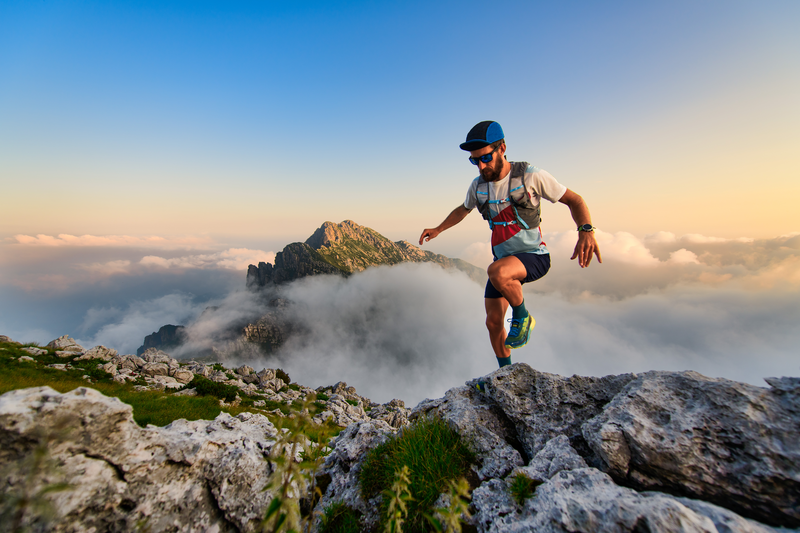
94% of researchers rate our articles as excellent or good
Learn more about the work of our research integrity team to safeguard the quality of each article we publish.
Find out more
ORIGINAL RESEARCH article
Front. Sustain. Food Syst. , 03 August 2023
Sec. Nutrition and Sustainable Diets
Volume 7 - 2023 | https://doi.org/10.3389/fsufs.2023.1183890
This article is part of the Research Topic New Challenges and Future Perspectives in Nutrition and Sustainable Diets in Africa View all 16 articles
Introduction: Bambara groundnut (BGN) is an underutilised, indigenous crop in sub-Saharan Africa. As an underutilised crop the nutritional contribution it can make is not well researched and documented. This study aims to better understand the nutritional profile and protein quality of BGN and the potential of this legume to combat food and nutritional security in rural and malnourished communities.
Methods: Nutritional analysis, including proximate analysis, minerals, total fat and amino acid profile of BGN from three different regions in the Mpumalanga (MP) province of South Africa (SA), were determined. The protein quality was evaluated with various methods including CSEAA, IEAA, PDCAAS and DIAAS and compared with other pulses and legumes in the diet.
Results: BGN consists of - 18,9% carbohydrates, 9,4% protein and 3% fat as consumed. The protein quality is lower than some other commonly consumed pulses but can still be valuable as a complementary protein in the current traditional diet of the region.
Discussion: There is the potential that BGN, together with other commonly consumed plant-based foods (e.g. maize) form a complete protein, that can contribute to protein intake and alleviate the burden of protein-energy malnutrition, specifically in developing countries.
- Carbohydrates and protein are the most abundant nutrients in BGN.
- The protein quantity of BGN does not predict protein quality.
- DIAAS score for BGN is lower than other legumes such as soybeans but higher than chickpeas.
- BGN is a potential complementary food that contributes to improving the protein quality of typical SSA dishes.
- BGN can contribute to dietary diversity as a plant-based protein source.
Food and nutrition security face several challenges, including climate change, the repercussions of the COVID-19 pandemic, the war in Ukraine, and the large-scale production of cash crops such as maize and wheat (Cloete and Idsardi, 2013; Mayes et al., 2019; Tan et al., 2020; FAO et al., 2022). In 2021, one in five people (20.2%) in Africa suffered from hunger and 29.3% of people globally faced moderate to severe food insecurity (FAO et al., 2022). Extreme droughts in Sub-Saharan Africa (SSA) in recent years have severely impacted the agricultural sector and further compromised food and nutrition security in the region. Climate change projections indicate a future of low and erratic rainfall with an increased frequency of drought occurrences in SSA (Mabhaudhi et al., 2018). Given the climate and sustainability challenges, Chivenge et al. (2015) emphasized the need for alternative approaches to ensure food and nutrition security. Researchers have suggested promoting indigenous, drought-resistant, and climate-smart crops as a solution (Massawe et al., 2015; Soumare et al., 2021).
Affording a healthy and balanced diet in the current economic climate has become increasingly difficult (FAO et al., 2022). Animal protein sources have seen a significant price increase, putting them out of reach of many consumers (Moore and Moseley, 2022). Poorer consumers, especially in low-and middle-income countries (LMICs) rely mainly on a cereal-based diet to fulfill their energy needs resulting in an inadequate intake of macro- and micronutrients. Cereal-based foods should be 15% of the diet and yet in many SSA, it comprises over 50% of the diet (Makumba et al., 2016; FAO et al., 2022). The consumption of energy-dense, nutrient-poor cereal-based diets has further contributed to the serious problem of protein-energy-malnutrition (PEM) in SSA along with the increasing rate of NCDs leading to a double-burden of disease (Labadarios et al., 2011; Makumba et al., 2016).
The most recent publication of “The State of Food Security and Nutrition in the World” by the FAO, IFAD, UNICEF, WFP and WHO (2022) calls for a transformed (agri) food system driven by policies that increase the availability of healthy and nutritious foods that are more affordable, sustainable and inclusive. The need for alternative, more affordable protein sources in the diet has become urgent as consumers transition to more climate-positive, plant-based dietary patterns that include plant-based protein sources (van den Berg et al., 2022). Policymakers, producers, and consumers must support this growing demand for good quality, affordable, plant-based protein foods to drive the necessary changes in the current food system (FAO et al., 2022).
