- 1College of Food and Biological Engineering, Chengdu University, Chengdu, China
- 2College of Food Science and Technology, Sichuan Tourism University, Chengdu, China
- 3Guangdong Province Key Laboratory of Marine Biotechnology, Shantou University, Shantou, China
- 4Zigong Taifu Agricultural and Sideline Products Processing Plant, Zigong, China
- 5Sichuan Dimeng Technology Co., Ltd, Zigong, China
A low-temperature and low-salt industrially fermented Chinese kohlrabi (LSCK) was developed in this study, with the salt usage decreased by approximately 70% compared to the traditional high-salt fermented Chinese kohlrabi (HSCK). The differences in physicochemical properties, metabolites and overall flavors during LSCK fermented for 0, 45 and 90 days (d) were analyzed by gas chromatography-time-of-flight mass spectrometry (GC-TOF-MS), electronic nose (E-nose) and other techniques. The results showed that the total acid content increased significantly from 3.68 to 8.59 g/kg. However, the protein content significantly decreased from 2.52/100 to 0.66 g/100 g. The number of lactic acid bacteria cells increased significantly from 3.69 to 4.46 log10CFU/g. Based on multivariate statistical analysis, 21, 14, and 15 differential metabolites were identified in the three treatment groups A1 (0 and 45 days), A2 (45 and 90 days), and A3 (0 and 90 days) respectively (VIP > 1, p < 0.05, |log2FC| ≥ 1.1). Carbohydrates, sugar alcohols, amino acids and their derivatives were the main differential metabolites in the LSCKs fermented for different periods. Aminoacyl−tRNA biosynthesis and glycine, serine and threonine metabolism pathways significantly correlated with the differential metabolites based on Kyoto Encyclopedia of Genes and Genomes (KEGG) analysis (p < 0.05). Furthermore, the overall odors were significantly different among the LSCKs with different fermentation periods, as detected by E-nose. The present study describes the change trend of metabolites during LSCK fermentation and elucidates important metabolic pathways in LSCK, providing a theoretical basis for the target regulation of functional metabolites in kohlrabi and the optimization of LSCK processing.
1. Introduction
Kohlrabi (Brassica juncea var. megarrhiza Tsen et Lee), belonging to cruciferous family with a strong spicy taste, which is rich in dietary fiber, vitamins C, B6, K and other essential elements. Regular intake of kohlrabi will effectively improve body’s immunity and prevent colds (Pasko et al., 2021). Kohlrabi originated in China and is mainly grown in Southwest China (Sichuan, Yunnan and Guizhou Provinces), Southeast China (Jiangsu and Zhejiang Provinces) and Central China (Hunan and Hubei Provinces). The fermented kohlrabi is normally used to prepare side dish in Chinese cuisine. It is well known that salt is one of the most important additives in fermented vegetables processing, which can increase osmotic pressure and reduce the water activity of fermented vegetables, resulting in cell water loss and thus inhibit the growth of spoilage−related microorganisms (Bansal and Mishra, 2020). Additionally, salt can maintain fermented foods texture by regulating the moisture content and moisture distribution and also the proportion of fermented food components (Lin et al., 2021). It has been well documented that salt affects fermented food flavor mainly through lipids and proteins oxidation (Wen et al., 2019; Lin et al., 2021). On the other hand, microorganism is another key factor to the flavor development and metabolite synthesis for fermented food. Wang D. et al. (2022) found that Actiplantibacillus, Halanaerobium, Halomonas, Pseudomonas, Enterobacteriaceae and Debayomyces hansenii were the dominant bacteria in fermented mustard. Sha et al. (2017) found that Proteobacteria, Firmicutes, and Actinobacteria were the dominant bacteria in Marcha and thiat. Lactic acid bacteria including Lactococcus, Leuconostoc, Weissella, Pediococcus, and Lactobacillus are considered as the main microorganisms in fermented vegetables (Hossain et al., 2020). It has been well demonstrated that lactic acid bacteria can produce organic acids and volatile compounds through lactic acid fermentation, having a positive impact on the taste and nutritional components (vitamins and amino acids) of food, and promoting to improve the sensory characteristics of food (Kumar Verma et al., 2022; Yilmaz et al., 2022).
Generally, approximately 15% (w/w) salt are required for production of traditional Chinese kohlrabi. Desalination is thus an important step before obtaining the finished kohlrabi products, which not only wastes a lot of water, but also increases the cost for sewage treatment. More importantly, water-soluble nutrients such as vitamins, amino acids and minerals will loss during desalination. Therefore, low-salt fermentation is attracting more and more attention from researchers all over the world (Hu et al., 2022).
In recent years, metabolomics has been an excellent approach for evaluating prebiotic changes (Kim et al., 2019), phenotype differentiation (Lee et al., 2018), palatability and unhealthiness (Singh et al., 2017) during vegetable fermentation. Liu D. Q. et al. (2021) suggested that halophilic bacteria and lactic acid bacteria were the dominate microorganisms during the spontaneous fermentation of potherb mustard (Brassica juncea var. multiceps) by metagenomics, which closely associated with the formation of organic acids, amino acids and volatile flavor compounds as evaluated by metabolomics. Kim et al. (2013) found that 4-aminobutyric acid, acetate and other 10 substances were the important metabolites for distinguishing Korean cabbages from Chinese cabbages by using of metabolomics analysis. Saleh et al. (2020) confirmed the anti-diabetic efficacy of salak fruit by metabolomics based on 1H nuclear magnetic resonance (NMR). Moreover, metabolomics methods have been used to explore the effects of lactic acid bacteria and molds on the formation of volatile compounds in cheese (Suzuki-Iwashima et al., 2020). Nissen et al. evaluated the fermentation performances of Zymomonas mobilis (Nissen et al., 2021a) and volatilome changes during probiotic fermentation of combined soy and rice drinks (Nissen et al., 2021b) by using a metabolomic approach.
Currently, progress on LSCK has been rarely reported. The metabolites and the associated metabolic pathways of LSCK have not yet been described. In the present work, the LSCK was produced, without desalination during the whole process. Furthermore, the usage of salt was decreased by approximately 70% in the low-salt fermentation compared to the traditional high-salt fermentation. Metabolomics combined with multivariate statistical analysis were employed to analyze the LSCK metabolites changes at different fermentation times for the first time. The present study described the differential metabolites and their change trends during LSCK fermentation and demonstrated the closely related pathways in LSCK, providing a theoretical basis for the target regulation of functional metabolites in kohlrabi and development of new LSCK products.
2. Materials and methods
2.1. Production of LSCKs
The kohlrabies were cultivated with the same variety (Queyecai) in Chengjia Town (latitude 29 degrees 39′ north and longitude 104 degrees 21′ east.) and grown from August 2021 to January 2022 in Zigong city, China. Freshly harvested kohlrabies were washed with water to remove sediment, and then dried outside for 30 days (average temperature 7–12°C). After natural air-dry, the kohlrabies were washed again by warm water (60 ± 2°C) for 10 min, which were subsequently dried in hot air vibrators at 35–40°C for 15 min. The kohlrabies and salt (2.5%, w/w) were transferred into a blender and stirred at a slow speed for 5 min, which were subsequently put into a 200 kg-tank containing 1,000–1,600 kohlrabies depending on the size of the kohlrabies, and pickled at 4°C ± 1°C for 2 days. The kohlrabies were taken to the blender again, yellow sugar (4%, w/w) and salt (1.5%, w/w) were added to the blender and subsequently stirred at a slow speed for 5 min, which were removed into 200 − kg tanks again. Finally, the treated kohlrabies were fermented at 4°C ± 1°C for 0, 45, and 90 days. The usage of salt in LSCK production was decreased by approximately 70% compared to the traditional high-salt fermented kohlrabi, as described in our previous study (Zhang et al., 2022; Chen et al., 2023). Moreover, desalination was not performed during the whole process. Fifteen kohlrabies were randomly selected from the upper, middle and lower layers of the fermentation tanks, which were ground and vacuum-packed in aluminum foil bags and stored at −80°C before analysis (Rubel Mozumder et al., 2020).
2.2. Determination of physicochemical properties and lactic acid growth for LSCK
The total acid, protein and reducing sugar were determined as described in our previous study (Nie et al., 2022). Total acid, reducing sugar and protein were, respectively, determined by acid–base titration, direct titration of copper tartrate alkaline solution and Kjeldahl nitrogen determination method. Lactic acid bacteria cells in LSCK was determined according to the method of Skonberg et al. (2021). Twenty-five grams of ground kohlrabi were resuspended in 225 ml of 0.90% NaCl and subsequently serial diluted (10−1–10−5) with 0.90% NaCl. A hundred microliters of the cell dilutions were plated on de Man Rogosa and Sharpe (MRS) agar plates (Solarbio, Beijing, China). Amino acid nitrogen was determined by the idea of Benjakul et al. (1997), using the formaldehyde value method. The ground kohlrabi was diluted by deionized water and subsequently titrated to pH9.2 by 0.05 M NaOH after the addition of formaldehyde solution. The amino acid nitrogen of the kohlrabi was expressed in g/100 g sample by calculation. All experiments were carried out in triplicate.
2.3. Extraction of LSCKs metabolites
Extraction of LSCK metabolites was performed according to the previous study (Want et al., 2013). The LSCK was accurately weighed 50 mg, added with 0.5 ml of a mixed solution (acetonitrile: isopropanol: water = 3:3:2, v/v/v), and vortex−mixed at 30 Hz for 20 s (Vortex Mixer, QL-866, Kylin−Bell Instrument Manufacturing Co., Ltd., Haimen, China). This operation was repeated eight time and followed by treated ultrasonically in an ice−water bath for 5 min. The solution was added with 0.5 ml of the same mixed solution and subsequently sonicated in an ice−water bath for 5 min again. After centrifugation at 15000 g for 2 min, precisely 500 μl of the supernatant were harvested and dried in a vacuum for 8–10 h. The dried residue was resuspended in 80 μl of 20 mg/ml methoxyamine pyridine solution, vortexed for 30 s and incubated at 60°C for 60 min (Ding et al., 2022). Finally, the solution was derivatized by 100 μl of BSTFA−TMCS reagent (99:1) for 90 min at 70°C. The final solution was centrifuged at 2,0000 g for 3 min, and 90–100 μl of the supernatant was gained and filled into the detection bottle. Quality control (QC) sample was prepared by mixing 20 μl of each extracts (Yang et al., 2022). All samples were analyzed in 24 h.
