- 1Laboratory Functional Food and Bioprocessing, Department of Food Industries, Universidad Nacional de Frontera, Sullana, Peru
- 2Faculty of Business Sciences and Tourism, Universidad Nacional de Frontera, Sullana, Peru
- 3Agroindustria La Perla del Huascarán S.R.L, Huaraz, Peru
- 4Departamento de Nutrición, Universidad Nacional Agraria La Molina, Lima, Peru
The present study focuses on the effect of different drying temperatures (DT) (40, 60 and 80°C) and the combination of banana slice thicknesses (BST) (2 and 4 mm) on the physicochemical properties and phytochemicals of green banana flour (GBF). The influence of the drying temperature and thickness of the banana pulp slice were significant (p < 0.05) on the TPC and % inhibition of the DPPH radical. As the temperature increased from 60 to 80°C and the thickness decreased from 4 to 2 mm, the TPC values (225.69 ± 5.13 GAE/100 g DW) and % inhibition of the DPPH radical (91.08 ± 2.28%) were higher, respectively. Physicochemical properties such as: soluble solids, titratable acidity, pH and ashes were not influenced by DT and BST; and the humidity values were < 10%. These findings indicate that drying with hot air from 60 to 80°C and thicknesses <4 mm favor a greater conservation of the antioxidant capacity in banana flour.
Introduction
Banana (Musa spp) is one of the most consumed fruits in the world, due to its flavor and nutritional content; this fruit is the fourth most important food after rice, wheat and corn (Salazar et al., 2021) and is considered a staple food in various countries. Its cultivation has spread to Asia, South America and Africa. Annual world banana production is around 115 million tons (Ahmed et al., 2020), and is the most produced fruit in the world (Meng et al., 2022). This fruit in its unripe state contains vitamins (B3, B6, B12, C, and E), minerals (P, Na, Ca, Mg, K, Zn, Fe, Cu, Mn), polyphenolic compounds, flavonoids, fiber, resistant starch and indigestible carbohydrates, which make it beneficial for human health (Padhi and Dwivedi, 2022).
Peru supplies approximately 3% of world banana production and almost all bananas grown in Peru are exported (FAO, 2021; Campos et al., 2022), especially the Cavendish type; this type of banana only grows in the states of Tumbes, Piura and Lambayeque. In 2021, about 2.5 million tons of bananas were produced (MIDAGRI, 2022) at a value of 145.24 million USD. The US market was the main importer, accumulating 22.31% of total banana exports from Peru (ITC, 2022). It is estimated that Peruvian exports for the year 2026 will reach 500 million USD, though this is a distant scenario.
Unfortunately, the conflict between Ukraine and Russia, added to other political and economic events, have impacted the global market, and have generated a 16% drop in Peruvian banana exports, In addition, production costs have increased by 91% since 2021 (García, 2022). Post-harvest and fruit processing losses are among the highest (40 to 50%) in Latin America (Felix e Silva et al., 2020), due to the quick expiration period of the banana. This forces producers and exporters to seek processing alternatives to reduce and avoid discarding this fruit. Consequently, the production of alternative and commercially viable products, such as banana flour, has been proposed to improve and guarantee the economic sustainability of producers, while providing a continuous supply of new ingredients for the food industry (Ahmed et al., 2020; Guadalupe-Moyano et al., 2022). Green banana flour (GBF) is an alternative to produce functional foods and it has been shown to help reduce the glycemic index, diabetes and prevent colon cancer and cardiovascular diseases (Padhi and Dwivedi, 2022).
There is an estimated average of 154.70 mg/100 g of phenolic content in bananas, such as catechins, epicatechins and phenolic acids all important antioxidants; these antioxidants are conserved in an adequate proportion during GBF manufacturing, even the free fraction tends to increase during the GBF production (Pico et al., 2019b; Chang et al., 2022). A study demonstrated the consumption of cakes supplemented with dehydrated and/or extruded banana flour showed an inhibition of glucose transport from 45.0 to 54.5% higher than the control (wheat cake), functionality attributed to the activity of catechins and myricetins — compounds present in GBF (Pico et al., 2019a).
Because GBF has many health benefits, it has been used as an ideal complement in products such as pasta, bread, spaghetti, cookies, noodles, baby food, and dairy products (Patiño-Rodríguez et al., 2018; Kumar et al., 2019; Felix e Silva et al., 2020; Guadalupe-Moyano et al., 2022). However, the functional properties of GBF are influenced by the variety of the fruit, its stage of maturation and the drying method (Guadalupe-Moyano et al., 2022).