Studies on plant-based protein sources from indigenous and traditional crops are necessary to promote climate-resilient indigenous foods that can contribute to dietary diversity, focusing on reintegrating indigenous and traditional foods into the diet (Cloete and Idsardi, 2013). Indigenous and traditional crops are specific to a region and subsequently form part of the endemic plant culture growing readily, despite harsh climate conditions, with minimal intervention as they are adapted to the environment (Modi and Mabhaudhi, 2016; Pieterse et al., 2022). For centuries, more than 7,000 indigenous plant species have been used globally for food, medicine and multiple other uses (Modi and Mabhaudhi, 2016; Akinola et al., 2020). Traditional crops can contribute significantly to social, economic and environmental outcomes. These crops provide subsistence farmers with livelihoods and contribute valuable macronutrients and other essential nutrients (Majola et al., 2021).
A significant proportion of the diverse indigenous and traditional foods available in our environment have been neglected over time despite the development of modern and improved agricultural practices, thereby decreasing dietary diversity and possibly narrowing global food security (Akinola et al., 2020). The lack of information and research on the production methods, post-harvest handling and the role of underutilized indigenous foods in the diet must be addressed to better understand how these foods can contribute to the alleviation of food insecurity in SSA (Cloete and Idsardi, 2013). Therefore, policy support and research on these neglected crops are essential for achieving improved nutrition outcomes, as with the Sustainable Development Goal (SDG) 2 (Zero Hunger) and the United Nations Decade of Action on Nutrition 2016–2025 (United Nations, 2015).
The promotion of underutilized indigenous crops, such as BGN, can furthermore contribute to achieving other SDGs:
• SDG 10—Reduce Inequalities: BGN, like many other indigenous crops, is mainly grown by female subsistence farmers in poor rural areas. The promotion of BGN production can contribute toward more equitable access to nutritious foods resulting in healthier diets and improved food and nutrition security.
• SDG 12—Responsible Consumption Patterns and SDG 13—Climate Action: the transition to plant-based diets becoming more popular in the developed world, climate-resilient foods with low impact on the environment (United Nations, 2015).
Bambara Groundnut (BGN; vigna subterranean (L.) Verdc) is an indigenous and traditional African crop mostly grown by rural female subsistence farmers in SSA (Omoikhoje, 2008). It is an underutilized crop, meaning it is not traded extensively globally, it is produced at low levels and under-researched (Omoikhoje, 2008; Halimi et al., 2019). BGN has gained popularity because of its ability to grow well, even under extreme conditions such as drought and poor soil conditions. Its ability to “fix” nitrogen in the soil makes it ideal for intercropping, especially for smallholder farmers (Mayes et al., 2019). BGN is classified as a drought-tolerant crop as it contains three important mechanisms as described by researchers—avoidance (reduced water loss due to various mechanisms including a prolific route system), escape (short lifecycle or growing period) and tolerance (ability to grow, flower and display economic yield under suboptimal water supply), respectively (Farooq et al., 2009; Mabhaudhi et al., 2018). BGN has confirmed drought avoidance and escape and low water use—giving it all three mechanisms (Chai et al., 2016).
In rural communities where BGN is grown, it is accepted and consumed as part of a regular diet. As BGN is mainly grown by subsistence farmers in SSA across rural and fragmented farmlands, it is very challenging to obtain adequate production and productivity data (Majola et al., 2021). Freshly harvested and dried seeds are purchased from local markets and usually cooked before consumption. However, urban and westernized communities in SSA are generally not familiar with the product and its preparation which pose a challenge to its uptake in the commercial market.
It is primarily grown as a plant-based protein alternative to meat in rural cuisines rather than an oil-producing seed, as commonly seen with other legumes, like soybeans. BGN is consumed in Southern, Eastern and Western Africa and is utilized by various cultures, as shown in Figure 1. Approximately 0.15 million tons of BGN are produced annually in SSA, with the majority being produced by West African countries (Majola et al., 2021). In Southern Africa, it is consumed roasted, boiled or dried with maize porridge or added to cowpea grain for improved flavor (Tan et al., 2020; Majola et al., 2021). Western African countries incorporate it into other foods such as grilled flatbreads, flour, snacks, and biscuits.
Figure 1. Different uses of BGN in SSA (Adapted from Tan et al., 2020 and Majola et al., 2021).
The nutritional contribution, specifically the amino acid and fatty acid composition of cooked BGN in SSA, is poorly researched and documented. Therefore, little is known about the potential role it can play in contributing to the nutritional quality of the diet in rural communities as a drought-resistant traditional crop.
This study aimed to determine the nutrient content of BGN and examines the protein quality of BGN as an underutilized, traditional and drought-resistant indigenous crop in Africa.
All the BGN samples were planted in December 2018 by rural subsistence farmers and harvested in May 2019. A total of 12 fresh samples of approximately 500 g each were collected from 12 farms in three regions (Gomoro, Langeloop and Mbombela) in the Ehlanzeni District Municipality in the Mpumalanga province of South Africa (SA; Table 1; Figure 2). Officials and extension officers from the Department of Agriculture, Rural Development, and Land and Environmental Affairs helped to identify the farms. Samples were harvested mid-morning in autumn directly from the soil. Farmers were compensated for a 500 g sample with the equivalent amount of maize meal. The BGN was placed in labeled brown paper bags and transported to the University of Pretoria in cooler boxes. Samples were placed in sealed plastic bags and in the freezer until cooking and sample preparation could take place.
Table 1. List of regions and farms in Ehlanzeni District Municipality, Mpumalanga, from where BGN samples were collected.
Figure 2. Map of South Africa and districts in the Mpumalanga province (Adapted from Municiplaities Mpumalanga, 2023).
The samples from the three regions were processed separately. The samples from each region were, thawed, combined, soaked in water for 120 min, and then thoroughly washed with tap water (two or three times) to remove all soil debris. Samples from different regions were kept separate. Each batch was cooked in tap water for 60 min at 100°C. After cooking, the samples were drained and cooled. Once cooled, the hull of each BGN was removed manually. The hull was not consumed and, therefore, not included in the analysis. The dehulled BGN samples from each region (Langeloop, Gomoro and Mbombela) were sorted into three colors—cream, mauve and brown (Figure 3). Two 100 g samples of each color from each region were weighed (n = 6 per region), packed, and frozen at −8°C until analysis. A total of n = 18 samples were analyzed.
