- 1Department of Forestry and Biodiversity, Tripura University, Agartala, India
- 2Regional Horticultural Research and Training Station, Dr. Y. S. Parmar University of Horticulture and Forestry, Kangra, India
- 3Department of Silviculture and Natural Resource Management (NRM), Tamil Nadu Agricultural University, Coimbatore, India
- 4Department of Silviculture and Agroforestry, Dr. Y. S. Parmar University of Horticulture and Forestry, Solan, India
- 5Division of Silviculture, Agroforestry and Forest Management, Tropical Forest Research Institute, Jabalpur, India
- 6Department of Botany, South Indian Vaniar Educational Trust College (SIVET) College, Chennai, India
- 7College of Forestry, Dr. Panjabrao Deshmukh Krishi Vidyapeeth, Akola, India
Food is a basic human requirement which sustains the dynamics of the Earth's inhabitants by satisfying hunger, providing nutrition and health, and catering to culture, tradition, and lifestyle. However, the rising global population coupled with climate change including calamities, diseases, conflicts, as well as poor agricultural practices put a huge constraint on the quantity and quality of food. Modern agriculture propelled by the green revolution has somehow been able to meet the food requirements of the ever-increasing population and is heavily dependent on chemical fertilizers, pesticides, and machinery, reducing the quality of food, and simultaneously posing a great risk of environmental quality degradation and genetic diversity reduction. The Integrated Organic Farming System (IOFS) is a novel approach that holds the potential in addressing the challenge of reconciling food production with environmental preservation. As this approach embraces zero or minimal chemical use, adopting the reprocessing and reuse of agricultural residues has led to a sustainable system that can be viewed as the closest approach to nature and a circular economy. However, certain constraints need to be addressed, such as ascertaining the effectiveness of organic fertilizers, the complexities associated with weed management, and the inadequacy of proficiency, financial resources, and technical expertise required to implement the IOFS. Therefore, this study emphasizes the comprehensive benefits that could be derived from IOFS, particularly agroforestry, including efficient food production, improved food quality, biodiversification of crops by the adoption of lesser-known crops to cater to cultural requirements and minimal capital input to achieve environmental sustainability and a carbon neutral economy.
Introduction
Agricultural systems are the foundation of human civilization, providing food, fiber, and fuel (Sharma U. et al., 2022). However, traditional farming practices have come under scrutiny due to their negative environmental impacts, involving soil degeneration in soil, water, and air with emissions of GHGs. This steered a proliferation of sustainable farming practices that balance food production with environmental conservation. Integrated Organic Farming Systems (IOFS) is an innovative approach that offers a promising solution to this challenge. Agricultural systems must have multi-functionality to achieve food security, economic gains, social functions, and environmental sustainability (Groenfeldt, 2005). In addition, an increase in the number of components and functions tends to enhance the stability of the food production and land use system (Price, 2000).
In the Indian context, IOFS is a novel concept of on-farm resource management strategy developed under the scheme (AL-NPOF)1 All India Network Program on Organic Farming (IIFSR-ICAR, 2014), designed to realize remunerative and sustained agricultural out-turn to satisfy the heterogeneous needs of the farmers as well as the consumers. IIFSR-ICAR (2016) developed the IOFS model for Meghalaya and Tamil Nadu. The model for Meghalaya is [(Cereals + legumes + vegetable crops +fruits + fodder) + Dairy (1 cow + 1 calf) + fishery]. While the IOFS model for Tamil Nadu is [(Green manure-cotton-sorghum; okra + cilantro-corn + cowpea (fodder), Desmanthus, 1 dairy cow with heifer and young bull + biofence of Gliricidia sepium and coconut)].2
IOFS is a holistic approach that integrates various agricultural practices, such as crop cultivation, livestock rearing, and tree farming, in a synergistic manner. An integral feature of an IOFS involves two fundamental attributes: residue recycling, whereby the waste or byproducts produced by one constituent are utilized as inputs for the other constituent, and improved land-use efficiency, i.e., two subsystems occupying a portion, or the entirety of the space required for each subsystem (Paramesh et al., 2022). The goal is to maximize resource utilization and reduce waste, leading to improved soil health, biodiversity, and enhanced ecosystem services. IOFS also emphasizes the application of organic resources and minimizes the application of chemical pesticides and fertilizers, promoting soil fertility with the reduction of environmental pollution. Several authors outline the benefits of IOFS for food production and environmental sustainability (Garima et al., 2021; Sharma U. et al., 2022; Kumar et al., 2023; Verma et al., 2023a,b).
For instance, a study conducted in India found that IOFS increased crop yields, reduced input costs, and improved soil quality compared to conventional farming practices (Das et al., 2017). Another study in China showed that IOFS improved soil organic matter content, reduced nitrogen losses, and increased biodiversity (Chen et al., 2019). Thus, the adoption of IOFS has the capacity to propel toward food and nutritional soundness through the systematic employment of available assets and the incorporation of essential components such as agricultural crops (Altieri et al., 2012; Wezel et al., 2014). However, understanding the distinct functions performed by different elements of IFOS is crucial in catering to the unique requirements of smallholder farmers, as this knowledge is pivotal in fulfilling the dietary and sustenance needs of agricultural households. Simultaneously, due to the intricate nature pertaining to the projected growth of food and nutritional demand, it is imperative to recognize the paramount significance of region-specific IFOS systems throughout the globe. These IFS initiatives play a pivotal role in effectively catering to and satiating the demand, thereby assuming a critical position in the overall food security framework (Paramesh et al., 2022).
Specialized agroecosystems tailored to cope with climatic stress are vital to expedite food security and sustenance. IOFS also has the prospect to attenuate climate change by impounding carbon into soil and limiting GHG emissions. The carbon captured by primary producers during photosynthesis practically nurtures each ecological consumer-resource system by serving as the channel for the transmission of solar energy in the biosphere (Pelletier et al., 2011). A study in Italy showed that IOFS had a lower carbon footprint than conventional farming practices due to reduced use of synthetic fertilizers and lower energy consumption (Chiriacò et al., 2017). Similarly, an investigation in China reported that IOFS minimized greenhouse gas emissions by 13.4% compared to conventional farming practices (Zhou et al., 2019). If all agricultural systems adopt IOFS, the oversight in manufacturing synthetic fertilizer and application can curtail agricultural emissions by about 20%, of which 10% will be due to reduced NO2 release while about 10% to low energy requirement (Niggli et al., 2009). Furthermore, these emissions reduction is again complemented by emission reparation by carbon sequestration of around 40–72% of the annual greenhouse gas emitted from food production systems. Overall, Barbosa et al. (2015) expressed that the adoption of IFOS presents a viable and encouraging approach for reducing greenhouse gas (GHG) emissions and curtailing nutrient loss. The optimization of available resources is achieved by implementing improved nutrient recycling techniques and utilizing crop residues as animal feed.
Despite the potential benefits of IOFS, its adoption has been limited due to several barriers, including a lack of knowledge and technical expertise, inadequate policy and institutional support, financial, sociocultural, and biophysical constraints, and market demand. Specifying the finitude of energy capital supporting food logistics combined with a restricted range of ecosystems to appropriate residues from energy production along with conversions, the ramifications for food systems deserve critical deliberation (Pelletier et al., 2008; Paramesh et al., 2022). Therefore, there is a need to undertake research and development in IOFS to universalize adoption. This review article intends to provide a conspectus of IOFS and its potential benefits for food production and environmental sustainability. The article will discuss the key principles of IOFS, its potential benefits and limitations, and the current state of research and development in this field. The article will also highlight the guide to the conversion of agrisystems to IOFS that can promote the adoption of IOFS and the challenges that need to be addressed.
Approached methodology
Data collection
For identifying relevant studies for this review, we carried out a systematic search of electronic databases, including PubMed, Scopus, Web of Science, and Google Scholar, using the following keywords: “Integrated organic farming,” “Organic farming systems,” “Sustainable agriculture,” “Food production,” and “Environmental sustainability.” We also hand-searched the reference lists of selected articles for additional relevant studies. The search encompasses appropriate literary materials from as early as 1713 to February 2023.
Data analysis
The authors TS and LP individualistically partitioned the titles and abstracts of the articles identified to assess their relevance to the review topic. The full texts of potential articles were obtained and evaluated for eligibility. Eligible studies were those that reported on the implementation and outcomes of integrated organic farming systems in different regions of the world. Overall, this review article provides a meticulous audit of integrated organic farming systems with their potential to provide a sustainable and efficient approach to food production and mitigate environmental impact.
The crisis and constraints in food production and food security
Before the beginning of the 19th century, the human population's growth was relatively slow. It took over 2 million years to reach the 1 billion milestone (Population Division, UN); now, the worldwide total population is about 8 billion (countrymeters.info). UNDESA (2021) forecasted that there will be 9.6 billion people by 2050, and providing food for humans sustainably will become a goliath task as total food production volumes are expected to increase appreciably through 2050 to nourish the population (FAO, 2006; Godfray et al., 2010). Taking 2000 as the base year, the FAO (2011) projected that it is mandatory to surge food production by 70% globally and 100% in cash-strapped countries. Sustainable nourishment of the human population is an impending obstacle as resources are finite.
The rapid growth of the human population in such a short period, combined with natural calamities, poor agricultural practices, and World Wars I and II, has put a mammoth impediment on the food supply, causing widespread famine and malnutrition. The end of the Second World War marked the emergence of several agricultural improvements termed the Green Revolution (Gaud, 1968), which was hastened by the efforts of Dr. Norman Borlaug through the creation of HYVs along with disease-resistant varieties of cereals especially wheat and rice which have been genetically modified from the purebred (Borlaug, 1953). The introduction of the multiline and the intensification of agriculture practices have produced huge agricultural output and saved a billion people from starvation (Easterbrook, 1997). Specialization of agriculture with a focus on incremental production was undertaken with reparations of agroecological principles including crop diversity, especially pulses that could reduce synthetic inputs (Watson et al., 2017).
Reliance on a few crops has resulted in a narrow genetic base, and diseases combined with hanging environmental scenarios such as land degradation and climate change have made such crops susceptible to devastation. Mention may be made of the Irish potato famine of the 1840s, where one in eight Irish people died of starvation as the potato was their main staple, and potato blight disease caused major crop failure. Moreover, the Southern Corn Leaf Blight in 1970 due to an attack of race T of fungus Helminthosporium maydis on the Southern and Mid-West US Corn Belt brought frantic turmoil in the economy of America as 15% of the crop was wiped out. The farmers and lenders who suffered the ripple effect of the blight had to endure the consequences of trying to recover from their losses (Doyle, 1985). During the 1980s in California, USA, 2 million acres of grape vines were replanted in Napa Valley as the same high-yielding clonal variety was unable to withstand attacks by aphid (Phylloxera vastatrix), which had devastated England and Europe from the grafts brought from California in the late 19th Century (New York Times, 1985). These have led to an economic breakdown, as depicted in Figure 1.
To discourse the issue of food insecurity in several regions by famine, the first-ever World Food Summit of 1974 convened by FAO promulgated that every human has the absolute right to be free from hunger and malnutrition to develop their physical and mental faculties (UN, 1975). In subsequent years, concerted efforts from multiple agencies and governments have discussed the issue of food insufficiency to some extent. However, most of these movements have been incremental that have failed to bring about a transformative mode of agroecological approaches considering the dynamics and interactions with the cropping systems, producer, and consumer (Rosati et al., 2021).
Conventional modern agricultural practices have also been criticized for being intensive and having high dependence on non-renewable resources, chemicals, and fuels (Carson, 1962; Pimentel, 1996; Geiger et al., 2010; Winqvist et al., 2012), degradation of land and water resources (Carson, 1962; Pimentel, 1996; Winqvist et al., 2012; Petrosillo et al., 2023), negative impacts on the native bio-resources through reliance on a few staples (Jennings, 1988; Fischer et al., 2014; Petrosillo et al., 2023) along with the higher output of greenhouse gases especially methane (UNDESA, 2021), and compromised nutritional quality (Sands et al., 2009). The input on agriculture to bring about enough food for all is so high that 70% of global freshwater withdrawals from both groundwater and freshwater are attributed to the irrigation of farmlands or processing of food produces (UNESCO, 2020). And an input of over 110 million tons of chemical fertilizers was made in the year 2018 (UNDESA, 2021). Kissinger et al. (2012) have attributed 80% of deforestation to agricultural land use. This projection was estimated using FAOSTAT (2009) deforestation values of 2005–2009 as a base, corresponding to the change in cropland areas in each country. The resulting ratio was furthermore employed to estimate deforestation values from area changes in the outlay. Meanwhile, half of the world's terrestrial habitable lands have been utilized for agricultural purposes, involving the conversion of huge areas (about one-third) of global forest lands (FAO, 2020a). The conversion of forests and thereby change in LULC to agroecosystems additionally poses a huge risk of loss of local biodiversity through habitat fragmentation and alteration (Gibbs et al., 2010).
