- 1Department of Biotechnology, Ambala College of Engineering and Applied Research, Ambala, Haryana, India
- 2Department of Biotechnology, Chaudhary Devi Lal University, Sirsa, Haryana, India
- 3Department of Biotechnology, Guru Jambheswar University, Hisar, Haryana, India
- 4University Institute of Biotechnology, Chandigarh University, Mohali Punjab, India
- 5Department of Plant-Pathology, Faculty of Agricultural Sciences, SGT University, Gurgaon, Haryana, India
- 6Department of Botany, Lucknow University, Lucknow, India
- 7Department of Food Technology, School of Applied and Life Sciences, Uttaranchal University, Dehradu, India
The presence of high tannin content in fruit juices and other beverages generates bitterness and haze formation with a concomitant deterioration of their quality. This problem could be circumvented by treating these food products with the enzyme tannase (tannin acyl hydrolase EC 3.1.1.20), which contains the ability to hydrolyze the ester and depside linkages present in hydrolyzable tannins. In the present study, a low molecular weight (38.20 kDa) tannase purified from Klebsiella pneumoniae KP715242 was used for clarification and detannification of orange juice, beer, and tea cream solubilization. The initial tannin content of orange juice was reduced to 42.45% upon treatment with 4.5 U/mL of purified tannase at 35°C for 3.5 h. Beer haze was clarified by incubating the colloidal suspension with the purified enzyme. Under the optimum condition of temperature (30°C), incubation time (3.5 h), and enzyme concentration (4.5 U/mL), the initial tannin content of beer was reduced to 46.47%. Tea cream solubilization was achieved by treating the tea extract with varying enzyme concentrations at different time intervals and temperatures. The maximum reduction in the tea tannin content (55%) was observed at 35°C when the enzyme was incubated for 3 h at a concentration of 6.0 U/mL. The results of this investigation could be further exploited at an industrial scale in the detannification of fruit juice, beer, and tea.
Introduction
Tannins are plant components known as the fourth most common group of these compounds, later to lignin, hemicellulose, and cellulose. Tannins are water-soluble polyphenols with molecular weights between 0.3 and 5 kDa. Tannins are a class of polyphenols with a high molecular weight naturally present in plants used for food and feed. They can be classified into two major categories: hydrolyzable and condensed tannins. Hydrolyzable tannins include gallotannins and ellagotannins, which can be hydrolyzed to yield glucose, gallic acid, and ellagic acid, respectively (Singh et al., 2021, 2022; Singh A. et al., 2023; Singh N. et al., 2023).
On the other hand, condensed tannins (proanthocyanidins) are oligomers and polymers of flavan-3-ol units consisting of various isomeric forms of catechin and gallocatechin linked by C4-C6 or C4-C8 bonds (Catelani et al., 2016; Wilhelmy et al., 2021; Mostafa, 2023). Tannase (tannin acyl hydrolase EC 3.1.1.20) is an inducible enzyme that possesses the capacity to hydrolyze ester and depside linkages. Tannic acid’s ester bond is broken, and gallic acid and glucose are released as a result (Jana et al., 2014). Although tannase has been discovered in various organisms, including yeast, bacteria, and filamentous fungi, they are the primary producers (Raveendran et al., 2018). The high tannin content in drinks, including iced tea, wine, beer, fruit juices, and coffee-flavored drinks, contributes to the precipitate development in beverages when combined with other molecules. Fruit juices are treated with tannases to eliminate these undesirable characteristics (Kanpiengjai et al., 2020).
Among these microorganisms, most of the study has focused on fungi. Fungal tannases are highly specific to hydrolyzing ester bonds of hydrolyzable tannins, producing gallic acid and glucose. This specificity makes them highly effective in various applications such as beverage processing, leather processing, and waste treatment. Additionally, fungal tannases can be produced in high yields using cost-effective fermentation processes. Nevertheless, a fungal strain is characterized by its comparatively sluggish growth rate and genetic complication, which discourages its use on an industrial scale.
In contrast, bacteria own a prominent growth rate and are easy to get manipulated at the genetic level. In addition, bacteria are also characterized by their ability to endure extreme temperatures, making these organisms a better choice for industrial use than fungi (Kumar et al., 2016). Studies have shown that bacterial tannases exhibit greater thermostability than fungal tannases. For example, bacterial tannases from Bacillus species have been found to be active over a broad range of temperatures, ranging from 20 to 80°C, with optimal activity around 60°C. In contrast, most fungal tannases have a narrow temperature range for activity and are typically active at temperatures below 50°C. This difference in thermostability can be attributed to differences in the molecular structure and composition of the two types of enzymes. Bacterial tannases are usually composed of one subunit, which confers more excellent structural stability and resistance to heat and aids in the ease of cloning, overexpression, and purification (Ren et al., 2013; Kumar et al., 2016).
Additionally, bacterial tannase can more effectively break down and hydrolyze tannic acid and natural tannins (Dhiman et al., 2018). In contrast, fungal tannases are often composed of multiple subunits, making them more susceptible to denaturation and loss of activity at high temperatures. This multimeric nature also makes the overexpression and purification steps more complex. Numerous bacterial species that are known to generate tannase have been reported, including Lactobacillus plantarum, L. paraplantarum, L. pentosus, Pseudomonas aeruginosa, Enterobacter cloacae, and Klebsiella pneumoniae (Osawa et al., 2000; Nishitani and Osawa, 2006; Selwal et al., 2010; Beniwal et al., 2013; Kumar et al., 2015b, 2016).