GBF can be produced by hot air drying, drip bed drying, ultrasonication, pulsed vacuum oven, microwave, spray drying and freeze drying (Khoozani et al., 2019). There are different drying techniques. Flavor and aroma retention are important properties when choosing a drying technique (Meng et al., 2022). Hot air drying (HAD) is frequently used in dehydrated food production instead of freeze-drying, since hot air drying has more economic viability, phytochemical content and aroma development (Jiang et al., 2019). However, the drying conditions affect the content of bioactive compounds, because cell rupture occurs during drying, promoting the release and/or degradation of phenolic compounds and generating changes in enzymes activities such as polyphenol oxidase. These enzymes react to light, oxygen, drying temperature, water content, pH and other intervening factors (Amini Khoozani et al., 2019). For example, high temperatures and long drying periods cause undesirable effects on quality parameters such as color, flavor, rehydration capacity, and the nutritional and antioxidant values of the products (Polat and Izli, 2022).
Rajoriya et al. (2021) demonstrated that convective drying of banana puree at temperatures of 90°C generates greater retention of ascorbic acid (78%) and total phenolic compounds (10.3 mg GAE/g DW), flavonoids (3.4 mg QE/g DW), as well as the antioxidant capacity (58.8 mM TE/g DW).
A similar situation was observed by Chikpah et al. (2022). The increase in the temperature of the drying air from 50 to 70°C, increased the total phenolic compounds and the flavonoid content in dehydrated fruits. However, it was observed that increasing the thickness of the slices (from 3 to 5 mm) extended the drying time, causing greater loss of bioactive compounds and antioxidant activity. These reports are limited regarding the influence of HAD on antioxidant capacity in GBF production, since attention is focused on the resistant starch in this product. Therefore, the objective of the study was to analyze the impact of hot air-drying conditions, drying temperature and slice thickness, and the antioxidant capacity of GBF to obtain a scenario that can be replicated by the food industry.
Materials and methods
Materials and reagents
Organic green bananas were collected (Musa cavendish) from small producers in the district of Miguel Checa, Sullana, Piura, Perú.
DPPH (2.2-diphenyl-1-picrylhydrazyl), gallic acid, Folin–Ciocalteu, sodium carbonate, ethanol (99.9% purity), and methanol (99.5% purity) were obtained from Sigma-Aldrich (ACS, Lima, Perú).
Green banana flour production
Banana flour production was carried out using the methodology proposed by Khoozani et al. (2019), with some modifications. The fruits were selected, washed and disinfected with sodium hypochlorite (50 ppm). Subsequently, the peel was removed, the pulp was cut into 2-and 4-mm thick slices, then immediately submerged in a 0.5% (w/v) citric acid solution for 15 min, since polyphenol oxidase enzymes present in banana fruit cause enzymatic oxidative reactions forming highly bonded polyphenols that absorb light in the full electromagnetic range extinguishing any internal blue fluorescence (Segura-Badilla et al., 2022). Banana slices were dried (hot air drying, dehydrator ST-01) at three different temperatures (40, 60, and 80°C) to equilibrium moisture. The air feeding the dryer had a relative humidity of 38%. The air flow rate was 2.5 m/s. The dried banana slices were ground in a blade mill (DAMAI High-speed Multifunction) and then sifted through an ASTM 70 mesh sieve (212 μm) and vacuum packed in polypropylene bags until analysis.
Chemical properties of banana flour
pH, titratable acidity, and soluble solids
The determination of pH, TA, and SS was carried out as reported by Padhi and Dwivedi (2022) with some modifications. One hundred milliliter of distilled water were added to 3 g of GBF, heated for 30 min, and then filtered. Twenty milliliter of filtered solution were used for pH analysis with a pH meter (HANNA-HI991001), calibrated by direct immersion of the electrode. Two drops of the solution were used and added to the prism of the refractometer (HANNA-H196801) for the determination of SS. The TA was estimated by titrating 20 mL of the filtrate with 0.1 N NaOH. Phenolphthalein was used as the indicator. The final point of the titration was with the color change to pink. The TA was determined using the Eq. (1).
*Malic acid equivalent = 0.067.
Humidity and ashes
GBF moisture and ash content was determined as described by Vega-Rojas et al. (2021) — by oven drying (Memmert, Mod: UN110) at 105°C and incineration in muffle (SEL-HORN “R-8 L”) at 550 ± 15°C respectively, both cases for 4 h.