AFOLU accounts for 25% of global human-induced GHG emissions (Ripple et al., 2014; Smith et al., 2014) of which 14.5% is contributed from livestock production. As specified by the IPCC, the agricultural industry was responsible for emitting an estimated annual quantity of greenhouse gases ranging from 5.1 to 6.1 gigatonnes of CO2 (Barker et al., 2007). The estimate which does not include emissions coming from post-harvest processing and distribution as the greatest consumption of energy and emission of GHGs in the food system is regional and global food trade upshot “food miles” (Pretty et al., 2005).
Moreover, conventional modern farming practices exacerbate soil quality degradation through topsoil runoff and loss of SOM, forbidding the future sustainability of crop production already facing peril under extreme climatic events (Pimentel et al., 1995; Ashby, 2001; Gomiero et al., 2011; Porter et al., 2014; Petrosillo et al., 2023). There is a strong correlation between agriculture and climate change as agriculture is very much responsible for producing GHGs, and prevailing climatic conditions highly influence the success of an agricultural food production system. Climate change-associated events such as rising temperatures, erroneous precipitation patterns along with the increased frequency of extreme weather occasions imperil agricultural fecundity, the feasibility of farming spiraling vulnerability, and ultimately leading to food insecurity (FAO, 2007).
Food security is indispensable to achieving sustainable development (Popkova and Shi, 2022; Sharma U. et al., 2022) especially MDG1-Hunger, i.e., Eradicate extreme poverty and hunger. But if people engaged in the agricultural sector do not strive to “ensure environmental sustainability”, i.e., MDG7- Environment, the efforts to increase food production are unsustainable (Rosati et al., 2021). Regrettably, the higher frequency of occurrence of climate change-associated hydro-meteorological uncertainties combined with rising global temperatures is exacerbating the existing food crisis (FAO, 2017). The predominant subsistence of over 60% of the population in Africa's Sub-Sahara region including 40–50% in Asia-Pacific regions is projected to be from the agriculture sector till 2030 (ILO, 2007). IPCC (2007) has also stated that by 2050 all global agroecosystems, inclusive of croplands, pastures, and meadows in temperate areas, are contemplated to be strained due to climate change. These can be supported by several reports of predictions on climate impacts and risks on agroecosystems by climate change through Table 1.
Additionally, The State of Food Security and Nutrition in the World 2020 and 2021 (FAO, 2020a; FAO et al., 2020) has pointed out that the world is “not on track” to realize Zero Hunger by 2030. As per this report, nearly 690 million people are hungry, ~8.9% of the total human population. It has also been highlighted that a global pandemic like COVID-19 can put pressure on health and nutritional security as the pandemic has disrupted production, supply chain, and adequate and appropriate food consumption (WHO, 2020).
Integrated organic farming systems and circular -organo-agroforestry practices
Existing sustainable land use/farming practices
Organic farming system
“Organic agriculture promotes and enhances agro-ecosystem health, through a holistic production management system by emphasize the use of management practices in preference to using off-farm inputs, considering the use of locally adapted systems at regional level. This is carried out by means of, possible, agronomic, biological, and mechanical methods, as opposed to using synthetic materials, to fulfill any specific function within the system.” (FAO, 1999; IFOAM, 2002). Organic farming strives to eliminate the dependence on chemical inputs and, since being labor intensive, provides rural employment and development opportunities. IFOAM (2010, 2020b) recognizes, “Organic Agriculture as the producing system which sustains the health of soils, ecosystems, and people”. These farming practices rely on the interactions between the components, promoting fairness of all life forms involved with the environment. It is distinguished by the prohibition of the input of chemical fertilizers and pesticides, particularly emphasizing soil health and cropping patterns, nutrient transfers, and oscillations within the agroecosystem (IFOAM, 2012). According to Pretty and Ball (2001), sustainable and organic systems in the United States demonstrated comparable yields to high-input industrialized systems while consuming 22–120% less energy. Simultaneously, according to several studies (Muller et al., 2012; Pimentel and Burgess, 2014), organic farming systems have been found to consume 20–50% less energy as compared to conventional farming systems (Zikeli et al., 2014). Moreover, the worldwide implementation of organic farming practices has the capacity to sequester an amount of greenhouse gas emissions equivalent to 32% of the total current human-induced emissions (Jordan et al., 2009; Zikeli et al., 2014).
Conservation agriculture (no-till agriculture)
At present, the cultivators-led transformation of agricultural land-use systems from tillage-based to conservation agriculture has garnered much thrust as a novel paragon for sustainable intensification in food production (Kassam et al., 2015). FAO (2014b) defined Conservation Agriculture as the approach of managing agroecosystems for greater and continuous productivity, improved profits, and food security while preserving and enhancing the resource base and the environment. This food production system is characterized by zero or minimal tool of soil to avoid disturbance of soil from cultivation, upkeep of permanent organic mulch over the soil with residues, crops, and cover crops, and enhanced crop diversity through mixing and sequencing of rotations especially of legume along non-legume. Kassam et al. (2015) have further added that CA can be complemented by the use of quality seeds, integrating management of water, pests, and soil nutrient. This particular land-use system can be executed on all land dimensions from an acre to some hectares (FAO, 2014c) and allows for maximum utilization of soil's ecosystem services with the use of locally adapted species. Simultaneously, conservative agricultural practices improve food accessibility and enhance the plasticity of the cropping systems against the perils of climate systems (Kassam et al., 2009; Friedrich et al., 2012; Farooq and Siddique, 2014; Jat et al., 2014; Sharma et al., 2020; Joshi et al., 2021; Sharma P. et al., 2022).
Natural farming system
In the Indian context, natural farming, an emerging practice, is providing new opportunities for efficient food production and environmental sustainability. The natural farming approach is a diversified farming system that incorporates crops, trees, and livestock, with a focus on functional biodiversity (Rosset and Martínez-Torres, 2012). According to Palekar (2005), the implementation of intercropping and mulching techniques, along with the substitution of chemical fertilizers and pesticides with locally produced alternatives such as Jeevamritham, Beejamritham, and Neemastra, results in a noteworthy reduction in production costs. Natural farming, which can also be referred to either as Eco-Agriculture or Eco-friendly Agriculture, is a modern methodology that seeks to improve both conventional and contemporary agricultural techniques while prioritizing the conservation of the environment, public health, and local communities (Mishra, 2013). Natural farming is a concept that is rooted in the principles of Masanobu Fukuoka, a Japanese farmer. It is based on the belief that it is essential to align oneself with the natural cycles and processes that exist in the environment. The potential effects of the extensive adoption of natural farming on greenhouse gas emissions are currently unclear. The anticipated outcome is a reduction in agricultural emissions per unit of land through the mitigation of fuel consumption, emissions from manufactured inputs, and N2O emissions resulting from a decrease in fertilizer use. According to CSTEP (2020), the implementation of natural farming systems has resulted in notable reductions in water consumption (50–60%), input energy (45–70%), and greenhouse gas emissions (55–85%). Similarly, Rosenstock et al. (2020) and Lohith et al. (2021) as well relayed a significant reduction in the GHG emission ranging from 23 to 60%.
Integrated farming system
Efforts for improvization of agricultural methods to increase food production and enhanced income from the farm has led many smallholder farmers to explore the integration of animal husbandry into traditional crop-based agricultural practices. Such efforts for efficient land use, to achieve sustenance and sustainability where the byproduct of one component became the input for another component and vice versa came to be known as Integrated Farming Systems. Several authors have defined IFS (Jayanthi et al., 2000; Singh and Ratan, 2009; Panke et al., 2010) but the core rationale of the definitions provided are maintenance of cropping patterns and combination along with optimal utilization of resources that accords in food security with income generation (FAO, 2001; Tipraqsa et al., 2007; Sasikala et al., 2015; Kumar et al., 2023). However, it ought to be reminded that the contradistinction between the integrated farming system and the conventional intensive farming system is not outright but is rather a matter of the degree of integration of resources in the farming practices (Tipraqsa, 2006) and hence environmental sustainability of IFS is not well-established.
Agroforestry
Agroforestry can be defined as the system of land usage or acreage where arboraceous perennials, such as trees, are raised along with herbaceous food crops in the same plot or piece of land that result in significant ecological interactions that further aid in maximizing financial returns to the practitioners (Young, 2002). In Lundgren's “Agroforestry Systems' (Lundgren, 1982), Agroforestry was defined as “a collective name for various land-use systems in which woody perennials (trees, shrubs, etc.) are grown in combination with herbaceous plants (crops, pasture) or livestock, in a spatial arrangement, a rotation, or both.” Agroforestry systems provide the optimum output of food crops and animal husbandry, even in the face of shortages in wood produce (Mantel, 1990). FAO (2004) states that, “organic agriculture and sustainable forest management produce commodities and build self-generating food systems and connectedness between protected areas.” The ubiquitous augmentation of these perspectives and their incorporation in landscape design is supposedly a cost-effective policy alternative for biodiversity conservation. A report of trial investigations by Ramesh et al. (2022) revealed that the inclusion of fodder in conjunction with the fodder trees is highly productive and could generate more effective farm income along with feed for livestock components.
The true essence of agroforestry lies in the anticipated position of on-farm and off-farm production from perennial woody components for facilitating sustainable land use and primal resources and maximizing economic returns for the farmers, which may be summarized as enhancing connectivity and stability among biodiversity, forests, landscape, and watershed (FAO, 2010; Nair, 2011; Murthy et al., 2016). An appropriate agroforestry system that usually follows organic norms can check against soil erosion with the help of the tree component and crop cover, maintain soil organic matter and augment nitrogen build-up through soil microbes and nitrogen-fixing species, and efficiently cycling of nutrients and energy within the system (Patiram and Bhaudauria, 2003).
Conforming and transforming agriculture systems to organic
Several organizations have given recommendations and suggestions to conform traditional and other sustainable agriculture systems to purely organic food production systems through conversion practices. PGS (Participatory Guarantee System) of the IFOAM provides an alternative to third-party certification systems (characterized by complicated regulatory procedures) for better on-farm practice and marketing strategies for smallholder agriculture practitioners such that food produce by such practices is guaranteed giving consumer satisfaction (IFOAM, 2020a). PGS allows for purchasers and growers assent on the circumstances in existing local food systems and the scheme; these are substantiated through the direct participation of all stakeholders especially in the options and descriptions of the norms along with the review and decision process to recognize farmers as organic. This system ensures transparency and integrity (IFOAM, 2009), that is, particularly adapted to local contexts and short supply chains, and which is sometimes referred to as “participatory certification” (IFOAM, 2020a). Detailed step-by-step conversion from existing food production land use to 100% organic production system especially applicable to larger conventional agricultural practices can be achieved by following FAO/TECA ID 8364 Step-by-step conversion to organic agriculture (FAO, 2020b). In the simplest words, it has three steps, viz., (1) Collecting information, (2) Trying out identified promising practices on a small scale, and (3) Implementing the organic practices to the entire farm. Initially intending farmers must collect reliable information from successful farmers, agriculture departments, and through websites such as those listed in Table 2. This should be followed by choosing practices that are simple and within the financial, intellectual, and technical grasp, which would also show results in short rotation/duration. These chosen practices are to be tested in designated plots within the farm and during such phase, the operation taken should be purely organic. When success is achieved, the farmer may proceed to convert the entire farm to the chosen organic practice. This allows for a certain period of observation and choice of combination of crop/livestock/woody components/horticultural crops/aquaculture/fodder grass/cover crops, etc., to be adopted in the IOFS to enhance maximum output with minimal external input. This would make preparations for sustainable food production systems that could mitigate food insecurity and are environmentally friendly.
