- 1Department of Geography and Resource Development (DGRD), University of Ghana, Accra, Ghana
- 2Soil Science Division, Cocoa Research Institute of Ghana (CRIG), New Tafo Akim, Ghana
- 3Institute for Environment and Sanitation Studies (IESS), University of Ghana, Accra, Ghana
Introduction: Soil health is critical for the efficient management of soil fertility and crop yield in “green” cocoa (GC) (Theobroma cacao L.) agroforestry systems. However, knowledge about agroecosystem factors that affect healthy soil productivity in “green” cocoa agroforestry systems is patchy in West Africa. Based on organic cocoa (OC) and conventional cocoa (CC) agroforestry systems in Ghana, this study examined the soil health and synergy of ecological factors that determine the yield of GC.
Methods: Using multi-stage random sampling, 11 CC and 11 OC farms were sampled from three soil types (ferralsols, lixisols, and leptosols) within selected agroecological zones. Socioeconomic and farm data, including bulked soil samples, were collected at 0–30 cm depth for analysis of soil chemical and physical properties.
Results: The results showed intricate relationships between the ecological factors and the yield of GC (1.07 t ha−1), which comprised dry beans of OC (1.24 t ha−1) and CC (0.89 t ha−1). The green cocoa yield increased for fields owned by female farmers and for native farmers who inherited or outrightly owned farmlands. The cocoa yield was also positively related to physicochemical factors such as soil organic carbon (0.21%), pH (5.8), and carbon–nitrogen ratio (40.8%). The carbon–nitrogen ratio and pH together exerted the highest positive influence (0.62%) on the yield. Biological factors such as plant density (>7 cocoa trees per 23.4 m2) and black pod rots reduced the cocoa yield.
Discussion: This study provides comprehensive empirical determinants of green cocoa productivity and offers a more reliable estimate of cocoa plant density. The findings suggest that Ghana's cocoa can be much greener if stakeholders promote healthy farm soil productivity and empower women who engage in soil organic carbon-conserving agroforestry.
1. Introduction
Cocoa (Theobroma cacao L.) produced on healthy farm soil supported by an agroforestry system is considered a “green” product (Schroth et al., 2016; Sdrolia and Zarotiadis, 2019). This is because it is believed that the process of production and the product are ecologically friendly, carbon-neutral, or climate-smart. The green products of cocoa agroforestry systems (CAS), such as cocoa yield, are produced in a way to prevent, reduce, and correct harmful environmental impacts of production systems (Rajab et al., 2016; Schroth et al., 2016). This involves the cultivation of cocoa with non-cocoa tree species of different canopy levels (multi-strata woody vegetation), with or without livestock simultaneously on the same unit of land (Tscharntke et al., 2011; Blaser et al., 2018; Castle et al., 2021, 2022). Evidence suggests that farm soils in CAS are healthier than those in cocoa monocropping systems (Rajab et al., 2016; Asare et al., 2019). A healthy cocoa farm soil can “sustain productivity, diversity, and environmental services of terrestrial ecosystems” (FAO ITPS, 2020). Hence, cocoa stakeholders are increasingly sourcing beans from “green” cocoa agroforestry systems that have healthy farm soils (Tscharntke et al., 2011; Fountain and Huetz-Adams, 2020). Nonetheless, previous studies show a paucity of empirical information on “green” cocoa farm soil productivity enhancement strategies (Hartemink, 2005; Fountain and Huetz-Adams, 2020; Amponsah-Doku et al., 2021). Therefore, it is important to examine the soil health and synergy of ecological factors influencing “green” cocoa farm soil productivity in agroforestry systems.
Healthy farm soils and trees of cocoa agroforestry systems can sequester 7 and 50 metric tons (t) of carbon (C) per hectare (ha), respectively, which provide ecosystem “green” benefits (Ofori-Frimpong et al., 2010; Lal, 2016; Quarles, 2018). Through photosynthesis, the trees absorb atmospheric carbon and store half of the carbon as soil organic carbon (Ofori-Frimpong et al., 2010; Rajab et al., 2016; Ingham, 2019). The soil organic carbon (SOC) constitutes a major component of cocoa farm soil health indicators (Amponsah-Doku et al., 2021; Arthur et al., 2022; Doe et al., 2022). The SOC serves as food for microbial organisms such as fungi and bacteria that play a crucial role in the mineralization of soil nutrients such as nitrogen (N), phosphorous (P), and potassium (K) for plant uptake (Ingham, 2019; Esmaeilzadeh-Salestani et al., 2021). The SOC also facilitates the release of soil nutrients through microbial actions, which improve soil structure and water and nutrient retention capacities (Rousk et al., 2010; Asigbaase et al., 2020, 2021). Other “green” benefits of CAS are temperature regulation, biodiversity, water cycling, and soil nutrient cycling (Asase and Tetteh, 2016; Rajab et al., 2016; Asigbaase et al., 2021).
A cocoa cropping system that makes efficient use of resources for optimum yield while maintaining soil health is also ecologically sustainable and may be described as green (Ofori-Frimpong et al., 2010; Dobermann et al., 2013; Sumberg and Giller, 2022). The same applies to a sustainable conventional cocoa (CC) cropping system, which aims at optimizing “green” cocoa yield by minimizing environmental liabilities through the rational use of timber species, food intercrops, synthetic pesticides, and mineral fertilizers. Similarly, a sustainable organic cocoa (OC) system uses non-synthetic inputs such as bioinsecticides, manure, or organic fertilizers with timber species (shade trees) and food intercrops to achieve an optimum “green” yield and soil health. Essentially, both cropping systems aim at optimizing the “green” benefits of cocoa agroforestry ecosystems (Sumberg and Giller, 2022). Hence, the composite of sustainable CC and OC is termed green cocoa (GC) in this study. The GC is akin to “mass balance,” where certified and non-certified sustainable cocoa beans are aggregated after harvest in Ghana and elsewhere (Mol and Oosterveer, 2015).
Ghana is the second-largest producer of the world's cocoa beans. In the year 2020, the country produced ~870,000 t of cocoa beans (FAOSTAT, 2021). In the same year, the cocoa sector employed 80,000 smallholder farmers and accounted for 19% (US$ 2.3 billion) of the country's export earnings (Fountain and Huetz-Adams, 2020; Amponsah-Doku et al., 2021). However, the suitability of climate and soil conditions for growing the crop is declining in the cocoa agroecological zones of the country (Läderach et al., 2013; Dossa et al., 2018a,b; Doe et al., 2022). As a result, despite improved innovations, resource inputs and several interventions in the cocoa sector (Fountain and Huetz-Adams, 2020), cocoa yields are reported to be declining with each harvest in farmers' fields (Appiah et al., 1997; Hartemink, 2005). At the national level, the cocoa yield barely increased (0.03 t ha−1) from 0.52 t ha−1 in 2017 to 0.55 t ha−1 in 2020, against a potential yield of 3.5 t ha−1 (FAOSTAT, 2021; Asante et al., 2022). The rigidity of national-level yield to rise and the declining yield at farmers' fields are inherently linked to ecological factors. Among the potential ecological factors are climate change and variability, soil type, plant age and density, pests and diseases, socioeconomic factors, and unsustainable management practices (Abdulai et al., 2020; Amponsah-Doku et al., 2021; Asitoakor et al., 2022).
However, studies on the determinants of cocoa yield have focused on single factors and soil fertility. For example, Aneani and Ofori-Frimpong (2013) adopted a socioeconomic approach to examine cocoa productivity and did not consider soil parameters. Those studies that examined soil fertility (Ofori-Frimpong et al., 2010; Ahenkorah, 2016; Asare et al., 2017; Kongor et al., 2019) did not consider soil health, social factors, and the magnitude of factor effects. The combined effects of the factors may be synergistic, which requires a more holistic study. In addition, the ecological concept of farm soil productivity suggests manipulations of social, biological, chemical, and physical ecological factors jointly impact crop yield and soil health. The complex interactions of these ecological factors generate stimuli at certain thresholds, below or beyond which desirable or undesirable feedback impacts the yield and soil health (van Ittersum and Rabbinge, 1997; Folke et al., 2010). For soil chemicals, the thresholds may be ideal or toxic to farm soil health (Tscharntke et al., 2012; Asare et al., 2017; Doe et al., 2022). Hence, limited predictive knowledge about the thresholds of these ecological factors hinders healthy cocoa farm soil productivity management.
The paucity of predictive knowledge of green cocoa farm soil productivity undermines local and international stakeholders in decision-making, aimed at enhancing the green benefits of cocoa agroforestry systems (van Vliet and Maja Slingerland, 2015; Dossa et al., 2018a). Particularly, it limits the work of smallholder cocoa farmers, buyers, extension officers, policymakers, and environmentalists, operating within different “soil ecotypes.” In this study, “soil ecotype” refers to a landscape characterized by a specific soil type in a specific agroecological zone or ecosystem. The present study, therefore, examines the soil health status of CC, OC, and GC systems, and the synergies and magnitudes of the ecological factors determining (±) green cocoa yield in Ghana.
2. Materials and methods
2.1. Study area
The study was conducted on smallholder cocoa farms in four communities, spread across three soil ecotypes in Ghana (Figure 1). The soil ecotypes were (i) Ferralsols (FR or Oxisols) of Wet Evergreen (WE) soil ecotype (FR.WE) in Elubo and Boinso cocoa districts of Western Region, (ii) Lixisols (LX or Alfisols) of dry semi-deciduous inner zone (DSIZ) soil ecotype (LX.DSIZ) in Suhum cocoa district in Eastern Region, and (iii) Leptosols (LE or Entisols) of moist semi-deciduous south-east (MSSE) soil ecotype (LE.MSSE) in Papase cocoa district of Oti Region. Average annual rainfall and cocoa production vary in the sequence of FR.WE > LE.MSSE > LX.DSIZ. Mean daily temperature (25°C) and annual rainfall (1,270–1,651 mm) of the LX.DSIZ are lower than those of the FR.WE (26°C and 1,732 mm). The mean daily temperature and annual rainfall are approximately 25°C and 1,400–1,800 mm, respectively, in the LE.MSSE. Soil quality varies in the different soil ecotypes, generally declining in the order as follows: LX.DSIZ > FR.WE > LE.MSSE (FAO and ITPS, 2015).
2.2. Study design, small size, sampling, and management practices
The study was designed as a quantitative cross-sectional agroecosystem analysis (Conway, 1987), which combined smallholder cocoa farmer socioeconomic and farm habitat survey data. Cocoa farmers and their respective cocoa farms were selected using a multi-stage stratified random sampling. In the first stage, three soil ecotypes were purposively selected out of six cocoa agroecological zones and seven soil types because they had both organic and conventional CAS. For each soil ecotype, 11 OC and 11 CC agroforests were randomly selected, making 33 CC and 33 OC systems, when combined 66 = GC farms (Figure 2). The OC system was managed by certified organic smallholder farmers of Yayra Glover Limited (YGL), while the CC system was managed by non-certified smallholder farmers in the same community.