Phytochemical properties
Determination of total phenolic content of GBF
The TPC was determined according to the method described by Cornelio-Santiago et al. (2019). To obtain the extracts, approximately 1 g of GBF was weighed, 5 mL of methanol were added in a 10 mL capacity tube, and then the whole solution was homogenized in a Vortex mixer at 2500 rpm for 20 min. Subsequently, it was centrifuged at 4500 rpm for 20 min and the first supernatant was recovered. With the residue obtained, the previous steps were repeated, obtaining a second supernatant; both recovered were mixed and homogenized.
For the colorimetric assay, the mixture of 1,364 μL of distilled water and 0.3 mL of methanolic extract (ME) was reacted with 136 μL of Folin–Ciocalteu a 2 N. The resulting solution was allowed to stand at room temperature for 8 min in a dark room. Later, 1.2 mL of 7.5% Na2CO3 were added, and the reaction was completed after remaining 2 h in the dark at room temperature (25°C), exposed to a dark blue color. The absorbance was recorded in a UV–Visible spectrophotometer (Genesys, S-150, 6,287,015) at 760 nm.
Determination of antioxidant capacity in GBF
The 2,2-diphenyl-1-picrylhydrazil (DPPH) assay was carried out to measure the antioxidant capacity according to the method reported by Tian et al. (2018). The assay consisted of reacting 100 uL of ME with 2 mL of DPPH solution (0.8 mmol/L). The necessary reaction time was 10 min of rest at room temperature in the dark. The absorbance values were measured at 517 nm using a UV–Visible spectrophotometer (Genesys, S-150, 6,287,015). The results were expressed in (%) percentage of inhibition of the DPPH radical, and it was calculated using the Eq. (2).
Where:
Abs. C: absorbance of DPPH solution at 0.8 mmol/L.
Abs. M: sample absorbance after 40 min rest.
Experimental design and statistical analysis
The experimental design considered two independent variables; thickness of the banana pulp slice (2 and 4 mm) and drying temperature (40, 60, and 80°C), applying a completely random 3×2 factorial arrangement. A total of 6 treatments with duplicates of each treatment and three replicates for each analysis were carried out. Average values in each case were taken and represented with means ± standard deviation. The results were subjected to analysis of variance (ANOVA) and the difference between the means was evaluated using the Tukey test. When the p probability level of the test turned out to be lower than the significance level set at p < 0.05, It was considered significantly different. Statistical analysis was performed using Minitab 19 statistical software. Ink. for Windows.
Results and discussion
Physical and chemical properties of GBF
The content of soluble solids, pH, and titratable acidity of the GBF (Table 1) was influenced only by the drying temperature, according to the analysis of variance. No significant difference was found between the treatments for these analyses. Slightly higher pH and TA values were observed when the temperature increased, which could be attributed to the thermal stability of fatty acids. This characteristic was demonstrated in ultrasonic-assisted airborne apple drying (Zhu et al., 2022).
Considering the analysis of variance, the drying conditions did not show an influence on the ash content of the GBF. The values were found within the range of 2.27 to 3.68%, reported in previous studies (Ahmed et al., 2020; Chang et al., 2022). Therefore, it was evident that the moisture content of the flours depended mainly on DT, followed by the thickness of the slice, and, to a lesser extent, on the interaction of both variables. The H values were less than 10% in all the treatments studied, considering that the Peruvian technical standard (NTP) 205.064 mentions 15% as the maximum permissible limit for this type of product; the GBF obtained in this study meets this requirement.
Effect of hot air drying on total phenolic content of GBF
Table 2 shows the TPC values ranging from 17.95 to 224.69 mg GAE/100 g DW in GBF, in the different treatments studied.
The TPC values of this study were higher than the values found by Chang et al. (2022), who reported from 93.82 to 117.77 mg/100 g. Khoza et al. (2021), however, observed higher values in GBF of different varieties (between 287.40 and 407.08 mg/100 g), these differences can be attributed to the variety of the fruit, state of maturity and cultivation conditions.
