- Unit of Microbiology, Department of Biology and Geology, CITE II-B, Agrifood Campus of International Excellence ceiA3, CIAIMBITAL, University of Almería, Almería, Spain
This work highlights the ability of various actinobacterial formulas, to control the incidence of gray-mold caused by Botrytis cinerea in cucumber seedlings. Protocols applied aimed at the preliminary characterization of the actinobacterial collection and the biopolymers used as carriers were very useful for predicting their phytotoxic, phytostimulating and biopesticidal capacity. First, the phytostimulatory or phytotoxic potential of 3 biopolymers at 3 different concentrations and a collection of 10 actinobacteria were analyzed by calculating the germination index in cucumber seeds by seed dipping (biopriming). In general, two-member consortia and independent actinobacteria previously selected reached a phytostimulant effect on cucumber seedlings after their application by biopriming. Likewise, the selected actinobacteria were characterized, sole and in co-cultures, according to its ability to inhibit the growth of B. cinerea by dual culture bioassays. Finally, after selecting the most effective actinobacterial formulas, a preventive gray-mold bioassay was performed based on cucumber seed biopriming. The strains A5 and A7, in axenic and co-culture, showed to be the most efficient strains against the in vitro growth of B. cinerea. Seed biopriming strategy with actinobacterial formulas revealed a remarkable promoter effect in the early stages of plant development and after the infection with the phytopathogen fungus was remained. Definitely, the microbial formulas used in this work showed a phytostimulant and biopesticide character, laying the foundations for subsequent studies that allow a deeper scrutiny of the mechanisms of action that grant the specialization of the effect that occurs between beneficial microorganisms and specific plant hosts.
1. Introduction
Cucumber (Cucumis sativus L.) is one of the most economically and nutritionally significant crops belonging to the Cucurbitaceae family. It has been widely cultivated worldwide, both in open fields and under plastic. Greenhouse cucumber from the southeast of Spain is one of the most exported crops to other European Union countries. Due to the importance of this horticultural crop in the economic market, avoiding production losses is crucial. Cucumber seedlings are highly susceptible to a wide range of pathogens (Toribio et al., 2021), being the necrotrophic pathogenic fungus Botrytis cinerea, one of the highest incidences (Kong et al., 2015).
B. cinerea, the causal agent of gray-mold, attacks leaves, stems, flowers and fruits of various vegetable crops grown in greenhouses. The broad spectrum of action of B. cinerea, together with the development of resistance to traditional pesticides, has forced the search for new control alternatives (Ben-Shalom et al., 2003; Kong et al., 2015). In this sense, the interest in the use of beneficial microbes as an environmentally sustainable option to replace chemical fertilizers and pesticides is increasing (Meena et al., 2017).
Biopriming is a beneficial process to coat or treat seeds with microorganisms under controlled hydration conditions. This practice improves the state of the seeds before germination, avoiding the appearance of premature radicles. Seed priming with appropriate microbial formulas is associated with increased hydrolytic enzyme activity, reactive oxygen species (ROS) production, changes in internal plant hormone levels, and differential gene expression of proteins related to pathogenesis (PR) from the host plant. After the application of biopriming, the response by the plant to activate the induced systemic resistance (ISR) stands out (Zeyad et al., 2022). This stimulation of the immune system, as well as the preactivation (through priming) of the molecular defense mechanisms against various phytopathogens, is favored by the ability of the microorganisms to colonize not only the seed coat, but later the roots, thanks to their viability in that environment (Aamir et al., 2019). All this leads to better plant growth and greater resistance to biotic and abiotic stresses (Mitra et al., 2021). Microbial biopriming represents an adaptive bio-based strategy to improve the defensive capacity of plants. For this, plant growth-promoting microorganisms (PGPM) are of great interest. PGPM proposed for use in biopriming include many strains of fungi, especially different species of Trichoderma; bacteria, such as Azospirillum spp., Bacillus spp. and Pseudomonas spp.; and actinobacteria, such as Streptomyces spp. or Microbacterium spp (Meena et al., 2017; Mitra et al., 2021; Zeyad et al., 2022; Suárez-Estrella et al., 2023). This last group, filamentous bacteria, is one of those receiving more attention due to its application as an agrobiotechnological tool based on the benefits provided by other areas of industrial microbiology. Actinobacteria, producers of endospores and a wide spectrum of antimicrobial compounds, are known to have antagonistic activity against plant pathogens and stimulate plant defense responses under biotic stress conditions (Jurado et al., 2019; Ebrahimi-Zarandi et al., 2022). Previous works have indicated the use of co-cultures of different strains of actinobacteria as responsible for the induction of the immune system of the plant, not only in vitro, but in vivo (Abbasi et al., 2022; Zeyad et al., 2022). Abbasi et al. (2022) pointed out that the application of actinobactorial cocktails that promote plant growth prior to the appearance of the pathogen showed great potential to reduce the use of synthetic pesticides, presenting a direct impact on the quality of the fruit.
The seed priming technique involving beneficial microorganisms has been previously studied using different crops of commercial interest such as lettuce, tomato or cucumber (Righini et al., 2021; Toribio et al., 2021; Suárez-Estrella et al., 2023), but mainly from the point of view of phytostimulation. In addition, cucumber is considered a model plant to study induced systemic resistance (ISR) against potential pathogens (Ben-Shalom et al., 2003). B. cinerea is one of the pathogens to which this crop is extremely susceptible and its control arouses enormous interest worldwide.
The aim of this work was to evaluate the best actinobacterial formulas to be applied as biological control tools against the gray-mold disease caused by Botrytis cinerea in cucumber seedbeds. Specific objectives were: (i) to check the phytotoxic or phytostimulant effect of different biopolymers to select the best coating film for cucumber seeds; (ii) to check the phytotoxic or phytostimulant effect of the actinobacterial collection to select the best strains for biopriming of cucumber seeds; (iii) to examine the in vitro efficacy of the selected actinobacterial strains on the inhibition of mycelial growth of B. cinerea, by dual culture technique; (iv) to investigate both biostimulant and biopesticide impact in vivo by biopriming preventive assay in cucumber seeds. This study will lay the foundations for future research on the use of actinobacteria, both in consortium and in pure culture, in a preventive manner through the novel seed biopriming technique. This work is part of an environmentally and economically sustainable mode of action, through a premise based on circular economy systems toward, which agricultural activity and the management of crop diseases tend.