2.4. Gas chromatography-time-of-flight mass spectrometry analysis
Gas chromatography-time-of-flight mass spectrometry analysis was performed according to the previous research (Mu et al., 2019). For separation, a DB−5MS capillary column (30 m × 250 μm i.d., 0.25 μm film thickness, Agilent J & W Scientific, Folsom, CA, United States) was used for gas chromatography. Derivatized species were separated at a constant flow of 1 ml/min, and 1 μl of the sample was injected through the autosampler in a split ratio of 1:10. The inlet temperature was 280°C, and the transfer line and ion source temperatures were 320°C and 230°C, respectively. The heating programs were as follows: initial temperature 50°C maintained for 0.5 min, elevated to 320°C at a rate of 15°C/min and held for 9 min. Full scan method was as follows: scan rate 10 spec/s, electron energy 70 V, and solvent delay 3 min. Three parallel experiments were performed for every sample.
2.5. Gas chromatography-time-of-flight mass spectrometry data preprocessing and analysis
The raw GC − TOF − MS data was converted into a common format by Analysis Base File Converter software, which was subsequently pre − processed by MSDIAL software (Xu et al., 2023). Differential metabolites were characterized according to the molecular weight (molecular weight error < 30 ppm). Fragmentation information obtained in MS/MS mode was further matched and annotated in HMDB, Metlin, Massbank, LipMaps, mzclound and BioNovoGene (Suzhou, China) databases. The matrix containing metabolite names and peak intensities was processed by missing value imputation and normalization and the missing values filled to one−half of the minimum value. The matrix was subsequently imported into SIMCA−P (version 14.1, Umetrics, Umea, Sweden) for principal component analysis (PCA) and orthogonal partial least squares discrimination analysis (OPLS−DA) (Liu Y. et al., 2021).
2.6. E-nose analysis
E − nose analysis was performed using a Fox 4,000 Sensory Array Fingerprint Analyzer (Alpha M.O.S., Toulouse, France), as described in our previous studies (Nie et al., 2022; Chen et al., 2023). For E − nose analysis, 0.5 g of LSCK was ground and transferred into a 10 − mL headspace bottle. The procedures were as follows: balance time 5 min at 70°C, injection temperature 70°C, injection speed 500 μl/s, injection period 1 s, data−acquisition time 120 s, detection time 180 s. A PCA plot of the E − nose was constructed to further analyze all the samples. Five parallel experiments were performed for every sample.
2.7. Statistical and bioinformatics analysis
Student’s t − test was performed to analyze the metabolites by using IBM SPSS Statistics (version 24). SIMCA 14.1 software (Umetrics, Umea, Sweden) was used for multivariate statistical analysis. To further clearly understand the changes in metabolite composition during kohlrabi fermentation, principal component analysis (PCA) and orthogonal partial least squares-discriminant analysis (OPLS-DA) were used to analyze the metabolic patterns. Based on the OPLS-DA model, the variable importance in the projection (VIP) values of metabolites were calculated (Mu et al., 2019). The fold change of each metabolite was calculated by EXCEL (version 2016). The metabolites with |log2FC| > 1.1, VIP > 1, and p < 0.05 from ANOVA were determined as significantly different metabolites. The curve for the relationship between mass spectrum intensity and time was drawn according to Wang’s method (Wang Z. et al., 2022). The correlation between the differential metabolites were calculated by Pearson’s correlation coefficients and visualized by R 4.2.1. Various public databases were searched, including the KEGG, PubChem to further validate the details of the identified metabolites. Metaboanalyst1 (accessed on December 27, 2022) was used for KEGG pathway enrichment analysis (Zhao et al., 2022).
3. Results
3.1. Analyses of the physicochemical properties and lactic acid bacteria growth for LSCK
The physicochemical properties and lactic acid bacteria growth are shown in Table 1. The total acid content increased significantly during the whole fermentation process (p < 0.05). However, the content of amino acid nitrogen, protein and reducing sugar decreased significantly within 0–45 days (p < 0.05) and there was no significant change after the first 45 days fermentation (p > 0.05). Similarly, the number of lactic acid bacteria cells increased significantly in 0–45 days (p < 0.05), which was not significantly changed in the later stage (p > 0.05).
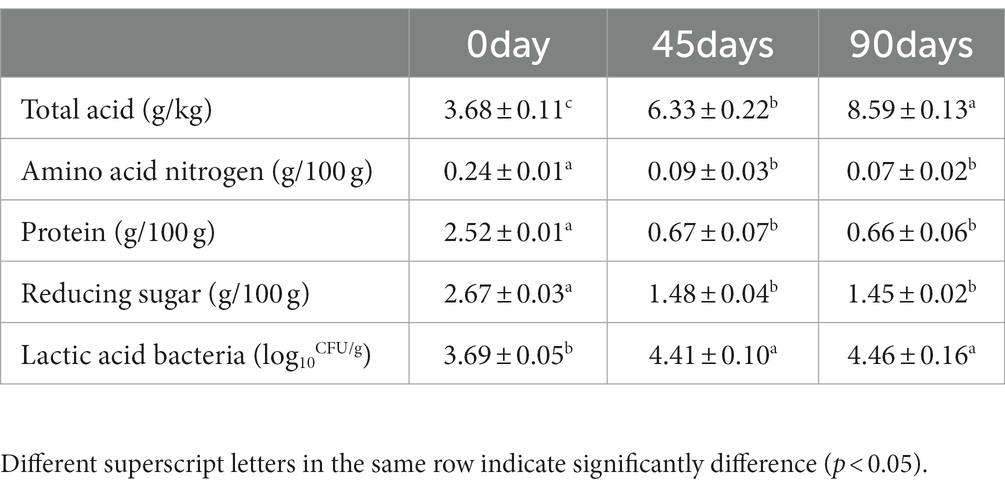
Table 1. Changes in physicochemical properties and lactic acid bacteria growth of LSCKs fermented for 0, 45, and 90 days.
3.2. Multivariate statistical analysis of the GC-TOF-MS data
A total of 98 metabolites were identified from the kohlrabies by using GC-TOF-MS, as shown in Supplementary Table S1. The PCA was performed to analyze the original data and preliminarily determine the differences in metabolites among LSCKs fermented for different periods (0, 45, and 90 days). The PCA result implied that all samples were well distributed in different regions, as revealed in Figure 1. The QC samples were also distributed around the origin with good repeatability, suggesting good system stability and high data reliability. In the PCA model, the score plot displayed variables of 44.7 and 30.1%, respectively. Cross−validation of the PCA model with R2X (R2X = 0.839 > 0.5) indicated that the established model exhibited a good interpretability. The three LSCKs were all significantly separated without overlapping, implying that there were significant differences in metabolites in LSCKs fermented for different periods (0, 45 and 90 days).
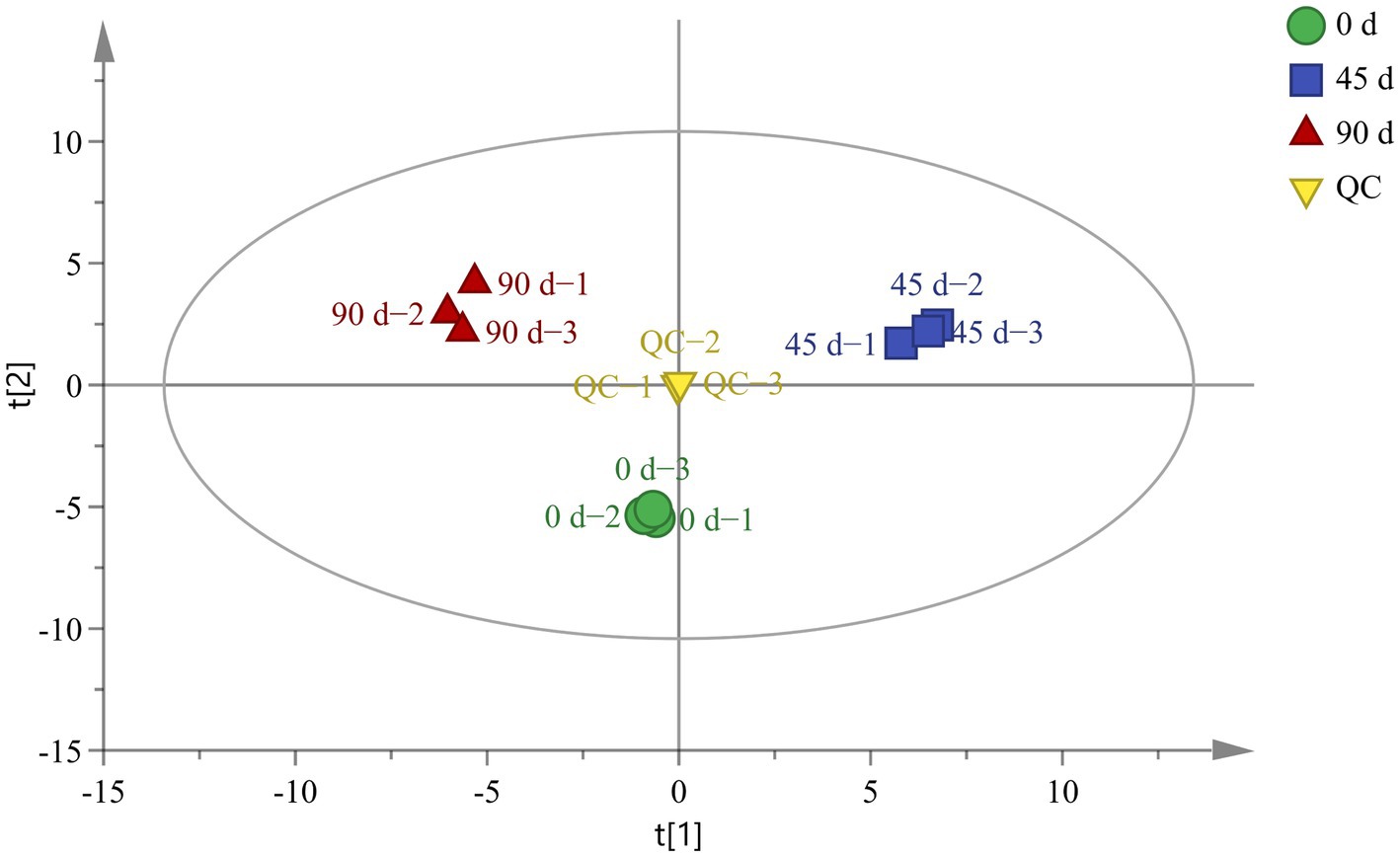
Figure 1. Principal component analysis (PCA) scores for LSCKs fermented for 0 days (circle), 45 days (square), and 90 days (regular triangle).