Figure 3. Different colors of dehulled BGN (cream, mauve, and brown) with scale in centimeters for reference.
Proximate analyses of the samples (n = 18) were carried out by Aspirata laboratory (AssureCloud, Cape Town, SA) to determine the total moisture (Official method of analysis, AOAC 930.15, 2000), ash (AOCS International, 2009) and nitrogen content (Official method of analysis 968.06, AOAC, 2000). The conversion factor of 5.71 (conversion factor for soybeans) was used to calculate the protein content [Food and Agriculture Organization (FAO), 2003]. The energy content for each macronutrient was calculated by multiplying the g/100 value with the respective conversion factors given in the list below (Greenfield and Southgate, 2003).
• Protein = 17
• Fat = 37
• Carbohydrates = 17
• Total dietary fiber = 8
The Agricultural Research Council’s analytical laboratory determined the amino acid profile in Pretoria, SA. Three separate analyses used high-performance liquid chromatography (HPLC) with fluorescence detection for amino acid determination. All three analyses were completed by hydrolysis.
The first hydrolysis analyzed arginine, serine, aspartic acid, glutamic acid, glycine, threonine, alanine, tyrosine, proline, hydroxyproline, methionine, valine, phenylalanine, isoleucine, leucine, histidine, and lysine, excluding cysteine and tryptophan. The freeze-dried BGN sample was hydrolyzed with 6-N-hydrochloric acid at 110°C for 24 h in a vacuum. An internal standard was added to the hydrolysate and filtered. Nitrogen flow was then used to dry a portion of the hydrolysate. The derivatization of the hydrolysate was done with 9-fluorenylmethyl chloroformate (FMOC-Cl). The amino acid content was determined with HPLC with an eluent of a tertiary gradient of pH, methanol and acetonitrile (Einarsson et al., 1983). The second hydrolysis determined cysteine and was the same as the first except for the cysteine oxidation to cystic acid before hydrolysis using a peroxide formic acid solution (Gehrke et al., 1985). The third hydrolysis determined the tryptophan content and involved enzymatic hydrolysis using protease. The hydrolysis was filtered and tryptophan content was determined by HPLC equipped with an AMinoTAg column and fluorescence detection (DeVries et al., 1980).
The total fat, saturated fat, polyunsaturated fat and monounsaturated fat content were determined for each sample (n = 18) at the Aspirata laboratory (AssureCloud, Cape Town, SA). Fat was determined by hydrolyzing the sample with concentrated hydrochloric acid in a boiling water bath for 3 h. Thereafter, the fat was extracted using petroleum ether and gravimetrically quantified. For the determination of the fatty acids, fat fractions were trans-methylated with methanol-potassium hydroxide. The fatty acid methyl esters were extracted with n-hexane and analyzed using gas chromatography with flame ionization detection (GC-FID) according to the official method of analysis AOAC 996.06 (2000).
Available carbohydrates were determined for each sample (n = 18) by Aspirata laboratory (AssureCloud, Cape Town, SA) using the Megazyme Available Carbohydrates and Dietary Fiber Assay Procedure from Kit K-ACHDF, 11/08 (Megazyme, 2018). The total dietary fiber reported was determined by the Aspirata laboratory (AssureCloud, Cape Town, SA) using an enzymatic-gravimetric method [AOAC 985.29 (2000)] method.
Iron and zinc were analyzed for each sample (n = 18) by the Aspirata laboratory (AssureCloud) according to the AOAC Official Method AOAC 999.10 (2012). Samples were digested with 3 M nitric acid and 30% (w/w) hydrogen peroxide in a closed vessel heated by microwaves. Iron and zinc were quantified by inductively coupled plasma optical emission spectroscopy (ICP-OES) using an acidified calibration range.
The assessment of protein quality did not involve regional or color differentiation; instead, the mean amino acid values were employed by aggregating data from all regions for the calculations and multiple protein quality assessment methods were utilized. These methods included the Chemical Score of the Essential Amino Acids (CSEAA) or CS (Mitchell and Block, 1946; Eq. 1), the Essential Amino Acid Index (IEAA; Oser, 1959; Rolinec et al., 2017; Huang et al., 2018; Abdualrahman et al., 2019; Eq. 2), Protein Digestibility-Corrected Amino Acid Score [PDCAAS; Food and Agriculture Organization (FAO), 2013; Eq. 3], and Digestible Indispensable Amino Acid Score [DIAAS; Food and Agriculture Organization (FAO), 2013, 2014; Eq 4].
The CSEAA/CS was calculated in relation to the amino acid pattern of the reference requirements outlined.
The Essential Amino Acid Index (EAAI) evaluates the presence of indispensable amino acids. In Equation 2 (Eq. 2), the variables a, b, c, …, j represent the content of histidine, isoleucine, leucine, lysine, methionine, phenylalanine, tyrosine, threonine, and valine in the sample. The variables ap, bp, cp, …, jp denote the content of histidine, isoleucine, leucine, lysine, methionine, phenylalanine, tyrosine, threonine, and valine in the reference protein according to the guidelines. The value of n corresponds to the total number of amino acids, accounting for pairs such as methionine and cysteine, which are treated as a single entity in the calculation.
The PDCAAS was calculated by dividing the amount of the limiting essential amino acid in the test protein by the amount of the same essential amino acid in a reference protein and then multiplying it by the digestibility coefficient (Eq. 3). The limiting essential amino acid is the amino acid present in the lowest quantity relative to the reference protein. PDCAAS values exceeding 1 were adjusted or truncated to 1 [Food and Agriculture Organization (FAO), 2013].
