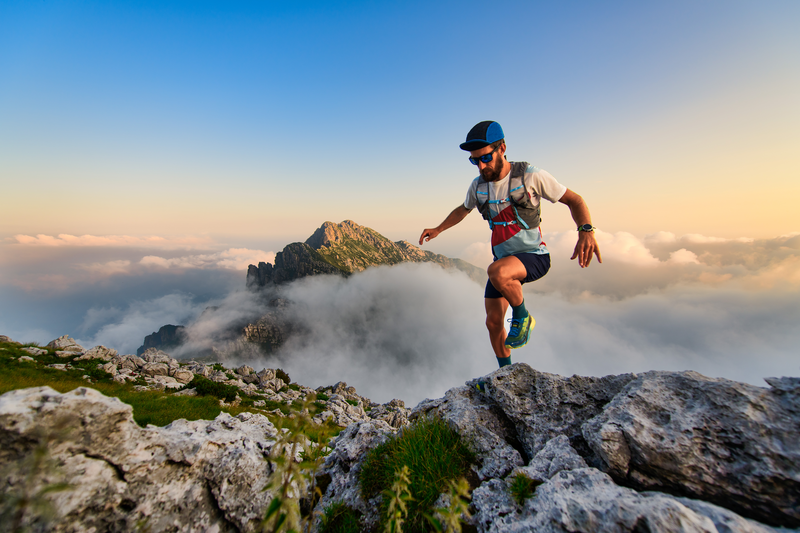
95% of researchers rate our articles as excellent or good
Learn more about the work of our research integrity team to safeguard the quality of each article we publish.
Find out more
ORIGINAL RESEARCH article
Front. Sustain. Food Syst. , 14 August 2023
Sec. Crop Biology and Sustainability
Volume 7 - 2023 | https://doi.org/10.3389/fsufs.2023.1163426
This article is part of the Research Topic Biostimulants for Climate-Smart and Sustainable Agriculture View all 12 articles
Most herbal plant farming operations still rely on conventional methods, negatively impacting human health and the environment. However, by using rhizobacteria to boost the yield and quality of herbal plants, farmers can make a more environmentally responsible and safe choice for consumers. Therefore, the present study aimed to determine the dosage of Brevibacillus agri added to the medicinal plant Piper caninum to boost its growth and phytochemical content. Piper caninum is a popularly used medicinal plant with antifungal and antibacterial properties and the ability to improve the quality of mouse sperm. The investigation was carried out in a greenhouse using a randomized group approach. The results indicated that the most effective formula for promoting growth and enhancing phytochemical composition was F1 (100 g of compost and 3 kg of soil plus 1% Brevibacillus agri), which contained 1% B. agri. Treating the Piper caninum plant with 1%, 2%, or 3% B. agri yielded positive results, likely due to the bacteria's nitrogen-fixing ability and favorable outcomes for the IAA test and protease enzyme. Brevibacillus agri was also found to colonize the roots of Piper caninum and produce the phytochemicals butanoic acid, propanediol, and cyclopropane. In conclusion, using rhizobacteria in sustainable agriculture was highly effective, providing an ecologically responsible and safe alternative to conventional farming methods.
Plants have been utilized as herbal medicines for centuries due to their high level of safety for consumption. In this modern era, there has been a significant global increase in using plants as herbs (Singh et al., 2021). These plants possess biologically active chemical components with medicinal properties in different parts, such as leaves, roots, rhizomes, stems, bark, flowers, fruits, and seeds. Various phytochemicals establish herbal plants' quality, including alkaloids, saponins, phenolics, flavonoids, tannins, and antioxidants (Nasab and Sayyed, 2021; Ali et al., 2022). Phytochemicals are essential daily as biopesticides, cosmetics, and medications to prevent and treat various diseases (Parbuntari et al., 2018). According to Firenzuoli and Gori (2007) research, there has been an increasing demand for herbal medicines. This trend underscores the need to cultivate plants sustainably, employ environmentally friendly methods, and refrain from using harmful chemicals (Egamberdieva and da Silva, 2015). Therefore, it is crucial to ensure that the plants used for herbal medicines are safe for consumption without harmful substances.
Due to practical reasons, chemical fertilizers and insecticides are still primarily used in producing herbal plants (Agbodjato et al., 2015). However, overusing pesticides and fertilizers has a negative impact on crop quality (Joko et al., 2020), as seen by the presence of chemical pesticide residues (Zou et al., 2023). Various businesses emerged in organic agriculture to obtain sustainable, environmentally friendly, and safe products. Furthermore, the study of plant growth promotion rhizobacteria (PGPR) present in plant roots has recently garnered considerable attention (Bhat et al., 2022; Desai et al., 2022; Tan et al., 2022). PGPR is safe to consume because there are no pesticide residues, and it is environmentally friendly and sustainable (Hamid et al., 2022). The application in plant cultivation has a good impact on plants (Chandran et al., 2021; Sharma et al., 2021). It creates a variety of chemicals for plants, including biofertilizers, biopesticides, growth hormones, and enzymes (Habib et al., 2016; Khan et al., 2021; Gowtham et al., 2022), which are beneficial to the development and productivity of plants (Mohanty et al., 2021).
Trichoderma biostimulants can increase the content of antioxidants, phenolics, and alkaloids in olive plants Egamberdieva and da Silva, 2015). Trichoderma album and Bacillus megaterium (Zope et al., 2019; Enshasy et al., 2020; Sagar et al., 2022a) can increase antioxidants in onion plants (Younes et al., 2023). Brevibacillus agri can improve the content of antioxidants, anthocyanins, vitamin C, vitamin A, fiber, tannins, and total phenols in Bali red rice (Unggulan et al., 2021). B. agri, found in the root of Bali rice plants, contains IAA hormones and protease enzymes. Furthermore, it fixes nitrogen from the atmosphere and boosts secondary metabolic chemicals and antioxidants (Suriani et al., 2022).
Piper caninum contains antioxidants, alkaloids, phenols, flavonoids, and steroids (Suriani et al., 2019, 2020b), and antimicrobial essential oils (Salleh et al., 2011, 2015). The quality of mice's sperm can be improved by P. caninum leaf extract (Gede et al., 2022). According to Suriani et al. (2020b), the plants growing at 600 meters in tropical forests are still in a wild state and have not been widely cultivated by the community. Additionally, several studies have been conducted on using the B. agri rhizobacteria formula to enhance growth, phytochemicals, and antioxidants in P. caninum leaf. This study aimed to get a better quantity and quality of P. caninum herbal plants using B. agri rhizobacteria.
The current study was conducted between January 2022 and October 2022 at the biopesticide lab at Udayana University in Bali, Indonesia, and the greenhouse in Munduk Paku Village in Senganan Penebel, Tabanan, Bali, Indonesia (8°22′49.3 “S 115°09′43.2”E). The climate of this region is categorized as Type A by Schmidt and Ferguson, and it experiences ~155.6 wet days per year, with an annual rainfall of between 2,000 and 2,800 mm. The region has 4 to 10 and 0 to 5 wet and dry months per year, respectively. Additionally, the average air temperature ranges from 25°C to 28°C (Suriani et al., 2022).
A randomized group design with four treatments and six replicates was used in the greenhouse, resulting in 24 experimental units, each comprising three clumps for 72 clusters. F0 is the control (untreated soil), F1 is treatment (100 g of compost and 3 kg of soil plus 1% B. agri), F2 is treatment (100 g of compost and 3 kg of soil plus 2% B. agri), and F3 is treatment (100 g of compost and 3 kg of soil plus 3% B. agri). Each polybag contains one ready-to-plant P. caninum plant. The P. caninum plants were obtained from the Bali villages of Munduk Paku and Senganan in the Penebel District and Tabanan Regency (Sudewi et al., 2020).