2. Materials and methods
2.1. Microorganisms
2.1.1. Collection of actinobacteria
Ten strains of the order Actinomycetales were selected from a collection belonging to the BIO175 group of the University of Almería (Table 1), which stood out for their ability to stimulate plant growth of reference seeds in vitro. Active pure cultures were kept on Standard Methods Agar (APHA) (ISO 4833:2003) (PanReac, Spain) at 4°C until use.
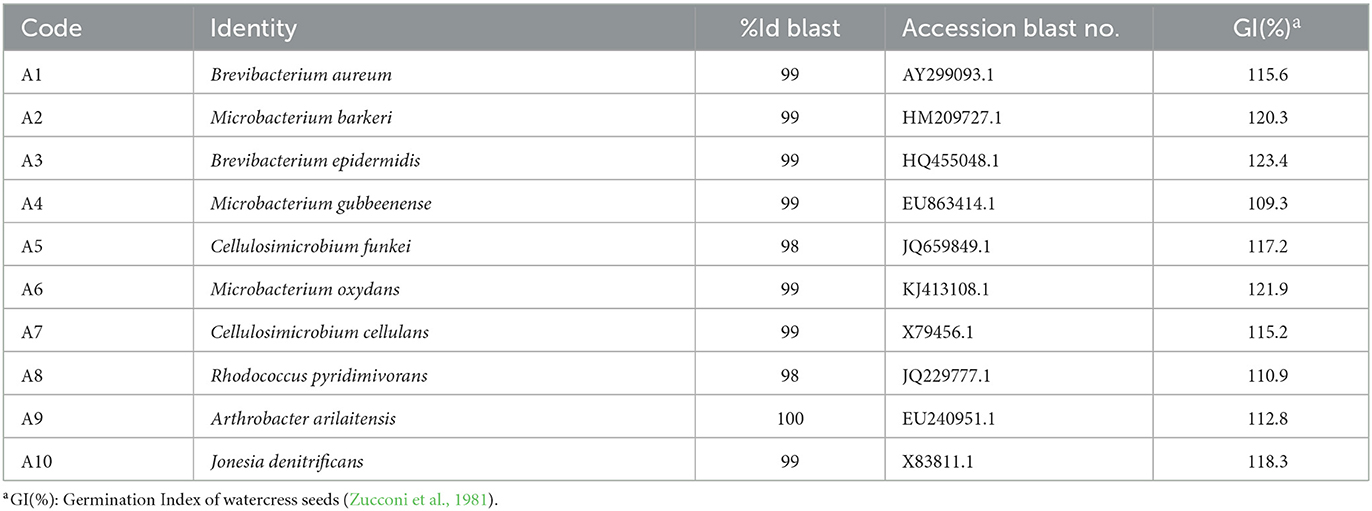
Table 1. Collection of selected strains based on their toxonomic order (Actynomicetales) and their phytostimulant potential on watercress seeds (GI ≥ 100%).
2.1.2. Phytopathogenic agent
A phytopathogenic strain was supplied by Spanish Type Culture Collection (CECT). Fungus culture of Botrytis cinerea CECT 2850 (BC) was kept in slant on Potato Dextrose Agar (PDA) (PanReac, Spain) at 4°C.
2.2. In vitro screening of strains and carriers by biopriming assay
The in vitro bioassay to select the most promising polysaccharide matrix and evaluate the stimulating effect of actinobacteria on cucumber seed (Cucumis sativus var. Ashley) germination was carried out as previously described by Zucconi et al. (1981) for reference seeds on watercress. Promotion effect was tested on four replicates of 25 seeds for each sample. To calculate germination index (GI), percentage of seed germination and elongation of the radicle (mm) were taken into account, based on the following formula:
where GI, germination index (%); Gc%, percentage of germinated seeds in presence of each sample; Gc%, percentage of germinated seeds in presence of distilled water as control; REs, mean of radicle elongation (mm) in presence of each sample; REc, mean of radicle elongation (mm) in presence of distilled water as control.
To select the most promising polysaccharide matrix, three different compounds were tested (sodium alginate-SA; carboxymethyl cellulose- CMC; and xanthan gum-XG) at five different concentrations (0.5, 1, 2, 5, and 10%). Cucumber seeds were washed with sterile distilled water 3 times before being subjected to the immersion bioassays. Then, they were immersed for 1 h in 10 mL volume containing the semi-solid matrix constituted by each concentration studied.
To evaluate the stimulating effect of each actinobacteria, a pre-inoculum was obtained from a culture in Nutrient Broth (NB) (PanReac, Spain) that was incubated at 30°C for 24-48 h. Cucumber seeds were washed with sterile distilled water 3 times before being subjected to the immersion bioassays. Then, they were immersed for 1 h in 10 mL volume containing the semi-solid matrix selected and an aliquot of each actinobacterial culture at a concentration of 105 cfu/mL. Subsequently, the selected strains were subjected to the same test, but using a co-culture strategy, applying the same amount of each one and in the concentration mentioned before.
In addition, an assay was carried out in a Petri plate to ensure that the co-cultures were compatible and that antagonistic phenomena were not observed between the selected strains.
2.3. In vitro inhibition: Dual cultures
The selected strains were cultured in nutrient broth (NB, Oxoid Ltd., UK) for 24 h at 30°C prior to use. Suppressive effect was demonstrated using the modified techniques of Landa et al. (1997). First, 2% water agar (WA) plates were prepared. After the agar solidified, four 8-mm-diameter steel cylinders were placed equidistantly from the edge. A second layer of PDA was added on the WA plates. Once the cylinders were removed, the wells were filled with 80 μL liquid culture of each antagonist, in axenic and mixed culture, to be assayed and a plug of 5-day-old phytopathogen agent (BC) culture, removed from a PDA plate, was placed at the center of the assay plate. Four replicates for each antagonist-PA combination were prepared. Plates were incubated at 30°C for 5 days and the inhibition index (I) was expressed as the percentage of PA inhibition in the presence of the antagonistic strain using the formula:
where I: Inhibition Index (%); C: growth of the phytopathogenic agent in absence of the antagonistic strain (mm); T: growth of the phytopathogenic agent in presence of the antagonistic strain (mm).