The treatments were actual farmer practices in organic (OC) and conventional (CC) cocoa cropping systems in Ghana. Many previous studies such as Arthur et al. (2017) and Asigbaase et al. (2020, 2021) have examined differences in the effects of agronomic practices by OC and CC farmers on their soil physiochemical quality and yield. Generally, the OC farmers use non-synthetic (organic) agrochemicals while the CC farmers use synthetic (inorganic) agrochemicals, which is the pivotal difference between organic and conventional agriculture. However, using copper-based fungicides (CBF) to control the fungal (Phytophthora spp.) disease of cocoa black pod rot is common in both OC and CC systems. The use of CBF in conventional and organic farming is common in many European countries (European Commission, 2021) and well accepted under the Japanese Agriculture Standard (JAS) for organic plants. The same applies to the United States Department of Agriculture (USDA) organic standard and the Rainforest Alliance Sustainable Agriculture standard.
In terms of actual management practices, the OC farmers used organic fertilizers such as Elite granular organic (NPK3:4:4 + 9Ca + 1 Mg + 0.04B + 0.08Zn + 11 Organic matter) and PhytoGreen liquid organic fertilizers. In addition, they use biosolids, organic manures, and compost. Copper-based fungicides such as Champion, Nordox, and Kocide were used to manage cocoa black pod diseases. Insect pests such as capsids (mirids) and aphids were managed using organic bioinsecticides such as pyrethrum, extracts from the seeds and leaves of a neem tree (Azadirachta indica A. Juss.), and AgroPy 5EW supplied by YGL through the Ghana COCOBOD. The CC farmers used mineral fertilizers such as Assase Wura (NKP0:22:18 + 9CaO + 7S + 6MgO), Sidalco (NPK10:10:10), and Cocofeed (NPK0:30:20). Copper-based fungicides such as Ridomil Gold Plus (metalaxyl cuprous oxide) and Fungikill as well as pesticides such as Confidor (imidacloprid) and Akatemaster (bifenthrin) supplied by the Ghana COCOBOD. The treatment given to the GC by combining the CC and OC yields is akin to the practice of mass balance in sustainable cocoa trading (Mol and Oosterveer, 2015), integrated soil fertility management (Quaye et al., 2021), and integrated organic farming (Layek et al., 2023). All the cropping systems were rainfed, zero-tillage with at least 18 timber species ha−1 (CHED/WCF, 2016).
2.3. Data collection, soil sampling, and measurement of variables
The socioeconomic data of the farmers were solicited using a semi-structured questionnaire, while the biological, chemical, and physical characteristics of their cocoa farms were collected using a checklist (see online Supplementary material, subsection 1).
2.4. Measurement of cocoa biological variables and yield
The farm biological data covered cocoa tree age (CAge), cocoa variety (cultivar), number of black pod rot diseases per tree (Bpod), planting density (Pltdn), and cocoa yield. The cocoa fields studied had irregular spacing of cocoa trees as pertains to most smallholder cocoa fields in Ghana. Therefore, to determine the cocoa plant density, which is representative of the entire farm, a 24 m transect line was laid within each farm, with three sets of regular hexagons (Figure 3), and the hexagons were 3 m apart along the transect line. Each hexagon was made up of three congruent rhombi (Figure 3A) or six equilateral triangles (Figure 3B) of 3 m sides (23.4 m2). Based on the recommended 3 m apart planting distance (3 m × 3 m) for cocoa (CHED/WCF, 2016), each hexagon, rhombus, and triangle is supposed to have seven, four, and three cocoa trees, respectively. The average cocoa plant density (Pltdn) per hexagon was computed by dividing the sum of cocoa trees within the delineated hexagons by the number of hexagons (Figure 3C).
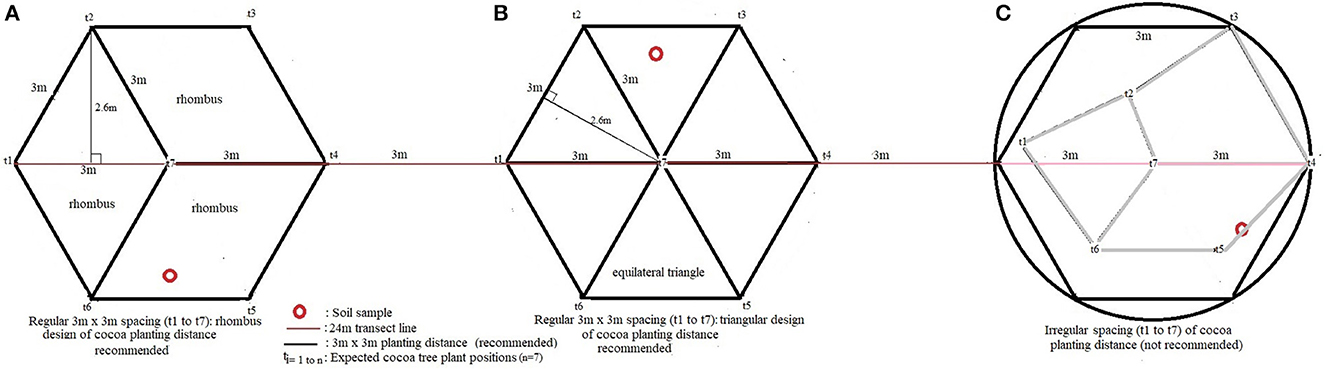
Figure 3. Schematic representation of expected cocoa plant position, plant density and soil sampling points for fields with (A) regular rhombus spacing, (B) regular triangle spacing, and (C) irregular spacing of cocoa planting distance.
The total count of black pod rots (Bpod) on the cocoa trees within the hexagons was divided by the number of cocoa trees to obtain the average number of black pod rots per tree. The age of the cocoa trees (CAge) and cocoa varieties (cultivars) were provided by the farmer and verified through observation by the researchers and cocoa extension agents. Cocoa cultivar indicator variables such as Amazonia, Hybrid, and their mixtures were also measured as dummy variables (1 = Yes or 0 = No).
Information on the annual cocoa production output was quantified as the number of bags of dry cocoa beans (a bag is 64.5 kg or 0.0645 t) obtained from the farm by the farmer during the last cropping calendar and verified by the cocoa passbook(s) of the farmer. Williams et al. (1989) and Wang et al. (2022) define crop yield as the magnitude of dry weight (t) of cocoa beans harvested per ha of land cultivated using a given cropping system. Therefore, the total annual cocoa yield in t ha−1 was computed by dividing the product of the number of bags of dry cocoa beans and 0.0645 t by the given farm size (ha) in the present study. The farm size was measured using a handheld GPS device (GPSMap 64, Garmin Ltd., United States).
2.5. Measurement of soil chemical and physical properties
One core of soil sample was taken at 0–30 cm depth from each hexagon along the transect line, mixed thoroughly in a plastic bucket and bulked to make a composite sample. The composite soil samples were well labeled and transported to the Ecological Laboratory of the University of Ghana for processing and analysis. In the laboratory, the samples were air-dried, crushed, and sieved through a 2 mm mesh after removing all visible plant materials for the determination of the soil's chemical and physical properties.
The soil chemical properties measured were pH, SOC, N, P, Ca, Mg, K, Na, Al, and H, and the physical properties were texture and soil ecotype. For the measurement of exchangeable bases (Ca, Mg, K, and Na) in cmolc kg−1, 10 g of the soil sample was extracted with 100 ml of 1 normal ammonium acetate (NH4OAc), buffered at pH 7, and measured using an atomic absorption spectrometer (PINAAcle 900T, Perkin Elmer Inc., Waltham Massachusetts, United States). The sum of Ca++, Mg++, K+, and Na+ yielded the total exchangeable bases (TEB). Effective cation exchange capacity (ECEC) was calculated by adding up TEB and acidity (H+ + Al+++), all in cmolc kg−1. The acidity of the soil was measured by the titration method (McLean, 1965). Base saturation (Bs) was expressed as a percentage of the TEB to the ECEC. Bray-1 method (0.03 M NH4F + 0.025 M HCl) (Bray and Kurtz, 1945) was used to determine available soil P (cmolc kg−1). Soil organic carbon (SOC) g/kg (%) was determined following Walkley and Black method (Walkley and Black, 1934). Soil CN was calculated based on the ratio of SOC to total N. The total N% was determined using a semi-micro Kjeldahl procedure (Bremner, 1996). The soil pH [water] was measured in 1:2 soil–water suspension using a well-maintained and calibrated electrode digital pH meter (EC PCS Testr35, Eutech Oakton Instruments Ltd, United States). The soil's texture (sand, silt, and clay) was determined using the pipette method (Robinson, 1922). All the properties are presented as continuous variables (Xi). The soil ecotypes were determined by superimposing shape files of the soil sampling points on the soil type (LX, FR, and LE) and agroecological zone (DSIZ, WE, and MSSE) maps using ArcGIS 10.4 and labeling the area of intersections (LX.DSIZ, FR.WE, and LE.MSSE) as a factor variable.
Soil biological properties were not measured. However, the soil pH, acidity (AI + H), and SOM/SOC are well known for influencing soil microbial activities and are often used as proxies for soil biological properties (Moebius-Clune, 2016). Thus, the soil parameters were chosen because they are well-known indices of soil health, soil fertility, and crop yield (Moebius-Clune, 2016). The soil health status was assessed based on the ideal range (Table 1) of the soil fertility indices or otherwise the extremes beyond which the indices become toxic (Gaspar and Labosk, 2016; Asare et al., 2017; Doe et al., 2022).
2.6. Measurement of socioeconomic variables
The socioeconomic data include the farmer's age (FAge) in years, number of cocoa training (Ext) attended by the farmer, gender (FSex), nativity (being an indigene or a migrant), and farmland tenancy (LT). Binary numbers 1 = Yes and 0 = No were used to represent social indicator variables such as gender (FSex) where female farmer = 1, male farmer = 0, and being a native farmer = 1 or otherwise = 0. The same applies to land tenancy (LT) arrangements such as outright and inherited farmland ownership. Sharecropping systems such as “abunu” where 2/3 of the cocoa yield goes to the farmer and “abusa” where 1/2 of the yield goes to the landlord were also treated as dummy variables.
2.7. Specification of the green cocoa farm soil productivity function
Plant production ecology theory underpins predictive crop production function and farm soil productivity (Odum, 1968; Scow, 1997; van Ittersum and Rabbinge, 1997). The theory suggests the interaction of biotic and abiotic ecological factors mediates yields of soil productivity. A certain threshold of the interaction generates stimuli (βi) that may trigger a positive (+) or negative (-) magnitude of crop yield and other ecosystem services (Folke et al., 2010; Lal et al., 2015; Lal, 2016). These interactions can be modeled using ecological functions (Odum, 1968; Zuur et al., 2009). In this study, the interaction of the agroecosystem (socioecological) factors (Xi) impacting the yield of green cocoa farm soil productivity (Yi) was expressed according to Zuur et al. (2009) and Zhao et al. (2017) as follows:
where Yi is the response (dependent) variable and Xi is an explanatory (independent or predictor) variable. The functional form of Equation 1 (Figure 4, fully expressed in Supplementary material) depends on the type of relationship between Yi and Xi and the distribution of Yi and Xi (Cobb and Douglas, 1928; Lloyd, 2001). We applied both double-log () and semi-log (αiXi) functional forms as shown in Equation 2.
where A denotes a positive constant (intercept). αi represents a proportionate (%) change (±) in Yi per unit change in a dummy (or factor) variable, Xi = 1 as opposed to Xi = 0, holding other factors constant. βi is technically known as elasticity or responsiveness (%) of Yi to changes in Xi. In other words, βi measures the percentage change in the yield of green cocoa due to a 1% change in each factor, holding other factors constant. The εi denote random error term.
