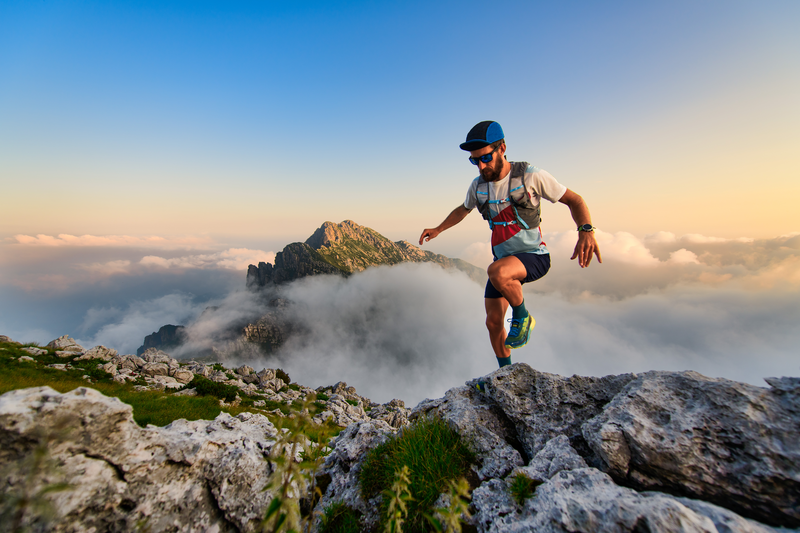
95% of researchers rate our articles as excellent or good
Learn more about the work of our research integrity team to safeguard the quality of each article we publish.
Find out more
ORIGINAL RESEARCH article
Front. Sustain. Food Syst. , 01 June 2023
Sec. Crop Biology and Sustainability
Volume 7 - 2023 | https://doi.org/10.3389/fsufs.2023.1197624
This article is part of the Research Topic ‘Save Soil’ by Managing Soil Nutrient Losses, Agronomic Practices and Crop-Microbial Interaction: World Soil Day 2022 View all 12 articles
Soil microbial communities are seen to be critical to the maintenance of soil health and quality. Many studies have suggested that soil microbial community composition is affected by different plant species. Based on this theory, we tried to improve the apple orchard soil microorganisms and nutrients by interplanting leguminous forage red clover (RC), meanwhile taking the natural mixed herb (NMH) naturally growing in the orchard as a control. The metagenomic analysis showed that interplanting red clover in the apple orchard markedly improved the soil microbial community composition, especially compared with natural mixed herbs, the abundance of Nitrospirae and Glomeromycota was higher. Compared with genus level, there are more unique bacteria species in RC, 84 species were unique to treatment (RC) soil and 43 species were unique to control group (NMH). The activities of glycoside hydrolase and carbohydrate-binding modules in RC were significantly higher than those in NMH, while compared with the control group (NMH), the activity of glycoside hydrolase and carbohydrate binding module in the treatment group (RC) was significantly higher, but the activity of glycosyl transferase was lower. In addition, only 5 of the 15 virulence factors they contain were lower in the RC, while the rest were higher in the control group. Based on the above results, we speculate that intercropping red clover can not only significantly improve soil quality, soil microbial community composition and soil fertility, but also effectively improve land use efficiency and reduce the use of chemical fertilizers.
Microorganisms drive all major biogeochemical cycles on Earth, and have profound effects on all other organisms (and their habitats) due to their different roles as pathogens, symbiotes, biotrophic converters, decomposing agents, and primary food sources (Stott and Taylor, 2016). The enormous metabolic diversity of soil microorganisms means that their activities play a driving or promoting role in the circulation of all major elements (such as C, N, and P), thereby affecting the structure and function of soil ecosystems and the ability of soil to provide services to people (Aislabie and Deslippe, 2013). Soil microbial community composition can be affected by different plant species (Grayston and Campbell, 1996; Grayston et al., 2001). This phenomenon has been demonstrated by a variety of methods, including characterization of microbial biofilms (Bossio et al., 1998; Pierra et al., 2015), DNA gene map (Duarte et al., 1998; Chen et al., 2016), and metabolic characteristics (Degens and Harris, 1997; Bowles et al., 2014). Nutrients required by microorganisms are usually derived from plant litter and root exudates (Grayston et al., 1998). Plants affect soil organic carbon stocks and short-term carbon cycling (Ward et al., 2009) through differences in the quantity and quality of root exudates and litters (Coleman et al., 2000; Deyn et al., 2008), thus affecting microbial community composition and function. Soil enzyme activities related to microbial function are also affected by plant species (Waldrop et al., 2000). The coexistence of different plant functional groups (e.g., gramineae and legume) plays a particularly important role in ecosystem function (De Deyn et al., 2009). Intercropping with leguminous crops can utilize the complementarity between different species to improve the utilization efficiency of water, sunlight, air, and nutrient resources, increase nutrient availability and soil quality, thereby increasing fertility and improving the growth performance of the two intercropping crops in the agricultural system (Tsubo et al., 2005; Monteiro and Lopes, 2007; Massawe et al., 2016). Among mixed grass coverts, leguminous grass species have the greatest influence on microbial function, mainly through roots (Breulmann et al., 2012). According to existing research, utilizing diversity planting such as intercropping can not only change the composition of plant rhizosphere microbial communities, but also help improve the abundance of beneficial microorganisms in the rhizosphere (Zhou et al., 2023). Although soil microbial community structure is generally considered to be limited by carbon nutrition, many studies suggest that it may also be limited by nitrogen nutrition (Chen and Stark, 2000). Schimel et al. (2005) found that nitrogen requirements seem to change the ratio between different microbial populations. Leguminous plants can obtain nitrogen by association with rhizobia or Frankia via differentiation on their respective host plants of a specialized organ, the root nodule (Franche et al., 2009).
