- 1ICAR-Central Coastal Agricultural Research Institute, Old Goa, Goa, India
- 2AICRP on Castor, University of Agricultural Sciences, Bengaluru, Karnataka, India
- 3ICAR-Directorate of Groundnut Research, Ananthapur, Andhra Pradesh, India
- 4ICAR-National Institute of Veterinary Epidemiology and Disease Informatics, Bengaluru, India
- 5Department of Ecology and Environmental Science, Assam University, Silchar, India
- 6ICAR-Indian Institute of Soil and Water Conservation, Vasad, Gujarat, India
Recently, most agrarian countries have witnessed either declining or stagnant crop yields. Inadequate soil organic matter (SOM) due to the poor physical, chemical, and biological properties of the soil leads to an overall decline in the productivity of farmlands. Therefore, the adoption of integrated nutrient management (INM) practices is vital to revive sustainable soil health without compromising yield potential. Integrated nutrient management is a modified nutrient management technique with multifarious benefits, wherein a combination of all possible sources of plant nutrients is used in a crop nutrition package. Several studies conducted in various parts of the world have demonstrated the benefits of INM in terms of steep gain in soil health and crop yields and at the same time, reducing greenhouse gas emissions and other related problems. The INM practice in the cropped fields showed a 1,355% reduction in methane over conventional nutrient management. The increase in crop yields due to the adoption of INM over conventional nutrient management was as high as 1.3% to 66.5% across the major cropping systems. Owing to the integration of organic manure and residue retention in INM, there is a possibility of significant improvement in soil aggregates and microbiota. Furthermore, most studies conducted to determine the impact of INM on soil health indicated a significant increase in overall soil health, with lower bulk density, higher porosity, and water-holding capacity. Overall, practicing INM would enhance soil health and crop productivity, in addition to decreasing environmental pollution, greenhouse gas emissions, and production costs.
1. Introduction
The increasing human population and their consequent need for food, combined with the depletion of healthy soil, have led to unprecedented damage to natural resources, making it difficult to meet the global demand for food. In the developing world, achieving food security through sustainable systems is a big task, yet it is vital for poverty alleviation. To get around this problem, farmers have resorted to overusing specific inputs like chemical fertilizers and pesticides, which have already begun to harm the ecosystem. During the initial days of fertilizer usage, the impact of crop fertilization with inorganic fertilizers has been prominent in world agriculture (Hossain and Singh, 2000). In most countries of agrarian background, manufacturing and service of chemical fertilizers have been practiced as prime agenda in securing nations’ food and nutritional security. India, a populous agrarian country, is the world’s third-largest producer and consumer of chemical fertilizers (Tandon and Tiwari, 2007). As per recent reports, the Indian fertilizer market reached a value of Rs. 887 billion and is expected to grow at a compound annual growth rate of 5.5% by 2026 [IFA (International Fertilizer Association), 2020]. Food production must increase significantly while agriculture’s environmental impact must decrease greatly to fulfill the world’s future food security and sustainability needs (Foley et al., 2011).
India is a country with diverse cropping practices. The nutrient mining by various crops and cropping systems is far higher than the nutrient additions annually through fertilizers (Kumar et al., 2015). For the past 40 years, a nutrient gap of 8 to 10 million tons of nitrogen (N), phosphorus (P), and potash (K) per year have been documented (Tandon, 2004). This condition is analogous to depleting the soil’s nutritional reserve. Long-term negative impacts of imbalance and indiscriminate use of inorganic fertilizers, particularly NPK-based formulations, have irreversibly damaged the soil resource base of many agroecosystems (Prasad et al., 2002; Singh et al., 2014). Despite the tremendous improvement in crop productivity in numerous crops due to improved varieties and increased use of agrochemicals, the goal of ensuring food and nutritional security remains challenging (Nath et al., 2018). The ever-increasing food demands of the burgeoning population have continuously exerted pressure on the agroecosystems. In the process of increasing agricultural production, the agriculture production units significantly contribute to environmental pollution (Wheeler and von Braun, 2013; Wu and Ma, 2015). For future generations, the goal is no longer to enhance agricultural production but also to optimize nutrients, energy, and water usage while minimizing environmental impact. Over-exploitation of nutrients from the soil and poor nutrient loss replenishment, depleted nutrients from the soil are often unable to be replenished by artificial crop fertilization, resulting in an imbalance in the soil nutrients pool (Paramesh et al., 2013a,b, 2014, 2020). Hence, the huge increase in global greenhouse gas (GHG) emissions by the agriculture sector, primarily due to the use of synthetic fertilizers and pesticides in recent years, both of which are rapidly expanding. One of the primary causes of environmental pollution, such as eutrophication and GHG emissions is the injudicious use of fertilizer, particularly nitrogen (Davidson et al., 2014). Thus, it is high time to search for innovative practices that guarantee higher yields with the least amount of additional environmental damage possible, especially for developing countries.
No single source of plant nutrients, such as chemical fertilizers, organic manures, crop residues, and bio-fertilizers, can meet the entire nutrient need of a crop in today’s intensive agriculture systems (Mahajan and Gupta, 2009). In this context, the integration of all possible sources of nutrients is ideal for enhancing the soil resource base besides contributing to crop productivity (Nath et al., 2018). Findings of various studies (Selim and Al-Owied, 2017; Selim, 2018; Wang et al., 2019; Song et al., 2020) suggest integrated nutrient management (INM) is a tool that can offer good options and economic choices to supply macro and micronutrients of plants and also contribute to reducing the dependence on externally purchased chemical fertilizers besides protecting soil health. Physical properties related to soil structure, are greatly influenced by adding organic manures (Das et al., 2014). An increasing number of research have suggested that INM has an impact on crop production, soil quality, and the environment while balancing food security and GHG mitigation. Numerous earlier studies largely examined how INM affected crop productivity, soil quality, or environmental performance. It is currently unknown how INM will affect crop productivity, soil bulk density, microbial biomass carbon, and the environment as a whole. This review addresses the synergistic effects of different nutrient sources and their combinations. This includes exploring the interactions between organic and inorganic fertilizers, biofertilizers, and crop residues on crop productivity and soil quality. By understanding how different nutrient sources interact, the researcher can develop more effective nutrient management strategies that optimize nutrient use efficiency and minimize environmental impacts. Further, this review highlights the long-term impacts of INM on soil quality parameters such as SMBC, bulk density, and dehydrogenase activity. This review article helps in understanding how INM affects soil quality, productivity, and environment. The researchers can develop more sustainable soil management practices that protect and improve soil health, and can develop more effective fertilizer recommendations and minimize nutrient losses. Finally, we can help farmers and policymakers make informed decisions about nutrient management.
2. Fertilizer consumption in India and the need for INM
Global use of inorganic fertilizers have increased almost fivefold since 1960 and have significantly supported population growth (FAO, 2017). The present world’s demography will change in many folds in the near future and will require the production of 70% extra food, fodder and fuel to meet the demands of the ever-growing population (FAO, 2017). On the contrary, the natural base resource required for additional food production is shrinking. A study conducted by FAO (2017) indicated that the growth of agriculture between 1960 and 2015 exhibited about a 28% increase in the production of 174 crops cultivated all over the world. This phenomenal gain in production over the past few decades has not only tripled production but also paved the way for land degradation by hampering the soil’s physical, chemical, and biological resource base (Power, 2013). High-input-driven and resource-intensive farming practices have caused massive and irreparable damage to soil and water resource in the agro-ecosystem and deteriorations in soil health besides contributing enormously to GHG emissions. Among agricultural practices, nutrient management is one such practice that plays a critical role in crop productivity and soil health. Additionally, after the green revolution, an over-reliance on fertilizers led to a decline in the efficiency of nutrient use and an increase in GHG emissions. The use of fertilizers can have implications for the accumulation of heavy metals in the soil and plants, which can subsequently enter the food chain, leading to potential pollution of water, soil, and air. Some mineral/chemical fertilizers contain low quantities of heavy metals and radionuclides, and their excessive application in agriculture can pose environmental problems. For example, the excessive use of urea has been identified as a major contributor to increased nitrate (NO3−) levels in drinking water and river systems. Studies in agricultural districts of Srikakulam in Andhra Pradesh, located in the Vamsadhara river basin, revealed elevated nitrate concentrations ranging from traces to 450 mg NO3−/L of water, particularly following fertilizer applications (Rao, 2006).
The current consequences of nitrate pollution in freshwater bodies in India reflect the detrimental effects of historical and ongoing excessive use of fertilizers and manures. The transport of phosphatic fertilizers through surface water flow can also contribute to increased phosphate content in drinking water and rivers. The rising levels of dissolved N and P loads are primarily associated with increased losses from agricultural and sewage systems. Moreover, the excessive application of nitrogen fertilizers has implications for GHG emissions. The relative contribution of fertilizer nitrogen application to total GHG emissions has increased from 8% in 1970 to 23% in 2010. NH3 volatilization from rice fields is higher when N fertilizers are surface-broadcasted, leading to reduced nitrogen use efficiency (Ladha et al., 2005). Additionally, excessive N fertilizer application can negatively affect soil health by degrading soil carbon and affecting the structure and function of soil biological communities. Long-term application of N fertilizer alone has been shown to significantly reduce soil pH at various experimental sites in India.
Further, phenomenal loss in nutrient use efficiency of various crops and cropping systems has gradually encouraged farmers to apply higher doses of nutrients (Figure 1). As a result, this injudicious practice of nutrient management has paved the way to impair soil health due to less or no use of organic manure, residue retention, and bioinoculant-mediated fertilizer management. This has strongly distracted the complementarity between bio-geo cycles of the agroecosystem and is the main reason for multiple nutrient deficiencies, declining fertilizer response, and crop productivity. To address the current challenges associated with excessive fertilizer use, including the potential accumulation of heavy metals, pollution of water sources, GHG emissions, and adverse effects on soil health. Implementing sustainable nutrient management practices that optimize fertilizer use, minimize losses, and protect both the environment and human health is imperative. Hence, INM is necessary to bring back harmony in the agroecosystem besides sustaining the productivity of crop and soil health.