2.4. Chromatographic analysis
The selected strains were cultured in NB and kept for 1 week in incubation at 30°C with shaking. Once this time had elapsed, the flasks were taken and centrifuged its content for 10 min at 5,000 rpm to precipitate the biomass and remove it. Next, ethyl acetate (1:1 ratio) was added to the supernatant, and left for 45 min under agitation. After this time, the phases were separated by decantation, and the acetate phase was preserved to search for the compounds of interest. Subsequently, the ethyl acetate was centrifuged for 10 min at 5,000 rpm to achieve excess CN residues precipitate. After this, a rotary evaporator was used to vaporize all the ethyl acetate and obtain a concentrated extract, which was suspended in 200 μL of methanol and then frozen at −20°C until analysis. Sterile culture medium without inoculum was used as a blank for chromatography (Jose et al., 2014).
The samples were subjected to a chromatographic analysis using an HPLC coupled to a QTrap mass analyzer. The chromatographic column used was Agela Technologies UHP AQ C18, 1.9 mm, 2.1 × 50 mm. Data processing was carried out by Software Analyst 1.6.2 and MultiQuant 3.0.2 which through the chromatograms and mass spectra identify the compounds present in each sample.
2.5. In planta evaluation of the biostimulant and biopesticide effect by biopriming assay
Firstly, a sterile substrate mixture (peat:vermiculite, 3:1 ratio) was prepared, sterilized and spread in seedling trays. The most effective strains selected from preliminary experiments were in vivo assayed to determine their potential as plant growth promoters of seedlings and for their protective effect against BC in bioprimed cucumber seeds. A randomized experimental design was established consisting of 30 pretreated cucumber seeds (Cucumis sativus var. Ashley) as previously described in Section 2.2. Seeds were germinated during3weeks at 25°C, 60% humidity and a photoperiod of 12 h light and 12 h dark. Four weeks after germination, the following plant growth parameters were measured to study biostimulant effect: number of leaves, stem and root length (cm), fresh and dry weight (g) and root/stem ratio (R/S ratio).
For the test of the suppressive effect in vivo, once the plants from the pretreated seeds by biopriming grew to present the first true (non-cotyledonous) leaves, seedlings were inoculated with the airborne pathogen (Botrytis cinerea). From a fresh culture of the fungus for 5 days in PDA nutrient medium, a suspension of BC spores was obtained. This suspension was prepared by adding 10 mL of sterile distilled water to the plate with the fungus culture. Next, using a sterile swab, the superficial part (mycelium and spores) of the medium was taken. The mycelium was removed by filtration with sterile muslin to obtain a spore suspension that was quantitated in a Neubauer chamber and then adjusted by adding tap water to an inoculum density of ~106 spores/mL. Finally, the solution was introduced into a sprinkler and applied via the foliar route, making about three sprays per leaf and per plant. The plants were kept under the same conditions as during germination to promote their adequate growth during the infection period.
2.6. Statistical analysis
Data obtained were subjected to statistical analysis using Statgraphics Centurion XIX (StatPoint Technologies Inc., Virginia). A multifactorial analysis of variance (ANOVA) and a multiple comparison test (Fisher's Least Significant Difference) were performed to compare mean values for different levels of repetition (P < 0.05).
3. Results
3.1. Selection of liquid coating: Type of substance and concentration
Three biopolymers were tested as coating materials for cucumber seeds: sodium alginate-SA; carboxymethyl cellulose- CMC; and xanthan gum-XG. Five different concentrations of each substance were tested, but data were only presented for the three smallest (0.5, 1, and 2%) (Figure 1), since at higher concentrations (5% and 10%) seed germination was difficult and the results were not satisfactory. In general, the percentages of the germination indices decreased as the percentage of each substance used in the seed priming suspension increased (Figure 1).
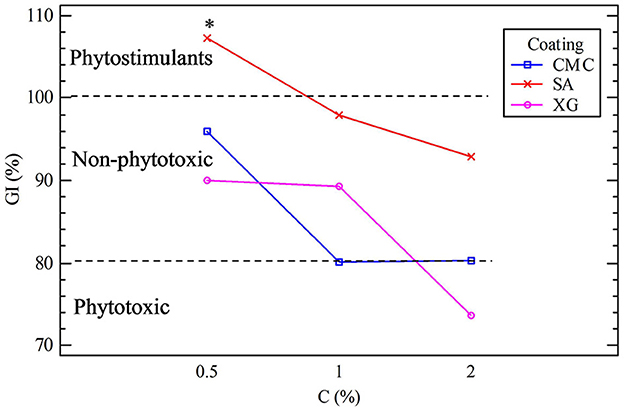
Figure 1. Interaction plot of Germination Index(%) to relate the substances tested (sodium alginate-SA; carboxymethyl cellulose- CMC; and xanthan gum-XG) at different concentrations (C%) (0.5, 1, and 2%) as liquid coatings for cucumber seed priming. Asterisks show different homogeneous groups with statistically significant differences (Fisher's LSD test, P < 0.05).
According to Zucconi et al. (1981), a GI value above 70–80% indicates the absence of phytotoxicity, while a value close to or >100% is indicative of a plant growth promoting effect. As shown on Figure 1, almost all compounds, and at different concentrations applied, were positioned in GI values above 80%, resulting lacking in phytotoxicity, except XG at 2%. It is worth noting the result obtained after the application of SA at 0.5%, since it acted as a promoter of plant growth reaching 107.2% of germination index (Figure 1). Being this compound (SA), and at that concentration (0.5%), the matrix chosen to be applied as film coating together with the microorganisms studied using the biopriming technique.
3.2. Selection of promising actinobacterial strains
Figure 2 shows the GI (%) of cucumber seeds subjected to a biopriming treatment with each of the actinobacteria in the work collection.