DIAAS evaluates protein quality based on the digestibility (bioavailability) of individual dietary indispensable amino acids. The measurement of amino acid digestibility is preferably conducted in humans, but if not feasible, it can be done in pigs or rats [Food and Agriculture Organization (FAO), 2013; Rutherfurd et al., 2015]. DIAAS was calculated by dividing the standardized ileal digestible amino acid content of the test protein by the standardized ileal digestible amino acid content of the reference protein, multiplied by 100 (Eq. 4). In the absence of a specific ileal digestibility value for BGN, the value reported for chickpeas, 84, reported by Han et al. (2020) was used instead. DIAAS values were not truncated.
The nutrient values from each region were consolidated (n = 6 per region), and a mean value was calculated and reported. Additionally, an overall mean for all the regions combined (n = 18) was determined and included in the analysis. Statistical analysis was conducted using GenStat for Windows (2008) software (Payne et al., 2009). The significance of all variables measured for each sample was tested using a one-way analysis of variance (ANOVA). The locality effect was tested with a Fisher’s protected t-test least significant difference at the 5% level of significance.
The nutrient composition of Bambara groundnut (BGN) was analyzed and compared in this study. The moisture content of the cooked edible portion ranged from 55.6 to 56.7%, with an average of 56.1% (Table 2). These values were similar to those reported in the West African Food Composition Tables [54.7%; Food and Agriculture Organization (FAO), 2019]. Factors such as long storage, thawing, cooking, and refreezing may have influenced the moisture content in the current study, along with differences in soil and climate conditions compared to the West African region.
Table 2. Summary of the nutrient composition of cooked Bambara Groundnut [per 100 g edible portion (EP)] from three different regions in South Africa.
Carbohydrates and protein were found to be the most abundant macronutrients in BGN. The carbohydrate content ranged from 17.5 to 20.4 g per 100 g of edible portion (EP), which did not significantly differ between regions (Table 2). It was slightly lower than a study by Tan et al. (2020) (30 g/100 g EP) and similar to the values reported in the West African Food Composition Tables [16.0 g/100 g EP; Food and Agriculture Organization (FAO), 2019]. The dietary fiber content averaged at 11.4 g/100 g EP and did not differ significantly between regions (Table 2). However, it was lower than the values reported in the West African Food Composition Tables for BGN and higher than the values for soybeans (4.8 g/100 g EP) and chickpeas (6.6 g/100 g EP) in South Africa (SAFOODS, 2017). The dietary fiber content can be influenced by factors such as maturity stage and processing methods (Tan et al., 2020).
The mean fat content of BGN was 2.97 g/100 g EP, similar to the values reported in the West African Food Composition Tables [2.70 g/100 g EP; Table 2; Food and Agriculture Organization (FAO), 2019]. The fat primarily consisted of unsaturated fatty acids (2.08 g/100 g EP). However, the total fat content showed significant differences between regions (Hlanga et al., 2021). These differences may be attributed to genotypical differences among landraces. Compared to other legumes like soybeans, BGN has a relatively low fat content and is not suitable for oil extraction (Halimi et al., 2019).
Iron content did not significantly differ between regions, and the mean value (0.827 mg/100 g EP) in this study was notably lower than the value reported in the West African Food Composition Tables [1.90 mg/100 g EP; Table 2; Food and Agriculture Organization (FAO), 2019]. Variations in iron content among different BGN landraces, influenced by water regimes, locations, seasons, and harvesting methods, may explain the differences between regions and the West African Food Composition Tables (Gqaleni, 2014). Zinc was not detected in the BGN samples, which may be attributed to the soaking and cooking process (Tan et al., 2020). However, the West African Food Composition Tables [1.08 mg/100 g EP; Food and Agriculture Organization (FAO), 2019] and other studies have reported BGN as a valuable source of zinc (Singh and Pratap, 2016; Halimi et al., 2019). It is important to note that these studies reported compositional values of raw BGN without considering the effects of storage, soaking, and cooking. Cooking processes can improve nutrient digestibility and bioavailability by breaking down antinutritional factors such as phytic acid and tannins (Oyeyinka et al., 2019).
The protein content of BGN was analyzed in the present study, yielding levels between 7.65 and 9.10 g per 100 g of EP (Table 2; Tan et al., 2020). Significant differences in protein content were observed among the three regions (Table 2). The mean protein value per 100 g of edible portion was determined to be 8.54 g (equivalent to 21.3 g per 100 g dry basis). A review by Nwadi et al. (2020) on available nutrient content data revealed a range of 9.6 to 40 g per 100 g dry basis for protein in BGN. Variations in protein content can be attributed to factors such as genetic background (landraces), growing conditions, and analytical techniques, including the specific nitrogen conversion factor employed (Friedman, 1996; Morris, 2006). It is worth noting that no formal classification of BGN species has been conducted in SA.
The essential amino acids (EAAs) necessary for human nutrition include phenylalanine, valine, tryptophan, threonine, isoleucine, methionine, histidine, leucine, and lysine, with arginine considered as a conditional EAA (Morris, 2006). In the present study, four amino acids (arginine, histidine, phenylalanine, threonine) among the nine EAAs displayed significant differences between the three regions (Table 3). Arginine, leucine, and lysine were found to be the most abundant EAAs, while sulfur-containing amino acids such as methionine and cysteine were present in relatively low concentrations. Methionine was identified as the limiting amino acid, consistent with the findings of Halimi et al. (2019). The United States Department of Agriculture [U.S Department of Agriculture, Agriculture Research Service (USDA), 2014] reported similar low concentrations of sulfur-containing amino acids in soybeans (0.224 g/100 g EP) and chickpeas (0.116 g/100 g EP) compared to BGN. Heat processing methods, such as boiling and roasting, can enhance the bioavailability of certain EAAs in BGN compared to their levels in raw seeds (Omoikhoje, 2008; Akande et al., 2009; Oyeyinka et al., 2019; van den Berg et al., 2022). However, prolonged boiling has been shown to reduce the availability of lysine in other legumes like soybeans, necessitating further investigation into heat processing methods for BGN (van den Berg et al., 2022).