Table 2 shows that DT, BST and the interaction of DT x BST, have a significant effect on the TPC of GBF. However, the greatest influence is given by BTS, as observed in the Pareto diagram of the main effects (Figure 1A). It was observed that the TPC increased with the increase of the temperature (Figure 1B). The highest TPC contents (225.65 and 160.47 mg GAE/100 g DW) were observed at a drying temperature of 80°C. These values coincide with those by Rajoriya et al. (2021) who worked with dried banana puree, and Yılmaz et al. (2017) who reported an increase in TPC as the drying temperature increased. A shorter drying time at 80°C compared to 40 and 60°C could have prevented the thermal and oxidative degradation of TPC, due to the rapid removal of moisture. This process during the first minutes of drying is very intense; the rapid evaporation of water causes the partial pressure of oxygen near the product to be reduced, which contributes to reducing the oxidation reactions promoted by O2. As a result, a greater retention of phenolic compounds is possible (Hernández-Santos et al., 2016). In addition, during drying with hot air, the product is subjected to stress conditions that stimulate the variation of the phenolic content through derivation reactions (Padhi and Dwivedi, 2022). High drying temperatures cause modification of the cell structure, which weakens the affinity of conjugated TPCs with the cell wall, increasing their availability (Zhu et al., 2022). Also, the formation of new phenolic complexes is generated by non-enzymatic interconversion between phenolic molecules at high temperatures (Rajoriya et al., 2021). However, at temperatures of 40 and 60°C, enzymatic oxidation reactions could have predominated, mainly by the enzyme polyphenol oxidase (PPO), because they are not sufficient temperatures to destroy the conformation of the enzymatic protein. PPO is generally inactivated at temperatures close to 80°C or higher, since it consists of two isoenzymes: the thermolabile and the thermoresistant. The heat-labile fraction is unstable and could be destroyed at low temperatures, while the heat resistant fraction could be inactivated when the blanching temperature was higher than 70°C (An et al., 2023).
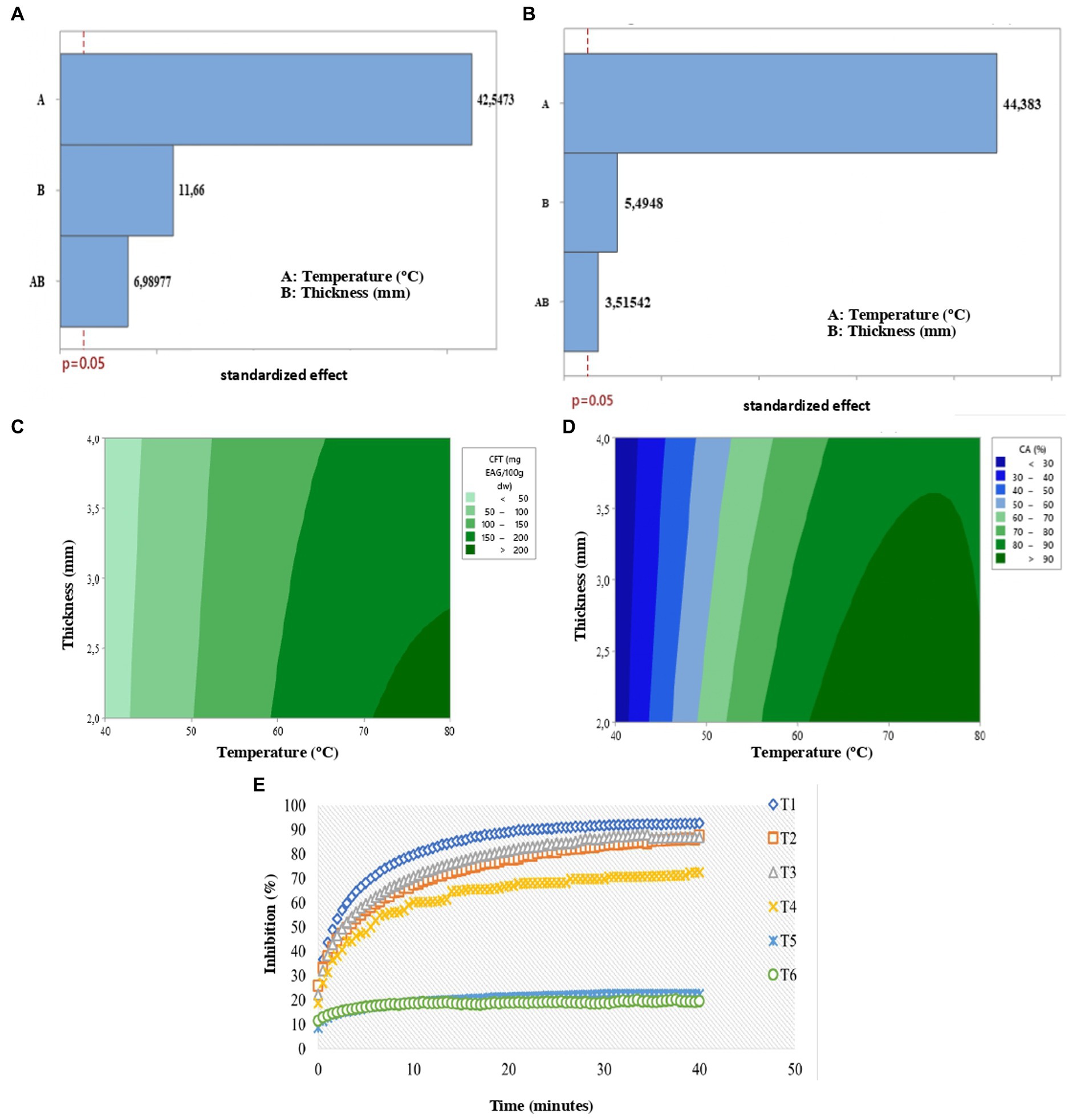
Figure 1. Effect of drying temperature and slice thickness on antioxidant capacity; (A) Pareto diagrams of main effects: Total phenolic compounds (mg GAE/100 g DW) and (C) Antioxidant capacity (% inhibition of the DPPH radical); (B) Contour plot for total phenolic compounds and (D) Antioxidant capacity; (E) Reaction time for the inhibition of the DPPH radical.
Regarding the BST factor, dried fruit, whose slice thickness was less than 4 mm, showed higher phenolic content (Figure 1B), due to short drying periods, which reduces TPC degradation reactions. When the BST is 2 mm, the exposed surface area is greater. This allows an increase in the speed of water removal through the hot air, which transports the water from the interior to the center, and from the center to the surface of the banana slice. It is argued that the temperature and the time of exposure to the treatment are crucial factors responsible for the retention of phenolic compounds (Padhi