In order to further analyze the LSCKs metabolites differences, the OPLS−DA method was employed, which removes the orthogonal variables that are not related to classification variables from metabolites to obtain more reliable information (Fu et al., 2019). With the extension of fermentation, the OPLS−DA score plot for all samples showed distinct separation (Figures 2A,C,E). All OPLS−DA models parameters of the 0, 45, and 90 d fermented LSCKs were R2X > 0.5 and Q2 > 0.9 (Table 2), indicating that the models could well interpret the differences. Furthermore, the 200 − cycle iteration permutation test was used to evaluate whether the OPLS−DA model was overfitting. The regression lines of all Q2 points intersected the negative semi−axis with the Y axis and no overfitting was observed (Figures 2B,D,F). The high R2Y (cum) and Q2 (cum) value, together with the negative Q2 value from the 200- cycle iteration permutation test, indicated that the established models were reliable (Westerhuis et al., 2008). Based on PCA and OPLS−DA results, it could be concluded that the three LSCK samples sets could be undoubtedly separated, suggesting that the metabolites among them varied distinctly.
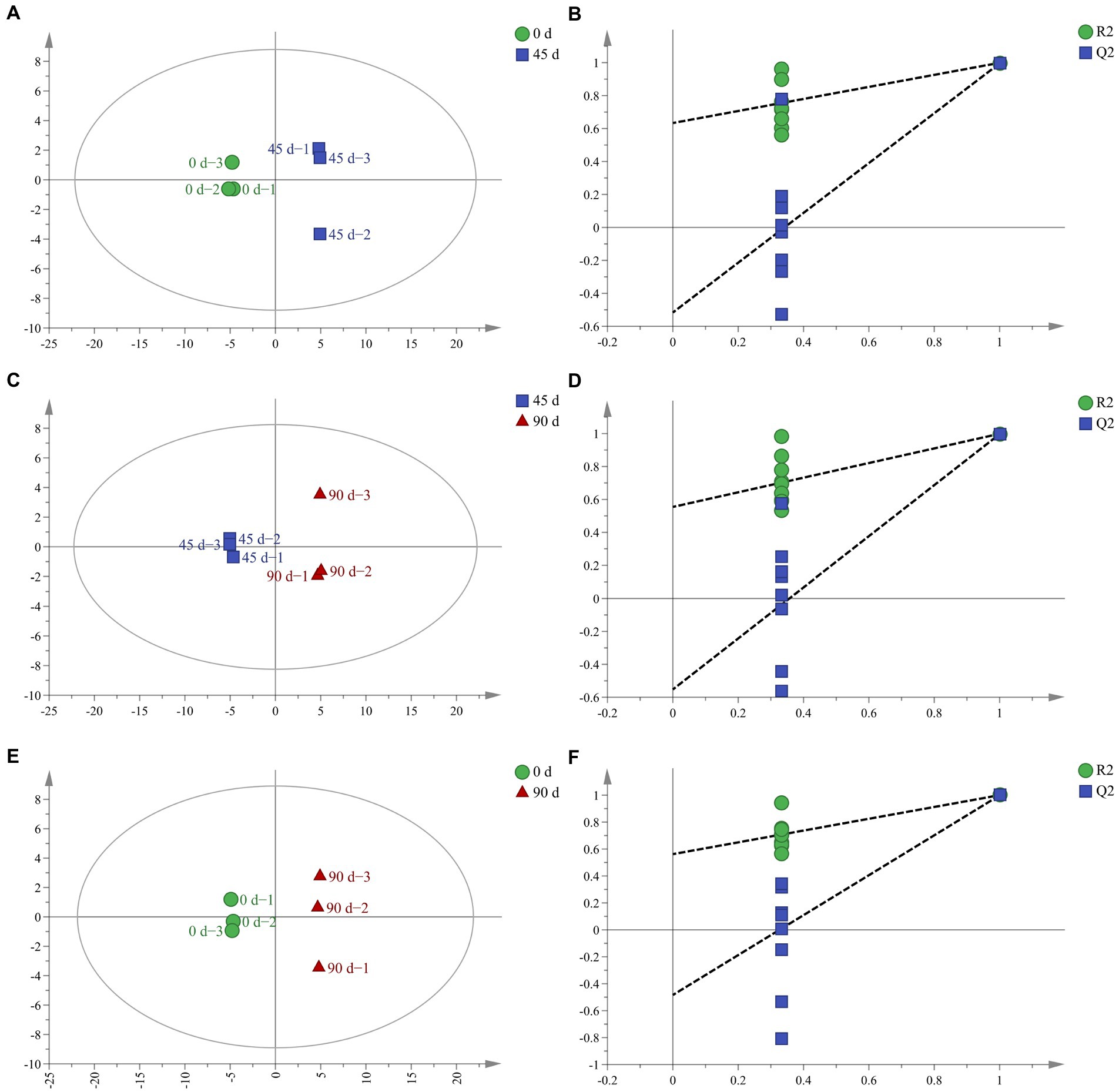
Figure 2. The OPLS−DA score and permutation test for LSCKs fermented for 0, 45, and 90 days. (A,C,E) Respectively, indicates the OPLS−DA analyses for A1 (0–45 days), A2 (45–90 days), and A3 (0–90 days); (B,D,F), respectively, represents permutation tests for A1 (0–45 days), A2 (45–90 days), and A3 (0–90 days).
3.3. Screening of differential metabolites in LSCKs
In the present study, the metabolites with VIP > 1, p < 0.05, and |log2FC| ≥ 1.1 were determined as the differential metabolites in the LSCKs. The volcano scatter plot was drawn to qualitatively analyze the differential metabolites, as shown in Figure 3. Twenty-one differential metabolites were identified in group A1 (0–45 days), with 19 metabolites up−regulated and 2 metabolites down-regulated. There were 14 differential metabolites in group A2 (45–90 days), with seven metabolites up-regulated and seven metabolites down-regulated. In group A3 (0–90 days), a total of 15 differential metabolites were screened, with 10 metabolites up−regulated and 5 metabolites down−regulated. Most of the differential metabolites were carbohydrates, sugar alcohols, amino acids and their derivatives, as listed in Supplementary Table S2. After 45 d fermentation, the sweet flavor substances in LSCKs gradually accumulated, including 2,3-butanediol, L-glutamine, D-arabitol, and L-rhamnose. L-pyroglutamic acid was the only one substance continuously decreased during the 90 days fermentation. However, the contents of 9 metabolites including L-glutamine, L-ornithine, β-D-fructose, α-D-glucose, D-arabinose, D-gluconic acid, glycine, L-proline and guanine continuously increased from the beginning to the end. In order to better evaluate the metabolic patterns of the main differential metabolites in LSCK, the relative contents of the 36 differential metabolites in LSCK were further analyzed by hierarchical clustering analysis (HCA) and displayed in the form of a heat map, as shown in Figure 4.
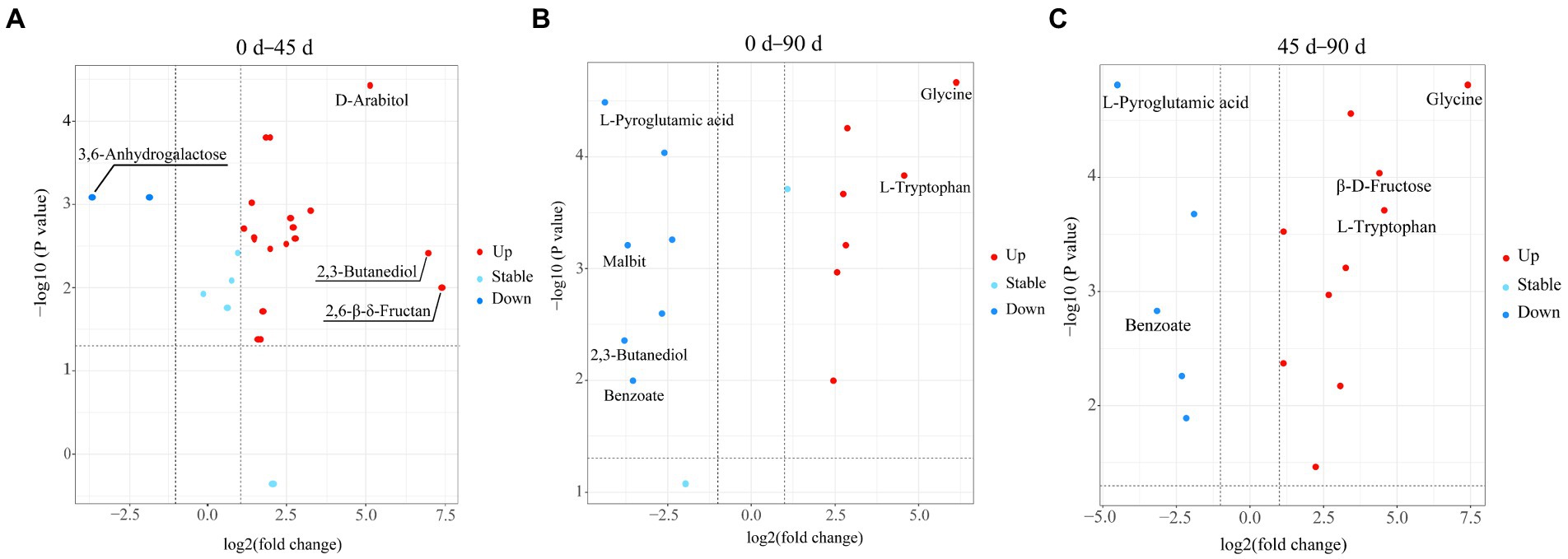
Figure 3. Volcano diagram of the differential metabolites in LSCKs fermented for 0, 45, and 90 days (A–C), respectively, represents group A1 (0–45 days), A2 (45–90 days), and A3 (0–90 days); blue and red, respectively, indicates the down−regulated and up−regulated differential metabolites.