Protein quality refers to the ability of a protein to fulfill metabolic functions by providing specific amino acid patterns, and it is equally important as protein content (Millward et al., 2008). Accurate measurement of protein quality and digestibility of food products relies on evaluating amino acid concentrations (Huang et al., 2018). Amino acid analysis can offer insights into a food’s ability to meet an individual’s amino acid requirements (Caire-Juvera et al., 2013; Huang et al., 2018; van den Berg et al., 2022).
Various methods exist to assess the protein quality of food products, including the Essential Amino Acid Index (EAAI), Protein Digestibility-Corrected Amino Acid Score (PDCAAS), and Digestible Indispensable Amino Acid Score (DIAAS). The protein quality measurements for this study are summarized in Table 4. EAAI evaluates protein quality based on the contribution of all essential amino acids, as described by Oser (1959) and Rao et al. (1959). The calculated EAAI index for the three regions is 1.49, slightly lower than that of canned chickpeas (1.66; Halimi et al., 2019). According to Oser’s method, the protein quality of BGN can be considered good.
PDCAAS is the most commonly used method for protein quality assessment due to its cost-effectiveness and efficiency (Schaafsma, 2012). This method was developed by the FAO Expert Consultation group on protein quality and human requirements [Food and Agriculture Organization (FAO)/World Health Organization (WHO), 1991]. The has limitations, such as truncating scores to 1.0, which may inaccurately estimate the importance of proteins in mixed diets (Huang et al., 2018). PDCAAS values exceeding 100% are truncated to 1.0 since the body cannot utilize more than what has been absorbed (Schaafsma, 2012). In the present study, the reported PDCAAS value for cooked BGN is 52% (Table 4), which is not truncated to 1.0. This value is lower than PDCAAS values reported for other commonly consumed pulses like chickpeas and soybeans, which range from 80% to 100%. The PDCAAS value for raw BGN was reported as 32% (Halimi et al., 2019). The increase in PDCAAS value after soaking and cooking suggests that heat treatment enhances the protein digestibility of BGN.
DIAAS is the current standard for assessing protein quality and was introduced in 2013 as the recommended method [Food and Agriculture Organization (FAO), 2013, 2014; van den Berg et al., 2022]. In the present study, the DIAAS value calculated for BGN is 69 (Table 4), indicating that BGN can meet just under three-quarters of an adult’s daily indispensable amino acid requirements. Similar to the PDCAAS value, the DIAAS value in the present study is lower than that reported for soybeans (DIAAS = 90/EP; Herreman et al., 2020) and (DIAAS = 86.4/EP; van den Berg et al., 2022) but higher than that of cooked chickpeas (DIAAS = 49/EP; Han et al., 2020). While the IEAA score suggests that BGN potentially contains high-quality protein, however, considering both the PDCAAS and DIAAS scores, the protein content of BGN can be considered lower quality compared to soybeans.
Table 4 presents the chemical score of essential amino acids, where the lowest score represents the first limiting amino acid. For BGN, methionine had a score below 1, indicating it as the first limiting amino acid. This finding is consistent with the results reported by Halimi et al. (2019) for BGN and similar to those reported for soybeans by van den Berg et al. (2022).
In SSA, the consumption of cereals forms the basis of local diets (Singh et al., 2007). The consumption of pulses in SSA is relatively low, at around 6% [Food and Agriculture Organization (FAO), 2008]. To improve dietary diversity and nutritional quality, an approach focusing on the inclusion of a variety of nutrient-rich foods is needed in SSA (Schönfeldt and Hall, 2012). As seen in Figure 1, BGN is primarily consumed with maize porridge in Southern Africa. Maize porridge, like many other grains consumed in large quantities in SSA, is rich in methionine but deficient in lysine (Boye et al., 2010; Makumba et al., 2016). The concept of combining different foods to enhance the amino acid profile is known as “complementation” (Tas et al., 2019). Due to its higher lysine concentration, BGN has the potential to complement maize porridge, resulting in a meal with improved protein quality. The recommended ratio of legume to maize for addressing lysine deficiency in maize is 70% cereal to 30% legume (Ejigui et al., 2007). The amino acid profile of maize meal in SSA is typically low in lysine and contains trace amounts of essential amino acids such as methionine and tryptophan [Food and Agriculture (FAO)/Government of Kenya, 2018], contradicting reports that maize porridge is high in methionine. Calculating the DIAAS score of maize meal porridge using this data reveals a score of zero due to the trace amounts of essential amino acids. However, combining maize meal porridge and BGN in a 70/30 ratio increases the DIAAS score from zero to 20.7%, thereby enhancing the protein quality and nutritional contribution of this common dish in SSA. The inclusion of BGN in the local diet can improve dietary diversity and nutrient quality.
Considering the adverse impact of climate change on agriculture and the high cost of animal protein sources, incorporating indigenous products like BGN along with local products such as maize meal porridge can promote dietary diversity and contribute to achieving SDG 2—Zero Hunger—by 2030. BGN can be considered an affordable plant-based protein source that has a positive climate impact in SSA. It is important to carefully consider the preparation and cooking methods of BGN, as these can affect the nutrient density and bioavailability of nutrients, particularly protein quality, as observed with soybeans (van den Berg et al., 2022).
In conclusion, the cooked BGN has an average carbohydrate content of 18.9 g per 100 g edible portion, while the fat content was 3 g per 100 g edible portion, predominantly consisting of unsaturated fatty acids. The iron content was 0.827 mg per 100 g edible portion, and zinc was not detected. The protein content of cooked BGN, calculated using a nitrogen conversion factor of 5.71, was 9.35 g per 100 g. However, when considering protein quality using the PDCAAS method, the value was below 1. Comparatively, the DIAAS value for BGN was lower than that of soybeans but similar to chickpeas. BGN exhibited a high lysine content but a low methionine content, aligning with the characteristics of other pulses.
BGN represents a climate-positive crop that has the potential to contribute to a more nutrient-dense diet in the face of the ongoing climate and cost-of-living challenges. The utilization of BGN as a complementary protein source with low-protein staples such as maize holds great potential for enhancing the protein quality of the diet.