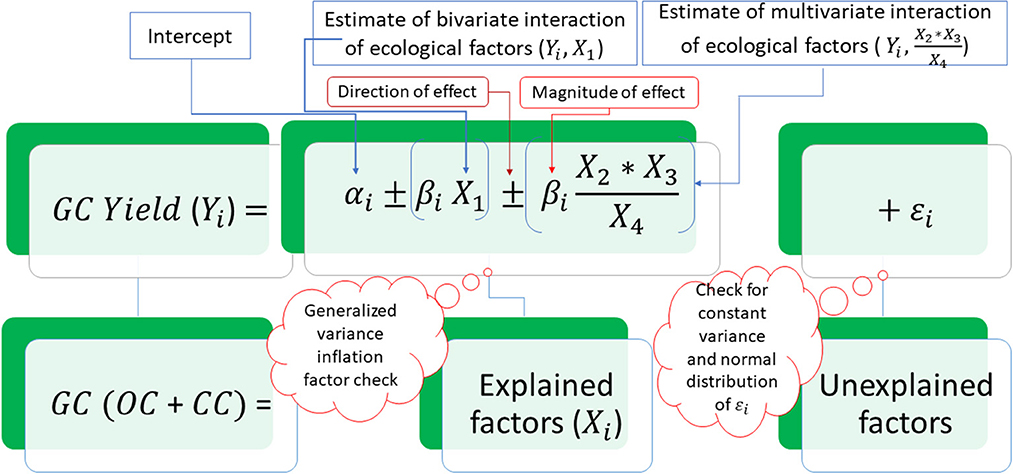
Figure 4. Schematic representation of multiple linear regression method for estimating factor effect on yield (Yi) of green cocoa (GC) based on conventional cocoa (CC) and organic cocoa (OC) agroforestry systems.
The double-log functional form (with the lowest Akaike information criteria) was chosen for this study because of its useful properties, which generate information for managing Yi and Xi to the optimum levels. In a strict double-log functional form, the sum of βi (∑β) is 1, but when generalized, the sum may be ≶ 1. ∑β also denotes a certain degree of homogeneity (λβ) factor for scaling up or down the Yi (Cobb and Douglas, 1928; Lloyd, 2001).
2.8. Statistical estimation and analysis of the data
The statistical analysis of the variables and estimation of the green cocoa productivity function were performed using R version 4.1.3 (R Core Team, 2019). Descriptive statistics such as the mean (μ), standard deviation (SD = σ) and variance (σ2), percentages (%), and boxplots of the variables were computed to describe the attributes of the cropping systems. The coefficient of variability (CV) of the attributes was expressed as σ /μ × 100. A CV ≤ 15% is low (homogenous), while a CV ≥ 36% is high (heterogeneous) (Wilding, 1985).
Bivariate Pearson's correlation coefficients (r) of the relationship between any pair of factors, including the yield, soil ecotypes, and OC and CC systems, were used to explain the synergy. The significant predictors of green cocoa productivity and their magnitudes of effects were examined using multiple regression (t-statistics) estimates of Equation 2. The combined significance of the predictors was determined using F-statistic analysis of variance (ANOVA).
Equation 2 was estimated using ordinary least-square (OLS) regression. The semi-log estimate of Equation 2 is known for minimizing the non-normality of the data, while the double-log minimizes multi-collinearity as well as differences in units of measurement of the variables (O'Brien, 2007). We followed O'Brien (2007) caution to avoid multi-collinearity by dropping some variables. Dropping key variables limits the representativeness of the function to reality, and too many variables would create multi-collinearity. We cautiously dropped a few variables that experienced perfect collinearity, hypo-heterogeneity (too small σ2), and hyper-scedasticity (too small R2 and too large standard errors).
We perform post-estimate regression diagnostics to validate the assumptions εi~N(0, σ2), underlining the use of the OLS. We checked for residual (εi) normality (N), zero (0) mean, and constant variance (σ2). We tested heteroscedasticity (multi-collinearity) using the generalized variance inflation factor (GVIF(1/(2*Df))), equivalence of VIF to satisfy the VIF's “ <10” rule of thumb (Fox and Monette, 1992). According to Fox and Monette (1992), VIF values <2, 5, or 10 have zero, minimum, and moderate (all acceptable) multi-collinearity, respectively. Obtaining these VIF values demonstrates that the estimated F-statistic (ANOVA) and T-statistic (OLS) are reliably valid for scientific interpretations and management decision-making.
3. Results
3.1. Soil health and fertility in soil ecotypes for conventional, organic, and green cocoa
Table 1 shows the soil fertility of the observed cocoa agroforestry systems within the soil ecotypes, with the ideal soil health indicators in parentheses. The SOC which largely defined the soil health of the systems was generally adequate (≥2.5%). It was, however, inadequate (<2.5%) for the conventional cocoa (CC) farms within the Lixisols of Dry Semi-deciduous Inner Zone (LX.DSIZ) and the Ferralsols of Wet Evergreen (FR.WE) soil ecotypes. The average CN ratio was mostly adequate (30.5–40.8%). The base saturation of all the systems and the soil ecotypes was within the range of 80–95% of the ECEC, indicating that all the systems had high soil fertility levels. The ECEC was lower (<16.6%) for the organic cocoa (OC) and CC systems in the FR.WE than in the other soil ecotypes. The FR.WE were more acidic (H+ = 14.7–26.1 cmolc kg−1 with pH = 5.0 to 5.4) than LX.DSIZ and the Leptosols of Moist-Semi-deciduous South-East (LE.MSSE) for both organic and conventional systems. The same applies to the Na. The texture of the investigated soils was generally loam based on United States Department of Agriculture (USDA) textural classes. Silt (43.5%) predominated, followed by sand (37.7%) and clay (18.7%).
3.2. Estimates of conventional, organic, and green cocoa farm soil productivity levels
The total yield of the organic system (1.22 t ha−1) was higher by 0.33 t ha−1 than the conventional system (0.89 t ha−1). The average yield of OC and CC (dash line) which denotes the yield of green cocoa was 1.07 t ha−1 (Figure 5).
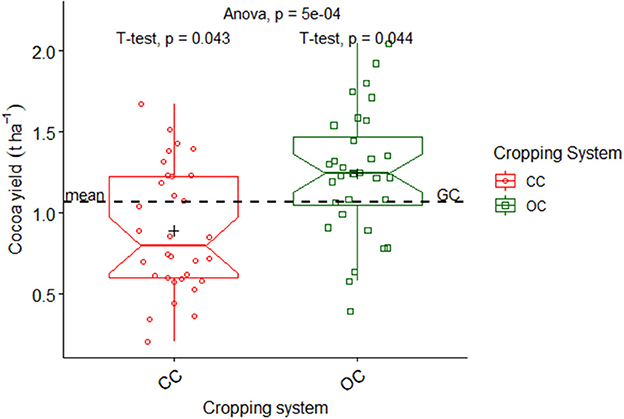
Figure 5. Yields of conventional cocoa (CC) and organic cocoa (OC); t-test compares the mean (+) yield of each cropping system to the overall mean (GC) represented by the dashed lines (—).
The cocoa varieties of the cropping systems were predominantly Amazonia (55.6%) and Hybrid (25.4%) cultivars. The mean cocoa tree plant density was 7.46 ± 2.58 per 23.4 m2, which implies, for each hexagon (six equilateral triangles) or three rhombi, the planting density was exceeded by one plant. The organic system was lower in plant density per 23.4 m2 (Pltdn = 6.43 ± 1.81) than the conventional system (Pltdn = 8.49 ± 2.85). The OC trees were older (17.40 ± 6.20 years) than the CC trees (13.70 ± 6.28 years). The mean number of black pods was 1 per tree (Bpod = 0.89).
The socioeconomic attributes of the farmers suggest that the cropping systems were predominantly managed by male cocoa farmers (79.9%) and a few females (20.1%). On average, both farmers were approximately 51 ± 15 years old. Approximately 57.1% of them had attended college (middle or junior high school). The farmers were natives (52.4%) and migrants (47.6%) who outrightly owned (25.4%) or inherited (44.4%) their cocoa farmlands. Each farmer had attended cocoa training (Ext) at least four times (Ext = 4.06 ± 2.24) during the last cropping calendar.
3.3. Synergy of bivariate correlation of the ecological factors
Figure 6 shows the bivariate (pairwise) correlation coefficients (r) explaining the relationships of the observed ecological factors. High blue color saturation signifies a high positive relationship. On the contrary, high red color saturation depicts a high negative relationship. The r illustrates the extent of desirability or undesirability of the relationships. There was a more desirable synergy of the factors, with the yield of OC and GC than the CC yield. For example, the cocoa yield had a significant positive correlation with female farmers (FSex) who also had a significant positive correlation with their farm soil organic CN ratio (Figure 6). In the three soil ecotypes, the cocoa yield increased significantly and insignificantly with aging of cocoa trees in the OC and CC systems, respectively (Figure 7). However, the correlation of the cocoa yield with soil available P was significantly positive for only the OC system in the FR.WE soil ecotype (Figure 8). The multiple regression analysis quantifies the effects of the factors.

Figure 6. Pairwise correlation matrix showing the interrelationship of ecological factors within the observed cocoa agroforestry systems. High blue color saturation signifies a high positive relationship. On the contrary, high red color saturation depicts a high negative relationship. The values of the r show the extent of desirability or undesirability of the relationships, to illustrate the synergy of interactions of the ecological factors in the agroecosystems. Yield refers to green cocoa yield.
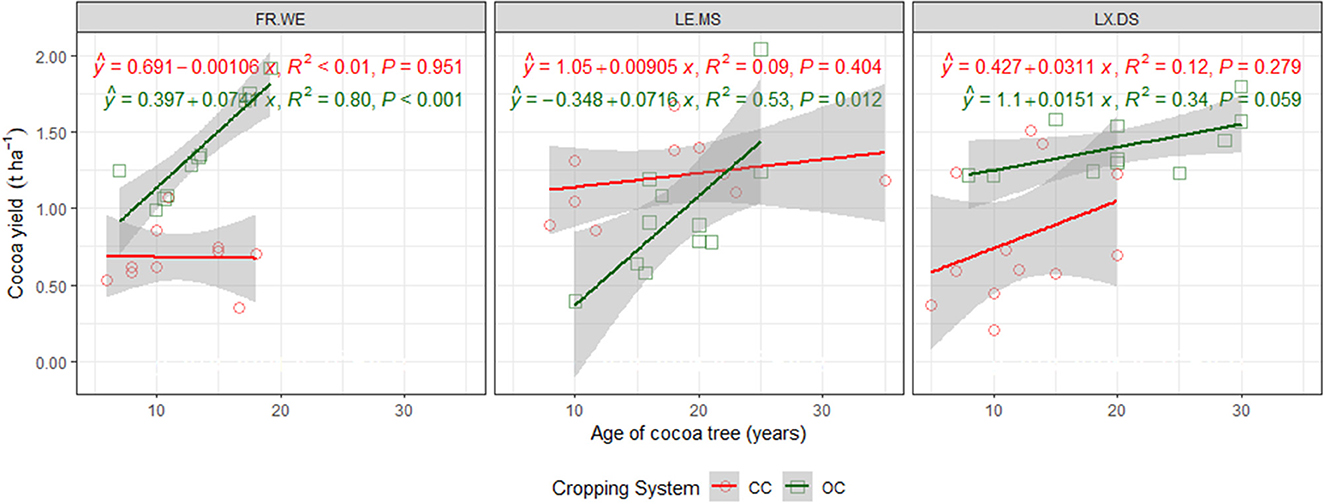
Figure 7. Trends of conventional (CC) and organic cocoa (OC) yields in relation to aging cocoa trees within the three soil ecotypes.
