- Department of Horticultural Sciences, Faculty of Agriculture and Natural Resources, University of Hormozgan, Bandar Abbas, Iran
Tomato fruits are usually characterized by a short postharvest life. Their quality decreases during storage due to losses in nutritional quality and decay. Salicylic acid (SA) has an important role in plant development and response to environmental stress. This research aimed at evaluating the effects of preharvest salicylic acid application on extending the storage life, and ripening of tomatoes (Solanum lycopersicum cv. Baraka). As a hybrid seed, the “Baraka” cultivar is appropriate for subtropical regions. Three consecutive weeks before harvest, foliar spraying with SA was applied at different concentrations (1, 2, and 4 mM). After storage for 10, 20, 30, and 40 days at 10°C with 85–95% relative humidity (RH), the tomatoes had decreased levels of a* value (redness), decay, electrolyte leakage, and chilling injury after being treated with salicylic acid. In comparison to the control, these characteristics were linked to increased levels of firmness, titratable acidity, ascorbic acid, and ascorbate peroxidase activity. According to the findings, fruit quality was best maintained at 4 mM SA, whereas it was least effective at 1 mM SA. Overall, the results showed that, SA can be considered a potent treatment that can effectively control postharvest loss.
1. Introduction
Tomato is a popular vegetable that has a predominant role in many diets worldwide (Manaa et al., 2014; Konagaya et al., 2020). Reportedly, physicochemical processes such as respiration rate, ethylene synthesis, cell wall, and chlorophyll degradation play essential roles in fruit softening and ripening. Therefore, during the ripening and senescence processes of tomato fruits, significant changes can occur in flavor, metabolite content, color, texture, and plant hormones (Bai et al., 2021). On the other hand, to preserve the quality and freshness of perishable products, it is necessary to limit the increase in respiratory rate during storage, thus advancing the senescence process and extending the shelf-life of perishable crops (Supapvanich and Promyou, 2013).
According to recent studies (Bai et al., 2021; Roohanitaziani et al., 2022), it is well known that managing the postharvest loss of fruits in modern agriculture is of great importance due to the globalization of markets, which may involve transits over very long distances between producers and consumers. In tomatoes, an approach to the early management of postharvest loss is to pick green or unripe fruits instead of red and ripe fruits. This is because the latter is more prone to damage during the delivery and postharvest periods. While picking unripe fruits can extend shelf life, it can also lead to a decrease in quality.
An efficient treatment that is commonly used in the horticulture industry is cold storage, through which postharvest life can be extended and fruit quality can be maintained (Bourne, 2006; Aghdam et al., 2013; Bai et al., 2021). Using cold storage entails specific limitations in the postharvest management of fruits and vegetables, which are usually susceptible to chilling injury (CI), especially when stored in cold storage (Ding et al., 2002; Aghdam et al., 2013). According to Elhadi and Jeffrey (2012), green mature tomatoes have a higher chance of being damaged by low temperatures than red and ripe tomatoes. The occurrence of chilling injury can be likely if the green tomatoes are stored at temperatures as low as 12.5°C or, 13°C (Rugkong, 2009) or, 10°C (Zhao et al., 2022). Saltveit (2001) reported that the severity of chilling injury (CI) depends on the duration of exposure and the specific temperature below the non-freezing threshold at around 12°C. CI symptoms include an inability to develop full fruit color, enhanced permeability of cellular membranes, and a higher prevalence of disease and decay (Saltveit, 2001; Ding et al., 2002).
Reactive oxygen species (ROS) are usually generated in plants after exposure to biotic and abiotic environmental stress. The activity of ROS tends to harm cellular structures. Nonetheless, the deleterious effects of ROS can be mitigated by a combination of non-enzymatic antioxidants, such as carotenoids and ascorbate, as well as enzymatic antioxidants, ascorbate peroxidase (APX) activity (Baninaiem et al., 2016). Therefore, the defensive response in plants is known as systemic acquired resistance (SAR), which can contribute to plant resistance against a wide range of pathogens (Ding et al., 2002).
Postharvest technology aims to enhance the natural resistance of horticultural produce against environmental stress during storage. The available literature cites several indications of safe, and environmentally-friendly techniques, like salicylic acid (SA), for postharvest management. SA is a safe compound for the maintenance of postharvest quality in vegetables and fruits (Supapvanich and Promyou, 2013; Aghdam et al., 2016). SA is a phenolic compound that acts as a plant hormone and exhibits a high potential for decreasing postharvest loss. Its mechanism of action relies on the regulation of membrane integrity, antioxidant system activity, and phenolic compounds. Also, it aids in reducing ethylene production and the spread of fungal contamination (Zavala et al., 2004; Cong et al., 2017; Amiri et al., 2021).
The initiation of SAR is related to elevated concentrations of SA, both at the infection site and frequently in remote tissues. Kumar et al. (2020) reported that the dose of SA for exogenous application on different kinds of fruits and vegetables is varied and limited. It is suggested in earlier studies that the optimum range for SA, which are non-toxic to fruit and vegetables, is approximately 0.5–2.0 mM and the high concentration can cause the harm to fruit skin and can lead to the attacks of fungi (Aghdam et al., 2016).
Hayat et al. (2010) reported that MeSA levels in plant tissues also parallel the increase in SA concentration locally and systemically after infections. The following results have been obtained from experiments conducted on tomatoes using MeSA: Zhang et al. (2011) mentioned that after 4 weeks at 2°C, the CI index of cherry tomato fruit was 26% higher than that of MeSA-treated (at a concentration of 0.05 mM) fruit. Tomato fruits at the mature green stage were treated with salicylic acid at concentrations of 1 and 2 mM and then stored at a temperature of 1°C for 3 weeks. The results showed that the application of salicylic acid treatment effectively reduced chilling injury in tomato fruits. Additionally, a concentration of 2 mM was found to be the most effective (Aghdam et al., 2012a). MeSA efficiently delayed CI in tomatoes treated at the pink maturity stage at a concentration of 10−4 M before cold storage at 0°C for 3 weeks (Fung et al., 2006).
Shi et al. (2018) reported that exogenous SA can enhance resistance to pathogens and reduce decay incidence in grapefruit. The results showed that the combination of SA with chitosan treatment provided more effective control against postharvest diseases than when either SA or chitosan was used alone. Also, Zhang et al. (2022) reported that combining blue light (BL) and SA reduced the rate of weight loss and delayed its onset, thereby preventing strawberry decay. Spraying with SA 2 weeks before the harvest of peach fruit caused an increase in postharvest shelf life (Salyari et al., 2021). According to research by Adhikary et al. (2020), SA treatment decreased decay incidence and maintained the postharvest quality of pear fruits for up to 60 days in cold storage.
In recent studies, exogenous SA could be used to enhance the chilling resistance of tomatoes (Ding et al., 2002), cucumber (Cao et al., 2009), peach (Benati et al., 2021), pomegranate (Sayyari et al., 2009), and sponge gourd (Cong et al., 2017). In the case of kiwifruit and banana, salicylic acid reportedly delayed ripening while the fruits were stored (Zhang et al., 2003). Given the possible long time it takes from the harvest to the consumption of tomatoes, this period is characterized by many physiological changes that occur in the fruit. The ultimate goal of this study was to investigate the effects of SA treatment, by spraying, with the aim of maintaining the qualitative attributes of tomatoes in cold storage while rendering their postharvest life longer.
2. Materials and methods
2.1. Plant materials and SA treatment
Tomato fruits (Solanum lycopersicum cv. Baraka) were cultivated in greenhouse conditions at University of Hormozgan, Iran. SA was applied as a preharvest spray on the tomatoes once a week, consecutively, for 3 weeks. The applied concentrations of SA were 1, 2, and 4 mM. After 3 weeks, harvesting was done when the fruits were in the mature green stage (fully expanded but unripe fruit with mature seed). The biological color chart was used to determine the fruit’s color and maturity level. The fruits were relocated to the laboratory and selected for uniformity in color, size, and weight. After dividing the fruits, they were washed with distilled water and air-dried. Each treatment group consisted of 60 fruits with three repetitions (i.e., 20 fruits per replicate). The tomatoes were maintained at 10°C and 85–95% relative humidity (RH) for 10, 20, 30, and 40 days (Hatami et al., 2013). They were sampled for quality evaluation every 10 days during the storage period.