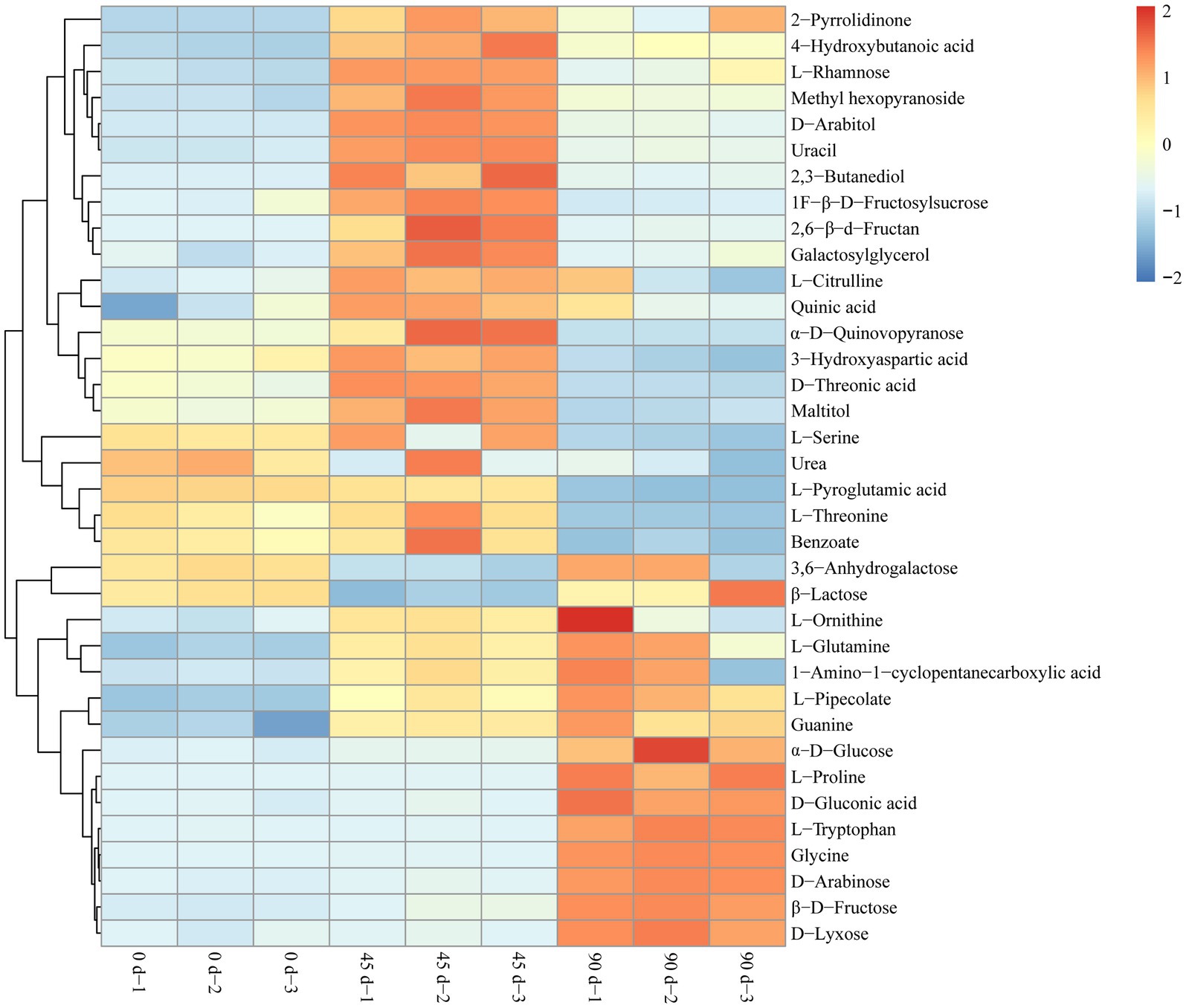
Figure 4. Hierarchical clustering analysis heat map of the main differential metabolites in LSCKs fermented for 0, 45, and 90 days.
3.4. Interaction analysis of the differential metabolites in LSCKs
The interactions between the differential metabolites are shown in Figure 5. Carbohydrates had a significant positive correlation with the metabolism of amino acids, such as α-D-quinovopyranose, which involved in aldose metabolism, exhibiting a significantly strong positive correlation with D-threonic acid (R = 0.929, p < 0.01). Similarly, galactosylglycerol positively correlated with D-threonic acid (R = 0.885, p < 0.01). On the other hand, β-D-fructose showed a significantly positive correlation with glycine (R = 0.993, p < 0.01) and L-proline (R = 0.981, p < 0.01). In this study, 10 carbohydrates including L-rhamnose, α-D-quinovopyranose, 3,6-anhydrogalactose, β-D-fructose, β-lactose, 1F-β-D-fructosylsucrose, 2,6-β-delta−fructan, D-lyxose, α-D-glucose, and D-arabinose were detected. α-D-glucose and D-fructose involved in galactose metabolism and amino sugar and nucleotide sugar metabolism, and their expression levels showed a significant positive correlation (R = 0.973, p < 0.01).
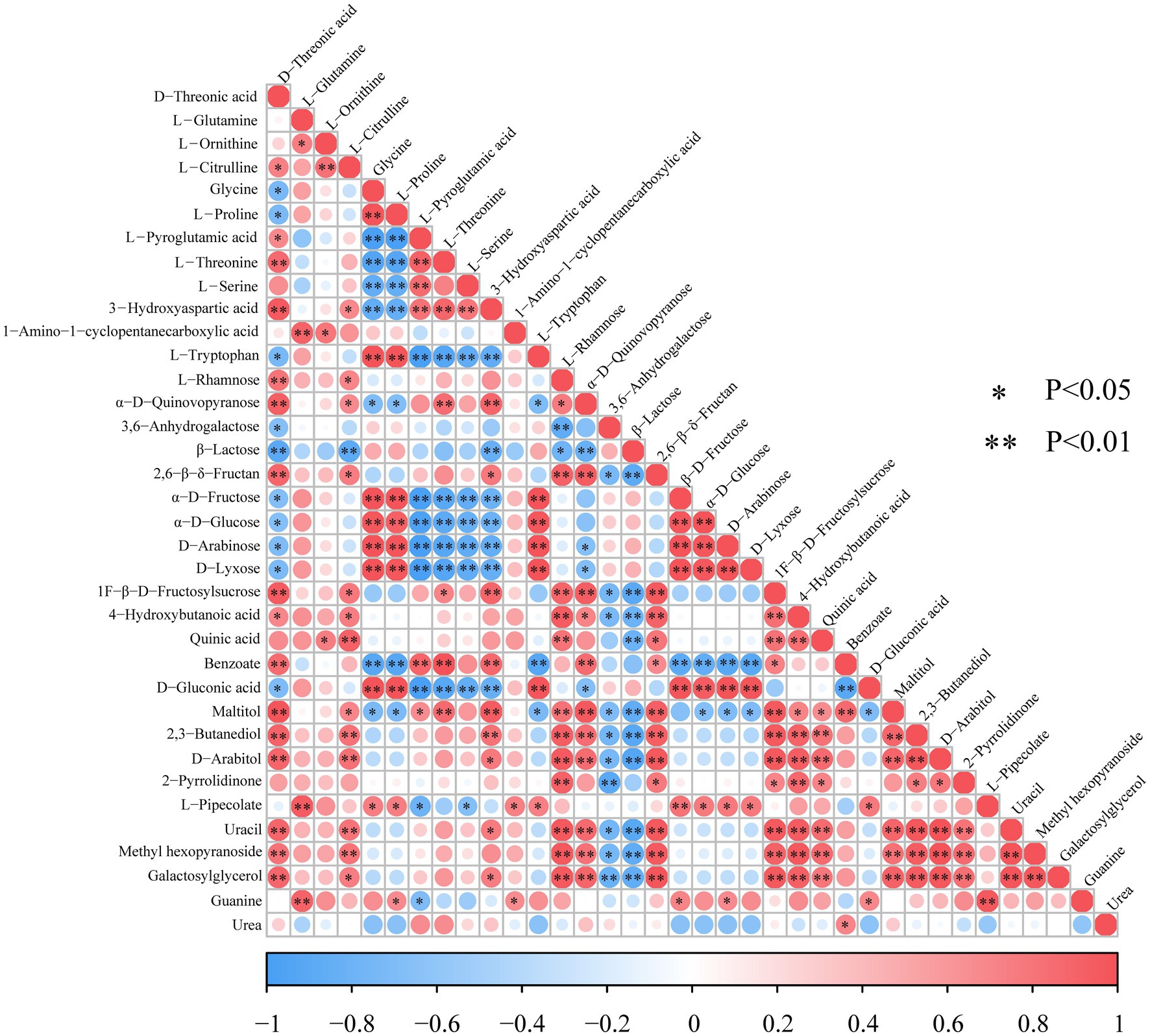
Figure 5. Heat map of differential metabolite correlations in LSCKs. Red and blue color, respectively, indicates a positive and negative correlation between the expression of the two metabolites. * and ** Indicates significant (p < 0.05) and highly significant differences, respectively (p < 0.01).
Amino acids are used to biosynthesize proteins, peptides and other nitrogen−containing substances, such as melanin and creatine (Da Silva, 2022). Most of the amino acids identified in this study were glycogenic amino acids, mainly including glycine, L-proline, L-threonine, L-serine, and L-tryptophan. Except for L-proline, all the amino acids can generate pyruvate or the intermediates involved in tricarboxylic acid cycle to participate in carbohydrates, lipids and fats metabolisms. By comparing the changing trends between amino acids, strong positive correlations between glycine and L-tryptophan (R = 0.999, p < 0.01), L-threonine and L-serine (R = 0.722, p < 0.05) were demonstrated. In addition, sugar alcohols including D-arabitol and D-sorbitol were also identified, which could be used to avoid the change of the cell membrane from liquid to gel at low temperature by increasing the fluidity of the cell membrane (Song et al., 2017).