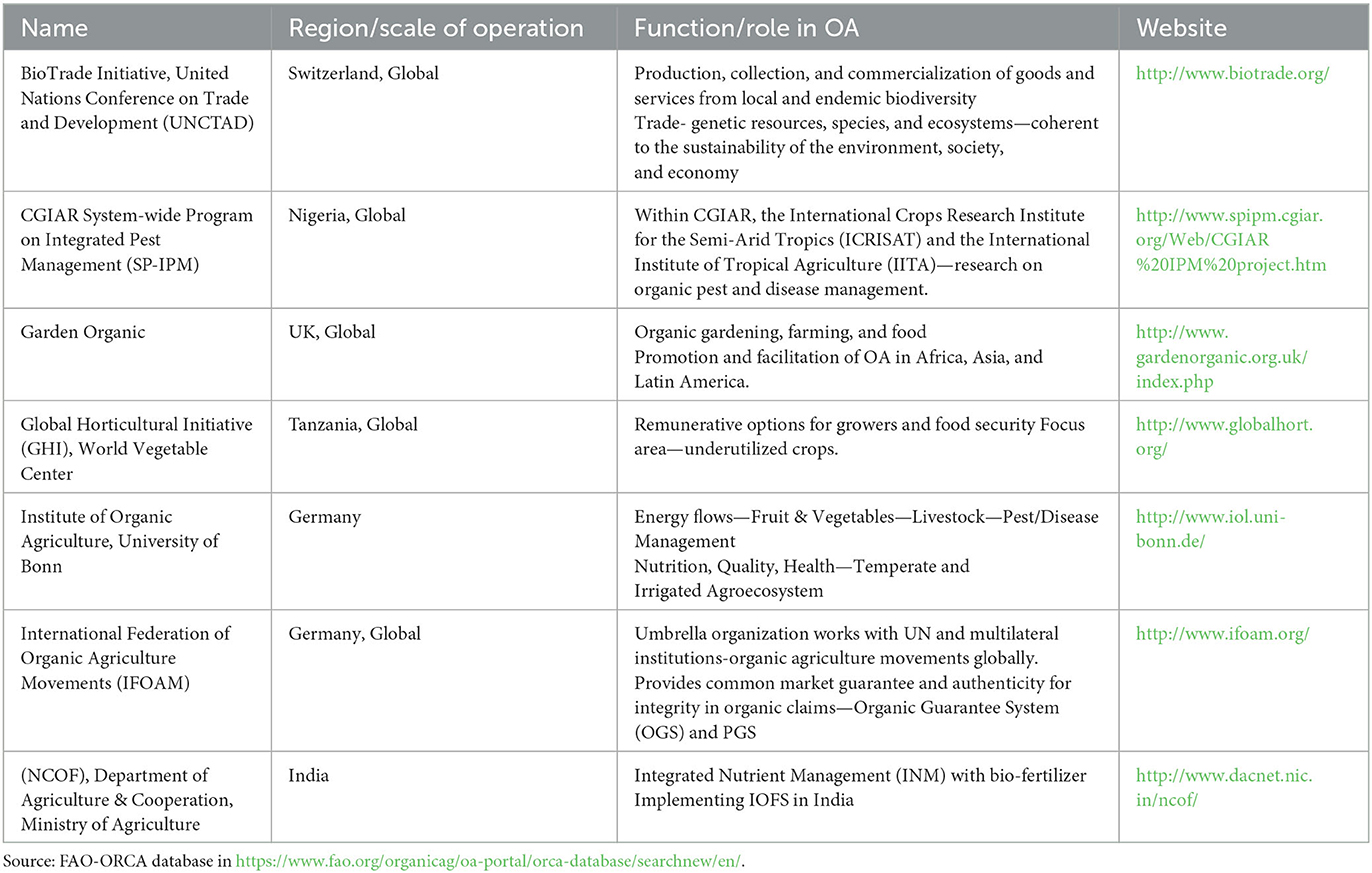
Table 2. List of prominent NGOs, other official government organizations, and academic institutions promoting OA.
Gliessman's (2015) description of the transition of agricultural systems toward sustainable agrifood systems has described five phases during the transition from conventional to sustainable agriculture systems. The first three phases operate at the agroecosystem level, while the last two phases involve more interaction between the producer and consumers, as well as involving the active participation of locals during food species selection and diversification of the agricultural systems' components. Rosati et al. (2021) have argued that despite the efforts to enhance sustainability, even organic farming systems could be just considered incremental in nature as they focus more on refusing chemical inputs and replacements by agroecological alternatives and fail to be transformative. They added that integrating agroforestry practices into organic farming systems can bring about a wholesome, sustained agroecological system inclined toward diversification of crop components with amplified interrelationships between the farm system and nature. Their argument stands true when we look at the various workers' descriptions and definitions of agroforestry, as discussed in the previous paragraph. Thus, we can safely declare that including agroforestry as part and parcel of organic farming systems has a duality in being transformative as well as incremental in nature and can address improved food security that gives a minimum cynical impact on the environmental health. The positive impact of organic practice in agriculture practice diversification, including poly-cropping, agroforestry, and integrated farming systems of crop and livestock with local varieties and breeds, produces good quality organic food. Moreover, certified organic products provide for increment in revenue for growers thereby forming a market-based incentive for ecological stewardship (Scialabba and Muller-Lindenlauf, 2010).
UNFCCC (2007) has compiled reactive and anticipatory adaptation means for Agriculture to ensure food security, which is coherent with the IOFS approach. The adaptation measures for agricultural and food security are laid out in Table 3. It is evident that adaptation for sustainability and resilience of agricultural systems may be enhanced through organic conversion, promotion of agroforestry, and water management.
Concept of circular economy and sustainability in integrated organic farming systems
Geissdoerfer et al. (2017) defined Circular Economy as “a recovering system in which resource input and waste, emission, and energy leaks are curtailed by slowing, closing and reducing material and energy loops”. The study adds that “a circular economy can be achieved through durable design, maintenance, repair, reuse, remanufacturing, refurbishing, and recycling.” An integrated organic farming system follows the 5R-concept: Reduction of inputs, Reuse agricultural residues, Recycle water, Refuse inorganic fertilizers and pesticides, and Replace conventional practices with closer to nature practices. For an efficient circular economy, agriculture should be taken as a primary sector with sustainability as its prerequisite, forming the fundamental of the economy and the living system involved (Jurgilevich et al., 2016). Agricultural systems adopting a circular approach such as IOFS circulate waste from one process to create resources for other means. The feedback-rich systems inspire the circular economy in the biosphere, that there are no wastes that would remain as wastes as decomposers process them, and the nutrient made available for use by producers is thus the system providing for itself perpetually (Pearce and Turner, 1989; Ellen MacArthur Foundation, 2013; Nattassha et al., 2020).
Circular agricultural practices as multifaceted sustainability efforts (Govindan and Hasanagic, 2018) are designed in such a way that all key elements involved in food production, from cultivation, harvesting, post-harvest activities, transportation, marketing, consumption, and disposal (Irani and Sharif, 2018) promote sustainable development through food security and sustainability across all the elements (Fassio and Minotti, 2019; Nattassha et al., 2020; UNDESA, 2021). This process can be considered to follow a rigid Cradle-to-Grave or Life Cycle-Assessment (LCA) approach as agricultural systems follow organic farming protocols with agroforestry and residue recycling that can reduce carbon emissions and efficiently deploy natural resources significantly by curtailing material and capital inputs. Integrated farming systems, when adopting the organic agricultural approaches, make the components of the farming systems synergistic (Csavas, 1992) and curtail risks, ensuring higher food production and economic profits in combination with proper utilization of organic crop residues (Radhamani et al., 2003; EC, 2012, 2014; McCarthy et al., 2019).
An ideal organic farming system assimilates the outputs or residue of one enterprise as feed for another enterprise in the confines of the same tillage (McDonough and Brungart, 2002; Argade and Wadkar, 2013). The long-term application of organic manure (Gomiero et al., 2011) in farms practicing livestock-based integrated organic farming was more stable and sustainable than conventional monoculture farming practices (Fließbach et al., 2007). The Brundtland Commission (Brundtland et al., 1987) defined sustainability as the “development that meets the wants of the current without compromising the capability of upcoming generations to meet their own needs,” and Geissdoerfer et al. (2017) have stated that the notion of sustainability has its origins in forestry. To support their statement, they have used the conceptualization of silvicultural principles of “Sylvicultura oeconomica” (Von Carlowitz, 1713), in which the amount of wood being reaped in a cycle should not transcend the regenerative capacity of the woodland. The sustainability of any system may be expressed in terms of the equation “I = P x A x T”, where I is the Environmental Impact of the system, P is Population (demographic factor), A is Affluence representing consumption, and T is Technologies involved (Holdren and Ehrlich, 1974). According to this equation, we can rate any agricultural land use system for its sustainability. Considering the parameters involved, we can conclude that Integrated Organic Farming Systems, including Agroforestry land use systems (McAdam et al., 2009), can be declared highly sustainable and ideal for substantial food production with the least environmental impact.
Thus, the integration of circular economy principles to organic agriculture system with agroforestry can be expressed with an amalgamated term “Circular-Organo-Agroforestry” as depicted with components and a model in Figures 2, 3 respectively and may be defined as “a system of agricultural farming practice which is organic and has rich species diversity which includes tree components, herb crop components and may include animal husbandry and fishery in which there are synergistic interactions between all components within the system following the principles of a circular economy that results in sufficient food production and helps in achievement of sustainable development.”
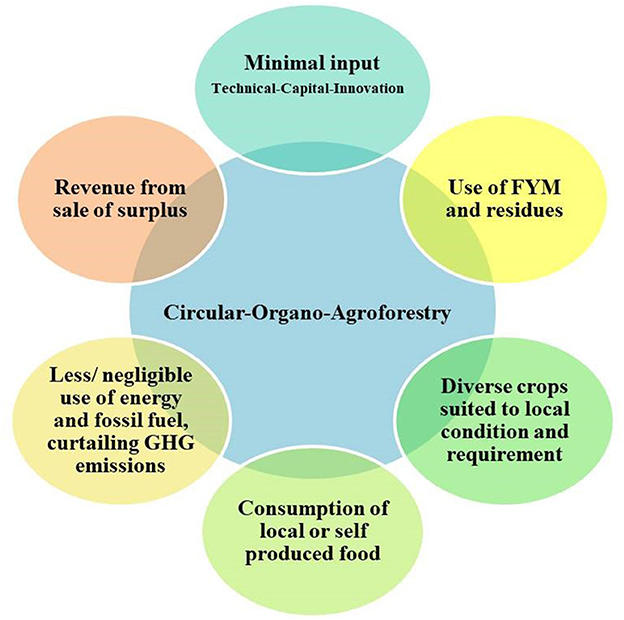
Figure 2. Components of Circular-Organo-Agroforestry to suit regional/local scenario for achieving MDGs.
Concept of food biodiversity and nutrition enrichment from organo-agroecosystem
Guidelines on Assessing Biodiverse Foods in Dietary Intake Surveys (FAO Biodiversity International, 2017) defined “food biodiversity” as “the diversity of plants, animals and other organisms used for food, covering the genetic resources within species, between species and provided by ecosystems.” A diverse diet augments the prospective of consuming sufficient nutrients essential to human health (WHO/FAO, 2003). Long-standing efforts to provide basic food for the human population have resulted in a biased focus toward more high-yielding starchy staples (Zhu et al., 2000). This further leads to dramatically simplifying landscape composition and a sharp decline in local biodiversity (Gomiero et al., 2011). This has put massive pressure on the diversity of food species from 5,000 to 70,000 plant species that have so far been documented as human food (Kunkel, 1984; Wilson, 1992; Schippmann et al., 2002, 2006), out of which almost exclusively three cereals, viz. rice, wheat, and maize, furnish 50% of the calories derived or having originated from the plant (FAO, 2015; Bailey, 2017; Futurism, 2017; FAO et al., 2021). Increased focus on the production of staples (Magrini et al., 2018) has generated reverberations in the ecological system and human wellbeing (IPES-Food, 2017). These reverberations can be attributed to the limitations of crop diversity combined with the intensification of livestock production methods (Tilman and Clark, 2014, 2015). Food security is not restricted solely to making food available and nevertheless must address the alarming sum of more than 800 million people in low-income countries suffering from chronic undernourishment and micro-nutrients deficiency (FAO, 2017), while overconsumption in high-income countries has propelled lifestyle diseases such as obesity, type II diabetes, and coronary heart disease (WHO, 2009; FAO, 2017). New solutions must develop solutions to overcome disparities in distribution and availability to food and nutrient requirements (Tilman and Clark, 2014). Healthy diets are unavailable to many people, especially the poor (FAO, 2020a), as over 3 billion people globally still get just adequate to fill their stomachs and do not have enough to purchase nutrient-rich food (FAO et al., 2020). This is very alarming as dietary guidelines that have been put forward in different regions of the world recommend a wholesome diet endowed with a variety of nutrients, which includes green leafy vegetables rich in vitamins, and micronutrients, whole grains and nuts, seeds, and pulses for proteins aside from starchy staples (Fischer and Garnett, 2016).
