- 1Affiliated Hangzhou Xixi Hospital, Zhejiang University School of Medicine, Hangzhou, China
- 2Hainan Institute of Zhejiang University, Sanya, China
- 3Institute of Preventive Veterinary Sciences and Department of Veterinary Medicine, Zhejiang University College of Animal Sciences, Hangzhou, China
- 4Laboratory of Engineering and Applied Technologies, Higher School of Technology, Sultan Moulay Slimane University, M’ghila University Campus, Beni Mellal, Morocco
- 5Department of Agricultural, Forest and Food Sciences, University of Turin, Torino, Italy
- 6Institute for Veterinary Food Science, Faculty of Veterinary Medicine, Justus-Liebig University Giessen, Giessen, Germany
- 7Zhejiang Provincial Key Laboratory of Preventive Veterinary Medicine, Hangzhou, China
- 8State Key Laboratory for Diagnosis and Treatment of Infectious Diseases, National Clinical Research Center for Infectious Diseases, National Medical Center for Infectious Diseases, The First Affiliated Hospital, College of Medicine, Zhejiang University, Hangzhou, China
Listeria species are exposed to various stressors throughout the food chain, which are crucial for microbe mitigation strategy in the food industry. However, the survival capabilities and development of antimicrobial resistance by Listeria spp. under different food processing environments (FPEs) stressors are not yet well understood. Hence, this study aims to determine the difference in survivability and antimicrobial susceptibility of L. monocytogenes (Lm) and other Listeria species (non-Lm) strains exposed to different FPEs stressors, including heat, acidic and alkaline pH, UV irradiation, and osmotic stress. For this, a collection of 11 Lm and 10 non-Lm strains were used to conduct experiments. This study showed that Lm strains were relatively more tolerant to environmental stresses than non-Lm strains (p > 0.05). Additionally, the evaluation of stress-induced resistance toward antimicrobials showed that anaerobic incubation, after exposition to environmental stresses, rendered Lm and non-Lm more resistant to antimicrobial agents than aerobic incubation. Furthermore, the study observed that different stressors induced an increase in minimum inhibitory concentrations (MICs) of certain antimicrobials. Specifically, heat stress persuaded an increase in MICs of tetracycline under aerobic incubation, and gentamicin and ciprofloxacin under anaerobic incubation. Acidic/alkaline pH induced an increase in MICs of gentamicin, ciprofloxacin, and trimethoprim-sulfamethoxazole, especially under anaerobic incubation. However, UV stress induced increase in MICs of tetracycline and trimethoprim-sulfamethoxazole under aerobic incubation and gentamicin, ciprofloxacin, and trimethoprim-sulfamethoxazole under anaerobic incubation. Additionally, osmotic stress induced an increase in MICs of tetracycline and ampicillin under aerobic incubation and gentamicin, tetracycline, and trimethoprim-sulfamethoxazole under anaerobic incubation. Collectively, this study highlights that stress tolerance may contribute to the predominance of Listeria species among FPEs and induce the development of antimicrobial resistance even without antibiotic selection pressure. The findings of this study may guide updated strategies to mitigate Listeria species in the food industry.
1. Introduction
Stress in foods and food processing environments (FPEs) leads to the adaptation of foodborne pathogens in unfavorable conditions, affecting their potential to survive and resist different treatments (Nic Aogáin and O’Byrne, 2016; Li et al., 2022). During food processing and preservation, foodborne pathogens are constantly exposed to a diverse range of stresses which could influence their viability and activity. These pathogens suffer stresses from the food-preserving process, food matrices, and animal or human consumption (Ruiz et al., 2017; Wang et al., 2023). Notably, the variation in foodborne pathogens’ response to such stresses is due to their differences in innate and acquired properties, including origin, serotype, membrane structure, efflux pump, mutations conferring altered target sites, and differences in the expression of protective mechanisms (Buncic et al., 2001; Francis and O’Beirne, 2005). Indeed, the long contact period between pathogens and environmental stressors may induce the development of antimicrobial resistance and virulence.
Listeria monocytogenes remains a major challenge for efficient control in the food industry. The persistence and survival of L. monocytogenes within food processing facilities are crucial risk factors for their presence in food products. In fact, L. monocytogenes may survive under adverse environmental conditions in FPEs and ready-to-eat (RTE) food, leading to human infections (Gandhi and Chikindas, 2007; Miladi et al., 2017; Shi et al., 2021). Hence, the persistence of L. monocytogenes in FPEs represents serious concerns about food safety, which has contributed to costly food recalls, the medical burden due to food-borne outbreaks, and high mortality and morbidity rates (Buchanan et al., 2017; Bouymajane et al., 2021; Shi et al., 2021; Anwar et al., 2022). Recently, there has been an increasing concern about food contamination due to the persistence of L. monocytogenes along with FPEs and RTE food (Lee et al., 2019), which may lead to human clinical infections (Liao et al., 2020).
L. monocytogenes infections cause gastroenteritis, meningitis, and neuroencephalitis in immunocompromised humans (Pilmis et al., 2020), while other species like L. ivanovii have been reported to be pathogenic for animals, by causing abortion, especially in sheep and cattle (Dunnett et al., 2020). In rare cases, L. ivanovii and L. seeligeri may be associated with human infections (Schmid et al., 2005). Importantly, it is reported that the contamination of food products with Listeria species is most likely due to their persistence in the processing environment (Leong et al., 2014). In fact, strains may resist cleaning and disinfection operations, persist in processing facilities, and then contaminate food products (Martínez-Suárez et al., 2016). Hence, despite introducing safe raw materials, contamination could be originated from contaminated facilities with persisting strains in FPEs (Muhterem-Uyar et al., 2015).
The development of antimicrobial resistance is a major threat to food safety and public health services. Moreover, persistence in different environmental stresses may induce resistance to different antibiotics by co-resistance mechanisms (Imran et al., 2019). However, the direct effect of environmental stressors on the development of antimicrobial-resistance is still obscure. In fact, we hypothesized that the exposition of foodborne pathogens to different stressors, including disinfectants, physical, and chemical treatments may induce the development of resistance toward different antibiotics and enhance the persistence of foodborne pathogens in unfavorable conditions. To bridge this knowledge gap, we conducted this study to better understand the bahaviour of both L. monocytogenes (Lm) strains and other Listeria spp. (non-Lm) strains toward environmental stress conditions, in addition, we investigated the effect of environmental stressors, including heat, acidic and alkaline pH, UV irradiation, and osmotic stress, on the variation of antimicrobial susceptibility of “Lm” and “non-Lm” strains incubated in both aerobic and anaerobic conditions. Finally, we believe that the outputs of this study could guide the risk estimation of emerging clinically relevant Listeria species, therefore, improving our understanding of the environmental stressors that may induce the development of antimicrobial resistance during the food processing chain.
2. Materials and methods
2.1. Bacterial strains
A total of twenty-one Listeria spp. isolates originating from diverse sources were selected and analyzed in this study, including those from food (n = 13), environment (n = 1), livestock (n = 5), and human isolates (n = 2), which were obtained over a period from 2002 to 2019. The studied isolates were obtained from the Laboratory of Professor Min Yue (Department of Veterinary Medicine, Zhejiang University, Hangzhou, China) and were grouped as L. monocytogenes (Lm) group (n = 11), including six different serotypes (1/2a, 1/2b, 1/2c, 4a, 4b, 4d), and non-L. monocytogenes (non-Lm) group (n = 10), including five common species, L. grayi, L. innocua, L. ivanovii, L. seeligeri, L. welshimeri. Detailed information about Lm and non-Lm strains is given in Table 1.