3.5. Metabolite pathway analysis for the differential metabolites
Based on the selected 36 differential metabolites, a general overview of the pathways of the differential metabolite enrichment was shown in Figure 6. Twenty−eight metabolic pathways were enriched, whereas only two pathways had topological analysis results with p-value<0.05 and impact value >0.1, namely aminoacyl−tRNA biosynthesis and glycine, serine and threonine metabolism. The fermentation period strongly affected the accumulation of metabolites in LSCKs. Amino acids and their derivatives were the dominant metabolites involved in these pathways. L-serine, glycine, L-threonine, and L-tryptophan were generally involved in the two enriched metabolic pathways. In addition, glycine, serine and threonine metabolism was the most significant differential metabolite pathway in LSCKs, which associated with L-glutamine, glycine, L-serine, L-threonine, L-tryptophan, and L-proline.
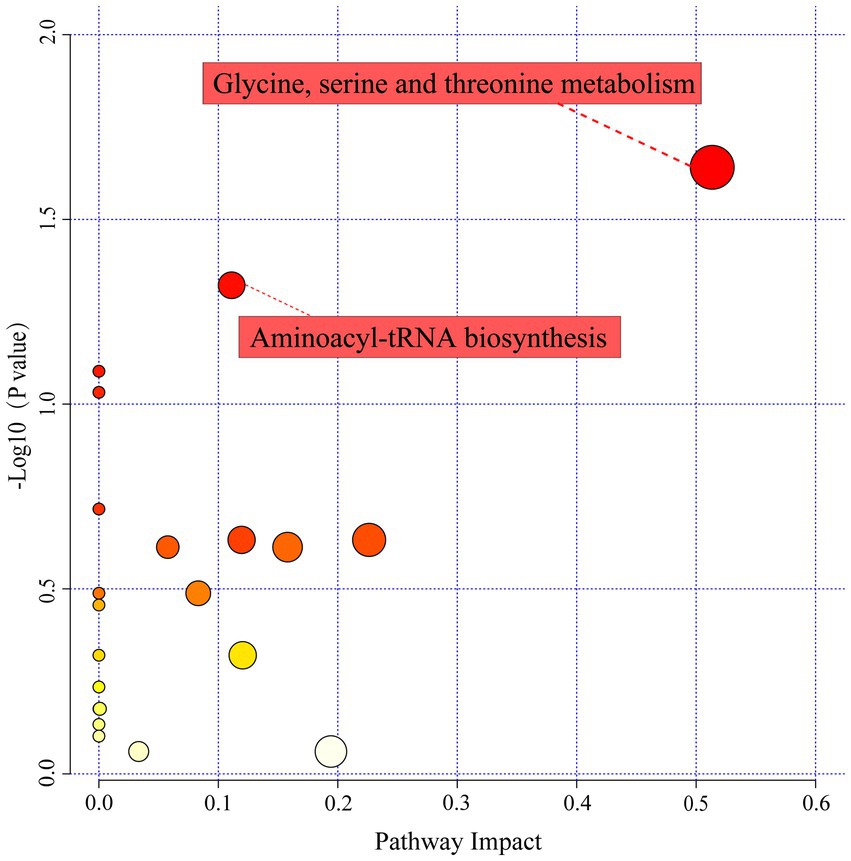
Figure 6. Influencing factors of metabolic pathways. A larger circle means a larger impact factor, and vice versa a smaller impact factor. The redder the color of the circle, the smaller the p-value.
3.6. E-nose analysis for LSCK
The overall odor profile for LSCK was drawn, as shown in Figure 7A. It can be seen that changes for nitrogen oxides, amine compounds and propane compounds were not significantly. However, ketones, alcohols, toluene and methane significantly increased along with the fermentation, which was in agreement with the metabolite analysis, as the 2,3 − butanediol continuously increased. The PCA analysis for E − nose result was performed, as revealed in Figure 7B. The cumulative contribution rate for the first two principal components was 99.51% (PC1 97.90% and PC2 1.61%), indicating that the established PCA model reflected most of the information. The 45 and 90 days fermented LSCKs distributed on the right side of the Y axis, while the 0 days fermented LSCK located on the left side of the Y axis, suggesting that the metabolism was stronger in the 0–45 days fermentation stage than that in the 45–90 days fermentation stage. The metabolites in the 90 days fermented LSCK significantly differed from those in the 0 and 45 days fermented LSCKs, which was consistent with the sensor response intensity in the radar map. The response value of LSCK fermented for 90 days was higher than those of 0 and 45 days fermented LSCKs, indicating that the major flavor of LSCK was mainly synthesized in 0–45 days.
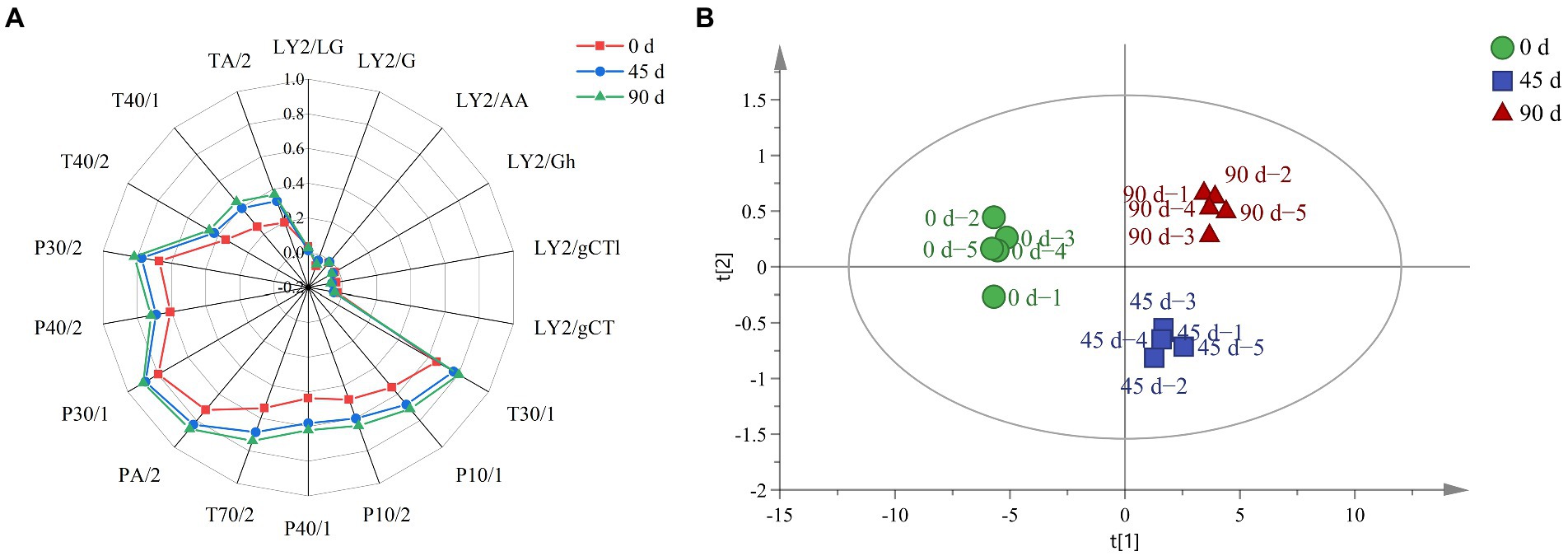
Figure 7. E-nose for LSCKs fermented for 0, 45, and 90 days. (A) Radar graph of the LSCK. Red, blue, and green indicates 0, 45, and 90 days fermented LSCKs, respectively. (B) PCA plot of the 0, 45, and 90 days fermented LSCKs.
4. Discussion
The physicochemical properties of LSCKs significantly changed along with the fermentation from 0 to 90 days. Sourness is the main taste of fermented vegetables, and total acid is one of the most important indicators for judging the sourness of fermented vegetables (Shang et al., 2022). The lactic acid bacteria cells significantly increased with the extension of low-salt fermentation at low temperature, which contributed to acid production in the first 45 days fermentation. After the 45 days fermentation, the number of lactic acid bacteria cells increased slightly (p > 0.05). Microorganisms such as Enterobacteriaceae can also use the soluble sugar in LSCK to produce acid during fermentation (Di Cagno et al., 2013). Proteases produced by microorganisms decomposed proteins, therefore the content of amino acid nitrogen increased, while the protein content decreased. Lee et al. (2012) found that the lactic acid bacteria Weissella koreensis isolated from Korean kimchi effectively decomposed proteins to produce amino acids such as β-aminobutyric acid and ornithine.
During fermentation, microorganisms can use the nutrients in kohlrabi to produce metabolites through various metabolic modes including lipid metabolism, amino acid metabolism and carbohydrate metabolism (Yang et al., 2020). Temperature and salt are major factors that affect the diversity and metabolism of microorganisms, possessing an important impact on the formation of metabolites in foods (Zhao et al., 2021; Mi et al., 2022). During low-temperature and low-salt fermentation, carbohydrates, amino acids and their derivatives including L-glutamine, L-ornithine, β-D-fructose, α-D-glucose, D-arabinose, D-gluconic acid, glycine, L-proline and guanine continuously increased, which agreed to Liu’s experimental results on mustard’s flavor metabolites (Liu D. Q. et al., 2021). The accumulation of flavor amino acids, such as glycine and aspartic acid, made the LSCKs more and more tasty along with the fermentation. L-serine, glycine, L-threonine, and L-tryptophan were mainly related to glycine, serine and threonine metabolism and aminoacyl−tRNA biosynthesis. L-pyroglutamic acid is an amino acid derivative produced by the cyclization of L-glutamic acid, which is widespread in animal brain tissue and plant tissues (Bachhawat, 2011). The decrease in L-pyroglutamic acid might be due to the activation of 5-hydroxyprolinase, which promoted the conversion of L-pyroglutamic acid into glutamic acid (Killiny et al., 2018). However, under low temperature condition, the activity of 5-hydroxyprolinase decreased. Therefore, the increase of glutamate was not significant (p > 0.05), which was thus not identified as a differential metabolite in this work.