The Convention on Biological Diversity advocates the combination of the conservation and sustainable use of biodiversity in cross-sectoral plans to achieve sustainable development goals that include poverty eradication, adaptation/mitigation to impacts of climate change through sector-specific initiatives such as agriculture and forestry (CBD, 2011).3 The Pacific Organic Standard emphasizes biodiversity enrichment through land use by requesting properties larger than five hectares to set aside a base land ratio of 5% of the certified area for wildlife excepting that the estates practice a traditional agroforestry or poly-culture system (Secretariat of the Pacific Community, 2008; Scialabba and Muller-Lindenlauf, 2010).
FAO (2014a) defined a sustainable food system as “a food system that brings food security and nutrition for all such that the economic, social, and environmental bases to create food security and nutrition for future generations are not compromised”. The FAO's Genetic Resources for Food and Agriculture Commission provided and adopted the Voluntary Guidelines for Mainstreaming Biodiversity into Policies, Programs, and National and Regional Plans of Action on Nutrition (FAO, 2016) supporting countries for incorporation of food biodiversity to encourage knowledge, conservation, promotion, and utilization of varieties and breeds used as food, as well as wild, neglected, and underutilized, underexplored species that could result in a better state of health and nutrition in respective countries. Species such as hanza (Boscia senegalensis) are a significant source of carbohydrates, Jessenia and patawa (Oenocarpus bataua) produce high-quality edible oil, Locust bean (Parkia bicolor) is rich in protein sources, Mongogo tree (Ricinodendro rautenenii) is a source of protein and fats, Bamboo shoots (Selvan and Tripathi, 2017), edible fungus, yams, etc. are significant lesser-known wild foods in which rural/forest dwellers extensively exploit in different areas around the world (Scoones et al., 1992; Selvan, 2022). Their utilization may be seen as signs of local populations' strategy to cope with food stress, and the incorporation of wild foods into local organic agricultural practices not only fulfills increased food production but enhances diverse nutrient availability (Nurhasan et al., 2022).
According to IFOAM (2002), farmers are to ensure maintenance and improvement of agrilandscape and enhance biodiversity. Organic farming systems, including agroforestry worldwide, use species that caters to the requirements of the local populace that includes but not limited to food staple, medicinal plants, ornamentals, culturally and traditionally important species, and fuelwood species, the inclusion of species that could provide fuel and fodder which may be considered as a step forward (Rosati et al., 2021) and to be aligned with principles of certified organic farming (Maeder et al., 2002). The species and varieties incorporated into these systems have better adaptability and enhanced agroecosystem resilience to environmental factors as well as market uncertainties (Zhang and Li, 2003; Smith et al., 2007, 2014).
IOFS that include animal husbandry considers ethical concerns in the context of the wellbeing of the livestock, and hence, amidst varied animal husbandry systems, encouraging natural grazing in open pastures and meadows, inhibiting synthetic feeds fortified with vitamins, and promoting the use of organically sourced feed (Lund, 2006), which leads to improved consumer health, such as consuming meat from livestock that may contain traces of growth promoters and antibiotics from the artificial feed and injections. Greater species diversity in these systems is conceived to improve food security (Parrot et al., 2006) and enhance the systems' ability to resist shocks, thereby reducing vulnerability and increasing resilience while maintaining the stability of the natural ecosystem (Fraser et al., 2005; Whitfield et al., 2018; Sherpa, 2023).
The species selected for such land use are often tailored to the local climatic and environmental conditions and involve less technical or zero chemical input, partly due to a lack of finance, knowledge, and resources. For instance, in the Northeastern Region of India, almost all traditional farming systems are usually of agroforestry type. They are organic by default as the farmers lack technical resources and prefer to rely on their traditional methods of agriculture using farmyard manures, green manures, indigenous ways of weed and pest control, and selection of crop species or animal husbandry to cater to their local preferences (Das et al., 2018). Indeed, they practice a different type of organic agroforestry land use systems (Selvan and Kumar, 2017), one of the reasons why the food security problems in the region are not as serious. The careful selection of species by the farmers in the region has also made their land use systems advantageous and sustainable (Pretty and Bharucha, 2014). Integrated organic farming uses agrobiodiversity to bring about variety and variability to increase yields, have higher disease resistance, requires less water input, have symbiotic relationship among the components of the system, produces food that has higher nutrient value, and produces more food groups to improve health of consumers (Bailey, 2017). Thus, it results in higher species richness and a greater abundance of taxa (Hole et al., 2005).
Status of organic food production systems
An area of 72.3 m ha of agriculture is under organic operations of which Australia alone has 35.69 million Ha which is ~50% of the total; followed by Argentina at 3.69 m ha and Spain at 2.35 m ha. The fourth and fifth largest area under organic agriculture is observed in the USA (2.33 m ha) and India (2.3 m Ha). Meanwhile, Europe showed steady growth in organic agriculture possessing 23% of the total. The increase in land under organic agriculture increased 555%-fold in the last two decades, i.e., 1999–2019. The report further showed that Australia showed an increase of 200% in the last decade itself. Despite the increase in organic agriculture areas in all regions of the world, the intensification of conventional agriculture is also growing simultaneously. Entry of synthetic fertilizers and pesticides into the food production systems is showing an increasing trend globally and in all regions of the world. This trend of input Kg/Ha is shown in Table 4. An exception is seen in Oceania for Kg/Ha fertilizer use from 2000 to 2020 which is actually −13.15%. This may be attributed to the proliferation of organic cropland areas.
However, it should be noted that only 1.5% of total global agricultural land are following organic practices despite the massive increase. Liechtenstein has more than 41% of agriculture land in organic agricultural practices (Schlatter et al., 2021; Willer et al., 2021).
Furthermore, it is reported that there are above 3.1 million organic producers worldwide of which 91% are concentrated in Asia, Africa, and Europe of which major import and export share is from tropical fruits, organic vegetables, fruit drinks, etc. (Sahota, 2021). An encouraging fact is that India itself has about 1.37 million organic producers (Sahota, 2021). The total organic food retail sale exceeds 106 billion Euros in 2019. The US is the major market for organic food at 44.7 billion Euros, which is even higher than the combined market share in the European Union, i.e., 41.4 billion Euros. The 3rd biggest market in the world and Asia's largest organic food market is China at 8.5 billion Euros (Sahota, 2021).
Limitations and constraints in the adoption of IOFS
Application of organic farmyard manures and vegetative residues are often-times proven to enhance sustainable and circular utilization of resources in integrated organic farming practices. Challenges exists in determining the fertilizing efficiency of organic materials as it depends on the mineralization processes, absorption along with losses such as gaseous emissions, and leaching or runoff (Burton and Turner, 2003; Sørensen et al., 2013; Bernal, 2017). A drawback of the organic land use systems is the difficulty in controlling of weeds as weeding is done manually which is labor-intensive increasing operation costs. Meanwhile, the use of vegetative mulches and FYMs with bio/green fertilizers must be taken with proper care as some ingredients may even exhibit allelopathic effects on the crops (Bond and Grundy, 2001). Muller et al. (2017) made a conservative estimate of organic yields exhibiting a median yield gap of 25% from the data in Seufert et al. (2012)'s “Comparing the yields of organic and conventional agriculture”. Clark et al. (1998) stated that the increase of soil organic matter after the transition from conventional to organic systems occurs rather too slowly and takes too long to manifest significant positive outcomes. Adoption of novel practices of transformative agricultural practices requires interactive discourse between local farmers and researchers/advisors for better acquisition of knowledge (on the complexity of the species compositions, functions, and services) which is itself a time-consuming and critical challenge (Geels and Schot, 2007; Hauggaard-Nielsen et al., 2021). Bernal (2017) further emphasizes that the contemporary lack of data and limited research on consequences to the agroecosystems due to increased microbial activities associated with organic fertilizers may cast doubts on the sustainability and put constraints on the adoption of IOFS. Moreover, locality factors play a crucial part in ascertaining the accomplishment of any particular farming systems as what is deemed sustainable could unfortunately yield desirable outcome in a different region or even within the same region (Smolik et al., 1995). FAO (2011) has expressed concerns over adoption of integrated systems as even though incorporation of food crops, animal husbandry, and pisciculture is ordinarily considered progressive, smallholder farmers need to have sufficient proficiency, financial, technical, and labor to operate this system in ways that yield economic and environmental sustainability in the long term. These above points may make IOFS unappealing and rather be viewed as utopian efforts of food production systems for poorer countries that are facing food insecurity as the aim to increase production is of primary importance.
Conclusion and future prospects
The primary target for escalating food production deprived of consideration for the ecological aspects, or good nutrition, is responsible for the drastic degradation of the environment while at the same time causing a pandemic of non-communicable diseases (Bailey, 2017). It could also be noted that monoculture, along with conventional agriculture practices depending on few staples, has caused a reduction of crop biodiversity. It is expository to scrutinize the correlation of Circular Economy with Sustainability, which can determine any sector's inventory management, trade ideals, and innovative approaches (Geissdoerfer et al., 2017). It is impetuous to develop food production systems characterized by resilience, sustainability, and justice inept in satiating the hunger and providing healthy, nutritious food. There is a need to interlink the essence of local producer–consumer relations and the generation of farm and food waste. IFOAM (2022) realizes the actions to galvanize with market players to ardently increase the value of organic products, creates strong and fair value chains, and allows easy access to wholesome and nutritious organic food and products created from organically sourced materials for every individual. Organically managed agriculture systems are designed for the production of high-grade, nutritious food that forms the foundation for preventative health management and wellbeing (IFOAM, 2020b). Integrated Organic Farming Systems, considered equivalent to circular agriculture, aim to ameliorate rising concerns of unsustainable global food production, environmental degradation, and the peril of biodiversity loss (UNDESA, 2021). Niggli et al. (2009) emphasized that there are three strategic research priorities in agriculture food production systems (Gomiero et al., 2011), i.e., (1) feasible options in empowerment of rural economy on a local, regional, and global scale; (2) safeguarding agroecosystems employing eco-functional agricultural consolidation; and (3) high-quality foods, a foundation for healthy and balanced diets, and a requisite for uplifting quality of life. It should be noted that Organic Agriculture practice is created on the four principles of Health, Ecology, Fairness, and Care (IFOAM, 2020b). All agriculture food producers should prioritize the utilization of organic agriculture diligently in panoramic policy solutions to exigency in climate change, biodiversity loss and food security, and relocate public grants to sustainable farming practices (IFOAM, 2022).
Meanwhile, farmers as well as researchers may explore various other organic agriculture and agroforestry practices such as Biodynamic agriculture, Regenerative agroforestry practices such as Permaculture, and Syntropic agriculture. A systematic step-by-step process of conversion of existing agricultural land use system to IOFS is depicted in Figure 4. These practices take a closer to nature approach by mimicking natural ecological processes in the acreage. In a particular agroforestry system called the Miyawaki, system the plots of urban areas are converted to natural forests using native species. This system utilizes the soil's ecosystem service to the fullest by growing several species in confined spaces where the crops grow rapidly in height while competing for sunlight. Any local fruit-bearing species or crops may be used to provide fertilizer and pesticide-free food options for the urban population. In addition, the mentioned farming strategies are practiced without synthetic inputs and emphasized the proper management and cycling of residues within the farming systems, sustaining the farmers and producing food for local markets. Farmers may follow any organic practices that are suited to farmer's existing conditions, integrating crop, livestock, trees, and fishponds accordingly.
Linkages and interactions between the various component species can considerably improve the sustainability of farming systems (Kumar and Jain, 2005). IOFS is an innovative approach that offers a promising solution for sustainable food production and environmental conservation. Its adoption can succor the global challenges of food security worsened by climate change and environmental degradation. The linear system of an economy based on a take–make–consume and dispose of pattern can only be done away with the adoption of Circular-Organo-Agroforestry practices. However, its implementation requires concerted efforts from various stakeholders, including farmers, policymakers, and researchers, to overcome the existing barriers and promote its adoption at a larger scale through financial and revival of traditional organic practices with technical assistance within a framework that rewards farms as multifunctional systems (Vermeulen et al., 2018; Lipper et al., 2022). Bernal (2017) has also stated that the scientific community needs to develop treatment technologies for ensuring nutrients (N and P), enhancing environmental conservation and sustainability and ensuring food safety as improvization from directly using manures in traditional methods.
More research and efforts need to be encouraged in looking for sustainable food production systems that are simultaneously low-cost and provide food security in the local, regional, and global context while attempting to achieve carbon neutrality from the food production systems. A more inclusive form of agriculture system that caters to local requirements and has a shorter supply chain, as well as producing minimum waste, is what the world requires at the moment. Integrated Organic Farming Systems seem to be the right choice of land use that could fulfill all these requirements.