Orchard grass mulch is a method of orchard management in which perennial herbs are planted as mulch between rows or throughout the orchard (Almeida et al., 2011; Qian et al., 2015). The soil microbial communities (SMC) in the vast majority of hot and arid regions of the Earth’s grasslands are mainly composed of 4 types of feed legumes, namely, alfalfa (Medicago sativa L.), red clover (Trifolium pratense L.), underground clover (T. subterranum L.), and white clover (T. repens L.; Zhou et al., 2017). The related changes of root development and root deposition during the growing season may change the spatial distribution and quality of organic matter (Philippot et al., 2013a,b), thus affecting the dynamics of microbial community over time. Leguminous forage can improve soil microbial community because of its nitrogen fixation function, and nitrogen is the basic element of microbial metabolism. Jiao et al. (2013) found that interplanting red clover in apple orchards could improve the functional diversity of soil microorganisms through Biolog Eco-Plate™. The decomposition of red clover stems and leaves cut into the soil in orchards can affect the abundance and proportion of soil bacteria and fungi (Zhou et al., 2019).
We assumed that planting a leguminous forage variety called red clover (RC) in an apple orchard can improve the soil microbial community and chemical parameters of the orchard. To verify this hypothesis, we planted RC in the orchard and used natural mixed herbaceous plants (NMH) as controls. In addition, the soil microbial community structure, carbohydrate enzyme activity and virulence factor abundance were also analyzed by using the metagenome technology. By comparing the differences between treatment and control, the improvement effect of planting red clover on the composition and function of soil microbial community was evaluated. In addition, we evaluated the differences between stem and leaf yields and soil chemistry parameters. This study explores a method to maintain the basic fertility of orchard soil, reduce fertilizer use, and improve soil ecological environment, laying the foundation for sustainable and efficient production in orchards.
The experiment was conducted at the National Modern Agriculture Demonstration Base of Heilongjiang Academy of Agricultural Sciences (45°84′N, 126°84′E), located in east of Harbin, Heilongjiang Province, China. The soil type of the apple orchard is an chernozem soil (pH, 6.4; soil organic matter, 5.41%; total nitrogen 1.5 g kg−1; total phosphorus 332.9 μg kg−1; total potassium 15.2 g kg−1; available nitrogen 14.6 mg kg−1; available phosphorus 26.2 μg g−1; available potassium 35.4 mg kg−1).
The apple cultivar “zhenxing1” seedlings, the rootstock is Malus baccata, were planted for orchard construction, and with east–west direction and plant row spacing of 1.5 m × 4.0 m. In the spring of 2019, the red clover (Trifolium pratense L.) was sown between rows of fruit trees as experimental treatment (RC). Before sowing, weeds were removed from the test plot, turned 20 ~ 25 cm deep, applied 5 kg·m−2 of high-quality organic fertilizer, mixed the soil fertilizer evenly, and then used a rake to smooth the land. Meanwhile native mixed herbs (NMH) growing naturally in the orchard, which is a common applied method, was set as the control. Each treatment area was 60 m2 with four replicates, 240 m2 in total. Red clover treatment was consistent with other orchard management practices. Other management measures such as fertilizer and water were consistent between the treatment group and the control group.
According to the orchard field management calendar, the herb was mowed three times on June 19, July 27 and September 20, 2020, and the yield of herb stems and leaves was measured at the same time. A 3 m × 1 m square was randomly drawn in the test plot to measure the yield, and the stubble height was 5 cm. The herb stems and leaves cut were collected and weighed to measure the fresh weight. The fresh herb stems and leaves was killed at 105°C for 30 min, and then dried in oven at 70–80°C until the weight did not change, in this way the dry weight was measured. Three repeated measurements were taken.
We collected soil samples in September 2020. Five sampling sites were selected according to “S” shape, respectively, for planting red clover treatment and native mixed herbs control treatment. After removing about 1 cm of the soil surface, samples were collected from different surface treatments at the sampling points using a soil drill that had been sterilized with 70% ethanol. The mixture was evenly mixed, and the soil depths were 0–20 cm, 20–40 cm, and 40–60 cm, respectively. Placed the soil sample in a plastic bag and evenly sieved it (2 mm) to remove debris such as crushed stones, stems, leaves, and dead roots from the soil.
Five gram soil from each sample was extracted into 10 ml sterile centrifuge tubes, stored in liquid nitrogen and brought back to the laboratory, sealed in dry ice, and then sent to MajorBio for metagenomic analysis of soil microbial communities. After DNA extraction, fragmentation, PE library construction and bridge PCR, sequence was performed using Illumina platform. After the quality control, assembly, prediction and redundancy removal of the original data, the non-redundant gene set was obtained. The non-redundant gene set was compared with the selected species or function database to obtain the species or function annotation information corresponding to each sample. Based on the annotated species or functional information, subsequent species composition analysis, samples comparison analysis, species difference analysis, correlation and model prediction analysis, etc. was carried out for these samples.
Setting the ratio of water to soil to 5:2, 10 g soil sample and 25 ml deionized water were added into a 50 ml tall beaker, and fully mixed with a glass rod. After standing for half an hour, the supernatant was poured into a small beaker with a capacity of 20 ml, and the pH meter (Cole-Parmer ph700) was used to determine the pH value of the soil. Each sample was measured 3 times repeatedly. After measuring 5–6 samples, recalibrated the pH meter with standard buffer solution. The K2Cr2O7 oxidation method was used for determining the soil organic matter (Nelson and Sommers, 1982). Soil total nitrogen (TN) was measured by the Automatic kjeldahl apparatus method (Meseguer Lloret et al., 2005). Soil total phosphorus (TP) was measured by the Molybdenum blue method (Stauffer, 1983). Soil total potassium was measured by the Sodium hydroxide melting process method. The available phosphorus (AP) in soil was determined by Olsen method (Recena et al., 2022). After extracting NH4OAc (1.0 mol/L), the available potassium (AK) in the soil is determined by a flame photometer (Xu et al., 2020). Mineral N was extracted from soil with 2 M KCl and quantified using a flow injection analyzer (Westco Scientific Ltd., Brookfield, CT, USA; Engedal et al., 2023).