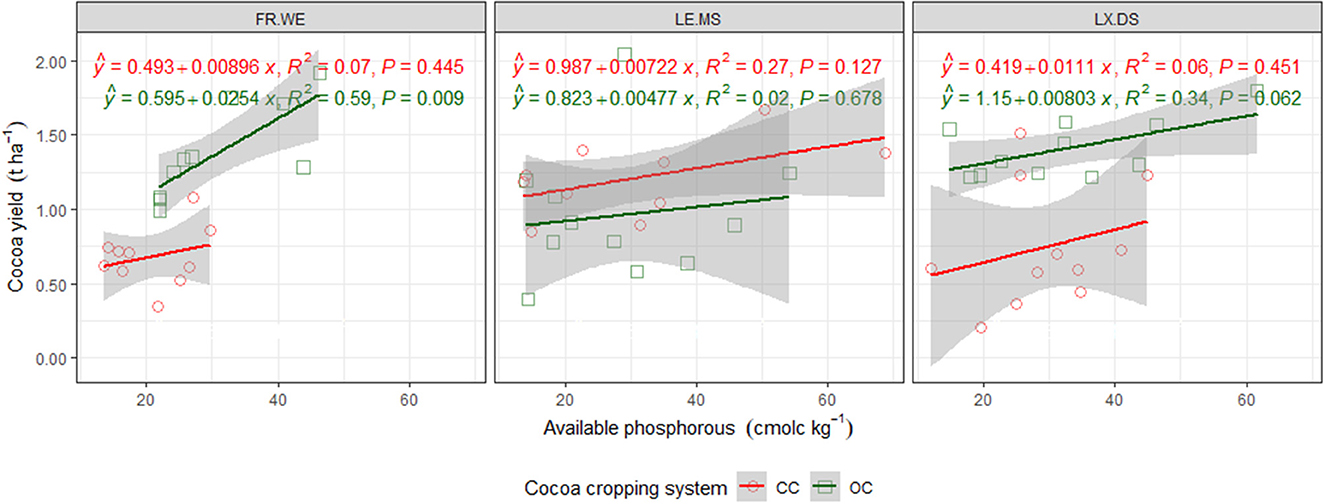
Figure 8. Trends of conventional (CC) and organic cocoa (OC) yields in relation to the concentration of available soil P (cmolc kg−1) within the three soil ecotypes.
3.3.1. Estimate and diagnostic results of the green cocoa farm soil productivity function
Figure 9 and Supplementary Table 1 presents the OLS regression estimates of the green cocoa productivity function, and Table 2 shows the GVIF diagnostic results. As shown in Table 1, the mean GVIF(1/(2*Df)) was close to 2 (2.034), which is equivalent to a VIF of 4.643, implying multi-collinearity was less than 5 (Fox and Monette, 1992; O'Brien, 2007). The diagnostics suggest that the and are reliable for testing hypotheses and making scientific interpretations and decisions since all the assumptions of OLS (Zuur et al., 2009) have been met. For instance, the mean residual ( = 0.005) and standard error (0.2375) are close to zero (SW-test of residual = 0.991, p = 0.931). The heteroscedasticity (non-constant variance) was not statistically significant (chi-square = 3.155, Df = 1, p = 0.076). In addition, the ANOVA was significant (F-statistic = 10.98, p < 0.0001), indicating the function (regression) fits the data well. The adjusted R2 (73.8%) suggests the explanatory variables jointly explained 73.8% of the variation in the observed cocoa yield.
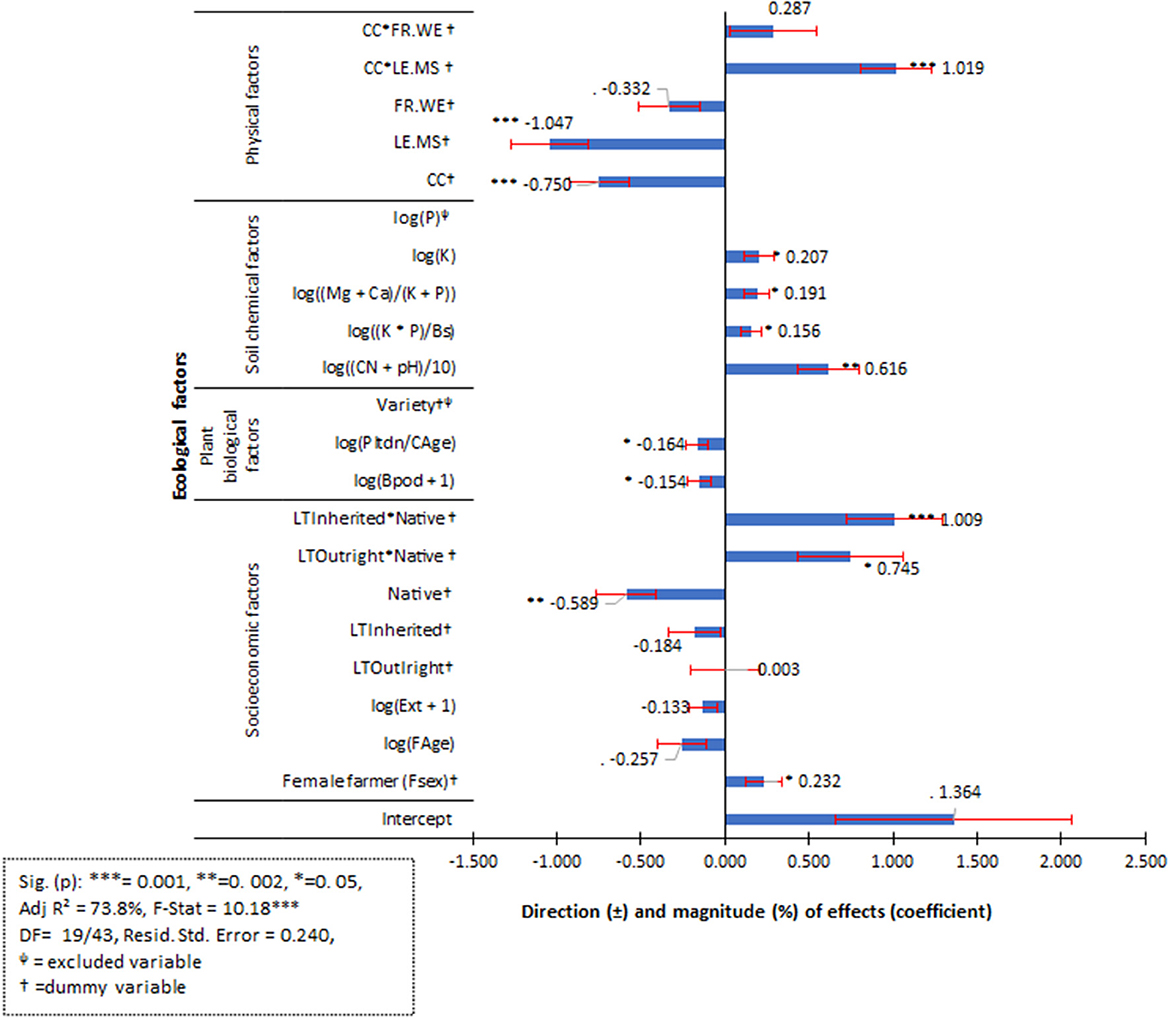
Figure 9. The ordinary least square regression estimates show factor influence on green cocoa farm soil productivity; † = dummy variable (Yes = 1, No = 0); φ = excluded variable.
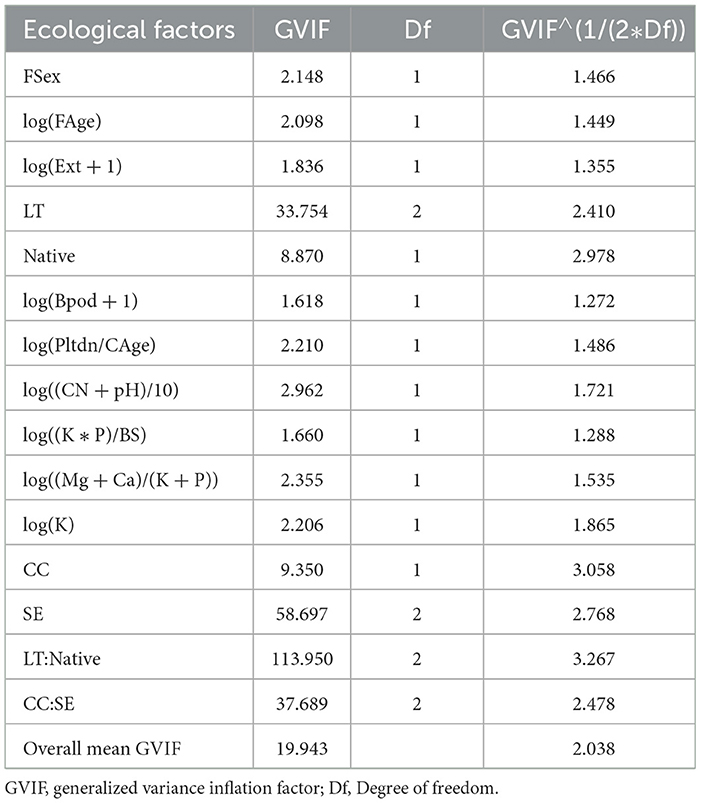
Table 2. Estimates of generalized variance inflation factors for the green cocoa productivity function.
3.3.2. Factors predicting the yield of green cocoa farm soil productivity and their magnitudes
There were 13 statistically significant (p < 5%) predictors of green cocoa farm soil productivity and their magnitudes of determining the yield, as presented in Figure 9. Among the significant physical factors are CC ( −0.750%) and LE.MSSE ( = −1.047%), which had negative effects on the yield, but their combined interactional (CC* LE.MSSE) effect was positive ( = 0.732%). The interaction of CC and LE.MSSE had the largest positive effect (1.019%). The LE.MSSE (−1.047%) effect was negative. The positive significant socioeconomic factors were gender (Fsex: 0.232%) and natives who had outright ( = 0.745%) or inherited ( 1.009%) land ownership. The individual effect of being a native ( = −0.589%) was negative. Of the crop biological factors, the cocoa black pod disease (Bpod: −0.154%) and the ratio of cocoa plant density to age (: = −0.164) exerted adverse effects on the yield. In terms of the soil chemical factors, the responsiveness of the yield to ( = 0.616%), and ( 0.191%) was positive. This implies, for instance, when the proportion of K and P in the Bs () increases by 1%, the yield would increase by 0.156%, holding other factors constant. When Bpod rises by 1%, the yield drops by 0.154%. The interaction of CN and pH exerted the largest impact of 0.616% among the soil chemical factors.