Author contributions
TS and LP: conceptualization, investigation, analysis, writing original draft, and project administration. TS, LP, KM, VG, KR, DB, CT, DKa, PS, RD, DKu, and HD: methodology, writing review, and editing. All authors contributed to the article and approved the submitted version.
Conflict of interest
The authors declare that the research was conducted in the absence of any commercial or financial relationships that could be construed as a potential conflict of interest.
Publisher's note
All claims expressed in this article are solely those of the authors and do not necessarily represent those of their affiliated organizations, or those of the publisher, the editors and the reviewers. Any product that may be evaluated in this article, or claim that may be made by its manufacturer, is not guaranteed or endorsed by the publisher.
Footnotes
1. ^https://iifsr.icar.gov.in/icar-iifsr/npof/
2. ^https://iifsr.icar.gov.in/icar-iifsr/npof/data/uploads/files/Tecnologies%20developed.pdf
References
Altieri, M. A., Funes-Monzote, F. R., and Petersen, P. (2012). Agroecologically efficient agricultural systems for smallholder farmers: contributions to food sovereignty. Agron. Sustain. Dev. 32, 1–13. doi: 10.1007/s13593-011-0065-6
Argade, S. D., and Wadkar, S. K. (2013). Sustainable rural livelihoods security through integrated farming systems-a review. Agric. Rev. 34, 207–215. doi: 10.5958/j.0976-0741.34.3.005
Ashby, J. A. (2001). Integrating research on food and the environment: an exit strategy from the rational fool syndrome in agricultural science. Conserv. Ecol. 5, 20. doi: 10.5751/ES-00296-050220
Bailey, A. (2017). Mainstreaming Agrobiodiversity in Sustainable Food Systems: Scientific Foundations for an Agrobiodiversity Index. - Summary. Rome: Bioversity International. Available online at: https://hdl.handle.net/10568/80360 (accessed May 16, 2022).
Barbosa, F. A., Soares Filho, B. S., Merry, F. D., de Oliveira Azevedo, H., Costa, W. L. S., Coe, M. T., et al. (2015). Cenários Para Apecuária de Corteamazônica. Belo Horizonte: IGC/UFMG, 1–154.
Barker, T. I., Bashmakov, L., Bernstein, J. E., Bogner, P. R., Bosch, R., Dave, O. R., et al. (2007). “Technical summary in climate change mitigation,” in Contribution of Working Group III to the Fourth Assessment Report of the Intergovernmental Panel on Climate Change, eds B. Metz, O. R. Davidson, P. R. Bosch, R. Dave, and L. A. Meyer (Cambridge; New York, NY: Cambridge University Press). Available online at: http://www.mnp.nl/ipcc/pages_media/FAR4docs/final_pdfs_ar4/TS.pdf (accessed June 12, 2023).
Bernal, M. P. (2017). Grand challenges in waste management in agro-ecosystems. Front. Sustain. Food Syst. 1, 1–4. doi: 10.3389/fsufs.2017.00001
Boko, M., Niang, I., Nyong, A., Vogel, C., Githeko, A., Medany, M., et al. (2007). “Africa,” in Climate Change 2007: Impacts, Adaptation and Vulnerability. Contribution of Working Group II to the Fourth Assessment Report of the Intergovernmental Panel on Climate Change, eds M. L. Parry, O. F. Canziani, J. P. Palutik, P. J. van der Linden, and C. E. Hanson (Cambridge: Cambridge University Press), 433–467.
Bond, W., and Grundy, A. C. (2001). Non-chemical weed management in organic farming systems. Weed Res. 41, 383–405. doi: 10.1046/j.1365-3180.2001.00246.x
Borlaug, N. E. (1953). New approach to the breeding of wheat varieties resistant to Puccinia graministritici. Phytopathology 43, 467.
Brundtland, G. H., Khalid, M., Agnelli, S., Al-Athel, S. A., Chidzero, B., Fadika, L. M., et al (1987). Our Common Future: Report of the 1987. World Commission on Environment and Development, United Nations, Oslo. Oxford: Oxford University Press. Available online at: https://repositorio.usp.br/item/000764409 (accessed June 5, 2022).
Burton, C., and Turner, C. (2003). Manure Management: Treatment Strategies for Sustainable Agriculture. Bedford: Silsoe Research Institute, Lister & Durling Printers. Available online at: https://researchonline.gcu.ac.uk/en/publications/manure-management-treatment-strategies-for-sustainable-agriculture (accessed May 30, 2023).
CBD (2011). NBSAP Training Modules Version 2.1 – Module 3. Mainstreaming Biodiversity Into National Sectoral and Cross-Sectoral Strategies, Policies, Plans and Programs. Montreal: Convention on Biological Diversity. Available online at: https://www.cbd.int/doc/training/nbsap/b1-train-intro-nbsap-revised-en.pdf (accessed August 29, 2022).
Chen, J., Zhu, R., Zhang, Q., Kong, X., and Sun, D. (2019). Reduced-tillage management enhances soil properties and crop yields in a alfalfa-corn rotation: case study of the Songnen Plain, China. Sci. Rep. 9, 1–10. doi: 10.1038/s41598-019-53602-7
Chiriacò, M. V., Grossi, G., Castaldi, S., and Valentini, R. (2017). The contribution to climate changeof the organic versus conventional wheat farming: a case study on the carbon footprint of whole meal bread production in Italy. J. Clean. Prod. 153, 309–319. doi: 10.1016/j.jclepro.2017.03.111
Christensen, J. H., Hewitson, B., Busuioc, A., Chen, A., Gao, X., Held, I., et al. (2007). “Regional climate projections,” in Climate Change 2007: The Physical Science Basis. Contribution of Working Group I to the Fourth Assessment Report of the Intergovernmental Panel on Climate Change, eds S. Solomon, D. Qin, M. Manning, Z. Chen, M. Marquis, K. B. Averyt, et al. (Cambridge; New York, NY: Cambridge University Press).
Clark, M. S., Horwath, W. R., Shennan, C., and Scow, K. M. (1998). Changes in soil chemical properties resulting from organic and low-input farming practices. Agron. J. 90, 662–671. doi: 10.2134/agronj1998.00021962009000050016x
Cruz, R. V., Harasawa, H., Lal, M., Wu, S., Anokhin, Y., Punsalmaa, B., et al. (2007). “Asia,” in Climate Change 2007: Impacts, Adaptation and Vulnerability. Contribution of Working Group II to the Fourth Assessment Report of the Intergovernmental Panel on Climate Change, eds M. L. Parry, O. F. Canziani, J. P. Palutik, P. J. van der Linden, and C. E. Hanson (Cambridge: Cambridge University Press), 469–506.
Csavas, I. (1992). “Regional review on livestock-fish production systems in Asia.” in Proceedings of the FAO/IPT Workshop on Integrated Livestock-Fish Production Systems, eds. T. K. Mukherjee, P. S. Moi, J. M. Panandam, and Y. S. Yang (Kuala Lumpur: Institute of Advance Studies, University of Malaya), 16–20 Available online at: https://www.fao.org/3/ac155E/AC155E05.htm (accessed May 26, 2022).
CSTEP (2020). Life Cycle Assessment of ZBNF and Non-ZBNF: A Study in Andhra Pradesh. Karnataka: Center For Study of Science, Technology & Policy.
Das, A., Layek, J., Ramkrushna, G. I., and Babu, S. (2018). “Integrated organic farming systems in 344 North East India”, in Conservation Agriculture for Advancing Food Security in Changing Climate (Adaptive Strategy, Resource Productivity and Economic Appraisal) eds A. Das, K. P. Mohapatra, S. V. Ngachan, A. S. Panwar, D. J. Rajkhowa, G. I. Ram krushna, et al. (New Delhi: Today & Tomorrow's Printers and Publishers), 301–318.
Das, A., Patel, D. P., Kumar, M., Ramkrushna, G. I., Mukherjee, A., Layek, J., et al. (2017). Impact of seven years of organic farming on soil and produce quality and crop yields in eastern Himalayas, India. Agric. Ecosyst. Environ. 236, 142–153. doi: 10.1016/j.agee.2016.09.007
Doyle, J. (1985). Altered Harvest: Agriculture, Genetics, and the Fate of the World's Food Supply. New York, NY: Viking Press.
Easterbrook, G. (1997). “Forgotten benefactor of humanity”. The Atlantic 397 Monthly, 74–8. Available online at: https://www.theatlantic.com/magazine/archive/1997/01/forgotten-benefactor-of-humanity/306101/ (accessed June 12, 2023).
EC (2012). “Innovating for sustainable growth: a bioeconomy for Europe,” in Communication from the Commission to the European Parliament, the Council, the European Economic and Social Committee and the Committee of The Regions (COM) (Brussels), 60.
EC (2014). “Towards a circular economy: a zero waste programme for Europe,” in Communication from the Commission to the European Parliament, the Council, the European Economic and Social Committee and the Committee of the Regions (COM) (Brussels), 398.
Ellen MacArthur Foundation (2013). Towards the Circular Economy: Economic and Business Rationale for an Accelerated Transition. Ellen MacArthur Foundation. Available online at: https://www.ellenmacarthurfoundation.org/assets/downloads/publications/Ellen-MacArthur-Foundation-Towards-the-Circular-Economy-vol.1.pdf (accessed June 12, 2023).
FAO (1999). Report of fifteenth session of Committee on Agriculture. Rome: Food and Agriculture Organization. Available online at: https://www.fao.org/unfao/bodies/Coag/coag15/X0226E.htm (accessed May 24, 2022)
FAO (2001). Mixed Crop-Livestock Farming: A Review of Traditional Technologies Based on Literature and Field Experience. Animal Production and Health Papers, 152. Rome: FAO. Available online at: https://www.fao.org/3/Y0501E/y0501e00.htm (accessed June 12, 2023).
FAO (2004). The Scope of Organic Agriculture, Sustainable Forest Management and Ecoforestry in Protected Area Management. Rome: FAO. Available online at: http://www.fao.org/docrep/007/y5558e/y5558e00.htm#toc (accessed June 12, 2023).
FAO (2006). World Agriculture Towards 2030-2050. Prospects for Food, Nutrition, Agriculture and Major Commodity Groups. Rome. Available online at: https://www.fao.org/3/a0607e/a0607e00.pdf (accessed June 12, 2023).
FAO (2007). Adaptation to Climate Change in Agriculture, Forestry and Fisheries: Perspective, Framework and Priorities. Rome: FAO. Available online at: https://www.fao.org/documents/card/fr/c/1be37c69-0147-44ea-af0b-d1487e223962/ (accessed June 13, 2023).
FAO (2010). Climate Smart Agriculture: Policies, Practices and Financing for Food Security, Adaptation and Mitigation. Rome: Food and Agriculture Organization. Available online at: https://www.fao.org/3/i1881e/i1881e00.htm (accessed May 24, 2022).
FAO (2011). Save and Grow, a Policymaker's Guide to Sustainable Intensification of Smallholder Crop Production. Rome: Food and Agriculture Organization. Available online at: https://www.fao.org/docrep/014/i2215e/i2215e.pdf (accessed June 13, 2023).
FAO (2014a). HLPE (High Level Panel of Experts on Food Security and Nutrition of the Committee on World Food Security of the FAO) -Food Losses and Waste in the Context of Sustainable Food systems. Rome: Food and Agriculture Organization. Available online at: https://edepot.wur.nl/309118 (accessed June 2, 2022).
FAO (2014b). What is Conservation Agriculture? FAO-CA. Available online at: http://www.fao.org/ag/ca/1a.html (accessed May 30, 2023).
FAO (2014c). CA Adoption Worldwide. FAO-CA. Available online at: http://www.fao.org/ag/ca/6c.html (accessed May 30, 2023).
FAO (2015). The Second Report on the State of the World's Plant Genetic Resources for Food and Agriculture. Rome: Food and Agriculture Organization. Available online at: https://www.fao.org/3/i3901e/i3901e.pdf (accessed June 15, 2022).
FAO (2016). Voluntary Guidelines for Mainstreaming Biodiversity into Policies, Programmes and National and Regional Plans of Action on Nutrition. Rome: Food and Agriculture Organization. Available online at: https://www.fao.org/3/i5248e/i5248e.pdf (accessed June 12, 2022).
FAO (2017). The State of Food Security and Nutrition in the World 2017. Building Resilience for Peace and Food Security. Rome: IFAD, FAO, UNICEF, WFP, and WHO. Available online at: https://www.fao.org/3/a-I7695e.pdf (accessed June 13, 2023).