Research on the classification of BGN species in South Africa is fragmented. Therefore research on the formal classification of BGN species can lead to targeted breeding, potentially optimizing the amino acid composition, to optimize BGN as a potential complimentary food for lysine-poor cereals. More research on seeds’ different species and origins is required for targeted breeding, which can improve the amino acid composition.
The original contributions presented in the study are included in the article/supplementary material, further inquiries can be directed to the corresponding author.
ZV and BP substantially contributed to the conception or design of the work or the acquisition, analysis, or interpretation of data for the work, drafted the work or critically revised for important intellectual content. HS provided approval for publication of the content. ZV, BP, and HS agreed to be accountable for all aspects of the work in ensuring that questions related to the accuracy or integrity of any part of the work are appropriately investigated and resolved. All authors contributed to the article and approved the submitted version.
This work was supported by the Economic and Social Research Council [grant number ES/T003871/1] under the ARUA-GCRF UKRI Partnership Program as part of the Capacity Building in Food Security (CaBFoodS-Africa) project.
The authors would like to thank the Department of Science and Technology (DSI) /National Research Foundation (NRF) South African Research Chairs Initiative (SARChI) in the National Development Plan Priority Area of Nutrition and Food Security (Unique number: SARCI170808259212).
The authors declare that the research was conducted in the absence of any commercial or financial relationships that could be construed as a potential conflict of interest.
All claims expressed in this article are solely those of the authors and do not necessarily represent those of their affiliated organizations, or those of the publisher, the editors and the reviewers. Any product that may be evaluated in this article, or claim that may be made by its manufacturer, is not guaranteed or endorsed by the publisher.
The grant holders acknowledge that opinions, findings and conclusions or recommendations expressed in any publication generated by NRF-supported research are those of the author(s) and that the NRF accepts no liability whatsoever.
Abdualrahman, M. A. Y., Ma, H., Yagoub, A. E. A., Zhou, C., Ali, A. O., and Yang, W. (2019). Nutritional value, protein quality and antioxidant activity of Sudanese sorghum-based kissra bread fortified with bambara groundnut (Voandzeia subterranea) seed flour. J. Saudi Soc. Agric. Sci. 18, 32–40. doi: 10.1016/j.jssas.2016.12.003
Akande, K. E., Abubakar, M. M., Adegbola, T. A., Bogoro, S. E., Doma, U. D., and Fabiyi, E. F. (2009). Nutrient composition and uses of Bambara groundnut (Vigna subterranea (L.) Verdcourt). Continent J Food Sci Technol 3, 8–13.
Akinola, R., Pereira, L. M., Mabhaudhi, T., De Bruin, F. M., and Rusch, L. (2020). A review of indigenous food crops in Africa and the implications for more sustainable and healthy food systems. Sustainability 12:3493. doi: 10.3390/su12083493
AOAC. (2000). Official method of analysis 974.24; 985.29; 992.16. Maryland, USA: Association of Official Analytical Chemists.
AOCS International. (2009). Official method of analysis ba 51-49. Illinois, USA: American Oil Chemist Society.
Boye, J., Zare, F., and Pletch, A. (2010). Pulse proteins: processing, characterization, functional properties and applications in food and feed. Food Res. Int. 43, 414–431. doi: 10.1016/j.foodres.2009.09.003
Caire-Juvera, G., Vázquez-Ortiz, F. A., and Grijalva-Haro, M. I. (2013). Amino acid composition, score and in vitro protein digestibility of foods commonly consumed in northwest Mexico. Nutr. Hosp. 28, 365–371. doi: 10.1007/s10681-015-1581-2
Chai, H. H., Massawe, F., and Mayes, S. (2016). Effects of mild drought stress on the morpho-physiological characteristics of a bambara groundnut segregating population. Euphytica 208, 225–236. doi: 10.1007/s10681-015-1581-2
Chivenge, P., Mabhaudhi, T., Modi, A. T., and Mafongoya, P. (2015). The potential role of neglected and underutilised crop species as future crops under water scarce conditions in sub-Saharan Africa. Int. J. Environ. Res. Public Health 12, 5685–5711. doi: 10.3390/ijerph120605685
Cloete, P. C., and Idsardi, E. F. (2013). Consumption of indigenous and traditional food crops: perceptions and realities from South Africa. Agroecol. Sustain. Food Syst. 37, 902–914. doi: 10.1080/21683565.2013.805179
DeVries, J. W., Koski, C. M., Egberg, D. C., and Larson, P. A. (1980). Comparison between a spectrophotometric and a high-pressure liquid chromatography method for determining tryptophan in food products. J. Agric. Food Chem. 28, 896–898. doi: 10.1021/jf60231a025
Einarsson, S., Josefsson, B., and Lagerkvist, S. (1983). Determination of amino acids with 9-fluorenylmethyl chloroformate and reversed-phase high-performance liquid chromatography. J. Chromatogr. A 282, 609–618. doi: 10.1016/S0021-9673(00)91638-8
Ejigui, J., Savoie, L., Marin, J., and Desrosiers, T. (2007). Improvement of the nutritional quality of a traditional complementary porridge made of fermented yellow maize (Zea mays): effect of maize–legume combinations and traditional processing methods. Food Nutr. Bull. 28, 23–34. doi: 10.1177/156482650702800103
FAO, IFAD, UNICEF, WFP and WHO. (2022). The state of food security and nutrition in the world 2022. Repurposing food and agricultural policies to make healthy diets more affordable. Rome, FAO.
Farooq, M., Wahid, A., Kobayashi, N., Fujita, D., and Basra, S. M. A. (2009). Plant drought stress: effects, mechanisms and management. Agron. Sustain. Dev. 29, 185–212.