and Dwivedi, 2022). Therefore, drying 2 mm thick banana slices requires a shorter drying time, reducing prolonged heat treatment, consequently retaining better phenolic content in the GBF (Chikpah et al., 2022). This result is consistent with the report of Jafari et al. (2016), which showed that an increase in the thickness of the pumpkin slices prolonged the drying time and caused a greater loss of bioactive compounds and antioxidant activity, in the same way in the drying of kiwi.
Antioxidant capacity of GBF
Antioxidants contribute to the prevention, reduction or repair of the deterioration caused by reactive oxygen or nitrogen species in cells and/or biomolecules. This prevention would help improve people’s immunity (Padhi and Dwivedi, 2022). The antioxidant capacity observed by GBF in the different treatments remained from 21.20 to 91.08% of inhibition of the DPPH radical (Table 2). The reaction time required for this study was 40 min (Figure 1E). According to the statistical analysis DT, BST and DT x BST showed a significant effect on the antioxidant capacity of GBF (Table 2). However, the effect of temperature was predominant, according to the Pareto diagram for the main effects (Figure 1C). In addition, the increase in the slice thickness favors a lower antioxidant capacity (Figure 1D). However, an opposite behavior was observed when the drying temperature increased. At 60 and 80°C the inhibition of the DPPH radical was between 80 and 91% respectively, which could be related to the presence of TPC. Bhat et al. (2022) revealed that polyphenols were largely responsible for the antioxidant capacity of kiwi; in fact, partially oxidized polyphenols demonstrated better antioxidant capacity than non-oxidized compounds. Additionally, Lobo et al. (2017) stated that temperatures close to 80°C favor a better quality of phenolic compounds, which contributes to increasing their antioxidant capacity. The values found at temperatures of 40°C suggest a high incidence of chemical, enzymatic or thermal degradation of antioxidant compounds.
Therefore, the present study confirms that hot air drying under different tested conditions, demonstrates a high content of TPC and CA. The importance of these findings lies in the use of GBF for the elaboration of functional foods, under optimal processing conditions using conventional drying.
The contribution of the manuscript is to recommend drying parameters at higher temperatures and intermediate air speed during banana drying in order to inactivate the highest amount of polyphenoloxylated enzymes, as well as to preserve the highest content of bioactive compounds in the final product.
Conclusion
The antioxidant properties of GBF were affected by different drying temperatures and slice thicknesses of banana pulp. Samples dried by hot air at temperatures from 60 to 80°C showed higher TPC and AC, while these properties decreased with the slice thickness increase from 2 to 4 mm. At 40°C the total phenolic content and antioxidant capacity were reduced by up to 87% compared to those at 80°C. The drying temperature and the thickness of the banana pulp slice do not have much effect on pH, SS, TA and A. The humidity in all the working conditions was less than 10%.