2.2. Firmness and weight loss
Fruit firmness was calculated by a penetrometer (6 mm diameter). The required force was provided through a flat probe that penetrated the fruit at two points. The amount of force was measured and the firmness was expressed as kg/cm-2 units (Shafiee et al., 2010). The measurement of weight loss (%) involved calculating the percentage of differences between tomato weights at the beginning and end of the experiment, according to the following formula:
2.3. Color assessment
Fruit color was determined as the values of L* (black-white), a* (green-red), and b* (blue-yellow). Mean values were calculated from color assessment at three different points on the fruit by a Minolta colorimeter model CR 400, Japan.
2.4. Chilling injury index and electrolyte leakage
The index of chilling injury (CI) was individually assessed by visible symptoms on the surface of tomato fruits (pitting and dehydration), the symptoms of which were estimated as scores according to a type of classification at 4 levels: 0 (no chilling injury), 1 (1–25%), 2 (25–50%), and 3 (>50%) (Sayyari et al., 2016). CI symptoms were calculated by the following equation:
According to a method by Mirdehghan et al. (2007), electrolyte-leakage (EL) was determined from 6 pieces of fruit tissue (10 mm in diameter) that were cut with a cork borer. The disks were put on a shaker to be incubated for 4 h in 25 mL (0.4 M) mannitol. Electrical conductivity at the beginning (E1) was determined with a conductivity-meter (Ttracon WTW 325). Then, to measure the final conductivity, autoclaving was carried out at 121°C for 15 min, and then the temperature was reduced to 20°C. The rate of EL (%) was calculated by the following formula:
2.5. Decay index
Decay incidences in fruits were evaluated on a subjective scale. The decay on fruit surfaces was scaled from 1 to 5, resulting differently from each treatment, based on which 1 = no decay, 2 = decay lower than 5% of the fruit surface, 3 = decay between 5 and 20% of the fruit surface, 4 = decay between 20 and 50% of the fruit surface, and 5 = decay more than 50% of the fruit surface (Babalar et al., 2007).
2.6. Ascorbic acid content
To measure ascorbic acid, a spectrophotometer was operated (Etemadipoor et al., 2020) after homogenizing the fruit samples (1 g) with distilled water (10 mL). The procedure was followed by filtering the solution. Metaphosphoric acid (1%) was incorporated into the filtered solution (0.1 mL). Then, 9 mL of colored 2, 6-dichlorophenolindophenol (0.0025%) was poured into the solution (1 mL). After thoroughly shaking it, a spectrophotometer was used to read the absorbance (Cecil, UK) (517 nm). The ascorbic acid was reported as mg per 100 g of fruit tissue.
2.7. Titratable acidity (TA), total soluble solid (TSS), and flavor index
TA was determined by titrating 5 mL of fruit juice with 0.1 N NaOH, which gradually reached pH 8.1, and TA was reported in percentages of citric acid (Saltveit, 2005). Total soluble solids were measured by a digital refractometer. The data were expressed as Brix values, and the flavor index was calculated as TSS/TA.
2.8. Ascorbate peroxidase activity
The activity of ascorbate peroxidase was measured according to the method of Nakano and Asada (1981), where 5.0 g was sampled from the pulp of fresh tissue and homogenized with potassium phosphate buffer (5 mL), pH 7.0, and 1 mM EDTA. Crude enzymes were extracted from the supernatant. The sample absorbance was measured at 290 nm for 1 min (UV–visible spectrophotometer, Cecil, UK).
2.9. The experimental design
Statistical analysis was carried out by a completely randomized factorial design (CRD), accompanied by three SA concentrations and three replications. The analysis of the data was performed using SAS software (version 9.4), and differences among the means were statistically compared with the Least Significant Difference (LSD) test at p ≤ 0.05.
3. Results
3.1. Firmness and weight loss
After 20 days, a rapid decline occurred in the firmness of the fruits when the storage period ended. However, this decrease in the firmness of treated fruits was slower than in the control. Salicylic acid at a 4 mM concentration caused better maintenance of fruit firmness compared to the other two treatments, and the difference was statistically significant compared to the control (Figure 1). An increase in weight loss was observed during storage. According to the results, the interaction effects of spraying concentration and time caused no significant changes in weight loss (data not shown).
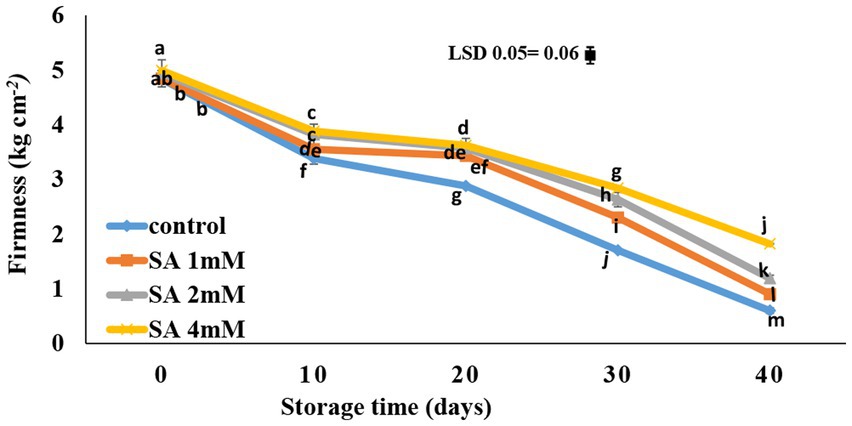
Figure 1. Effects of pre-harvest SA treatments on the firmness of tomatoes stored at 10°C for up to 40 days. Bars show standard errors (±SE) of mean values of the three replications.
3.2. Color assessment
As shown in (Table 1A), lightness (L*) was measured in SA-treated fruits at harvest time. Ultimately, the treated fruits had higher lightness values compared to the fruits of the control group, and the 4 mM SA concentration made L* decrease slowly during storage. According to the results, no significant changes were observed in the L* value during the 40 days of storage.
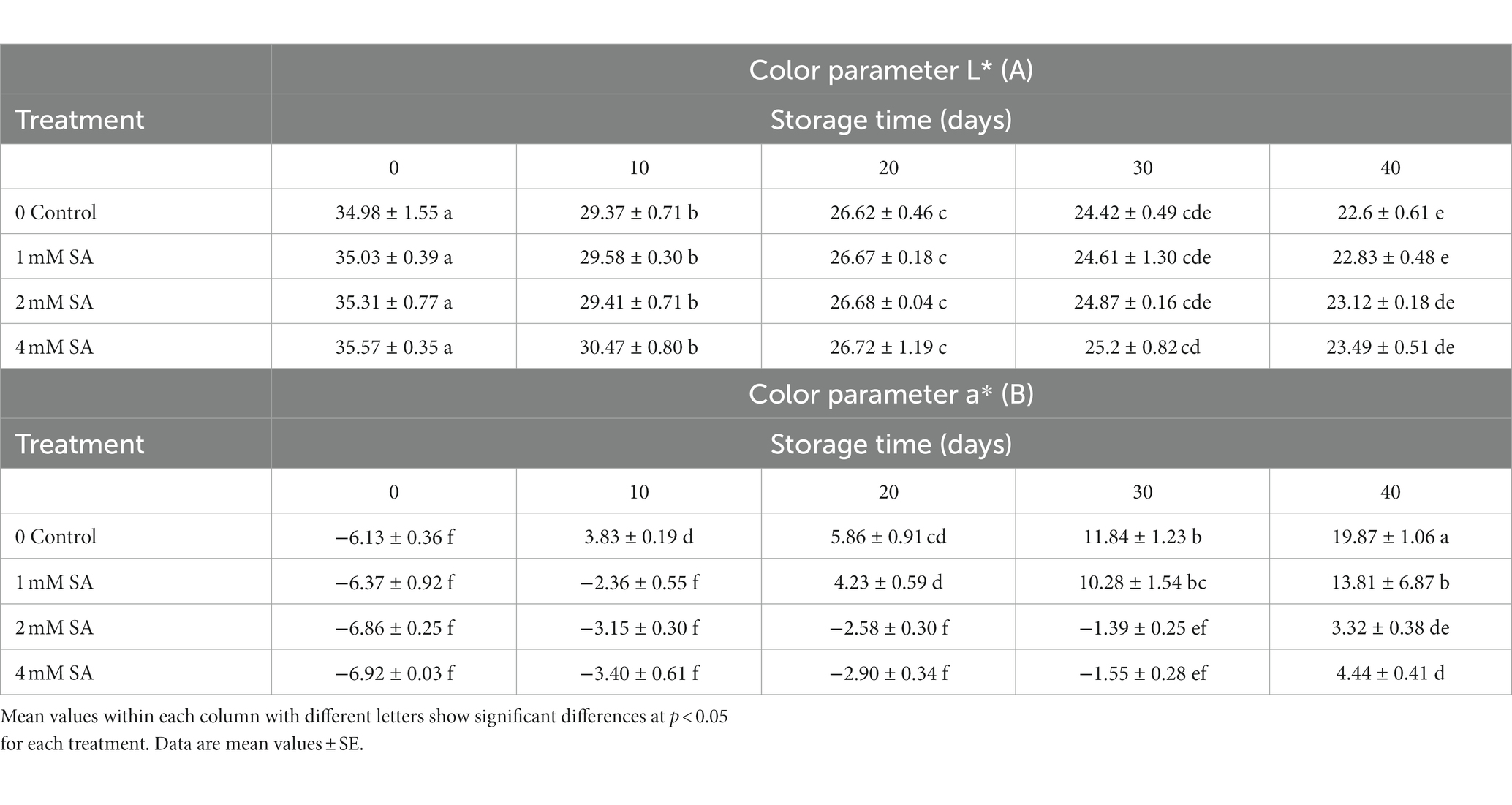
Table 1. Effect of preharvest treatments of salicylic acid on L*, a* of tomatoes stored at 10°C for up to 40 days.