The double-log portion of the function suggests that the sum of ( = 0.8.71) is < 1, meaning there is a decreasing return to scale. In other words, when all the observed factors increase by λ0.871, the GC yield increases by less than λ0.871. Without the disease incidence (Bpod) and the interaction of cocoa plant density and age (), would be equal to 1.163. The 1.163 () denotes an increasing return to scale, suggesting that the yield would be more than double when all chemical inputs are doubled. Table 3 provides details on the marginal effects of each factor.
4. Discussion
4.1. Soil health status of the observed cocoa agroforestry systems
In general, the soil fertility indices (SOC, CN, pH, P, K, Na, H, Bs, and ECEC) measured in the three soil ecotypes were within the ideal soil health indicator ranges (Table 1). The findings are consistent with the reports of Ofori-Frimpong et al. (2010), Arthur et al. (2017), and Kongor et al. (2019) who also reported similar soil fertility indices. However, we found that the indices exhibited high variability, evident in the coefficient of variability (CV). The CV was either moderate (16–35%) or high (>35%) in SOC, CN, P, K, and ECEC. The moderate and high levels of variability raise soil health risks if the usual blanket approach to soil fertility management is to be applied (Dossa et al., 2018a,b; Doe et al., 2022). Hence, we recommend soil ecotype-specific soil fertility management or fertilizer regimes to minimize soil health risks and sustain healthy cocoa farm soil productivity in the study area.
4.2. The synergy of interrelationships of the factors affecting cocoa yield
The results showed significant (p < 0.05) correlations that demonstrate the synergy of interacting factors in the cocoa agroforestry systems, though most of the correlation coefficients (r±) were weak (< 0.5). The factors interacting in the green and organic cocoa systems were in more harmonic sync with cocoa farm soil productivity than the conventional system. For instance, the soil pH (r = 0.30), female cocoa farmers (r = 0.37), SOM (r = 0.39), P (r = 0.40), OC (r = 0.42), cocoa tree age (r = 0.48), SOC (r = 0.49), and CN (r = 0.71) correlated positively with the green cocoa yield. These factors were also positively correlated with the organic system but negatively correlated with the conventional system. These observations affirm Ofori-Frimpong et al. (2010) and Asigbaase et al. (2020, 2021) who recounted high SOC, SOM, pH, CN, P, and K in organic cocoa agroforestry systems.
In addition, the observed positive relationship (r = 0.41) between the green cocoa yield and CN/SOC in fields owned by female farmers suggests that CN/SOC accumulation was impacted by gender differences in cocoa management practices. This finding is congruent with the previous report by Mensah et al. (2021) that women were more environmentally conscious than men. The finding suggests that prioritizing gender support for women can improve soil health and productivity for green, conventional, and organic cocoa cropping systems. This could be achieved through intensifying agricultural extension services, financing, and mechanical or physical labor support for the women.
Corroborating Ofori-Frimpong et al. (2010), the SOC (r = −44) and CN (r = −45) were negatively related to overstocked cocoa tree density. Similarly, correlations of the green cocoa yield with black pod rot (r = −0.18), silt (r = −0.30), and planting density (r = −0.56) were negative. These findings confirm the report by Asante et al. (2021), who argued that poor cocoa planting distance and disease management are bottlenecks to closing the conventional cocoa yield gap in Ghana.
Consistent with Arthur et al. (2017) and Quaye et al. (2021), we observed that the green cocoa system had negative correlations with soil pH (r = −0.65), K (r = −0.37), and CEC (r = −0.82) in the Ferralsols of Wet Evergreen (FR.WE) soil ecotype. The FR.WE are usually acidic (< 5.6) in nature due to high rainfall and leaching, and high acidity reduces their cation exchange capacity (CEC).
On the contrary, the Lixisols of the Dry Semi-deciduous Inner Zone (LX.DSIZ) (r = 0.44) and Leptosols of Moist-Semi-deciduous South-East (LE.MSSE) (r = 0.36) were positively correlated with CEC and soil pH. While the LE.MSSE had positive correlations with soil pH (r = 0.31), SOC (r = 36), K (r = 0.39), and SOM (r = 0.41), the LX.DSIZ had adverse correlations with soil pH (r = −0.32), SOC (r = −35), K (r = −0.02), and SOM (r = −0.46). These findings are consistent with FAO and ITPS (2015), who reported that the correlation between the observed soil ecotypes and their soil properties is due to parent materials and the climate of the soil ecotypes.
4.3. Yields of organic and conventional cocoa combined to form green cocoa yield
The observed average green cocoa yield (1.07 t ha−1) exceeded the national mean of 0.50 t ha−1 for Ghana (FAOSTAT, 2021), and the farm level yield (0.21–0.65 t ha−1) was reported by Abdulai et al. (2020). However, it is lower than the 23-year experimental cocoa yield (1.37 t ha−1), reported by Ramírez-Argueta et al. (2022), and the potential/water-limited yields (2.5 to 3.5 t ha−1) reported by van Vliet and Maja Slingerland (2015) and Asante et al. (2022). The average crop yield curve of farmers usually plateaus (flattens) around 75-80 % of the potential yield ceiling (Dobermann et al., 2013). The maximum water-limited/potential yields are usually high because they are obtained in a controlled environment (climate), with sufficient plant water, soil nutrients, and low pests and diseases Dobermann et al., 2013; Van Ittersum et al., 2013). Smallholder farmers generally operate under rainfed conditions, characterized by all the potential yield-limiting factors, including soil ecotype and socioeconomic constraints. To reduce the yield gap, high-yielding local cocoa varieties that are resistant to abiotic and biotic stresses and healthy soil fertility management practices are required. In addition, financial constraints, limited availability of organic and inorganic fertilizers, and agrochemicals to smallholders (Amponsah-Doku et al., 2021) need to be addressed per soil ecotype. Given the conditions described above, we recommend an integrated ecological cropping system such as the green cocoa cropping system to enhance the sustainability of agroecosystem services while closing yield gaps.
We also found a higher yield for OC (1.24 t ha−1) compared with the CC (0.89 t ha−1), which confirms the accounts of Badgley et al. (2007), Rajab et al. (2016), and Bandanaa et al. (2021) that OC systems and cocoa shade diversity are equally productive as the conventional monocrop cocoa systems. However, the results contradict the findings of Asigbaase et al. (2020) who found that the yield of OC was 30% lower than that of CC. Several ecological factors explain the aforementioned superiority of the GC and OC yields to the CC yield. Ofori-Frimpong et al. (2010), Tscharntke et al. (2012), and Somarriba et al. (2013) attributed high yield in OC systems to superior biodiversity and physicochemical soil quality build-up over time. On the contrary, Herzog et al. (2019) and Smith et al. (2019) found that the duration (age) of organic cocoa cropping systems had no relationship with the yield. The present study demonstrated that the cocoa yield of the organic cropping system increased with aging of the cocoa trees and their physicochemical properties compared with the CC system. This demonstration is consistent with the findings by Ofori-Frimpong et al. (2010), Tscharntke et al. (2012), Somarriba et al. (2013), and Asigbaase et al. (2020) who argued that the OC system builds up more soil physicochemical properties over time than the CC cropping system. Thus, improved soil physicochemical properties in the OC and GC systems simulate plant growth, prolong plant life, and increase yield. The build-up of physicochemical properties in the OC and GC systems emanates from integrated organic soil and crop husbandry practices. Integrated organic husbandry practices such as the use of bioinsecticides and biological pest control and application of organic manure and compost increase soil organic matter. The soil organic matter provides a binding agent that improves soil structure and stimulates soil microbial activities (Asigbaase et al., 2020, 2021). An improved soil structure retains soil moisture and nutrients, while soil microbial activities aid in the mineralization of other soil nutrients, such as plant available P and exchangeable K for uptake by cocoa plants. Furthermore, the use of bioinsecticides and biological (natural) pest controls in the OC and GC systems enhances the defense mechanisms of cocoa plants against insect pests that cause yield loss (Krey et al., 2020; Akesse-Ransford et al., 2021). On the contrary, the sole use of mineral fertilizers and pesticides in pure CC can kill or reduce the population of the natural enemies of the pests, leading to higher pest yield losses in pure CC relative to the OC and GG systems.
4.4. Ecological factors determining green cocoa yield and magnitude
For the ecological determinants (predictors) of green cocoa farm soil productivity, we found several significant factors based on the estimated cocoa productivity function. We found that the green cocoa yield responded positively to the LE.MSSE but negatively to the FR.WE. This is probably because of the higher concentrations of physicochemical properties in the LE.MSSE and LX.DSIZ than the FR.WE. The concentrations of P and K and percentages of CN, ECEC, and base saturation in the LE.MSSE and the LX.DSIZ were higher than those in FR.WE. These findings are not surprising because of the relatively higher rainfall regimes of the FR.WE compared with the LE.MSSE and the LX.DSIZ soil ecotypes. Doe et al. (2022) indicated that Leptosols in the Volta Region of Ghana have healthy soils due to their relatively larger clay loam content and deep SOM layer.
We also found that, while the age of the cocoa trees (CAge = 16 ± 7 years) positively affected the yield, an overstocked cocoa plant density (per 23.4 m2) and black pod rot disease occurrence inversely affected the yield, corroborating Ahenkorah (2016) and Kongor et al. (2018). The yield within the organic system appears more resilient to aging cocoa trees than the conventional system. This implies that older OC trees (17.40 ± 6.20 years old) were more productive than the younger CC trees (13.70 ± 6.28 years old). In addition, overstocking mature cocoa trees (>7 cocoa trees per 23.4 m2) and having one black pod rot disease on each tree would reduce the cocoa yield. This observation underscores the undesirable humid condition associated with an overstocked cocoa plant population. The results reiterate the previous findings indicating that non-conformity to recommended regular plant distance (or plant density) reduces cocoa yield (Ofori-Frimpong et al., 2010; Asante et al., 2021). An overcrowded cocoa plant density redirects photosynthates and encourages competition (trunk and stem elongations) for sunlight at the expense of maturation, flowering, fruiting, and pod development. It retards ventilation and creates undesirable humid conditions that facilitate fungal diseases such as cocoa black pod rots (Ofori-Frimpong et al., 2010; Akrofi et al., 2015). Our findings suggest a need to intensify good agronomic practices such as regular pruning to improve plant spacing (Pltdn) and disease management using bioinsecticides.
The present study corroborates previous findings on planting distance (Ofori-Frimpong et al., 2010; Asante et al., 2021), and the novelty of our study lies in the method used to estimate the cocoa plant density on the field. Our method differs from the usual indicator variable approach (regular or irregular planting distance) reported in the study by Asante et al. (2021) and many other studies. Our method quantified the number of cocoa trees within a 23.4 m2 hexagon, which is equivalent to three congruent rhombi of 3 m sides, which must ideally have seven cocoa trees, based on COCOBOD recommendation (CHED/WCF, 2016). This method unbiasedly permitted a more accurate measure of the cocoa planting density in often irregular stands of cocoa trees planted at stakes during the establishment by smallholder farmers. The method is, however, more laborious and time-consuming than the indicator variable approach, but it is certainly more accurate than the indicator variable approach.