Tannase is a versatile enzyme used in various sectors for the industrial processing and clarity of fruit juice, beer, and Acron wine. Additionally, tannase finds applications in detannifying food, removing tannins from high-quality leather, and imparting coffee flavor to soft beverages (Aracri et al., 2019; Cavalcanti et al., 2020). However, gallic acid production and instant tea processing represent tannase’s main industrial uses. Gallic acid is frequently used as a component of a developer in printing inks and photography. Additionally, it serves as a precursor for the industrial manufacturing of certain dyestuffs, propyl gallate, a food preservative, and the anti-microbial medication trimethoprim. Tannase is also employed in the pharmaceutical and chemical industries, as well as in the bioremediation of tannery effluent (Lekha et al., 1993; Aguilar and Gutierrez-Sanchez, 2001; Chhokar et al., 2010; Sharma et al., 2014). Because of the immense industrial potential of this enzyme, commercial usage of tannase has augmented in recent years. Juelich Chiral Solutions GmbH (Germany) in Europe was the first company that commercialized tannase in extract form (Aguilar and Gutierrez-Sanchez, 2001).
The elevated tannin content in fruit juices is the reason for haze and sediment formation, which deteriorates their quality upon storage. In the case of tea, the high tannin content is responsible for tea cream formation while it is kept at or below 4°C. This lowers the solubility of tea in cold water and hinders the manufacturing of instant tea (Lekha and Lonsane, 1997). Similarly, tannins are also responsible for the deterioration of beer quality due to haze formation after storage. The conventional non-enzymatic methods to remove tannins from juices and other beverages have not yielded satisfactory results.
Moreover, these methods also lead to the loss of important nutritional constituents as well as the aroma of drinks. Hence, a more efficient clarification or detannification method is vital in enhancing the quality and commercial value of these food products (Coggon et al., 1975). In this regard, enzymatic treatment of fruit juices and other beverages seems to be a better alternative to eliminate the problems associated with conventional methods of removing tannins. Because of these aspects, The current investigation was started to exploit the potential of purified tannase from Klebsiella pneumoniae KP715242 to attain a higher quality of fruit juice, beer, and instant tea by reducing the tannin content in these drinks. Moreover, To our knowledge, this is the initial report about the use of bacterial tannase in the detannification of a wide range of fruit juice and beverages.
Materials and methods
Chemicals
We purchased tannic acid and microbiological medium from Hi-media in Mumbai, India. Other chemicals with high analytical grades were also used. Orange, beer, and tea leaves were purchased from the local market of Ambala Cantt. India.
Microorganism and tannase production
Based on data obtained from morphological, biochemical and 16S ribosomal RNA sequences, a tannase-producing bacteria employed in the current investigation was isolated from rhizospheric soil of Acacia species and identified as Klebsiella pneumoniae (GenBank Accession Number KP715242; Kumar et al., 2015b).
A 250 mL Erlenmeyer flask was used to produce the enzymes, and it contained 50 mL of basal medium (K2HPO4:0.5 g/L, KH2PO4:0.5 g/L, MgSO4:2.0 g/L, CaCl2:1.0 g/L, NH4Cl:3.0 g/L, supplemented with 1 percent tannic acid, pH 5.5). The medium was inoculated with 2% of 24 h old inoculums and was incubated at 37°C for 48 h at 150 rpm.
Estimation of tannin content
The Hagerman and Butler technique was employed to determine the tannin concentration of orange juice, beer, and tea (Hagerman and Butler, 1978). A centrifuge tube combined 2.0 mL of BSA solution (1.0 mg/ml) and 1 ml of tannin extract. The mixture’s components were combined, and after around 20 min, the solution was centrifuged at 5,000 g for 15 min at room temperature. The pellet surface and tube wall were rinsed with buffer without disturbing the particle, and the supernatant was discarded afterward. The precipitates were dissolved in 3 ml of SDS-triethanolamine solution, followed by adding 1.0 ml of the ferric chloride reagent and mixing. Following a 30-min incubation period, the absorbance at 510 nm was measured, and the content of tannins in the test sample was calculated using a standard tannic acid curve.
Tannase assay
In a 0.2 M acetate buffer (pH 5.5) containing 0.3 ml of 1.0 percent (w/v) tannic acid, the enzyme solution was incubated at 40°C for 40 min. The remaining tannic acid was precipitated when the reaction was stopped by adding 3 ml of BSA (1 mg/ml). BSA has a strong attraction to tannins and can connect with any unconsumed tannins in the sample. This bond creates a physical obstruction between the tannins and the tannase enzyme, hindering additional enzymatic activity. The heat-denatured enzyme was used as a control as well. The precipitates were liquefied in 3 ml of SDS-triethanolamine solution (1 percent w/v, triethanolamine) after centrifuging the test and control tubes (12,000 g, 10 min). The mixture was then combined with 1.0 ml of FeCl3 reagent (0.13 M) and allowed to sit for 15 min to ensure the color’s stability. At 530 nm, the test and control tubes’ absorbance was compared to a blank (without tannic acid). The tannase that can hydrolyze 1 mM of substrate tannic acid in 1 min under typical test conditions was determined as one unit (Mondal et al., 2001).
Tannase purification
Ammonium sulfate precipitation and dialysis
The 85% (NH4)2SO4 saturation came after the crude enzyme extract had been concentrated by osmosis against concrete sucrose. The precipitates were then dissolved in a buffer solution of 0.02 M acetate at a pH of 5.5 following centrifugation of the fractions at 12,000 g for 20 min (containing 5 mM EDTA). The dissolved sample was dialyzed for 24 h with frequent buffer changes against 0.01 M acetate buffer (pH 5.5).
Ion exchange chromatography
DEAE-Cellulose was packed into a glass chromatography column measuring 18 cm by 2.5 cm, and 0.02 M acetate buffer was used to equilibrate it (pH 5.5). The dialyzed concentrated enzyme was delicately placed on the column’s top surface. Elution was performed with a linear gradient of 0.02 M acetate buffer (pH 5.5) with a volumetric time of 0.5 ml/min. The resulting fractions were then tested for tannase activity, and those with high tannase activity were combined and kept at 4°C for later use.