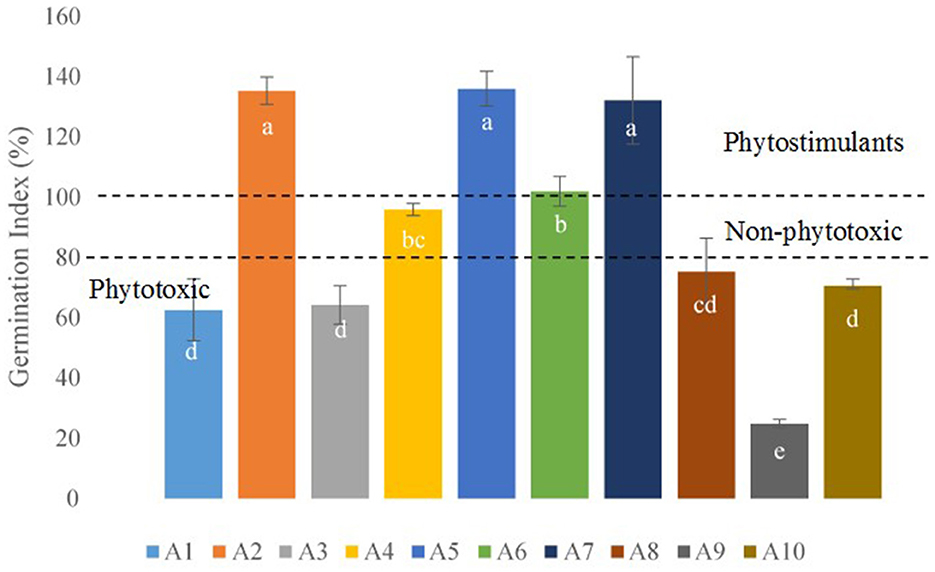
Figure 2. Germination Index (%) derived from the application of actinobacteria with a sodium alginate matrix (0.5%) by biopriming on cucumber seeds. Bars indicate standard error. The letters inside the bars indicate different homogeneous groups with statistically significant differences (Fisher's LSD test, P < 0.05).
Although the application on this same type of seeds of the polysaccharide substance provided benefits during germination in the pre-emergence stage of the seeds, the incorporation of the biological factor yielded diverse results (Figure 2). In fact, 50% of the actinobacteria contributed phytotoxicity to the formulation (GI < 80%). On the other hand, the A2 (135.3% GI), A5 (135.9% GI) and A7 (132.1% GI) strains presented phytostimulant values, significantly different to the other microbial formulations (Figure 2). These strains even notably increased the beneficial effect already shown in the exclusive presence of the alginate matrix (Figure 1). In view of the remarkable results, these three actinobacteria (A2, A5, and A7) were selected as the most promising candidates for the following trials.
Additionally, in the present work the effect of the combination of different strains was tested. For this, two-member consortia were developed combining the three previously selected strains (Consortium 1-C1: A2+A5; Consortium 2-C2: A2+A7; and Consortium 3-C3: A5+A7). Figure 3 shows the GI (%) using co-cultures by biopriming. A compatibility test was also performed to rule out inhibition reactions between any of the proposed co-cultures (Supplementary Figure S1).
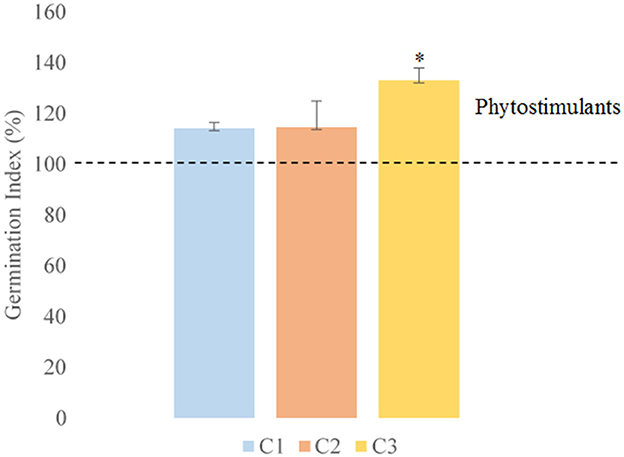
Figure 3. Germination Index (%) derived from the application of two-member consortia (Consortium 1-C1: A2+A5; Consortium 2-C2: A2+A7; and Consortium 3-C3: A5+A7) with a sodium alginate matrix (0.5%) by biopriming on cucumber seeds. Bars indicate standard error Asterisks show different homogeneous groups with statistically significant differences (Fisher's LSD test, P < 0.05).
C1 (114.2% GI) and C2 (114.7% GI) (Figure 3) presented a slight decrease in the percentages of germination index compared to the values presented by the strains when tested independently (Figure 2). In any case, with results considered for plant-growth promoting microorganisms (GI > 100%). While the consortium formed by the A5 and A7 (C3) strains gave a result similar to best values recorded in Figure 2 (133.1% GI). Taking these results into account, the A5 and A7 strains presented significant differences when they were used in consortium, standing out from the others as potential plant growth promoting agents using the biopriming technique.
3.3. In vitro antagonism against Botrytis cinerea: Axenic cultures and consortia
In parallel to the in vitro phytostimulation test, the selected strains (A2, A5, and A7) were subjected to an inhibition assay by dual culture in Petri plate, both alone and in co-culture, against the phytopathogenic fungus B. cinerea (Figure 4).
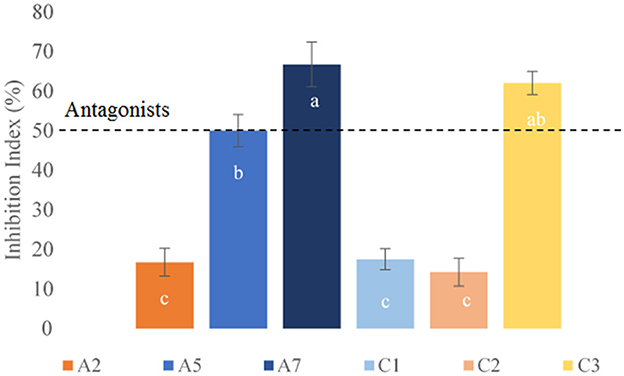
Figure 4. Inhibition Index (I%) derived from the application of three actinobacteria selected and two-member consortia (Consortium 1-C1: A2+A5; Consortium 2-C2: A2+A7; and Consortium 3-C3: A5+A7) against Botrytis cinerea (BC) in vitro. Bars indicate standard error. The letters inside the bars indicate different homogeneous groups with statistically significant differences (Fisher's LSD test, P < 0.05).