Compost is made from rice straw, chicken manure, and cow dung. Subsequently, a minor bit of water was added to bring the entire weight up to about 500 kg, with 1 liter of liquid biostater B. agri. The container was locked for 20 days before thoroughly stirring the liquid. It was then closed again for 40 days following the process described by Shilviana et al. (2021). The appropriate amounts of cow dung, chicken manure, and rice straw were combined and then slightly moistened with water to achieve a total weight of around 500 kg to create the fertilizer. Additionally, 1 liter of liquid biostater B. agri was added, and after locking the container for 20 days, the liquid was thoroughly agitated upon opening to release any available gas. The container was closed again for 40 days to complete the process; after that, the compost was ready to be used as a mixed media in research (Shilviana et al., 2021).
The isolates were first grown in a 5 ml test tube of tryptic soy broth, where they were kept in the dark at 28°C for 48 h. One cc isolate was first grown in a 5 mL test tube of tryptic soy broth and added with one cc of Salkowski's solution to the test tube; the hue changed. A pink tinge in the suspension indicates that the rhizobacteria can produce IAA. Spectrophotometry at 520 nm or higher was also used to quantify the data (Delgado-Ramírez et al., 2021).
Bacterial isolates were grown on a bromothymol blue malate medium that lacked nitrogen and contained 5 g of malic acid, 4 g of KOH, 0.5 g of K2HPO4, and 0.05 g of FeSO4. Additionally, 0.01 g of MnSO4 and 7H2O and 0.01 g of MgSO4 in 7H2O, 0.02% NaCl, 0.01% CaCl2, and 0.002% Na2 were added. The cultures were maintained at 28°C for 48 h, and rhizobacteria actively fixed nitrogen when the colonies turned yellow (Tang et al., 2019).
Bacterial isolates were cultivated on 2% SMA media to test for proteolytic activity (Skimmed Milk Agar). The media was prepared by mixing 2 g and 3 g of skimmed milk and Nutrient Agar, diluted with distilled water to a final volume of 100 mL. The mixture was sterilized using an autoclave at 121°C for 20 min. Meanwhile, the isolated bacteria were grown on 2% SMA media at 35–37°C for 24–48 h. A clear zone around the bacterial colony indicated protease enzyme activity (Kusuma et al., 2021).
Rhizobacteria with assessment number: OM510267 were found in Senganan village, Penebel sub-district, Tabanan Regency, Bali, Indonesia. It is the bacterium that produces the most potent IAA hormone compared to 20 others from Badung Regency and Tabanan Bali (Suriani et al., 2022). B. agri bacteria is grown in Nutrient Agar (NA) media containing Nystatin as much as 500 mg/L. To make 1 liter of biostimulant, 1 liter of Potato Dextrose Broth (PDB) media is prepared, media containing Nystatin as much as 500 mg/L, then five needles of Ose culture of B. agri are then incubated for three days between 28°C and 30°C.
The compound's analysis in the control and treatment cases was analyzed using GC-MS to determine the phytochemical compound. The isolate was incubated in potato dextrose broth medium for seven days, after which the culture was centrifuged at 10.000 rpm for 15 minutes to collect the supernatant. Subsequently, the supernatant from the biomass experiment was filtered through a 0.45 μm Millipore membrane (LTD, Yonezawa, Japan) for analysis (Maulina et al., 2022).
The supernatant was dissolved in a 1:1 v/v ratio of methanol and chloroform (5 mL total) to prepare the sample for GC-MS analysis. The sample was then subjected to GC-MS analysis using liquid nitrogen as the eluent. Meanwhile, the column had the following specifications: 4.6 x 200 mm, 1 mL/min flow rate, 250°C temperature, and UV detection at 254 nm. The compound was identified by comparing the isolated compound's molecular weight and fragmentation pattern with those in the GC-MS library. The bacteria were isolated by inoculating the compound in a Potato Dextrose Broth medium for seven days (Akubugwo et al., 2022).
About 0.5 g of specimens were weighed after being smoothed out and placed into a Kjeldahl flask. Next, 25 mL of sulfuric-salicylic acid solution was added, shaken, and allowed to sit overnight. The mixture was then heated at a low temperature until the bubbles disappeared after 4 g of Na2S2O2.5H2O was added. The temperature was gradually increased until a maximum of 300°C (~2 h) was reached and then allowed to cool. The solution was transferred into a 500 mL measuring flask, diluted with distilled water, shaken, and adjusted to the line mark. Distillation was terminated when the distillation yield reached 100 mL. Subsequently, 25 mL was pipetted and added to a distillation flask with 150 mL of distilled water, 10 mL of 40% NaOH solution, a 20 mL of 1% boric acid solution. The solution was titrated with an H2SO4 0.05 N solution until the endpoint of the titration was reached (the green color changed to pink). Meanwhile, work was also carried out on the blank solution. Furthermore, nitrogen levels were determined using a UV-Vis spectrophotometer at a wavelength of 400 nm (Liu et al., 2022).
A total of 0.5 g of soil is subjected to the ashing process with the addition of concentrated H2SO4 and HNO3; after that, it is heated over a hot plate. Then 2.5 ml of concentrated H2SO4 was added, so it turned black such as ash, then added concentrated HNO3 until the smoke from the sample is gone black. The addition of HNO3 was gradual until the sample did not emit black smoke after adding HNO3. After the ashing process, the sample was added to 50 ml of distilled water, shaken, filtered, and kept in the Erlenmeyer flask, followed by adding 2.5 ml of vanadate molybdate, which will produce a yellow color. Furthermore, phosphorus levels were determined using a UV-Vis spectrophotometer at a wavelength of 400 nm (Elbasiouny et al., 2020).
About 2.5 g of test-ready samples were weighed in a 250 mL flask, and 50 mL of 4% (NH4)2C2O4 and 125 mL of distilled water were used for the K analysis. The mixture was brought to a boil, boiled for 30 min, and then cooled. Once the mixture reached the mark on the flask, it was transferred to a 250 mL measuring flask and diluted with distilled water. The 15 mL solution was then filtered or left to stand until clear and was transferred into a 100 mL measuring flask to prepare the analysis solution. Furthermore, 2 mL of NaOH (20%), 5 mL of HCHO, and 1 mL of STPB for every 1% K2O were added. After filling the flask with distilled water to the mark and stirring for 5 to 10 minutes, the solution was strained using Whatman filter paper No. 12, and about 50 mL of the filtrate was taken for further analysis (Alhaj Hamoud et al., 2019).
Scanning electron microscopy (SEM) was utilized to investigate the effects of rhizobacteria treatment on bacterial colonization of plant roots. Control samples were created using the roots of P. caninum, while treatment samples were prepared by immersing the roots in a 2% solution of B. agri for 3 days. The samples were then dehydrated for 8 hours and ventilated for 1 week at 50 degrees until a constant weight was established. The root samples were examined using an FE-SEM MICROSCOPE SCAN ENERGY X-ray spectrometry, ZEISS Merlin, with a beam current of 0.2–30 kV to 400 nA and a lowest vacuum of few pA-300 nA. In this study, a 3kV acceleration was used for imaging, and 15 kV was used for EDX experiments, except on the skin where 10 keV was sufficient. The analysis was conducted in the Lab. UGM (Maulina et al., 2022).
A specific concentration is mixed with compost after the soil medium (20 cm below the surface) has been boiled, prepared, and combined with the media under treatment. The material was ready for usage.
The seedlings used are previously prepared and treated using biostimulants. The healthy seedlings are uniform at ± 20 cm in height, free from pests and diseases. Planting is perpendicular to a depth of ± 5 cm (Suriani et al., 2020b).