FAO (2020a). World Food and Agriculture - Statistical Yearbook 2020. Rome: Food and Agriculture Organization.
FAO (2020b). FAO/TECA-Step by Step Conversion to Organic Agriculture. Available online at: https://teca.apps.fao.org/teca/en/technologies/8364 (accessed May 30, 2023).
FAO (2022). World Food and Agriculture – Statistical Yearbook 2022. Rome: Food and Agriculture Organization of the United Nations.
FAO Biodiversity International (2017). Guidelines on Assessing Biodiverse Foods in Dietary Intake Surveys. Rome: Food and Agriculture Organization. Available online at:https://www.fao.org/documents/card/fr/c/5d2034ff-a949-482a-801c-44b7b675f1dd/ (accessed May 18, 2022).
FAO IFAD, UNICEF, WFP, and WHO. (2020). The State of Food Security and Nutrition in the World 2020. Transforming Food Systems for Affordable Healthy Diets. Rome: Food andAgriculture Organization.
FAO IFAD, UNICEF, WFP, and WHO. (2021). The State of Food Security and Nutrition in theWorld 2021. Transforming Food Systems for Affordable Healthy Diets. Rome: FAO.
FAOSTAT (2009). FAO Statistical Database Domain on Fertilizers: ResourceSTAT-Fertilizers. Rome: Food and Agriculture Organisation of the United Nations (FAO).
Farooq, M., and Siddique, K. H. M., (eds.). (2014). Conservation Agriculture. Switzerland: Springer International. Available online at: https://link.springer.com/chapter/10.1007/978-3-319-11620-4_1 (accessed April 23, 2023).
Fassio, F., and Minotti, B. (2019). Circular economy for food policy: the case of the RePoPP project in the City of Turin (Italy). Sustainability. 11, 6078 doi: 10.3390/su11216078
Fischer, C. G., and Garnett, T. (2016). Plates, Pyramids, Planet: Developments in National Healthy and Sustainable Dietary Guidelines: A State of Play Assessment. Oxford: Food and Agriculture Organization of the United Nations, The Food Climate Research Network at the University of Oxford. Available online at: https://www.fao.org/documents/card/en/c/d8dfeaf1-f859-4191-954fe8e1388cd0b7/ (accessed April 6, 2022).
Fischer, T., Byerlee, D., and Edmeades, G. (2014). Crop Yields and Global Food Security: Will Yield Increase to Feed the World? 1st Edn. Canberra, ACT: Two foot Consulting Group, 462–467.
Fließbach, A., Oberholzer, H. R., Gunst, L., and Mader, P. (2007). Soil organic matter and biological soil quality indicators after 21 years of organic and conventional farming. Agric. Ecosyst. Environ.118, 273–284. doi: 10.1016/j.agee.2006.05.022
Fraser, E. D., Mabee, W., and Figge, F. (2005). A framework for assessing the vulnerability of food systems to future shocks. Futures 37, 465–479. doi: 10.1016/j.futures.2004.10.011
Friedrich, T., Derpsch, R., and Kassam, A. (2012). Overview of the global spread of agriculture. Field Act. Rep. 6, 1–7.
Futurism (2017). World Food Supplies Threatened by Sixth Mass Extinction. About 75 Percent of the World's Food Comes From 12 Crops and Five Animal Species — and That's a Problem'. Karla Lant. Available online at: https://futurism.com/world-food-supplies-threatened-by-sixth-mass-extinction (accessed October 21, 2022).
Garima, B. D. R., Thakur, C. L., Kaushal, R., Sharma, P., Kumar, D., and Kumari, Y. (2021). Bamboo-based agroforestry system effects on soil fertility: Ginger performance in the bamboo subcanopy in the Himalayas (India). Agron. J. 113, 2832–2845. doi: 10.1002/agj2.20684
Gaud, W. S. (1968). The Green Revolution: Accomplishments and Apprehensions. Available online at: https://archives.yale.edu/repositories/6/archival_objects/2948845 (accessed July 17, 2022).
Geels, F. W., and Schot, J. (2007). Typology of sociotechnical transition pathways. Res. Policy 36, 399–417. doi: 10.1016/j.respol.2007.01.003
Geiger, F., Bengtsson, J., Berendse, F., Weisser, W. W., Emmerson, M., Morales, M. B., et al. (2010). Persistent negative effects of pesticides on biodiversity and biological control potential on European farmland. Basic Appl. Ecol. 11, 97–105. doi: 10.1016/j.baae.2009.12.001
Geissdoerfer, M., Paulo, S., Nancy, M. P. B., and Hultink, E. J. (2017). The circular economy – a new sustainability paradigm? J. Clean. Prod. 143, 757–768. doi: 10.1016/j.jclepro.2016.12.048
Gibbs, H. K., Ruesch, A. S., Achard, F., Clayton, M. K., Holmgren, P., Ramankutty, N., et al. (2010). Tropical forests were the primary sources of new agricultural land in the 1980s and 1990s. Proc. Natl. Acad. Sci. U. S. A. 107, 16732–16737. doi: 10.1073/pnas.0910275107
Gliessman, S. R. (2015). Agroecology-The Ecology of Sustainable Food Systems, 3rd Edn. Boca Raton: CRC Press, Taylor & Francis Group. Available online at: https://www.taylorfrancis.com/books/mono/10.1201/b17881/agroecology-stephen-gliessman (accessed April 23, 2023).
Godfray, C., Beddington, J., Crute, I., Haddad, L., Lawrence, D., et al. (2010). Food Security: the challenge of feeding 9 billion people. Science 327, 812–818 doi: 10.1126/science.1185383
Gomiero, T., Pimentel, D., and Paoletti, M. G. (2011). Environmental impact of different agricultural management practices: conventional vs. organic agriculture. Crit. Rev. Plant Sci. 30, 95–124. doi: 10.1080/07352689.2011.554355
Govindan, K., and Hasanagic, M. A. (2018). systematic review on drivers, barriers, and practices towards circular economy: a supply chain perspective. Int. J. Prod. Res.56, 278–311. doi: 10.1080/00207543.2017.1402141
Groenfeldt, D. (2005). “Multi functionality of agricultural water: Looking beyond food production and ecosystem service,” in Paper Prepared for the FAO/Netherlands International Conference on Water for Food and Ecosystems (The Hague).
Hauggaard-Nielsen, H., Lund, S., Aare, A., Watson, C. A., Bedoussac, L., Aubertot, J.-N., et al. (2021). Translating the multifactor approach to research into practise using a workshop approach focusing on species mixtures. Front. Agr. Sci. Eng. 8, 460–473. doi: 10.15302/J-FASE-2021416
Holdren, J., and Ehrlich, P. (1974). Human population and the global environment. Am. Sci. 62, 282–292.
Hole, D. G., Perkins, A. J., Wilson, J. D., Alexander, I. H., Grice, P. V., and Evans, A. D. (2005). Does organic farming benefit biodiversity? Biol. Conserv. 122, 113–130. doi: 10.1016/j.biocon.2004.07.018
IFOAM (2002). Basic Standards for Organic Production and Process Approved by the IFOAM General Assembly. Bonn.
IFOAM (2009). The Full Diversity of Organic Agriculture: What we call Organic, Position Paper. Available online at: www.ifoam.bio/sites/default/files/position_full_diversity_of_oa.pdf (accessed April 23, 2023).
IFOAM (2010). Definition of Organic Agriculture. Available online at: http://www.ifoam.org/growingorganic/definitions/doa/index.html (accessed June 13, 2023).
IFOAM (2012). The IFOAM Norms for Organic Production and Processing. Available online at: http://www.ifoam.org (accessed June 13, 2023).
IFOAM (2020a). IFOAMPolicy brief on How Governments Can Recognize and Support Participatory Guarantee Systems (PGS) ifoam.bio Edition 2018. Available online at: https://www.ifoam.bio/sites/default/files/2020-03/policybrief_how_governments_can_support_pgs.pdf (accessed June 13, 2023).
IFOAM (2020b). Principles Of Organic Agriculture. Available online at: https://ifoam.bio/principles-organic-agriculture-brochure (accessed June 13, 2023).
IFOAM (2022). Declaration from the 50th Anniversary Conference of IFOAM- Organics International 1-3 October, 2022. Goesan. Available online at: https://www.ifoam.bio/declaration-50th-anniversary-conference-ifoam-organics (accessed June 13, 2023).
IIFSR-ICAR. (2014). All India Network Programme on Organic Farming. Available online at: https://iifsr.icar.gov.in/icar-iifsr/npof/ (accessed June 12, 2023).
IIFSR-ICAR. (2016). Scientific Package of Practices (PoPs) for Organic Production of Crops in Cropping Systems. p. 227.
ILO (2007). “Employment by sector,” in Key Indicators of the Labour Market (KILM), 5th Edn. Geneva: International Labour Organisation (ILO). Available online at: http://www.ilo.org/public/english/employments/strat/kil04.pdf (accessed June 10, 2023).
IPCC (2007). “Summary for policymakers,” in Climate Change 2007: Impacts, Adaptation and Vulnerability. Contribution of Working Group II to the Fourth Assessment Report of the Intergovernmental Panel on Climate Change, eds M. L. Parry, O. F. Canziani, J. P. Palutikof, P. J. van derLinden, and C. E. Hanson (Cambridge: Cambridge University Press), 7–22.
IPES-Food (2017). Unravelling the Food–Health Nexus: Addressing Practices, Political Economy, and Power Relations to Build Healthier Food Systems. The Global Alliance for the Future of Food and IPES-Food. Available online at: http://www.ipes-food.org/images/Reports/Health_FullReport.pdf (accessed June 10, 2023).
Irani, Z., and Sharif, A. M. (2018). Food security across the enterprise: a puzzle, problem or mess for a circular economy? J. Enterprise Inf. Manag. 33, 2–9. doi: 10.1108/JEIM-03-2017-0045
Jat, R. A., and Sahrawat, K. L. Kassam, A. H. (eds.),., (2014). Conservation Agriculture: Global Prospects and Challenges. Wallingford: CABI, 393 p. Available online at: https://agris.fao.org/agris-search/search.do?recordID=XF2015004173 (accessed May 30, 2023).
Jayanthi, C., Rangasamy, A., and Chinnusamy, C. (2000). Water budgeting for components in lowland integrated farming systems. Agric. J. 87, 411–414. doi: 10.29321/MAJ.10.A00486
Jennings, B. H. (1988). Foundations of International Agricultural Research: Science and Politics in Mexican Agriculture. Boulder: Westview Press.
Jordan, R., Müller, A., and Oudes, A. (2009). High Sequestration, Low Emission, Food Secure Farming. Organic Agriculture-a Guide to Climate Change & Food Security. Bonn: International Federation of Organic Agriculture Movements.
Joshi, D. R., Ghimire, R., Kharel, T., Mishra, U., and Clay, S. A. (2021). Conservation agriculture for food security and climate resilience in Nepal. Agron. J. 113, 4484–4493. doi: 10.1002/agj2.20830
Jurgilevich, A., Birge, T., Kentala-Lehtonen, J., Korhonen-Kurki, K., Pietikainen, J., Saikku, L., et al. (2016). Transition towards circular economy in the food system. Sustainability 8, 69. doi: 10.3390/su8010069
Kassam, A., Friedrich, T., Derpsch, R., and Kienzle, J. (2015). Overview of the worldwide spread of conservation agriculture. Field Act. Sci. Rep. 8, 1–11.
Kassam, A., Friedrich, T., Francis Shaxson, F., and Pretty, J. (2009). The spread of conservation agriculture: Justification, sustainability and uptake. Int. J. Agri. Sustain. 7, 292–320. doi: 10.3763/ijas.2009.0477
Kissinger, G., Herold, M., and De Sy, V. (2012). Drivers of Deforestation and Forest Degradation: A Synthesis Report for REDD+Policymakers. Vancouver: Lexeme Consulting.
Kumar, J., Thakur, C. L., Bhardwaj, D. R., Kumar, S., and Dutt, B. (2023). Effects of integrated nutrient management on performance of bhringraj (Eclipta prostrata L.) and soil fertility under the Grewia optiva Drummond. canopy in a mid-hill agro-ecosystem of north western Himalayas. Agrofor. Syst. 97, 711–726. doi: 10.1007/s10457-023-00822-6
Kumar, S., and Jain, D. K. (2005). Are linkages between crops and livestock important for the sustainability of the farming system? Asian Econ. Rev. 47, 90–101.