All the strains and consortia presented a suppressive character, but to a different extent, with the A7 strain being the most prominent with 66.8% of inhibition. However, this strain (A7) did not present significant differences with the C3 consortium (62.0%) (Figure 4), and this, in turn, did not present significant differences with the A5 strain. This makes the three formulations A5, A7, and C3, the most promising as biopesticide agents using the biopriming technique.
3.4. Chromatographic profile of the selected strains
Table 2 lists the most interesting analytes detected in the microbial suspensions analyzed (A2, A5, and A7) by HPLC. All the selected strains were capable to produce antimicrobial compounds. A7 was especially effective, since showed a wide range of production, being associated to all the analytes founded: Clindamycin, Sulfapyridine, Azidamphenicol, Lincomycin, and Sulfamethizole (Table 2, Supplementary Figure S2).

Table 2. Analytes detected after the chromatogram carried out on the acellular extracts of strains Microbacterium barkeri A2; Cellulosimicrobium funkei A5; and Cellulosimicrobium cellulans A7 concentrated in methanol.
3.5. Early phytostimulation and plant protection against Botrytis cinerea by seed biopriming with actinobacterial strains
After the screening carried out in vitro, in which the A5 and A7 strains were selected as the most promising, both in co-culture and axenic, in vivo tests were carried out to study the phytostimulant and bioprotective effect through plant production parameters from seedlings subjected to biopriming pre-treatment (Figure 5).
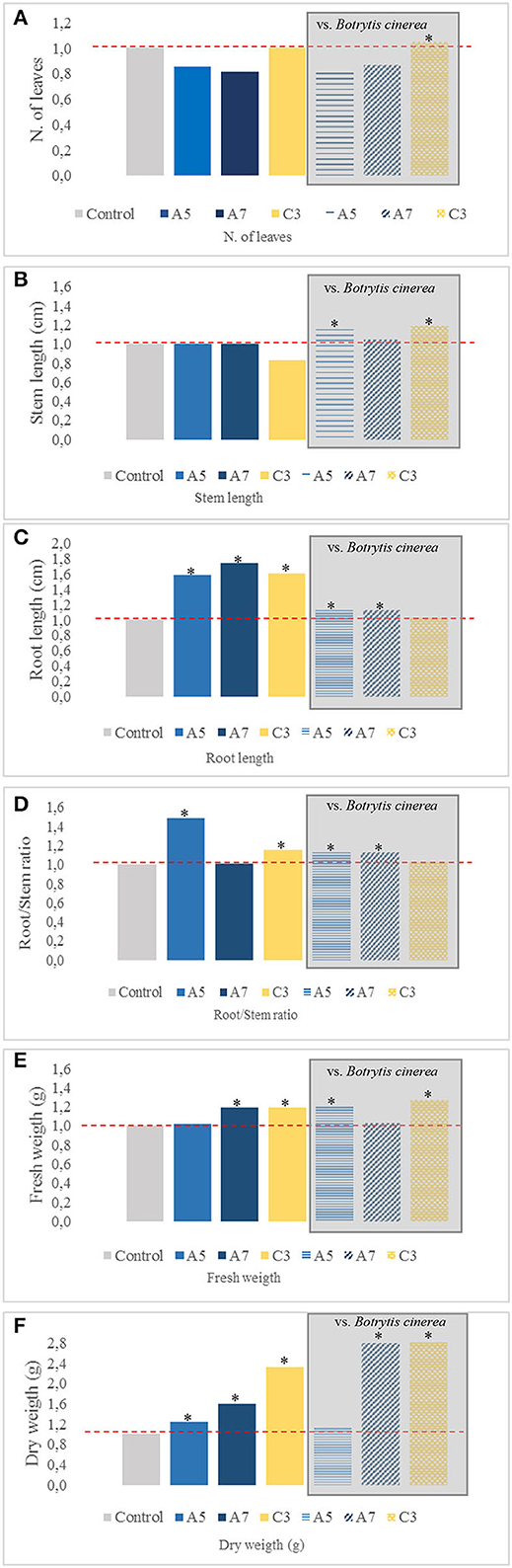
Figure 5. Measurement of the increase with respect to the control of plant growth promoting effect by biopriming application of the three microbial formulations selected: strains A5, and A7, and Consortium-3 (C3: A5+A7). Before (bars with color fill) and after the inoculation with Botrytis cinerea. (A) Number of leaves; (B) Stem length (cm); (C) Root length (cm); (D) Root/Stem ratio; (E) Fresh weight (g); and (F) Dry weight (g). Asterisks show different homogeneous groups with statistically significant differences (Fisher's LSD test, P < 0.05).
During the first part of the in vivo test, the ability of the microbial formulations to stimulate plant growth without incidence of the pathogen was studied. In Figure 5, a strengthening of the root system can be observed, especially notable in the seeds primed with bioformulas A5 and C3 (Figures 5C, D). The fresh and dry weight parameters (Figures 5E, F) also showed an increase with respect to the control seeds during the test of the biostimulant effect of the biopriming technique.
In the subsequent trial, after inoculation with the pathogenic fungus (BC), the seedlings from the different biopriming pretreatments continued to show improvements in the different parameters analyzed. A5 and A7 continued to stand out in terms of the root system, presenting increases in the length of the leaves and in the R/S ratio (Figures 5C, D). In this second part of the assay, the stimulation of the aerial part of the plants from the seeds pretreated with A5 and C3 was observed (Figures 5A, B). The microbial formulations applied to the seeds by means of biopriming resulted in techniques that were not only directly effective as plant growth promoting agents, but also acted as preventive biopesticide agents. In general, the three formulations (A5, A7, and C3) after infection with the phytopathogenic fungus (BC) presented values equal to or higher than the negative control in the different plant protection parameters studied (Figure 5).