Corroborating Asigbaase et al. (2021) and Li et al. (2021), we found that the combined effect of CN (40.1 ± 13.8%) and soil pH (5.8 ± 0.7) dominates the chemical determinants of green cocoa yield. The CN facilitates microbial activities for decomposing more SOM to enhance CEC and retention of cocoa plant nutrients (Somarriba et al., 2013; Rajab et al., 2016; Eddy and Yang, 2022). This explains why soil pH, acidity, SOM, and SOC management are widely accepted as a technique for restoring soil health and fertility for sustainable food crop production and mitigating climate change.
We also found that the overall base saturation (Bs = 0.917) was high (91.7%), but a majority of the individual elements (Ca, Mg, K, and Na) were insignificant. This implies that the decomposition of the individual effects of the elements is essential for farm-level soil fertility and productivity management decisions. However, the combined effect of the elements is even more essential. The positive effect of is congruent with Ahenkorah (2016) and Dogbatse et al. (2021). This finding suggests that when the proportion of K*P to Bs () increases by 1%, the yield of GC would increase by 0.156%, holding all other factors constant. In addition, the significance of implies holding all other factors constant, this set () of determinants would generate a 0.191% extra green cocoa yield. Another implication of the interaction effects of both and is that managing the proportion of Ca+Mg to K+P well is critical for green cocoa farm soil productivity. This is essential, particularly when the individual elements have insignificant effects on the yield.
We are not recommending a continuous application of P, Ca, K, and Mg beyond their maximum thresholds. As a rule of thumb, the theory of base cation saturation ratio (Gaspar and Labosk, 2016) limits the proportions of Na+, H+, K+, Mg++, and Ca++ to 0–1%, 0–10%, 4–8%, 12–25%, and 65–80% of the base saturation (Bs), respectively. The results showed that the proportion of K (1.2%) to the Bs of the observed soil was far lower than the ideal minimum of 4%. The same applies to the proportions of Mg (40.9%) and Ca (53.9%) to Bs. On the contrary, the Bs of Na and H exceeded their maximum proportions of 1% and 10%, respectively.
The observed soils appear fertile on the face value of Mg and Ca but limiting in K base saturations. Therefore, the positive influence of the combined effects of K, Ma, and Ca on green cocoa yield is only attainable through effective management of soil fertility and soil health. This can be carried out by enriching the SOC, Ca, Mg, and K contents while reducing the sodic (salty) and acidic effects of excessive Na and H, respectively. Common SOC sources in Ghana are organic manure, compost, rice husks, corncobs, biochar, cocoa pod husk potash, and empty oil palm bunches.
It was also noted that the marginal effect of exchangeable K on the green cocoa yield was greater than the marginal effect of available P, which implies P is the most limiting factor, as reported by Dossa et al. (2018a,b). According to Liebig's law of the minimum, soil productivity is determined by the most limiting soil nutrient or factor. The available soil P limitation can be addressed by tapping total P or developing a P-efficient cocoa variety to limit the amount of P lost to the environment.
Improving the efficiency of P-use can also be achieved using soil microorganisms in a root-foraging strategy, where plants uptake more P at a lower P critical level (Richardson et al., 2011). For instance, Richardson et al. (2011) employed P-mining strategies to enhance P-desorption, solubilization, and mineralization of soil nutrients when P was sparingly available in the soil.
Nevertheless, the dominant effect of the exchangeable K and the limiting effect of the available P have important implications for soil nutrient amendment and cocoa site-specific fertilizer formulation. The K effect suggests that the conventional farms in LX.DSIZ and FR.WE, require more P than K. The organic farms in the LE.MSSE require more of the same because the Ferralsols and Lixisols have a lower soil pH than the Leptosols. Liming materials (Calciprill, limestone, and dolomite) are effective in acidic soils and may help in P dissolution, unlocking available P, decreasing A+++, and increasing exchangeable base cations (Ca++, Mg++, and K+).
5. Conclusion
The study proved that the observed cocoa systems exhibit high soil health, fertility, and yield. The cocoa farm soil productivity was high in the order as follows: conventional cocoa (CC) < green cocoa (GC) < organic cocoa (OC), particularly in the landscapes of FR.WE, LX.DSIZ and LE.MSSE soil ecotypes of Ghana. For all three soil ecotypes, it was concluded that green cocoa yield responds positively to female cocoa farmers because female cocoa farmers correlate positively with the soil organic carbon and nitrogen ratio in their farms. The cocoa productivity within the organic system appears more resilient to aging cocoa trees than the conventional system. One black pod rot disease in an overstocked mature cocoa plant density per 23.4 m2 can reduce cocoa yield. While soil ecotype predominates soil physical factor effects, the combined effect of soil chemical factors such as CN ratio and pH dominates the yield of green cocoa productivity. Doubling the sum () interaction effects of soil pH, CN, P, Ca, Mg, K (, and ) more than doubles the yield, holding limiting factors like diseased pods, aged and overcrowded cocoa tree density constant, among others. Thus, the critical determinants of green cocoa (integrated CC+OC) productivity are not limited to the individual effects of CN ratio, pH, P, Ca, Mg, and K but their combined synergy of effects including soil ecotype, cocoa plant density, black pod disease, and aging of the cocoa trees. In addition, gendered support for female cocoa farmers owning farmlands is likely to be a major determinant of green cocoa farm soil productivity. Hence, promoting female cocoa farmers with SOC, CN, and pH conservation is critical to soil health and green cocoa development in Ghana. These conclusions are pertinent to the LE.MSSE, LX.DSIZ, and FR.WE soil ecotypes.
The research has expanded the boundaries of ecological theory, specifically the concepts of cocoa green productivity, soil health, cocoa farm diagnostics, and yield improvement strategies in smallholder cocoa agroforestry systems. The study demonstrates a quantitative ecology methodological pathway for generating empirical evidence on the determinants of green cocoa productivity and cocoa agroecosystem assessment at the farmer/farm level. This includes methods for quantifying cocoa plant density in an irregularly spaced field of cocoa trees.
Limitations: we recognized that statistical regression models of crop production are a simplified version of the actuals. Topographic factors such as altitude and slope of farmland were held constant. Owing to the rainfed nature of cocoa farming in the study area, soil moisture, and climatic factors such as rainfall, relative humidity, sunshine, and evapotranspiration were assumed to be constants per soil ecotype.
Data availability statement
The raw data supporting the conclusions of this article will be made available by the authors, without undue reservation.
Ethics statement
The studies involving human participants were reviewed and approved by Ethics Committee for Humanities, University of Ghana. The patients/participants provided their written informed consent to participate in this study.
Author contributions
ED: research idea, concept and design, fieldwork, data analysis, and writing of the manuscript. EA, PO, and AQ: supervision, contributing, and commenting. BF-M: contributing, commenting, and editing. All authors reviewed and approved the manuscript submitted.
Funding
The study was funded in part by the Queen Elizabeth Scholarship – Advanced Scholars Program (QES-AS), with the University of Ghana and partly by the Banga-Africa Project supported by Carnegie Foundation New York. The QES-AS is made possible through the Canadian Queen Elizabeth II Diamond Jubilee Scholarship Program and funded by Canada's International Development Research Centre (IDRC), the Social Science and Humanities Research Council of Canada (SSHRC), and Carleton University.
Acknowledgments
The authors are grateful to the Queen Elizabeth Scholarship Advanced Scholars Program, University of Ghana, and the Banga-Africa Project for financing the field and lab costs. We would like to thank Samuel Senyo Koranteng of the Institute of Environmental Sanitation and Studies (IESS), Prince Owusu, Amoako Ofori, Amarteifio Nii Amartei, and Christian Drah of the University of Ghana ECOLAB for their immense technical support. We are grateful to Yayra Glover Ltd. and staff George Dotse, Christian Asiedu, Emmanuel Aryee, Kwasi Owusu, and Obuoba Sikahene. We also would like to thank Ghana COCOBOD and staff Eric Koka Bani (CHED), Frank Osei (REC), Kwaku Agyepong (DEC), Samuel Oduro (DEC), Forgor Richard Basig (CEA), Prince Adansi. Bonna (CEA), and SugloWisdom Maaliyiri (CEA). We would like to appreciate all cocoa farmers and PCs who participated in the study.
Conflict of interest
The authors declare that the research was conducted in the absence of any commercial or financial relationships that could be construed as a potential conflict of interest.
Publisher's note
All claims expressed in this article are solely those of the authors and do not necessarily represent those of their affiliated organizations, or those of the publisher, the editors and the reviewers. Any product that may be evaluated in this article, or claim that may be made by its manufacturer, is not guaranteed or endorsed by the publisher.
Supplementary material
The Supplementary Material for this article can be found online at: https://www.frontiersin.org/articles/10.3389/fsufs.2023.1169015/full#supplementary-material
References
Abdulai, I., Hoffmann, M. P., Jassogne, L., Asare, R., Graefe, S., Tao, H. H., et al. (2020). Variations in yield gaps of smallholder cocoa systems and the main determining factors along a climate gradient in Ghana. Agric. Syst. 181, 102812. doi: 10.1016/j.agsy.2020.102812
Ahenkorah, Y. (2016). Towards Advancement of Knowledge: Scientific Research into Cocoa Nutrition and Other Soils. Edited by Y. Ahenkorah, C. O. Ofosu, and K. Nu. Appiah. Accra, Ghana: Ghana Academy of Arts and Sciences.
Akesse-Ransford, G., Owusu, E. O., Kyerematen, R., and Adu-Acheampong, S. (2021). Arthropod diversity of cocoa farms under two management systems in the Eastern and Central regions of Ghana. Agroforest. Sys. 95, 791–803. doi: 10.1007/s10457-020-00568-5
Akrofi, A. Y., Amoako-Atta, I., Assuah, M., and Asare, E. K. (2015). Black pod disease on cacao (Theobroma cacao, L) in Ghana: spread of Phytophthora megakarya and role of economic plants in the disease epidemiology. Crop. Prot. 72, 66–75. doi: 10.1016/j.cropro.2015.01.015
Amponsah-Doku, B., Daymond, A., Robinson, S., Atuah, L., and Sizmur, T. (2021). Improving soil health and closing the yield gap of cocoa production in Ghana–a review. Scient. Af. 15, e01075. doi: 10.1016/j.sciaf.2021.e01075
Aneani, F., and Ofori-Frimpong, K. (2013). An analysis of yield gap and some factors of cocoa (theobroma cacao) yields in Ghana. Sustain. Agric. Res. 2, 117. doi: 10.5539/sar.v2n4p117
Appiah, M. R., Sackey, S. T., Ofori-Frimpong, K., and Afrifa, A. A. (1997). The consequences of cocoa production on soil fertility in Ghana: a review. Ghana J. Agricult. Sci. 30, 183–190. doi: 10.4314/gjas.v30i2.1970
Arthur, A., Acquaye, S., Cheng, W., Dogbatse, J. A., Konlan, S., Domfeh, O., et al. (2022). Soil carbon stocks and main nutrients under cocoa plantations of different ages. Soil Sci. Plant Nutr. 68, 99–103. doi: 10.1080/00380768.2022.2029219
Arthur, A., Afrifa, A. A, and Dogbatse, J. A. (2017). “Assessment of Soil Fertility Status of Cocoa Farms around the Ankasa National Park in the Jomoro District of the Western Region of Ghana.” in International Symposium on Cocoa Research (ISCR), 13–17. Lima, Peru.
