- 1Animal and Human Health Program, International Livestock Research Institute, Addis Ababa, Ethiopia
- 2World Vegetable Center Eastern and Southern Africa, Addis Ababa, Ethiopia
- 3International Institute of Tropical Agriculture, Cotonou, BP, Benin
- 4College of Veterinary Medicine, Haramaya University, Dire Dawa, Ethiopia
- 5World Vegetable Center, Tainan, Taiwan
- 6World Vegetable Center, Nairobi, Kenya
- 7Natural Resources Institute, University of Greenwich, Kent, United Kingdom
- 8Animal and Human Health Program, International Livestock Research Institute, Nairobi, Kenya
- 9Bless Agri Food Laboratory Services, Addis Ababa, Ethiopia
Background: In Ethiopia, pesticides are widely used in vegetable production. However, if used incorrectly they may harm consumers of vegetables contaminated with pesticide residues, as well as producers handling the pesticides and lead to ecological damage. We performed a cross-sectional survey to assess pesticide residues in vegetables produced in central and eastern Ethiopia.
Methods: A total of 232 vegetable samples (91 tomatoes, 106 cabbages and 35 Swiss chard) were collected from fields and retail markets, and were screened for 35 pesticides (16 organochlorine, 11 organophosphate, 3 pyrethroids, 2 carbamates and 3 other agrochemicals) using GC–MS analysis.
Results: Pesticides residues were detected in 60% of Swiss chard, 47% of cabbage and 45% of tomato samples. Two or more pesticides were detected in 20% of cabbages, 13% of tomatoes and over half of Swiss chard samples. Bendiocarb, diazinon, endrin, piperonyl butoxide, profenofos and propargite were detected, but only diazinon, propargite and profenofos had residual values above EU Maximum Residue Level (MRL), with diazinon commonly detected at relatively high levels. About 15% of the total analyzed samples, 10% of cabbages and tomatoes, and nearly half of Swiss chard samples had pesticide concentration exceeding EU MRL. However, none of the tested samples had residues exceeding Codex Alimentarius Commission (Codex) MRLs.
Conclusion: This study showed widespread contamination of vegetables with pesticides, mainly organophosphates. We recommend monitoring and regulation of pesticides usage, with promotion of good agricultural practices.
1. Introduction
Pesticides improve crop production by protecting from pests (Tarannum et al., 2019), but their inappropriate use creates health risks for vegetable producers and consumers, and impact on the wider ecology and environment (Sharma et al., 2012; Negatu et al., 2016).
Unsafe use of pesticides in agriculture increases the presence of their residues in produce such as vegetables after harvest (Kapeleka et al., 2020). Intake of foods contaminated with pesticide residues exposes consumers to pesticides with potential long-term health risks (Darko and Akoto, 2008; Ferré et al., 2018; Nougadère et al., 2020). Farm workers may also be exposed to pesticides during application on farm; as may local residents exposed through inhalation of pesticide drift and/or volatilization from the environment (Hanssen et al., 2015; Teysseire et al., 2020; Polledri et al., 2021).
Studies have demonstrated that exposure to pesticides such as organophosphates and organochlorines can result in increased risk of cancers (De Roos et al., 2003), disruption of circulating hormones, reproductive problems (Meeker et al., 2008) and neurological disorders (Eskenazi et al., 2007). In addition to affecting human health, pesticides can have negative impacts on the environment including, but not limited to, non-point source pollution (Mekonen et al., 2016; Teklu et al., 2018), loss of pollinators and biodiversity (Fikadu, 2020), and losses to birds and aquatic wildlife (Yohannes et al., 2014).
Good Agricultural Practices (GAP) and pesticide usage regulations have been put in place with MRLs for pesticide levels in foods specified in national and international standards (WHO, FAO, 2010). MRLs are set to avoid potentially toxic high pesticide exposures while still allowing sufficiently high exposures needed for effective pest control. The EU defines sampling strategies and MRLs for relevant foods sold within the EU with removal from market and potentially prosecution if these MRLs are exceeded. The Codex Alimentarius Commission (Codex) also publishes MRLs that are expected to be applied as minimum requirements for foods traded internationally. In Ethiopia, pesticide MRLs have not yet been established for vegetables but the country uses the Codex MRLs as a reference (WHO, FAO, 2010).
Monitoring of pesticide residue concentrations in vegetables should be performed routinely to assess MRL compliance and protect consumers. Demonstrable effective monitoring can also help build trust between exporting and importing countries by showing oversight and control of food safety (Mahugija et al., 2017; Ali et al., 2020; Omwenga et al., 2021).