4. Discussion
4.1. In vitro bioassays
Adak et al. (2016) defined seed coating as a reliable technique to apply exogenous materials on seeds in order to improve their germination, which indirectly translates into an improvement in the seed quality and yield. This application, known as film coating, allows to encapsulate seeds with a fine layer usually of polymers, but also plasticizers, pigments and solvents, in the form of a synthetic suspension. The thin layer does not change the size and shape of the seeds much, however, improves its handling characteristics. The use of biopolymers in the seed coat has been described as an improvement for seed germination (Adak et al., 2016; Ma, 2019). In fact, the coating agent is considered critical in ensuring the success of seed biopriming. Thus, to select an adequate matrix for the collection of microorganisms studied in this work, three different types of substances, based on the multiple possibilities mentioned in literature, were used: sodium alginate, carboxymethyl cellulose and xanthan gum (Angelopoulou et al., 2014; O'Callaghan, 2016). The types of substances chosen were biopolymers because they have no adverse effect on radicle length and seed germination. Deaker et al. (2004) pointed out that the adhesives polymers such as xanthan gum, methylcellulose, arabic gum, polyvinylpyrolidone, and polyacrylamide, had long been credited with the ability to improve seed survival. Some of the protective properties of biopolymers have been attributed to their abilities to maintain optimal water activity levels for the organism on which they are applied, as well as to limit the transfer of heat.
In this work, SA 0.5% showed the higher coating efficacy in view of the germination index results (Figure 1). Other authors confirm that the use of sodium alginate in microbial formulations favors the colonization of plant roots by the inoculated microorganism, without harming the viability of their cells (Schoebitz et al., 2013; Chin et al., 2021; Toribio et al., 2021; Suárez-Estrella et al., 2023). In this sense, the application of biopriming technique with the collection of strains was done using SA at 0.5% (Figure 1).
The actinobacteria used in this work came from a collection previously characterized by their potential to be applied as agents of interest in agrobiotechnology (Jurado et al., 2019). Of all the strains tested, those belonging to the genus Microbacterium (A2, A4, and A6) and Cellulosimicrobium (A5 and A7) were not phytotoxic to cucumber seeds after being applied by biopriming with SA 0.5% as coating agent (Figure 2). Previous works demonstrated the potential of strains from the Microbacteriaceae family as outstanding microorganisms based on the production of interesting substances (volatile organic compounds, siderophores, chitinase enzymes, salicylic acids and hydrogen cyanide) and suppressive capacity against various phytopathogenic fungi related to damping-off and gray-mold diseases (Jurado et al., 2019; Suárez-Estrella et al., 2023). Similarly, isolates belonging to the genus Cellulosimicrobium have been reported as plant growth promoting agents and antagonists of phytopathogenic fungi such as Botrytis cinerea, Fusarium oxysporum and Verticillium dahliae due to their ability to solubilize phosphate, produce indoleacetic acid, siderophores and beneficial enzymes for plant growth and soil fertility as protease, chitinase, amylase and urease (Nabti et al., 2014). However, the strains that offered the best results were A2 (Microbacterium barkeri), A5 (Cellulosimicrobium funkei), and A7 (Cellulosimicrobium cellulans) (Figure 2), which significantly outperformed the rest with very high values of GI (Figure 2) that suggest a great capacity to promote germination in seeds embedded in these microbial solutions.
The use of artificial formulations based on microbial consortia has shown to have positive synergistic effects on plant growth (Toribio et al., 2022; Zeyad et al., 2022), which is of enormous agrobiotechnological interest. Thus, in this work the three selected actinobacteria were co-cultivated to study the behavior of mixed cultures (Consortium 1-C1: A2+A5; Consortium 2-C2: A2+A7; and Consortium 3-C3: A5+A7) after being used to cover seeds by biopriming (Figure 3), as well as their effect against B. cinerea (Figure 4). Although the three applied consortia were phytostimulants, only C3 reached values similar to those observed after the use of the strains in axenic culture (Figure 3). The combination of the two Cellulosimicrobium strains (C. funkei and C. cellulans) presented the best germination indices. Likewise, these two actinobacteria, both individually and in consortium, presented the highest inhibition indices against the phytopathogenic fungus tested (Figure 4). The potential of Cellulosimicrobium, and specifically of C. cellulans, as biocontrol against fungi such as B. cinerea, and plant growth promoting agent, has been previously described. Among others, it has been associated with the ability to produce chitinases (Nabti et al., 2014).
These results were complemented with chromatographic analyses that showed an antimicrobial potential profile of the strains (Table 2). C. cellulans (A7) stood out, since it presented all the detected analytes (Table 2). The antimicrobial compounds detected by chromatography generally corresponded to substances with broad spectrum antibacterial power. Nevertheless, some substances of specific interest for the present work can be observed in Table 2. For example, clindamycin, produced by the three strains studied, is not a compound related to research against phytopathogens, but there are records of its ability to combat other types of pathogenic fungi, such as Pneumocystis jiroveci, the causative agent of pneumonia in immunocompromised patients (Guay, 2007). On the other hand, sulfapyridine, produced exclusively in the A7 strain, is an antibiotic broad-spectrum synthetic for most gram-positive and gram-negative bacteria. This active compound has also been shown to reduce infections due to phytopathogenic fungi, as well as induces the mechanisms of ISR of plants, since it acts as a functional analog of chemical elicitors, such as salicylic acid (Tripathi et al., 2019).
4.2. In vivo bioassays
The results of plant production revealed that the use of appropriate microorganisms in interaction with the seeds (biopriming) improves the size of plants, with special incidence in the root system of seedlings. However, the fact of using a combination of actinobacteria that separately offer promising results, even without worsening with respect to the result obtained in the control plants, neither does it substantially improve all the parameters analyzed in relation to the application of each strain individually (Figure 5). That is, the synergistic effect expected is not as significant as would be desirable in all the parameters studied. This could incline the choice toward the use of each strain separately, which a priori appears to be more economically viable. However, the use of consortia in different fields of agrobiotechnology is being imposed as it generally results in greater environmental and economic efficiency, since consortia allow to expand the potential of action of the microbial formula (Toribio et al., 2022; Zeyad et al., 2022).
On the other hand, it should be noted that the positive impact on the tested crops remains over time and protects against the development of diseases. Despite the inoculation with the phytopathogenic fungus once the seedling stage has been reached, the parameters analyzed showed that the promotion of plant growth continued and even improved the condition of the seedlings (Figure 5). This could be due to the close interaction achieved by soaking the seeds in a solution containing the appropriate microorganisms and carriers, which strongly influences the survival and colonization ability of the actinobacteria in the roots and even in the adjacent soil. This level of interaction reached by biopriming can be considered similar to the effect produced by bacterial endophytes on plant health, inducing local and systemic defense responses (Singh et al., 2020).