SDS-polyacrylamide gel electrophoresis
The molecular weight of the purified tannase was determined by sodium dodecyl sulfate-polyacrylamide gel electrophoresis (Laemmli, 1970) following ion-exchange chromatography (DEAE Cellulose). The Coomassie Brilliant Blue staining and subsequent destaining with a solution of methanol, glacial acetic acid, and distilled water allowed us to see the protein bands on the gel. Alphaview determined the purified tannase’s molecular weight (Alpha Innotec Gel Doc system).
Applications of tannase
Clarification of Orange juice
One liter of the orange juice was preserved for 4 days at 4°C, which resulted in the settling of the tannin-protein complex. The upper layer was decanted off, and a colloidal suspension was prepared by mixing the lower portion. Ten ml of the colloidal juice suspension was treated with the purified enzyme. Reduction in tannin content was maximized by optimizing the values of different factors like enzyme concentrations (0.5–7.5 U/ml), temperature (20–50°C), and incubation time (30–240 min). The residual tannin content of juice was determined earlier and later in tannase treatment following the method of Hagerman and Butler (1978).
Clarification of beer
To clarify the beer, 750 ml with chill haze was incubated at 4°C for 3 days to allow the haze to settle. After discarding the supernatant, purified tannase was added to 10 ml of the colloidal haze solution. The tannin content of the colloidal haze solution was determined before and after tannase treatment (Hagerman and Butler, 1978). Maximal tannin degradation was achieved by optimizing the optimum values of enzyme concentrations (0.5–7.5 U/ml), temperature (20–50°C) and incubation time (30–240 min).
Solubilization of tea cream
Fifty grams of raw tea leaves were dissolved in 200 ml of boiling distilled water. The mixture was allowed to settle for 30 min before filtering through Whatman No.1 filter paper. The filtrate was well blended and suspended in 100 ml of distilled water.
Ten milliliters of this colloidal tea solution were treated with the purified tannase at different conditions. Tannin degradation was maximized by optimizing the above variables, and the residual tannin content was determined before and after tannase treatment (Hagerman and Butler 1978).
Results and discussion
Tannase production and purification
Tannase-producing bacterium K. pneumoniae KP715242 was screened and isolated from the rhizospheric soil of Acacia species. The enzyme production was maximized statistically by optimizing the diverse parameters affecting tannase production through central composite design (CCD) using response surface methodology (RSM). The ideal conditions of pH 5.2, temperature 34.97°C, agitation speed 103.34 rpm, and incubation period 91.34 h, as reported in our earlier work, resulted in a 3.25-fold increase in enzyme production (Kumar et al., 2015b).
Using traditional purification techniques, including ammonium sulfate precipitation and anion exchange chromatography (DEAE cellulose), tannase was extracted from K. pneumoniae with a 9.5% enzyme yield and an 8.55 purification fold (Table 1). In a prior study (Kumar et al., 2015a), it was reported that the purified tannase from K. pneumoniae had a molecular weight of 38.20 kDa, as evidenced by a single band observed in SDS-PAGE (Figure 1). The enzyme was found to exhibit a Km of 0.00375 M and a Vmax of 3.64 mM/S.

Table 1. Purification of tannase isolated from Klebsiella pneumoniae KP715242 (Kumar et al., 2015a).
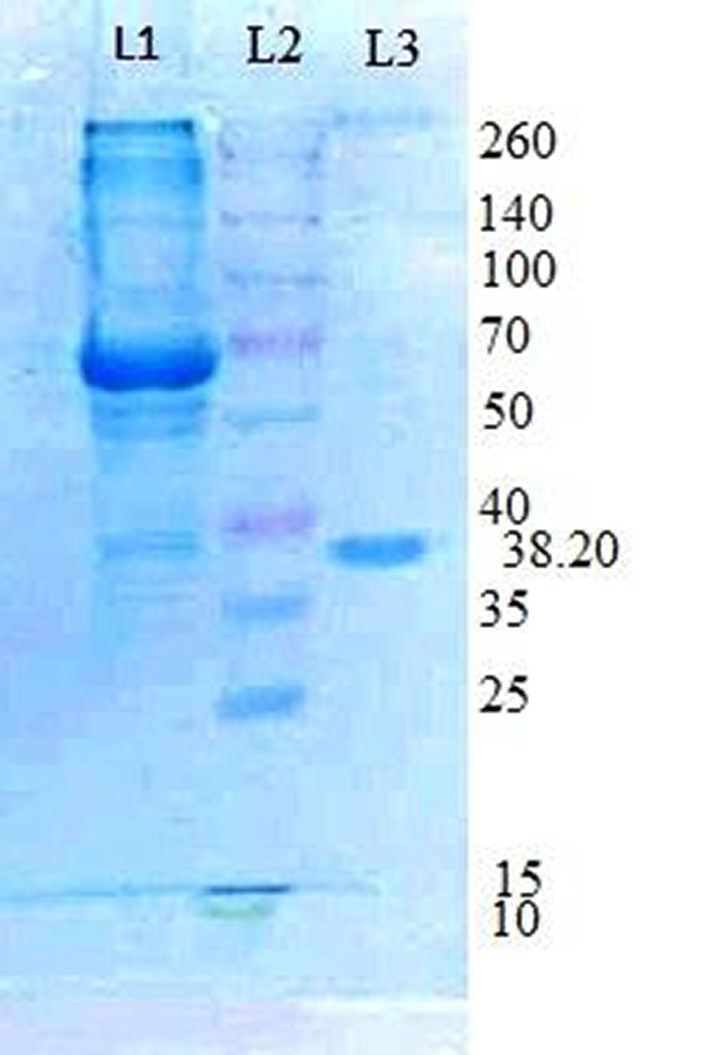
Figure 1. The molecular weight of purified Klebsiella pneumoniae KP715242 tannase was estimated by SDS-PAGE. (Lane 1) Crude extract, (Lane 2) Marker proteins (kDa), and (Lane 3) Purified tannase obtained after Ion-exchange chromatography (Kumar et al., 2015b).