During 40 days of cold storage, SA-treated fruits had significantly lower a* values, compared to the fruits of the control group (Table 1B). This meant that the fruits appeared less red compared to the control group. During 40 days, the a* value of tomato fruits increased constantly, irrespective of the treatments. Spraying with 2 and 4 mM SA led to effective maintenance of a* compared to the control and fruits of the 1 mM SA treatment. When tomatoes are green and reach the pink stage, the a* value changes from negative to positive. With the progress in fruit maturity, the a* value of tomatoes increased at a faster rate. In the control, the value of redness (a*) increased more rapidly compared to the treated fruits 10 days after storage, and the coloring process of the treated fruits was slower. The a* value was greatest in fruits that were treated with 1 mM SA after 40 days of storage (Table 1B).
3.3. Chilling injury and electrolyte leakage
Chilling symptoms were not observed until the 20th day of storage. On day 30, all treatment groups showed symptoms of chilling injury, except for the 4 mM treatment group. The smallest chilling injury occurred in response to the 4 mM SA concentration, whereas lower SA concentrations (1 mM and 2 mM) led to higher levels of chilling injury (Figure 2A). Generally, electrolyte leakage increased during storage. At harvest time, the amount of electrolyte leakage in the control was higher compared to the amount observed in the treatment groups. In comparison with the control, significantly lower levels of electrolyte leakage were observed in response to 2 and 4 mM SA at the end of storage life. There was no significant difference between the control and the treatment groups at 1 mM SA (Figure 2B).
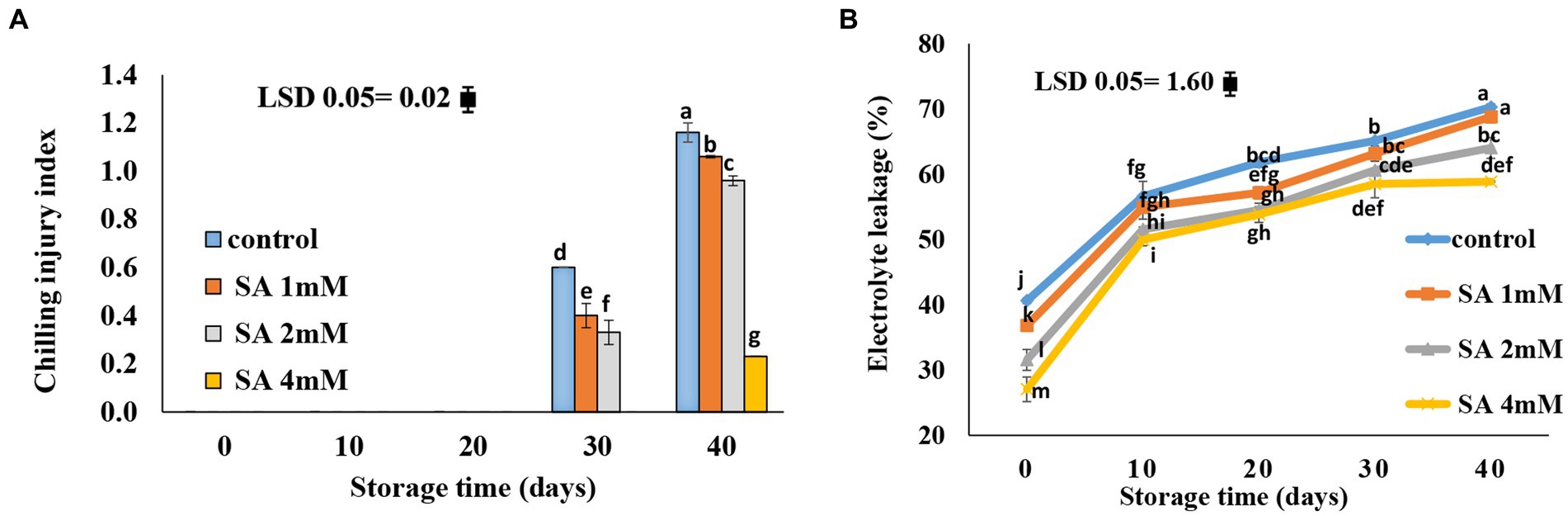
Figure 2. Effects of pre-harvest SA treatments on chilling injury and electrolyte leakage of tomatoes stored at 10°C for up to 40 days. (A) Chilling injury, (B) electrolyte leakage. Bars show standard errors (±SE) of the mean values of the three replications.
3.4. Fruit decay index (scores)
Decay symptoms were not observed until the 20th day of storage. On day 30, all treatment groups showed symptoms of decay except the 4 mM SA treatment group. A slower rate of decay was observed in response to the application of 4 mM SA (Figure 3).
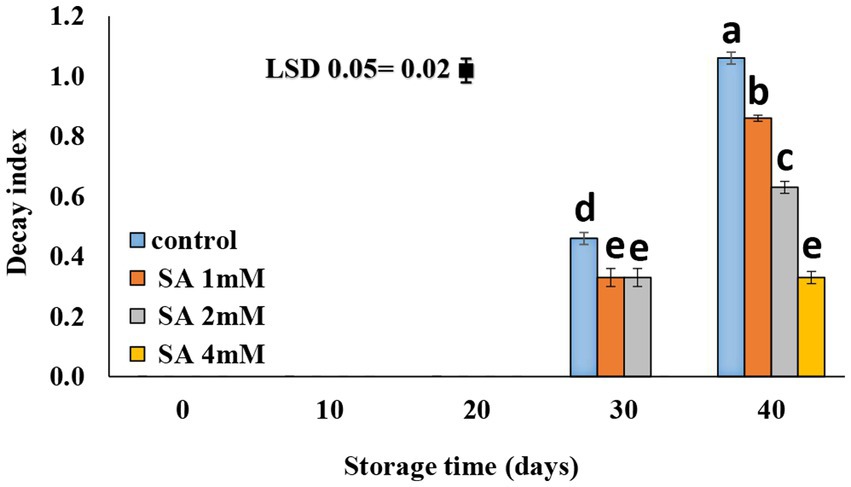
Figure 3. Effect of pre-harvest SA treatments on decay content of tomato fruits during storage at 10°C for up to 40 days. The bars show standard errors (±SE) for the mean values of the three replications.
3.5. Ascorbic acid
At harvest time, the highest vitamin C content was observed in the fruits of the 4 mM treatment group. No significant difference was observed in the vitamin C content of fruits in treatment groups of 1 and 2 mM on the 40th day of storage (Figure 4). There was a gradual decline in ascorbic acid content in all fruits until the storage period ended. The least amount was observed in the control, compared to the other treatments.