Data availability statement
The original contributions presented in the study are included in the article/supplementary material, further inquiries can be directed to the corresponding author.
Author contributions
LE-E: conceptualization, investigation, funding acquisition, methodology, supervision and revision. CJ-O: conceptualization, investigation and preparation of the original draft. LR-F: investigation, writing, data curation, and formal analysis. LM-Q: data curation, review, and parcial funding. MA-P and HC-Q: supervision, review, and visualization.
Funding
This research received financial support from the National Programme for Scientific Research and Advanced Studies (PROCIENCIA), Peru. Contract No. 049-2021-FONDECYT, and the Universidad Nacional de Frontera grants a partial funding for the payment of APC by RESOLUTION No. 217-2023-UNF/FCET.
Acknowledgments
The authors thank the laboratory staff of the Universidad Nacional de Frontera.
Conflict of interest
The authors declare that the research was conducted in the absence of any commercial or financial relationships that could be construed as a potential conflict of interest.
Publisher’s note
All claims expressed in this article are solely those of the authors and do not necessarily represent those of their affiliated organizations, or those of the publisher, the editors and the reviewers. Any product that may be evaluated in this article, or claim that may be made by its manufacturer, is not guaranteed or endorsed by the publisher.
References
Ahmed, J., Thomas, L., and Khashawi, R. (2020). Influence of hot-air drying and freeze-drying on functional, rheological, structural and dielectric properties of green banana flour and dispersions. Food Hydrocoll. 99:105331. doi: 10.1016/j.foodhyd.2019.105331
Amini Khoozani, A., Birch, J., and Bekhit, A. E. D. A. (2019). Production, application and health effects of banana pulp and peel flour in the food industry. J. Food Sci. Technol. 56, 548–559. doi: 10.1007/s13197-018-03562-z
An, N., Lv, W. Q., Li, D., Wang, L. J., and Wang, Y. (2023). Effects of hot-air microwave rolling blanching pretreatment on the drying of turmeric (Curcuma longa L.): physiochemical properties and microstructure evaluation. Food Chem. 398:133925. doi: 10.1016/j.foodchem.2022.133925
Bhat, T. A., Hussain, S. Z., Wani, S. M., Rather, M. A., Reshi, M., Naseer, B., et al. (2022). The impact of different drying methods on antioxidant activity, polyphenols, vitamin C and rehydration characteristics of kiwifruit. Food Biosci. 48:101821. doi: 10.1016/j.fbio.2022.101821
Campos, J. C., Manrique-Silupú, J., Dorneanu, B., Ipanaqué, W., and Arellano-García, H. (2022). A smart decision framework for the prediction of thrips incidence in organic banana crops. Ecol. Model. 473:110147. doi: 10.1016/j.ecolmodel.2022.110147
Chang, L., Yang, M., Zhao, N., Xie, F., Zheng, P., Simbo, J., et al. (2022). Structural, physicochemical, antioxidant and in vitro digestibility properties of banana flours from different banana varieties (Musa spp.). Food Biosci. 47:101624. doi: 10.1016/j.fbio.2022.101624
Chikpah, S. K., Korese, J. K., Sturm, B., and Hensel, O. (2022). Colour change kinetics of pumpkin (Cucurbita moschata) slices during convective air drying and bioactive compounds of the dried products. J. Agric. Food Res. 10:100409. doi: 10.1016/j.jafr.2022.100409
Cornelio-Santiago, H. P., Mazalli, M. R., Rodrigues, C. E. C., and de Oliveira, A. L. (2019). Extraction of Brazil nut kernel oil using green solvents: effects of the process variables in the oil yield and composition. J. Food Process Eng. 42:e13271. doi: 10.1111/jfpe.13271
FAO (2021). Banana market review – Preliminary results 2020. Roma Available at: https://www.fao.org/publications/card/en/c/CB5150EN/ (accessed November 13, 2022).
Felix e Silva, A., Copatti, C. E., de Oliveira, E. P., Bonfá, H. C., Melo, F. V. S. T., Camargo, A. C. S., et al. (2020). Effects of whole banana meal inclusion as replacement for corn meal on digestibility, growth performance, haematological and biochemical variables in practical diets for tambaqui juveniles (Colossoma macropomum). Aquac. Rep. 17:100307. doi: 10.1016/j.aqrep.2020.100307
García, E. (2022). El costo de producción del banano en Perú sube un 91% y su exportación a agosto cae un 16%. Gestion Available at: https://gestion.pe/economia/costo-de-produccion-del-banano-sube-91-y-su-exportacion-a-agosto-cae-en-16-noticia/?ref=gesr (accessed November 13, 2022).