In the process of glucose metabolism, mannitol and glycerol are important stress molecules when kohlrabi fermented at low temperature (Wang et al., 2017). Glycerol and mannitol generated into pyruvate and fructose 6 − phosphate by glycerol kinase and glycerol dehydrogenase, which subsequently participated in the glycolytic pathway (Wang Z. et al., 2022). Glucose interacts with phosphoenolpyruvate and 4-phosphoerythrose pyruvate to produce quinic acid. With the extension of the low temperature fermentation, the kohlrabi was gradually stressed by the lower temperature, and a variety of secondary metabolites were thereby synthesized to fight against the threat of the environment (Bordenave et al., 2013). In this study, it was easy to understand why the content of quinic acid increased during the first 45 days fermentation. 2,3-Butanediol was the most significantly up−regulated differential metabolite in A1 (FC = 123.622), which belonged to the anaerobic fermentation of glucose. Glucose generates pyruvate through the Embden−Meyerhof pathway (Verhees et al., 2003). Under the synergism of some Erwinia, Enterobacter and Serratia, two molecules of pyruvates interact with one molecule of NADH produce two molecules of CO2 and one molecule of 2,3-butanediol (Reshamwala et al., 2017). In addition, D-arabitol was also the differential substance in group A1 (FC = 34.237), which was a sugar alcohol generated from D-arabinitol 4-dehydrogenase through the pentose phosphate pathway (Marks, 1956). Glycine was the most significantly up−regulated differential metabolite in group A2 (FCA2 = 69.896) and A3 (FCA3 = 169.239), mainly produced by threonine metabolism through the catalysis of aldolase and threoninase (Darling et al., 2000). Glycine also associates with plant antioxidant properties (He et al., 2022) and affects energy metabolism by regulating γ-aminobutyric acid metabolism (Liu and von Wirén, 2017). Generally, glycine made the fermented vegetables with sweetness characteristics during fermentation, which would be increased when Lactobacillus (L.) plantarum SCYAPCZN11–1, L. buchneri SCYAPCZN11–4 and Pediococcus ethanoliduran SCYAPCZN11–8 worked together (Zhao et al., 2016). With the fermentation extended, the D − gluconic acid gradually increased. It has been well known that D-gluconic acid is widespread in fruits and honey (Sainz et al., 2016), which is also detected in ultra−long−term fermented Chinese kohlrabi as described in our previous study (Nie et al., 2022). So, it is interesting to use LSCK as a source for producing D-gluconic acid, which has the potential to replace the commercial production by microorganisms such as Aspergillus niger in the future (Bauer et al., 2022).
In the present study, the LSCKs were processed under low-temperature and low-salt condition. Low-temperature increases the water retention capacity of cells, induces changes in soluble carbohydrates content, leads to the gradual accumulation of carbohydrates in plants (González et al., 2009). Furthermore, the intracellular NADPH/NADP ratio and ATP content increased, which provided sufficient reducing power and energy for carbohydrates anabolism (Savitch et al., 2010). Previous studies have suggested that free carbohydrates can be rapidly consumed to produce glycerol and ethanol by yeast and lactic acid bacteria during fermentation (Chen et al., 2017). Moreover, free carbohydrates can be hydrolyzed to generate monosaccharides and release energy to promote various biochemical reactions (Jeong et al., 2013). The changes in carbohydrate content probably be caused by various environmental stresses. It has been reported that mannitol metabolism possibly plays a key role in plant responses to stress (Lau et al., 2020). α-D-glucose and D-fructose, respectively, produced from glycolysis and fructose and mannose metabolism (Guo et al., 2022), indicating a significant synergy between glycolysis and fructose and mannose metabolism during the fermentation of LSCK under low temperature. In addition, carbohydrate is considered as an important component of signal pathway, connecting and constructing pathway network to control plant metabolic response. Sami et al. (2016) suggested that carbohydrate could induce stress response and increase plant resistance to abiotic stress.
The main function of amino acids is to form proteins, and also to synthesize polypeptides and other nitrogen-containing substances, such as melanin, creatine (Jeon et al., 2021; Da Silva, 2022). Free amino acid is an important kind of differential metabolite in fermented kohlrabi, which contributes greatly to the flavor of fermented foods. For example, D-threonic acid, glycine, and L-serine have sweet taste, while L-citrulline, L-proline, and L-tryptophan show bitter taste. The free amino acid not only directly affecting food taste, but also provides fermented vegetables with unique color, aroma and taste, considered as a precursor of volatile flavor. Amino acid catabolism can lead to the formation of a wide range of flavor substances, such as aldehydes, esters, acids, alcohols, ether, nitrogen−containing compounds, pyrazines and pyridines. Free amino acid can be converted into aromatic substances by lactic acid bacteria during differentiation (Xiao et al., 2020). It has been documented that L. sakei can generate aroma−active volatile substances including aldehydes, alcohols and carboxylic acids from α-keto acids by transamination of free amino acid (Gutsche et al., 2012). Furthermore, various species of Lactobacillus are well−known contributors to the formation of volatile aroma compounds in cheeses by catabolizing proteins to amino acids and subsequent conversions (Peralta et al., 2014).
Metabolites including L-glutamine, glycine, L-serine, L-threonine, L-tryptophan, and L-proline were closely related to aminoacyl−tRNA biosynthesis. L-glutamine exists in plants, animals and other organisms. As a component of proteins, L-glutamine involved in regulation of immunity and cell regulation (Sevastos et al., 2018). L-serine is a non-essential amino acid, which is an important precursor for the synthesis of phospholipids (Cao et al., 2018), promoting the flowering of plants (Tanaka et al., 1997). It has been demonstrated that tryptophan plays an important role in salt stress of highland barley in Tibet (Wang et al., 2019). Glycine is often used as a nutritional supplement for flavoring. L-glutamine and glycine continuously increased along with the fermentation, and the metabolism of glyoxylic acid and dicarboxylic acid consequently increased. Additionally, with the increase of L-glutamine, glycine and L-proline, the biosynthesis of aminoacyl−tRNA enhanced. The changes of these flavor precursors may have an impact on the flavor of LSCK. The relationship between the precursor flavor substances and the flavor of LSCK is complex and needs to be further elucidated.
The differential metabolites including 1 − octanol, 2 − pyrrolidinone and ethanolamine agreed well with the overall odor profile for LSCK, as shown in Figure 7. Metabolic complementation among different microorganisms and interactions between metabolites during fermentation increased the content of volatile substances and promoted the production of new volatile substances. For example, co − inoculation of L. plantarum and Pediococcus pentosus increased the content of alcohols, aldehydes and esters (Da Silva, 2022). The ketones and alcohols substances contents in LSCK increased with the fermentation extended, which was consistent with the metabolites changes in pickled radish (Di Cagno et al., 2013).
5. Conclusion
In the present work, GC-TOF-MS and multivariate statistics analyses were used to analyze the LSCK metabolites changes during low-temperature and low-salt fermentation for different periods. The LSCK with longer fermentation period had higher total acid content and lactic acid bacteria cells, while the contents of amino acid nitrogen, protein and reducing sugar were higher in LSCK with shorter fermentation period. Carbohydrates, sugar alcohols, and amino acids and their derivatives were considered as the main differential metabolites of LSCK. There was a significant correlation within the carbohydrate metabolism and amino acid metabolism. In addition, glycine, serine and threonine metabolism and aminoacyl−tRNA biosynthesis pathways were the most significant pathways closely correlated with the differential metabolites, as analyzed by KEGG. In the future, further experiments should be performed to precisely demonstrate what compounds are derived from the microbial fermentation. Moreover, it is necessary to use liquid chromatography–tandem mass spectrometry (LC–MS/MS) to analyze the non-volatile metabolites of the LSCKs during fermentation. The present work provides a theoretical support for optimization of LSCK production procedure.
Data availability statement
The original contributions presented in the study are included in the article/Supplementary material, further inquiries can be directed to the corresponding author.
Author contributions
ZZ: conceptualization, formal analysis, methodology, and writing – original draft. HC: data curation, formal analysis, methodology, and writing – original draft. YZ: formal analysis and writing – original draft. XN: conceptualization, data curation, formal analysis, funding acquisition, methodology, project administration, validation, visualization, and writing – review and editing. LX: writing – review and editing. TP: writing – original draft. DL, HL, and AW: formal analysis. All authors have read and agreed to the published version of the manuscript.
Funding
This work was supported by grants from the Science and Technology Department of Sichuan Province (2022NSFSC1702, 2021YFQ0072, 2021YJ0275) and Guangdong Province Key Laboratory of Marine Biotechnology (GPKLMB202104).
Conflict of interest
HL was employed by Sichuan Dimeng Technology Co., Ltd.
The remaining authors declare that the research was conducted in the absence of any commercial or financial relationships that could be construed as a potential conflict of interest.
Publisher’s note
All claims expressed in this article are solely those of the authors and do not necessarily represent those of their affiliated organizations, or those of the publisher, the editors and the reviewers. Any product that may be evaluated in this article, or claim that may be made by its manufacturer, is not guaranteed or endorsed by the publisher.
Supplementary material
The Supplementary material for this article can be found online at: https://www.frontiersin.org/articles/10.3389/fsufs.2023.1156173/full#supplementary-material
Footnotes
References
Bachhawat, A. (2011). Pyroglutamic acid: Throwing light on a lightly studied metabolite. Curr. Sci. 102, 288–297.