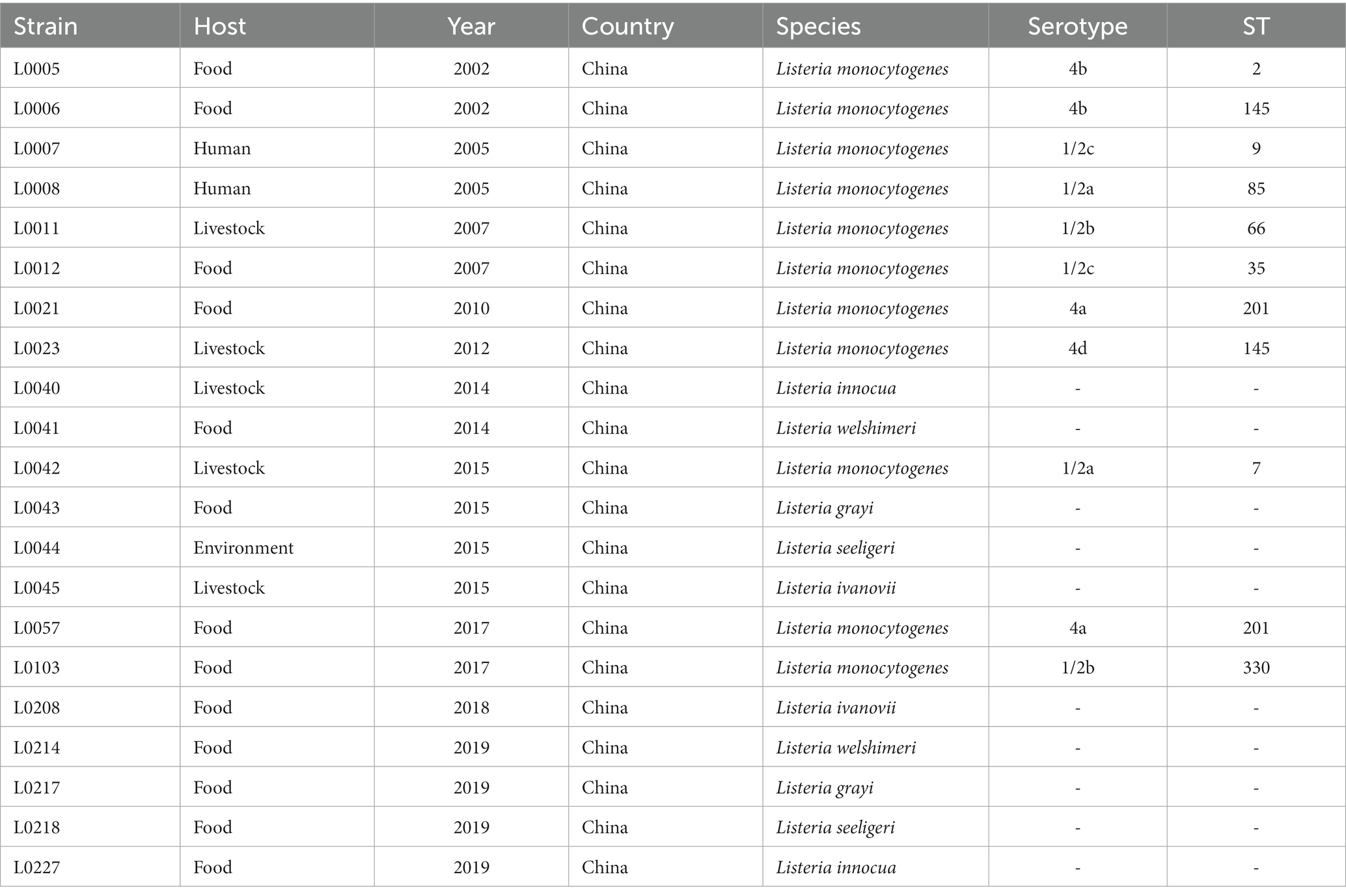
Table 1. The examined strains of Listeria species (Lm and non-Lm) with their host, year, country, serotypes, and STs.
2.2. Bacterial culture
For each Listeria spp. strain, a frozen (−80°C) stock culture (500 μl culture suspension supplemented with 50% glycerol) was thawed, and a loopful was streaked onto brain heart infusion (BHI) agar and incubated at 37°C overnight. Afterward, a single colony was transferred into BHI broth and incubated at 37°C overnight.
2.3. Stress exposure under different conditions
Bacterial survivability after stress exposure was evaluated by log10 (N0/N) where N0 = colony-forming units (CFU) of bacteria before exposure to stress; N = CFU of bacteria after exposure to stress.
2.3.1. Heat stress
Bacterial survivability under heat stress was evaluated as reported previously, with minor modification (Wałecka-Zacharska et al., 2018). A total of 10 μl of bacterial culture (OD600 = 0.15) were added to 990 μl of sterile Phosphate-Buffered Saline (PBS) (pH 7.4, pre-heated to 65°C) and treated at 65°C in a water bath for 2, 3, and 4 min, respectively. After treatment, the bacterial suspensions were immediately placed on ice. Then, 100 μl of bacterial suspension and its serial 10-fold dilutions were plated onto the BHI agar, and then incubated overnight at 37°C before the enumeration of colonies. The experiments were conducted in triplicates.
2.3.2. Acid and alkaline stress
Bacterial survivability under the acid and alkaline stresses was evaluated using a previously established protocol (Koutsoumanis et al., 2003). Hydrochloric acid (HCl) and sodium hydroxide (NaOH) were used to prepare physiological saline solutions with various ranges of pH = 2.0 and pH = 12.0. A total of 10 μl of the resuspended bacterial solution (OD600 = 0.15) were mixed with 990 μl used to prepare PBS of pH = 2.0 and pH = 12.0. The mixtures were incubated at room temperature for 10, 20, and 30 min, respectively. Then, we conducted a serial 10-fold dilution using PBS (pH 7.4). After that, 100 μl of the original solution and each dilution were spread onto the BHI agar plates. Plates were incubated with three replicates for each sample at 37°C for 24 h, the number of CFUs was counted (Koutsoumanis et al., 2003).
2.3.3. UV stress
Bacterial response to UV stress was conducted according to the previous method (McKinney et al., 2009). For each strain, a standardized suspension (OD600 = 0.15) was prepared and then serially diluted in PBS (pH 7.4) to achieve 10-fold dilutions series of different cell concentrations. Then, 10 μl of the original suspension, along with each dilution were spread-plated onto BHI agar in triplicate and allowed to dry. Afterward, the plates were vertically irradiated with the UV lamp (8 W, 254 nm) for 60, 80s, and 100 s and incubated at 37°C for 24 h. Then the number CFUs was enumerated.
2.3.4. Osmotic stress
Bacterial survivability under osmotic stress was investigated by treating Listeria strains with 10% sodium chloride (NaCl) (Bergholz et al., 2010). Briefly, 10 μl of bacterial suspension (OD600 = 0.15) were mixed with 990 μl of 10% NaCl in buffered peptone water (BPW) and incubated at 37°C under static conditions. To determine the survivability of strains exposed to osmotic stress, samples aliquots were removed from these cultures on days 1, 3, 5, 7, 9, 11, 13, 15, 27, and 55, serially diluted and then plated on BHI agar. Colonies were enumerated following overnight incubation at 37°C.
2.4. Antimicrobial susceptibility testing
The antimicrobial susceptibility of isolates was assayed with seven antibiotics using the broth micro-dilution to determine the minimum inhibitory concentrations (MICs) as recommended by guidelines of the Clinical and Laboratory Standards Institute (CLSI) (CLSI, 2021). Breakpoints for ampicillin, trimethoprim-sulfamethoxazole, and imipenem were found in CLSI documents M45-A3 (CLSI, 2021). Since there are no relevant criteria for tetracycline, gentamicin, ciprofloxacin, and chloramphenicol, the susceptibility results for these antimicrobials were interpreted based on the breakpoints of Staphylococcus spp. as reported previously (Zhang et al., 2019). The multiple classes of antimicrobials along with MIC range (μg/mL) used in this study are as follow: β-lactams (ampicillin: AMP, 0.032–16); penems (imipenem: IMP, 0.032–16); sulphonamides (trimethoprim-sulfamethoxazole: SXT, 0.032–16); aminoglycosides (gentamicin: GEN,0.032–16); tetracyclines (tetracycline: TET,0.032–16); quinolones (ciprofloxacin: CIP, 0.032–16); phenicols (chloramphenicol: CHL, 0.125–64). Escherichia coli ATCC 25922 was used as a quality control strain.
2.5. Effect of stress conditions on antimicrobial susceptibility of Listeria spp.
Antimicrobial susceptibility testing was performed based on the determination of MICs before (pre-stress) and after (post-stress) the application of stress conditions on both “Lm” and “non-Lm” groups. The antimicrobial susceptibility was evaluated under both aerobic and anaerobic incubations. For aerobic conditions, MICs were determined by using 96-well plates incubated overnight at 37°C, while to achieve the anaerobic conditions, the 96-well plates were placed in anaerobic jars containing AnaeroPack-Anaero (disposable oxygen absorbing and carbon-dioxide generating agent) (Mitsubishi Gas Chemical CO., INC, Japan) and incubated overnight at 37°C (Hinnu et al., 2022). Moreover, to understand the effect of different stress conditions on the antimicrobial susceptibility of both “Lm” and “non-Lm” groups, the bacterial culture obtained after stress conditions application was adjusted to the OD600 = 0.12–0.15 (approximately 5 × 108 CFU/mL) and then used to determine the MIC of each antibiotic. The following post-stress treatments (heat stress at 65°C for 3 min; pH = 2 for 5 min and pH = 12 for 30 min; UV at 60s and 10% of NaCl for 27 days.) were used to evaluate the effect of stress conditions on the antimicrobial susceptibility behavior of Listeria groups.
2.6. Statistical analysis
The data interpretation and figures generation were performed by GraphPad Prism 7 software (GraphPad Software, Inc., United States). The significance level was set at a value of p <0.05, and ANOVA test was used to analyze the statistical difference between groups using GraphPad Prism 7 software.
3. Results
3.1. Bacterial survivability under various stresses
In order to provide in-depth knowledge about the persistence of Listeria spp. in FPEs, we evaluated the survival ability of both L. monocytogenes (Lm) and non-L. monocytogenes (non-Lm) groups under different stress conditions, including heat stress, acid/alkaline stress, UV stress, and osmotic stress. Our results showed that Lm isolates group presented greater tolerance to the tested environmental stresses that the non-Lm isolates group, but without significant difference (p > 0.05) (Figures 1A–E).