The data processing and analysis of metagenomic was carried out in the MajorBio data analysis platform.1 The significance of the differences between the treatment group and the control group analyzed by SPSS 19.0 software (SPSS Co., Ltd., Chicago, Illinois, USA).
Herbaceous stems can be used as soil green fertilizers, therefore, their yield can be used as a major indicator for evaluating the quality of herbaceous plants in orchards. In this study, we measured the yield of herb stems and leaves on June 19, July 27 and September 20, respectively, in 2020 (Table 1).
Compared with the first mowing yield measured on June 19, the fresh weight and dry weight of red clover (RC) were significantly (p < 0.05) higher (2.1 times) than those of native mixed herbs (NMH). Compared with the second mowing yield measured on July 27, there were no significant (p > 0.05) differences in both fresh weight and dry weight between RC and NMH. Compared with the third mowing yield measured on September 20, the fresh weight and dry weight of stems and leaves of RC were significantly (p < 0.05) higher (1.8 times) than that of NMH. Compared with the total annual yield, the fresh weight and dry weight yield of RC were significantly (p < 0.05) higher (2.4 times) than those of NMH. In summary, compared to NMH, the stem and leaf yield of red clover was higher during the same period. Moreover, according to field observation, red clover has the characteristics of early greening, late dormancy, robust growth and high coverage. These factors combine to make it have the characteristics of high yield of stems and leaves. In addition, according to the results of three yield measurements, red clover showed better growth in spring and autumn, indicating that it is more adaptable to cool climate.
Figure 1A showed the annotated column diagram of horizontal species in the NR (RefSeq non-redundant proteins: Non-redundant protein sequences from GenPept, Swissprot, PIR, PDF, P) database. The abundance of bacteria, fungi and viruses in soil samples of red clover treatment and native mixed herbs control were visually studied by using the visualization method of the column diagram.
Figure 1. Phyla level of microbial community composition of orchard soils. (A) Community barplot analysis. (B) Wilcoxon rank-sum test.
The results showed that the microbial community composition was significantly different between the red clover treatment group (RC_1, RC_2, and RC_3) and the natural clover control group (NMH_1, NMH_2, and NMH_3), which indicated that the soil microbial community composition was affected by different herb varieties in the orchard. In particular, the abundance of Nitrospirae in the red clover soil is 1.4 times higher than that of the native mixed herbs soil. The Nitrospirae is a class of Gram-negative bacteria, among which Nitrospira, as the Nitrifier, can oxidize nitrite into nitrate. Planting red clover in orchard can effectively improve the abundance of soil nitrogen-fixing bacteria. Figure 1B analyzed the significant differences of 15 dominant phyla with the highest abundance between treatment and control (p < 0.05). The abundance of 7 phyla was significantly higher in the treatment group, including: Proteobacteria, Gemmatimonadetes, Nitrospirae, Candidatus_Rokubacteria, Bacteroidetes, Candidatus_Tectomicrobia, and Planctomycetes. The remaining 8 phyla are more abundant in the control group, including: Actinobacteria, Acidobacteria, Chloroflexi, Thaumarchaeota, Firmicutes, unclassified bacteria, Verrucomicrobia and Euryarchaeota. In conclusion, planting red clover had significant effects on 15 dominant phyla with the largest abundance.
A heatmap analysis of phyla level microorganisms was conducted for the treatment soil samples (RC_1, RC_2 and RC_3) and control soil samples (NMH_1, NMH_2 and NMH_3) of the native mixed herbs control (Figure 2). The heatmap uses hierarchical clustering analysis to cluster species with high and low abundance. Color gradient and similarity degree reflect the similarity and difference of species composition in multiple samples communities.
According to Figure 2, the Glomeromycota abundance of soil samples planted with red clover for 3 repeats (RC_1, RC_2, and RC_3) had a much higher abundance of Glomeromycota compared to the control group (NMH_1, NMH_2, and NMH_3). Although Glomeromycota is a phylum of fungi of which only about 400 species have been found, it can form Arbuscular mycorrhizas with terrestrial plants. This symbiotic structure can assist plants in the absorption of inorganic salts, especially phosphorus, from the soil and is considered to have been an important key for plants to adapt to the terrestrial environments in the early stage. In the present study, planting red clover in the orchard increased the abundance of the Glomeromycota, which theoretically indicated that planting red clover can improve the soil microbial community composition and function of the orchard.
In Figure 3A, Venn diagram was used to analyze the common and unique microbiome genera in different soil treatments, which can intuitively show the similarity and overlap of soil microbial community composition. As it can be seen from Figure 3A, in the treatment group (RC) and the control group (NMH), there were 2,461 species of microorganisms belonging to the same genus, 84 species were unique to treated soil and 43 species were unique to control group. In conclusion, planting red clover in orchards can not only increase the variety of bacteria, but also make orchards have more unique bacterial species. In this study, principal component analysis was used to reflect the degree of difference between the treatment group and the control group at the generic level, which were shown in Figure 3B. The greater the distance between points, the greater the difference. As can be seen from Figure 3B, the distance between the treatment group and control group was significantly different, indicating that planting red clover in the orchard had a great effect on soil microbial community composition.
Figure 3. Comparison of soil microbial community composition between interplanting red clover and native mixed herbs in orchard at the genus level. (A) The similarity and overlap of soil microbial community composition. (B) PCA analysis.