Applications of tannase
Clarification of orange juice
Fruit juices typically taste bitter, which is unpleasant due to the presence of plentiful tannins. Hence, clarification or detannification of fruit juices is essential for improving the quality and commercial value of these juices. Since tannase exhibits the potential to hydrolyze tannins, clarification of orange fruit juice was carried out using the purified enzyme. Orange juice was treated with different enzyme concentrations to obtain the optimum enzyme concentration. Tannase at a concentration of 4.5 U/ml was found to be most efficient in maximally decreasing the tannin content of orange juice after an initial incubation period of 2.5 h (Figure 2). The reaction mixture was allowed to incubate for 2.5 h at various temperatures to attain the optimum temperature. Maximum decline in tannin content was obtained at a temperature of 35°C (Figure 3), beyond which no further reduction was observed. For the maximum reduction of the tannin content, the orange juice was incubated for different intervals, keeping the enzyme concentration and temperature at the optimum values. The ideal incubation period was found to be 3.5 h. There was no further decline in tannin with a further increase in the incubation period. Figure 4 shows that under these optimum conditions of enzyme units (4.5 U/ml), temperature (35°C), and incubation time (3.5 h), the initial orange juice tannin content (1,060 μg/ml) was reduced to 43.46%. The optimum temperature obtained in this case lies well in the documented thermostability range of 30–50°C from K. pneumoniae tannase (Laemmli, 1970). Literature reveals that reports suggest the decline in the tannin content of various juices upon treatment with tannase. Rout and Banerjee (2006) observed a 25% decline in the tannin content of pomegranate juice upon treatment with tannase from Aspergillus foetidus and Rhizopus oryzae co-culture at 37°C for 2 h. Likewise, Srivastava and Kar (2009) also reported a 73.6 and 45.2% reduction in the tannin content of fresh amla (Phyllanthus emblica) juice by immobilized and soluble tannase from Aspergillus niger. Similar applications of tannase from Trichoderma harzianum MTCC 10841 and Aspergillus niger have been reported in pomegranate and guava juice, respectively (Kapoor and Iqbal, 2014; Sharma et al., 2014). However, all of these studies have been conducted on fungal tannase, whereas the present report is probably the first one suggesting the use of bacterial tannase for fruit juice clarification.
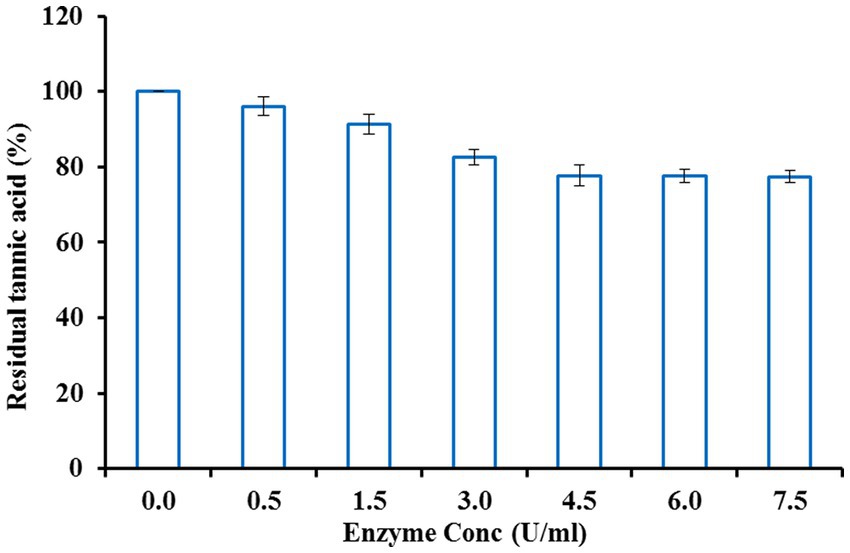
Figure 2. Effect of enzyme concentration on fruit juice detannification by tannase from Klebsiella pneumoniae.
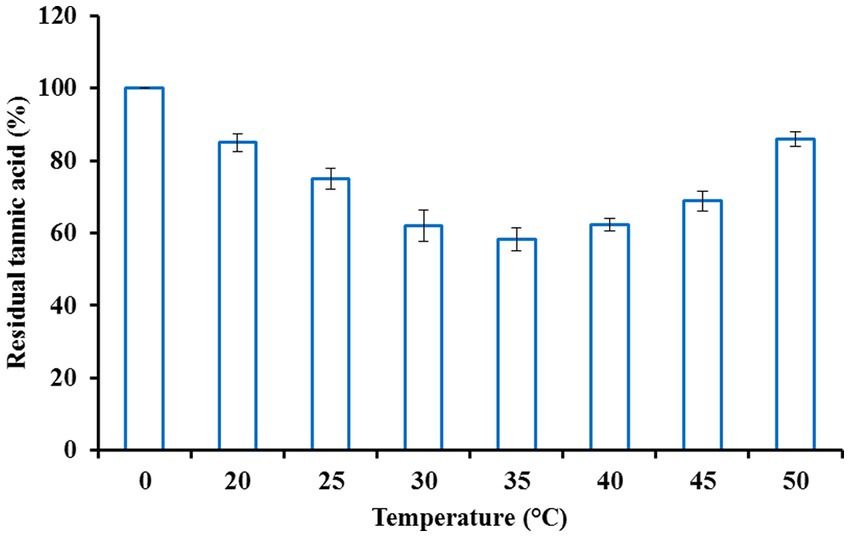
Figure 3. Effect of temperature on fruit juice detannification by tannase from Klebsiella pneumoniae.