The application of B. agri, under the previously designed plan, was carried out at 2, 4, and 6 weeks after planting (MST).
Plant maintenance involves several important tasks, such as watering, weeding, fertilizing, and pruning. Embroidery, which consists of creating plant patterns, is typically conducted on plants growing uniformly without irregularities. To ensure consistent growth, these plants are prepared in advance. Watering is performed weekly in the morning to induce stress and promote plant resilience. Weeding is crucial to prevent the growth of unwanted plants competing for nutrients. This helps to maintain healthy plant growth and to avoid damage (Suriani et al., 2020b).
Harvesting is carried out after the P. caninum plant within four months. The harvested leaves were washed, dried in a clean indoor wind, and served 8 hours before the oven.
Parameters measured in the field include plant height, root length, leaf area, laboratorium analysis of chlorophyll content, N, P, K, Cu, Cd, Pb leaf, then analyzed phenolic content, flavonoids, and antioxidant activity.
To prepare P. caninum leaves for chemical analysis, they are first chopped into 2 mm thick pieces and dried for 8 hours in a clean, dry room. Once the leaves are dry, they are ventilated at a temperature of 50°C for 10 hours until a constant weight and moisture content of 4% is obtained. Next, the leaves are macerated using ethanol, and the resulting solution is evaporated using a rotary evaporator (Lee et al., 2022). Finally, the leaves are tested for their phenolic, flavonoid, and antioxidant content.
A 7% Na2CO3 reagent was prepared by dissolving 3.5 g of Na2CO3 in 50 mL aqua bidestillata. Total phenolic compounds were measured using the colorimetric approach with gallic acid (GAE) as the reference. Meanwhile, a standard solution of gallic acid was created by dissolving 10 mg of gallic acid in 10 mL of ethanol to make a solution with a concentration of 1,000 ppm. To obtain a concentration of 100 ppm, 2.5 mL of the stock solution was diluted with ethanol to a volume of 25 mL. Subsequently, 1, 2, 3, 4, and 5 mL of the solution were diluted with 10 mL of ethanol simultaneously to create concentrations of 10, 20, 30, and 50 ppm. For the gallic acid standard solution measurement, 0.4 mL of the Folin-Ciocalteau reagent was added to each concentration of 10, 20, 30, 40, and 50 ppm. The mixture was whipped for 4–8 min, and then 4.0 mL of the 7% Na2CO3 was added and stirred until smooth. Subsequently, up to 10 mL of aquabidestillata was added, and the mixture was allowed to stand for two hours at room temperature. A calibration curve was created by measuring the absorbance at a maximum wave of 744.8 nm and relating it to the gallic acid content (g/mL). To prepare the P. caninum extract solution, 10 mg of the extract was weighed and dissolved in 10 mL of ethanol. Up to 1 mL of the solution was pipetted into the mixture to measure total phenol levels.
Furthermore, 0.4 mL of the Folin-Ciocalteau reagent was added, and the mixture was agitated for 4–8 min before being mixed with 4.0 mL of the 7% Na2CO3 solution. Up to 10 mL of aquabidestillata was added, and the mixture was stirred and allowed to stand for two hours at room temperature. The maximum absorption was measured at a wave of 744.8 nm. This process was repeated three times, and the phenol levels were measured as mg of gallic acid equivalent per g of extract (Redondo-Gómez, 2022).
A colorimetric method determined the total flavonoid levels with the steps and quercetin (QE). Standard quercetin solutions were created by measuring and dissolving 10 mg of standard quercetin in 10 ml of ethanol every hour to achieve a concentration of 1000 ppm. A typical quartzine solution of 1,000 ppm was diluted in 10 mL of p.a. ethanol for 100 ppm before being pumped into a range of 10 ppm, 20 ppm, 30 ppm, 40 ppm, and 50 ppm concentrations. To each standard solution, three milliliters of quercetin, 0.2 milliliters of 10% AlCl3, 0.2 milliliters of potassium acetate, and up to ten milliliters of aquadestilata were added. After incubating for another 30 minutes at room temperature, the sample was subjected to UV-Vis spectrophotometry at a wavelength of 431 nm to measure its absorbance. To determine the total flavonoid content of a 100 mg cadaver extract solution in 10 mL of ethanol, 0.2 mL of 10% AlCl3, 0.2 mL of potassium acetate, and 10 mL of distilled water were added to the solution. The absorbance was then measured using UV-Vis spectrophotometry at 431 nm after allowing the mixture to sit in a dark room at room temperature for 30 min. Meanwhile, 3 replicates of the sample solution were prepared to obtain the flavonoid levels as quercetin equivalents (Perisoara et al., 2022).
A standard gallic acid curve was created with different concentrations (0–2 mg/L). The sample was treated by weighing 0.05 g and diluting with 99.9% ethanol to a volume of 5 mL in a measuring flask, then centrifuging for 15 min at 3000 rpm. After adding the standard and supernatants, pipetting was used to introduce 0.5 mL of DPPH 0.1 mm (in 99.9% ethanol solvent) to the test tube. It was incubated at 25°C for 30 min to give DPPH enough time to react with the hydrogen atoms of the sample's antioxidants. Furthermore, its absorbance at 517 nm was measured. The antioxidant capacity was estimated using the formula y from the linear regression equation: y = ax + b (Cappellari et al., 2020).
Sample analysis was carried out for the control and treated leaves of P. caninum. P. caninum leaf samples weighing 0.5 g were placed in a Kjeldahl flask with 5 ml of concentrated HNO3 and H2SO4. The sample was then wet-digested until a dark powdered solution was obtained, which appeared slightly yellow. The resulting solution was diluted with ion-free water using a 100 mL measuring flask and filtered until a filtrate was obtained. Furthermore, the filtrates were analyzed using AAS for metal grades and mineral standards (Aslanidis and Golia, 2022).
The data obtained were analyzed quantitatively using analysis of variance (ANOVA). This is continued with the Duncans Multiple Range Test (DMRT) tests at a level of 5% when the treatment causes differences in the observed variables (Suriani et al., 2020a; Hosseini et al., 2022).
Table 1 shows that B. agri has produced qualitatively positive IAA hormones and quantitatively acquired the concentrations from 7 tests, ranging from 602.96–681.77 ppm. The results are highly favorable, demonstrating its capacity to bind nitrogen from the air and break down proteins, as shown in Table 2. Balinese rice contains the IAA hormone, which enhances rapid development (Suriani et al., 2022). Rhizobacteria can produce IAA hormones to promote the growth of plant roots and shoots. For example, tomato plants' roots and shoots can grow faster when rhizobacteria like Bacillus Substilis and Azospirillum brasilense are present (Lobo et al., 2022). Plants benefit from Arthrobacter pascens ZZ21, located in the rhizosphere. These rhizobacteria can generate the phytohormone indole-3-acetic acid (IAA), promoting plant development and purging soil contaminated with fluoranthene. IAA synthesis increased by 4.5 times when tryptophan in the culture medium was supplied at 200 mg/L. (Li et al., 2018).
Meanwhile, rhizobacteria can also fix nitrogen, boost rice plants' willingness to take up N, and associate with the roots of rice plants (Sagar et al., 2020; Jabborova et al., 2022; Mir et al., 2022). In the Bali region, this bacteria can also raise the N content of maize crops and soil. Protease enzymes break down proteins into smaller components for plant nutrition, facilitating the synthesis of nutrients in bean plants (Flores-Duarte et al., 2022). At the early seedling stage, PGPR increased leaf gas exchange rates, including photosynthesis, stomatal conductance, and transpiration in potato plants (Liu et al., 2022).