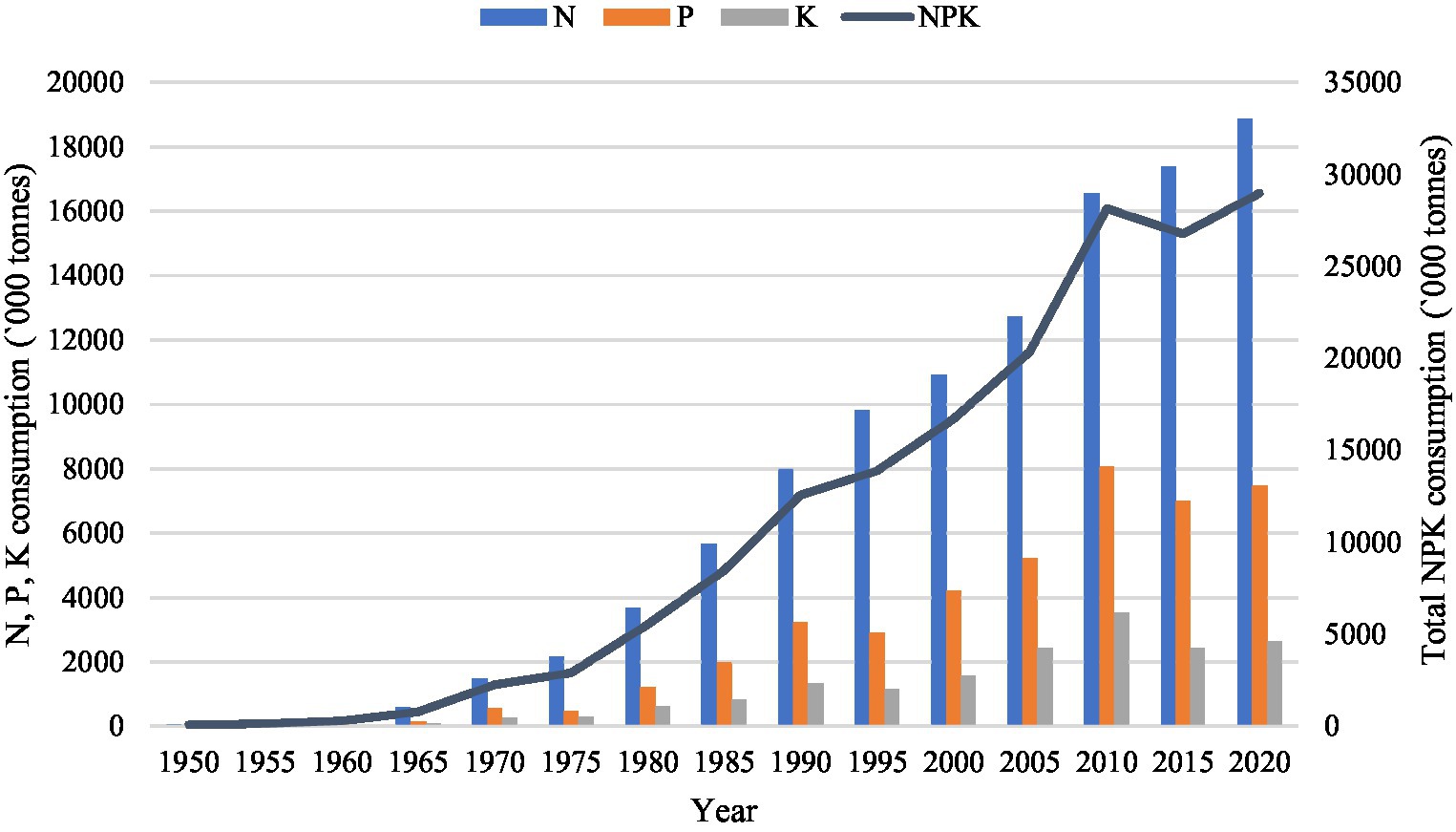
Figure 1. Annual fertilizer consumption in India (Fertilizer Association of India, 2021).
The INM primarily refers to the judicious, efficient, and integrated use of all available sources of organic, inorganic, and biological components to combine traditional and modern techniques of nutrient management into an environmentally sound and economically optimal agricultural system (Janssen, 1993). To synchronize nutrient demand by the crop and its release in the environment, it optimizes all elements of the nutrient cycle, including N, P, K, and other macro- and micronutrient inputs and outputs. The INM techniques reduce losses due to leaching, runoff, volatilization, emissions, and immobilization while maximizing nutrient use efficiency (Zhang et al., 2012). Additionally, INM aims to improve the physical, chemical, biological, and hydrological aspects of the soil to increase agricultural production and reduce land degradation (Janssen, 1993; Esilaba et al., 2005). There is now a deeper understanding that INM may concurrently and very invisibly protect soil resources while also increasing crop yield. Farmyard manures, farm wastes, soil amendments, crop residues, chemical fertilizers, green manures, cover crops, intercropping, crop rotations, fallows, conservation tillage, irrigation, and drainage are all used in its methods to increase plant nutrition and preserve water (Janssen, 1993). The INM practice also promotes methods designed to reduce nutrient losses and enhance plant uptakes, such as the deep placement of fertilizers and the use of inhibitors or urea coatings (Zhang et al., 2012). Instead of merely concentrating on yield-scaled profit, these techniques urge farmers to focus on long-term planning and offer scope for the reduction of environmental impact.
2.1. Nutrient mobilization/mineralization in soil under INM practice
Farmers generally apply significant amounts of N fertilizer at the time of sowing or planting under conventional practice. Typically, 80% of the total N fertilizer is applied as a basal dressing, and the remaining N is applied within the first 10 days after transplanting for rice or within the first 30-days after the date of seeding for wheat, maize, and sorghum (Peng et al., 2002; Chen et al., 2011). Due to the inefficient synchronization between the soil’s supply and crop demand caused by this N application pattern, a significant amount of inorganic N is available in the soil before it is needed by rapidly growing crops (Chen et al., 2006). However, a material with a high N content (fertilizers) favors net mineralization, whereas one with a low N concentration (manures) results in net immobilization. Interestingly, Reddy et al. (2008) stated that organic manures (farmyard manure-FYM) with a C:N ratio of 29% to 33% immobilized N during the initial 40 days of incubation. This caused net N mineralization to begin during the first week of incubation, and then urea fertilizer has been added to lower the C:N ratio to 18%–22%. Therefore, a rapid increase in the mineral N near the end of incubation was the result of the combined application of fertilizer with FYM. The combined usage of organic manures with that of chemical fertilizer assures the continuous supply of nutrients by acting as a multi-nutrient pool, further during organic matter mineralization nutrients release slowly and gradually so that the crop enjoys the soil nutrient pool throughout its requirement. Consequently, the continual application of inorganic fertilizers in combination with FYM or lime, significantly altered soil microbial biomass carbon, soil N and P, fulvic acid (FA), and humic acid (HA; Srinivasarao et al., 2020). In addition, integrated use of fertilizer, manure, and lime application in soybean-wheat rotation led to improvements in soil water retention, soil aggregates, microporosity, and water holding capacity as well as a decrease in the soil’s bulk density (BD) in the top 30 cm of the soil when compared to fertilizer application alone (Hati et al., 2008). Thus, the use of organic manures enhances fertilizer use efficiency and serves as an alternative source of nutrients (Dwivedi et al., 2016). The INM system synchronizes the nutrient demand set by plants, both in time and space, with the supply of nutrients from the labile soil pool and applied nutrient sources (Cassman et al., 2002). As INM encourages the split application of N fertilizers during critical stages of crop growth in small quantities it has the potential to increase crop productivity and quality and also reduce nutrient losses (Tilman et al., 2002; Witt and Dobermann, 2004).
2.2. Data collection and rice equivalent yield
The key data collected from the publications that qualified for review included grain yield, soil bulk density (BD), SOC, soil microbial biomass carbon (SMBC), dehydrogenase (DHA), GHG emission. In this analysis, change (δ) in measured variables between the conventional and INM practice was expressed as a percentage, i.e., δ = 100*(INM − Conventional)/Conventional because of its ease of interpretation. To compare different monocrops and cropping systems, rice equivalent yield (REY; equation 1) was determined by converting the economic yield of different crops on the basis of their marketable price prevailing during the period for each crop, including rice, and expressed in ton per unit area.
3. Effect of INM on crop productivity, soil bulk density, microbial biomass carbon, and reducing environmental impact
3.1. Crop yield
Yield is the utmost concern in agriculture production systems. To achieve a desirable yield, a plant nutrient management system is one of the prime agro-practices. The impact of integrated nutrient management on rice equivalent yield was synthesized by taking into consideration of yield obtained under INM-treated plots against the conventional nutrient management of various crops and cropping systems and presented in Table 1. The results of rice equivalent yield indicated the gain in productivity of most of the cropping systems. The increment in crop yields under INM over conventional nutrient management across the cropping system studied was 1.3% to 66.5%. Furthermore, significant improvement in crop yields was more noticeable in field crops, especially rice, wheat, and soybean. However, vegetable crops like okra, tomato, and onion crops also demonstrated increased yields under INM. A long-term field experiment in India from 1973 to 2004, observed a substantial decrease trend in soybean yield for both the control and NPK treatments whereas the NPK + FYM treatment had a statistically significant (p < 0.05) increase in yield over time (Bhattacharyya et al., 2008). Increased microbial activity, better supply of macro- and micronutrients like S, Zn, C, and B, which are not supplied by NPK (straight) fertilizers, and lower nutrient losses from the soil are some of the additional benefits of organic matter over N, P, and K supply that may have contributed to the higher yields of soybean and wheat obtained with the FYM + NPK treatment. Therefore, INM system enhances the yield potential of crops over and above achievable with recommended fertilizers. In the future, there are several promising areas of work that can be explored in INM to further enhance crop yield. One avenue is the development and utilization of precision nutrient management techniques. As precision nutrient management uses advanced technologies such as remote sensing, geospatial analysis, and machine learning algorithms to assess crop nutrient requirements at a fine-scale level and deliver precise and site-specific nutrient applications. This approach minimizes nutrient loss and ensures that crops receive nutrients when and where they are most needed. Another important issue to be addressed is the exploration of microbial interventions in nutrient management. Microbial interventions in nutrient management use beneficial microorganisms like mycorrhizal fungi, rhizobacteria, and other plant growth-promoting microbes to improve nutrient availability and uptake efficiency, and enhance crop productivity. Scope of INM should be extended to the use cover crops and crop rotations to build soil fertility, enhance nutrient cycling, and crop yield.