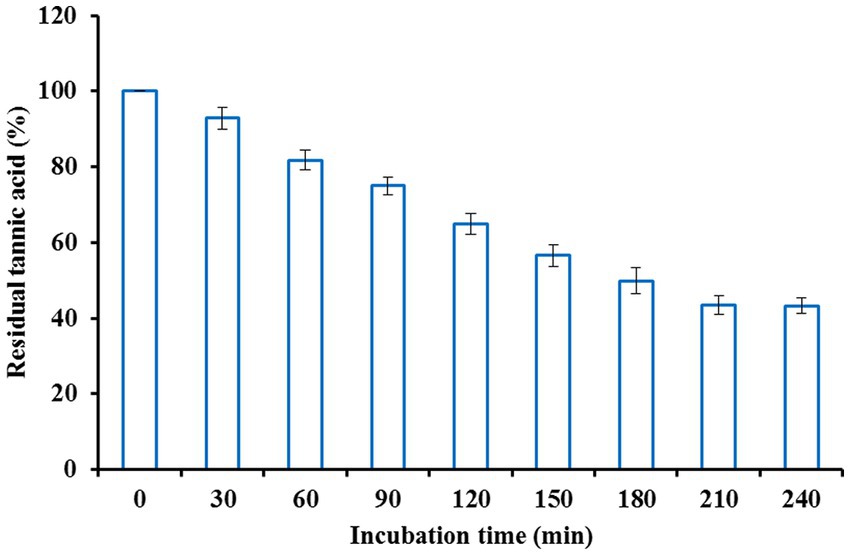
Figure 4. Effect of incubation time on fruit juice detannification by tannase from Klebsiella pneumoniae.
Clarification of beer
A high concentration of tannins in beer and other beverages induces sediment formation, haziness, and a bitter taste upon storage. Such kinds of problems could be circumvented by treatment with tannase. In the present study, beer haze clarification was achieved by treating colloidal beer suspension with purified tannase at optimized enzyme concentration, temperature, and incubation period conditions. The colloidal beer haze suspension was mixed with different enzyme concentrations ranging from 0.5–7.5 U/ml and incubated for 2.5 h. Tannase at a concentration of 4.5 U/ml was found to be most efficient in bringing the residual tannic acid to 71.51%. Further, an increase in enzyme dose beyond this level did not affect tannin content reduction (Figure 5). Thereafter, the enzyme (4.5 U/ml) and beer haze mixture was incubated at various temperatures ranging from 20 to 50°C. Maximum decomposition of tannin was observed at 30°C with a residual tannic acid of 59.29% (Figure 6). Incubation period was optimized by incubating the colloidal beer suspension for different time intervals keeping enzyme concentration (4.5 U/ml) and temperature (30°C) at their optimized values. After an incubation period of 3.5 h, the colloidal beer suspension became reasonably clear. The initial tannin content (425 μg/ml) was reduced to 46.47% (Figure 7). The current finding is in agreement with the earlier reports highlighting the significant reduction in tannin content of stored beer haze upon treatment with fungal tannase from Aspergillus flavus (Masschelein and Batum, 1981; Rossi et al., 1988; Giovanelli, 1989) These results exhibit the potential contribution of tannase in the hydrolysis of wort phenolics that are responsible for beer haze formation. However, there is a scarcity of reports suggesting the use of bacterial tannase to clarify beer haze.
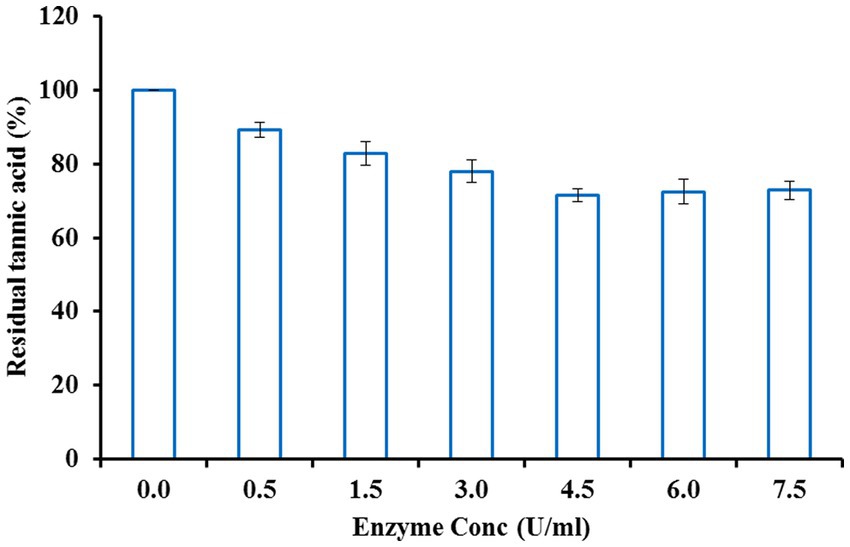
Figure 5. Effect of enzyme concentration on beer haze clarification by tannase from Klebsiella pneumoniae.
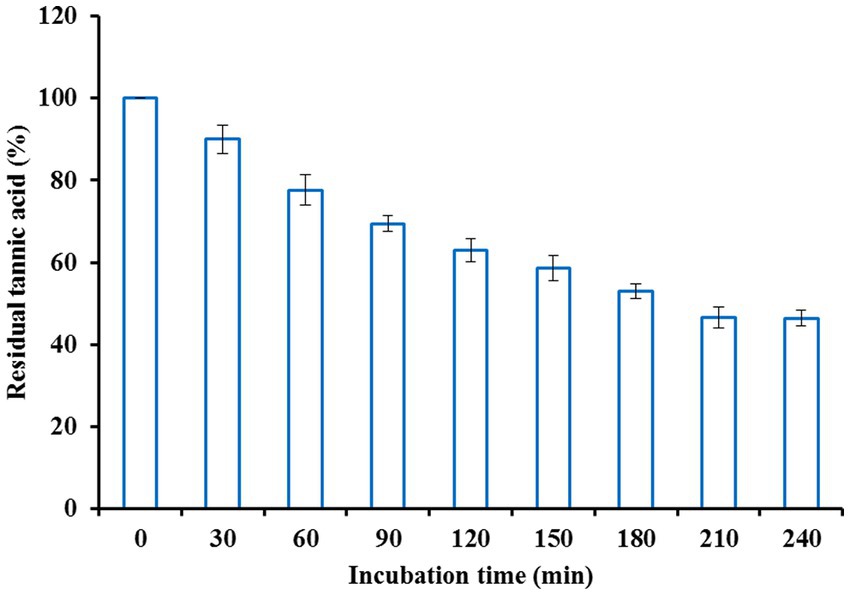
Figure 7. Effect of incubation time on beer haze clarification by tannase from Klebsiella pneumoniae.