Definitely, seed biopriming, which consists of soaking seeds in a liquid suspension of microbial culture for a specific period, allows physiological processes to be initiated within the seed, increasing plant growth promoting levels in the spermosphere, which in turn increases the proliferation of microbial cells inside the seeds (Bisen et al., 2015). As shown in Figure 5, biopriming with actinobacterial formulas allows the plant to resist adverse conditions, including the protection of the seed from pathogen attack.
5. Conclusions
The biopriming technique facilitates the germination of the seeds, in addition, it favors the adhesion of the microorganisms included in the formulation on the plants subjected to biostimulation and bioprotection treatments. The actinobacteria Microbacterium barkeri (A2), Cellulosimicrobium funkei (A5) and Cellulosimicrobium cellulans (A7) present adequate metabolic and physiological qualities for their use as agrobiotechnological tools. The potential of strains has been studied from plant growth promoting and gray-mold disease suppressors point of view. The actinobacteria selected for the in vivo tests, Cellulosimicrobium funkei (A5) and Cellulosimicrobium cellulans (A7), cause very significant changes in the development of the seedlings, even after subjecting the seedlings to the attack of the studied pathogen (Botrytis cinerea). The use of the most promising strains in a combined treatment (consortium) improves the efficacy of the strains involved, especially acting as a plant growth promoting bioformula. However, due to the multiple factors that intervene in the effectiveness of the consortia with respect to a specific crop, it is necessary to continue studying the mechanisms in more depth in subsequent works. It is worth noting the compatibility of the actinobacteria studied as consortia and their potential as promising agrobiotechnological tools.
Data availability statement
The raw data supporting the conclusions of this article will be made available by the authors, without undue reservation.
Author contributions
MJ: conceptualization, investigation, methodology, data curation, and writing—original draft preparation. FS-E and ML: conceptualization, methodology, and supervision. AT, MM-G, ME-G, and JL-G: investigation and methodology. All authors contributed to the article and approved the submitted version.
Funding
This research has been funded by the projects AGL2009-08405 and AGL2012-36434 of the Spanish Ministry of Science and Innovation, as well as by the own funds of the BIO-175 Research Group of the University of Almeria (Spain).
Conflict of interest
The authors declare that the research was conducted in the absence of any commercial or financial relationships that could be construed as a potential conflict of interest.
Publisher's note
All claims expressed in this article are solely those of the authors and do not necessarily represent those of their affiliated organizations, or those of the publisher, the editors and the reviewers. Any product that may be evaluated in this article, or claim that may be made by its manufacturer, is not guaranteed or endorsed by the publisher.
Supplementary material
The Supplementary Material for this article can be found online at: https://www.frontiersin.org/articles/10.3389/fsufs.2023.1158722/full#supplementary-material
References
Aamir, M., Kashyap, S. P., Zehra, A., Dubey, M. K., Singh, V. K., Ansari, W. A., et al. (2019). Trichoderma erinaceum bio-priming modulates the WRKYs Defense programming in tomato against the Fusarium oxysporum f. sp. Lycopersici (Fol) challenged Condition. Front. Plant Sci. 10, 911. doi: 10.3389/fpls.2019.00911
Abbasi, S., Kafi, S. A., Karimi, E., and Sadeghi, A. (2022). Streptomyces consortium improved quality attributes of bell pepper fruits, induced plant defense priming, and changed microbial communities of rhizosphere under commercial greenhouse conditions. Rhizosphere 23, 100570. doi: 10.1016/j.rhisph.2022.100570
Adak, T., Kumar, J., Shakil, N. A., and Pandey, S. (2016). Role of nano-range amphiphilic polymers in seed quality enhancement of soybean and imidacloprid retention capacity on seed coatings. J. Sci. Food Agric. 96, 4351–4357. doi: 10.1002/jsfa.7643
Angelopoulou, D. J., Naska, E. J., Paplomatas, E. J., and Tjamos, S. E. (2014). Biological control agents (BCAs) of Verticillium wilt: influence of application rates and delivery method on plant protection, triggering of host defence mechanisms and rhizosphere populations of BCAs. Plant Pathol. 63, 1062–1069. doi: 10.1111/ppa.12198
Ben-Shalom, N., Ardi, R., Pinto, R., Aki, C., and Fallik, E. (2003). Controlling gray mould caused by Botrytis cinerea in cucumber plants by means of chitosan. Crop Prot. 22, 285–290. doi: 10.1016/S0261-2194(02)00149-7
Bisen, K., Keswani, C., Mishra, S., Saxena, A., Rakshit, A., and Singh, H. B. (2015). “Unrealized potential of seed biopriming for versatile agriculture,” in Nutrient Use Efficiency: from Basics to Advances, eds A. Rakshit, H. B. Singh, and A. Sen (New Delhi: Springer). doi: 10.1007/978-81-322-2169-2_13
Chin, J. M., Lim, Y. Y., and Ting, A. S. Y. (2021). Biopolymers for biopriming of Brassica rapa seeds: a study on coating efficacy, bioagent viability and seed germination. J. Saudi Soc. Agric. Sci. 20, 198–207. doi: 10.1016/j.jssas.2021.01.006
Deaker, R., Roughley, R. J., and Kennedy, I. R. (2004). Legume seed inoculation technology—a review. Soil Biol. Biochem. 36, 1275–1288. doi: 10.1016/j.soilbio.2004.04.009
Ebrahimi-Zarandi, M., Saberi Riseh, R., and Tarkka, M. T. (2022). Actinobacteria as effective biocontrol agents against plant pathogens, an overview on their role in eliciting plant defense. Microorganisms 10, 1739. doi: 10.3390/microorganisms10091739