Bansal, V., and Mishra, S. K. (2020). Reduced-sodium cheeses: implications of reducing sodium chloride on cheese quality and safety. Compr. Rev. Food Sci. Food Saf. 19, 733–758. doi: 10.1111/1541−4337.12524
Bauer, J. A., Zámocká, M., Majtán, J., and Bauerová-Hlinková, V. (2022). Glucose oxidase, an enzyme "Ferrari": its structure, function, production and properties in the light of various industrial and biotechnological applications. Biomol. Ther. 12:472. doi: 10.3390/biom12030472
Benjakul, S., Seymour, T. A., Morrissey, M. T., and An, H. (1997). Physicochemical changes in Pacific whiting muscle proteins during iced storage. J. Food Sci. 62, 729–733. doi: 10.1111/j.1365−2621.1997.tb15445.x
Bordenave, C. D., Escaray, F. J., Menendez, A. B., Serna, E., Carrasco, P., Ruiz, O. A., et al. (2013). Defense responses in two ecotypes of Lotus japonicus against non-pathogenic Pseudomonas syringae. PLoS One 8:e83199. doi: 10.1371/journal.pone.0083199
Cao, K., Liu, Y., Tian, Y., Zhang, Q., Cong, P., Li, H., et al. (2018). Reaction specificity of phospholipase D prepared from Acinetobacter radioresistens a2 in transphosphatidylation. Lipids 53, 517–526. doi: 10.1002/lipd.12057
Chen, G., Chen, C., and Lei, Z. (2017). Meta-omics insights in the microbial community profiling and functional characterization of fermented foods. Trends Food Sci. Technol. 65, 23–31. doi: 10.1016/J.TIFS.2017.05.002
Chen, H., Nie, X., Peng, T., Xiang, L., Liu, D., Luo, H., et al. (2023). Effects of low-temperature and low-salt fermentation on the physicochemical properties and volatile flavor substances of Chinese kohlrabi using gas chromatography-ion mobility spectrometry. Fermentation 9:146. doi: 10.3390/fermentation9020146
Da Silva, R. P. (2022). The dietary supplement creatyl-l-leucine does not bioaccumulate in muscle, brain or plasma and is not a significant bioavailable source of creatine. Nutrients 14:701. doi: 10.3390/nu14030701
Darling, P. B., Grunow, J., Rafii, M., Brookes, S., Ball, R. O., and Pencharz, P. B. (2000). Threonine dehydrogenase is a minor degradative pathway of threonine catabolism in adult humans. Am. J. Physiol. Endocrinol. Metab. 278, E877–E884. doi: 10.1152/ajpendo.2000.278.5.E877
Di Cagno, R., Coda, R., De Angelis, M., and Gobbetti, M. (2013). Exploitation of vegetables and fruits through lactic acid fermentation. Food Microbiol. 33, 1–10. doi: 10.1016/j.fm.2012.09.003
Ding, M., Fan, J. L., Huang, D. F., Jiang, Y., Li, M. N., Zheng, Y. Q., et al. (2022). From non-targeted to targeted GC-MS metabolomics strategy for identification of TCM preparations containing natural and artificial musk. Chin. Med. 17:41. doi: 10.1186/s13020-022-00594-8
Fu, T., Feng, Y., Zhang, L., Li, X., and Wang, C. (2019). Metabonomics study on rice from different geographical areas based on gas chromatography-mass spectrometry. Food Sci. 40, 176–181. doi: 10.7506/spkx1002-6630-20180621-412
González, M. V., Sadras, V. O., Equiza, M. A., and Tognetti, J. A. (2009). Suboptimal temperature favors reserve formation in biennial carrot (Daucus carota) plants. Physiol. Plant. 137, 10–21. doi: 10.1111/j.1399−3054.2009.01247.x
Guo, X. M., Luo, T., Han, D. M., Zhu, D. F., Li, Z. Y., Wu, Z. Y., et al. (2022). Multi-omics analysis revealed room temperature storage affected the quality of litchi by altering carbohydrate metabolism. Sci. Hortic. 293:110663. doi: 10.1016/j.scienta.2021.110663
Gutsche, K. A., Tran, T. B. T., and Vogel, R. F. (2012). Production of volatile compounds by lactobacillus sakei from branched chain α-keto acids. Food Microbiol. 29, 224–228. doi: 10.1016/j.fm.2011.06.010
He, Y., Wang, Y., Hu, C., Hu, W., Zhu, W., and Xu, N. (2022). Potential biomarkers of low-temperature tolerance in Ulva prolifera strains. J. Appl. Phycol. 34, 1099–1108. doi: 10.1007/s10811−021−02666−y
Hossain, M. I., Mizan, M. F. R., Ashrafudoulla, M., Nahar, S., Joo, H.-J., Jahid, I. K., et al. (2020). Inhibitory effects of probiotic potential lactic acid bacteria isolated from kimchi against listeria monocytogenes biofilm on lettuce, stainless-steel surfaces, and MBEC™ biofilm device. LWT 118:108864. doi: 10.1016/j.lwt.2019.108864
Hu, Y., Zhang, L., Badar, I. H., Liu, Q., Liu, H., Chen, Q., et al. (2022). Insights into the flavor perception and enhancement of sodium-reduced fermented foods: a review. Crit. Rev. Food Sci. Nutr., 1–15. doi: 10.1080/10408398.2022.2121909
Jeon, J. S., Kang, H. M., Park, J. H., Kang, J. S., Lee, Y. J., Park, Y. H., et al. (2021). A comparative study on photo-protective and anti-Melanogenic properties of different Kadsura coccinea extracts. Plan. Theory 10:1633. doi: 10.3390/plants10081633
Jeong, S. H., Lee, S. H., Jung, J. Y., Choi, E. J., and Jeon, C. O. (2013). Microbial succession and metabolite changes during long-term storage of Kimchi. J. Food Sci. 78, M763–M769. doi: 10.1111/1750−3841.12095
Killiny, N., Nehela, Y., Hijaz, F., and Vincent, C. I. (2018). A plant pathogenic bacterium exploits the tricarboxylic acid cycle metabolic pathway of its insect vector. Virulence 9, 99–109. doi: 10.1080/21505594.2017.1339008
Kim, J., Choi, K. B., Park, J. H., and Kim, K. H. (2019). Metabolite profile changes and increased antioxidative and antiinflammatory activities of mixed vegetables after fermentation by lactobacillus plantarum. PLoS One 14:e0217180. doi: 10.1371/journal.pone.0217180
Kim, J., Jung, Y., Song, B., Bong, Y.-S., Ryu, D. H., Lee, K.-S., et al. (2013). Discrimination of cabbage (Brassica rapa ssp. pekinensis) cultivars grown in different geographical areas using 1H NMR-based metabolomics. Food Chem. 137, 68–75. doi: 10.1016/j.foodchem.2012.10.012
Kumar Verma, D., Al-Sahlany S, T. G., Kareem Niamah, A., Thakur, M., Shah, N., Singh, S., et al. (2022). Recent trends in microbial flavour compounds: a review on chemistry, synthesis mechanism and their application in food. Saudi J. Biol. Sci. 29, 1565–1576. doi: 10.1016/j.sjbs.2021.11.010
Lau, H., Laserna, A. K. C., and Li, S. F. Y. (2020). 1H NMR-based metabolomics for the discrimination of celery (Apium graveolens L. var. dulce) from different geographical origins. Food Chem. 332:127424. doi: 10.1016/j.foodchem.2020.127424
Lee, J. H., Bae, J. W., and Chun, J. (2012). Draft genome sequence of Weissella koreensis KCTC 3621T. J. Bacteriol. 194, 5711–5712. doi: 10.1128/JB.01356−12
Lee, K. B., Kim, Y. J., Kim, H. J., Choi, J., and Kim, J. K. (2018). Phytochemical profiles of Brassicaceae vegetables and their multivariate characterization using chemometrics. Appl. Biol. Chem. 61, 131–144. doi: 10.1007/s13765−017−0340−6
Lin, X., Tang, Y., Hu, Y., Lu, Y., Sun, Q., Lv, Y., et al. (2021). Sodium reduction in traditional fermented foods: challenges, strategies, and perspectives. J. Agric. Food Chem. 69, 8065–8080. doi: 10.1021/acs.jafc.1c01687
Liu, Y., Mei, B., Chen, D., and Cai, L. (2021). GC-MS metabolomics identifies novel biomarkers to distinguish tuberculosis pleural effusion from malignant pleural effusion. J. Clin. Lab. Anal. 35:e23706. doi: 10.1002/jcla.23706
Liu, Y., and Von Wirén, N. (2017). Ammonium as a signal for physiological and morphological responses in plants. J. Exp. Bot. 68, 2581–2592. doi: 10.1093/jxb/erx086
Liu, D. Q., Zhang, C. C., Zhang, J. M., Xin, X. T., and Liao, X. J. (2021). Metagenomics reveals the formation mechanism of flavor metabolites during the spontaneous fermentation of potherb mustard (Brassica juncea var. multiceps). Food Res. Int. 148:110622. doi: 10.1016/j.foodres.2021.110622
Marks, P. A. (1956). A newer pathway of carbohydrate metabolism; the pentose phosphate pathway. Diabetes 5, 276–283. doi: 10.2337/diab.5.4.276
Mi, T., Wang, D., Yao, S., Yang, H., Che, Y., and Wu, C. (2022). Effects of salt concentration on the quality and microbial diversity of spontaneously fermented radish paocai. Food Res. Int. 160:111622. doi: 10.1016/j.foodres.2022.111622
Mu, Y., Su, W., Yu, X., Mu, Y., Jiang, L., and Wang, H.-L. (2019). Untargeted metabolomics based on GC-TOF-MS reveals the optimal pre-fermentation time for black glutinous rice wine. Int. J. Food Prop. 22, 2033–2046. doi: 10.1080/10942912.2019.1705481
Nie, X., Chen, H., Xiang, L., Zhang, Y., Liu, D., and Zhao, Z. (2022). GC-TOF-MS-based non-targeted metabolomic analysis of differential metabolites in Chinese ultra-long-term industrially fermented kohlrabi and their associated metabolic pathways. Meta 12:991. doi: 10.3390/metabo12100991
Nissen, L., Casciano, F., Babini, E., and Gianotti, A. (2021a). Prebiotic potential and bioactive volatiles of hemp byproduct fermented by lactobacilli. LWT 151:112201. doi: 10.1016/j.lwt.2021.112201
Nissen, L., Casciano, F., and Gianotti, A. (2021b). Volatilome changes during probiotic fermentation of combined soy and rice drinks. Food Funct. 12, 3159–3169. doi: 10.1039/d0fo03337e
Pasko, P., Galanty, A., Tyszka-Czochara, M., Zmudzki, P., Zagrodzki, P., Gdula-Argasinska, J., et al. (2021). Health promoting vs anti-nutritive aspects of kohlrabi sprouts, a promising candidate for novel functional food. Plant Foods Hum. Nutr. 76, 76–82. doi: 10.1007/s11130−020−00877−1