Solubilization of tea cream
Because tea cream forms when instant tea is stored at or below 4°C, high cold water solubility is a significant issue with instant tea processing. The primary flavonoids found in caffeine and tea, including epicatechin, epicatechin gallate, epigallocatechin, and epigallocatechin gallate, as well as their condensation products, make up this haze formation. The hot water extract of tea is heated to low temperatures while being stirred, and then the tea cream is centrifuged, according to a traditional method of making instant tea (Nagalakshmi et al., 1985). Typically, the tea cream is discarded, which results in a significant loss of the key flavorings (Coggon et al., 1975).
Given the above problems, tea cream solubilization was accomplished with purified tannase from Klebsiella pneumoniae. Tea cream solubilization was optimized concerning enzyme concentration, temperature, and incubation time. Tea extract was incubated with different enzyme concentrations varying from 0.5–7.5 U/ml. An increase in enzyme dose led to a decrease in the tannin content of the tea extract up to a concentration of 6 U/ml, beyond which no further decline was observed in tannin content (Figure 8). Incubation was done at different temperatures ranging from 20 to 50°C and maximum reduction in tannin content was observed at 35°C with residual tannic acid of 63.82% (Figure 9). For maximizing the tannin reduction, tea extract was incubated for different time intervals while keeping the enzyme concentration and temperature at their optimum values. After 3 h of incubation, tea cream was dissolved, and the initial tannin content (94 μg/g) was reduced to 55%. No further reduction in tannin content was observed with a further increase in the incubation period (Figure 10). Our results are following Selwal et al. (2011) who treated tea extract with the partially purified tannase from Penicillium atramentosum KM at 35°C and observed a 74% reduction in the tannin content after 3 h of the incubation period. Yao et al. (2014) also reported a significant reduction in the tannins from green tea infusion through immobilized tannase from the metagenomic library. In this regard, Sanderson et al. (1977) have a patent for preparing cold-water-soluble leaf tea extract with good color, yield, and flavor through tannase. However, to the best of our knowledge, none of the reports have suggested so much reduction in the tannin content of tea cream as was obtained in the present study.
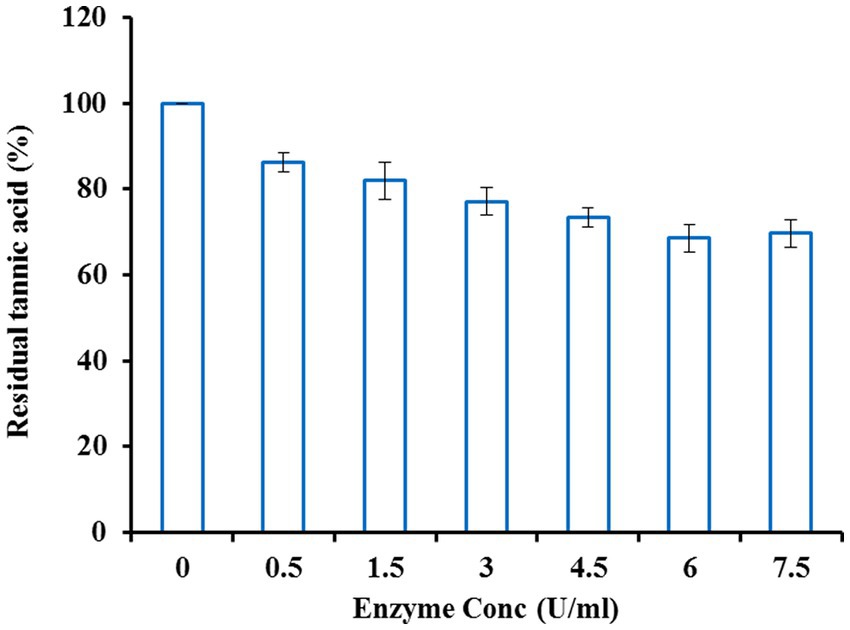
Figure 8. Effect of enzyme concentration on tea solubilization by tannase from Klebsiella pneumoniae.
Conclusion
Fruit juices and other beverages have important dietary implications. The bitterness and haziness are the most critical limiting factors causing major problems to the fruit juice and beverage industries. In this regard, the literature reveals the use of enzymes, particularly tannase, from several fungal sources as a remedial measure. However, given the problems associated with the fungi, as discussed in the introduction part, we have attempted, probably for the first time to report the potential applications of purified bacterial tannase in the clarification and detannification of fruit juice, beer, and tea cream solubilization. A significant reduction in the tannic acid content of orange juice, beer, and tea cream was observed, suggesting the possible use of this tannase in the quality improvement of fruit juices and beverages. Moreover, the low molecular mass of the present tannase also makes it a better candidate for cloning, overexpression, and purification as compared to fungal tannase. Such desirable properties of the bacterial tannase might be useful in the downstream processing of various fruit juices and beverages on an industrial scale.
Author’s note
The quality of various fruit juices and beverages deteriorates due to the high tannin content. The current study provides a biological way to overcome this problem by treating these food products with the bacterial enzyme tannase. This enzyme removes the tannins responsible for bitterness and improves the quality of juices and other beverages.
Data availability statement
Publicly available datasets were analyzed in this study. This data can be found here: https://www.ncbi.nlm.nih.gov/−, KP715242.
Author contributions
All authors listed have made a substantial, direct, and intellectual contribution to the work and approved it for publication.