Guay, D. (2007). Update on clindamycin in the management of bacterial, fungal and protozoal infections. J. Expert Opin. Pharmacother. 8, 2401–2444. doi: 10.1517/14656566.8.14.2401
Jose, P. A., Sivakala, K. K., Rajeswari, P., and Jebakumar, S. R. D. (2014). Characterization of antibiotic producing rare actinomycete Nonomuraea sp. JAJ18 derived from an Indian coastal solar saltern. Sci. World J. 2014, 456070. doi: 10.1155/2014/456070
Jurado, M. M., Suárez-Estrella, F., López, M. J., López-González, J. A., and Moreno, J. (2019). Bioprospecting from plant waste composting: actinobacteria against phytopathogens producing damping-off. Biotechnol. Rep. 23, e00354. doi: 10.1016/j.btre.2019.e00354
Kong, W., Chen, N., Liu, T., Zhu, J., Wang, J., He, X., et al. (2015). Large-scale transcriptome analysis of cucumber and Botrytis cinerea during infection. PLoS ONE 10, e0142221. doi: 10.1371/journal.pone.0142221
Landa, B., Hervás, A., Bettiol, W., and Jiménez-Díaz, R. M. (1997). Antagonistic activity of bacteria from the chickpea rhizosphere against Fusarium oxysporum f.sp. ciceris. Phytoparasitica 25, 305–318. doi: 10.1007/BF02981094
Ma, Y. (2019). Seed coating with beneficial microorganisms for precision agriculture. Biotechnol. Adv. 37, 107423. doi: 10.1016/j.biotechadv.2019.107423
Meena, V. S., Meena, S. K., Verma, J. P., Kumar, A., Aeron, A., Mishra, P. K., et al. (2017). Plant beneficial rhizospheric microorganism (PBRM) strategies to improve nutrients use efficiency: a review. Ecol. Eng. 107, 8–32. doi: 10.1016/j.ecoleng.2017.06.058
Mitra, D., Mondal, R., Khoshru, B., Shadangi, S., Mohapatra, P. K. D., and Panneerselvam, P. (2021). Rhizobacteria mediated seed bio-priming triggers the resistance and plant growth for sustainable crop production. Curr. Res. Microbial Sci. 2, 100071. doi: 10.1016/j.crmicr.2021.100071
Nabti, E., Bensidhoum, L., Tabli, N., Dahel, D., Weiss, A., Rothballer, M., et al. (2014). Growth stimulation of barley and biocontrol effect on plant pathogenic fungi by a Cellulosimicrobium sp. strain isolated from salt-affected rhizosphere soil in northwestern Algeria. Eur. J. Soil Biol. 61, 20–26. doi: 10.1016/j.ejsobi.2013.12.008
O'Callaghan, M. (2016). Microbial inoculation of seed for improved crop performance: issues and opportunities. Appl. Microbiol. Biotechnol. 100, 5729–5746. doi: 10.1007/s00253-016-7590-9
Righini, H., Francioso, O., Di Foggia, M., Prodi, A., Quintana, A. M., and Roberti, R. (2021). Tomato seed biopriming with water extracts from Anabaena minutissima, Ecklonia maxima and Jania adhaerens as a new agro-ecological option against Rhizoctonia solani. Sci. Hortic. 281, 109921. doi: 10.1016/j.scienta.2021.109921
Schoebitz, M., López, M. D., and Roldán, A. (2013). Bioencapsulation of microbial inoculants for better soil–plant fertilization. A review. Agron. Sust. Dev. 33, 751–765. doi: 10.1007/s13593-013-0142-0
Singh, S., Singh, U. B., Malviya, D., Paul, S., Sahu, P. K., Trivedi, M., et al. (2020). Seed biopriming with microbial inoculant triggers local and systemic defense responses against Rhizoctonia solani causing banded leaf and sheath blight in maize (Zea mays L.). Int. J. Environ. Res. Public Health 17, 1396. doi: 10.3390/ijerph17041396
Suárez-Estrella, F., Jurado, M. M., López-González, J. A., Toribio, A. J., Martínez-Gallardo, M. R., Estrella-González, M. J., et al. (2023). Seed priming by application of Microbacterium spp. strains for control of Botrytis cinerea and growth promotion of lettuce plants. Sci. Horticult. 313, 111901. doi: 10.1016/j.scienta.2023.111901
Toribio, A. J., Jurado, M. M., Suárez-Estrella, F., López, M. J., López-González, J. A., and Moreno, J. (2021). Seed biopriming with cyanobacterial extracts as an eco-friendly strategy to control damping off caused by Pythium ultimum in seedbeds. Microbiol. Res. 248, 126766. doi: 10.1016/j.micres.2021.126766
Toribio, A. J., Suárez-Estrella, F., Jurado, M. M., López-González, J. A., Martínez-Gallardo, M. R., and López, M. J. (2022). Design and validation of cyanobacteria-rhizobacteria consortia for tomato seedlings growth promotion. Sci. Rep. 12, 13150. doi: 10.1038/s41598-022-17547-8
Tripathi, D., Raikhy, G., and Kumar, D. (2019). Chemical elicitors of systemic acquired resistance-Salicylic acid and its functional analogs. Curr. Plant Biol. 17, 48–59. doi: 10.1016/j.cpb.2019.03.002
Keywords: actinobacteria, biopriming, phytostimulant, biopesticide, Botrytis cinerea
Citation: Jurado MM, Suárez-Estrella F, Toribio AJ, Martínez-Gallardo MR, Estrella-González MJ, López-González JA and López MJ (2023) Biopriming of cucumber seeds using actinobacterial formulas as a novel protection strategy against Botrytis cinerea. Front. Sustain. Food Syst. 7:1158722. doi: 10.3389/fsufs.2023.1158722
Received: 04 February 2023; Accepted: 09 March 2023;
Published: 04 April 2023.
Edited by:
Edel Pérez-López, Laval University, CanadaReviewed by:
Mohamed Anli, Cadi Ayyad University, MoroccoFrutos Carlos Marhuenda-Egea, University of Alicante, Spain
Copyright © 2023 Jurado, Suárez-Estrella, Toribio, Martínez-Gallardo, Estrella-González, López-González and López. This is an open-access article distributed under the terms of the Creative Commons Attribution License (CC BY). The use, distribution or reproduction in other forums is permitted, provided the original author(s) and the copyright owner(s) are credited and that the original publication in this journal is cited, in accordance with accepted academic practice. No use, distribution or reproduction is permitted which does not comply with these terms.
*Correspondence: Macarena M. Jurado, mjr956@ual.es