Food and Agriculture (FAO)/Government of Kenya, (2018). Kenya food composition tables. Nairobi, 254 pp. Available at: http://www.fao.org/3/I9120EN/i9120en.pdf
Food and Agriculture Organization (FAO), (2003). Food energy—methods of analysis and conversion factors. Report of a technical workshop. Rome, Italy: Food and Agriculture Organization of the United Nations.
Food and Agriculture Organization (FAO). (2008). FAOSTAT database. Available at http://faostat3.fao.org.
Food and Agriculture Organization (FAO), (2013). Dietary protein quality evaluation in human nutrition. Rome: Food and Agriculture Organization of the United Nations.
Food and Agriculture Organization (FAO), (2014). Research approaches and methods for evaluating the protein quality of foods. Rome: Food and Agriculture Organization of the United Nations.
Food and Agriculture Organization (FAO), (2019). West African food composition table. Rome: Food and Agricultural Organisation.
Food and Agriculture Organization (FAO)/World Health Organization (WHO), (1991). Protein quality evaluation. Rome, Italy: Food and Agriculture Organization of the United Nations.
Friedman, M. (1996). Nutritional value of proteins from different food sources. A review. J. Agric. Food Chem. 44, 6–29. doi: 10.1021/jf9400167
Gehrke, C. W., Wall, L. L. Sr., Absheer, J. S., Kaiser, F. E., and Zumwalt, R. W. (1985). Sample preparation for chromatography of amino acids: acid hydrolysis of proteins. J. Assoc. Off. Anal. Chem. 68, 811–821. doi: 10.1093/jaoac/68.5.811
Gqaleni, P., (2014). “Nutitional value of bambara groundnut (vigna subterranea (l.) verdc.): A human and animal perspective”, MSc Thesis. University of Kwazulu Natal, South Africa.
Greenfield, H., and Southgate, D. A., (2003). Food composition data: Production, management, and use. Rome, Italy: Food and Agriculture Organization.
Halimi, R. A., Barkla, B. J., Mayes, S., and King, G. J. (2019). The potential of the underutilized pulse bambara groundnut (Vigna subterranea (L.) Verdc.) for nutritional food security. J. Food Compos. Anal. 77, 47–59. doi: 10.1016/j.jfca.2018.12.008
Han, F., Moughan, P. J., Li, J., and Pang, S. (2020). Digestible indispensable amino acid scores (DIAAS) of six cooked Chinese pulses. Nutrients 12:3831. doi: 10.3390/nu12123831
Herreman, L., Nommensen, P., Pennings, B., and Laus, M. C. (2020). Comprehensive overview of the quality of plant-and animal-sourced proteins based on the digestible indispensable amino acid score. Food Sci. Nutr. 8, 5379–5391. doi: 10.1002/fsn3.1809
Hlanga, N. C., Modi, A. T., and Mathew, I. (2021). Evaluating nutritional content among Bambara groundnut lines. J. Food Compos. Anal. 102:104053. doi: 10.1016/j.jfca.2021.104053
Huang, S., Wang, L. M., Sivendiran, T., and Bohrer, B. M. (2018). Amino acid concentration of high protein food products and an overview of the current methods used to determine protein quality. Crit. Rev. Food Sci. Nutr. 58, 2673–2678. doi: 10.1080/10408398.2017.1396202
Labadarios, D., Steyn, N. P., and Nel, J. (2011). How diverse is the diet of adult south Africans? Nutr. J. 10, 1–11.
Mabhaudhi, T., Chibarabada, T. P., Chimonyo, V. G. P., and Modi, A. T. (2018). Modelling climate change impact: a case of bambara groundnut (Vigna subterranea). Phys Chem Earth Parts A/B/C 105, 25–31. doi: 10.1016/j.pce.2018.01.003
Majola, G. N., Gerrano, A. S., and Shimelis, H. (2021). Bambara groundnut (Vigna subterranean [L.] Verdc.) production, utilisation and genetic improvement in sub-Saharan Africa. Agronomy 11, 1–16. doi: 10.3390/agronomy11071345
Makumba, C. T., Njobeh, P. B., Oluwafemi, A. A., Olugbile, A. O., and Kayitesi, E. (2016). The role of compositing cereals with legumes to alleviate protein energy malnutrition in Africa. Int. J. Food Sci. Technol. 51, 543–554. doi: 10.1111/ijfs.13035
Massawe, F. J., Mayes, S., Cheng, A., Chai, H. H., Cleasby, P., Symonds, R., et al. (2015). The potential for underutilised crops to improve food security in the face of climate change. Procedia Environ. Sci. 29, 140–141. doi: 10.1016/j.proenv.2015.07.228
Mayes, S., Ho, W. K., Chai, H. H., Gao, X., Kundy, A. C., Mateva, K. I., et al. (2019). Bambara groundnut: an exemplar underutilised legume for resilience under climate change. Planta 250, 803–820. doi: 10.1007/s00425-019-03191-6
Megazyme, (2018). Available carbohydrates assay kit. Available at: https://www.megazyme.com/available-carbohydrates-assay-kit (Accessed 12 March 2022).
Millward, D. J., Layman, D. K., Tomé, D., and Schaafsma, G. (2008). Protein quality assessment: impact of expanding understanding of protein and amino acid needs for optimal health. Am. J. Clin. Nutr. 87, 1576S–1581S. doi: 10.1093/ajcn/87.5.1576S
Mitchell, H. H., and Block, R. J. (1946). Some relationships between the amino acid contents of proteins and their nutritive values for the rat. J. Biol. Chem. 163, 599–620. doi: 10.1016/S0021-9258(17)41289-0
Modi, A. T., and Mabhaudhi, T., (2016). Developing a research agenda for promoting underutilised, indigenous and traditional crops. Pretoria: Water Research Commission.
Moore, H. L., and Moseley, K. L. (2022). Prosperity for whom? Systemic injustice and the UK economy. UCL Institute for Global Prosperity: London, UK.
Municiplaities Mpumalanga. (2023). National Government of South Africa. Available at: https://municipalities.co.za/provinces/view/6/mpumalanga (Accessed 19 February 2023).