The use of chemical pesticides in agriculture is rapidly increasing in Ethiopia (Negatu et al., 2016), but there has been inadequate awareness and enforcement of good practices when using pesticides (Mengistie, 2016), and misuse of pesticides is widespread in Ethiopia (Negatu et al., 2016, 2021). A report generated from a larger project, which encompass this pesticide assessment as one of its activities, assessing vegetable and chicken food safety in Harar and Dire Dawa, eastern Ethiopia1 found high levels of consumer concern over contamination of foods with agricultural chemicals such as pesticides. In addition, experts reported anecdotes of inappropriate pesticide usage by farmers, repeated application of pesticides close to harvest (Amenu et al., 2021). Although these evidence shows poor GAP use of pesticides implying their higher presence on produce, there is little information on pesticide residue levels in vegetables in Ethiopia. To partially address this gap, we performed a cross-sectional screening survey to assess levels of pesticide residues in selected vegetables from fields and retail markets in central and eastern Ethiopia.
2. Materials and methods
2.1. Study areas
Vegetable samples were collected from markets, fields (farmers’ vegetable farms) and small-scale vegetable producers between October 2020 and January 2021 at fields in the central Rift-Valley of Ethiopia and the Akaki Kality areas of Addis Ababa. Samples from Harar and Addis Ababa retail markets were also collected (Figure 1).
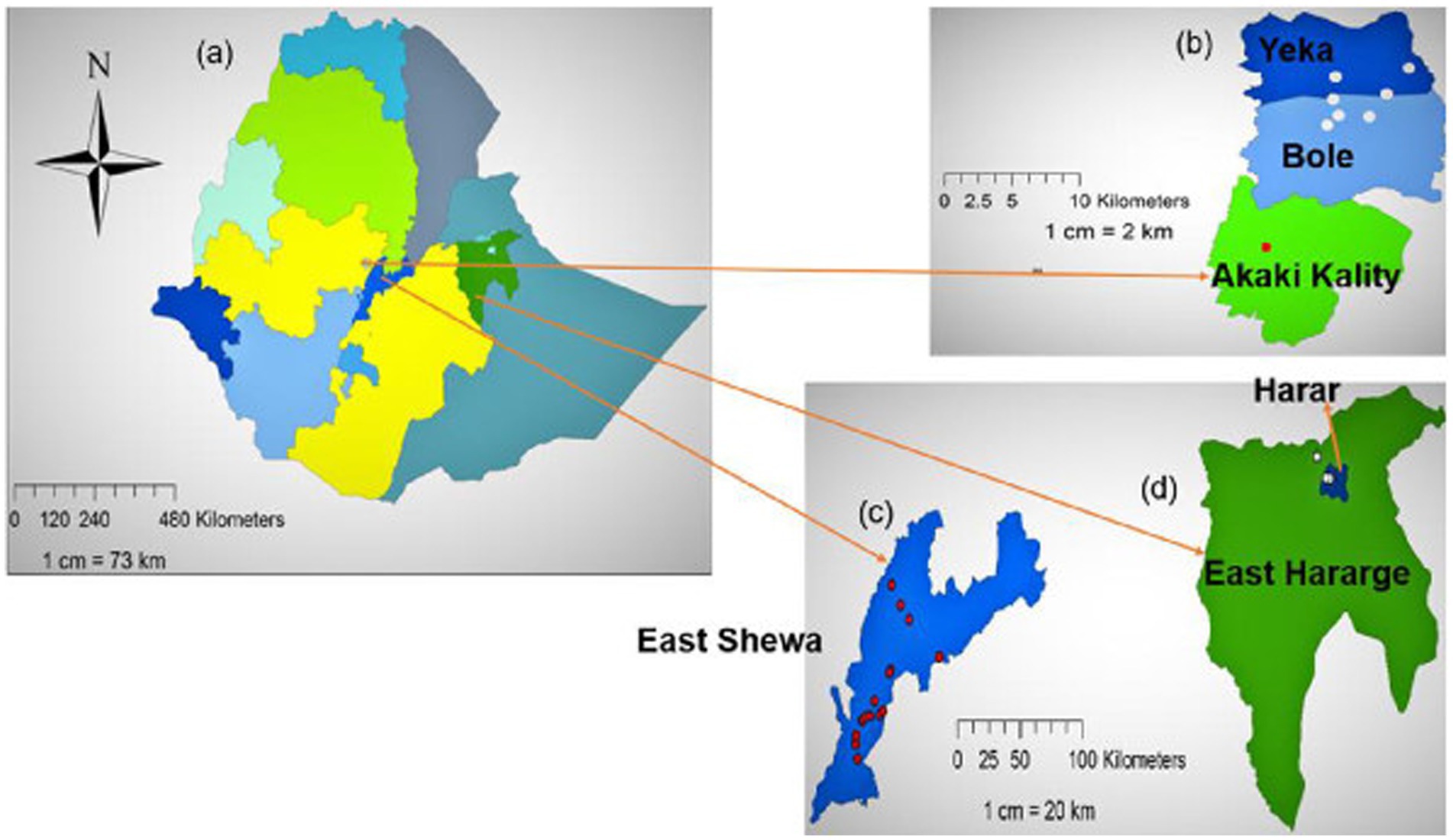
Figure 1. (A) Map of Ethiopia showing regions, with expanded maps showing vegetable sample collection sites in (B) Addis Ababa, (C) East Shewa zone, the vegetable production heartlands of Ethiopia and (D) Harar and East Hararge zone, a focus of the Pull-Push Project within which this study was conducted. Samples from fields are shown in red and samples from food markets are shown in white.