3.2. Greenhouse gas emission
Globally, agriculture and its associated systems are viewed as a massive contributor of GHG emissions (Ravikumar et al., 2021). To reduce its contribution, reduction in excess nutrient application and balanced application are the key mitigation strategies (Sapkota et al., 2021). Enhancing crop yields by application of plant nutrients become an ambiguous practice in most farmlands (Garnett et al., 2013). On the contrary, the imbalanced application of fertilizer in croplands is a major source of anthropogenic greenhouse gas emissions (Sutton et al., 2013). Therefore, to keep these anthropogenic GHG emissions under control, devising, and practicing proper fertilizer management is essential (Carlson et al., 2017). Of the several means of nutrient management, INM is proven better at minimizing GHG emissions. The work conducted by Nyamadzawo et al. (2017) in Zimbabwe in maize indicated practicing INM reduced about 17.1% GHGs over conventional nutrient management (Table 2). Another study in China demonstrated a significant (p < 0.05) reduction (1,268%) in GHG emissions against conventional nutrient management in the rice-wheat cropping system (Ma et al., 2010). Similarly, several studies conducted in India indicated a considerable reduction in GHG emissions (20%–1,355%) in rice, wheat, and mustard crops (Majumdar et al., 2002; Nyamadzawo et al., 2014; Sharma et al., 2019).
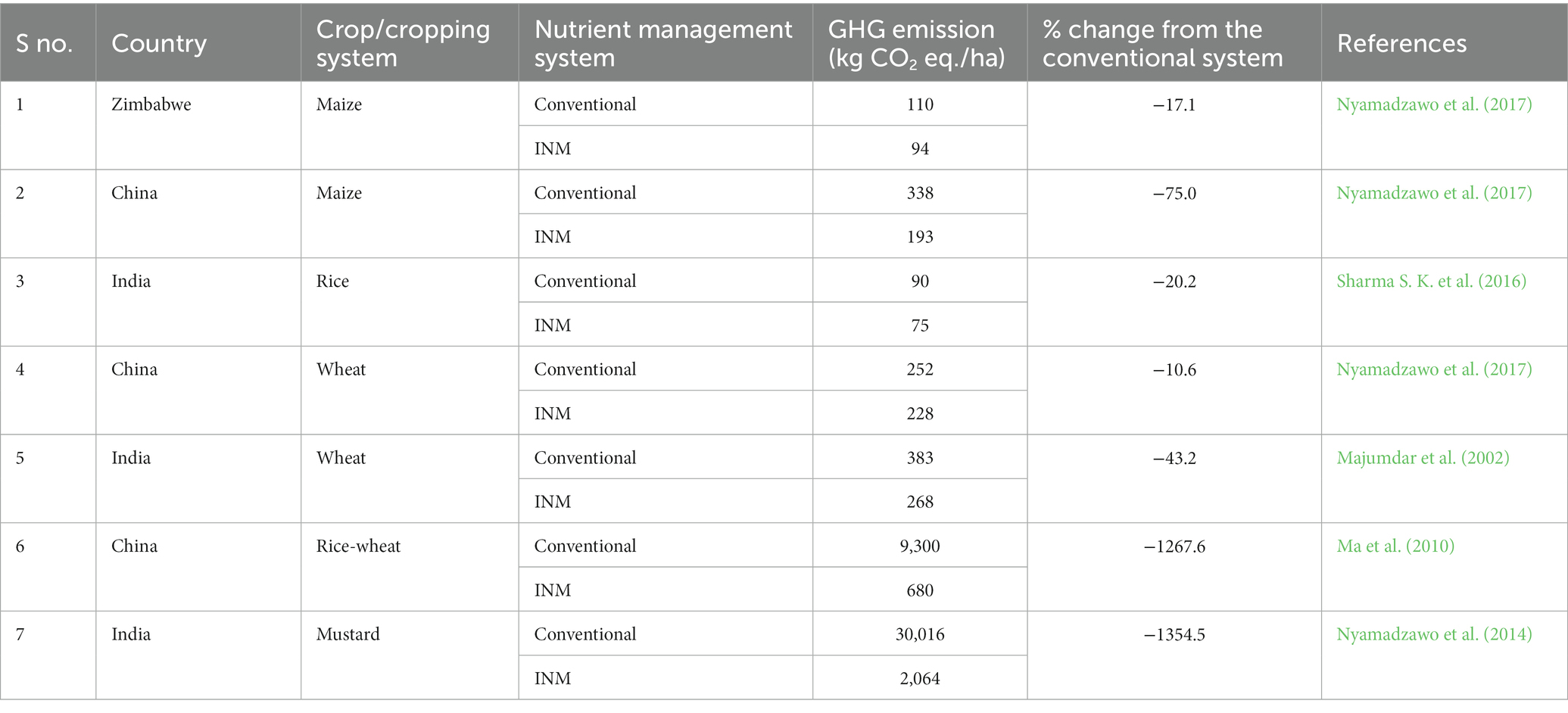
Table 2. Comparison of GHG emissions from the conventional and integrated nutrient management system.
Uncontrolled use of fertilizers increases emissions into the atmosphere and groundwater leaching of nutrients. The INM promotes high agricultural yields while reducing N losses and associated detrimental consequences on the environment (Gruhn et al., 2000). The ultimate fate of applied fertilizers is a combined effect of crop nutrient intake, immobilization, and soil residues, as well as nitrogen losses to the environment as ammonia volatilization, NOX emissions, denitrification, N leaching, and runoff (Wu and Ma, 2015). Additionally, the pattern of N application, crop features, soil characteristics, climate, and management approaches affect the efficacy of applied fertilizers. INM thus recommends deep urea placement, which can greatly boost N-use efficiency with low NH3 volatilization and reduces nitrate-leaching (Jambert et al., 1997). Because nitrification occurs mostly following fertilizer N application (Ma et al., 2010) and irrigation, the use of nitrification inhibitors can also minimize N2O emissions (Ju et al., 2011). Additionally, INM supports the use of organic nutrient sources since they provide both greater potential for agriculture’s sustainability and more immediate environmental advantages. Combining organic manure with other management techniques, such as incorporating agricultural residues and creating conservation tillage (such as no-till or reduced-tillage practices), can also help to lower GHG emissions, enhance soil quality, and boost carbon sequestration (Huang and Sun, 2006).
Reducing environmental pollution can be achieved by developing precision nutrient management techniques, promoting nutrient recycling and reuse strategies, exploring the use of cover crops and diversified cropping systems. Precision nutrient management techniques minimize nutrient losses to the environment by refining nutrient application methods, such as incorporating controlled-release fertilizers or using site-specific technologies to target nutrient placement. In INM, nutrient recycling and reuse strategies efficiently capture and recycle nutrients from various sources, such as agricultural residues, livestock manure, and wastewater. Practices like anaerobic digestion, composting, and biochar production can be used to convert these nutrient-rich materials into valuable organic amendments. Intercropping and crop rotation can also be made an integral part of INM systems that incorporate nitrogen-fixing crops can also reduce the need for synthetic nitrogen fertilizers and decrease nitrogen runoff.
3.3. Carbon sequestration
Soil OM is the most important indicator of soil fertility, quality, and productivity, and is usually estimated by determining SOC (Rasmussen et al., 1998). It is generally believed that fertilizer application increases residues, including roots, returned to the soil, and as a result, can increase SOM content and C sequestration (Lu et al., 2009; Paustian et al., 2019). The INM through green manuring, crop residue incorporation and other animal-based manures has a profound influence on soil carbon stock. From their study, Sujata et al. (2007) revealed application of inorganic fertilizers in combination with organic manure has a positive influence on all soil properties, especially soil organic carbon. Similar, observations were also made by Singh et al. (2009) where the addition of inorganic fertilizers with various organic manures in rice-wheat system enhanced the soil particle aggregation which in turn resulted in higher storage of soil organic carbon content. For instance, several studies conducted in India to know the effect of integrated nutrient supply on soil organic carbon status indicated significant gain in soil organic carbon, especially under intensive cropping systems like rice-wheat (Singh et al., 2000; Nayak et al., 2012; Ramteke et al., 2017), maize-wheat (Paramesh et al., 2014, 2020), maize-mustard (Saha et al., 2010; Moharana et al., 2012), and rice-groundnut (Prasad et al., 2002; Table 3).
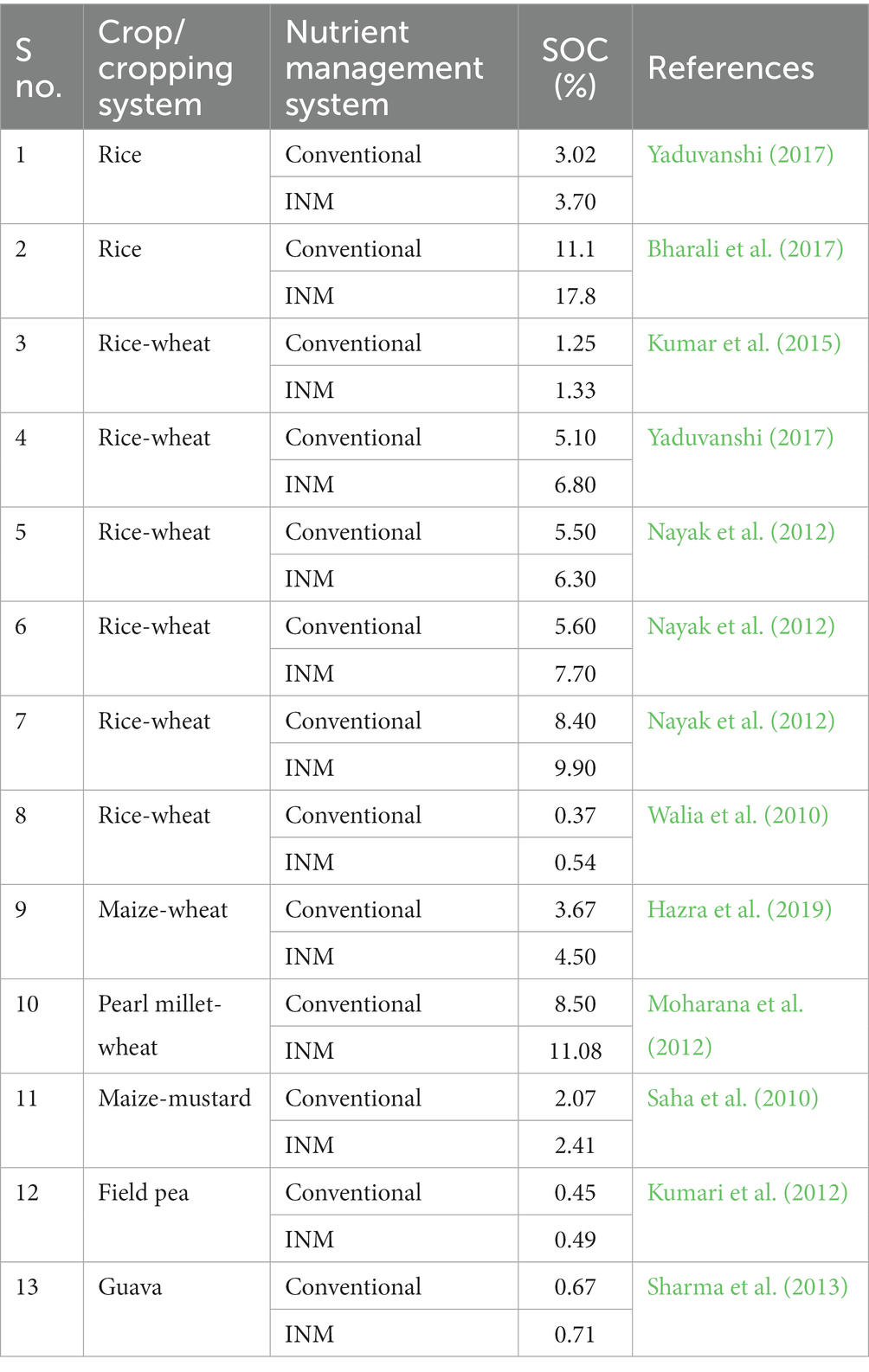
Table 3. Variation in soil organic carbon due to conventional and integrated nutrient management system in India.