The results of the GC-MS analysis of B. agri (Figure 1) obtained 3 types of compounds, where propanediol has the most significant area at 39.25%, as shown in Table 3. Propanediol is a cosmetic ingredient produced by the bacterium Propionibacterium freudenreichii (Dank et al., 2021). Meanwhile, butanoic acid is antimicrobial (Suriani et al., 2022), and marine bacteria Labrenzia sp. 011, producing cyclopropane, can be antifungals (Moghaddam et al., 2018).
Data on P. caninum growth also indicated improvement following B. agri treatment, where 1% (F1) and 2% (F2) produced the best growth (Figures 2, 3). Rhizobacteria are the reason for the treatment's increased growth, significantly different from the control at all concentrations. IAA is a hormone that B. agri generates to promote plant development (Tables 1, 2). According to research, B. agri can improve Balinese rice growth at a concentration of 2% (Suriani et al., 2022). It creates phytochemicals with antifungal and antibacterial properties (Table 3). By directly promoting plant development through processes like nitrogen (N) fixation, phytohormone synthesis, and phosphate solubilization, PGPR can be employed as biofertilizers and biopesticides (Shah et al., 2021). It can increase vegetable growth and yield by creating hormones like IAA and phytochemicals inhibiting bacterial and fungal infections (Kumar et al., 2021). A plant's metabolism can be affected immediately by bacteria that support plant growth and use their metabolism to solubilize phosphates, create hormones, and fix nitrogen.
Figure 2. Everage groeth of P. canium. Letters a, b, c, d indicate significant differences between the values at P > 0.05.
Furthermore, PGPR enhances root growth and raises plant enzymatic activity. Several studies have shown numerous benefits of the application in maize and sugarcane crops (Kusale et al., 2021a; Saboor et al., 2021; Sagar et al., 2022b). PGPR can also stimulate other bacteria as part of a synergistic effect to improve their influence on plants, increasing growth or development (dos Santos et al., 2020). It increases the growth of medicinal plants because it can produce biofertilizers, dissolve phosphate and potassium, and fix nitrogen (Kumar et al., 2022).
Table 4 analyzes flavonoids, polyphenols, antioxidants, and chlorophyll. P. caninum plants treated with B. agri have been demonstrated to contain higher flavonoids, polyphenols, antioxidants, and chlorophyll than controls. Furthermore, 1–2% of B. agri treatment yields the best effects, whereas 3% results in lower levels of chlorophyll. Every treatment is significantly different from the control because of the influence of B. agri. The plant P. caninum has higher phytochemicals, antioxidants, and chlorophyll concentrations. According to studies (Ghorbanpour et al., 2016), PGPR boosts antioxidant activity in chickpeas (Cicer arietinum L.) and the activity of several antioxidant enzymes. S. meliloti increases the contents of phenolic compounds, flavonoids, and antioxidant capacity in the plant, which can be attributed to the ability of pounds, flavonoids, and antioxidants (Zapata-Sifuentes et al., 2022). Hyssopus officinalis, a member of the Lamiaceae family and one of the most significant medicinal plants producing essential oils can raise the chlorophyll content a, b, and total chlorophyll in plants by promoting rhizobacteria, Azospirillum, Pseudomonas, and Bacillus (Sharifi, 2017). Furthermore, the combination of B. subtilis and B. amyloliquefaciens had the most substantial significant impact, where the content of chlorophyll A increased by 30% and 27%, chlorophyll B by 20% and 16%, and total chlorophyll by 54% and 43%. Ascorbic acid also increased in tomato plants (Plants et al., 2022). Thiobacillus thiooxidans, Frateuria aurantia, and Bacillus megaterium can boost phenolic and antioxidant content (Eren, 2022). After being treated with PGPR Glomus aggregatum, Trichoderma harzianum, and Bacillus coagulans, Glycyrrhiza glabra L. (licorice) plants produced more phenols, ortho-dihydroxy phenols, tannins, flavonoids, and alkaloids (Egamberdieva and da Silva, 2015). Heavy metal data for Cu, Pb, and Cd were not detected, and there was no significant difference between the control and treatment. Therefore, the P. caninum plant is safe to consume and devoid of heavy metal contamination. There is no rich metal content of Cu, Pb, or Cd in the leaves of P. caninum due to the effects of B. agri. By changing the bioavailability of metal in the soil and enhancing metal translocation, PGPR may reduce phytotoxicity, supported by the analysis of soils showing no detected Cu (Table 5). PGPR can oxidize hydrocarbons and improve plant biodegradation activity (Vocciante et al., 2022). B. cereus inoculation increased the antioxidant enzyme activities in walnut seedlings and changed their photosynthetic characteristics (Ji and Huang, 2007).
ARAC 3221, ARAC 221, ARSI 2112, ARAI 3312, and ARAI 3221 were among the actinobacteria isolates that were successful in IAA is created by dissolving phosphate and producing chitinase enzymes. Only ARAI 3312 could not absorb nitrogen among the four isolates (Yanti et al., 2023). Most plants use indole-3-acetic acid (IAA) as their primary auxin. At the early stages of leaf development, in young leaves, and during seed germination, IAA is synthesized from tryptophan or indole. IAA also lowers intercellular concentrations and speeds up transpiration, stomatal conductance, and photosynthesis (Zou et al., 2023). Both nitrogen and phosphate, which are components of the nucleotides involved in the synthesis of amino acids and proteins, are macro elements that plants require for metabolism. Moreover, the primary component of plant chlorophyll is nitrogen (Etienne et al., 2018).
Tables 5, 6 show notable differences in the NPK content of the soil and P. caninum leaves compared to the control group and the 1% treatment exhibited. There was a significant difference from the control in the case of P. caninum leaf NPK content. The 1% treatment yielded the highest N and K content, while the highest P was found in the 2%. B. agri plays a crucial role in increasing the NPK content in the soil, as it can fix nitrogen and dissolve phosphate and potassium. Therefore, the content in the leaf is also high because plants can easily absorb it after the nutrients are available. Rhizobacteria play a crucial role in providing nutrients, and PGPR can boost wheat plants' uptake of NPK minerals (Hafez et al., 2019). In the nitrogen fixation process, soil-dwelling microbes bind atmospheric nitrogen, making it available to plants as ammonia. Both non-symbiotic (free-living diazotrophs, Azospirillum) and symbiotic (Azotobacter spp., Bacillus spp., etc.) methods are possible with PGPR (Bhat et al., 2023). In the potato plant, PGPR and compost treatment enhanced P and k by 82.1% and 51.1% (Ekin, 2019). The concentration of N, P, and K in soil and maize crops can be increased by PGPR isolated from Bali (Maulina et al., 2022). P's solubilization and mineralization depend on the soil bacteria's actions. On the other side, phosphatase hydrolysis of phosphoric esters results in the mineralization of organic phosphorus (Vocciante et al., 2022). Experiments on cucumber and pepper plants show that Paenibacillus can enhance K solubility in the soil. Meanwhile, bacillus can also raise the willingness of K in the soil.