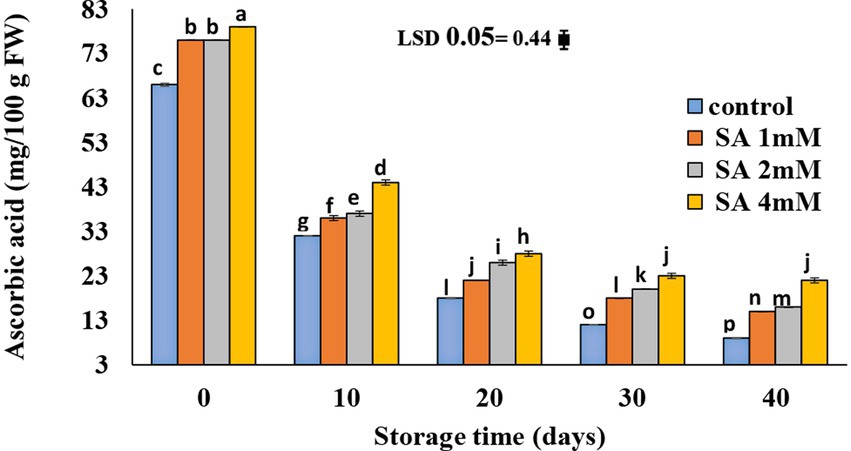
Figure 4. Effects of pre-harvest SA treatments on ascorbic acid of tomatoes stored at 10°C for up to 40 days. Bars show standard errors (±SE) of mean values of the three replications.
3.6. TA, TSS, and TSS/TA ratio
The titratable acidity (TA) content decreased in all treated tomatoes, whereas TSS showed a reverse trend during storage. The highest TSS content (19.02%) was observed in the control tomatoes (Figures 5A,B). As depicted in Figure 5C, among the three concentrations of SA, the 4 mM concentration caused the lowest flavor index, whereas the fruits of the control group (22.17%) had a higher index compared to the other treatments.
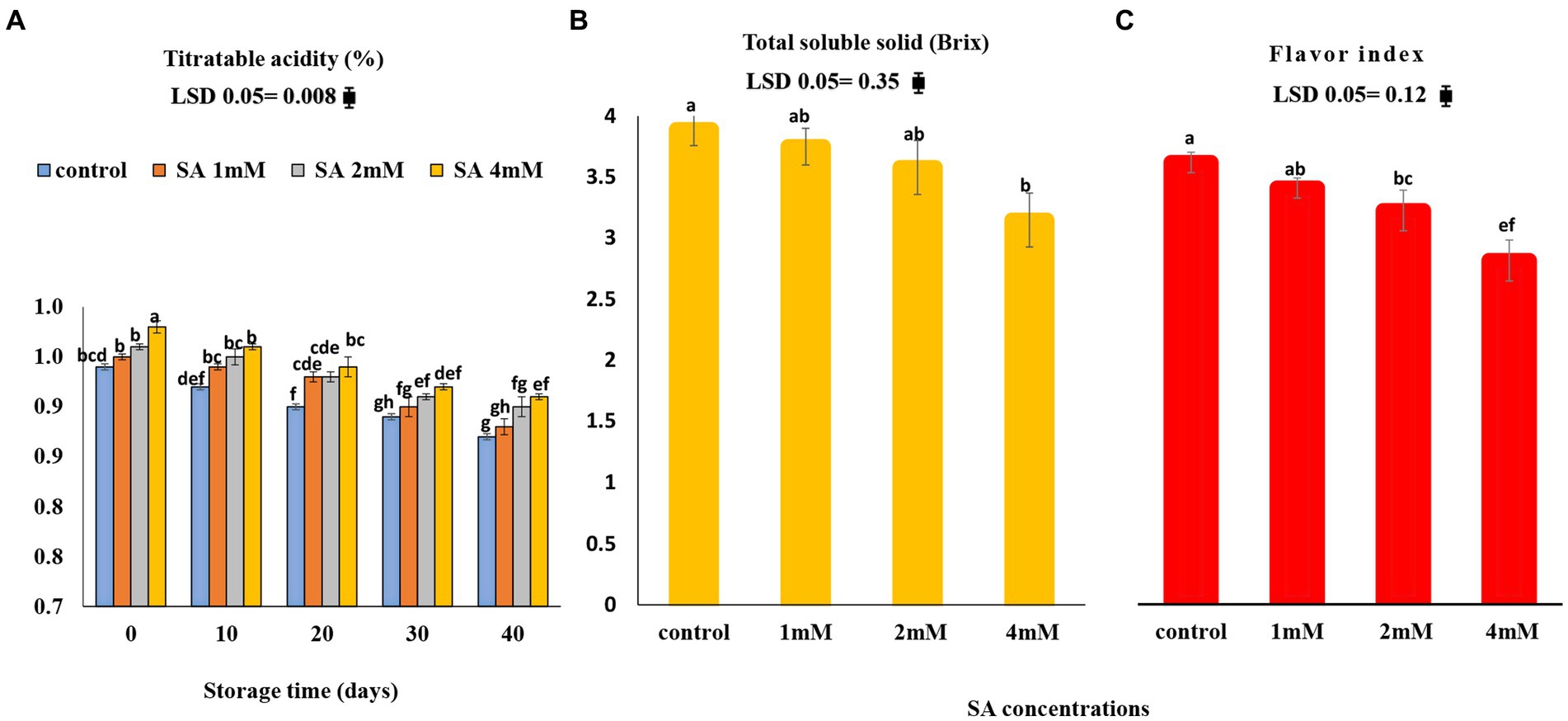
Figure 5. Effects of pre-harvest SA treatments on the flavor index of tomatoes stored at 10°C for up to 40 days. (A) Titratable acidity, (B) total soluble solid, (C) TSS/TA ratio. Bars show standard errors (±SE) of mean values of the three replications.
3.7. Ascorbate peroxidase activity
According to the results, the APX activity gradually decreased in the treated fruits (Figure 6). After 10 days of storage, there was a rapid decrease in the activity of APX in the fruits of the control group. In response to 4 mM SA, the APX content became significantly different compared to the control on the 30th and 40th days. At the end of storage, the highest APX activity was observed in response to 4 mM SA, whereas the control group had the lowest content.
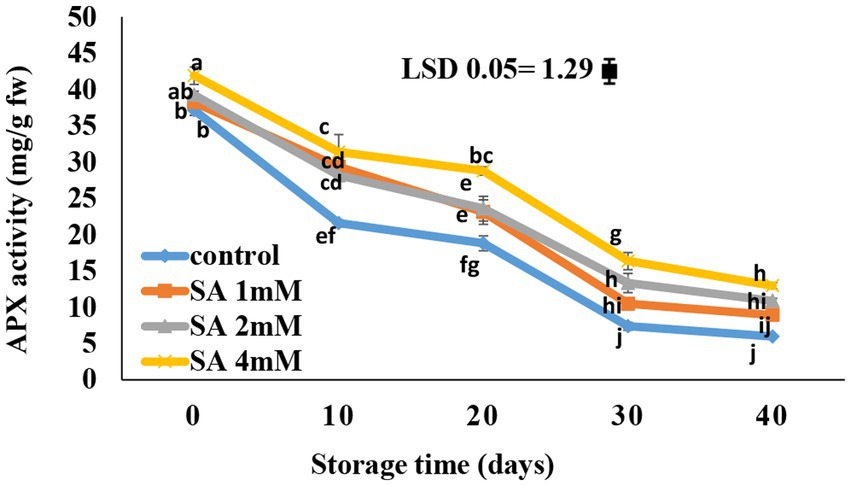
Figure 6. Effects of pre-harvest SA treatments on ascorbate peroxidase activity of tomatoes stored at 10°C for up to 40 days. Bars show standard errors (±SE) of the mean values of the three replications.
4. Discussion
According to the results, salicylic acid caused the maintenance of fruit firmness compared to the control (Figure 1). Aghdam et al. (2016) suggested that SA has a high potential for maintaining postharvest quality, delaying softening, prolonging the shelf life, and controlling the postharvest loss of vegetables and fruits. Also, high concentrations of SA were reportedly associated with greater fruit firmness. While the application of salicylic acid (4 mM) significantly maintained the fruit firmness in the current study, the results are in agreement with other reports; e.g., in the case of table grapes, SA led to greater firmness in the fruits at the postharvest stage (Champa et al., 2014). Similar results were also reported in pepper fruit (Elwan and El-Hamahmy, 2009), kiwifruit (Zhang et al., 2003; Aghdam et al., 2010), and apple (Mo et al., 2008). Furthermore, many studies confirmed that SA could be applied to maintain firmness and extend the storage life of vegetables and fruits (Sayyari et al., 2011; Supapvanich and Promyou, 2013).