The direct application of organic manure to the soil, which encouraged the development and activity of microorganisms, as well as improved root growth, which led to increased biomass output, crop stubbles, and residues, are both associated with increasing the amount of organic carbon in the INM (Yilmaz and Alagöz, 2010; Singh et al., 2011; Moharana et al., 2012). The increased carbon content of the soil have been caused by the eventual decomposition of these components. Additionally, the inclusion of FYM facilitated the synthesis of humic acid, which subsequently raised the soil’s organic carbon content (Bajpai et al., 2006). Further, they opined that increased residue return, and minimum or zero tillage practices as the contributing factors to increasing SOC under INM practice.
A review of the long-term fertilizer experiment in China by Lu et al. (2009) observed linear relationships between the amount of N application and straw incorporation to soil C sequestration. Contrary to this belief, Khan et al. (2007) observed a decline in SOC after 50 years of chemical fertilizer application despite crop residue incorporation due to excessive N removal by crops to the tune of 60%–190%. The possible reasons for the decrease in SOC under continuous chemical fertilizer application were (i) acid forming ammonium fertilizers application delay soil C decomposition, (ii) enhancement in the activities of heterotrophic soil microorganisms that use C derived from crop residues (Mack et al., 2004; Khan et al., 2007), and (iii) decrease in C:N ratio lead to bacterial-dominated microbial communities, this further results in faster decomposition of SOC (Moore et al., 2003). Increased decomposition of SOC will result in more dissolved organic C, which can be lost through leaching (Mack et al., 2004; Van Kessel et al., 2009).
Enhancing carbon sequestration through integrated nutrient management can be achieved by exploring soil management practices, investigating the role of plant–soil-microbial interactions, exploring the integration of agroforestry practices, developing precision nutrient management techniques, and using modeling tools and decision support systems. Soil management practices that promote carbon sequestration include the use of organic amendments, cover crops, and crop rotations. These practices can help to increase soil organic matter, which is a major sink for atmospheric carbon. Beneficial soil microorganisms, such as mycorrhizal fungi, can help to improve nutrient uptake, promote root growth, and enhance soil aggregation. These activities can create conditions that are favorable for carbon sequestration. Agroforestry systems can provide multiple benefits, including increased biomass production and carbon storage. Precision nutrient management techniques can indirectly contribute to carbon sequestration by improving nutrient use efficiency and minimizing nutrient losses. Adaption of modeling tools can consider factors such as soil type, climate conditions, and crop management practices to estimate carbon sequestration rates and provide recommendations for optimizing nutrient management strategies that enhance carbon sequestration.
3.4. Soil properties
Crops grow better under friable and well-aggregated soils with optimum soil bulk density as it greatly influencing crop root growth and nutrient uptake. Practicing long-term INM brings a favorable difference in soil bulk density (Saha et al., 2010). The main reason for decreasing bulk density under INM was aggregation of soil particles due to increasing organic matter as well as stability of aggregates which leads to an increase in the total pore space in the soil. Islam et al. (2012) also concluded that the addition of organic matter through organic manure decreases the bulk density of soil. Integration of organic sources of nutrients such as crop residue and organic manures has a significant effect on soil bulk density (Celik et al., 2004). Nevertheless, studies undertaken to know the influence of integrated nutrient management on soil bulk density indicated favorable and convincing results. A study conducted by Nayak et al. (2012) in rice-wheat cropping systems at various places indicated significantly lower values of soil bulk density in the plots treated with integrated nutrient management systems over conventional chemical fertilizers. Similarly, Saha et al. (2010) and Salahin et al. (2011) also revealed the positive benefits of integrated nutrient management on soil bulk density in maize-mustard and tomato-mung-toria cropping sequence (Table 4). A long-term fertilizer experiment conducted in a rice-wheat cropping system at Chattisgarh, India ascertained that the incorporation of organic sources with chemical fertilizer application decreased bulk density, increased infiltration rate, and available NPK status of the soil (Bajpai et al., 2006). Significant reduction of bulk density in INM may be due to better soil aggregation (Singh et al., 2000), higher organic carbon, and more pore space (Selvi et al., 2005). A similar reduction in bulk density of soil due to the application of FYM with 100% NPK was also observed by Bellakki et al. (1998) and Bhattacharyya et al. (2010).
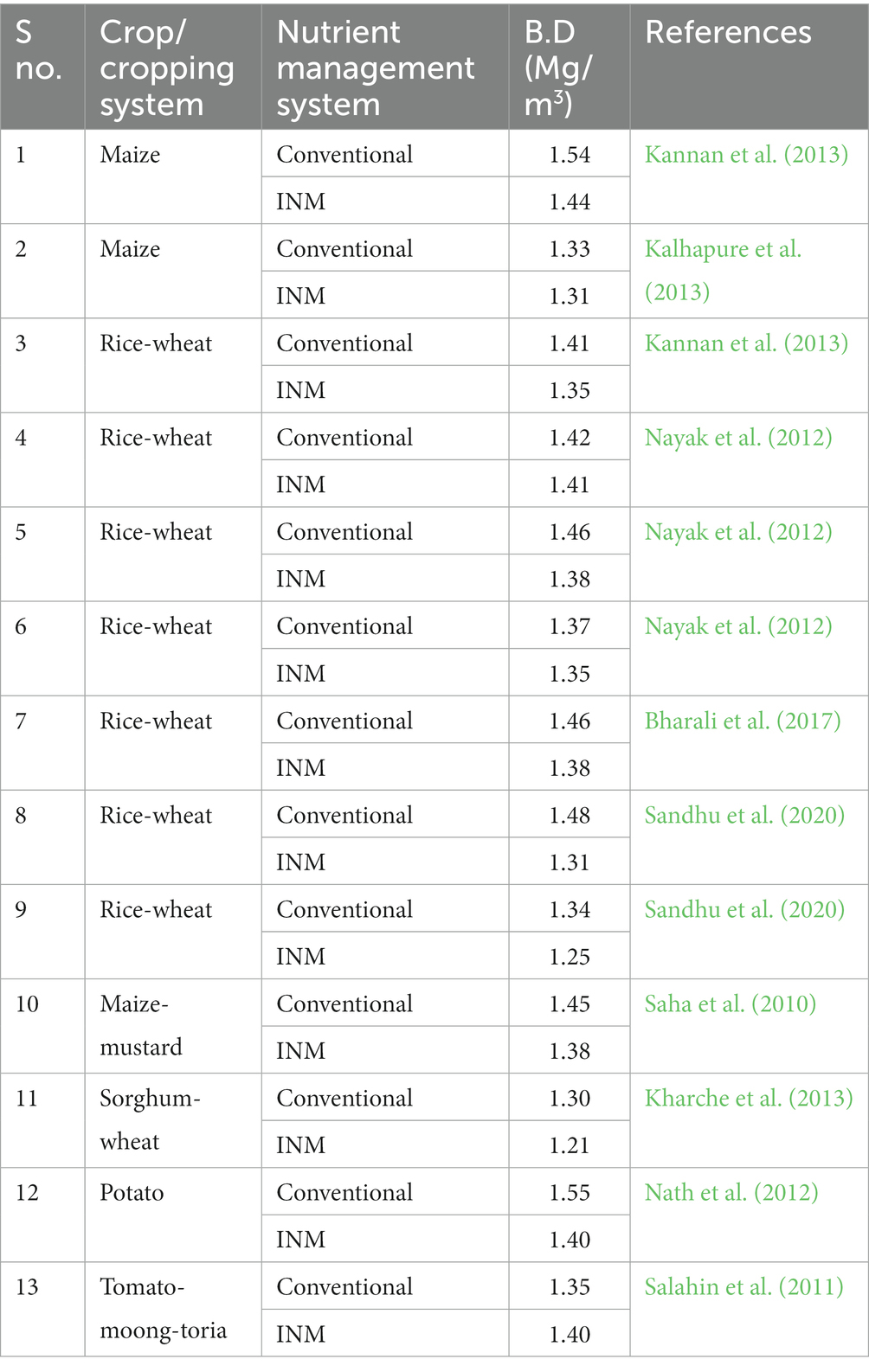
Table 4. Variation in soil bulk density due to conventional and integrated nutrient management system of India.
Table 5 shows an increase in SMBC and DHA under INM over conventional chemical fertilization. The increase in microbial biomass is mostly driven by the microbial biomass found in the organic byproducts and the addition of carbon from the substrate, both of which encourage the naturally occurring soil microbiota. The combined effect of FYM and chemical fertilizers in raising the SMBC under the maize-wheat system was also highlighted by Verma and Mathur (2009). The application of biofertilizers is known to create a variety of growth-promoting compounds in addition to their basic effects, which help explain the rapid expansion of microbial growth. Additionally, the crops’ rhizodeposition (Jones et al., 2009) contributed to the elevated SMBC under INM practice. Nayak et al. (2007) similarly demonstrated enhanced dehydrogenase activity with both compost and inorganic fertilizer application under continuous rice-growing situations and explained a significant relationship between SMBC. The addition of fly ash and FYM to a rice-wheat cropping system in Alfisol and Vertisol significantly enhanced the microbial biomass carbon and dehydrogenase activity in soil (Ramteke et al., 2017). Kanchikerimath and Singh (2001) found that the inorganic fertilizer (NPK) increased crop yield, SOC, total N, mineralizable C and N, microbial biomass C and N, and dehydrogenase, urease, and alkaline phosphatase activities, while manure applied together with inorganic fertilizer increased these parameters more strongly. However, when more organic residues are added to the soil, they undergo microbial decomposition, which releases organic compounds like polysaccharides, which act as a strong binding agent in the formation of large and stable aggregates that help to improve the physical properties of soil (Manickam, 1993). Accordingly, Yuhui et al. (2004) highlighted the significance of organic manure addition with chemical fertilizers to improve yield, and soil health, and enhance the earthworm population in the soil. In another long-term experiment in Germany, Marhan and Scheu (2005) observed an increase in earthworm biomass by 42.8% in NPK + FYM treatment, with a decrease of 9.4% in NPK treatment. They ascertained an increase in earthworm biomass in NPK + FYM could be due increased utilizable SOM pool.