Asante, P. A., Rahn, E., Zuidema, P. A., Rozendaal, D. M., van der Baan, M. E., Läderach, P., et al. (2022). The cocoa yield gap in Ghana: a quantification and an analysis of factors that could narrow the gap. Agric. Syst. 201, 103473. doi: 10.1016/j.agsy.2022.103473
Asante, P. A., Rozendaal, D. M., Rahn, E., Zuidema, P. A., Quaye, A. K., Asare, R., et al. (2021). Unravelling drivers of high variability of on-farm cocoa yields across environmental gradients in Ghana. Agric. Syst. 193, 103214. doi: 10.1016/j.agsy.2021.103214
Asare, R., Asare, R. A., Asante, W. A., Markussen, B. O., and Ræbild, A. (2017). Influences of shading and fertilization on on-farm yields of cocoa in Ghana. Exp. Agricult. 53, 416–431. doi: 10.1017/S0014479716000466
Asare, R., Markussen, B., Asare, R. A., Anim-Kwapong, G., and Ræbild, A. (2019). On-farm cocoa yields increase with canopy cover of shade trees in two agro-ecological zones in Ghana. Clim. Develop. 11, 435–445. doi: 10.1080/17565529.2018.1442805
Asase, A., and Tetteh, D. A. (2016). Tree diversity, carbon stocks, and soil nutrients in cocoa-dominated and mixed food crops agroforestry systems compared to natural forest in southeast Ghana. Agroecol. Sustain. Food Sys. 40, 96–113. doi: 10.1080/21683565.2015.1110223
Asigbaase, M., Dawoe, E., Lomax, B. H., and Sjogersten, S. (2021). Biomass and carbon stocks of organic and conventional cocoa agroforests, Ghana. Agric. Ecosyst. Environ. 306, 107192. doi: 10.1016/j.agee.2020.107192
Asigbaase, M., Lomax, B. H., Dawoe, E., and Sjogersten, S. (2020). Influence of organic cocoa agroforestry on soil physico-chemical properties and crop yields of smallholders' cocoa farms, Ghana. Ren. Agricult. Food Sys. 36, 255–264. doi: 10.1017/S1742170520000290
Asitoakor, B. K., Asare, R., Ræbild, A., Ravn, H. P., Eziah, V. Y., Owusu, K., et al. (2022). Influences of climate variability on cocoa health and productivity in agroforestry systems in Ghana. Agricult. Forest Meteorol. 327, 109199. doi: 10.1016/j.agrformet.2022.109199
Badgley, C., Moghtader, J., Quintero, E., Zakem, E., Chappell, M. J., Aviles-Vazquez, K., et al. (2007). Organic agriculture and the global food supply. Ren. Agricult. Food Sys. 22, 86–108. doi: 10.1017/S1742170507001640
Bandanaa, J., Asante, I. K., Egyir, I. S., Schader, C., Annang, T. Y., Blockeel, J., et al. (2021). Sustainability performance of organic and conventional cocoa farming systems in Atwima Mponua District of Ghana. Environ. Sustain. Indicat. 11, 100121. doi: 10.1016/j.indic.2021.100121
Blaser, W. J., Oppong, J., Hart, S. P., Landolt, J., Yeboah, E., and Six, J. (2018). Climate-smart sustainable agriculture in low-to-intermediate shade agroforests. Nat. Sustain. 1, 234–239. doi: 10.1038/s41893-018-0062-8
Bray, R. H., and Kurtz, L. T. (1945). Determination of total, organic, and available forms of phosphorus in soils. Soil Sci. 59, 39–46. doi: 10.1097/00010694-194501000-00006
Bremner, J. M. (1996). Nitrogen-total. Methods of soil analysis. Part 3 Chem. Meth. 5, 1085–1121. doi: 10.2136/sssabookser5.3.c37
Castle, S. E., Miller, D. C., Merten, N., Ordonez, P. J., and Baylis, K. (2022). Evidence for the impacts of agroforestry on ecosystem services and human well-being in high-income countries: a systematic map. Environ. Evid. 11, 1–27. doi: 10.1186/s13750-022-00260-4
Castle, S. E., Miller, D. C., Ordonez, P. J., Baylis, K., and Hughes, K. (2021). The impacts of agroforestry interventions on agricultural productivity, ecosystem services, and human wellbeing in low-and middle-income countries: a systematic review. Campbell Sys. Rev. 17, e1167. doi: 10.1002/cl2.1167
CHED/WCF (2016). Manual for Cocoa Extension in Ghana. 2016th ed. COCOBOD-Cocoa Health and Extension Division and World Cocoa Foundation. doi: 10.1017/CBO9781107415324.004
Cobb, C. W., and Douglas, P. H. (1928). A Theory of Production. Am Econ Rev. 18, 139–165. Available online at: https://www.jstor.org/stable/1811556
Conway, G. R. (1987). The properties of agroecosystems. Agric. Syst. 24, 95–117. doi: 10.1016/0308-521X(87)90056-4
Dobermann, A., Nelson, R., Beever, D., Bergvinson, D., Crowley, E., Denning, G., et al. (2013). Solutions for sustainable agriculture and food systems. Sustain. Develop. Solut. Network. Available online at: https://resources.unsdsn.org/solutions-for-sustainable-agriculture-and-food-systems (accessed April 20, 2023).
Doe, E. K., Attua, E. M., Dogbatse, J. A., and Fosu-Mensah, B. Y. (2022). Assessing the condition and capability of soils in cocoa districts of Ghana using geovisualization. Soil Sec. 7, 100058. doi: 10.1016/j.soisec.2022.100058
Dogbatse, J. A., Arthur, A., Awudzi, G. K., Quaye, A. K., Konlan, S., and Amaning, A. A. (2021). Effects of organic and inorganic fertilizers on growth and nutrient uptake by Young cacao (Theobroma cacao L.). Int. J. Agron. 2021, 1–10. doi: 10.1155/2021/5516928
Dossa, E. L., Arthur, A, Dogbe, W, Mando, A, Snoeck, D, and Acquaye, S. (2018a). “An Assessment of Inherent Chemical Properties of Soils for Balanced Fertilizer Recommendations for Cocoa in Ghana.” in Improving the Profitability, Sustainability and Efficiency of Nutrients Through Site Specific Fertilizer Recommendations in West Africa Agro-Ecosystems. doi: 10.1007/978-3-319-58789-9_18
Dossa, E. L., Arthur, A, Dogbe, W, Mando, A, Snoeck, D., Afrifa, A.A., et al. (2018b). “Improving Fertilizer Recommendations for Cocoa in Ghana Based on Inherent Soil Fertility Characteristic.” in Improving the Profitability, Sustainability and Efficiency of Nutrients Through Site Specific Fertilizer Recommendations in West Africa Agro-Ecosystems, edited by Andre Bationo, Djimasbe Ngaradoum, Sansan Youl, Francois Lompo, and Joseph Opoku Fening, 1:287–99. Springer International Publishing. doi: 10.1007/978-3-319-58789-9
Eddy, W. C., and Yang, W. H. (2022). Improvements in soil health and soil carbon sequestration by an agroforestry for food production system. Agric. Ecosyst. Environ. 333, 107945. doi: 10.1016/j.agee.2022.107945
Esmaeilzadeh-Salestani, K., Bahram, M., Seraj, R. G. M., Gohar, D., Tohidfar, M., Eremeev, V., et al. (2021). Cropping systems with higher organic carbon promote soil microbial diversity. Agric. Ecosyst. Environ. 319, 107521. doi: 10.1016/j.agee.2021.107521
European Commission (2021). Consolidated Text: Commission Implementing Regulation (EU) 2021/1165 of 15 July 2021 Authorising Certain Products and Substances for Use in Organic Production and Establishing Their Lists. EU. Available online at: http://data.europa.eu/eli/reg_impl/2021/1165/2023-02-07 (accessed April 20, 2023).
FAO ITPS (2020). “Towards a Definition of Soil Health.” Soil Letters. 2020. Available online at: https://www.fao.org/3/cb1110en/cb1110en.pdf (accessed April 20, 2023).
FAOSTAT (2021). “Crops: Production Quantities of Cocoa Beans by Country Average from 1994–2020.” 2021. Available online at: http://www.fao.org/faostat/en/#data/QC (accessed April 20, 2023).
Folke, C., Carpenter, S. R., Walker, B., Scheffer, M., Chapin, T., and Rockström, J. (2010). Resilience thinking: integrating resilience, adaptability and transformability. Ecol. Soc. 15(4). doi: 10.5751/ES-03610-150420
Fountain, A., and Huetz-Adams, F. (2020). Cocoa barometer 2020. In Cocoa barometer 2020: Fountain, Antonie. [Bonn]: SÜDWIND eV-Institut für Ökonomie und Ökumene. Available online at: https://www.voicenetwork.eu/wp-content/uploads/2021/03/2020-Cocoa-Barometer-EN.pdf (accessed April 20, 2023).
Fox, J., and Monette, G. (1992). Generalized collinearity diagnostics. J. Am. Stat. Assoc. 87, 178-183. doi: 10.1080/01621459.1992.10475190
Gaspar, A. P., and Labosk, C. A. (2016). “Base Saturation: What Is It? Should I Be Concerned? Does It Affect My Fertility Program.” in Proceedings of the 2016 Wisconsin Crop Management Conference, 5:69–72. Available online at: https://extension.soils.wisc.edu/wcmc/base-saturation-what-is-it-should-i-be-concerned-does-it-affect-my-fertility-program/ (accessed April 20, 2023).
Hartemink, A. E. (2005). Nutrient stocks, nutrient cycling, and soil changes in cocoa ecosystems: a review. Adv. Agron. 86, 227–253. doi: 10.1016/S0065-2113(05)86005-5
Herzog, C., Honegger, A., Hegglin, D., Wittwer, R., de Ferron, A., Verbruggen, E., et al. (2019). Crop yield, weed cover and ecosystem multifunctionality are not affected by the duration of organic management. Agric. Ecosyst. Environ. 284, 106596. doi: 10.1016/j.agee.2019.106596
Ingham, E. R. (2019). “Soil Health- Soil Food Web.” Natural Resources Conservation Service Soils United States Department of Agriculture. Available online at: https://www.nrcs.usda.gov/wps/portal/nrcs/detailfull/soils/health/biology/?cid=nrcs142p2_053868 (accessed February 14, 2020).