In addition, the firmness of the cucumber fruit was maintained at a higher level when treated with SA at a concentration of 0.5 mM (Cao et al., 2009). According to the results of Wang et al. (2006), peaches treated with 1 mM SA had a higher firmness compared to the control group.
Weight loss was not significant. However, some results were found to be contradictory regarding strawberries (Shafiee et al., 2010), pepper fruit (Elwan and El-Hamahmy, 2009), and table grapes (Champa et al., 2014). A report by Shafiee et al. (2010) indicated that weight loss in fruits and vegetables occurs because of transpiration, respiration, and metabolic activity. While SA can decrease fruit weight loss and respiration rate by stomatal closure, this is attributed to its role as an electron donor, which mitigates the effects of free radicals on normal respiration.
During storage, the L* value showed no significant changes (Table 1A). The results are in line with earlier findings reported by Shafiee et al. (2010), who reported that SA treatment did not affect the color lightness (L*) of strawberries. Also, these results are in agreement with Champa et al. (2014), who found that SA treatment on table grapes caused a gradual decrease in their L* values, irrespective of the treatments during the 75 days of cold storage. SA-treated fruits had lower a* values compared to the control (Table 1B). Batu (2004) and Cheema et al. (2014) suggested that the a* value is a good parameter for the assessment of color change and, thus, the degree of ripening in tomatoes. It is one of the quality factors of fresh tomatoes in consumer perception. Furthermore, Supapvanich and Promyou (2013) reported on fruit color during storage, which involved large changes in the ratio of pigments as indexes of ripening and senescence. The importance of these indexes through color assessment can define the quality attributes of postharvest horticultural products. Spraying with SA caused an increase in the lycopene of tomatoes and decreased the rates of lycopene and B-carotene breakdown. It seems that SA activated lycopene biosynthesis while contributing to the regulation of enzymes relating to the maintenance of lycopene (Huang et al., 2008). These results are in line with earlier ones by Champa et al. (2014) about table grapes. Fruit clusters of grapes that were treated with 2.0 mM SA significantly maintained higher a* values than in the control and the 1.0 mM SA treatment. Similar findings were reported in pepper fruits (Elwan and El-Hamahmy, 2009) and pomegranates (Sayyari et al., 2011), although controversial results were reported on strawberries, where the preharvest application of SA was not effective on a* values compared to the control (Shafiee et al., 2010).
The lower chilling injury occurred in the 4 mM SA concentration, whereas the 1 mM and 2 mM, observed the higher chilling injury (Figure 2A), and electrolyte leakage was lower in the 2 and 4 mM SA concentrations than in the control (Figure 2B). Beckles (2012) reported that tomato fruits are usually placed in cold storage (10–15°C) to prolong their storage life. However, at temperatures below 12.5°C, some cultivars of tomato fruits may decay from chilling injury. The severity of chilling injury symptoms is greater in green fruits than in red fruits, and the response to chilling injury increases as a function of temperature and storage time.
According to previous results, Sayyari et al. (2011) suggested that chilling injury occurs primarily on the plasma membrane, with changes occurring in phospholipid compositions that initiate a cascade of secondary reactions leading to the destruction of cell wall structures. This membrane injury can be measured by electrolyte leakage. In the case of pomegranate, the electrolyte leakage occurred more significantly on the fruit skin of the control than on that of the treated pomegranate fruits. Furthermore, Aghdam et al. (2016) suggested that treatments of MeJA and MeSA vapor at times of fruit storage alleviated chilling injury in pomegranate fruits and maintained nutrition-related qualities in the fruits at low temperatures. SA plays an important role in triggering defense mechanisms against chilling stress. Oxidative stress, which is a precursor of reactive oxygen species in the plasma membrane, is reportedly associated with oxidative injury under chilling stress. However, plant tolerance to chilling stress is mostly dependent on the level of antioxidant enzyme activity (Cao et al., 2010). Using SA on table grapes at preharvest caused lower values of electrolyte leakage during the postharvest period (4°C) (Champa et al., 2014). Application of SA at concentrations of 0.4, 0.8, and 1.2 mM during storage effectively preserved the membrane integrity (Mo et al., 2008), and SA treatment at the postharvest stage reduced the severity of CI in tomatoes (Ding et al., 2001, 2002; Fung et al., 2006; Zhang et al., 2011; Aghdam et al., 2012a,b) and peaches (Wang et al., 2006). Cao et al. (2009) demonstrated that the application of SA at 0.5 mM was most effective in reducing CI in cucumber fruit during cold storage. The application of MeSA and MeJA enhanced the resistance of green bell pepper against chilling injury (Fung et al., 2004). The smallest rate of decay was observed at a 4 mM SA concentration (Figure 3). SA is known for its effective application at the pre-harvest stage. It is capable of inducing defense resistance against postharvest diseases, which tend to produce promising results in controlling postharvest decay. Therefore, SA can be used as a suitable alternative to chemicals in postharvest management (Asghari and Babalar, 2010). Shafiee et al. (2010) reported that all treatments were able to postpone decay in fruits that grew in nutrient solutions, with or without SA. There was no difference in the severity of decay between fruits that received or did not receive SA, although the effect of heat treatment was an exception. Therefore, if using postharvest treatments is not possible or feasible, adding SA to the nutrient solution can postpone fruit decay. In a relevant case of research, it was reported that dipping fruits in water (25°C) containing calcium or SA led to a decrease in the severity of the decay compared to fruits treated with heat. Gholami et al. (2010) indicated that the application of SA at the preharvest stage, precisely 3 weeks before harvesting the fruits, enhanced quality attributes and lowered fungal contamination in “Mashhad” sweet cherry fruits. A similar observation was made on table grapes (Champa et al., 2014), sweet cherries (Yao and Tian, 2005), and pears (Cao et al., 2006), where preharvest SA treatments effectively reduced fruit decay during storage. SA maintained the postharvest quality and extended the shelf life of the orange fruits (Amiri et al., 2021) and peaches (Wang et al., 2006).
The findings of Nasrin et al. (2008) suggested that the tomato fruits shelf life was extended up to 17 days without significant quality degradation by treating the chlorine fruits, packaging them in perforated polyethylene bags, and storing them at room temperature for just 7 days in comparison to the control. Also, Tabaestani et al. (2013) reported that it can increase the storage life of tomatoes stored at 20°C by using basil gum as an edible coating. The antifungal group composed of thymol and salicylic acid was applied to control postharvest decay in tomatoes, and it had the best synergistic effect, according to a study conducted by Kong et al. (2016). The rate of SA decay was noticeably reduced in apple fruits, delaying the ripening process after 10 days during storage (Mo et al., 2008).
The highest ascorbic acid content was found in the treatment group (Figure 4). SA reduces the damage to fruits because of its action as an antioxidant. Accordingly, this is carried out by eliminating free radicals at the ripening stage (Elwan and El-Hamahmy, 2009; Etemadipoor et al., 2020). Similarly, Shafiee et al. (2010) mentioned that SA treatments increased the antioxidant capacity in plants and assisted with anti-stress mechanisms while reducing the rate of ascorbic acid destruction. According to previous research (Elwan and El-Hamahmy, 2009), higher amounts of ascorbic acid in pepper fruits led to lower levels of ROS, which ultimately resulted in fewer ROS and less damage after salt stress. SA treatments reportedly maintained ascorbic acid (AA) in pomegranate (Sayyari et al., 2009). Similar results were observed in cornelian cherry fruits (Dokhanieh et al., 2013) and litchi fruits (Kumar et al., 2013). The AA content in sweet pepper, as a result of the SA and CaCl2 treatments, acted against ascorbic acid oxidase (AAO) enzyme activity, leading to a smaller loss of AA content compared with the control (Rao et al., 2011). As an antioxidant, ascorbic acid plays an important role in protecting plants from oxidative damage since it scavenges free radicals and ROS. In fact, a higher ascorbic acid content can reduce ROS levels in pepper fruits, thereby resulting in less ROS-mediated damage (Elwan and El-Hamahmy, 2009).