Carbohydra-active enZYmes (CAZy) Database2 is a professional database on the synthesis and breakdown of complex carbohydrate and sugar complex enzymes. According to the similarity of amino acid sequences in protein domain, carbohydrate active enzymes from different sources could be divided into six types of enzymes, namely glycoside hydrolase, glycosyl transferases, polysaccharide clease, carbohydrate esterase, carbohydrate-binding modules and auxiliary REDOX reductase. Compared the activity of soil carbohydrate active enzymes in the treated group (RC) and the control group (NMH) in the CAZy database. In the soil samples of this study, the activities of three type enzymes, namely glycoside hydrolase, glycosyltransferase and carbohydrate binding module, were higher of all six type enzymes (Figure 4). The activities of glycoside hydrolase and carbohydrate-binding modules in RC were significantly higher than those in NMH, while the activities of glycosyl transferases were significantly lower than those in NMH (Figure 4).
Figure 4. Comparison of soil microbial carbohydrate active enzyme activities between planting red clover and growing natural mixed herbs in orchard.
In this study, virulence factor genes were counted by comparing virulence factor database,3 and the difference significance analysis was conducted for the top 15 virulence factors with the highest abundance (Figure 5). Of these, 10 virulence factors were higher in the control group (NMH), and only 5 were higher in the treatment group (RC). In general, planting red clover in orchards has a positive effect on reducing soil microbial virulence factors.
Figure 5. Comparison of soil microbial virulence factors between interplanting red clover and native mixed herbs in the orchard.
According to the results shown in Figure 6A, when the soil depth was 0–20 cm and 40–60 cm, the soil pH value of the treatment group and the control group was almost the same, but when the soil depth was 20–40 cm, the pH value of the control group was higher. For soil organic matter content, soil at depths of 0–20 cm and 20–40 cm contained more organic matter in the treatment group, while the organic matter content in soil at 40–60 cm depth were not significantly different from those in the control group (Figure 6B). It can be seen that the organic matter content of the soil in the orchard increased after planting red clover. The total nitrogen content of soil at depths of 0 to 20 cm and 20 to 40 cm was much higher in the treatment group, but the soil nitrogen content in the two groups at depths of 40 to 60 cm was almost the same (Figure 6C). The available nitrogen content in 0–20 cm and 20–40 cm soil depth in treatment group was significantly higher than that in control group, while the available nitrogen content in 40–60 cm soil depth was significantly lower than that in control group (Figure 6D). In general, planting red clover increased the nitrogen content of orchard soil. There was no significant difference in total P content between the treatment group and the control group at three depths (Figure 6E). Soil at a depth of 20–40 cm contained more available P in the treatment group, but at depths of 0–20 cm and 40–60 cm, the two groups of soil contained similar available P (Figure 6F). There was no significant difference in total potassium content between the treatment group and the control group at three depths (Figure 6G). The available potassium content in soil at 0–20 cm and 40–60 cm depth in treatment group was significantly lower than that in control group, while the available potassium content in soil at 20–40 cm depth was not significantly different from that in control group (Figure 6H).
Figure 6. Comparison of soil nutrient contents between planting red clover and growing natural mixed herbs in orchard. (A) The pH value of soil at different depths. (B) Organic matter content of soil at different depths. (C) Total nitrogen content of soil at different depths. (D) Available nitrogen content in soil layers at different depths. (E) Total p content of soil at different depths. (F) Available phosphorus content in soil at different depths. (G) Total potassium content of soil at different depths. (H) Available potassium content in soil at different depths.
This work provided evidence that growth of red clover has a major impact on microbial community structure in soils (Figures 1, 3). In particular, the abundance of Nitrospirae (Figure 1), which has the effect of nitrogen fixation, and the abundance of the beneficial fungus Glomeromycota were significantly increased (Figure 2). Nitrospirae is important bacteria in soil nitrogen cycle, usually involved in nitrite oxidation (Attard et al., 2010; Florine et al., 2017). Liu et al. (2019) showed that Alpha diversity of surface soil fungi increased significantly 30 years after grassland restoration. In this study, the Glomeromycota abundance in orchard soil after 2 years of intercropping red clover was significantly higher than that in native mixed herbs soil. Although Glomeromycota is a phylum of fungi of which only about 400 species have been found, it can form Arbuscular mycorrhizas with terrestrial plants (Brundrett, 2002). More than 80% of vascular plants have arbuscular mycorrhiza composed of bulbous bacteria, and arboreal mycorrhiza can also be found in bryophytes and other plants without real root structure, indicating the great significance of arbuscular mycorrhizas in terrestrial ecosystems (Schwarzott et al., 2001). It is concluded that intercropping red clover has a significant effect on improving the community and function of fungi. Soil microorganisms as indicators of soil quality are an important part of soil (Cui et al., 2021). Existing studies have shown that planting grass in orchards can effectively improve the ground temperature and air relative humidity, enhance soil water retention (Huang et al., 2014), and improve soil physical and chemical properties (Merwin et al., 1994). These changes will directly affect the environmental conditions for the survival of soil microorganisms. At the same time, more plant roots and litters are imported into the soil after planting grass. Their input and decomposition can improve the soil environment, promote the formation and accumulation of soil organic matter, and increase the soil nutrient content (Gomez et al., 2009; Rodrigues et al., 2013). Therefore, the food source and growth environment of soil microorganisms are changed, thus affecting the survival and reproduction ability of soil microorganisms (Zhang et al., 2015). The changes of soil microbial composition and soil enzyme activity were closely related to grass species. It was found that deep root leguminous herbage alfalfa could leave a large amount of root residue in deep soil, which was conducive to improving the urease activity and comprehensive fertilizer supply capacity in deep soil (Manici et al., 2015). Red clover root system was developed and distributed deeply, so it could influence the resource utilization in deep soil (Jiao et al., 2013). On the other hand, the different root exudates of different plants will also have a great influence on the rhizosphere soil microorganisms and enzyme activities (Sturz et al., 2011). Due to the complex physicochemical structure of plant cells, microorganisms usually secrete a large number of degrading enzymes, mainly glycoside hydrolases, lyases, esterases, and helper enzymes to utilize plant residues as an important nutrient source (Gilbert, 2010). In this study, Carbohydrate enzymes were classified according to the CAZy database (Zhong et al., 2018). The activities of glycoside hydrolase and carbohydrate-binding modules in interplanting red clover soils were significantly higher than those in native mixed herbs soils, while the activities of glycosyl transferases were significantly lower than those in native mixed herbs soils (Figure 4). The activity of enzymes related to carbon and nitrogen cycling in soil increased due to grass mulching, usually because the return of organic materials containing carbon and nitrogen to the soil stimulated the activity of these enzymes (Wang et al., 2020). The difference of stem, leaf and root exudates between red clover and native mixed herbs should be the reason for the difference of soil enzyme activity. Virulence factors are derived from microorganisms and can promote their own infection and cause specific host diseases. It mainly includes bacterial toxins, cell surface proteins that regulate bacterial adhesion, proteins that protect bacteria, cell surface carbohydrates and hydrolytic enzymes that are pathogenic to bacteria (Cai et al., 2018). Compared with native mixed herbs, interplanting red clover in orchard effectively reduced the content of virulence factors (Figure 6).