Acknowledgments
The authors thank the Chairperson, Department of Biotechnology, Chaudhary Devi Lal University, Sirsa, Haryana, India, for providing the necessary laboratory facilities to carry out this work.
Conflict of interest
The authors declare that the research was conducted in the absence of any commercial or financial relationships that could be construed as a potential conflict of interest.
Publisher’s note
All claims expressed in this article are solely those of the authors and do not necessarily represent those of their affiliated organizations, or those of the publisher, the editors and the reviewers. Any product that may be evaluated in this article, or claim that may be made by its manufacturer, is not guaranteed or endorsed by the publisher.
References
Aguilar, C. N., and Gutierrez-Sanchez, G. (2001). Review: sources properties, applications and potential uses of tannin acyl hydrolase. Int. J. Food Sci. Technol. 7, 373–382. doi: 10.1106/69M3-B30K-CF7Q-RJ5G
Aracri, F. M., Cavalcanti, R. M. F., and Guimaraes, L. H. S. (2019). Extracellular tannase from Aspergillus ochraceus: influence of the culture conditions on biofilm formation, enzyme production, and application. J. Microbiol. Biotechnol. 29, 1749–1759. doi: 10.4014/jmb.1903.03060
Beniwal, V., Kumar, A., and Goel, G. (2013). Chhokar V (2013) a novel low molecular weight acido-thermophilic tannase from Enterobacter cloacae MTCC 9125. Biocatal. Agric. Biotechnol. 2, 132–137. doi: 10.1016/j.bcab.2013.03.002
Catelani, T. A., Bittar, D. B., Pezza, L., and Pezza, H. R. (2016). A rapid and eco-friendly method for determination of hydrolysable tannins and its application to honey samples. Food Anal. Methods 9, 2552–2559. doi: 10.1007/s12161-016-0454-1
Cavalcanti, R. M. F., Martinez, M. L. L., Oliveira, W. P., and Guimaraes, L. H. S. (2020). Stabilization and application of spray-dried tannase from Aspergillus fumigatus CAS21 in the presence of different carriers. 3 Biotech 10:177. doi: 10.1007/s13205-020-2164-z
Chhokar, V., Sangwan, M., Beniwal, V., Nehra, K., and Nehra, K. S. (2010). Effect of additives on the activity of tannase from Aspergillus awamori MTCC 9299. Appl. Biochem. Biotechnol. 160, 2256–2264. doi: 10.1007/s12010-009-8813-7
Dhiman, S., Mukherjee, G., and Singh, A. K. (2018). Recent trends and advancements in microbial tannase-catalyzed biotransformation of tannins: a review. Int. Microbiol. 21, 175–195. doi: 10.1007/s10123-018-0027-9
Giovanelli, G. (1989). Enzymatic treatment of malt polyphenols for stabilization. Ind. Bevande 18, 497–502.
Hagerman, A. E., and Butler, L. G. (1978). Protein precipitation method for the quantitative determination of tannins. J. Agric. Food Chem. 26, 809–812. doi: 10.1021/jf60218a027
Jana, A., Halder, S. K., Banerjee, A., Paul, T., Pati, B. R., Mondal, K. C., et al. (2014). Biosynthesis, structural architecture and biotechnological potential of bacterial tannase: a molecular advancement. Bioresour. Technol. 157, 327–340. doi: 10.1016/j.biortech.2014.02.017
Kanpiengjai, A., Khanongnuch, C., Lumyong, S., Haltrich, D., Nguyen, T. H., and Kittibunchakul, S. (2020). Co-production of gallic acid and a novel cell-associated tannase by a pigment-producing yeast, Sporidiobolus ruineniae A45.2. L. Microb. Cell Factories 19:95. doi: 10.1186/s12934-020-01353-w
Kapoor, A., and Iqbal, H. (2014). Efficiency of tannase produced by Trichoderma harzianum MTCC 10841 in pomegranate juice clarification and natural tannin degradation. Int. J. Biotechnol. Bioeng. Res. 4, 641–650.
Kumar, M., Beniwal, V., and Salar, R. K. (2015a). Purification and characterization of a thermophilic tannase from Klebsiella pneumoniae KP715242. Biocatal. Agric. Biotechnol. 4, 745–751. doi: 10.1016/j.bcab.2015.10.011
Kumar, M., Rana, S., Beniwal, V., and Salar, R. K. (2015b). Optimization of tannase production by a novel Klebsiella pneumoniae KP715242 using central composite design. Biotechnol. Rep. 7, 128–134. doi: 10.1016/j.btre.2015.06.002
Kumar, M., Singh, A., Beniwal, V., and Salar, R. K. (2016). Improved production of tannase by Klebsiella pneumoniae using Indian gooseberry leaves under submerged fermentation using Taguchi approach. AMB Express 6:46. doi: 10.1186/s13568-016-0217-9
Laemmli, U. K. (1970). Cleavage of structural proteins during the assembly of the head of bacteriophage T4. Nature 227, 680–685. doi: 10.1038/227680a0
Lekha, P. K., and Lonsane, B. K. (1997). Production and application of tannin acyl hydralose: state of the art. Adv. Appl. Microbiol. 44, 215–260. doi: 10.1016/S0065-2164(08)70463-5
Lekha, P., Ramakrishna, M., and Lonsane, B. (1993). Strategies for isolation of potent culture capable of producing tannin acyl hydrolase in higher titers. Chem. Mikrobiol. Technol. Lebensm. 15, 5–10.
Masschelein, C. A., and Batum, M. S. (1981) Enzyme degradation and participation of ester linked beer polyphenols in chill haze formation. Proc Cong Eur Brew Conv 359-370.