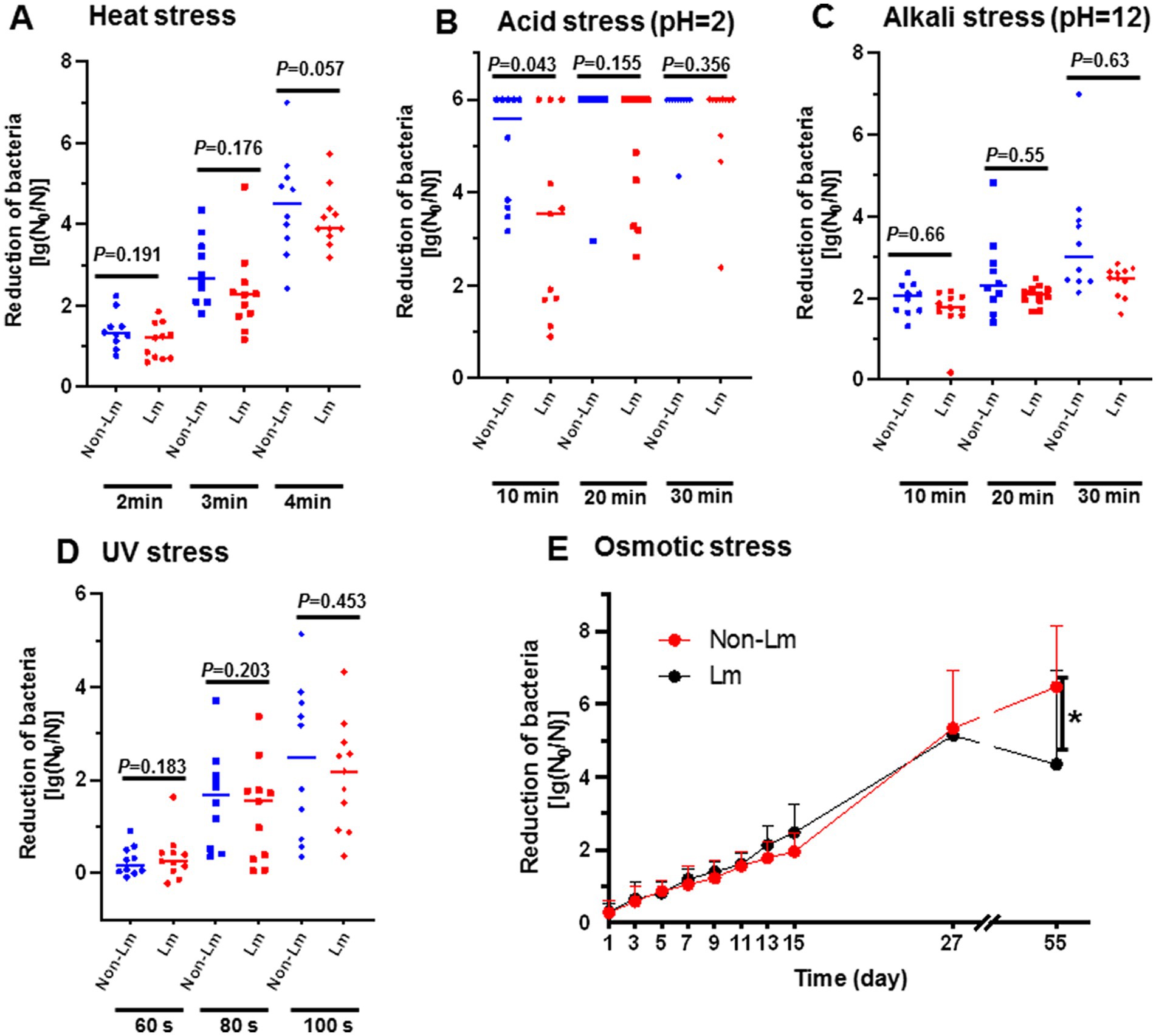
Figure 1. Effect of various stress conditions on survival of L. monocytogenes (Lm) isolates and non-L. monocytogenes (non-Lm) isolates. Reduction of bacteria was calculated as log(N0/N), N0 = colony forming unit of organisms before exposure to stress, N = colony forming unit of organisms after exposure to stress. (A) Bacterial survivability under heat stress. The logarithmic reduction value showed bacterial numbers of (non-Lm) and (Lm) strains in response to heat stress at 65°C for 2 min, 3 min, and 4 min. (B) Bacterial survivability under acid stress. The logarithmic reduction value showed bacterial numbers of (non-Lm) and (Lm) strains were treated with acid stress (pH 2.0) for 10 min, 20 min, and 30 min. (C) Bacterial survivability under alkaline stress. The logarithmic reduction value showed bacterial numbers of (non-Lm) and (Lm) strains treated with alkaline stress (pH 12.0) for 10 min, 20 min, and 30 min. (D) Bacterial survivability under UV stress. The logarithmic reduction value showed bacterial numbers of (non-Lm) and (Lm) strains under UV stress for the 60s, 80s, and 100 s. (E) Bacterial survivability under osmotic stress. The logarithmic reduction value showed bacterial numbers of (non-Lm) and (Lm) strains were under 10% NaCl stress for 55 days.
3.2. Antimicrobial susceptibility of pre-stressed isolates of Listeria spp.
The antimicrobial susceptibility of both Lm and non-Lm groups of Listeria spp. was evaluated by the determination of MIC of each antimicrobial under both aerobic and anaerobic incubation conditions. Our results showed that two isolates from the non-Lm group and one isolate from the Lm group presented resistance to CIP and SXT under aerobic incubation (Figure 2A). While anaerobic incubation showed that three non-Lm isolates were resistant to CIP and CHL, and three isolates from the Lm group displayed multi-resistance toward CIP, CHL, and SXT (Figure 2B); in addition, two non-Lm isolates showed resistance to tetracycline, and another from the Lm group presented resistance to ampicillin. Notably, anaerobic incubation revealed a high resistance level to CIP, CHL, and SXT among both the Lm and non-Lm groups. However, all the tested strains were susceptible to GEN and IPM under both aerobic and anaerobic incubation, where no susceptibility difference has been reported toward these antimicrobials.
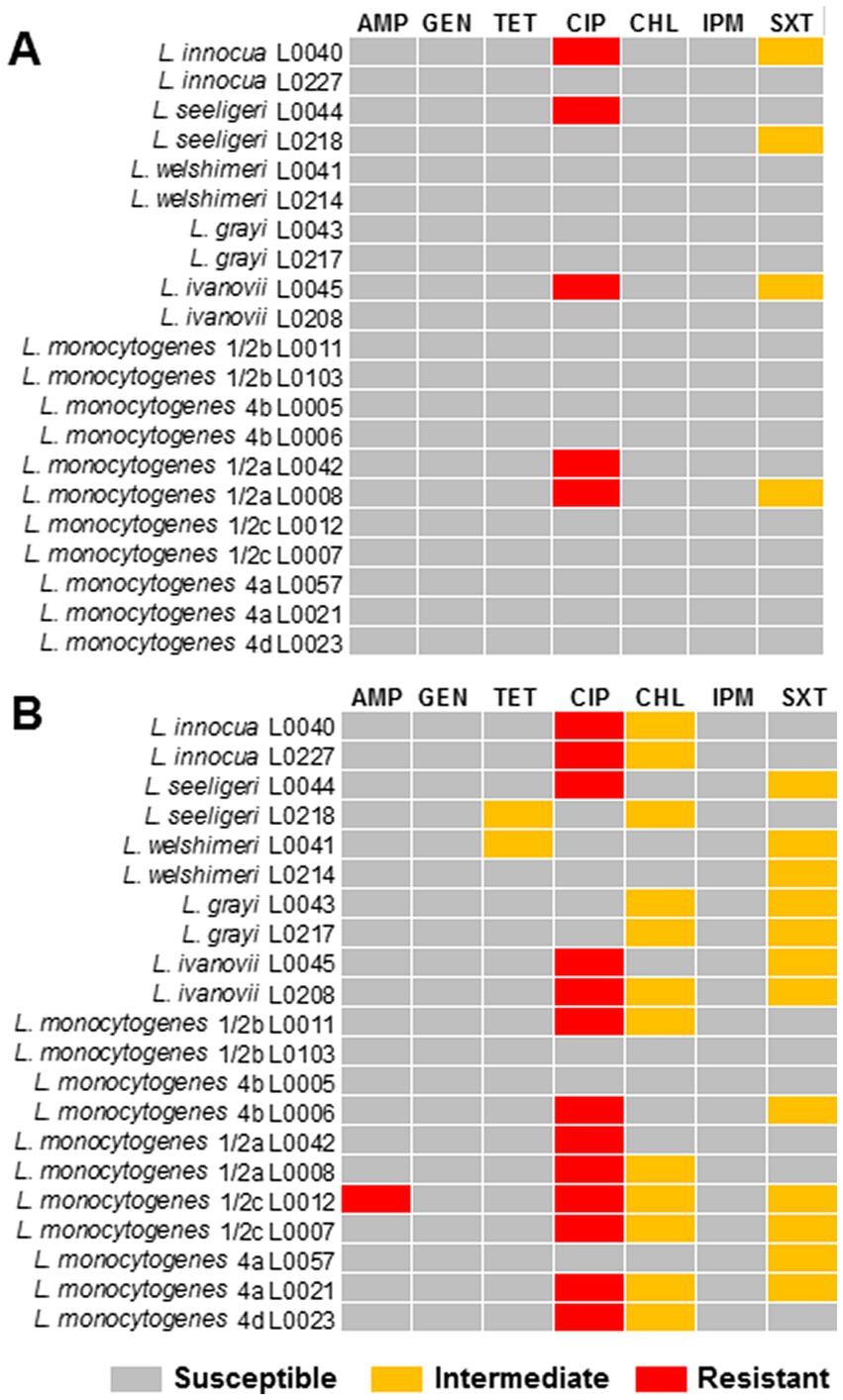
Figure 2. Antimicrobial susceptibility testing under the aerobic and anaerobic conditions. AMP: ampicillin, GEN: gentamicin, TET: tetracycline, CIP: ciprofloxacin, CHL: chloramphenicol, IPM: imipenem, SXT: trimethoprim-sulfamethoxazole. (A) The isolates were resistant (red), intermediate resistant (orange) or susceptible (gray) to the tested antibiotics under aerobic conditions. (B) The isolates were resistant (red), intermediate resistant (orange), or susceptible (grey) to the tested antibiotics under anaerobic conditions.