The most favorable situation for plants is when rhizobacteria colonize their roots to gain the most benefits. According to a recent study, B. agri successfully colonized the roots of P. caninum after F1, F2, and F3 treatments but not the control plant, as shown in Figure 4. These three species can colonize the roots of maize plants, allowing for close interactions with the bacteria (Maulina et al., 2022). Free-living bacteria surrounding plant roots can exchange amino acids, proteins, enzymes, vitamins, and growth hormones in root exudates for nitrogen (Santoyo et al., 2021). Furthermore, PGPR alters the root system architecture by generating phytohormones and other signals that promote more lateral root branching and hair formation. It alters the plant's physiology, improves nutrition, and modifies the function of the root (Vacheron et al., 2013). To enhance their benefits to plants, PGPR colonizes roots, producing chemicals, fixing nitrogen, and dissolving phosphates (Ahemad and Kibret, 2014; Sayyed et al., 2015). Colonization of rooted rhizobacteria is closely related to the ability of rhizobacteria to produce hormones, enzymes (Sagar et al., 2022b), antibiotics (Vinay et al., 2016; Zakaria et al., 2016; Reshma et al., 2018), and biological fertilizers (Vejan et al., 2016; Kusale et al., 2021b). The rhizosphere should be created appropriately for plant growth, the bioavailability of N, P, K, and antagonistic characteristics should be increased, and PGPRs should be able to colonize host plant roots sufficiently. For PGPRs to be used as effective and successful bioinoculants, they must have specific properties. It must survive in soil, be compatible with the crop being inoculated, and interact with both abiotic and biotic soil microorganisms. The bioinoculants should be stabilized in soil systems, and any non-target effects should be prevented by taking the necessary precautions. These actions will ensure the longevity of the plant growth effect and the successful application of PGPRs as bioinoculants (Basu et al., 2021).
Treating 1%, 2%, and 3% B. agri on the P. caninum plant effectively improves growth and phytochemicals compound, with F1 (1% B. agri) as the best formula. B. agri is positive for the IAA test, protease enzyme, and can fix nitrogen. Furthermore, it can colonize the plant's roots and produce phytochemicals compounds butanoic acid, propanediol, and cyclopropane.
The original contributions presented in the study are included in the article/supplementary material, further inquiries can be directed to the corresponding author.
Conceptualization, methodology, and writing original draft: NS and NR. Data analysis: DS, KP, and JA. Writing and review: IS. Editing: EH, Rusdianasari, KP, and JA. Fund acquisition: KP.
This research was funded by LPPM Udayana University No. B/78.197/UN14.4.A/PT.01.03/2022 through Udayana University Innovation awards and the Researchers Supporting Project Number RSP2023R358, King Saud University, Riyadh, Saudi Arabia.
The authors would like to acknowledge the support provided by Researchers Supporting Project Number RSP2023R358, King Saud University, Riyadh, Saudi Arabia. The authors are grateful to LPPM Udayana University for funding through the Udayana University Innovation Awards 2022, the Laboratory of Biopesticide at Udayana University in Bali, Indonesia, and the Department of Biology Study Program at Udayana University in Bali, Indonesia.
The authors declare that the research was conducted in the absence of any commercial or financial relationships that could be construed as a potential conflict of interest.
All claims expressed in this article are solely those of the authors and do not necessarily represent those of their affiliated organizations, or those of the publisher, the editors and the reviewers. Any product that may be evaluated in this article, or claim that may be made by its manufacturer, is not guaranteed or endorsed by the publisher.
Agbodjato, N. A., Noumavo, P. A., Baba-Moussa, F., Salami, H. A., Sina, H., Sèzan, A., et al. (2015). Characterization of potential plant growth promoting rhizobacteria isolated from Maize (Zea mays L.) in central and Northern Benin (West Africa). Appl. Environ. Soil Sci. 2015, 1687–7667. doi: 10.1155./2015/901656
Ahemad, M., and Kibret, M. (2014). Mechanisms and applications of plant growth promoting rhizobacteria: Current perspective. J. King Saud Univ. Sci. 26, 1–20. doi: 10.1016/j.jksus.2013.05.001
Akubugwo, E. I., Emmanuel, O., Ekweogu, C. N., Ugbogu, O. C., Onuorah, T. R., Egeduzu, O. G., et al. (2022). GC-MS analysis of the phytochemical constituents, safety assessment, wound healing and anti-inflammatory activities of cucurbita pepo leaf extract in rats. Sci. Pharm. 90, 64. doi: 10.3390./scipharm90040064
Alhaj Hamoud, Y., Shaghaleh, H., Sheteiwy, M., Guo, X., Elshaikh, N. A., Ullah Khan, N., et al. (2019). Impact of alternative wetting and soil drying and soil clay content on the morphological and physiological traits of rice roots and their relationships to yield and nutrient use-efficiency. Agri. Water Manag. 223, 105706. doi: 10.1016/j.agwat.2019.105706
Ali, H., Hasi, R. Y., Islam, M., Haque, M. S., Alkhanani, M. F., Almalki, A. H., et al. (2022). Antioxidant, cytotoxic and apoptotic activities of the rhizome of Zingiber zerumbet Linn. in Ehrlich ascites carcinoma bearing Swiss albino mice. Sci. Rep. 12, 12150. doi: 10.1038/s41598-022-15498-8
Aslanidis, P. S. C., and Golia, E. E. (2022). Urban sustainability at risk due to soil pollution by heavy metals—Case study: Volos, Greece. Land, 11, 16. doi: 10.3390./land11071016
Basu, A., Prasad, P., Das, S. N., Kalam, S., Sayyed, R. Z., Reddy, M. S. El., et al. (2021). Plant growth promoting rhizobacteria (PGPR) as green bioinoculants:r developments, constraints, and prospects. Sustainability 13, 1–20. doi: 10.3390/su13031140
Bhat, B. A., Tariq, L., Nissar, S., Islam, S. T., Islam, S. U., Mangral, Z., et al. (2022). and Dar TH*. Plant-associated rhizobacteria in plant growth and metabolism as a tool for sustainable agriculture. J. Appl. Microbiol. 00, 1–25. doi: 10.1111/jam.157962022
Bhat, M. A., Mishra, A. K., Jan, S., Bhat, M. A., Kamal, M. A., Rahman, S., et al. (2023). Plant growth promoting rhizobacteria in plant health?: A perspective study of the underground interaction. Plants. 12, 629. doi: 10.3390/plants12030629
Cappellari, L. D., Chiappero, J., Palermo, T. B., Giordano, W., and Banchio, E. (2020). Volatile organic compounds from rhizobacteria increase the biosynthesis of secondary metabolites and improve the antioxidant status in mentha piperita l. grown under salt stress. Agronomy. 10, 1094. doi: 10.3390/agronomy10081094
Chandran, H., Meena, M., and Swapnil, P. (2021). Plant growth-promoting rhizobacteria as a green alternative for sustainable agriculture. Sustainability. 13, 10986. doi: 10.3390/su131910986
Dank, A., Zeng, Z., Boeren, S., Notebaart, R. A., Smid, E. J., Abee, T., et al. (2021). Bacterial microcompartment-dependent 1, 2.-propanediol utilization of propionibacterium freudenreichii. Front. Microbiol. 12, 1–10. doi: 10.3389/fmicb.2021.679827
Delgado-Ramírez, C. S., Hernández-Martínez, R., and Sepúlveda, E. (2021). Rhizobacteria associated with a native solanaceae promote plant growth and decrease the effects of fusarium oxysporum in tomato. Agronomy. 11, 579. doi: 10.3390/agronomy11030579
Desai, A., Ruparelia, J., Jha, C. K., Sayyed, R. Z., Mitra, D., Priyadarshini, A., et al. (2022). Articulating Beneficial rhizobacteria mediated plant defenses through induced systemic resistances. Pedosphere 4, 3. doi: 10.1016/j.pedsph.10003
dos Santos, R. M., Diaz, P. A. E., Lobo, L. L. B., and Rigobelo, E. C. (2020). Use of plant growth-promoting rhizobacteria in maize and sugarcane: characteristics and applications. Front. Sustain. Food Sys., 4(September), 1–15. doi: 10.3389./fsufs.2020.00136
Egamberdieva, D., and da Silva, T. J. A. (2015). Medicinal plants and PGPR. New Front. Phytoche. 3, 287–303. doi: 10.1007./978-3-319-13401-7_14
Ekin, Z. (2019). Integrated use of humic acid and plant growth promoting rhizobacteria to ensure higher potato productivity in sustainable agriculture. Sustainability. 11, 3417. doi: 10.3390/su11123417
Elbasiouny, H., Elbehiry, F., and Brevik, E. C. (2020). Phosphorus availability and potential environmental risk assessment in alkaline soils. Agriculture. 10, 172. doi: 10.3390/agriculture10050172
Enshasy, H. E. A., Ambehati, K. K., Ashraf, F., Ramchuran, S., Sayyed, R. Z., Amalin, D., et al. (2020). “Trichoderma : Biocontrol agents for promoting plant growth and soil health,” in Agriculturally Important Fungi for Sustainable Agriculture: Vol 2: Functional Annotation for Crop Protection. p. 239–259.