2.2. Sampling and data collection
2.2.1. Sample collection in fields
A total of 113 vegetable samples [31 cabbages (Brassica oleracea var. capitata), 55 tomatoes (Solanum lycopersicum) and 27 Swiss chard (Beta vulgaris)] were collected from farmers’ fields in major vegetable producing areas of East Shewa zone in Oromia and from small-scale producers’ vegetable plots within Addis Ababa (Akaki Kality sub-city) during October–November 2020. Experts identified these vegetables as high risk because of the common use of pesticides in these vegetables, in addition tomatoes are a focus of the project within which this survey was performed (Amenu et al., 2021). Fields growing these vegetables were randomly selected and samples were collected from different corners of a particular field. Depending on the size of the field, two to four samples were collected, packed, labeled, and placed in a container in shade. Within a field, samples from up to five individual plants were collected to create a 1 kg sample. Samples were kept cold until they reached the laboratory.
2.2.2. Sample collection in food markets
A total of 119 vegetable samples were collected from food markets (75 cabbages, 36 tomatoes and 8 Swiss chard) between 28 December 2020 and 22 January 2021. Samples from five major vegetable markets (both wholesale and retail) were collected in Harar city (market names: Dakar, Shewa ber, Shankor, Arategna, and Bate). The number of retail and wholesale traders sampled from each market was calculated with probability proportional to size based on a recent census of vegetable outlets (unpublished project activity), sampling a fixed proportion within each market. The main market in the nearby town of Haramaya was also sampled systematically selecting stalls evenly spread-out across the market, as were major retail markets in Bole and Yeka sub-cities of Addis Ababa (market names: Yerer, Goro, Gurd shola, Summit, Meri, Hayat, and Kotebe 02).
Within each market, the first encountered vegetable stall on entering the market was sampled and then every Kth vegetable stalls encountered during a walk covering the market were sampled; where K was the number of vegetable stalls in the market divided by the number of predefined vegetable samples required from that market. At a stall, a 1 kg sample of firm but mature fresh vegetables were collected and bagged. The stall-keeper selected the vegetables as for a normal customer and was not aware that the samples were collected as part of a study. The vegetable samples were kept cool until transported to the laboratory (Bless Agri Food Laboratory Services, Legetafo Legedadi, Addis Ababa, Ethiopia) within 0–4 days.
2.3. Chemicals and reagents
Pesticide standards of high purity (99.6%) from Sigma-Aldrich (United states, Saint Louis, MO) were used consisting of:
• 16 organochlorine pesticides (alpha-BHC, beta-BHC, delta-BHC, dieldrin, endrin, endrin aldehyde, endosulfan I (alpha), endosulfan II (beta isomer), endrin ketone, heptachlor epoxide isomer B, hexachlorobenzene, indoxacarb, lindane, 4,4-DDD, 4.4-DDE, and 4,4-DDT);
• 11 organophosphate pesticides (bromophos-ethyl, chlorpyrifos-methyl, diazinon, ethion, famphur, fenitrothion, fenthion, malathion, parathion, profenofos, thionazin);
• 3 pyrethroids [deltamethrin, cypermethrin 1 (Zeta), cyfluthrin 1];
• 2 carbamates (bendiocarb, propoxur); and
• 3 other agrochemicals (dichlorobenzonitrile, propargite, piperonyl butoxide).
All reagents and solvents were of analytical grade including acetonitrile, acetic acid, magnesium sulfate, sodium acetate and primary secondary amine (PSA) which were used for extraction and clean-up of the samples.