3.3. Effect of stress conditions “post-stress” on antimicrobial susceptibility of Listeria spp.
To understand whether stress conditions affect the antimicrobial susceptibility profiles of Listeria species, antimicrobial susceptibility testing was performed by conducting MIC assay after applying heat, acid and alkaline, UV, and osmotic stresses on the Lm and non-Lm isolates under aerobic and anaerobic incubations.
3.3.1. Osmotic stress
To evaluate the effect of heat stress on the antimicrobial susceptibility of Listeria spp., we determined the MICs of the tested antimicrobials toward 21 strains of Listeria species survived after 3 min of heat treatment at 65°C. Our results showed that under aerobic incubation, heat stress at 65°C for 3 min led to an apparent increase in MICs of TET and SXT for non-Lm isolates compared to Lm isolates (Figure 3A). However, an increase in MIC was evidenced for GEN and SXT under anaerobic conditions for both Lm and non-Lm isolates (Figure 3B). Compared to the results of aerobic conditions, anaerobic incubation under heat stress increased the MICs of GEN, CIP, IPM, and SXT for both Lm and non-Lm isolates (Figure 3B). Additionally, the Lm isolates appeared to be more resistant to CIP and IPM than non-Lm isolates after heat treatment under anaerobic conditions (Figure 3B). Moreover, heat-stress induced resistance for the non-Lm isolates to TET under aerobic incubation and GEN under anaerobic incubation (Figures 3A,B). Detailed information about the antimicrobial susceptibility of the Lm and the non-Lm isolates after applying heat stress is shown in Supplementary Data, Supplementary Table S1.
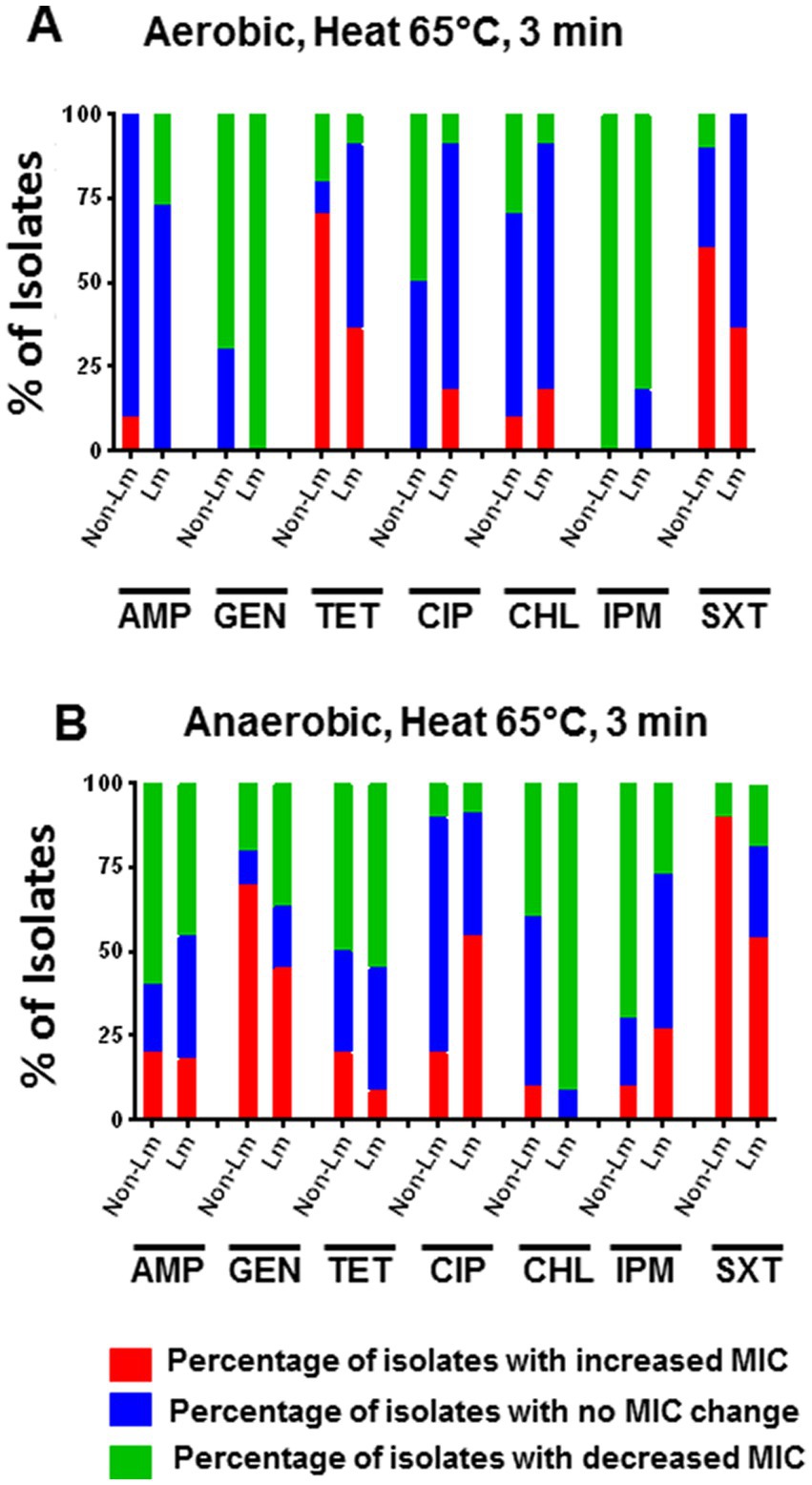
Figure 3. Changes in antimicrobial susceptibility of Lm and non-Lm isolates incubated in aerobic and anaerobic conditions after heat stress of 65°C for 3 min. AMP: ampicillin, GEN: gentamicin, TET: tetracycline, CIP: ciprofloxacin, CHL: chloramphenicol, IPM: imipenem, SXT: trimethoprim-sulfamethoxazole. (A) Change of MIC (increase/decrease) after heat stress compared with pre-stress (before stress) under aerobic condition, (B) Change of MIC (increase/decrease) after heat stress compared with pre-stress (before stress) under anaerobic conditions.
3.3.2. Effect of acid and alkaline stress on antimicrobial susceptibility
Experiments were conducted to determine whether acid and alkaline stress influence the susceptibility of the studied Listeria isolates to antimicrobials under aerobic and anaerobic incubations. The obtained results were summarized in Supplementary Data, Supplementary Tables S2, S3.
Results showed that acid stress (5 min at pH = 2.0) has led to a slight increase in MIC of TET and SXT for the non-Lm strains compared to the Lm strains under aerobic incubation (Figure 4A). It also led to an apparent increase in MICs of GEN and SXT for both Lm and non-Lm groups incubated in anaerobic conditions (Figure 4B). Moreover, both Lm and non-Lm groups appear to be more resistant to GEN, CIP, and SXT when incubated in anaerobic conditions rather than aerobic conditions (Figure 4B). However, no difference in MICs of AMP, TET, CHL, and IPM has been reported in both Lm and non-Lm groups under anaerobic incubation (Figure 4B). On the other hand, both Lm and non-Lm groups were susceptible to GEN and IPM when incubated in aerobic condition and susceptible to CHL and IPM when incubated in anaerobic conditions (Figures 4A,B).