Eren, E. (2022). The effect of plant growth promoting rhizobacteria (PGPRs) on yield and some quality parameters during shelf life in white button mushroom (Agaricus bisporus L.). J. Fungi 8, 1016. doi: 10.3390/jof8101016
Etienne, P., Diquelou, S., Prudent, M., Salon, C., Maillard, A., Ourry, A., et al. (2018). Macro and micronutrient storage in plants and their remobilization when facing scarcity: the case of drought. Agriculture, 8, 14. doi: 10.3390./agriculture8010014
Firenzuoli, F., and Gori, L. (2007). Herbal medicine today: clinical and research issues. Evid. Based Complem. Alt. Med. 4(SUPPL 1), 37–40. doi: 10.1093./ecam/nem096
Flores-Duarte, N. J., Mateos-Naranjo, E., Redondo-Gómez, S., Pajuelo, E., Rodriguez-Llorente, I. D., Navarro-Torre, S., et al. (2022). Role of nodulation-enhancing rhizobacteria in the promotion of medicago sativa development in nutrient-poor soils. Plants 11, 1164. doi: 10.3390./plants11091164
Gede, D., Artha, N., Made, N., Suarni, R., Suriani, N. L., Gusti, N., et al. (2022). The Quality of Male Mice (Mus musculus L.) Spermatozoes Which are given Ethanol Leaves Extract of Piper caninum. East. J. Agric. Biol. Sci. 23–30.
Ghorbanpour, M., Hatami, M., Kariman, K., and Abbaszadeh Dahaji, P. (2016). Phytochemical variations and enhanced efficiency of antioxidant and antimicrobial ingredients in salvia officinalis as inoculated with different rhizobacteria. Chem. Biodiv. 13, 319–330. doi: 10.1002/cbdv.201500082
Gowtham, H. G., Singh, S. B., Shilpa, N., Aiyaz, M., Nataraj, K., Udayashankar, A. C., et al. (2022). Insight into recent progress and perspectives in improvement of antioxidant machinery upon pgpr augmentation in plants under drought stress: a review. Antioxidants 11, 9. 1763. doi: 10.3390/antiox11091763
Habib, S. H., Kausar, H., and Saud, H. M. (2016). Plant growth-promoting rhizobacteria enhance salinity stress tolerance in okra through ROS-scavenging enzymes. BioMed Res. Int. 2016, 4547. doi: 10.1155./2016/6284547
Hafez, M., Popov, A. I., and Rashad, M. (2019). Influence of agro-industrial wastes and azospirillum on nutrients status and grain yield under corn plant growth in arid regions. Biosci. Res. 16, 2119–2130.
Hamid, B., Bashir, Z., Yatoo, A. M., Mohiddin, F., Majeed, N., Bansal, M., et al. (2022). Cold-Active enzymes and their potential industrial applications—A review. Molecules 27, 5885. doi: 10.3390/molecules27185885
Hosseini, A., Hosseini, M., and Schausberger, P. (2022). Plant growth-promoting rhizobacteria enhance defense of strawberry plants against spider mites. Front. Plant Sci. 12(January), 1–12. doi: 10.3389/fpls.2021
Jabborova, D., Annapurna, K., Azimov, A., Tyagi, S., Pengani, K. R., Sharma, S., et al. (2022Co-inoculation of biochar and arbuscular mycorrhizae for growth promotion and nutrient fortification in soybean under drought conditions. Front. Plant Sci. 13, 947547. doi: 10.3389/fpls.2022.947547.
Ji, Y. X., and Huang, X. D. (2007). Effects of plant growth-promoting rhizobacteria on the growth of oat seedlings under salt stress. Dalian Haishi Daxue Xuebao J. Dalian Mari. Univ. 33, 86–89.
Joko, T., Dewanti, N. A. Y., and Dangiran, H. L. (2020). Pesticide poisoning and the use of personal protective equipment (PPE) in Indonesian farmers. J. Environ. Public Health 2020, 9619. doi: 10.1155./2020/5379619
Khan, N., Ali, A., Shahi, M. A., Mustafa, A., Sayyed, R. Z., and Curaá, J. A. (2021). Insights into the interactions among roots, rhizosphere and rhizobacteria for improving plant growth and tolerance to abiotic stresses: a review. Cells 10, 1551. doi: 10.3390/cells10061551
Kumar, M., Giri, V. P., Pandey, S., Gupta, A., Patel, M. K., Bajpai, A. B., et al. (2021). Plant-growth-promoting rhizobacteria emerging as an effective bioinoculant to improve the growth, production and stress tolerance of vegetable crops. Int. J. Mol. Sci. 22, 12245. doi: 10.3390/ijms222212245
Kumar, R., Swapnil, P., Meena, M., Selpair, S., and Yadav, B. G. (2022). Plant growth-promoting rhizobacteria (PGPR): approaches to alleviate abiotic stresses for enhancement of growth and development of medicinal plants. Sustainability 14, 5514. doi: 10.3390./su142315514
Kusale, S. P., Attar, Y. C., Sayyed, R. Z., Enshasy, H. E., Hanapi, Z., et al. (2021a). Inoculation of Klebsiella variicola Alleviated slat stress salinity and improved growth and nutrients in wheat and maize. Agronomy. 11, 927. doi: 10.3390/agronomy11050927
Kusale, S. P., Attar, Y. C., Sayyed, R. Z., Malek, R. A., Ilyas, N., Suriani, N. L., et al. (2021b). and Enshasy HE. Production of plant beneficial and antioxidants metabolites by Klebsiella variicola under salinity Stress. Molecules 26, 1894. doi: 10.3390/molecules26071894
Kusuma, P., Swan, B., and Bugbee, B. (2021). Does green really mean go? Increasing the fraction of green photons promotes growth of tomato but not lettuce or cucumber. Plants. 10, 637. doi: 10.3390/plants10040637
Lee, C. S., Gibbons, L. E., Lee, A. Y., Yanagihara, R. T., Blazes, M. S., Lee, M. L., et al. (2022). Association between cataract extraction and development of dementia. JAMA Intern Med. 182, 134–141. doi: 10.1001/jamainternmed.2021
Li, M., Guo, R., Yu, F., Chen, X., Zhao, H., Li, H., et al. (2018). Indole-3-acetic acid biosynthesis pathways in the plant-beneficial bacterium Arthrobacter pascens zz21. Int. J. Mol. Sci. 19, 443. doi: 10.3390./ijms19020443
Liu, J., Zhang, J., Zhu, M., Wan, H., Chen, Z., Yang, N., et al. (2022). Effects of plant growth promoting rhizobacteria (PGPR) strain bacillus licheniformis with biochar amendment on potato growth and water use efficiency under reduced irrigation regime. Agronomy 12, 31. doi: 10.3390./agronomy12051031
Lobo, L. L. B., and Andrade, d. e., da Silva, M. S. R., Castellane, T. C. L., Carvalho, R. F., and Rigobelo, E. C. (2022). Effect of Indole-3-Acetic Acid on Tomato Plant Growth. Microorganisms 10, 2212. doi: 10.3390/microorganisms10112212
Maulina, N. M., Suprapta, D. N., Temaja, I. G., Adnyana, I. M., and Suriani, N. L. (2022). Rhizobacteria of bali with obvious growth-promoting properties on corn (Zea mays L.). Front. Sustain Food Syst. 6, 899736. doi: 10.3389/fsufs.2022.899736