Guadalupe-Moyano, V., Palacios-Ponce, A. S., Rosell, C. M., and Cornejo, F. (2022). Impact of drying methods on banana flour in the gluten-free bread quality. LWT 168:113904. doi: 10.1016/j.lwt.2022.113904
Hernández-Santos, B., Martínez-Sánchez, C. E., Torruco-Uco, J. G., Rodríguez-Miranda, J., Ruiz-López, I. I., Vajando-Anaya, E. S., et al. (2016). Evaluation of physical and chemical properties of carrots dried by Refractance window drying. Dry. Technol. 34, 1414–1422. doi: 10.1080/07373937.2015.1118705
ITC (2022). Trade Map - Lista de los mercados importadores para un producto exportado por Perú. Available at: https://www.trademap.org/Country_SelProductCountry_TS.aspx?nvpm=6040803412212111 (accessed March 4, 2023).
Jafari, S. M., Azizi, D., Mirzaei, H., and Dehnad, D. (2016). Comparing quality characteristics of oven-dried and Refractance window-dried kiwifruits. J. Food Process. Preserv. 40, 362–372. doi: 10.1111/jfpp.12613
Jiang, G. H., Lee, K. C., Ameer, K., and Eun, J. B. (2019). Comparison of freeze-drying and hot air-drying on Asian pear (Pyrus pyrifolia Nakai ‘Niitaka’) powder: changes in bioaccessibility, antioxidant activity, and bioactive and volatile compounds. J. Food Sci. Technol. 56, 2836–2844. doi: 10.1007/s13197-019-03700-1
Khoozani, A. A., Bekhit, A. E. D. A., and Birch, J. (2019). Effects of different drying conditions on the starch content, thermal properties and some of the physicochemical parameters of whole green banana flour. Int. J. Biol. Macromol. 130, 938–946. doi: 10.1016/j.ijbiomac.2019.03.010
Khoza, M., Kayitesi, E., and Dlamini, B. C. (2021). Physicochemical characteristics, microstructure and health promoting properties of green banana flour. Foods 10:2894. doi: 10.3390/foods10122894
Kumar, P. S., Saravanan, A., Sheeba, N., and Uma, S. (2019). Structural, functional characterization and physicochemical properties of green banana flour from dessert and plantain bananas (Musa spp.). LWT 116:108524. doi: 10.1016/j.lwt.2019.108524
Lobo, F. A., Nascimento, M. A., Domingues, J. R., Falcão, D. Q., Hernanz, D., Heredia, F. J., et al. (2017). Foam mat drying of Tommy Atkins mango: effects of air temperature and concentrations of soy lecithin and carboxymethylcellulose on phenolic composition, mangiferin, and antioxidant capacity. Food Chem. 221, 258–266. doi: 10.1016/j.foodchem.2016.10.080
Meng, Z., Cui, X., Liu, Y., Hu, R., du, C., Wang, S., et al. (2022). Drying characteristics of banana slices under heat pump-electrohydrodynamic (EHD) combined drying. Sustain. Energy Technol. Assess. 54:102907:102907. doi: 10.1016/j.seta.2022.102907
MIDAGRI (2022). Boletín estadístico mensual “El agro en cifras: Diciembre 2021.” Lima Available at: https://siea.midagri.gob.pe/portal/ (accessed November 18, 2022).