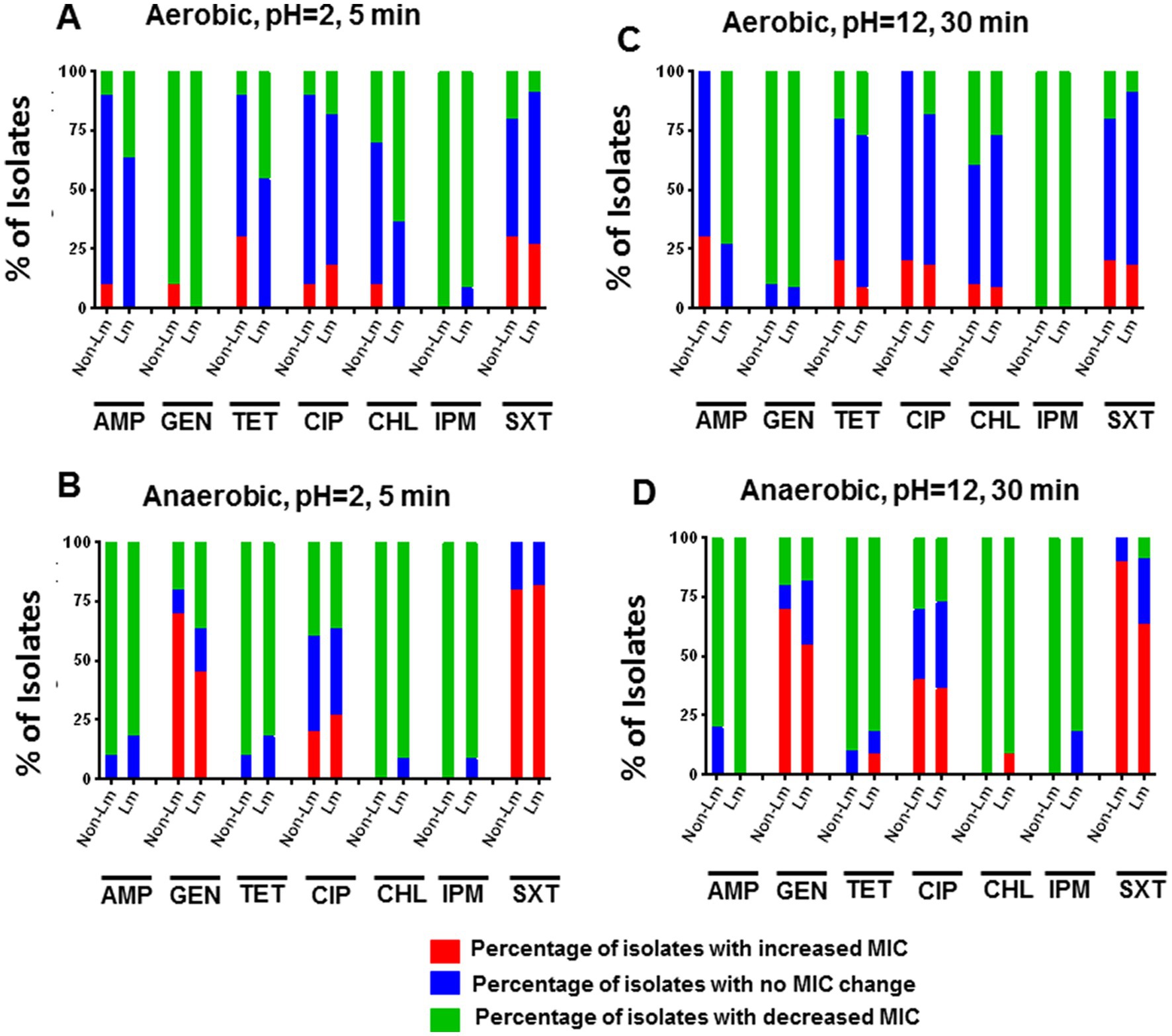
Figure 4. Changes in antimicrobial susceptibility in aerobic and anaerobic conditions after acid stress (pH 2, 5 min) and alkaline stress (pH 12, 30 min). AMP: ampicillin, GEN: gentamicin, TET: tetracycline, CIP: ciprofloxacin, CHL: chloramphenicol, IPM: imipenem, SXT: trimethoprim-sulfamethoxazole. (A) Change of MIC (increase/decrease) after acid stress compared with pre-stress (before stress) under aerobic incubation. (B) Change of MIC (increase/decrease) after acid stress compared with pre-stress (before stress) under anaerobic incubations. (C) Change of MIC (increase/decrease) after alkaline stress, compared with pre-stress (before stress) under aerobic incubation. (D) Change of MIC (increase/decrease) after alkaline stress, compared with pre-stress (before stress) under anaerobic incubations.
Alkaline stress (30 min at pH = 12.0) has resulted in a slight increase in MICs of TET and AMP for non-Lm than Lm isolates under aerobic incubation (Figure 4C). It also led to an apparent increase in MICs of GEN, CIP, and SXT for non-Lm isolates under anaerobic conditions (Figure 4D). Additionally, Alkaline stress resulted in a decreased MIC of IPM for nearly all isolates regardless of aerobic and anaerobic incubations (Figures 4C,D). Moreover, the overall shift in greater sensitivity to AMP was more dramatic for non-Lm isolates under anaerobic incubation (Figure 4D).
3.3.3. Effect of UV stress on antimicrobial susceptibility
Experiments were carried out to understand whether UV stress (exposition to UV during 60s) significantly impacts the antimicrobial susceptibility of Lm and non-Lm isolates under aerobic and anaerobic incubation. The outputs of this test were summarized in Supplementary Data, Supplementary Table S4. Our results showed that under aerobic conditions, UV stress increased MIC values of TET and SXT in a high percentage of non-Lm isolates compared to Lm isolates (Figure 5A). On the other hand, the application of UV stress followed by anaerobic incubation has led to increased MIC values of GEN and CIP in a high percentage of non-Lm compared to Lm isolates, while it increases the MIC values of SXT and IPM in a high rate of Lm compared to non-Lm isolates (Figure 5B). Furthermore, UV stress enhanced antimicrobial resistance of both Lm and non-Lm toward GEN when incubated in anaerobic conditions (Figure 5B).
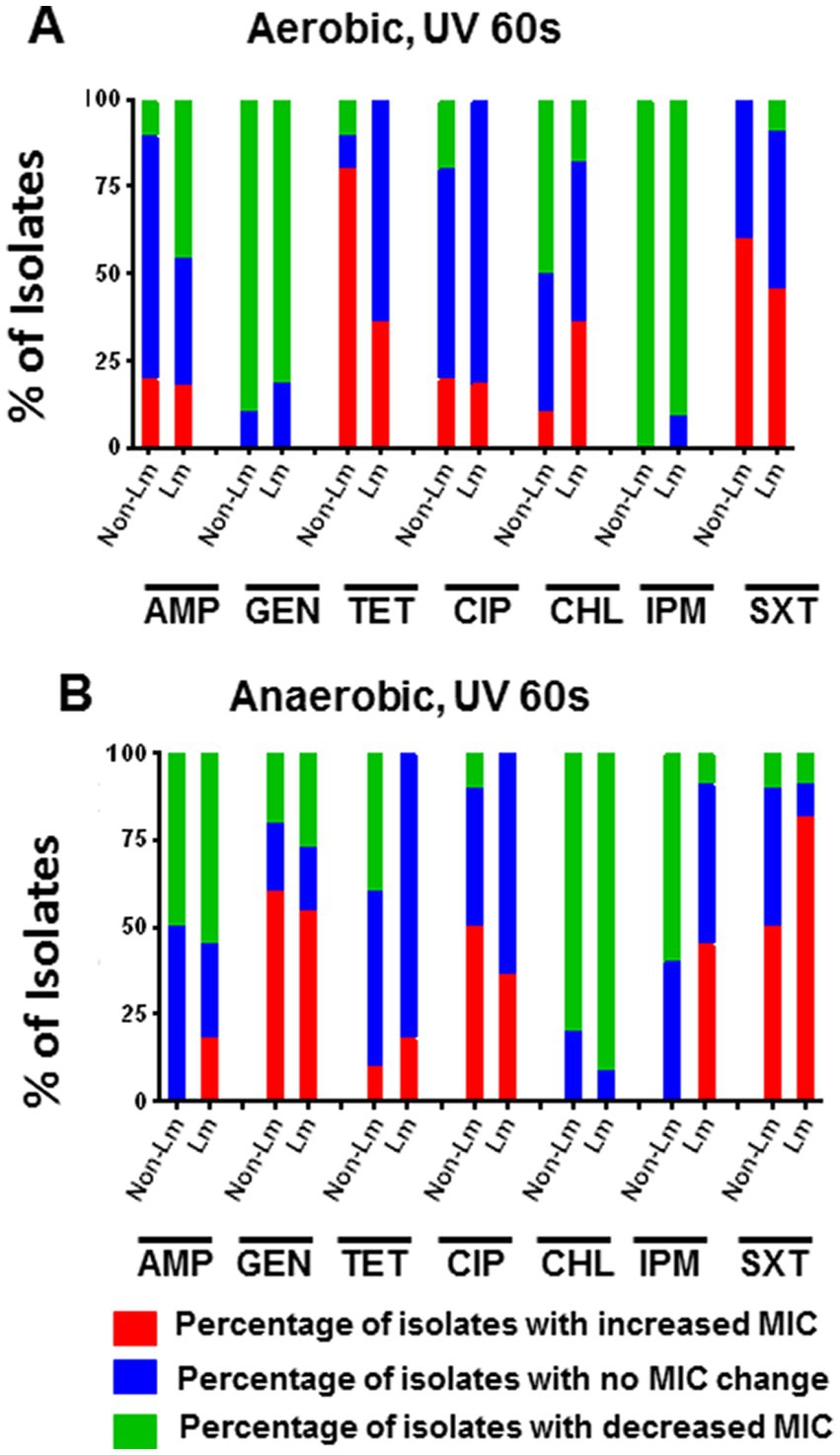
Figure 5. Changes in antimicrobial susceptibility in aerobic and anaerobic conditions after UV stress of the 60s. AMP: ampicillin, GEN: gentamicin, TET: tetracycline, CIP: ciprofloxacin, CHL: chloramphenicol, IPM: imipenem, SXT: trimethoprim-sulfamethoxazole. (A) Change of MIC (increase/decrease) after UV stress compared with pre-stress (before stress) under aerobic incubation. (B) Change of MIC (increase/decrease) after UV stress compared with pre-stress (before stress) under anaerobic incubations.