In this study, interplanting red clover significantly increased soil organic matter content (Figure 6B) and nitrogen fertilizer content (Figures 6C,D). Organic matter has been widely recognized as one of the most important indicators of soil quality and farmland fertility (Kleber and Lehmann, 2015), and plays an important role in maintaining ecosystem productivity and sustainability (Liang et al., 2017). Intercropping red clover effectively increased the content of soil organic matter, which may be related to two reasons. On the one hand, red clover has a higher yield of stems and leaves (Table 1), which are decomposed into soil organic matter. On the other hand, the developed root system (Gu et al., 2016) and high quality root exudates (Kong and Six, 2010) of red clover are another reason to increase organic matter content. Interplanting red clover had a positive effect on total and available nitrogen content in apple orchards, consistent with other reported results (Franche et al., 2009; Manici et al., 2015). Stefani et al. (2018) believed that NO3− leaching was the main way of nitrogen loss in farmland, and that NO3− leaching in soil could be reduced by crop mulching. Nitrogen-fixing rhizobia of red clover can absorb and fix nitrogen in the atmosphere, thereby increasing soil nitrogen levels (Franche et al., 2009).
In summary, the results of this study indicated that intercropping red clover can significantly affect the community structure of soil microorganisms, increase the abundance of Glomeromycota in soil, and improve soil physicochemical properties, which greatly improved the living environment of soil microorganisms. In addition, interplanting red clover can also effectively improve land use efficiency, reduce the content of virulence factor in the soil, increase the content of organic matter, and reduce the use of chemical fertilizers.
As a special terrestrial ecosystem, soil habitats covered by grass in orchards contain abundant microbial resources. The production of specific microbial functional populations and bioactive substances can play a role in regulating and monitoring the healthy growth of fruit trees and soil quality evaluation. In this study, intercropping of red clover in orchards significantly increased the abundance of Glomeromycota, a finding that is worthy of further study. It can be used to isolate, identify and function analysis the fungi and explore the symbiotic relationship between mycorrhizal fungi and fruit roots. In addition, more attention should be paid to the effect mechanism of red clover on soil biological health in orchard. On the one hand, the change characteristics of soil food web and its effect on soil available nutrient supply capacity of this management modes should be studied. On the other hand, it is also necessary to study the mechanism of this management mode on soil pathogenic microorganisms, so as to provide basis for improving the health level of soil and fruit trees. It has guiding significance in improving soil quality, using land efficiently and reducing chemical fertilizer use in terms of application. Breeding industry can be developed based on the excellent herbage production capacity (Table 1) and fertilizer production capacity (Figure 6) of red clover. In order to establish a green production model combining fruit, pasture and livestock, it is necessary to study the carbon cycle and nitrogen cycle mechanism under this model.
The datasets presented in this study can be found in online repositories. The names of the repository/repositories and accession number(s) can be found in the article/supplementary material.
This work was supported by the Natural Science Foundation of Heilongjiang Province under Grant LH2019C062 and the Scientific Research Funds of Heilongjiang Provincial Research Institutes under Grant CZKYF2023-1-C020.
KJ wrote the draft of the manuscript and contributed to manuscript revision. YW, BG, LZ, YL, and PS contributed to data analysis and visualization. All authors contributed to the article and approved the submitted version.
The authors declare that the research was conducted in the absence of any commercial or financial relationships that could be construed as a potential conflict of interest.
All claims expressed in this article are solely those of the authors and do not necessarily represent those of their affiliated organizations, or those of the publisher, the editors and the reviewers. Any product that may be evaluated in this article, or claim that may be made by its manufacturer, is not guaranteed or endorsed by the publisher.
Aislabie, J., and Deslippe, J. R. (2013). “Soil microbes and their contribution to soil services” in Ecosystem services in New Zealand–conditions and trends. ed. J. R. Dymond (New Zealand, Manaaki Whenua Press: Lincoln), 112–161.