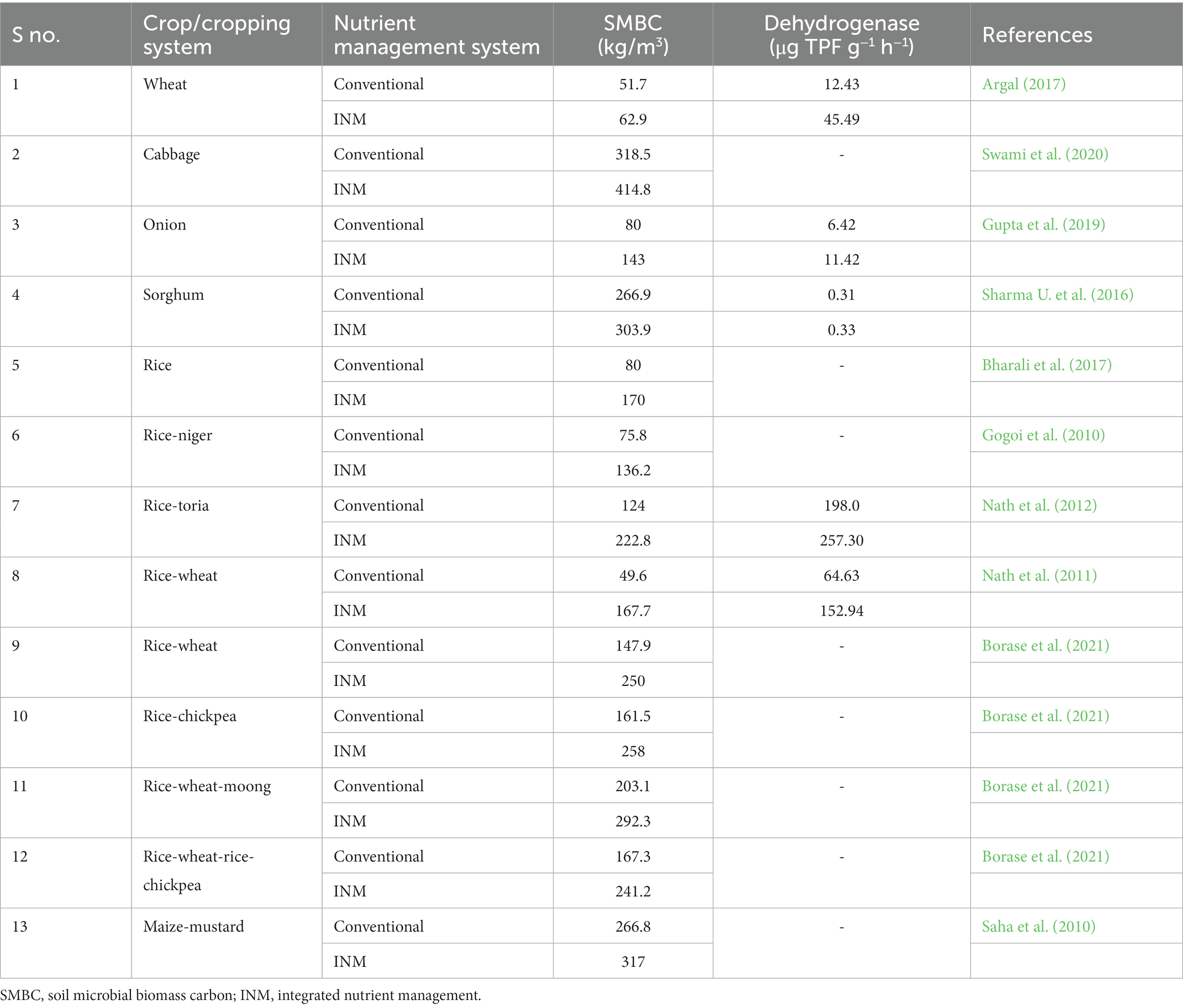
Table 5. Variation in SMBC and dehydrogenase activity due to conventional and integrated nutrient management system.
Future research in integrated nutrient management can prioritize enhancing soil quality, a critical aspect of sustainable agriculture. One promising area is the exploration of practices that enhance soil organic matter content and composition. Efforts can focus on identifying effective organic amendments, cover crops, and crop rotations that facilitate the accumulation of stable organic matter in soils. Understanding the interactions among nutrient management practices, organic matter dynamics, and soil microbial communities will provide valuable insights for optimizing soil quality enhancement. Additionally, research should emphasize nutrient management strategies that promote soil nutrient cycling and enhance nutrient use efficiency. This involves investigating the synergistic relationships between organic and inorganic fertilizers, biofertilizers, and soil microorganisms in nutrient cycling processes. Developing nutrient management approaches that minimize nutrient losses through leaching, volatilization, and runoff, while simultaneously ensuring sufficient nutrient availability for crops, will improve nutrient use efficiency and contribute to soil quality enhancement. Moreover, such practices will help mitigate environmental pollution associated with nutrient runoff.
3.5. Integrated nutrient management on soil health and crop quality
The current review has shown a positive effect of Integrated nutrient management (INM) on yield, soil microbial activity, SOC, and BD over conventional chemical fertilizer application. These summarized results further highlighted reduction in use of chemical fertilizer under INM practices, this in turn resulting in reduction of environmental burden associated with fertilizer production, transport, and its use. The main objective of INM is to wisely utilize its three primary components. These include harnessing the existing synergy between dual-purpose microbes (which promote growth and control soil-borne pathogens), limiting the use of chemical fertilizers, promoting the multiplication of native soil microbial diversity through organic substrates, and maintaining a nutrient inflow that exceeds outflow. Additionally, the aim is to ensure that production economics are favorable in the market. Nevertheless, there are still several crucial areas that require urgent attention to make INM a globally dynamic nutrient management approach. Through enhancement of SOC and soil microbial activity INM increases soil nutrient availability and it is having positive impact on crop quality.
4. Conclusion
Farmers have rich experiences in integrated and efficient utilization of different sources of organic materials to produce modest crop yields and maintain soil fertility using traditional farming practices. However, during the last two decades, to achieve food security with declining land and other resources, this practice is gradually being abandoned, and nutrient management is being shifted to over-reliance on chemical fertilizers. Over-application of N has become more common in intensive agricultural regions, leading to low nutrient-use efficiency and environmental pollution, which threaten the long-term sustainability of the agricultural system. Many factors might have contributed to the over-application problems including obtaining higher yields as a top priority, small land holding, lack of nitrogen management, and lack of effective extension systems. A review of experiments conducted globally indicates that chemical fertilizers alone are not enough to improve yield and soil quality at high levels. Further, the review also highlighted the negative effects of the continuous use of synthetic N fertilizers on soil organic carbon, bulk density, soil enzymatic activity, SMBC, and the environment. Crop yield responses to nutrient management may vary significantly from year to year due to variations in weather conditions and indigenous N supply, and thus the commonly adopted prescriptive approach to N management needs to be replaced by a responsive in-season management approach based on the diagnosis of crop growth and N status and demand.
Author contributions
VP, RM, SG, GR, AN, ST, and YM designed the research, data collection, and analysis. VP, RM, SB, AN, DJ, and SG were involved in the interpretation of results, data analysis, draft manuscript preparation, and final manuscript preparation and editing. All authors contributed to the article and approved the submitted version.
Acknowledgments
We thank the Indian Council of Agricultural Research, New Delhi and ICAR-Central Coastal Agricultural Research Institute, Goa, for providing the necessary facility.
Conflict of interest
The authors declare that the research was conducted in the absence of any commercial or financial relationships that could be construed as a potential conflict of interest.
Publisher’s note
All claims expressed in this article are solely those of the authors and do not necessarily represent those of their affiliated organizations, or those of the publisher, the editors and the reviewers. Any product that may be evaluated in this article, or claim that may be made by its manufacturer, is not guaranteed or endorsed by the publisher.
References
Argal, M. S. (2017). Impact of nutrient management on plant nutrient content and nutrient uptake of wheat (Triticum aestivum L.) under degraded land of Chambal ravine. Int. J. Pure Appl. Biosci. 5, 1672–1682. doi: 10.18782/2320-7051.6180
Bajpai, R. K., Chitale, S., Upadhyay, S. K., and Urkurkar, J. S. (2006). Long-term studies on soil physico-chemical properties and productivity of rice-wheat system as influenced by integrated nutrient management in Inceptisol of Chhattisgarh. J. Indian Soc. Soil Sci 54, 24–29.
Baviskar, M. N., Bharad, S. G., Dod, V. N., and Barne, V. G. (2011). Effect of integrated nutrient management on yield and quality of sapota. Plant Arch. 11, 661–663.
Bellakki, M. A., Badanur, V. P., and Setty, R. A. (1998). Effect of long-term integrated nutrient management on some important properties of a vertisol. J. Indian Soc. Soil Sci 46, 176–180.
Bharali, A., Baruah, K. K., Bhattacharyya, P., and Gorh, D. (2017). Integrated nutrient management in wheat grown in a Northeast India soil: impacts on soil organic carbon fractions in relation to grain yield. Soil Tillage Res. 168, 81–91. doi: 10.1016/j.still.2016.12.001
Bhattacharyya, R., Kundu, S., Prakash, V., and Gupta, H. S. (2008). Sustainability under combined application of mineral and organic fertilizers in a rainfed soybean–wheat system of the Indian Himalayas. Eur. J. Agron. 28, 33–46. doi: 10.1016/j.eja.2007.04.006
Bhattacharyya, R., Prakash, V., Kundu, S., Srivastva, A. K., Gupta, H. S., and Mitra, S. (2010). Long term effects of fertilization on carbon and nitrogen sequestration and aggregate associated carbon and nitrogen in the Indian sub-Himalayas. Nutr. Cycl. Agroecosyst. 86, 1–16. doi: 10.1007/s10705-009-9270-y
Biswas, P. P., and Sharma, P. D. (2008). A new approach for estimating fertiliser response ratio-the Indian scenario. Indian J. Fertil. 4:59.