3.3.4. Effect of osmotic stress on antimicrobial susceptibility
To evaluate the effect of osmotic stress on the antimicrobial susceptibility of Listeria species, MIC values were determined based on Listeria isolates exposed to 10% NaCl for 27 days. The obtained results were shown in Supplementary Data, Supplementary Table S5. In fact, we noticed that under aerobic incubation, osmotic stress has resulted in an apparent increase in MIC value of AMP for non-Lm than Lm isolates (Figure 6A). Moreover, increase in MIC values of GEN and SXT were evidenced for both Lm and non-Lm isolates under anaerobic conditions when compared with aerobic conditions (Figure 6B), while aerobic incubation has increased MIC value of TET for both Lm and non-Lm isolates compared to anaerobic incubation (Figure 6A). Furthermore, it seems that anaerobic incubation rendered Lm isolates more resistant to SXT than non-Lm isolates (Figure 6B).
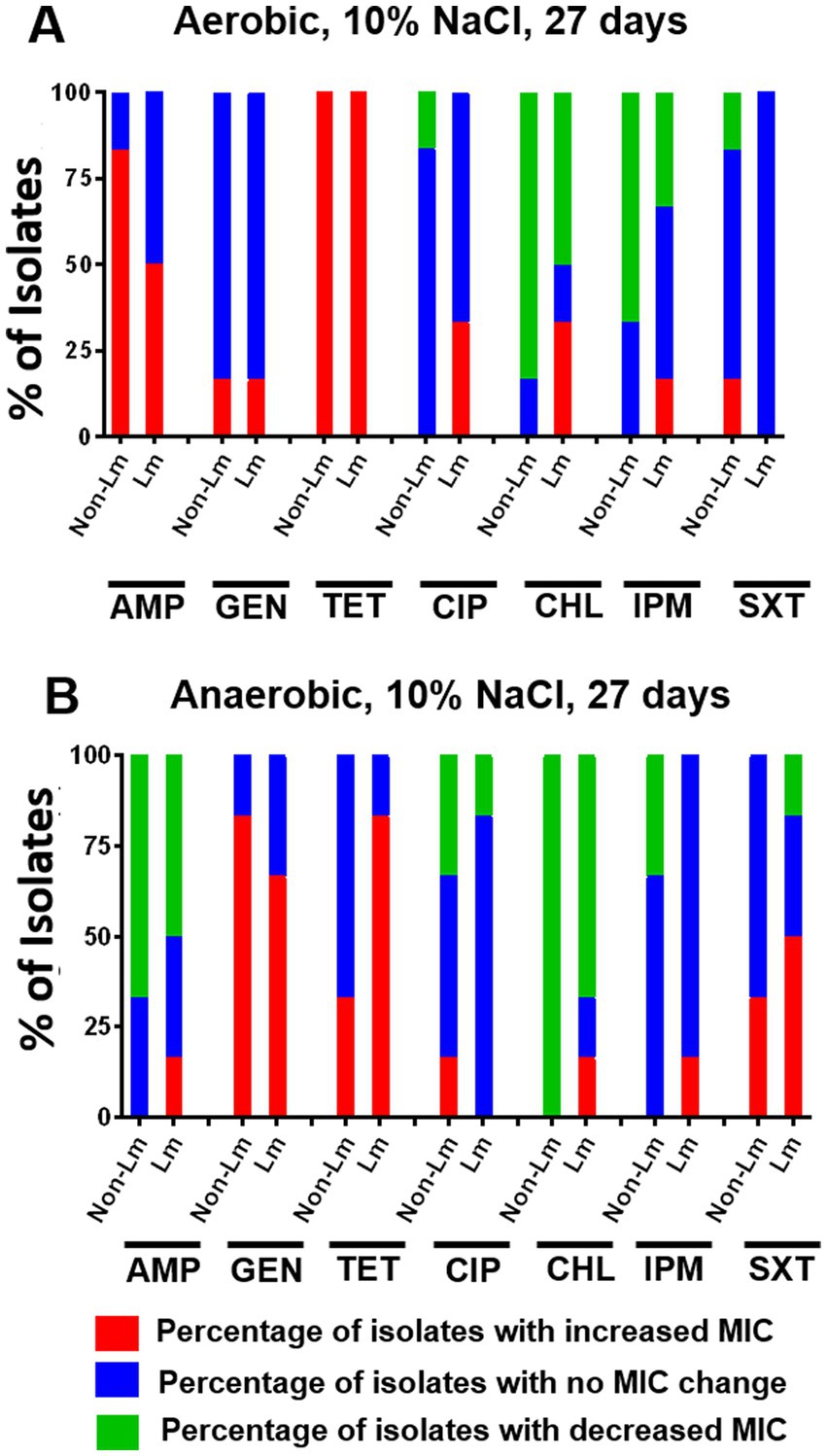
Figure 6. Changes in antimicrobial susceptibility in aerobic and anaerobic conditions after osmotic stress of 27 days. AMP: ampicillin, GEN: gentamicin, TET: tetracycline, CIP: ciprofloxacin, CHL: chloramphenicol, IPM: imipenem, SXT: trimethoprim-sulfamethoxazole. (A) Change of MIC (increase/decrease) after osmotic stress of 27 days compared with pre-stress (before stress) under aerobic incubation. (B) Change of MIC (increase/decrease) after osmotic stress of 27 days compared with pre-stress (before stress) under anaerobic incubations.
4. Discussion
Contamination of various food products along the food chain by L. monocytogenes is a significant risk to the food industry and public health. Additionally, the large scale of using disinfectants during the pandemic of COVID-19 in food industries may influence the behavior of foodborne pathogens toward different antimicrobial agents, in which, we hypothesized that selective pressure exerted by disinfectants could induce the antimicrobial resistance of bacteria, especially Listeria species through cross-and co-resistance mechanisms (Wu et al., 2023). Indeed, some previous studies have demonstrated the ability of stress exposures to induce resistance mutations and influence bacterial susceptibility to antimicrobials (Foster, 2008; Al-Nabulsi et al., 2015; Nambiar and Yue, 2022). Hence, we evaluated in this study the behavior of Lm and non-Lm species to heat treatment, acidic and alkaline pH, UV irradiation, and 10% sodium chloride, as well as the effect of stress conditions on the change in antimicrobial susceptibility under both aerobic and anaerobic incubations.
Our findings showed that the Lm strains are relatively more tolerant to environmental stress conditions, including heat treatment, acidic and alkaline pH, UV stress, and osmotic stress, than the non-Lm strains (p > 0.05). As known, Listeria species, especially L. monocytogenes has the capabilities to survive under extreme environmental conditions like a wide range of temperature (0°C–45°C), a wide range of pH (4.1–9.6), low water activity (<0.9), and high osmotic stress (up to 10% w/v of NaCl) (Chen et al., 2019; Anwar et al., 2022). Moreover, previous studies have demonstrated the ability of L. monocytogenes to resist stress during food processing and storage (Bucur et al., 2018), including tolerance to heat stress (60°C for 10 min) (Shen et al., 2014), acidic pH (pH = 3), alkaline pH (pH = 12), and saturated NaCl (Liu et al., 2005), and exposition to UV (Gayán et al., 2015). We could argue that the pathogenic species of Listeria (L. monocytogenes) shares similar morphological, biochemical, and genetic characteristics with other non-pathogenic species of Listeria (Li et al., 2021). In this regard, previous studies have reported the close genetic relationships between L. monocytogenes and L. innocua coexisted in similar ecological niches, these species shared similar plasmids and resistance/virulence genes and may horizontally transfer common genetic elements (Li et al., 2021).
Recent findings have shown the increase in antimicrobial resistance of Listeria spp. recovered from different sources, including foods, environment, and humans (Bouymajane et al., 2021; Anwar et al., 2022). Indeed, the increase in antimicrobial resistance has been attributed to the misuse and overuse of antibiotics in veterinary and medical fields (Chang et al., 2019; Shao et al., 2021). However, advanced studies have concluded that bacteria could develop antimicrobial resistance even in absence of antibiotics use (Li et al., 2022). This may be due to different mechanisms where bacteria develop resistance and adaptation mechanisms to different unfavorable conditions and resist by the same mechanisms to different antibiotic classes (co-and cross-resistance mechanisms). In this study, we evidenced that the adaptation to environmental stresses such as heat stress, acidic/alkaline stress, and osmotic stress induces the development of resistance by increasing the MIC of gentamicin and trimethoprim-sulfamethoxazole in anaerobic incubation. Moreover, the application of UV stress increased the MIC of trimethoprim-sulfamethoxazole in Lm group and MICs of gentamicin in both Lm and non-Lm groups. This increase in MICs may be attributed to changes in binding protein and transport of antibiotics across the cell membrane (Taber et al., 1987; Guinane et al., 2006). Interestingly, we observed increased resistance to some antibiotics of both Lm and non-Lm strains incubated in anaerobic conditions, this finding is alarming because the anaerobic packaging and storage of food products under different stressors may help bacteria, especially Listeria species, to develop resistance to the critical antimicrobial agents.