Kongor, J. E., Boeckx, P., Vermeir, P., Van de Walle, D., Baert, G., Afoakwa, E. O., et al. (2019). Assessment of soil fertility and quality for improved cocoa production in six cocoa growing regions in Ghana. Agroforest. Sys. 93, 1455-1467. doi: 10.1007/s10457-018-0253-3
Kongor, J. E., De Steur, H., Van de Walle, D., Gellynck, X., Afoakwa, E. O., Boeckx, P., et al. (2018). Constraints for future cocoa production in Ghana. Agroforestry Systems, 92, 1373-1385. doi: 10.1007/s10457-017-0082-9
Krey, K. L., Nabity, P. D., Blubaugh, C. K., Fu, Z., Van Leuven, J. T., Reganold, J. P., et al. (2020). Organic farming sharpens plant defenses in the field. Front. Sustain. Food Sys. 4, 97. doi: 10.3389/fsufs.2020.00097
Läderach, P., Martinez-Valle, A., Schroth, G., and Castro, N. (2013). Predicting the future climatic suitability for cocoa farming of the world's leading producer countries, Ghana and Côte d'Ivoire. Clim. Change 119, 841–854. doi: 10.1007/s10584-013-0774-8
Lal, R., Singh, B. R., Mwaseba, D. L., Kraybill, D., Hansen, D. O., and Eik, L. O. (Eds.). (2015). Sustainable Intensification to Advance Food Security and Enhance Climate Resilience in Africa. Cham: Springer International Publishing. doi: 10.1007/978-3-319-09360-4
Layek, J., Anup, D, Meraj, A. A, Vinay, K. M, Krishnappa, R, and Natesan, R.. (2023). An integrated organic farming system: innovations for farm diversification, sustainability, and livelihood improvement of hill farmers. Front. Sustain. Food Sys. 7, 193. doi: 10.3389/fsufs.2023.1151113
Li, Z., Jiao, Y., Yin, J., Li, D., Wang, B., Zhang, K., et al. (2021). Productivity and quality of banana in response to chemical fertilizer reduction with bio-organic fertilizer: Insight into soil properties and microbial ecology. Agric. Ecosyst. Environ. 322, 107659. doi: 10.1016/j.agee.2021.107659
Lloyd, P. J. (2001). The Origins of the von Thünen-Mill-Pareto-Wicksell-Cobb-Douglas Function. History Pol. Econ. 3, 1. doi: 10.1215/00182702-33-1-1
McLean, E. O. (1965). “Aluminum,” in Methods of Soil Analysis: Part 2 Chemical and Microbiological Properties, edited by A. G. Norman, 9:978–98. Madison, Wisconsin USA: Wiley Online Library. doi: 10.2134/agronmonogr9.2.c16
Mensah, M., Villamor, G. B., Fosu-Mensah, B. Y., and Vlek, P. L. (2021). Exploring the gender-specific adaptive responses to climate variability: application of grazing game in the semi-arid Region of Ghana. Agriculture 11, 1048. doi: 10.3390/agriculture11111048
Moebius-Clune, B. N. (2016). Comprehensive Aassessment of Soil Health: The Cornell Framework Manual. Geneva: Cornell University.
Mol, A. P., and Oosterveer, P. (2015). Certification of markets, markets of certificates: tracing sustainability in global agro-food value chains. Sustainability 7, 12258–78. doi: 10.3390/su70912258
O'Brien, R. M. (2007). A caution regarding rules of thumb for variance inflation factors. Qual. Quant. 41, 673–690. doi: 10.1007/s11135-006-9018-6
Odum, E. P. (1968). Energy flow in ecosystems: a historical review. Am. Zool. 8, 11–18. doi: 10.1093/icb/8.1.11
Ofori-Frimpong, K., Afrifa, A. A., and Acquaye, S. (2010). Impact of shade and cocoa plant densities on soil organic carbon sequestration rates in a cocoa growing soil of Ghana. Af. J. Environ. Sci. Technol. 4, 621–624. doi: 10.4314/ajest.v4i9.71321
Quarles, W. (2018). Regenerative agriculture can reduce global warming. IPM Practitioner, 36, 1–8. Berkeley, CA: Bio-Integral Resources Center (BIRC).
Quaye, A. K., Doe, E. K., Amon-Armah, F., Arthur, A., Dogbatse, J. A., and Konlan, S. (2021). Predictors of integrated soil fertility management practice among cocoa farmers in Ghana. J. Agricult. Food Res. 5, 100174. doi: 10.1016/j.jafr.2021.100174
R Core Team (2019). “R: A Language and Environment for Statistical Computing.” Vienna, Austria: R Foundation for Statistical Computing. Available online at: https://www.r-project.org/ (accessed April 20, 2023).
Rajab, Y. A., Leuschner, C., Barus, H., Tjoa, A., and Hertel, D. (2016). Cacao cultivation under diverse shade tree cover allows high carbon storage and sequestration without yield losses. PLoS ONE 11, e0149949. doi: 10.1371/journal.pone.0149949
Ramírez-Argueta, O., Orozco-Aguilar, L., Dubón, A. D., Díaz, F. J., Sánchez, J., and Casanoves, F. (2022). Timber growth, cacao yields, and financial revenues in a long-term experiment of cacao agroforestry systems in northern Honduras. Front. Sustain. Food Sys. 6, 434. doi: 10.3389/fsufs.2022.941743
Richardson, A. E., Lynch, J. P., Ryan, P. R., Delhaize, E., Smith, F. A., Smith, S. E., et al. (2011). Plant and microbial strategies to improve the phosphorus efficiency of agriculture. Plant Soil 349, 121-156. doi: 10.1007/s11104-011-0950-4
Robinson, G. W. (1922). A new method for the mechanical analysis of soils and other dispersions. J. Agric. Sci. 12, 306–321. doi: 10.1017/S0021859600005360
Rousk, J., Bååth, E., Brookes, P. C., Lauber, C. L., Lozupone, C., Caporaso, J. G., et al. (2010). Soil bacterial and fungal communities across a pH gradient in an arable soil. ISME J. 4, 1340–1351. doi: 10.1038/ismej.2010.58
Schroth, G., Jeusset, A., Gomes, A. D. S., Florence, C. T., Coelho, N. A. P., Faria, D., et al. (2016). Climate friendliness of cocoa agroforests is compatible with productivity increase. Mitig. Adaptat. Strat. Glob. Change 21, 67–80. doi: 10.1007/s11027-014-9570-7
Scow, K. M. (1997). “Soil Microbial Communities and Carbon Flow in Agroecosystems,” in Ecology in Agriculture, 367–413. Elsevier. doi: 10.1016/B978-012378260-1/50012-9
Sdrolia, E., and Zarotiadis, G. (2019). A comprehensive review for green product term: From definition to evaluation. J. Econ. Surv. 33, 150–178. doi: 10.1111/joes.12268
Smith, O. M., Cohen, A. L., Rieser, C. J., Davis, A. G., Taylor, J. M., Adesanya, A. W., et al. (2019). Organic farming provides reliable environmental benefits but increases variability in crop yields: A global meta-analysis. Front. Sustain. Food Syst. 3, 82. doi: 10.3389/fsufs.2019.00082
Somarriba, E., Cerda, R., Orozco, L., Cifuentes, M., Dávila, H., Espin, T., et al. (2013). Carbon stocks and cocoa yields in agroforestry systems of Central America. Agric. Ecosyst. Environ. 173, 46–57. doi: 10.1016/j.agee.2013.04.013
Sumberg, J., and Giller, K. E. (2022). What is ‘conventional' agriculture? Global Food Sec. 32, 100617. doi: 10.1016/j.gfs.2022.100617
Tscharntke, T., Clough, Y., Bhagwat, S. A., Buchori, D., Faust, H., Hertel, D., et al. (2011). Multifunctional shade-tree management in tropical agroforestry landscapes–a review. J. Appl. Ecol. 48, 619–629. doi: 10.1111/j.1365-2664.2010.01939.x
Tscharntke, T., Clough, Y., Wanger, T. C., Jackson, L., Motzke, I., Perfecto, I., et al. (2012). Global food security, biodiversity conservation and the future of agricultural intensification. Biol. Conserv. 151, 53–59. doi: 10.1016/j.biocon.2012.01.068
Van Ittersum, M. K., Cassman, K. G., Grassini, P., Wolf, J., Tittonell, P., and Hochman, Z. (2013). Yield gap analysis with local to global relevance—A review. Field Crops Res. 143, 4–17. doi: 10.1016/j.fcr.2012.09.009
van Ittersum, M. K., and Rabbinge, R. (1997). Concepts in production ecology for analysis and quantification of agricultural input-output combinations. Field Crops Res. 52, 197–208. doi: 10.1016/S0378-4290(97)00037-3
van Vliet, J. A., and Maja Slingerland, K. E. Giller. (2015). Mineral Nutrition of Cocoa: A Review. Advances in Agronomy. Vol. 141. Wageningen: Wageningen University and Research Centre. doi: 10.1016/bs.agron.2016.10.017
Walkley, A., and Black, I. A. (1934). An examination of the Degtjareff method for determining soil organic matter, and a proposed modification of the chromic acid titration method. Soil Sci. 37, 29–38. doi: 10.1097/00010694-193401000-00003
Wang, Z., Ye, L., Jiang, J., Fan, Y., and Zhang, X. (2022). Review of application of EPIC crop growth model. Ecol. Modell. 467, 109952. doi: 10.1016/j.ecolmodel.2022.109952
Wilding, L. P. (1985). “Spatial Variability: Its Documentation, Accommodation and Implication to Soil Surveys,” in Soil Spatial Variability.
Williams, J. R., Jones, C. A., Kiniry, J. R., and Spanel, D. A. (1989). The EPIC crop growth model. Transact. ASAE 32, 497–511. doi: 10.13031/2013.31032
Zhao, H., Sun, B., Lu, F., Wang, X., Zhuang, T., Zhang, G., et al. (2017). Roles of nitrogen, phosphorus, and potassium fertilizers in carbon sequestration in a Chinese agricultural ecosystem. Clim. Change. 142, 587–596. doi: 10.1007/s10584-017-1976-2
Keywords: cocoa agroforestry, crop yield, soil fertility, soil organic carbon, ferralsols, lixisols, leptosols, plant density
Citation: Doe EK, Attua EM, Obour PB, Quaye AK and Fosu-Mensah BY (2023) Soil health and synergy of ecological determinants of green cocoa productivity in different soil ecotypes in Ghana. Front. Sustain. Food Syst. 7:1169015. doi: 10.3389/fsufs.2023.1169015
Received: 18 February 2023; Accepted: 24 April 2023;
Published: 19 May 2023.
Edited by:
Sanjay Singh Rathore, Indian Agricultural Research Institute (ICAR), IndiaReviewed by:
Ana I. F. Ribeiro-Barros, University of Lisbon, PortugalMusibau Azeez, Obafemi Awolowo University, Nigeria
Copyright © 2023 Doe, Attua, Obour, Quaye and Fosu-Mensah. This is an open-access article distributed under the terms of the Creative Commons Attribution License (CC BY). The use, distribution or reproduction in other forums is permitted, provided the original author(s) and the copyright owner(s) are credited and that the original publication in this journal is cited, in accordance with accepted academic practice. No use, distribution or reproduction is permitted which does not comply with these terms.
*Correspondence: Peter Bilson Obour, cGJvYm91ciYjeDAwMDQwO3VnLmVkdS5naA==; Eric Kofi Doe, ZXJpY2RvZTFzdCYjeDAwMDQwO2dtYWlsLmNvbQ==; ZWtkb2UmI3gwMDA0MDtzdC51Zy5lZHUuZ2g=