Almeida, D. D. O., Klauberg Filho, O., Almeida, H. C., Gebler, L., and Felipe, A. F. (2011). Soil microbial biomass under mulch types in an integrated apple orchard from southern Brazil. Sci. Agric. 68, 217–222. doi: 10.1590/S0103-90162011000200012
Attard, E., Poly, F., Commeaux, C., Laurent, F., Terada, A., Smets, B. F., et al. (2010). Shifts between Nitrospira- and Nitrobacter-like nitrite oxidizers underlie the response of soil potential nitrite oxidation to changes in tillage practices. Environ. Microbiol. 12, 315–326. doi: 10.1111/j.1462-2920.2009.02070.x
Bossio, D. A., Scow, K. M., Gunapala, N., and Graham, K. J. (1998). Determinants of soil microbial communities: effects of agricultural management, season, and soil type on phospholipid fatty acid profiles. Microb. Ecol. 36, 1–12. doi: 10.1007/s002489900087
Bowles, T. M., Acosta-Martínez, V., Calderón, F., and Jackson, L. E. (2014). Soil enzyme activities microbial communities and carbon and nitrogen availability in organic agroecosystems across an intensively-managed agricultural landscape. Soil Biol. Biochem. 68, 252–262. doi: 10.1016/j.soilbio.2013.10.004
Breulmann, M., Schulz, E., Weißhuhn, K., and Buscot, F. (2012). Impact of the plant community composition on labile soil organic carbon, soil microbial activity and community structure in semi-natural grassland ecosystems of different productivity. Plant Soil 352, 253–265. doi: 10.1007/s11104-011-0993-6
Brundrett, M. C. (2002). Coevolution of roots and mycorrhizas of land plants. New Phytol. 154, 275–304. doi: 10.1046/j.1469-8137.2002.00397.x
Cai, P., Liu, X., Ji, D., Yang, S., Walker, S. L., Wu, Y., et al. (2018). Impact of soil clay minerals on growth, biofilm formation, and virulence gene expression of Escherichia coli O157:H7. Environ. Pollut. 243, 953–960. doi: 10.1016/j.envpol.2018.09.032
Chen, L., Brookes, P. C., Xu, J., Zhang, J., Zhang, C., Zhou, X., et al. (2016). Structural and functional differentiation of the root-associated bacterial microbiomes of perennial ryegrass. Soil Biol. Biochem. 98, 1–10. doi: 10.1016/j.soilbio.2016.04.004
Chen, J., and Stark, J. M. (2000). Plant species effects and carbon and nitrogen cycling in a sagebrush-crested wheatgrass soil. Soil Biol. Biochem. 32, 47–57. doi: 10.1016/S0038-0717(99)00124-8
Coleman, M. D., Dickson, R. E., and Isebrands, J. G. (2000). Contrasting fine-root production, survival and soil CO2 efflux in pine and poplar plantations. Plant Soil 225, 129–139. doi: 10.1023/A:1026564228951
Cui, Q., Xia, J., Yang, H., Liu, J., and Shao, P. (2021). Biochar and effective microorganisms promote Sesbania cannabina growth and soil quality in the coastal saline-alkali soil of the Yellow River Delta, China. Sci. total Environ. 756:143801. doi: 10.1016/j.scitotenv.2020.143801
De Deyn, G. B., Quirk, H., Yi, Z., Oakley, S., Ostle, N. J., and Bardgett, R. D. (2009). Vegetation composition promotes carbon and nitrogen storage in model grassland communities of contrasting soil fertility. J. Ecol. 97, 864–875. doi: 10.1111/j.1365-2745.2009.01536.x
Degens, B. P., and Harris, J. A. (1997). Development of a physiological approach to measuring the catabolic diversity of soil microbial communities. Soil Biol. Biochem. 29, 1309–1320. doi: 10.1016/S0038-0717(97)00076-X
Deyn, G. B., Cornelissen, J. H. C., and Bardgett, R. D. (2008). Plant functional traits and soil carbon sequestration in contrasting biomes. Ecol. Lett. 11, 516–531. doi: 10.1111/j.1461-0248.2008.01164.x
Duarte, G. F., Rosado, A. S., Seldin, L., Keijzer-Wolters, A. C., and van Elsas, J. D. (1998). Extraction of ribosomal RNA and genomic DNA from soil for studying the diversity of the indigenous bacterial community. J. Microbiol. Methods 32, 21–29. doi: 10.1016/S0167-7012(98)00004-9
Engedal, T., Karlsson, M., Andersen, M. S., Rasmussen, J., Thorup-Kristensen, K., Jensen, L. S., et al. (2023). Legume-based cover crop mixtures can overcome trade-offs between C inputs, soil mineral N depletion and residual yield effects. Agric. Ecosyst. Environ. 349:108408. doi: 10.1016/j.agee.2023.108408
Florine, D., Nicolas, T., Gilles, C., Marie-Pierre, H., Bernard, B., Bernard, T., et al. (2017). Temporal dynamics of soil microbial communities below the seedbed under two contrasting tillage regimes. Front. Microbiol. 8:1127.