Overall, these findings could be relevant to FPEs, specifically where food products are exposed to various stresses such as temperature, pH, salt, and UV stress to prolong their shelf life, preserve their nutritional value, and prevent microbial spoilage (Singh and Shalini, 2016; Amit et al., 2017). We may argue that contamination of food products by antimicrobial-resistant strains in FPEs and laterally consumed by consumers may be more difficult to treat in clinical settings. Subsequently, the strains are more difficult to treat by conventional drugs in vivo within anaerobic host environments (Sydnor and Perl, 2011; Nguyen et al., 2018); thereby leading to unsuccessful therapeutic outcomes and possibly higher mortality (Sydnor and Perl, 2011). To our knowledge, this is the first study covering the Lm and non-Lm species antimicrobials susceptibility under aerobic and anaerobic incubations with various stress conditions.
Nevertheless, the adaptive response to various food-related stresses is a complicated process. It depends on several physical and chemical stresses which challenge the bacterial cell and determine the fate of the pathogen along the food chain. Subsequently, this impacts the disease-causing potential of strains and the outcome of the disease. In a nutshell, we have found a greater tendency for tolerance, resistance and/or cross-protection for the “Lm” species compared to the “non-Lm” species. These findings showed that resistant L. monocytogenes in FPEs, can cause contamination of food products and clinical infection. Additionally, stressed L. monocytogenes showed high MIC values by displaying antimicrobial resistance potential against critical antimicrobials such as trimethoprim-sulfamethoxazole, gentamicin, and ampicillin, which may pose profound implications for clinical practices that are also involved with numerous physical and chemical stresses. From this perspective, the findings of the current study suggested potential risks to food technology and public health. Therefore, keeping the food chain or FPEs free from L. monocytogenes contamination is fundamental for designing improved food processing conditions and public health safety.
5. Conclusion
The findings of this study showed higher tolerance capabilities among Lm strains than non-Lm strains to various stresses. More importantly, our findings evidenced that the exposition to environmental stress like heat, acidic, alkali, UV, and osmotic stress rendered Listeria species more resistant to antimicrobial agents, especially in anaerobic conditions which is often used in food packaging and storage. Hence, the findings of this study highlight the significance of stress tolerance in affecting antimicrobial resistance phenotypes of Listeria species regarding the clinically critical antimicrobials, posing significant public health concerns. Finally, we believe that further studies based on more widely sampling Listeria isolates are needed to confirm these results and conclusions.
Data availability statement
The original contributions presented in the study are included in the article/Supplementary material, further inquiries can be directed to the corresponding authors.
Author contributions
HW, MF, TA, and WC conducted lab experiments, analyzed data, and generated figures. AE-D analyzed data and revised the draft manuscript. XK, KR, and CK revised and critically commented on the draft manuscript. MY and YL conceived the project and provided critical comments for the draft. All authors have read and agreed to the published version of the manuscript.
Funding
This work was supported by the National Program on Key Research Project of China (2019YFE0103900) as well as the European Union’s Horizon 2020 Research and Innovation Programme under Grant Agreement No. 861917 – SAFFI, Scientific Research Foundation of Zhejiang Province (2022C03188), Zhejiang Provincial Key R&D Program of China (2022C02024 and 2021C02008), Key Research and Development Program of Hangzhou (202203A08) and District-level project for high-level innovative and entrepreneurial talents of “Zijinshan Talents Gaochun Plan” (202100677).
Conflict of interest
The authors declare that the research was conducted in the absence of any commercial or financial relationships that could be construed as a potential conflict of interest.
Publisher’s note
All claims expressed in this article are solely those of the authors and do not necessarily represent those of their affiliated organizations, or those of the publisher, the editors and the reviewers. Any product that may be evaluated in this article, or claim that may be made by its manufacturer, is not guaranteed or endorsed by the publisher.
Supplementary material
The Supplementary material for this article can be found online at: https://www.frontiersin.org/articles/10.3389/fsufs.2023.1179835/full#supplementary-material
References
Al-Nabulsi, A. A., Osaili, T. M., Shaker, R. R., Olaimat, A. N., Jaradat, Z. W., Zain Elabedeen, N. A., et al. (2015). Effects of osmotic pressure, acid, or cold stresses on antibiotic susceptibility of listeria monocytogenes. Food Microbiol. 46, 154–160. doi: 10.1016/J.FM.2014.07.015
Amit, S. K., Uddin, M. M., Rahman, R., Islam, S. M. R., and Khan, M. S. (2017). A review on mechanisms and commercial aspects of food preservation and processing. Agric. Food Secur. 6, 1–22. doi: 10.1186/S40066-017-0130-8/TABLES/21
Anwar, T. M., Pan, H., Chai, W., Ed-Dra, A., Fang, W., Li, Y., et al. (2022). Genetic diversity, virulence factors, and antimicrobial resistance of listeria monocytogenes from food, livestock, and clinical samples between 2002 and 2019 in China. Int. J. Food Microbiol. 366:109572. doi: 10.1016/j.ijfoodmicro.2022.109572
Bergholz, T. M., den Bakker, H. C., Fortes, E. D., Boor, K. J., and Wiedmann, M. (2010). Salt stress phenotypes in listeria monocytogenes vary by genetic lineage and temperature. Foodborne Pathog. Dis. 7, 1537–1549. doi: 10.1089/FPD.2010.0624
Bouymajane, A., Rhazi Filali, F., Oulghazi, S., Lafkih, N., Ed-Dra, A., Aboulkacem, A., et al. (2021). Occurrence, antimicrobial resistance, serotyping and virulence genes of listeria monocytogenes isolated from foods. Heliyon 7:e06169. doi: 10.1016/j.heliyon.2021.e06169
Buchanan, R. L., Gorris, L. G. M., Hayman, M. M., Jackson, T. C., and Whiting, R. C. (2017). A review of listeria monocytogenes: an update on outbreaks, virulence, dose-response, ecology, and risk assessments. Food Control 75, 1–13. doi: 10.1016/J.FOODCONT.2016.12.016
Bucur, F. I., Grigore-Gurgu, L., Crauwels, P., Riedel, C. U., and Nicolau, A. I. (2018). Resistance of listeria monocytogenes to stress conditions encountered in food and food processing environments. Front. Microbiol. 9:2700. doi: 10.3389/fmicb.2018.02700
Buncic, S., Avery, S. M., Rocourt, J., and Dimitrijevic, M. (2001). Can food-related environmental factors induce different behaviour in two key serovars, 4b and 1/2a, of listeria monocytogenes? Int. J. Food Microbiol. 65, 201–212. doi: 10.1016/S0168-1605(00)00524-9
Chang, Y., Chusri, S., Sangthong, R., McNeil, E., Hu, J., Du, W., et al. (2019). Clinical pattern of antibiotic overuse and misuse in primary healthcare hospitals in the southwest of China. PLoS One 14:e0214779. doi: 10.1371/JOURNAL.PONE.0214779
Chen, M., Cheng, J., Zhang, J., Chen, Y., Zeng, H., Xue, L., et al. (2019). Isolation, potential virulence, and population diversity of listeria monocytogenes from meat and meat products in China. Front. Microbiol. 10:946. doi: 10.3389/fmicb.2019.00946
Dunnett, E., Florea, L., Thurston, L., Floyd, T., Collins, R., and Otter, A. (2020). Deaths of weaned lambs with visceral listeria ivanovii i nfections. Vet. Rec. Case Rep. 8:e001254. doi: 10.1136/VETRECCR-2020-001254
Foster, P. L. (2008). Stress-Induced Mutagenesis in Bacteria. Crit. Rev. Biochem. Mol. Biol. 42, 373–397. doi: 10.1080/10409230701648494
Francis, G. A., and O’Beirne, D. (2005). Variation among strains of listeria monocytogenes: differences in survival on packaged vegetables and in response to heat and acid conditions. Food Control 16, 687–694. doi: 10.1016/J.FOODCONT.2004.06.005
Gandhi, M., and Chikindas, M. L. (2007). Listeria: a foodborne pathogen that knows how to survive. Int. J. Food Microbiol. 113, 1–15. doi: 10.1016/J.IJFOODMICRO.2006.07.008
Gayán, E., Serrano, M. J., Pagán, R., Álvarez, I., and Condón, S. (2015). Environmental and biological factors influencing the UV-C resistance of listeria monocytogenes. Food Microbiol. 46, 246–253. doi: 10.1016/J.FM.2014.08.011