Lipper, L., Puri, J., Cavatassi, R., and McCarthy, N. (2022). How doesclimate change affect the evidence we need foragricultural development? Agric. Econ. 53, 911–923. doi: 10.1111/agec.12732
Lohith, R. V., Murthy, M., and Sanjay, M. T. (2021). Studies on methane and nitrous oxide emission from zero budget natural farming organic and conventional farming in direct seeded aerobic rice. Mysore J. Agric. Sci. 55, 230–236.
Lund, V. (2006). Natural living—a precondition for animal welfare in organic farming. Livest. Sci. 100, 71–83. doi: 10.1016/j.livprodsci.2005.08.005
Maeder, P., Fliessbach, A., Dubois, D., Gunst, L., Fried, P., and Niggli, U. (2002). Soil fertility and biodiversity in organic farming. Science. 96, 1694–1697. doi: 10.1126/science.1071148
Magrini, M. B., Anton, M., Chardigny, J. M., Duc, G., Duru, M., Jeuffroy, M. H., et al. (2018). Pulses for sustainability: breaking agriculture and food sectors out of lock-in. Front. Sustain. Food Syst. 2, 64. doi: 10.3389/fsufs.2018.00064
McAdam, J. H., Burgess, P. J., Graves, A. R., Rigueiro-Rodríguez, A., and Mosquera-Losada, M. R. (2009).“Classifications and functions of agroforestry systems in Europe,” in Agroforestry in Europe- Current Status and Future Prospects, eds A. Rigueiro-Rodríguez, J. McAdam, and M. R. Mosquera-Losada (Dordrecht: Springer Science + Business Media B.V), 21–41.
McCarthy, B., Kapetanaki, A. B., and Wang, P. (2019). Circular agri-food approaches: will consumers buy novel products made from vegetable waste? Rural Soc. 28, 91–107. doi: 10.1080/10371656.2019.1656394
McDonough, W., and Brungart, M. (2002). Design for the triple top line:new tools for sustainable commerce. Corp Environ. Strat. 9, 251–258. doi: 10.1016/S1066-7938(02)00069-6
Mimura, N., Nurse, L., McLean, R. F., Agard, J., Briguglio, L., Lefale, P., et al. (2007). “Small islands,” in Climate Change 2007: Impacts, Adaptation and Vulnerability. Contribution of Working Group II to the Fourth Assessment Report of the Intergovernmental Panel on Climate Change, eds M. L. Parry, O. F. Canziani, J. P. Palutikof, P. J. van der Linden, and C. E. Hanson (Cambridge: Cambridge University Press), 687–716.
Mishra, M. (2013). Role of eco-friendly agricultural practices in indian agriculture development. Int. J. Agric. Food Sci. Technol. 4, 25–29.
Muller, A., Olesen, J., Davis, J., Smith, L., Dytrtová, K, Gattinger, A., et al. (2012). Reducing Global Warming and Adapting to Climate Change: The Potential of Organic Agriculture. Working Paper, Draft Version. Suisse: FiBL Schweiz.
Muller, A., Schader, C., El-HageScialabba, N., Brüggemann, J., Isensee, A., Erb, K.-H., et al. (2017). Strategies for feeding the world more sustainably with organic agriculture. Nat. Commun. 8, 1–13. doi: 10.1038/s41467-017-01410-w
Murthy, I. K., Dutta, S., Varghese, V., Joshi, P. P., and Kumar, P. (2016). Impact of agroforestry systems on ecological and socio-economic systems: a review. Glob. J. Sci. Front. Res. 16, 14–28.
Nair, P. K. R. (2011). Agroforestry systems and environmental quality: introduction. J. Environ. Qual. 40, 784–790. doi: 10.2134/jeq2011.0076
Nattassha, R., Handayati, Y., Simatupang, T. M., and Siallagan, M. (2020). Understanding circular economy implementation in the agri-food supply chain: the case of an Indonesian organic fertiliser producer. Agri. Food Sec. 9, 1–16. doi: 10.1186/s40066-020-00264-8
New York Times (1985). Insect That Ruined Vineyards Reappears in California. KatherineBishop. Available online at: https://www.nytimes.com/1985/07/01/us/insect-that-ruinedvineyards-reappears-in-california.html (accessed November 19, 2022).
Niggli, U., Fließbach, A., Hepperly, P., and Scialabba, N. (2009). Low Greenhouse Gas Agriculture: Mitigation and Adaptation Potential of Sustainable Farming Systems. Rome: FAO. Available online at: ftp://ftp.fao.org/docrep/fao/010/ai781e/ai781e00.pdf (accessed May 30, 2023).
Nurhasan, M., Maulana, A. M., Ariesta, D. L., Usfar, A. A., Napitupulu, L., Rouw, A., et al. (2022). Toward a sustainable food system in west Papua, Indonesia: Exploring the links between dietary transition, food security, and forests. Front. Sustain. Food Syst. 5, 789186. doi: 10.3389/fsufs.2021.789186
Palekar, S. (2005). The Philosophy of Spiritual Farming, 2nd Edn. Amravati: Zero Budget Natural Farming Research, Development & Extension Movement.
Panke, S. K., Kadam, R. P., and Nakhate, C. S. (2010). “Integrated farming system for sustainable rural livelihood security,” in 22nd National Seminar on “Role of Extension in Integrated Farming Systems for Sustainable Rural Livelihood” (Maharashtra), 33–35.
Paramesh, V., Ravisankar, N., Behera, U., Arunachalam, V., Kumar, P., Solomon Rajkumar, R., et al. (2022). Integrated farming system approaches to achieve food and nutritional security for enhancing profitability, employment, and climate resilience in India. Food Energy Sec. 11, e321. doi: 10.1002/fes3.321
Parrot, N., Olesen, J. E., and Hogh-Jensen, H. (2006). “Certified and non-certified organic farming in the developing world” in Global Development of Organic Agriculture: Challenges and Prospects, eds N. Halberg, H. F. Alroe, M. T. Knudsen, and E. S. Kristensenet (Cambridge: CABI), 153–179.
Patiram, A. R. K., and Bhadauria, S. B. S. (2003). Sustainable land use planning for the Sikkim Himalayas-perspectives and options. ENVIS Bull. Himalayan Ecol. 11, 37–49.
Pearce, D. W., and Turner, R. K. (1989). Economics of Natural Resources and the Environment. Baltimore, MD: JHU Press.
Pelletier, N., Arsenault, N., and Tyedmers, P. (2008). Scenario-modeling potential eco-efficiency gains from a transition to organic agriculture: life cycle perspectives on Canadian canola, corn, soy and wheat production. Environ. Manag. 42, 989–1001 doi: 10.1007/s00267-008-9155-x
Pelletier, N., Audsley, E., Garnett, T., Henriksson, P., Kendall, A., Kramer, K. J., et al. (2011). Energy intensity of agriculture and food systems. Annu. Rev. Environ. Resourc. 36, 223–246 doi: 10.1146/annurev-environ-081710-161014
Petrosillo, I., Valente, D., and Scavuzzo, C. M. Selvan, T. (2023). Editorial: land degradation pattern and ecosystem services. Front. Environ. Sci. 11, 1137768. doi: 10.3389/fenvs.2023.1137768
Pimentel, D. (1996). Green revolution agriculture and chemical hazards. Sci. Total Environ. 188, S86–S98. doi: 10.1016/0048-9697(96)05280-1
Pimentel, D., and Burgess, M. (2014). An environmental, energetic and economic comparison of organic and conventional farming systems. Integr. Pest Manag. Pestic. Prob. 3, 141–166. doi: 10.1007/978-94-007-7796-5_6
Pimentel, D., Harvey, C., Resosudarmo, P., Sinclair, K., Kurz, D., McNair, M., et al. (1995). Environmental and economic costs of soil erosion and conservation benefits. Science 267, 1117–1123 doi: 10.1126/science.267.5201.1117
Popkova, E. G., and Shi, X. (2022). Economics of climate change: global trends, country specifics and digital perspectives of climate action. Front. Environ. Econ. 1. 935368. doi: 10.3389/frevc.2022.935368
Porter, J. R., Xie, L., Challinor, A. J., Cochrane, K., Howden, S. M., Iqbal, M. M., et al. (2014). “Food security and food production systems,” in Climate Change 2014: Impacts, Adaptation, and Vulnerability. Part A: Global and Sectoral Aspects. Contribution of Working Group II to the Fifth Assessment Report of the Intergovernmental Panel on Climate Change, eds C. B. Field, V. R. Barros, D. J. Dokken, K. J. Mach, M. D. Mastrandrea, T. E. Bilir, et al. (Cambridge; New York, NY: Cambridge University Press).
Pretty, J., and Ball, A. (2001). Agricultural Influences on Carbon Emissions and Sequestration: A Review of Evidence and the Emerging Trading Options. Centre for Environment and Society Occasional Paper 2001-03, 3 Centre for Environment and Society and Department of Biological Sciences, University of Essex, Colchester, United Kingdom. p. 31.
Pretty, J., and Bharucha, Z. P. (2014). Sustainable intensification in agricultural systems. Ann. Bot. 114, 1571–1596. doi: 10.1093/aob/mcu205
Pretty, J. N., Ball, A. S., Lang, T., and Morison, J. I. L. (2005). Farm costs and food miles: an assessment of the full cost of the UK weekly food basket. Food Policy 30, 1–19. doi: 10.1016/j.foodpol.2005.02.001
Price, T. (2000). Cultivation our futures. Final Paper. OECD Publications No. 2. Organization for Economic Co-operation and Development.
Radhamani, S., Balasubramanian, A., Ramamoorthy, K., and Geethalakshmi, V. (2003). Sustainable integrated farming systems for drylands: a review. Agric. Rev. 24, 204–210.
Ramesh, K. R., Devanand, P. S., Senthil, K., Balasubramanian, P., Thiyagarajan, G., Selvan, T., et al. (2022). Growth and yield performance of fodder trees and grasses under silvipastoral systems in dryland farming. Agri. Mech. Asia Africa Latin America. 53, 11231–11238.
Ripple, W. J., Smith, P., Haberl, H., Montzka, S. A., Mcalpine, C., and Boucher, D. H. (2014). Ruminants, climate change and climate policy. Nat. Clim. Change 4, 2–5. doi: 10.1038/nclimate2081
Rosati, A., Borek, R., and Canali, S. (2021). Agroforestry and organic agriculture. Agrofor. Syst. 95, 805–821. doi: 10.1007/s10457-020-00559-6
Rosenstock, T. S., Mayzelle, M., Nambi, N., and Fantke, P. (2020). Climate impacts of natural farming: A cradle to gate comparison between conventional practice and Andhra Pradesh Community Natural Farming. J. Agri. Allied. Sci. 2020, 1–50. doi: 10.31220/agriRxiv.2020.00013
Rosset, P. M., and Martínez-Torres, M. E. (2012). Rural social movements and agroecology: context, theory, and process. Ecol. Soc. 17, 1–12. doi: 10.5751/ES-05000-170317
Sahota, A. (2021). “The global market for organic food and drink,” in The World of Organic Agriculture. Statistics and Emerging Trends 2021, eds H. Willer, J. Trávníček, C. Meier, and B. Schlatter (Bonn: Research Institute of Organic Agriculture FiBL, Frick, and IFOAM - Organics International), 136–139.
Sands, D. C., Morris, C. E., Dratz, E. A., and Pilgeram, A. (2009). Elevating optimal human nutrition to a central goal of plant breeding and production of plant-based foods. Plant Sci. 177, 377–389. doi: 10.1016/j.plantsci.2009.07.011
Sasikala, V., Tiwari, R., and Saravan, M. (2015). A review of integrated farming systems. J. Int. Acad. Res. Multidiscip. 3, 319–328.
Schippmann, U., Leaman, D. J., and Cunningham, A. B. (2002). Impact of Cultivation and Gathering of Medicinal Plants on Biodiversity: Global Trends and Issues. Rome: Food and Agriculture Organization. Available online at: https://www.fao.org/3/aa010e/AA010e00.htm (accessed August 13, 2022).
Schippmann, U., Leaman, D. J., and Cunningham, A. B. (2006). “A comparison of cultivation and wild collection of medicinal and aromatic plants under sustainability aspects” in Medicinal andAromatic Plants, eds. R. Bogers, L. Craker, and D. Lange (Dordrecht: Springer Netherlands), 75–95.
Schlatter, B., Travnicek, J., Meier, C., Keller, O., and Willer, H. (2021). “Current statistics on organic agriculture worldwie: Area, operators and market,” in The World of Organic Agriculture. Statistics and Emerging Trends 2021, eds H. Willer, J. Trávníček, C. Meier, and B. Schlatter (Bonn: Research Institute of Organic Agriculture FiBL, Frick, and IFOAM - Organics International), 31–69.