The titratable acidity decreased in all the treated fruits, and TSS showed a reverse trend on the 40th day of storage. TA correlates with the organic acid content of fruits. During the ripening process, TA and total organic acid contents decreased (Kazemi et al., 2011). TA in the pomegranate was not affected by SA treatments (Sayyari et al., 2009). In kiwifruits, however, SA caused the maintenance of a higher TA than in the control (Kazemi et al., 2011). According to Supapvanich and Promyou (2013), sucrose is the most commonly produced carbohydrate during photosynthesis. Its source-to-sink transfer causes higher amounts of total soluble solids (TSS) in fruits. At the postharvest stage, they accumulate due to the metabolism of stored proteins, lipids, and carbohydrates, which ultimately culminates in senescence (Beckles, 2012). The preharvest application of SA on pepper fruits regulated their sugar content (Elwan and El-Hamahmy, 2009). In a study conducted by Beckles (2012), it was concluded that fruit maturation is the most important factor affecting TSS, TA, and the TSS/TA ratio. During postharvest, the climacteric fruit continues its respiration and ethylene production, which gives it a palatable taste, followed by fruit senescence. Accordingly, the fruits can be managed at 12.5°C in postharvest storage. On the other hand, the ratio of TSS to TA is important for the sweetness and sourness of tomatoes (Cheema et al., 2014). It is recognized that SA reduced the ripening process during storage, which decreased the rate of respiration and ethylene biosynthesis and led to a change in parameters such as firmness, TA, and TSS (Babalar et al., 2007; Valero et al., 2011; Champa et al., 2014). At harvest, table grapes showed higher values of TSS/TA when treated with 2.0 mM SA compared to the effects of the other two treatments (1.0 and 1.5 mM). Thus, an increase in TSS/TA was significant in the control for up to 45 days but then decreased rapidly (Cheema et al., 2014). As for the litchi fruits that were treated with salicylic acid, Kumar et al. (2013) observed a balanced TSS:TA ratio during storage.
The control had the lowest APX activity, and the highest content was observed in 4 mM SA (Figure 6). Aghdam et al. (2016) suggested that enhancements in some enzyme activities of the antioxidant system usually result in a decrease in plasma membrane damage because of less lipid peroxidation in cell membranes and, ultimately, the avoidance of reactive oxygen species accumulation. These factors are known to assist with CI tolerance in plants, which is reflected in more stable membrane integrity. This was emphasized by Cao et al. (2010) and Shafiee et al. (2010), where SA was reportedly effective in reducing CI during storage, thereby increasing ascorbate peroxidase activity (APX) and antioxidant capacity in the fruits. Therefore, enhancing APX activity could contribute to the more efficient removal of hydrogen peroxide (Cao et al., 2010). In previous research, Cao et al. (2010) concluded that the combined treatment might alleviate oxidative stress in peach fruits. Thus, the actions of APX, and other enzymes may be linked to one another as major factors that trigger plant tolerance to CI in peach fruits after heat treatment, combined with SA. In fact, APX enzyme activity was significantly reduced in immature pear fruits by SA spraying (Cao et al., 2006). Similar results were observed in peach (Wang et al., 2006), tomato (Ding et al., 2001, 2002; Fung et al., 2006; Zhang et al., 2011; Aghdam et al., 2012a,b), tomato seedlings (Senaratna et al., 2000), and pomegranate (Sayyari et al., 2009, 2011).
5. Conclusion
In sum, the results indicated that the SA treatment before harvest can assist in maintaining the quality of tomato fruits, delaying their ripening during storage, and extending their storage life. Meanwhile, SA is a simple phenolic compound that exhibits high potential for reducing post-harvest decay. It also helps in maintaining the amounts of firmness, TA, and ascorbic acid in fruits, reducing chilling injury and electrolyte leakage, while increasing the activation of ascorbate peroxidase (APX). SA can prevent the postharvest loss of tomato fruits at 10°C storage for 40 days, which makes this type of treatment feasible as a preharvest approach for making improvements in product quality.
Data availability statement
The original contributions presented in the study are included in the article/Supplementary material, further inquiries can be directed to the corresponding author.
Author contributions
AD and EB contributed to the conception and design of the study. EB performed the experiments, analyzed the data, and wrote the manuscript, which AD edited. All authors contributed to the article and approved the submitted version.
Conflict of interest
The authors declare that the research was conducted in the absence of any commercial or financial relationships that could be construed as a potential conflict of interest.
Publisher’s note
All claims expressed in this article are solely those of the authors and do not necessarily represent those of their affiliated organizations, or those of the publisher, the editors and the reviewers. Any product that may be evaluated in this article, or claim that may be made by its manufacturer, is not guaranteed or endorsed by the publisher.
References
Adhikary, T., Gill, P. S., Jawandha, S. K., Bhardwaj, R. D., and Anurag, R. K. (2020). Browning and quality management of pear fruit by salicylic acid treatment during low temperature storage. J. Sci. Food Agric. 101, 853–862. doi: 10.1002/jsfa.10692
Aghdam, M. S., Asghari, M., Babalar, M., and Sarcheshmeh, M. A. A. (2016). “Impact of salicylic acid on postharvest physiology of fruits and vegetables” in Eco-Friendly Technology for Postharvest Produce Quality, 243–268.
Aghdam, M. S., Asghari, M. R., Khorsandi, O., and Mohayeji, M. (2012a). Alleviation of post-harvest chilling injury of tomato fruit by salicylic acid treatment. J. Food Sci. Technol. 51, 2815–2820. doi: 10.1007/s13197-012-0757-1
Aghdam, M. S., Asghari, M. R., Moradbeygi, H., Mohamadkhani, N., Mmohayeji, M., and Rezapour-Fard, J. (2012b). Effect of postharvest salicylic acid treatment on reducing chilling injury in tomato fruit. Rom. Biotechnol. Lett. 17, 7466–7473.
Aghdam, M. S., Motallebiazar, A., Mostofi, Y., Moghaddam, J. F., and Ghasemnezhad, M., (2010). Effects of MeSA vapor treatment on the postharvest quality of ‘Hayward’ kiwifruit. 6th international postharvest symposium. Acta Hort 877 ISHS, 2010. 743–748. doi: 10.17660/ActaHortic.2010.877.98
Aghdam, M. S., Sevillano, L., Flores, F. B., and Bodbodak, S. (2013). Heat shock proteins as biochemical stress markers for postharvest chilling in fruits and vegetables. Sci. Hortic. 160, 54–64. doi: 10.1016/j.scienta.2013.05.020
Amiri, S., Nicknam, Z., Radi, M., Sayadi, M., Bagheri, F., Karimi Khorrami, N., et al. (2021). Postharvest quality of orange fruit as inluenced by salicylic acid, acetic acid, and carboxymethyl cellulose coating. J. Food Meas. Charact. 15, 3912–3930. doi: 10.1007/s11694-021-00966-y
Asghari, M. R., and Babalar, M., (2010). Use of salicylic acid to increase strawberry fruit Total antioxidant activity. Eds.: M. Erkan and U. Aksoy. 6th International Postharvest Symposium Acta Horticulture, 877, 1117–1122.