Guinane, C. M., Cotter, P. D., Ross, R. P., and Hill, C. (2006). Contribution of penicillin-binding protein homologs to antibiotic resistance, cell morphology, and virulence of listeria monocytogenes EGDe. Antimicrob. Agents Chemother. 50, 2824–2828. doi: 10.1128/AAC.00167-06/ASSET/D73488E3-F530-4EF5-8691-18A324495231/ASSETS/GRAPHIC/ZAC0080659680002.JPEG
Hinnu, M., Putrinš, M., Kogermann, K., Bumann, D., and Tenson, T. (2022). Making antimicrobial susceptibility testing more physiologically relevant with bicarbonate? Antimicrob. Agents Chemother. 66:e0241221. doi: 10.1128/AAC.02412-21/SUPPL_FILE/AAC.02412-21-S0001.PDF
Imran, M., Das, K. R., and Naik, M. M. (2019). Co-selection of multi-antibiotic resistance in bacterial pathogens in metal and microplastic contaminated environments: an emerging health threat. Chemosphere 215, 846–857. doi: 10.1016/j.chemosphere.2018.10.114
Koutsoumanis, K. P., Kendall, P. A., and Sofos, J. N. (2003). Effect of food processing-related stresses on acid tolerance of listeria monocytogenes. Appl. Environ. Microbiol. 69, 7514–7516. doi: 10.1128/AEM.69.12.7514-7516.2003/ASSET/51EA25C7-DB4B-40E1-9352-AB26A810A14A/ASSETS/GRAPHIC/AM1230608GR1.JPEG
Lee, B. H., Cole, S., Badel-Berchoux, S., Guillier, L., Felix, B., Krezdorn, N., et al. (2019). Biofilm formation of listeria monocytogenes strains under food processing environments and Pan-genome-wide association study. Front. Microbiol. 10:2698. doi: 10.3389/FMICB.2019.02698/BIBTEX
Leong, D., Alvarez-Ordóñez, A., and Jordan, K. (2014). Monitoring occurrence and persistence of listeria monocytogenes in foods and food processing environments in the Republic of Ireland. Front. Microbiol. 5:436. doi: 10.3389/FMICB.2014.00436/BIBTEX
Li, Y., Ed-Dra, A., Tang, B., Kang, X., Müller, A., Kehrenberg, C., et al. (2022). Higher tolerance of predominant salmonella serovars circulating in the antibiotic-free feed farms to environmental stresses. J. Hazard. Mater. :129476. doi: 10.1016/J.JHAZMAT.2022.129476
Li, M., Yan, S., Fanning, S., Li, F., and Xu, J. (2021). Whole genome analysis of three multi-drug resistant listeria innocua and genomic insights into their relatedness with resistant listeria monocytogenes. Front. Microbiol. 12:694361. doi: 10.3389/FMICB.2021.694361/FULL
Liao, X., Ma, Y., Daliri, E. B. M., Koseki, S., Wei, S., Liu, D., et al. (2020). Interplay of antibiotic resistance and food-associated stress tolerance in foodborne pathogens. Trends Food Sci. Technol. 95, 97–106. doi: 10.1016/J.TIFS.2019.11.006
Liu, D., Lawrence, M. L., Ainsworth, A. J., and Austin, F. W. (2005). Comparative assessment of acid, alkali and salt tolerance in listeria monocytogenes virulent and avirulent strains. FEMS Microbiol. Lett. 243, 373–378. doi: 10.1016/J.FEMSLE.2004.12.025
Martínez-Suárez, J. V., Ortiz, S., and López-Alonso, V. (2016). Potential impact of the resistance to quaternary ammonium disinfectants on the persistence of listeria monocytogenes in food processing environments. Front. Microbiol. 7:638. doi: 10.3389/FMICB.2016.00638/BIBTEX
McKinney, J., Williams, R. C., Boardman, G. D., Eifert, J. D., and Sumner, S. S. (2009). Dose of UV light required to inactivate listeria monocytogenes in distilled water, fresh brine, and spent brine. J. Food Prot. 72, 2144–2150. doi: 10.4315/0362-028X-72.10.2144
Miladi, H., Elabed, H., Ben Slama, R., Rhim, A., and Bakhrouf, A. (2017). Molecular analysis of the role of osmolyte transporters opu CA and bet L in listeria monocytogenes after cold and freezing stress. Arch. Microbiol. 199, 259–265. doi: 10.1007/S00203-016-1300-Y/METRICS
Muhterem-Uyar, M., Dalmasso, M., Bolocan, A. S., Hernandez, M., Kapetanakou, A. E., Kuchta, T., et al. (2015). Environmental sampling for listeria monocytogenes control in food processing facilities reveals three contamination scenarios. Food Control 51, 94–107. doi: 10.1016/J.FOODCONT.2014.10.042
Nambiar, R. B., and Yue, M. (2022). Persistence Phenotype. In: Ding, T., Liao, X., Feng, J. (eds)Stress Responses of Foodborne Pathogens. Springer, Cham.doi: 10.1007/978-3-030-90578-1_15
Nguyen, L., Garcia, J., Gruenberg, K., and Mac Dougall, C. (2018). Multidrug-resistant pseudomonas infections: hard to treat, but Hope on the horizon? Curr. Infect. Dis. Rep. 20, 1–10. doi: 10.1007/S11908-018-0629-6/METRICS
Nic Aogáin, K., and O’Byrne, C. P. (2016). The role of stress and stress adaptations in determining the fate of the bacterial pathogen listeria monocytogenes in the food chain. Front. Microbiol. 7:1865. doi: 10.3389/FMICB.2016.01865/BIBTEX
Pilmis, B., Leclercq, A., Maury, M. M., Moura, A., Bracq-Dieye, H., Thouvenot, P., et al. (2020). Cutaneous listeriosis, a case series of 16 consecutive patients over 25 years. J. Infect. 80, 232–254. doi: 10.1016/J.JINF.2019.10.004
Ruiz, L., Aertsen, A., Nguyen-The, C., Gänzle, M. G., and Alvarez-Ordóñez, A. (2017). Industrial and host associated stress responses in food microbes. Implications for food technology and food safety. Front. Microbiol. 8:1522. doi: 10.3389/FMICB.2017.01522/BIBTEX
Schmid, M. W., Ng, E. Y. W., Lampidis, R., Emmerth, M., Walcher, M., Kreft, J., et al. (2005). Evolutionary history of the genus listeria and its virulence genes. Syst. Appl. Microbiol. 28, 1–18. doi: 10.1016/J.SYAPM.2004.09.005
Shao, Y., Wang, Y., Yuan, Y., and Xie, Y. (2021). A systematic review on antibiotics misuse in livestock and aquaculture and regulation implications in China. Sci. Total Environ. 798:149205. doi: 10.1016/J.SCITOTENV.2021.149205
Shen, Q., Jangam, P. M., Soni, K. A., Nannapaneni, R., Schilling, W., and Silva, J. L. (2014). Low, medium, and high heat tolerant strains of listeria monocytogenes and increased heat stress resistance after exposure to sublethal heat. J. Food Prot. 77, 1298–1307. doi: 10.4315/0362-028X.JFP-13-423
Shi, D., Anwar, T. M., Pan, H., Chai, W., Xu, S., and Yue, M. (2021). Genomic determinants of pathogenicity and antimicrobial resistance for 60 global listeria monocytogenes isolates responsible for invasive infections. Front. Cell. Infect. Microbiol. 11:706. doi: 10.3389/fcimb.2021.718840
Singh, S., and Shalini, R. (2016). Effect of hurdle technology in food preservation: a review. Crit. Rev. Food Sci. Nutr. 56, 641–649. doi: 10.1080/10408398.2012.761594
Sydnor, E. R. M., and Perl, T. M. (2011). Hospital epidemiology and infection control in acute-care settings. Clin. Microbiol. Rev. 24, 141–173. doi: 10.1128/CMR.00027-10/ASSET/4276AE64-8B7E-493F-927C-D0D2514D47F2/ASSETS/GRAPHIC/ZCM9990923430001.JPEG
Taber, H. W., Mueller, J. P., Millert And, P. F., and Arrow2, A. S. (1987). Bacterial uptake of aminoglycoside antibiotics. Microbiol. Rev. 51, 439–457. doi: 10.1128/mr.51.4.439-457.1987
Wałecka-Zacharska, E., Gmyrek, R., Skowron, K., Kosek-Paszkowska, K., and Bania, J. (2018). Duration of Heat Stress Effect on Invasiveness of L. monocytogenes strains. Biomed. Res. Int. 2018:2018. doi: 10.1155/2018/1457480
Wang, Z., Liao, S., Huang, G., Feng, M., Yin, R., Teng, L., et al. (2023). Infant food production environments: a potential reservoir for vancomycin-resistant enterococci non-nosocomial infections. Int. J. Food Microbiol. 389:110105. doi: 10.1016/J.IJFOODMICRO.2023.110105
Wu, R. A., Feng, J., Yue, M., Liu, D., and Ding, T. (2023). Overuse of food-grade disinfectants threatens a global spread of antimicrobial-resistant bacteria. Crit. Rev. Food Sci. Nutr., 1–10. doi: 10.1080/10408398.2023.2176814
Keywords: Listeria monocytogenes, Listeria ivanovii, Listeria innocua, food processing environment, stress resistance, antimicrobial resistance
Citation: Wang H, Feng M, Anwar TM, Chai W, Ed-Dra A, Kang X, Rantsiou K, Kehrenberg C, Yue M and Li Y (2023) Change in antimicrobial susceptibility of Listeria spp. in response to stress conditions. Front. Sustain. Food Syst. 7:1179835. doi: 10.3389/fsufs.2023.1179835
Edited by:
Shoukui He, Shanghai Jiao Tong University, ChinaReviewed by:
Xiangan Han, Chinese Academy of Agricultural Sciences, ChinaChengming Wang, Auburn University, United States
Copyright © 2023 Wang, Feng, Anwar, Chai, Ed-Dra, Kang, Rantsiou, Kehrenberg, Yue and Li. This is an open-access article distributed under the terms of the Creative Commons Attribution License (CC BY). The use, distribution or reproduction in other forums is permitted, provided the original author(s) and the copyright owner(s) are credited and that the original publication in this journal is cited, in accordance with accepted academic practice. No use, distribution or reproduction is permitted which does not comply with these terms.
*Correspondence: Yan Li, yanli3@zju.edu.cn
†These authors have contributed equally to this work