Padhi, S., and Dwivedi, M. (2022). Physico-chemical, structural, functional and powder flow properties of unripe green banana flour after the application of Refractance window drying. Future Foods 5:100101. doi: 10.1016/j.fufo.2021.100101
Patiño-Rodríguez, O., Bello-Pérez, L. A., Flores-Silva, P. C., Sánchez-Rivera, M. M., and Romero-Bastida, C. A. (2018). Physicochemical properties and metabolomic profile of gluten-free spaghetti prepared with unripe plantain flours. LWT 90, 297–302. doi: 10.1016/j.lwt.2017.12.025
Pico, J., Corbin, S., Ferruzzi, M. G., and Martinez, M. M. (2019a). Banana flour phenolics inhibit trans-epithelial glucose transport from wheat cakes in a coupled: in vitro digestion/Caco-2 cell intestinal model. Food Funct. 10, 6300–6311. doi: 10.1039/c9fo01679a
Pico, J., Xu, K., Guo, M., Mohamedshah, Z., Ferruzzi, M. G., and Martinez, M. M. (2019b). Manufacturing the ultimate green banana flour: impact of drying and extrusion on phenolic profile and starch bioaccessibility. Food Chem. 297:124990. doi: 10.1016/j.foodchem.2019.124990
Polat, A., and Izli, N. (2022). Drying characteristics and quality evaluation of ‘Ankara’ pear dried by electrohydrodynamic-hot air (EHD) method. Food Control 134:108774. doi: 10.1016/j.foodcont.2021.108774
Rajoriya, D., Bhavya, M. L., and Hebbar, H. U. (2021). Impact of process parameters on drying behaviour, mass transfer and quality profile of refractance window dried banana puree. LWT 145:111330. doi: 10.1016/j.lwt.2021.111330
Salazar, D., Arancibia, M., Lalaleo, D., Rodríguez-Maecker, R., López-Caballero, M. E., and Montero, M. P. (2021). Physico-chemical properties and filmogenic aptitude for edible packaging of Ecuadorian discard green banana flours (Musa acuminanta AAA). Food Hydrocoll. 122:107048. doi: 10.1016/j.foodhyd.2021.107048
Segura-Badilla, O., Kammar-García, A., Mosso-Vázquez, J., Ávila-Sosa Sánchez, R., Ochoa-Velasco, C., Hernández-Carranza, P., et al. (2022). Potential use of banana peel (Musa cavendish) as ingredient for pasta and bakery products. Heliyon 8:e11044. doi: 10.1016/j.heliyon.2022.e11044
Tian, X., Liu, Y., Feng, X., Khaskheli, A. A., Xiang, Y., and Huang, W. (2018). The effects of alcohol fermentation on the extraction of antioxidant compounds and flavonoids of pomelo peel. LWT Food Sci. Technol. 89, 763–769. doi: 10.1016/j.lwt.2017.11.049
Vega-Rojas, L. J., Londoño-Restrepo, S. M., and Rodriguez-García, M. E. (2021). Study of morphological, structural, thermal, and pasting properties of flour and isolated starch from unripe plantain (Musa paradisiaca). Int. J. Biol. Macromol. 183, 1723–1731. doi: 10.1016/j.ijbiomac.2021.05.144
Yılmaz, F. M., Yüksekkaya, S., Vardin, H., and Karaaslan, M. (2017). The effects of drying conditions on moisture transfer and quality of pomegranate fruit leather (pestil). J. Saudi Soc. Agric. Sci. 16, 33–40. doi: 10.1016/j.jssas.2015.01.003
Zhu, R., Jiang, S., Li, D., Law, C. L., Han, Y., Tao, Y., et al. (2022). Dehydration of apple slices by sequential drying pretreatments and airborne ultrasound-assisted air drying: study on mass transfer, profiles of phenolics and organic acids and PPO activity. Innov. Food Sci. Emerg. Technol. 75:102871. doi: 10.1016/j.ifset.2021.102871
Keywords: banana, flour, convective drying, phenolic compounds, antioxidant capacity
Citation: Espinoza-Espinoza LA, Juárez-Ojeda CE, Ruiz-Flores LA, Moreno-Quispe LA, Anaya-Palacios MS and Cárdenas-Quintana H (2023) Influence of convection drying with hot air on the physicochemical and phytochemical properties of green banana flour (Musa cavendish). Front. Sustain. Food Syst. 7:1204349. doi: 10.3389/fsufs.2023.1204349
Edited by:
Anand Mohan, University of Georgia, United StatesReviewed by:
Vivaldo Silveira Junior, Campinas State University, BrazilRungsinee Sothornvit, Kasetsart University, Thailand
Copyright © 2023 Espinoza-Espinoza, Juárez-Ojeda, Ruiz-Flores, Moreno-Quispe, Anaya-Palacios and Cárdenas-Quintana. This is an open-access article distributed under the terms of the Creative Commons Attribution License (CC BY). The use, distribution or reproduction in other forums is permitted, provided the original author(s) and the copyright owner(s) are credited and that the original publication in this journal is cited, in accordance with accepted academic practice. No use, distribution or reproduction is permitted which does not comply with these terms.
*Correspondence: Luis Alfredo Espinoza-Espinoza, bGVzcGlub3phQHVuZi5lZHUucGU=