Scialabba, N., and Muller-Lindenlauf, M. (2010). Organic agriculture and climate change. Renew. Agric. Food Syst. 25, 158–169. doi: 10.1017/S1742170510000116
Scoones, I., Melnyk, M., and Pretty, J. (1992). The Hidden Harvest: Wild Foods and Agricultural Systems. A Literature Review and Annotated Bibliography. London: International Institute for Environment and Development.
Secretariat of the Pacific Community. (2008). Annual Report -2008. Secretariat of the Pacific Community. Land Resources Division. SPC Headquarters, New Caledonia. p. 44.
Selvan, T. (2022). NMHS-Final Technical Report (FTR)- Detailed Report on Conservation of Biodiversity and Livelihood Enhancement through Community-Based Forest Management and Ecotourism in and around Submergence of Small Hydropower Project. New Delhi: NMHS-PMU National Mission on Himalayan Studies, GBP NIHE HQs Ministry of Environment, Forest & Climate Change (MoEF&CC), 63.
Selvan, T., and Kumar, S. (2017). “Agroforestry in the North-Eastern Himalayas” in Agroforestry for Increased Production and Livelihood Security, eds S. K. Gupta, P. Panwar, and R. Kaushal (New Delhi: New India Publishing Agency), 23–40.
Selvan, T., and Tripathi, K. M. (2017). Economic potential of bamboo for rural livelihood. Agrobios. News Letter 15, 42–44.
Seufert, V., Ramankutty, N., and Foley, J. A. (2012). Comparing the yields of organic and conventional agriculture. Nature 485, 229–232. doi: 10.1038/nature11069
Sharma, P., Bhardwaj, D. R., Singh, M. K., Nigam, R., Pala, N. A., Kumar, A., et al. (2022). Geospatial technology in agroforestry: Status, prospects, and constraints. Environ. Sci. Pollut. Res. doi: 10.1007/s11356-022-20305-y
Sharma, P., Singh, M. K., Verma, K., and Prasad, S. K. (2020). Changes in the weed seed bank in long-term establishment methods trials under rice-wheat cropping system. Agronomy 10, 292. doi: 10.3390/agronomy10020292
Sharma, U., Bhardwaj, D. R., Sharma, S., Sankhyan, N., Thakur, C. L., Rana, N., et al. (2022). Assessment of the efficacy of various mulch materials on improving the growth and yield of ginger (Zingiber officinale) under bamboo-based agroforestry system in NW-Himalaya. Agrofor. Syst. 96, 925–940. doi: 10.1007/s10457-022-00753-8
Sherpa, T. O. (2023). Indigenous people's perception of indigenous agricultural knowledge for climate change adaptation in Khunbu, Nepal. Front. Clim. 4, 1067630. doi: 10.3389/fclim.2022.1067630
Singh, R. P., and Ratan, P. (2009). “Farming system approach for growth in Indian Agriculture,” in National Seminar on Enhancing Efficiency of Extension for Sustainable Agriculture and Livestock Production (Izatnagar: Indian Veterinary Research Institute).
Smith, P., Clark, H., Dong, H., Elsiddig, E., Haberl, H., Harper, R., et al. (2014). “Agriculture, forestry and other land use (AFOLU),” in Climate Change 2014: Mitigation of Climate Change. Contribution of Working Group III to the Fifth Assessment Report of the Intergovernmental Panel on Climate Change, eds O. Edenhofer, R. Pichs-Madruga, Y. Sokona, E. Farahani, S. Kadner, K. Seyboth, et al. (Cambridge: Cambridge University Press), 811–922.
Smith, P., Martino, D., Cai, Z., Gwary, D., Janzen, H., Kumar, P., et al. (2007). “Agriculture,” in Climate Change 2007: Mitigation. Contribution of Working Group III to the Fourth Assessment Report of the Inter-governmental Panel on Climate Change, eds B. Metz, O. R. Davidson, P. R. Bosch, R. Dave, and L. A. Meyer (Cambridge: Cambridge University Press).
Smolik, J. D., Dobbs, T. L., and Rickerl, D. H. (1995). The relative sustainability of alternative, conventional and reduced-till farming system. Am. J. Altern. Agric. 10, 25–35. doi: 10.1017/S0889189300006081
Sørensen, C., Jensen, L., Sommer, S. G., Bochtis, D., and Rotz, C. A. (2013). “Technologies and logistics for handling, transport and distribution of animal manure,” in Animal Manure Recycling: Treatment and Management, 1st Edn, eds S. G. Sommer, M. L. Christensen, T. Schmidt, and L. S. Jensen (Chichester: John Wiley & Sons, Ltd).
Tilman, D., and Clark, M. (2014). Global diets link environmental sustainability and human health. Nature 515, 518–522. doi: 10.1038/nature13959
Tilman, D., and Clark, M. (2015). Food, agriculture and the environment: can we feed the world and save the Earth? Daedalus 144, 8–23. doi: 10.1162/DAED_a_00350
Tipraqsa, P. (2006). “Opportunities and constraints of integrated farming system in Northeast Thailand. A case study of the Huai Nong Ian catchment, Khon Kaen Province,” in Ecology Development Series No. 35 (Cuvillier Verlag, Göttingen: University of Bonn).
Tipraqsa, P., Craswell, E. T, Noble, A. D., and Schmidt, V. D. (2007). Resource integration for multiple benefits: multifunctionality of integrated farming systems in Northeast Thailand. Agric. Syst. 94, 694–703 doi: 10.1016/j.agsy.2007.02.009
UN (1975). Report of the World Food Conference Rome, 5-16 November 1974. New York, NY: United Nations Publication.
UNDESA (2021). UN/DESA Policy Brief #105: Circular Agriculture for Sustainable Rural Development. Available online at: http://www.un.org/development/desa/dpad (accessed April 08, 2022).
UNESCO. (2020). United Nations World Water Development Report 2020: Water and Climate Change. Paris: UNESCO.p. 219.
UNFCCC (2007). Climate Change: Impacts, Vulnerabilties and Adaptation in Developing Countries. Bonn: Climate Change Secretariat (UNFCCC) Martin-Luther-King-Strasse. Available online at: www.unfccc.int (accessed June 19, 2023).
Verma, K., Prasad, S. K., Singh, M. K., and Sharma, P. (2023a). Assessment of agronomic zinc biofortification of alley cropped pearl millet. Bang. J. Bot. 52, 203–209. doi: 10.3329/bjb.v52i1.65252
Verma, K., Prasad, S. K., Singh, M. K., and Sharma, P. (2023b). Response of alley-cropped pearl millet (Pennisetum glaucum) to nitrogen and zinc schedules under semi-arid regions. Indian J. Agron. 68, 105–109. doi: 10.59797/ija.v68i1.213
Vermeulen, S. J., Dinesh, D., Howden, S. M., Cramer, L., and Thornton, P. K. (2018). Transformation in practice: a review of empirical cases of transformational adaptation in agriculture under climate change. Front. Sustain. Food Syst. 2, 65. doi: 10.3389/fsufs.2018.00065
Von Carlowitz, H. C. (1713). Sylvicultura Oeconomica. Leipzig: Hausswirthliche Nachricht und Naturmäßige Anweisungzur Wilden Baum-Zucht Faksimile der Erstauflage.
Watson, C. A., Reckling, M., Preissel, S., Bachinger, J., Bergkvist, G., Kuhlman, T., et al. (2017). Grain legume production and use in European agricultural systems. Adv. Agron. 144, 235–303 doi: 10.1016/bs.agron.2017.03.003
Wezel, A., Casagrande, M., Celette, F., Vian, J. F., Ferrer, A., and Peigné, J. (2014). Agroecological practices for sustainable agriculture. A review. Agron. Sustain. Dev. 34, 1–20. doi: 10.1007/s13593-013-0180-7
Whitfield, S., Challinor, A. J., and Rees, R. M. (2018). Frontiers in climate smart food systems: outlining the research space. Front. Sustain. Food Syst. 2, 2. doi: 10.3389/fsufs.2018.00002
WHO (2009). Global Health Risks: Mortality and Burden of Disease Attributable to Selected Major Risks. Geneva: World Health Organization.
WHO (2020). As More Go Hungry and Malnutrition Persists, Achieving Zero Hunger by 2030 in Doubt, UN Report Warns. Available online at: https://www.who.int/news/item/13-07-2020 (accessed December 02, 2022).
WHO/FAO (2003). Joint WHO/FAO Expert Consultation. WHO Technical Report 916: Diet, Nutrition and the Prevention of Chronic Diseases. World Health Organization Technical Report Series. Available online at: https://www.ncbi.nlm.nih.gov/pubmed/12768890 (accessed May 28, 2022).
Willer, H., Travnicek, J., Meier, C., and Schlatter, B. (2021). The World of Organic Agriculture. Statistics and Emerging Trends 2021. Research Institute of Organic Agriculture FiBL, Fricks and IFOAM- Organics International, Rheinbreitbach, Germany. p. 340.
Wilson, E. O. (1992). The Diversity of Life. Cambridge, MA: Harvard University Press; Belknap Press. p.424.
Winqvist, C., Ahnstrom, J., and Bengtsson, J. (2012). Effects of organic farming on biodiversity and ecosystem services: taking landscape complexity into account. Ann NY Acad Sci. 1249, 191–203. doi: 10.1111/j.1749-6632.2011.06413.x
Young, A. (2002). Agroforestry for Soil Management. Wallingford: Centre for Agriculture and Bioscience International.
Zhang, F., and Li, L. (2003). Using competitive and facilitative interactions in intercropping systems enhance crop productivity and nutrient use efficiency. Plant Soil. 248, 305–312 doi: 10.1023/A:1022352229863
Zhou, J., Li, B., Xia, L., Fan, C., and Xiong, Z. (2019). Organic-substitute strategies reduced carbon and reactive nitrogen footprints and gained net ecosystem economic benefit for intensive vegetable production. J. Clean. Prod. 225, 984–994. doi: 10.1016/j.jclepro.2019.03.191
Zhu, Y., Chen, H., Fan, J., Wang, Y., Li, Y., Chen, J., et al. (2000). Genetic diversity and disease control in rice. Nature 406, 718–722 Available online at: https://www.nature.com/articles/35021046 (accessed May 5, 2023).
Zikeli, S., Rembiałkowska, E., Załecka, A., and Badowski, M. (2014). “Organic farming and organic food quality: prospects and limitations,” in Sustainable Food Production Includes Human and Environmental Health, Issues in Agroecology-Present Status and Future Prospects, eds W. B. Campbell and S. Lopez-Ortiz (Dordrecht: Springer Science + Business Media), 85–164. doi: 10.1007/978-94-007-7454-4_3
Keywords: crop biodiversification, lesser-known crops, sustainability, 5R-concept, Circular-Organo-Agroforestry, organo-agroecosystem, agroforestry
Citation: Selvan T, Panmei L, Murasing KK, Guleria V, Ramesh KR, Bhardwaj DR, Thakur CL, Kumar D, Sharma P, Digvijaysinh Umedsinh R, Kayalvizhi D and Deshmukh HK (2023) Circular economy in agriculture: unleashing the potential of integrated organic farming for food security and sustainable development. Front. Sustain. Food Syst. 7:1170380. doi: 10.3389/fsufs.2023.1170380
Received: 20 February 2023; Accepted: 27 June 2023;
Published: 24 July 2023.
Edited by:
Subhash Babu, Indian Agricultural Research Institute (ICAR), IndiaReviewed by:
Lalit Mohan Gupta, Sher-e-Kashmir University of Agricultural Sciences and Technology, IndiaAmol Vasishth, Uttarakhand University of Horticulture and Forestry, India
Jayanta Kumar Patra, Dongguk University Seoul, Republic of Korea
Copyright © 2023 Selvan, Panmei, Murasing, Guleria, Ramesh, Bhardwaj, Thakur, Kumar, Sharma, Digvijaysinh Umedsinh, Kayalvizhi and Deshmukh. This is an open-access article distributed under the terms of the Creative Commons Attribution License (CC BY). The use, distribution or reproduction in other forums is permitted, provided the original author(s) and the copyright owner(s) are credited and that the original publication in this journal is cited, in accordance with accepted academic practice. No use, distribution or reproduction is permitted which does not comply with these terms.
*Correspondence: Thiru Selvan, dHNlbHZhbiYjeDAwMDQwO3RyaXB1cmF1bml2LmFjLmlu