Babalar, M., Asghari, M., Talaei, A., and Khosroshahi, A. (2007). Effect of pre- and postharvest salicylic acid treatment on ethylene production, fungal decay and overall quality of Selva strawberry fruit. Food Chem. 105, 449–453. doi: 10.1016/j.foodchem.2007.03.021
Bai, C. H., Wu, C., Ma, L., Fu, A., Zheng, Y., Han, J., et al. (2021). Transcriptomics and metabolomics analyses provide insights into postharvest ripening and senescence of tomato fruit under low temperature. Hortic. Plant J 9, 109–121. doi: 10.1016/j.hpj.2021.09.001
Baninaiem, E., Mirzaaliandastjerdi, A. M., Rastegar, S., and Abbaszade, K. (2016). Effect of pre- and postharvest salicylic acid treatment on quality characteristics of tomato during cold storage. Adv. Hortic. Sci. 30, 183–192. doi: 10.13128/ahs-20281
Batu, A. (2004). Determination of acceptable firmness and colour values of tomatoes. J. Food Eng. 61, 471–475. doi: 10.1016/S0260-8774(03)00141-9
Beckles, D. M. (2012). Factors affecting the postharvest soluble solids and sugar content of tomato (Solanum lycopersicum L.) fruit. Postharvest Biol. Technol. 63, 129–140. doi: 10.1016/j.postharvbio.2011.05.016
Benati, J. A., Barreto, C. F., Navroski, R., Farias, R., Roberto, D. M., Martins, C. R., et al. (2021). Efect of salicylic acid in post-harvest quality of ‘BRS Kampai’peaches submitted to diferent cold storage periods. Aust. J. Crop. Sci. 15, 196–200. doi: 10.21475/ajcs.21.15.02.p2549
Bourne, M. C. (2006). Selection and use of postharvest technologies as a component of the food chain. J. Food Sci. 69, 43–46. doi: 10.1111/j.1365-2621.2004.tb15491.X
Cao, S. F., Hu, Z. C., and Wang, H. O. (2009). Effect of salicylic acid on the activities of anti-oxidant enzymes and phenylalanine ammonia-lyase in cucumber fruit in relation to chilling injury. Hortic. Sci. Biotech. 84, 125–130. doi: 10.1080/14620316.2009.11512492
Cao, S., Hu, Z., Zheng, Y., and Lu, B. (2010). Synergistic effect of heat treatment and salicylic acid on alleviating internal browning in cold-stored peach fruit. Postharvest Biol. Technol. 58, 93–97. doi: 10.1016/j.postharvbio.2010.05.010
Cao, J., Zeng, K., and Jiang, W. (2006). Enhancement of postharvest disease resistance in Ya Li pear (Pyrus bretschneideri) fruit by salicylic acid sprays on the trees during fruit growth. Eur. J. Plant Pathol. 114, 363–370. doi: 10.1007/s10658-005-5401-8
Champa, W. A. H., Gill, M. I. S., Mahajan, B. V. C., and Arora, N. K. (2014). Preharvest salicylic acid treatments to improve quality and postharvest life of table grapes (Vitis vinifera L.) cv. Flame seedless. J. Food Sci. Technol. 52, 3607–3616. doi: 10.1007/s13197-014-1422-7
Cheema, A., Padmanabhan, P., Subramanian, J., Blom, T. H., and Paliyath, G., Improving (2014). Quality of greenhouse tomato (Solanum lycopersicum L.) by pre- and postharvest applications of hexanal-containing formulations. Postharvest Biol. Technol. 95, 13–19. doi: 10.1016/j.postharvbio.2014.03.012
Cong, H., Jin-hua, Z., Qing, W., Hai-zhou, D., and Li-pu, G. (2017). Salicylic acid alleviates postharvest chilling injury of sponge gourd (Luffa cylindrica). J. Integr. Agric. 16, 735–741. doi: 10.1016/S2095-3119(16)61390-4
Ding, C.-K., Wang, C. Y., Gross, K. C., and Smith, D. L. (2001). Reduction of chilling injury and transcript accumulation of heat shock proteins in tomato fruit by methyl jasmonate and methyl salicylate. Plant Sci. 161, 1153–1159. doi: 10.1016/S01689452(01)00521-0
Ding, C. K., Wang, C. Y., Gross, K. C., and Smith, D. L. (2002). Jasmonate and salicylate induce the expression of pathogenesis-related-protein genes and increase resistance to chilling injury in tomato fruit. Planta 214, 895–901. doi: 10.1007/s00425-001-0698-9
Dokhanieh, A. Y., Aghdam, M. S., Rezapour Fard, J., and Hassanpour, H. (2013). Post- harvest salicylic acid treatment enhances antioxidant potential of cornelian cherry fruit. Sci. Hortic. 154, 31–36. doi: 10.1016/j.scienta.2013.01.025
Elhadi, M. Y., and Jeffrey, K. B. (2012). “Tomatoes” in Crop post- harvest: science and technology, perishables. eds. D. Rees, G. Farrell, and J. Orchard ( Blackwell Publishing Ltd.), 14.
Elwan, M. W. M., and El-Hamahmy, M. A. M. (2009). Improved productivity and quality associated with salicylic acid application in green house pepper. Sci. Hortic. 122, 521–526. doi: 10.1016/j.scienta.2009.07.001
Etemadipoor, R., Mirzaalian Dastjerdi, A., Ramezanian, A., and Ehteshami, S. (2020). Ameliorative efect of gum arabic, oleic acid and/or cinnamon essential oil on chilling injury and quality loss of guava fruit. Sci. Hortic. 266:109255. doi: 10.1016/j.scienta.2020.109255
Fung, R. W. M., Wang, C. Y., Smith, D. L., Gross, K. C., Tao, Y., and Tian, M. (2006). Charac- terization of alternative oxidase (AOX) gene expression in response to methyl salicylate and methyl jasmonate pre-treatment and low temperature in tomatoes. Plant Physiol. 163, 1049–1060. doi: 10.1016/j.jplph.2005.11.003
Fung, R. W. M., Wang, C. Y., Smith, D. L., Gross, K. C., and Tian, M. (2004). MeSA and MeJA increase steadyestate transcript levels of alternative oxidase and resistance against chilling injury in sweet peppers. Plant Sci. 166, 711–719. doi: 10.1016/j.plantsci.2003.11.009
Gholami, M., Sedighi, A., Ershadi, A., and Sarikhani, H. (2010). Effect of pre- and postharvest treatment of salicylic acid and geibberellic acid on ripening and some physicochemical properties of ‘Mashhad’ sweet cherry (Prunnus avium L.) fruit. Acta Hortic. 884, 257–264. doi: 10.17660/ActaHortic.2010.884.30
Hatami, M., Kalantari, S., and Delshsd, M. (2013). Responses of different maturity stages of tomato fruit to different storage conditions. Acta Hortic. 101, 857–864. doi: 10.17660/ActaHortic.2013.1012.116
Hayat, Q., Shamsul Hayat, S., Irfan, M., and Ahmad, A. (2010). Effect of exogenous salicylic acid under changing environment: a review. Environ. Exp. Bot. 68, 14–25. doi: 10.1016/j.envexpbot.2009.08.005
Huang, R., Xia, R., Lu, Y., Hu, L., and Xu, Y. (2008). Effect of pre-harvest salicylic acid spray treatment on post-harvest antioxidant in the pulp and peel of ‘Cara cara’ navel orange (Citrus sinenisis L. Osbeck). Sci. Food Agric. 88, 229–236. doi: 10.1002/jsfa.3076
Kazemi, M., Aran, M., and Zamani, S. (2011). Effect of calcium chloride and salicylic acid treatment on quality characteristics of kiwifruit (Actinidia deliciosa cv. Hayward) during storage. Am. J. Plant Physiol. 6, 183–189. doi: 10.3923/ajpp.2011.183.189
Konagaya, K., Al Riza, D. F., Nie, S., Yoneda, M., Hirata, T., Takahashi, N., et al. (2020). Monitoring mature tomato (red stage) quality during storage using ultraviolet-induced visible fluorescence image. Postharvest Biol. Technol. 160:111031. doi: 10.1016/j.postharvbio.2019.111031
Kong, J., Xie, Y. F., Guo, Y. H., Cheng, Y., Qian, H., and Yao, W. R. (2016). Biocontrol of postharvest fungal decay of tomatoes with a combination of thymol and salicylic acid screening from 11 natural agents. LWT Food Sci. Technol. 72, 215–222. doi: 10.1016/j.lwt.2016.04.020
Kumar, D., Mishra, D., Chakraborty, B., and Kumar, P. (2013). Pericarp browning and quality management of litchi fruit by antioxidants and salicylic acid during ambient storage. Food Sci. Technol. 50, 797–802. doi: 10.1007/s13197-011-0384-2
Kumar, N., Tokas, J., Raghavendra, M., and Singal, H. R. (2020). Impact of exogenous salicylic acid treatment on the cell wall metabolism and ripening process in postharvest tomato fruit stored at ambient temperature. Inter. J. Food Sci. Technol. doi: 10.1111/ijfs.14936
Manaa, A., Gharbi, E., Mimouni, H., Wasti, S., Aschi-Smiti, S., Lutts, S., et al. (2014). Simultaneous application of salicylic acid and calcium improves salt tolerance in two contrasting tomato (Solanum lycopersicum) cultivars. S. Afr. J. Bot. 95, 32–39. doi: 10.1016/j.sajb.2014.07.015
Mirdehghan, S. H., Rahemi, M., Martinez-romero, D., Guillen, F., Valverde, J. M., Zapata, P. J., et al. (2007). Reduction of pomegranate chilling injury during storage after heat treatment: role of polyamines. Postharvest Biol. Technol. 44, 19–25. doi: 10.1016/j.postharvbio.2006.11.001
Mo, Y., Gong, D., Liang, G., Han, R., Xie, J., and Li, W. (2008). Enhance preservation effects of sugar apple fruits by salicylic acid treatment during postharvest storage. J. Sci. Food Agric. 88, 2693–2699. doi: 10.1002/jsfa.3395
Nakano, Y., and Asada, K. (1981). Hydrogen peroxide is scavenged by ascorbate-specific peroxidases in spinach chloroplasts. Plant Cell Physiol. 22, 867–880. doi: 10.1093/oxfordjournals.pcp.a076232
Nasrin, T. A. A., Alam, M. S., Molla, M. M., Hossaen, M. A., and Yasmin, L. (2008). Effect of postharvest tretments on shelf life and quality of tomato. Aust. J. Agric. Res. 33, 579–585.