Franche, C., Lindstrm, K., and Elmerich, C. (2009). Nitrogen-fixing bacteria associated with leguminous and non-leguminous plants. Plant Soil 321, 35–59. doi: 10.1007/s11104-008-9833-8
Gilbert, H. J. (2010). The biochemistry and structural biology of plant cell wall deconstruction. Plant Physiol. 153, 444–455. doi: 10.1104/pp.110.156646
Gomez, J. A., Guzman, G. M., Giraldez, J. V., and Fereres, E. (2009). The influence of cover crops and tillage on water and sediment yield, and on nutrient, and organic matter losses in an olive orchard on a sandy loam soil. Soil Tillage Res. 106, 137–144. doi: 10.1016/j.still.2009.04.008
Grayston, S. J., and Campbell, C. D. (1996). Functional biodiversity of microbial communities in the rhizospheres of hybrid larch (Larix eurolepis) and Sitka spruce (Picea sitchensis). Tree Physiol. 16, 1031–1038. doi: 10.1093/treephys/16.11-12.1031
Grayston, S. J., Griffith, G. S., Mawdsley, J. L., Campbell, C. D., and Bardgett, R. D. (2001). Accounting for variability in soil microbial communities of temperate upland grassland ecosystems. Soil Biol. Biochem. 33, 533–551. doi: 10.1016/S0038-0717(00)00194-2
Grayston, S. J., Wang, S., Campbell, C. D., and Edwards, A. C. (1998). Selective influence of plant species on microbial diversity in the rhizosphere. Soil Biol. Biochem. 30, 369–378. doi: 10.1016/S0038-0717(97)00124-7
Gu, C., Liu, Y., Mohamed, I., Xiao, W., Nie, X., Jiang, M., et al. (2016). Dynamic changes of soil surface organic carbon under different mulching practices in citrus orchards on sloping land. PLoS One 11:e0168384. doi: 10.1371/journal.pone.0168384
Huang, J., Wang, J., Zhao, X., Wu, P., Qi, Z., and Li, H. (2014). Effects of permanent ground cover on soil moisture in jujube orchards under sloping ground: a simulation study. Agric. Water Manag. 138, 68–77. doi: 10.1016/j.agwat.2014.03.002
Jiao, K., Qin, S., Lyu, D., Liu, L., and Ma, H. (2013). Red clover intercropping of apple orchards improves soil microbial community functional diversity. Acta Agric. Scand. 63, 466–472. doi: 10.1080/09064710.2013.799219
Kleber, J., and Lehmann, M. (2015). The contentious nature of soil organic matter. Nature 528, 60–68. doi: 10.1038/nature16069
Kong, A., and Six, J. (2010). Tracing root vs. residue carbon into soils from conventional and alternative cropping systems. Soil Sci. Soc. Am. J. 74, 1201–1210. doi: 10.2136/sssaj2009.0346
Liang, C., Schimel, J. P., and Jastrow, J. D. (2017). The importance of anabolism in microbial control over soil carbon storage. Nat. Microbiol. 2:17105. doi: 10.1038/nmicrobiol.2017.105
Liu, Y., Chen, X., Liu, J., Liu, T., Cheng, J., Wei, G., et al. (2019). Temporal and spatial succession and dynamics of soil fungal communities in restored grassland on the Loess Plateau in China. Land Degrad. Dev. 30, 1273–1287. doi: 10.1002/ldr.3289
Manici, L. M., Kelderer, M., Caputo, F., Nicoletti, F., De Luca Picione, F., and Topp, A. R. (2015). Impact of cover crop in pre-plant of apple orchards: relationship between crop health, root inhabiting fungi and rhizospheric bacteria. Can. J. Plant Sci. 95, 947–958. doi: 10.4141/cjps-2015-013
Massawe, P. I., Mtei, K. M., Munishi, L. K., and Ndakidemi, P. A. (2016). Improving soil fertility and crops yield through maize-legumes (Common bean and Dolichos lablab) intercropping systems. J. Agric. Sci., 8:148. doi: 10.5539/jasv8n12p148
Merwin, L. A., and Stiles, W. C. (1994). Orchard Groundcover Management Impacts on Apple Tree Growth and Yield, and Nutrient Availability and Uptake. J. Am. Soc. Hortic Sci. 119, 209–215. doi: 10.21273/jashs.119.2.209
Meseguer Lloret, S., Verdú Andrés, J., Molins Legua, C., and Campíns Falcó, P. (2005). Determination of ammonia and primary amine compounds and Kjeldahl nitrogen in water samples with a modified Roth's fluorimetric method. Talanta 65, 869–875. doi: 10.1016/j.talanta.2004.08.050
Monteiro, A., and Lopes, C. M. (2007). Influence of cover crop on water use and performance of vineyard in Mediterranean Portugal. Agric. Ecosyst. Environ. 121, 336–342. doi: 10.1016/j.agee.2006.11.016
Nelson, D. W., and Sommers, L. E. (1982). “Total carbon, organic carbon and organic matter” in Methods of soil analysis, ASA and SSSA, Madison (Wisconsin USA: Methods of Soil Analysis Part 2. Chemical and Microbial Properties), 534–580.
Philippot, L., Raaijmakers, J. M., Lemanceau, P., and van der Putten, W. H. (2013a). Going back to the roots: the microbial ecology of the rhizosphere. Nat. Rev. Microbiol. 11, 789–799. doi: 10.1038/nrmicro3109
Philippot, L., Spor, A., Hénault, C., Bru, D., Bizouard, F., Jones, C. M., et al. (2013b). Loss in microbial diversity affects nitrogen cycling in soil. ISME J 7, 1609–1619. doi: 10.1038/ismej.2013.34
Pierra, M., Carmona-Martínez, A. A., Trably, E., Godon, J.-J., and Bernet, N. (2015). Microbial characterization of anode-respiring bacteria within biofilms developed from cultures previously enriched in dissimilatory metal-reducing bacteria. Bioresour. Technol. 195, 283–287. doi: 10.1016/j.biortech.2015.07.010
Qian, X., Gu, J., Pan, H. J., Zhang, K. Y., Sun, W., Wang, X. J., et al. (2015). Effects of living mulches on the soil nutrient contents, enzyme activities, and bacterial community diversities of apple orchard soils. Eur. J. Soil Biol. 70, 23–30. doi: 10.1016/j.ejsobi.2015.06.005
Recena, R., García-López, A. M., Quintero, J. M., Skyttä, A., Ylivainio, K., Santner, J., et al. (2022). Assessing the phosphorus demand in European agricultural soils based on the Olsen method. J. Clean. Prod. 379:134749. doi: 10.1016/j.jclepro.2022.134749
Rodrigues, M. Â., Correia, C. M., Claro, A. M., Ferreira, I. Q., Barbosa, J. C., Moutinho-Pereira, J. M., et al. (2013). Soil nitrogen availability in olive orchards after mulching legume cover crop residues. Sci. Hortic. 158, 45–51. doi: 10.1016/j.scienta.2013.04.035
Schimel, J. P., Bennet, J., and Fierer, N. (2005). “Microbial community composition and soil nitrogen cycling: is there really a connection?” in Biological diversity and function in soils. eds. R. D. Bardgett, M. B. Usher, and D. W. Hopkins (New York: Cambridge University Press), 171–188.