Borase, D. N., Murugeasn, S., Nath, C. P., Hazra, K. K., Singh, S. S., Kumar, N., et al. (2021). Long-term impact of grain legumes and nutrient management practices on soil microbial activity and biochemical properties. Arch. Agron. Soil Sci. 67, 2015–2032. doi: 10.1080/03650340.2020.1819532
Carlson, K. M., Gerber, J. S., Mueller, N. D., Herrero, M., MacDonald, G. K., Brauman, K. A., et al. (2017). Greenhouse gas emissions intensity of global croplands. Nat. Clim. Chang. 7, 63–68. doi: 10.1038/nclimate3158
Cassman, K. G., Dobermann, A., and Walters, D. T. (2002). Agroecosystems, nitrogen-use efficiency, and nitrogen management. AMBIO A J. Hum. Environ. 31, 132–140. doi: 10.1579/0044-7447-31.2.132
Celik, I., Ortas, I., and Kilic, S. (2004). Effects of compost, mycorrhiza, manure and fertilizer on some physical properties of a Chromoxerert soil. Soil Tillage Res. 78, 59–67. doi: 10.1016/j.still.2004.02.012
Chaturvedi, S., Chandel, A. S., and Singh, A. P. (2012). Nutrient management for enhanced yield and quality of soybean (Glycine max.) and residual soil fertility. Legum. Res. Int. J. 35, 175–184.
Chaudhari, L. S., Mane, S. S., and Giri, S. N. (2019). Growth, yield and quality of soybean as influenced by INM. Int. J. Pure Appl. Biosci. 7, 209–212. doi: 10.18782/2320-7051.7228
Chen, X.-P., Cui, Z.-L., Vitousek, P. M., Cassman, K. G., Matson, P. A., Bai, J.-S., et al. (2011). Integrated soil–crop system management for food security. Proc. Natl. Acad. Sci. 108, 6399–6404. doi: 10.1073/pnas.1101419108
Chen, X., Zhang, F., Römheld, V., Horlacher, D., Schulz, R., Böning-Zilkens, M., et al. (2006). Synchronizing N supply from soil and fertilizer and N demand of winter wheat by an improved Nmin method. Nutr. Cycl. Agroecosyst. 74, 91–98. doi: 10.1007/s10705-005-1701-9
Damse, D. N., Bhalekar, M. N., and Pawar, P. K. (2014). Effect of integrated nutrient management on growth and yield of garlic. Bioscan 9, 1557–1560.
Das, S., and Adhya, T. K. (2014). Effect of combine application of organic manure and inorganic fertilizer on methane and nitrous oxide emissions from a tropical flooded soil planted to rice. Geoderma 213, 185–192. doi: 10.1016/j.geoderma.2013.08.011
Das, B., Chakraborty, D., Singh, V. K., Aggarwal, P., Singh, R., Dwivedi, B. S., et al. (2014). Effect of integrated nutrient management practice on soil aggregate properties, its stability and aggregate-associated carbon content in an intensive rice–wheat system. Soil Tillage Res. 136, 9–18. doi: 10.1016/j.still.2013.09.009
Davidson, E. A., Galloway, J. N., Millar, N., and Leach, A. M. (2014). N-related greenhouse gases in North America: innovations for a sustainable future. Curr. Opin. Environ. Sustain. 9-10, 1–8. doi: 10.1016/j.cosust.2014.07.003
Dwivedi, V. (2013). Effect of integrated nutrient management on yield, quality and economics of guava. Ann. Plant Soil Res. 15, 149–151.
Dwivedi, B. S., Singh, V. K., Meena, M. C., Dey, A., and Datta, S. P. (2016). Integrated nutrient management for enhancing nitrogen use efficiency. Indian J. Fertil 12, 62–71. doi: 10.3390/plants10112547
Esilaba, A. O., Byalebeka, J. B., Delve, R. J., Okalebo, J. R., Ssenyange, D., Mbalule, M., et al. (2005). On farm testing of integrated nutrient management strategies in eastern Uganda. Agr. Syst. 86, 144–165. doi: 10.1016/j.agsy.2004.09.005
Farhad, I. S. M., Rahman, M. A., Jahan, E., Azam, M. G., and Khan, N. R. (2017). Integrated nutrient management on soybean in a coastal charland of Bangladesh. Bangladesh Agron. J. 20, 77–83. doi: 10.3329/baj.v20i1.34886
Fertilizer Association of India (2021). Statistical database. Available at: https://www.faidelhi.org/statistics/statistical-database.
Foley, J. A., Ramankutty, N., Brauman, K. A., Cassidy, E. S., Gerber, J. S., Johnston, M., et al. (2011). Solutions for a cultivated planet. Nature 478, 337–342. doi: 10.1038/nature10452
Garai, T. K., Datta, J. K., and Mondal, N. K. (2014). Evaluation of integrated nutrient management on boro rice in alluvial soil and its impacts upon growth, yield attributes, yield and soil nutrient status. Arch. Agron. Soil Sci. 60, 1–14. doi: 10.1080/03650340.2013.766721
Garnett, T., Appleby, M. C., Balmford, A., Bateman, I. J., Benton, T. G., Bloomer, P., et al. (2013). Sustainable intensification in agriculture: premises and policies. Science 341, 33–34. doi: 10.1126/science.1234485
Gogoi, B., Barua, N. G., and Baruah, T. C. (2010). Effect of integrated supply of nutrients on soil microbial biomass carbon in an Inceptisol of Assam. J. Indian Soc. Soil Sci 58:241.
Gruhn, P., Goletti, F., and Yudelman, M. (2000). Integrated nutrient management, soil fertility, and sustainable agriculture: Current issues and future challenges. Washington, D.C., USA: Intl Food Policy Res Inst.
Gupta, R., Rai, A. P., and Swami, S. (2019). Soil enzymes, microbial biomass carbon and microbial population as influenced by integrated nutrient management under onion cultivation in sub-tropical zone of Jammu. J. Pharmacogn. Phytochem. 8, 194–199.
Hati, K. M., Swarup, A., Mishra, B., Manna, M. C., Wanjari, R. H., Mandal, K. G., et al. (2008). Impact of long-term application of fertilizer, manure and lime under intensive cropping on physical properties and organic carbon content of an Alfisol. Geoderma 148, 173–179. doi: 10.1016/j.geoderma.2008.09.015
Hazra, K. K., Nath, C. P., Singh, U., Praharaj, C. S., Kumar, N., Singh, S. S., et al. (2019). Diversification of maize-wheat cropping system with legumes and integrated nutrient management increases soil aggregation and carbon sequestration. Geoderma 353, 308–319. doi: 10.1016/j.geoderma.2019.06.039
Hossain, M., and Singh, V. P. (2000). Fertilizer use in Asian agriculture: implications for sustaining food security and the environment. Nutr. Cycl. Agroecosyst. 57, 155–169. doi: 10.1023/A:1009865819925
Huang, Y., and Sun, W. (2006). Changes in topsoil organic carbon of croplands in mainland China over the last two decades. Chin. Sci. Bull. 51, 1785–1803. doi: 10.1007/s11434-006-2056-6
IFA (International Fertilizer Association) (2020). “Short-term fertilizer outlook 2020-2021” in Public summary of IFA virtual strategic forum (Paris, France: International Fertilizer Association), 17–19.
Islam, M. R., Sikder, S., Bahadur, M. M., and Hafiz, M. H. R. (2012). Effect of different fertilizer management on soil properties and yield of fine rice cultivar. J. Environ. Sci. Nat. Resour. 5, 239–242. doi: 10.3329/jesnr.v5i1.11588
Jambert, C., Serca, D., and Delmas, R. (1997). Quantification of N-losses as NH3, NO, and N2O and N2 from fertilized maize fields in southwestern France. Nutr. Cycl. Agroecosyst. 48, 91–104. doi: 10.1023/A:1009786531821
Janssen, B. H. (1993). Integrated nutrient management: the use of organic and mineral fertilizers. In H. Reulervan and W. H. Prins (Eds.), The role of plant nutrients for sustainable food crop production in Sub-Saharan Africa (pp. 89–105). Ver. Kunstmest Producenten.
Jat, M. K., Tikkoo, A., Yadav, P. K., Yadav, S. S., and Yadav, P. V. S. (2017). Effect of integrated nutrient management on yield, soil fertility and economics in Abelmoschus esculentus–Allium cepa cropping system in semi arid zone of Haryana. J. Pharmacogn. Phytochem. 6, 1142–1145.
Jones, D. L., Nguyen, C., and Finlay, R. D. (2009). Carbon flow in the rhizosphere: carbon trading at the soil–root interface. Plant and Soil 321, 5–33. doi: 10.1007/s11104-009-9925-0
Ju, X., Lu, X., Gao, Z., Chen, X., Su, F., Kogge, M., et al. (2011). Processes and factors controlling N2O production in an intensively managed low carbon calcareous soil under sub-humid monsoon conditions. Environ. Pollut. 159, 1007–1016. doi: 10.1016/j.envpol.2010.10.040
Kalhapure, A. H., Shete, B. T., and Dhonde, M. B. (2013). Integrated nutrient management in maize (Zea Mays L.) for increasing production with sustainability. Int. J. Agric. Food Sci. Technol. 4, 195–206.
Kanchikerimath, M., and Singh, D. (2001). Soil organic matter and biological properties after 26 years of maize–wheat–cowpea cropping as affected by manure and fertilization in a Cambisol in semiarid region of India. Agric. Ecosyst. Environ. 86, 155–162. doi: 10.1016/S0167-8809(00)00280-2
Kannan, R. L., Dhivya, M., Abinaya, D., Krishna, R. L., and Krishnakumar, S. (2013). Effect of integrated nutrient management on soil fertility and productivity in maize. Bull. Environ. Pharmacol. Life Sci. 2, 61–67.
Khan, S. A., Mulvaney, R. L., Ellsworth, T. R., and Boast, C. W. (2007). The myth of nitrogen fertilization for soil carbon sequestration. J. Environ. Qual. 36, 1821–1832. doi: 10.2134/jeq2007.0099
Kharche, V. K., Patil, S. R., Kulkarni, A. A., Patil, V. S., and Katkar, R. N. (2013). Long-term integrated nutrient management for enhancing soil quality and crop productivity under intensive cropping system on vertisols. J. Indian Soc. Soil Sci 61, 323–332.
Kumar, R. M., Hiremath, S. M., and Nadagouda, B. T. (2015). Effect of single-cross hybrids, plant population and fertility levels on productivity and economics of maize (Zea mays). Indian J. Agron. 60, 431–435.