Rao, T. V. R., Gol, N. B., and Shah, K. K. (2011). Effect of postharvest treatments and storage temperatures on the quality and shelf life of sweet pepper (Capsicum annum L.). Sci. Hortic. 132, 18–26. doi: 10.1016/j.scienta.2011.09.032
Roohanitaziani, R., Lammers, M., Molthoff, J., Tikunov, Y., Meijer-Dekens, F., Visser, R. G. F., et al. (2022). Phenotyping of a diverse tomato collection for postharvest shelf-life. Postharvest Biol. Technol. 188:111908. doi: 10.1016/j.postharvbio.2022.111908
Rugkong, A., (2009). Effects chilling on tomato fruit ripening. A dissertation presented to the Faculty of the Graduate School of Cornell University. 62, Available at: https://hdl.handle.net/1813/12807.
Saltveit, M. E. (2001). Chilling injury is reduced in cucumber and rice seedlings and in tomato pericarp discs by heat-shocks applied after chilling. Postharvest Biol. Technol. 21, 169–177. doi: 10.1016/S0925-5214(00)00132-0
Saltveit, M. E. (2005). “Postharvest Biology and Technology” in Tomatoes. ed. E. Heuvelink (Wallingford: CABI), 305, 352–324.
Salyari, R., Seii, E., Varasteh, F., and Alizadeh, M. (2021). Efects of pre-harvest salicylic acid treatment on the postharvest quality of peach cultivar Robin. Chem. Health Risks 11, 1–8. doi: 10.22034/JCHR.2021.1875114.1041
Sayyari, M., Aghdam, M. S., Salehi, F., and Ghanbari, F. (2016). Salicyloyl chitosan alleviates chilling capacity injury and maintains antioxidant of pomegranate fruits during cold storage. Sci. Hortic. 211, 110–117. doi: 10.1016/j.scienta.2016.08.015
Sayyari, M., Babalar, M., Kalantari, S., Serrano, M., and Valero, D. (2009). Effect of salicylic acid treatment on reducing chilling injury in stored pomegranates. Postharvest Biol. Technol. 53, 152–154. doi: 10.1016/j.postharvbio.2009.03.005
Sayyari, M., Castillo, S., Valero, D., Díaz-Mula, H. M., and Serrano, M. (2011). Acetyl salicylic acid alleviates chilling injury and maintains nutritive and bioactive compounds and antioxidant activity during postharvest storage of pomegranates. Postharvest Biol. Technol. 60, 136–142. doi: 10.1016/j.postharvbio.2010.12.012
Senaratna, T., Touchell, D., Bunn, E., and Dixon, K. (2000). Acetyl salicylic acid (aspirin) and salicylic acid induce multiple stress tolerance in bean and tomato plants. Plant Growth Regul. 30, 157–161. doi: 10.1023/A:1006386800974
Shafiee, M., Taghavi, T. S., and Babalar, M. (2010). Addition of salicylic acid to nutrient solution combined with postharvest treatments (hot water, salicylic acid, and calcium dipping) improved postharvest fruit quality of strawberry. Sci. Hortic. HORTI-3462:6. doi: 10.1016/j.scienta.2009.12.004
Shi, Z., Wang, F., Lu, Y., and Deng, J. (2018). Combination of chitosan and salicylic acid to control postharvest green mold caused by Penicillium digitatum in grapefruit fruit. Sci. Hortic. 233, 54–60. doi: 10.1016/j.scienta.2018.01.039
Supapvanich, S., and Promyou, S. (2013). “Efficiency of salicylic acid application on postharvest perishable crops” in Salicylic acid. eds. S. Hayat, A. Ahmad, and M. Alyemeni (Dordrecht: Springer Science + Business Media), 339–355.
Tabaestani, H. S. H., Sedaghat, N., Pooya, E. S., and Alipour, A. (2013). Shelf-life improvement and postharvest quality of cherry tomato (Solanum lycopersicum L.) fruit using basil mucilage edible coating and cumin essential oil. Int. J. Agron. Plant Prod. 4, 2346–2353. Available at: http://www.ijappjournal.com
Valero, D., Díaz-Mula, H. M., Zapata, P. J., Castillo, S., Guillén, F., Martínez-Romero, D., et al. (2011). Postharvest treatments with salicylic acid, acetylsalicylic acid or oxalic acid delayed ripening and enhanced bioactive compounds and antioxidant capacity in sweet cherry. J. Agric. Food Chem. 59, 5483–5489. doi: 10.1021/jf200873j
Wang, L., Chen, S., Kong, W., Li, S., and Archbold, D. D. (2006). Salicylic acid pretreatment alleviates chilling injury and affects the antioxidant system and heat shock proteins of peaches during cold storage. Postharvest Biol. Technol. 41, 244–251. doi: 10.1016/j.postharvbio.2006.04.010
Yao, H. J., and Tian, S. P. (2005). Effects of pre-and postharvest application of SA or MeJA on inducing disease resistance of sweet cherry fruit in storage. Postharvest Biol. Technol. 35, 253–262. doi: 10.1016/j.postharvbio.2004.09.00
Zavala, J. F. A., Wang, S. Y., Wang, C. Y., and Aguilar, G. A. G. (2004). Effects of storage temperatures on antioxidant capacity and aroma compounds in strawberry fruit. LWT- Food Sci. Technol. 37, 687–695. doi: 10.1016/j.lwt.2004.03.002
Zhang, Y., Chen, K., Zhang, S., and Ferguson, I. (2003). The role of salicylic acid in postharvest ripening of kiwifruit. Postharvest Biol. Technol. 28, 67–74. doi: 10.1016/S0925-5214(02)00172-2
Zhang, Y., Li, S., Deng, M., Gui, R., Liu, Y., Chen, X., et al. (2022). Blue light combined with salicylic acid treatment maintained the postharvest quality of strawberry fruit during refrigerated storage. Food Chem. 15:100384. doi: 10.1016/j.fochx.2022.100384
Zhang, X., Shen, L., Li, F., Meng, D., and Sheng, J. (2011). Methyl salicylate-induced arginine catabolism is associated with up-regulation of polyamine and nitric oxide levels and improves chilling tolerance in cherry tomato fruit. J. Agric. Food Chem. 59, 9351–9357. doi: 10.1021/jf201812r
Keywords: salicylic acid, tomato, ascorbate peroxidase, postharvest, chilling injury, storage, shelf life
Citation: Baninaiem E and Dastjerdi AM (2023) Enhancement of storage life and maintenance of quality in tomato fruits by preharvest salicylic acid treatment. Front. Sustain. Food Syst. 7:1180243. doi: 10.3389/fsufs.2023.1180243
Edited by:
Laurent Dufossé, Universitéde la Réunion, FranceReviewed by:
Mahmood Ghasemnezhad, University of Guilan, IranZakir Hussain, Indian Agricultural Research Institute (ICAR), India
Chen Huan, Zhejiang Gongshang University, China
Copyright © 2023 Baninaiem and Dastjerdi. This is an open-access article distributed under the terms of the Creative Commons Attribution License (CC BY). The use, distribution or reproduction in other forums is permitted, provided the original author(s) and the copyright owner(s) are credited and that the original publication in this journal is cited, in accordance with accepted academic practice. No use, distribution or reproduction is permitted which does not comply with these terms.
*Correspondence: Abdolmajid Mirzaalian Dastjerdi, mirzaalian@hormozgan.ac.ir