Mondal, K. C., Banerjee, D., Jana, M., and Pati, B. R. (2001). Colorimetric assay for determination of tannin acyl hydrolase (E.C.3.1.1.20) activity. Anal. Biochem. 295, 168–171. doi: 10.1006/abio.2001.5185
Mostafa, H. S. (2023). Production of low-tannin Hibiscus sabdariffa tea through D-optimal design optimization of the preparation conditions and the catalytic action of new tannase. Food Chem. 17:100562. doi: 10.1016/j.fochx.2023.100562
Nagalakshmi, S., Jayalakshmi, R., and Seshadri, R. (1985). Approaches to decreaming of black tea infusions by solvent decaffeination and tannase treatment. J. Food Sci. Technol. 22, 198–201.
Nishitani, Y., and Osawa, R. (2006). Involvement of tannase in the acquisition of manganese from tannin-rich medium by tannase-producing Lactobacillus plantarum. Jpn. J. Lactic Acid Bact. 17, 125–131. doi: 10.4109/jslab.17.125
Osawa, R., Kuroiso, K., Goto, S., and Shimizu, A. (2000). Isolation of tannin-degrading Lactobacilli from humans and fermented foods. Appl. Environ. Microbiol. 66, 3093–3097. doi: 10.1128/AEM.66.7.3093-3097.2000
Raveendran, S., Parameswaran, B., and Madhavah, A. (2018). Applications of microbial enzymes in food industry. Food Technol. Biotechnol. 56, 16–30. doi: 10.17113/ftb.56.01.18.5491
Ren, B., Wu, M., Wang, Q., Peng, X., Wen, H., Mckinstry, W. J., et al. (2013). Crystal structure of tannase from Lactobacillus plantarum. J. Mol. Biol. 425, 2737–2751. doi: 10.1016/j.jmb.2013.04.032
Rossi, M., Glovanelli, G., Cantarelli, C., and Brenna, O. (1988) Bulletins Liaision Groupe Polyphenols 14: 85–88.
Rout, S., and Banerjee, R. (2006). Production of tannase under mSSF and its application in fruit juice debittering. Ind. J. Biotechnol. 5, 351–356.
Sanderson, G. W., Hoefler, A. C., Graham, H. N., and Coggon, P. (1977) Cold water extractable tea leaf and process. US 4051264.
Singh, N., Jiwani, G., Rocha, L. S., and Mazaheri, R. (2023) “Bioagents and Volatile Organic Compounds: An Emerging Control Measures for Rice Bacterial Diseases,” in Bacterial Diseases of Rice and Their Management. Apple Academic Press. 255–274.
Singh, N., Mansoori, A., Jiwani, G., Solanke, A. U., Kumar, R., and Kumar, A. (2021). Evaluation of antioxidant and antimicrobial potential of thespesia lampas ROOT EXTRACTS. JEBAS 9, 87–99. doi: 10.18006/2021.9(1).87.99
Singh, N., Pandey, R., Chandraker, S. K., Pandey, S., Malik, S., and Patel, D. (2022). “Use of wild edible plants can meet the needs of future generation,” in Agro-biodiversity and Agri-ecosystem Management. Singapore: Springer Nature Singapore. 341–366.
Singh, A., Singh, N., Singh, S., Srivastava, R. P., Singh, L., Verma, P. C., et al. (2023). The industrially important genus Kaempferia: An ethnopharmacological review. Front. Pharmacol. 14:1099523. doi: 10.3389/fphar.2023.1099523
Selwal, M. K., Yadav, A., Selwal, K. K., Aggarwal, N. K., Gupta, R., and Gautam, S. K. (2010). Optimization of cultural conditions for tannase production by Pseudomonas aeruginosa IIIB 8914 under submerged fermentation. World J. Microbiol. Biotechnol. 26, 599–605. doi: 10.1007/s11274-009-0209-x
Selwal, M. K., Yadav, A., Selwal, K. K., Aggarwal, N. K., Gupta, R., and Gautam, S. K. (2011). Tannase production by Penicillium atramentosum KM under SSF and its applications in wine clarification and tea cream solubilization. Braz. J. Microbiol. 42, 374–387. doi: 10.1590/S1517-83822011000100047
Sharma, N. K., Beniwal, V., Kumar, N., Kumar, S., Pathera, A. K., and Ray, A. (2014). Production of tannase under solid-state fermentation and its application in detannification of guava juice. Prep. Biochem. Biotechnol. 44, 281–290. doi: 10.1080/10826068.2013.812566
Srivastava, A., and Kar, R. (2009). Characterization and application of tannase produced by Aspergillus Niger ITCC 6514.07 on pomegranate rind. Braz. J. Microbiol. 40, 782–789. doi: 10.1590/S1517-83822009000400008
Wilhelmy, C., Pavez, C., Bordeu, E., and Brossard, N. (2021). A review of tannin determination methods using spectrophotometric detection in red wines and their ability to predict astringency. South Afr. J. Enol. Vis. 42. doi: 10.21548/42-1-3852
Keywords: Detannification, fruit juice, Klebsiella pneumoniae, tannic acid, tea cream solubilization
Citation: Kumar M, Mehra R, Yogi R, Singh N, Salar RK, Saxena G and Rustagi S (2023) A novel tannase from Klebsiella pneumoniae KP715242 reduces haze and improves the quality of fruit juice and beverages through detannification. Front. Sustain. Food Syst. 7:1173611. doi: 10.3389/fsufs.2023.1173611
Edited by:
Mireille Fouillaud, Universitéde la Réunion, FranceReviewed by:
Vikas Beniwal, Maharishi Markandeshwar University, Mullana, IndiaJitender Kumar, Central University of Himachal Pradesh, India
Copyright © 2023 Kumar, Mehra, Yogi, Singh, Salar, Saxena and Rustagi. This is an open-access article distributed under the terms of the Creative Commons Attribution License (CC BY). The use, distribution or reproduction in other forums is permitted, provided the original author(s) and the copyright owner(s) are credited and that the original publication in this journal is cited, in accordance with accepted academic practice. No use, distribution or reproduction is permitted which does not comply with these terms.
*Correspondence: Rajesh Yogi, cmFqZXNoeW9naTk5OUBnbWFpbC5jb20=