Peralta, G. H., Wolf, I. V., Bergamini, C. V., Perotti, M. C., and Hynes, E. R. (2014). Evaluation of volatile compounds produced by lactobacillus paracasei I90 in a hard-cooked cheese model using solid-phase microextraction. Dairy Sci. Technol. 94, 73–81. doi: 10.1007/S13594−013−0143−4
Reshamwala, S. M. S., Deb, S. S., and Lali, A. M. (2017). A shortened, two-enzyme pathway for 2,3-butanediol production in Escherichia coli. J. Ind. Microbiol. Biotechnol. 44, 1273–1277. doi: 10.1007/s10295−017−1957−5
Rubel Mozumder, N. H. M., Lee, Y.-R., Hwang, K.-H., Lee, M.-S., Kim, E.-H., and Hong, Y.-S. (2020). Characterization of tea leaf metabolites dependent on tea (Camellia sinensis) plant age through 1H NMR-based metabolomics. Appl. Biol. Chem. 63:10. doi: 10.1186/s13765-020-0492-7
Sainz, F., Navarro, D., Mateo, E., Torija, M. J., and Mas, A. (2016). Comparison of D-gluconic acid production in selected strains of acetic acid bacteria. Int. J. Food Microbiol. 222, 40–47. doi: 10.1016/j.ijfoodmicro.2016.01.015
Saleh, M. S. M., Siddiqui, M. J., Mediani, A., Ahmed, Q. U., Mat So'ad, S. Z., Saidi-Besbes, S., et al. (2020). Modulation of metabolic alterations of obese diabetic rats upon treatment with Salacca zalacca fruits extract using (1)H NMR-based metabolomics. Food Res. Int. 137:109547. doi: 10.1016/j.foodres.2020.109547
Sami, F., Yusuf, M., Faizan, M., Faraz, A., and Hayat, S. (2016). Role of sugars under abiotic stress. Plant Physiol. Biochem. 109, 54–61. doi: 10.1016/j.plaphy.2016.09.005
Savitch, L. V., Harney, T., and Huner, N. (2010). Sucrose metabolism in spring and winter wheat in response to high irradiance, cold stress and cold acclimation. Physiol. Plant. 108, 270–278. doi: 10.1034/j.1399−3054.2000.108003270.x
Sevastos, A., Kalampokis, I. F., Panagiotopoulou, A., Pelecanou, M., and Aliferis, K. A. (2018). Implication of Fusarium graminearum primary metabolism in its resistance to benzimidazole fungicides as revealed by (1)H NMR metabolomics. Pestic. Biochem. Physiol. 148, 50–61. doi: 10.1016/j.pestbp.2018.03.015
Sha, S. P., Jani, K., Sharma, A., Anupma, A., Pradhan, P., Shouche, Y., et al. (2017). Analysis of bacterial and fungal communities in Marcha and Thiat, traditionally prepared amylolytic starters of India. Sci. Rep. 7:10967. doi: 10.1038/s41598-017-11609-y
Shang, Z., Ye, Z., Li, M., Ren, H., Cai, S., Hu, X., et al. (2022). Dynamics of microbial communities, flavor, and physicochemical properties of pickled chayote during an industrial-scale natural fermentation: correlation between microorganisms and metabolites. Food Chem. 377:132004. doi: 10.1016/j.foodchem.2021.132004
Singh, D., Lee, S., and Lee, C. H. (2017). Metabolomics for empirical delineation of the traditional Korean fermented foods and beverages. Trends Food Sci. Technol. 61, 103–115. doi: 10.1016/J.TIFS.2017.01.001
Skonberg, D. I., Fader, S., Perkins, L. B., and Perry, J. J. (2021). Lactic acid fermentation in the development of a seaweed sauerkraut-style product: microbiological, physicochemical, and sensory evaluation. J. Food Sci. 86, 334–342. doi: 10.1111/1750−3841.15602
Song, Y., Liu, L., Wei, Y., Li, G., Yue, X., and An, L. (2017). Metabolite profiling of adh1 mutant response to cold stress in Arabidopsis. Front. Plant Sci. 7:2072. doi: 10.3389/fpls.2016.02072
Suzuki-Iwashima, A., Matsuura, H., Iwasawa, A., and Shiota, M. (2020). Metabolomics analyses of the combined effects of lactic acid bacteria and Penicillium camemberti on the generation of volatile compounds in model mold-surface-ripened cheeses. J. Biosci. Bioeng. 129, 333–347. doi: 10.1016/j.jbiosc.2019.09.005
Tanaka, O., Nakayama, Y., Emori, K., Takeba, G., Sato, K., and Sugino, M. (1997). Flower-inducing activity of lysine in Lemna paucicostata 6746. Plant Cell Physiol. 38, 124–128. doi: 10.1093/oxfordjournals.pcp.a029141
Verhees, C. H., Kengen, S. W., Tuininga, J. E., Schut, G. J., Adams, M. W., de Vos, W. M., et al. (2003). The unique features of glycolytic pathways in Archaea. Biochem. J. 375, 231–246. doi: 10.1042/BJ20021472
Wang, D., Chen, G., Tang, Y., Li, J., Huang, R., Ye, M., et al. (2022). Correlation between autochthonous microbial communities and flavor profiles during the fermentation of mustard green paocai (Brassica juncea Coss.), a typical industrial-scaled salted fermented vegetable. LWT 172:114212. doi: 10.1016/j.lwt.2022.114212
Wang, J., Ying, S. H., Hu, Y., and Feng, M. G. (2017). Vital role for the J-domain protein Mdj1 in asexual development, multiple stress tolerance, and virulence of Beauveria bassiana. Appl. Microbiol. Biotechnol. 101, 185–195. doi: 10.1007/s00253−016−7757−4
Wang, Y., Zeng, X., Xu, Q., Mei, X., Yuan, H., Jiabu, D., et al. (2019). Metabolite profiling in two contrasting Tibetan hulless barley cultivars revealed the core salt-responsive metabolome and key salt-tolerance biomarkers. AoB Plants 11:plz021. doi: 10.1093/aobpla/plz021
Wang, Z., Zhao, Y., Chen, D., Peng, J., Gao, J., Lin, Z., et al. (2022). Study on the differences of chemical components in different subtypes of Yunnan white tea based on metabolomics method. Food Sci. 43, 221–231. doi: 10.7506/spkx1002-6630-20220121-215
Want, E. J., Masson, P., Michopoulos, F., Wilson, I. D., Theodoridis, G., Plumb, R. S., et al. (2013). Global metabolic profiling of animal and human tissues via UPLC-MS. Nat. Protoc. 8, 17–32. doi: 10.1038/nprot.2012.135
Wen, R., Hu, Y., Zhang, L., Wang, Y., Chen, Q., and Kong, B. (2019). Effect of NaCl substitutes on lipid and protein oxidation and flavor development of Harbin dry sausage. Meat Sci. 156, 33–43. doi: 10.1016/j.meatsci.2019.05.011
Westerhuis, J. A., Hoefsloot, H. C. J., Smit, S., Vis, D. J., Smilde, A. K., Van Velzen, E. J. J., et al. (2008). Assessment of PLSDA cross validation. Metabolomics 4, 81–89. doi: 10.1007/s11306-007-0099-6
Xiao, Y., Huang, T., Huang, C., Hardie, J., Peng, Z., Xie, M., et al. (2020). The microbial communities and flavour compounds of Jiangxi yancai, Sichuan paocai and Dongbei suancai: three major types of traditional Chinese fermented vegetables. LWT 121:108865. doi: 10.1016/j.lwt.2019.108865
Xu, N., Zeng, X., Li, L., Zhang, X., Wang, P., Han, M., et al. (2023). Effects of post-mortem aging process on characteristic water-soluble taste-active precursors in yellow-feathered broilers. Food Sci. Human Wellness 12, 242–253. doi: 10.1016/j.fshw.2022.07.004
Yang, X., Hu, W., Xiu, Z., Jiang, A., Yang, X., Saren, G., et al. (2020). Microbial community dynamics and metabolome changes during spontaneous fermentation of northeast sauerkraut from different households. Front. Microbiol. 11:1878. doi: 10.3389/fmicb.2020.01878
Yang, K., Jian, S., Guo, D., Wen, C., Xin, Z., Zhang, L., et al. (2022). Fecal microbiota and metabolomics revealed the effect of long-term consumption of gallic acid on canine lipid metabolism and gut health. Food Chem X 15:100377. doi: 10.1016/j.fochx.2022.100377
Yilmaz, B., Bangar, S. P., Echegaray, N., Suri, S., Tomasevic, I., Manuel Lorenzo, J., et al. (2022). The impacts of Lactiplantibacillus plantarum on the functional properties of fermented foods: a review of current knowledge. Microorganisms 10:826. doi: 10.3390/microorganisms10040826
Zhang, Y., Chen, H., Zhao, Z., Wang, W., Nie, X., Cheng, J., et al. (2022). Analysis of differential metabolites and related metabolism pathways in long-term fermented kohlrabi fermented for different periods. Food Sci. 43, 192–198. doi: 10.7506/spkx1002-6630-20211220-236
Zhao, N., Lai, H., He, W., Wang, Y., Huang, Y., Zhao, M., et al. (2021). Reduction of biogenic amine and nitrite production in low-salt Paocai by controlled package during storage: a study comparing vacuum and aerobic package with conventional salt solution package. Food Control 123:107858. doi: 10.1016/j.foodcont.2020.107858
Zhao, N., Zhang, C., Yang, Q., Guo, Z., Yang, B., Lu, W., et al. (2016). Selection of taste markers related to lactic acid bacteria microflora metabolism for Chinese traditional Paocai: a gas chromatography-mass spectrometry-based metabolomics approach. J. Agric. Food Chem. 64, 2415–2422. doi: 10.1021/acs.jafc.5b05332
Keywords: low−salt fermentation, non-targeted metabolomic, GC-TOF-MS, differential metabolites, metabolic pathway, fermented Chinese kohlrabi
Citation: Zhao Z, Chen H, Zhang Y, Nie X, Xiang L, Peng T, Liu D, Luo H and Wang A (2023) Metabolites changes of a low-temperature and low-salt fermented Chinese kohlrabi during fermentation based on non-targeted metabolomic analysis. Front. Sustain. Food Syst. 7:1156173. doi: 10.3389/fsufs.2023.1156173
Edited by:
Paula Jauregi, Centro Tecnológico Experto en Innovación Marina y Alimentaria (AZTI), SpainCopyright © Zhao, Chen, Zhang, Nie, Xiang, Peng, Liu, Luo and Wang. This is an open-access article distributed under the terms of the Creative Commons Attribution License (CC BY). The use, distribution or reproduction in other forums is permitted, provided the original author(s) and the copyright owner(s) are credited and that the original publication in this journal is cited, in accordance with accepted academic practice. No use, distribution or reproduction is permitted which does not comply with these terms.
*Correspondence: Xin Nie, niexin@sctu.edu.cn