Schwarzott, D., Walker, C., and Schüßler, A. (2001). Glomus, the largest genus of the arbuscular mycorrhizal fungi (Glomales), is nonmonophyletic. Mol. Phylogenet. Evol. 21, 190–197. doi: 10.1006/mpev.2001.1007
Stauffer, R. E. (1983). Determination of arsenic and phosphous compounds in groundwater with reduced molybdenum blue. Anal. Chem. 55, 1205–1210. doi: 10.1021/ac00259a006
Stefani, D., Fu, B., Wang, L., Jacinthe, P. A., and Zhao, W. (2018). Quantitative synthesis on the ecosystem services of cover crops. Earth Sci. Rev. 185, 357–373. doi: 10.1016/j.earscirev.2018.06.013
Stott, M. B., and Taylor, M. W. (2016). Microbial ecology research in New Zealand. N. Z. J. Ecol. 40, 12–28. doi: 10.20417/nzjecol.40.2
Sturz, A. V., Christie, B. R., and Matheson, B. G. (2011). Associations of bacterial endophyte populations from red clover and potato crops with potential for beneficial allelopathy. Can. J. Microbiol. 44, 162–167. doi: 10.1139/w97-146
Tsubo, M., Walker, S., and Ogindo, H. O. (2005). A simulation model of cereallegume intercropping systems for semi-arid regions. Field Crops Res. 93:22. doi: 10.1016/j.fcr.2004.09.002
Waldrop, M. P., Balser, T. C., and Firestone, M. K. (2000). Linking microbial community composition to function in a tropical soil. Soil Biol. Biochem. 32, 1837–1846. doi: 10.1016/S0038-0717(00)00157-7
Wang, Y. J., Liu, L., Tian, Y. L., Wu, X., Yang, J., Luo, Y., et al. (2020). Temporal and spatial variation of soil microorganisms and nutrient under white clover cover. Soil Tillage Res. 202:104666. doi: 10.1016/j.still.2020.104666
Ward, S. E., Bardgett, R. D., McNamara, N. P., and Ostle, N. J. (2009). Plant functional group identity influences short-term peatland ecosystem carbon flux: evidence from a plant removal experiment. Funct. Ecol. 23, 454–462. doi: 10.1111/j.1365-2435.2008.01521.x
Xu, Y. M., Wang, X. X., Bai, J. H., Wang, D. W., Wang, W., and Guan, Y. N. (2020). Estimating the spatial distribution of soil total nitrogen and available potassium in coastal wetland soils in the Yellow River Delta by incorporating multi-source data. Ecol. Indic. 111:106002. doi: 10.1016/j.ecolind.2019.106002
Zhang, N., Sun, Y., Wang, E., Yang, J., Yuan, H., and Scow, K. M. (2015). Effects of intercropping and Rhizobial inoculation on the ammonia-oxidizing microorganisms in rhizospheres of maize and faba bean plants. Appl. Soil Ecol. 85, 76–85. doi: 10.1016/j.apsoil.2014.09.008
Zhong, Y., Yan, W., Wang, R., Wang, W., and Shangguan, Z. (2018). Decreased occurrence of carbon cycle functions in microbial communities along with long-term secondary succession. Soil Biol. Biochem. 123, 207–217. doi: 10.1016/j.soilbio.2018.05.017
Zhou, X., Zhang, J., Rahman, M. K., Gao, D., Wei, Z., Wu, F., et al. (2023). Interspecific plant interaction via root exudates structures the disease suppressiveness of? rhizosphere microbiomes. Mol. Plant. 16, 849–864. doi: 10.1016/j.molp.2023.03.009
Zhou, T., Jiao, K., Qin, S., and Lyu, D. (2019). The impact of cover crop shoot decomposition on soil microorganisms in an apple orchard in Northeast China. Saudi J. Biol. Sci. 26, 1936–1942. doi: 10.1016/j.sjbs.2019.07.004
Keywords: interplanting, metagenomic analysis, orchard soil, red clover, soil microorganism
Citation: Jiao K, Wu Y, Guo B, Zhang L, Li Y and Song P (2023) Regulation of microbial community structure, function, and nutrient of apple orchard soil by interplanting red clover. Front. Sustain. Food Syst. 7:1197624. doi: 10.3389/fsufs.2023.1197624
Received: 31 March 2023; Accepted: 15 May 2023;
Published: 01 June 2023.
Edited by:
Sudhakar Srivastava, Banaras Hindu University, IndiaReviewed by:
Xingang Zhou, Northeast Agricultural University, ChinaCopyright © 2023 Jiao, Wu, Guo, Zhang, Li and Song. This is an open-access article distributed under the terms of the Creative Commons Attribution License (CC BY). The use, distribution or reproduction in other forums is permitted, provided the original author(s) and the copyright owner(s) are credited and that the original publication in this journal is cited, in accordance with accepted academic practice. No use, distribution or reproduction is permitted which does not comply with these terms.
*Correspondence: Kuibao Jiao, enhzamtiMTk4M0AxNjMuY29t
Disclaimer: All claims expressed in this article are solely those of the authors and do not necessarily represent those of their affiliated organizations, or those of the publisher, the editors and the reviewers. Any product that may be evaluated in this article or claim that may be made by its manufacturer is not guaranteed or endorsed by the publisher.
Research integrity at Frontiers
Learn more about the work of our research integrity team to safeguard the quality of each article we publish.