Kumari, A., Singh, O. N., and Kumar, R. (2012). Effect of integrated nutrient management on growth, seed yield and economics of field pea (Pisum sativum L.) and soil fertility changes. J. Food Legum. 25, 121–124.
Ladha, J. K., Pathak, H., Krupnik, T. J., Six, J., et al. (2005). Efficiency of fertiliser nitrogen in cereal production: retrospect and prospect. Advances in Agronomy 87, 85–156.
Lal, G., and Dayal, H. (2014). Effect of integrated nutrient management on yield and quality of acid lime (Citrus aurantifolia Swingle). African J. Agric. Res. 9, 2985–2991. doi: 10.5897/AJAR2014.8902
Lu, F. E. I., Wang, X., Han, B., Ouyang, Z., Duan, X., Zheng, H. U. A., et al. (2009). Soil carbon sequestrations by nitrogen fertilizer application, straw return and no-tillage in China’s cropland. Glob. Chang. Biol. 15, 281–305. doi: 10.1111/j.1365-2486.2008.01743.x
Ma, B. L., Wu, T. Y., Tremblay, N., Deen, W., Morrison, M. J., McLaughlin, N. B., et al. (2010). Nitrous oxide fluxes from corn fields: on-farm assessment of the amount and timing of nitrogen fertilizer. Glob. Chang. Biol. 16, 156–170. doi: 10.1111/j.1365-2486.2009.01932.x
Mack, M. C., Schuur, E. A. G., Bret-Harte, M. S., Shaver, G. R., and Chapin, F. S. (2004). Ecosystem carbon storage in arctic tundra reduced by long-term nutrient fertilization. Nature 431, 440–443. doi: 10.1038/nature02887
Mahajan, A., and Gupta, R. D. (2009). Integrated nutrient management (INM) in a sustainable rice-wheat cropping system. Berlin, Germany: Springer Science & Business Media.
Majumdar, D., Pathak, H., Kumar, S., and Jain, M. C. (2002). Nitrous oxide emission from a sandy loam Inceptisol under irrigated wheat in India as influenced by different nitrification inhibitors. Agric. Ecosyst. Environ. 91, 283–293. doi: 10.1016/S0167-8809(01)00223-7
Manickam, T. S. (1993). “Organics in soil fertility and productivity management” in Organics in soil health and crop production. ed. P. K. Thampan (India, Peekay Tree Crops Developments Foundation: Cochin), 87–104.
Marhan, S., and Scheu, S. (2005). The influence of mineral and organic fertilisers on the growth of the endogeic earthworm Octolasion tyrtaeum (Savigny). Pedobiologia 49, 239–249. doi: 10.1016/j.pedobi.2004.11.002
Marimuthu, S., Surendran, U., and Subbian, P. (2014). Productivity, nutrient uptake and post-harvest soil fertility as influenced by cotton-based cropping system with integrated nutrient management practices in semi-arid tropics. Arch. Agron. Soil Sci. 60, 87–101. doi: 10.1080/03650340.2013.771259
Moharana, P. C., Sharma, B. M., Biswas, D. R., Dwivedi, B. S., and Singh, R. V. (2012). Long-term effect of nutrient management on soil fertility and soil organic carbon pools under a 6-year-old pearl millet–wheat cropping system in an Inceptisol of subtropical India. F. Crop. Res. 136, 32–41. doi: 10.1016/j.fcr.2012.07.002
Moore, J. C., McCann, K., Setälä, H., and De Ruiter, P. C. (2003). Top-down is bottom-up: does predation in the rhizosphere regulate aboveground dynamics? Ecology 84, 846–857. doi: 10.1890/0012-9658(2003)084[0846:TIBDPI]2.0.CO;2
Nath, A. J., Brahma, B., Sileshi, G. W., and Das, A. K. (2018). Impact of land use changes on the storage of soil organic carbon in active and recalcitrant pools in a humid tropical region of India. Sci. Total Environ. 624, 908–917. doi: 10.1016/j.scitotenv.2017.12.199
Nath, D. J., Ozah, B., Baruah, R., Barooah, R. C., and Borah, D. K. (2011). Effect of integrated nutrient management on soil enzymes, microbial biomass carbon and bacterial populations under rice (Oryza sativa)-wheat (Triticum aestivum) sequence. Indian J. Agric. Sci. 81:1143.
Nath, D. J., Ozah, B., Baruah, R., Barooah, R. C., Borah, D. K., and Gupta, M. (2012). Soil enzymes and microbial biomass carbon under rice-toria sequence as influenced by nutrient management. J. Indian Soc. Soil Sci 60, 20–24.
Nayak, D. R., Babu, Y. J., and Adhya, T. K. (2007). Long-term application of compost influences microbial biomass and enzyme activities in a tropical Aeric Endoaquept planted to rice under flooded condition. Soil Biol. Biochem. 39, 1897–1906. doi: 10.1016/j.soilbio.2007.02.003
Nayak, A. K., Gangwar, B., Shukla, A. K., Mazumdar, S. P., Kumar, A., Raja, R., et al. (2012). Long-term effect of different integrated nutrient management on soil organic carbon and its fractions and sustainability of rice–wheat system in indo Gangetic Plains of India. Field Crop Res 127, 129–139. doi: 10.1016/j.fcr.2011.11.011
Nyamadzawo, G., Shi, Y., Chirinda, N., Olesen, J. E., Mapanda, F., Wuta, M., et al. (2017). Combining organic and inorganic nitrogen fertilisation reduces N2O emissions from cereal crops: a comparative analysis of China and Zimbabwe. Mitig. Adapt. Strat. Glob. Chang. 22, 233–245. doi: 10.1007/s11027-014-9560-9
Nyamadzawo, G., Wuta, M., Nyamangara, J., Smith, J. L., and Rees, R. M. (2014). Nitrous oxide and methane emissions from cultivated seasonal wetland (dambo) soils with inorganic, organic and integrated nutrient management. Nutr. Cycl. Agroecosyst. 100, 161–175. doi: 10.1007/s10705-014-9634-9
Paramesh, V., Dhar, S., Dass, A., Kumar, B., Kumar, A., El-Ansary, D. O., et al. (2020). Role of integrated nutrient management and agronomic fortification of zinc on yield, nutrient uptake and quality of wheat. Sustainability 12:3513. doi: 10.3390/su12093513
Paramesh, V., Dhar, S., Vyas, A. K., and Dass, A. (2014). Studies on impact of phosphoenriched compost, chemical fertilizer and method of zinc application on yield, uptake and quality of maize (Zea mays). Indian J. Agron. 59, 613–618.
Paramesh, V., Sridhara, C. J., and Shashidhar, K. S. (2013a). Effect of integrated nutrient management and planting geometry on root parameter and nutrient uptake of aerobic rice. Agric. Updat. 8, 217–220.
Paramesh, V., Sridhara, C. J., Shashidhar, K. S., and Vinoda, K. S. (2013b). Soil nutrient balance in aerobic rice as affected by integrated nutrient management and planting geometry. J. Inter. Des. 17, 441–446.
Pati, P., and Mahapatra, P. K. (2015). Yield performance and nutrient uptake of Indian mustard (Brassica juncea L.) as influenced by integrated nutrient management. J. Crop Weed 11, 58–61.
Paustian, K., Collins, H. P., and Paul, E. A. (2019). “Management controls on soil carbon” in Soil organic matter in temperate agroecosystems. eds. E. A. Paul, K. H. Paustian, E. T. Elliott, and C. V. Cole (Boca Raton, Florida, United States: CRC Press), 15–49.
Peng, S., Huang, J., Zhong, X., Yang, J., Wang, G., Zou, Y., et al. (2002). Challenge and Opportunity in Improving Fertilizer-nitrogen Use Efficiency of Irrigated Rice in China. Agricultural Sciences in China 1, 776–785.
Power, A. G. (2013). “Ecology of agriculture” in Encyclopedia of biodiversity. Cornell University, Ithaca, NY, USA: (Elsevier), 9–15.
Prasad, P. V. V., Satyanarayana, V., Murthy, V. R. K., and Boote, K. J. (2002). Maximizing yields in rice–groundnut cropping sequence through integrated nutrient management. Field Crop Res 75, 9–21. doi: 10.1016/S0378-4290(01)00214-3
Prativa, K. C., and Bhattarai, B. P. (2011). Effect of integrated nutrient management on the growth, yield and soil nutrient status in tomato. Nepal J. Sci. Technol. 12, 23–28.
Ramteke, L. K., Sengar, S. S., and Porte, S. S. (2017). Effect of fly ash, organic manure and fertilizers on soil microbial activity in ricewheat cropping system in Alfisols and Vertisols. Int. J. Curr. Microbiol. App. Sci. 6, 1948–1952. doi: 10.20546/ijcmas.2017.607.231
Rao, N. S. (2006). Nitrate pollution and its distribution in the groundwater of Srikakulam district, Andhra Pradesh, India. Environmental Geology 51, 631–645.
Rasmussen, P. E., Goulding, K. W. T., Brown, J. R., Grace, P. R., Janzen, H. H., and Körschens, M. (1998). Long-term agroecosystem experiments: assessing agricultural sustainability and global change. Science 282, 893–896. doi: 10.1126/science.282.5390.893
Ravikumar, C., Ganapathy, M., Karthikeyan, A., Senthilvalavan, P., and Manivannan, R. (2021). Integrated nutrient management—promising way to reduce carbon dioxide and methane emission in flooded rice ecosystem: a review. J. Appl. Nat. Sci. 13, 385–395. doi: 10.31018/jans.v13i1.2570
Reddy, K. S., Mohanty, M., Rao, D. L. N., Singh, M., Dalal, R. C., Rao, A. S., et al. (2008). Nitrogen mineralization in a vertisol from organic manures, green manures and crop residues in relation to their quality. Agrochimica 52, 377–388.
Saha, R., Mishra, V. K., Majumdar, B., Laxminarayana, K., and Ghosh, P. K. (2010). Effect of integrated nutrient management on soil physical properties and crop productivity under a maize (Zea mays)–mustard (Brassica campestris) cropping sequence in acidic soils of Northeast India. Commun. Soil Sci. Plant Anal. 41, 2187–2200. doi: 10.1080/00103624.2010.504799
Salahin, N., Islam, M. S., Begum, R. A., Alam, M. K., and Hossain, K. M. F. (2011). Effect of tillage and integrated nutrient management on soil physical properties and yield under tomato-mungbean-t. aman cropping pattern. Int. J. Sustain. Crop Prod 6, 58–62.