Nwadi, O. M., Uchegbu, N., and Oyeyinka, S. A. (2020). Enrichment of food blends with bambara groundnut flour: past, present, and future trends. Legume Sci 2:e25. doi: 10.1002/leg3.25
Omoikhoje, S. O. (2008). Assessment of the nutritive value of Bambara groundnut as influenced by cooking time. Livest. Res. Rural. Dev. 20, 1–10.
Oser, B. L. (1959). “An integrated essential amino acid index for predicting the biological value of proteins” in Protein and amino acid nutrition. ed. A. A. Albanese (New York: Academic Press), 295–311.
Oyeyinka, A. T., Pillay, K., and Siwela, M. (2019). Full title-in vitro digestibility, amino acid profile and antioxidant activity of cooked Bambara groundnut grain. Food Biosci. 31, 100428–100410. doi: 10.1016/j.fbio.2019.100428
Payne, R. W., Murray, D. A., Harding, S. A., Baird, D. B., and Soutar, D. M., (2009). Gen stat for windows introduction. Hemel Hempstead: VSN International.
Pieterse, E., Millan, E., and Schönfeldt, H. C. (2022). Consumption of edible flowers in South Africa: nutritional benefits, stakeholders' views, policy and practice implications. Br. Food J. 125, 2099–2122. doi: 10.1108/BFJ-10-2021-1091
Rao, P. R., Metta, V. C., and Johnson, B. C. (1959). The amino acid composition and the nutritive value of proteins: I. essential amino acid requirements of the growing rat. J. Nutr. 69, 387–391. doi: 10.1093/jn/69.4.387
Rolinec, M., Daniel, B. Í. R. O., Galik, B., Šimko, M., Juráček, M., and Hanušovský, O. (2017). Essential amino acid index of sow’s colostrum. J. Cent. Eur. Agric. 19, 95–101. doi: 10.5513/JCEA01/19.1.2028
Rutherfurd, S. M., Fanning, A. C., Miller, B. J., and Moughan, P. J. (2015). Protein digestibility-corrected amino acid scores and digestible indispensable amino acid scores differentially describe protein quality in growing male rats. J. Nutr. 145, 372–379. doi: 10.3945/jn.114.195438
SAFOODS. (2017). SAMRC food composition tables for South Africa. 5th Edn. Cape Town: South African Medical Research Council.
Schaafsma, G. (2012). Advantages and limitations of the protein digestibility-corrected amino acid score (PDCAAS) as a method for evaluating protein quality in human diets. Br. J. Nutr. 108, S333–S336. doi: 10.1017/S0007114512002541
Schönfeldt, H. C., and Hall, N. G. (2012). Dietary protein quality and malnutrition in Africa. Br. J. Nutr. 108, S69–S76. doi: 10.1017/S0007114512002553
Singh, R. J., Chung, G. H., and Nelson, R. L. (2007). Landmark research in legumes. Genome 50, 525–537. doi: 10.1139/G07-037
Singh, N. P., and Pratap, A. (2016). Food legumes for nutritional security and health benefits. Biofortification of food crops, 41–50.
Soumare, A., Diedhiou, A. G., and Kane, A. (2021). Bambara groundnut: a neglected and underutilized climate-resilient crop with great potential to alleviate food insecurity in sub-Saharan Africa. J. Crop Improv. 36, 747–767. doi: 10.1080/15427528.2021.2000908
Tan, X. L., Azam-Ali, S., Goh, E. V., Mustafa, M., Chai, H. H., Ho, W. K., et al. (2020). Bambara groundnut: an underutilized leguminous crop for global food security and nutrition. Front. Nutr. 7, 1–16. doi: 10.3389/fnut.2020.601496
Tas, A. A., Umit, N., Alkan, R. O., Boynak, A., and Yeral, S. D. (2019). Approximation of protein quality (DIAAS) of vegetarian dishes served in restaurants. Madridge J Agric Environ Sci. 1, 1–5. doi: 10.18689/ijons-1000101
U.S Department of Agriculture, Agriculture Research Service (USDA). (2014). USDA nutrient database for standard reference, release 27. Nutrient Data Laboratory Home Page. Available at: http://ndb.nal.usda.gov/ndb/search/visit.
United Nations, (2015). ‘Transforming our world: the 2030 agenda for sustainable development’, Sustainable Development. Available at: https://sustainabledevelopment.un.org/post2015/transformingourworld/publication (Accessed 12 March 2022).
Keywords: Bambara Groundnut, legumes, underutilized foods, protein quality, dietary diversity, plant-based protein sources, drought resistant
Citation: Veldsman Z, Pretorius B and Schönfeldt HC (2023) Examining the contribution of an underutilized food source, Bambara Groundnut, in improving protein intake in Sub-Saharan Africa. Front. Sustain. Food Syst. 7:1183890. doi: 10.3389/fsufs.2023.1183890
Received: 10 March 2023; Accepted: 20 July 2023;
Published: 03 August 2023.
Edited by:
Rakesh Bhardwaj, Indian Council of Agricultural Research (ICAR), IndiaReviewed by:
Sapna Langyan, Indian Council of Agricultural Research (ICAR), IndiaCopyright © 2023 Veldsman, Pretorius and Schönfeldt. This is an open-access article distributed under the terms of the Creative Commons Attribution License (CC BY). The use, distribution or reproduction in other forums is permitted, provided the original author(s) and the copyright owner(s) are credited and that the original publication in this journal is cited, in accordance with accepted academic practice. No use, distribution or reproduction is permitted which does not comply with these terms.
*Correspondence: Zani Veldsman, emFuaWRwbEBnbWFpbC5jb20=
Disclaimer: All claims expressed in this article are solely those of the authors and do not necessarily represent those of their affiliated organizations, or those of the publisher, the editors and the reviewers. Any product that may be evaluated in this article or claim that may be made by its manufacturer is not guaranteed or endorsed by the publisher.
Research integrity at Frontiers
Learn more about the work of our research integrity team to safeguard the quality of each article we publish.