Mir, M. I., Bee, H., Quadriya, H., Kumar, B. K., Ilyas, I., Kasem, H. S., et al. (2022). and Sayyed RZ. Multifarious indigenous diazotrophic rhizobacteria of rice (Oryza sativa L.) rhizosphere and their effect on plant growth promotion. Front. Nutri. 8, 781764. doi: 10.10.3389/fnut.2021.781764
Moghaddam, J. A., Dávila-Céspedes, A., Kehraus, S., Crüsemann, M., Köse, M., Müller, C. E., et al. (2018). Cyclopropane-containing fatty acids from the marine bacterium Labrenzia sp. 011 with antimicrobial and GPR84 activity. Marine Drugs 16, 369. doi: 10.3390./md16100369
Mohanty, P., Singh, P. K., Chakraborty, D., Mishra, S., and Pattnaik, R. (2021). insight into the role of pgpr in sustainable agriculture and environment. Front. Sustain Food Syst. 5, 667150. doi: 10.3389/fsufs.2021.667150
Nasab, B. F., and Sayyed, R. Z. (2021). In silico molecular docking analysis of and amp;amp;alpha;-pinene: an antioxidant and anticancer drug obtained from Myrtus communis. Int. J. Cancer Manag. 14, e89116. Available online at: https://brieflands.com/articles/ijcm-89116.html
Parbuntari, H., Prestica, Y., Gunawan, R., Nurman, M. N., and Adella, F. (2018). Preliminary phytochemical screening (qualitative analysis) of cacao leaves (Theobroma cacao L.). EKSAKTA: Berkala Ilmiah Bidang MIPA 19, 40–45. doi: 10.24036/eksakta/vol19-iss2/142
Perisoara, A., Marinas, I. C., Geana, E. I., Constantin, M., Angheloiu, M., Pirvu, L., et al. (2022). Phytostimulation and synergistic antipathogenic effect of tagetes erecta extract in presence of rhizobacteria. Horticulturae 8, 779. doi: 10.3390./horticulturae8090779
Plants, S. L., Alshallash, K. S., El-Taher, A. M., Azab, E. S., El-Raouf, H. S. A., Ibrahim, M. F. M., et al. (2022). Cyanobacteria on Botanical Characteristics of Tomato. 1–16.
Redondo-Gómez, S., Romano-Rodríguez, E., Mesa-Marín, J., Sola-Elías, C., and Mateos-Naranjo, E. (2022). Consortia of plant-growth-promoting rhizobacteria isolated from halophytes improve the response of swiss chard to soil salinization. Agronomy. 12, 468. doi: 10.3390/agronomy12020468
Reshma, P., Naik, M. K., Aiyaz, M., Niranjana, S. R., Chennappa, G., Shaikh, S. S., et al. (2018). Induced systemic resistance by 2,4diacetylphloroglucinol positive fluorescent Pseudomonas strains against rice sheath blight. Indian J. Exp. Biol. 56, 207–12. Available online at: http://nopr.niscpr.res.in/handle/123456789/43660
Saboor, A., Muhammad, A. A., Hussain, S., El Enshasy, H. E., Hussain, S., Ahmed, N., et al. (2021). S, Datta R. Zinc nutrition and arbuscular mycorrhizal symbiosis effects on maize (Zea mays L.) growth and productivity. Saudi J. Biol. Sci. 28, 6339–6351. doi: 10.1016/j.sjbs.06096
Sagar, A., Sayyed, R. Z., Ramteke, P. W., Ramakrishna, W., Poczai, P., Obaid, S. A., et al. (2022b). Synergistic effect of Azotobacter nigricans and NPK fertilizer on agronomic and yield traits of Maize (Zea mays L.). Front. Plant Sci. 13, 952212. doi: 10.3389/fpls.2022.952212
Sagar, A., Sayyed, R. Z., Ramteke, P. W., Sharma, S., Marraiki, N., Elgorban, A. M., et al. (2020). deaminase and antioxidant enzymes producing halophilic Enterobacter sp. PR14 promotes the growth of rice and millets under salinity stress. Physiol. Mol. Biol. Plants 26, 1847–54. doi: 10.1007/s12298-020-00852-9
Sagar, A., Yadav, S. S., Sayyed, R. Z., Sharma, S., and Ramteke, P. W. (2022a). Bacillus subtilis: A Multifarious Plant Growth Promoter, Biocontrol Agent, and Bioalleviator of Abiotic Stress, Bacilli in Agrobiotechnology, Islam, M.T., Rahman, M., Pandey, P,. (eds) Bacilli in Agrobiotechnology. Bacilli in Climate Resilient Agriculture and Bioprospecting. Springer, pp 561-580
Salleh, W. M. N. H. W., Ahmad, F., and Yen, K. H. (2015). Chemical constituents from Piper caninum and antibacterial activity. J. Appl. Pharm. Sci. 5, 020–025. doi: 10.7324/JAPS.2015.50604
Salleh, W. M. N. H. W., Ahmad, F., Yen, K. H., and Sirat, H. M. (2011). Chemical compositions, antioxidant and antimicrobial activities of piper caninum blume essential oils. Int. J. Mol. Sci. 12, 7720–7731. doi: 10.3390/ijms12117720
Santoyo, G., Alberto, C., Damián, P., and Glick, B. R. (2021). Rhizosphere Colonization Determinants by Plant Growth-Promoting Rhizobacteria (PGPR). Biology. 10, 475. doi: 10.3390/biology10060475
Sayyed, R. Z., Patel, P. R., and Shaikh, S. S. (2015). Plant growth promotion and root colonization by EPS producing enterobacter sp. RZS5 under Heavy metal contaminated soil. Indian J. Exp. Biol. 53, 116–123. Available online at: http://nopr.niscpr.res.in/handle/123456789/30443
Shah, A., Nazari, M., Antar, M., Msimbira, L. A., Naamala, J., Lyu, D., et al. (2021). PGPR in agriculture: A sustainable approach to increasing climate change resilience. Front. Sustain. Food Syst. 5, 1–22. doi: 10.3389/fsufs.2021.667546
Sharifi, P. (2017). The effect of plant growth promoting rhizobacteria (PGPR), salicylic acid and drought stress on growth indices, the chlorophyll and essential oil of hyssop (Hyssopus officinalis). Biosci. Biotechnol. Res. Asia 14, 1033–1042. doi: 10.13005/bbra/2538
Sharma, A., Gupta, A., Dalela, M., Sharma, S., Sayyed, R. Z., Enshasy, H. E., et al. (2021). Linking organic metabolites as produced by Purpureocillium lilacinum 6029 cultured on Karanja deoiled cake medium for the sustainable management of root-knot nematodes. Sustainability. 12, 8276. Available online at: https://www.mdpi.com/2071-1050/12/19/827
Shilviana, S. D., Suriani, N. L., and Sundra, I. K. (2021). Utilization of organic fertilizer compost made from purple sweet potato waste (Ipomoea Batatas L.) to increase the production of pakchoy (Brassica Chinensis L.). AJARCDE. Asian J. Appl. Res. Comm. Develop. Empower. 5, 3–8. doi: 10.29165/ajarcde.v5i3.70
Singh, S., Singh, V., Mishra, B. N., Sayyed, R. Z., and Haque, S. (2021). Lilium philadelphicum flower as a novel source of antimicrobial agents: a study of bioactivity, phytochemical analysis and partial identification of antimicrobial metabolites. Sustainability 13, 8471. doi: 10.3390/su13158471
Sudewi, S., Palu, U. A., Baharuddin, B., Hasanuddin, U., and Saleh, A. R. (2020). Scereening Of Plant Growth Promotion Rhizobacteria (PGPR) to increase local aromatic rice plant growth. Int. J. Pharmaceut. Res. 13.