Sandhu, P. S., Walia, S. S., Gill, R. S., and Dheri, G. S. (2020). Thirty-one years study of integrated nutrient management on physico-chemical properties of soil under rice–wheat cropping system. Commun. Soil Sci. Plant Anal. 51, 1641–1657. doi: 10.1080/00103624.2020.1791156
Sangeeta, S., Singh, V. K., and Ravi, K. (2014). Effect of integrated nutrient management on yield and quality of cauliflower (Brassica oleracea var. botrytis L.). Bioscan 9, 1053–1058.
Sapkota, T. B., Jat, M. L., Rana, D. S., Khatri-Chhetri, A., Jat, H. S., Bijarniya, D., et al. (2021). Crop nutrient management using nutrient expert improves yield, increases farmers’ income and reduces greenhouse gas emissions. Sci. Rep. 11:1564. doi: 10.1038/s41598-020-79883-x
Selim, M. (2018). Potential role of cropping system and integrated nutrient management on nutrients uptake and utilization by maize grown in calcareous soil. Egypt. J. Agron. 40, 297–312. doi: 10.21608/agro.2018.6277.1134
Selim, M. M., and Al-Owied, A.-J. A. (2017). Genotypic responses of pearl millet to integrated nutrient management. Biosci. Res. 14, 156–169.
Selvi, D., Santhy, P., and Dhakshinamoorthy, M. (2005). Effect of inorganics alone and in combination with farmyard manure on physical properties and productivity of Vertic Haplustepts under long-term fertilization. J. Indian Soc. Soil Sci 53, 302–307.
Sharma, P., Meena, R. S., Kumar, S., Gurjar, D. S., Yadav, G. S., and Kumar, S. (2019). Growth, yield and quality of cluster bean (Cyamopsis tetragonoloba) as influenced by integrated nutrient management under alley cropping system. Indian J. Agric. Sci. 89, 1876–1880. doi: 10.56093/ijas.v89i11.95333
Sharma, S. K., Singh, Y. V., Tyagi, S., and Bhatia, A. (2016). Influence of rice varieties, nitrogen management and planting methods on methane emission and water productivity. Paddy Water Environ. 14, 325–333. doi: 10.1007/s10333-015-0502-2
Sharma, U., Subehia, S. K., and Rana, S. S. (2016). Long term effect of integrated nutrient management on yield and economics of rice (Oryza sativa L.)-wheat (Triticum aestivum L.) system in north-western Himalaya. Res. Crop. 17, 173–177. doi: 10.5958/2348-7542.2016.00031.0
Sharma, A., Wali, V. K., Bakshi, P., and Jasrotia, A. (2013). Effect of integrated nutrient management strategies on nutrient status, yield and quality of guava. Indian J. Hortic. 70, 333–339.
Singh, A. K., Amgain, L. P., and Sharma, S. K. (2000). Root characteristics, soil physical properties and yield of rice (Oryza sativa) as influenced by integrated nutrient management in rice-wheat (Triticum aestivum) system. Indian J. Agron. 45, 217–222.
Singh, A. K., Chakraborti, M., and Datta, M. (2014). Improving Rice-based cropping pattern through soil moisture and integrated nutrient Management in mid-Tropical Plain Zone of Tripura. India. Rice Sci. 21, 299–304. doi: 10.1016/S1672-6308(13)60190-0
Singh, G., Kumar, S., Sidhu, G. S., and Kaur, R. (2018). Effect of integrated nutrient management on yield of wheat (Triticum aestivum L.) under irrigated conditions. Int. J. Chem. Stud. 6, 904–907.
Singh, G., Kumar, S., Singh, G., and Singh, N. (2019). Effect of integrated nutrient management on nutrient uptake and grain yield of wheat (Triticum aestivum L.) under irrigated conditions. J. Pharmacogn. Phytochem. 8, 1077–1080.
Singh, R. N., Singh, S., Prasad, S. S., Singh, V. K., and Kumar, P. (2011). Effect of integrated nutrient management on soil fertility, nutrient uptake and yield of rice-pea cropping system on an upland acid soil of Jharkhand. J. Indian Soc. Soil Sci 59, 158–163.
Singh, R., Singh, Y. P., Yaduvanshi, N. P. S., and Sharma, D. K. (2009). Effect of irrigation scheduling and integrated nutrient management on. J. Indian Soc. Soil Sci 57, 280–286.
Song, F., Xu, M., Duan, Y., Cai, Z., Wen, S., Chen, X., et al. (2020). Spatial variability of soil properties in red soil and its implications for site-specific fertilizer management. J. Integr. Agric. 19, 2313–2325. doi: 10.1016/S2095-3119(20)63221-X
Srinivasarao, C., Sumanta Kundu, C., Subha Lakshmi, M., Babu, V. S., Gabhane, V. V., Sarma, P. K., et al. (2020). “Manures versus fertilizers in rainfed dryland production systems of India” in Soil and Fertilizers. ed. R. Lal (Boca Raton, Florida, United States: CRC Press), 131–168.
Sujata, P., Rathod, P. K., Ravankar, H. N., Patil, Y. G., Yewale, A. C., and Dhammadina, P. (2007). Effect of long-term fertilization in vertisols on soil properties and yield of sorghum wheat sequence. Asian J. Soil Sci. 2, 74–78.
Sutton, M. A., Bleeker, A., Howard, C. M., Erisman, J. W., Abrol, Y. P., Bekunda, M., et al. (2013). Our nutrient world. The challenge to produce more food & energy with less pollution Centre for Ecology and Hydrology.
Swami, S., Singh, S., and Konyak, C. P. W. (2020). Physico-chemical and microbiological properties of acid Inceptisol as influenced by INM practices under cabbage (Brassica oleracea L. var. capitata) production. J. Chem. Res. Adv. 1, 1–9.
Swarup, A., and Yaduvanshi, N. P. S. (2000). Effects of integrated nutrient management on soil properties and yield of rice in alkali soils. J. Indian Soc. Soil Sci 48, 279–282.
Tandon, H. L. S. (2004). Fertilizers in Indian agriculture from 20th to 21st century Fertiliser Development and Consultation Organisation.
Tandon, H. L. S., and Tiwari, K. N. (2007). Fertiliser use in Indian agriculture—an eventful half century. Better Crop. 1, 3–5.
Tilman, D., Cassman, K. G., Matson, P. A., Naylor, R., and Polasky, S. (2002). Agricultural sustainability and intensive production practices. Nature 418, 671–677. doi: 10.1038/nature01014
Van Kessel, C., Clough, T., and van Groenigen, J. W. (2009). Dissolved organic nitrogen: an overlooked pathway of nitrogen loss from agricultural systems? J. Environ. Qual. 38, 393–401. doi: 10.2134/jeq2008.0277
Verma, K., Bindra, A. D., and Singh, J. (2018). Effect of integrated nutrient management on System’s Total productivity, System’s Total uptake and System’s Total economics of maize and wheat in maize-wheat cropping sequence in Mid Hills of HP, India. Int. J. Curr. Microbiol. App. Sci. 7, 3488–3502. doi: 10.20546/ijcmas.2018.712.395
Verma, G., and Mathur, A. K. (2009). Effect of integrated nutrient management on active pools of soil organic matter under maize-wheat system of a Typic Haplustept. J. Indian Soc. Soil Sci 57, 317–322.
Verma, S. N., Sharma, N., and Verma, A. (2017). Effect of integrated nutrient management on growth, quality and yield of soybean (Glycine max). Ann. Plant Soil Res. 19, 372–376.
Walia, M. K., Walia, S. S., and Dhaliwal, S. S. (2010). Long-term effect of integrated nutrient Management of Properties of Typic Ustochrept after 23 cycles of an irrigated Rice (Oryza sativa L.) – wheat (Triticum aestivum L.) system. J. Sustain. Agric. 34, 724–743. doi: 10.1080/10440046.2010.507519
Wang, H., Wang, X., Bi, L., Wang, Y., Fan, J., Zhang, F., et al. (2019). Multi-objective optimization of water and fertilizer management for potato production in sandy areas of northern China based on TOPSIS. Field Crop Res 240, 55–68. doi: 10.1016/j.fcr.2019.06.005
Wheeler, T., and von Braun, J. (2013). Climate change impacts on global food security. Science 341, 508–513. doi: 10.1126/science.1239402
Witt, C., and Dobermann, A. (2004). “17 toward a decision support system for site-specific nutrient management” in Increasing product. Intensive Rice Syst. through site Rice Syst. through site-specific Nutr. Manag (Los Baños: Science Publishers, Inc.), 359.
Wu, W., and Ma, B. (2015). Integrated nutrient management (INM) for sustaining crop productivity and reducing environmental impact: a review. Sci. Total Environ. 512-513, 415–427. doi: 10.1016/j.scitotenv.2014.12.101
Yaduvanshi, N. P. S. (2017). Nutrient management for sustained crop productivity in sodic soils: a review. Soil Salin. Manag. Agric., 365–394. doi: 10.1201/9781315365992-15
Yilmaz, E., and Alagöz, Z. (2010). Effects of short-term amendments of farmyard manure on some soil properties in the Mediterranean region-Turkey. J. Food Agric. Environ. 8, 859–862.
Yuhui, Q., Zhiping, C., Baoqing, W., and Qin, X. (2004). Impact of soil fertility maintaining practice on earthworm population in low production agro-ecosystem in North China. Acta Ecol. Sin. 24, 700–705.
Keywords: agroecosystem, crop nutrition, greenhouse gas, nutrient management, soil health
Citation: Paramesh V, Mohan Kumar R, Rajanna GA, Gowda S, Nath AJ, Madival Y, Jinger D, Bhat S and Toraskar S (2023) Integrated nutrient management for improving crop yields, soil properties, and reducing greenhouse gas emissions. Front. Sustain. Food Syst. 7:1173258. doi: 10.3389/fsufs.2023.1173258
Edited by:
Andrews Opoku, Kwame Nkrumah University of Science and Technology, GhanaReviewed by:
Mavis Brempong, CSIR Crops Research Institute, GhanaBenedicta Essel Ayamba, Council for Scientific and Industrial Research (CSIR), Ghana
Rechiatu Asei, Sunyani Technical University, Ghana
Copyright © 2023 Paramesh, Mohan Kumar, Rajanna, Gowda, Nath, Madival, Jinger, Bhat and Toraskar. This is an open-access article distributed under the terms of the Creative Commons Attribution License (CC BY). The use, distribution or reproduction in other forums is permitted, provided the original author(s) and the copyright owner(s) are credited and that the original publication in this journal is cited, in accordance with accepted academic practice. No use, distribution or reproduction is permitted which does not comply with these terms.
*Correspondence: R. Mohan Kumar, mohanomkey@gmail.com; Sathish Gowda, cssg86@gmail.com