Suriani, N. L., Darmadi, A. A. K., Parwanayoni, N. M. S., Hamid, M. H. N. A., and Yamin, B. M. (2019). The combination of piper Caninum Blume leaf extract and compost fertilizer for pressing blast disease and improving the growth of Bali red rice (Oryza Sativa Linn). Int. J. Adv. Sci. Engin. Inform. Technol. 9, 518–525. doi: 10.18517/ijaseit.9.2.7449
Suriani, N. L., Suprapta, D., Novizar, N., Parwanayoni, N., Darmadi, A., Dewi, D., et al. (2020a). A mixture of piper leaves extracts and rhizobacteria for sustainable plant growth promotion and biocontrol of blast pathogen of organic bali rice. Sustainability 12, 8490. Available online at: https://www.mdpi.com/2071-1050/12/20/8490
Suriani, N. L., Suprapta, D. N., Nazir, N., Darmadi, A. A. K., Parwanayoni, N. M. S., Sudatri, N. W., et al. (2020b). Inhibitory activity of piper caninum leaf extract against curvularia spotting disease on rice plants. Indian J. Agri. Res. 54, 411–419. doi: 10.18805/IJARe.A-560
Suriani, N. L., Suprapta, D. N., Suarsana, I. N., Reddy, M. S., Gunawan, S., Herlambang, S., et al. (2022). Piper caninum extract and Brevibacillus agri mixture suppress rice leaf spot pathogen; Nigrospora oryzae and improves the production of red rice (Oryza sativa L). Front. Sustain. Food Sys. 6, 481. doi: 10.3389./fsufs.2022.1080481
Tan, C., Kalhoro, M. T., Faqir, Y., Ma, J., Osei, M. D., Khaliq, G., et al. (2022). Climate-resilient microbial biotechnology: a perspective on sustainable agriculture. Sustainability 14, 1–29. doi: 10.3390/su14095574
Tang, W., Wang, S., Fonseca-Batista, D., Dehairs, F., Gifford, S., Gonzalez, A. G, et al. (2019). Revisiting the distribution of oceanic N2 fixation and estimating diazotrophic contribution to marine production. Nat Commun. 10, 831.
Unggulan, B., Kode, L., Penelitian, T., Ilmu, K. R., Umum, B., Indrayani, A. W., et al. (2021). Bidang Unggulan: Ke tahanan Pangan, Energi dan Lingkungan Kode Topik Penelitian: B1 Kode Rumpun Ilmu: 113 Biologi (Bioteknologi Umum).
Vacheron, J., Desbrosses, G., Bouffaud, M. L., Touraine, B., Moënne-Loccoz, Y., Muller, D., et al. (2013). Plant growth-promoting rhizobacteria and root system functioning. Front. Plant Sci. 4, 1–19. doi: 10.3389/fpls.2013.00356
Vejan, P., Abdullah, R., Khadiran, T., Ismail, S., and Nasrulhaq Boyce, A. (2016). Role of plant growth promoting rhizobacteria in agricultural sustainability—A review. Molecules 21, 1–17. doi: 10.3390/molecules21050573
Vinay, J. U., Naik, M. K., Rangeshwaran, R., Chennappa, G., Shaikh, S. S., Sayyed, R. Z., et al. (2016). Detection of antimicrobial traits in fluorescent pseudomonads and molecular characterization of an antibiotic pyoluteorin. 3 Biotech. 6, 1–11. doi: 10.1007/s13205-016-0538-z
Vocciante, M., Grifoni, M., Fusini, D., Petruzzelli, G., and Franchi, E. (2022). The role of plant growth-promoting rhizobacteria (PGPR) in mitigating plant's environmental stresses. Appl. Sci. 12, 1231. doi: 10.3390./app12031231
Yanti, Y., Hamid, H., Suriani, N. L., and Reddy, M. S. (2023). Screening of indigenous actinobacteria as biological control agents of Colletotrichum capsici and increasing chili production. Egypt. J. Biol. Pest Cont. 4, 9. doi: 10.1186./s41938-023-00660-9
Younes, N. A., Anik, T. R., Rahman, M. M., Wardany, A. A., Dawood, M. F. A., Phan Tran, L-. S., et al. (2023). Effects of microbial biostimulants (Trichoderma album and Bacillus megaterium) on growth, quality attributes, and yield of onion under field conditions. Heliyon 9, e14203. doi: 10.1016/j.heliyon.2023.e14203
Zakaria, A. K., Sayyed, R. Z., and Enshasy, H. E. (2016). “Biosynthesis of antibiotics by PGPR and their roles in biocontrol of plant diseases,” in Plant Growth Promoting Rhizobacteria for Sustainable Stress Management Vol II Rhizobacteria in Biotic Stress Management, ed R. Z. Sayyed (Singapore: Springer), 1–36. doi: 10.1007/978-981-13-6986-5_1
Zapata-Sifuentes, G., Hernandez-Montiel, L. G., Saenz-Mata, J., Fortis-Hernandez, M., Blanco-Contreras, E., Chiquito-Contreras, R. G., et al. (2022). Plant growth-promoting rhizobacteria improve growth and fruit quality of cucumber under greenhouse conditions. Plants 11, 1–9. doi: 10.3390/plants11121612
Zope, V. P., Jadhav, H. P., and Sayyed, R. Z. (2019). Neem cake carrier prolongs the shelf life of biocontrol fungus Trichoderma viridae. Indian J. Exp. Biol. 57, 372–75. Available online at: http://nopr.niscpr.res.in/handle/123456789/47162
Keywords: compost, hormones, phytochemicals, plant herb, rhizobacteria
Citation: Suriani NL, Suprapta DN, Perveen K, Suarsana IN, Resiani NMD, Hamidah E, Rusdianasari, Alsulami JA, Parray JA and Ho TS (2023) Formulation of Brevibacillus agri and compost to improve growth and phytochemicals compound of Piper caninum herbal plant. Front. Sustain. Food Syst. 7:1163426. doi: 10.3389/fsufs.2023.1163426
Received: 10 February 2023; Accepted: 24 July 2023;
Published: 14 August 2023.
Edited by:
Abdelilah Meddich, Cadi Ayyad University, MoroccoReviewed by:
Mohamed Anli, Cadi Ayyad University, MoroccoCopyright © 2023 Suriani, Suprapta, Perveen, Suarsana, Resiani, Hamidah, Rusdianasari, Alsulami, Parray and Ho. This is an open-access article distributed under the terms of the Creative Commons Attribution License (CC BY). The use, distribution or reproduction in other forums is permitted, provided the original author(s) and the copyright owner(s) are credited and that the original publication in this journal is cited, in accordance with accepted academic practice. No use, distribution or reproduction is permitted which does not comply with these terms.
*Correspondence: Ni Luh Suriani, bmlsdWhzdXJpYW5pQHVudWQuYWMuaWQ=
Disclaimer: All claims expressed in this article are solely those of the authors and do not necessarily represent those of their affiliated organizations, or those of the publisher, the editors and the reviewers. Any product that may be evaluated in this article or claim that may be made by its manufacturer is not guaranteed or endorsed by the publisher.
Research integrity at Frontiers
Learn more about the work of our research integrity team to safeguard the quality of each article we publish.