2.4. Sample preparation
Vegetable sample extraction and clean-up was performed using the QuEChERS (quick, easy, cheap, effective, rugged, and safe) method as indicated in the AOAC Official Method 2007.01 with slight modifications (Association of Official Analytical Chemists International, 2007). Briefly, a 1 kg of each vegetable sample was thoroughly chopped and homogenized using a high-speed multi-function comminutor. Fifteen grams (15 g) of the homogenized vegetable sample were weighed into a 50 mL Teflon tube. Then, 15 mL of 1% acetic acid in acetonitrile was added and the tube was sealed and vigorously shaken by hand for 1 min. Following this, a 1.5 g anhydrous sodium acetate and 6 g anhydrous MgSO4 were added into the Teflon tube and vortexed for 1 min and then centrifuged at 4,000 rpm for 10 min. After this, 4 mL aliquot of the upper layer extract was transferred to a 10 mL Teflon tube containing 200 mg primary secondary amine (PSA) and 150 mg anhydrous MgSO4. The tubes were sealed and vortexed for 1 min and then centrifuged at 4,000 rpm for 10 min. Finally, a 1.5 mL aliquot of the cleaned extracts was transferred into GC vials and injected into Gas Chromatography–Mass Spectrometry (GC-MS) system for pesticide analysis.
2.5. Method validation
Pesticide standard stock solutions were prepared in acetonitrile at 2,000 mg/L and stored in the dark at −20°C. Standard working solutions were prepared by dissolving appropriate amounts of stock solution with a mixture of acetone (9:1, v/v). The obtained standard working solutions were used to plot calibration curves as a function of peak area vs. concentrations of the selected pesticides. The concentration range was from 5 to 50 μg/kg for organochlorines, while that of organophosphates, pyrethroids, carbamates and the other agrochemicals was from 2 to 40 μg/kg. Our analytical method was validated using linearity (expressed as correlation coefficient), precision (expressed as repeatability relative standard deviation), mean recovery/reliability (as a measure of trueness) and sensitivity (expressed as slope of the regression equation). Moreover, the Limit of Detection (LOD) and Limit of Quantification (LOQ) of each screened pesticide were evaluated using 3S/m and 10S/m, respectively (where S is the standard deviation of the intercept and m is the slope of the regression line). Representative samples from each commodity group (tomatoes, cabbages and Swiss chard) were spiked with known concentrations of each standard dilution of pesticide and recovery values were determined. A non-spiked sample was also analyzed and used as a control.
2.6. GC-MS analysis
This study was performed on an Agilent 7890B GC equipped with G4513A auto-sampler coupled to a 7000C GC/MS Triple Quadrupole mass detector system (Agilent Technologies, Inc., CA, United States). An Agilent J&W DB-5 ms Ultra Inert capillary GC column with 30 m length, 0.25 mm internal diameter, and 0.25 μm film thickness was used to provide analyte separation and a highly inert flow path into the detector. The carrier gas was helium (99.999%) at a column flow rate of 1.2 mL/min. The interface and the injector were programmed at 280°C for splitless injection of 1 μL. The eluent from the GC column was transferred through a transfer line at a temperature of 280°C and fed into a 70 eV electron impact ionization source at a source temperature of 280°C. The analysis was done in the selected ion monitoring mode.
2.7. Statistical analysis
The pesticide residues (μg/kg) of samples were compared with MRLs from Codex Alimentarius Commission standards (Codex MRLs) and European Commission legislation and standards (EU MRLs; Codex, 2021; European Commission, 2021). For a specific pesticide, its concentration in a given vegetable sample was compared with its MRL in that vegetable in EU or Codex pesticide database but a default MRL of 10 μg/kg (0.01 mg/kg) for EU MRL and 2,000 μg/kg (2 mg/kg) for Codex MRL was used when a pesticide is not specifically mentioned (Codex, 2021; European Commission, 2021).
Pesticide residues concentration was log-transformed using to improve the normality of our data distribution. The distribution of pesticide residues was described, stratifying by sample location (market, field) and vegetable type, with associations tested using Chi-square tests and One-way ANOVA. Analysis was done using R (version 4.1.0; R Core Team, 2021) and maps produced in ArcGIS (version 10.2, ESRI, California, United States; ESRI, 2020).
3. Results
3.1. Method validation
Our results showed excellent linearity and reproducibility indicating good fitness of the model with R2 ≥ 0.99 for all analytes. The good reliability of our method was expressed by recovery values obtained to be in the range from 71% to 112%, which are better comparing to the acceptable limits for individual recovery results that should normally be within the range of 60%–140%. The relative standard deviations showed a good repeatability and precision of the method, was under 5%, which is in the recommended range, RSD ≤ 20%. The LOD and LOQ values of the tested pesticides were very low in the order of picogram/kg. The obtained values of LOD and LOQ in this study were below the Codex MRLs (Table 1).
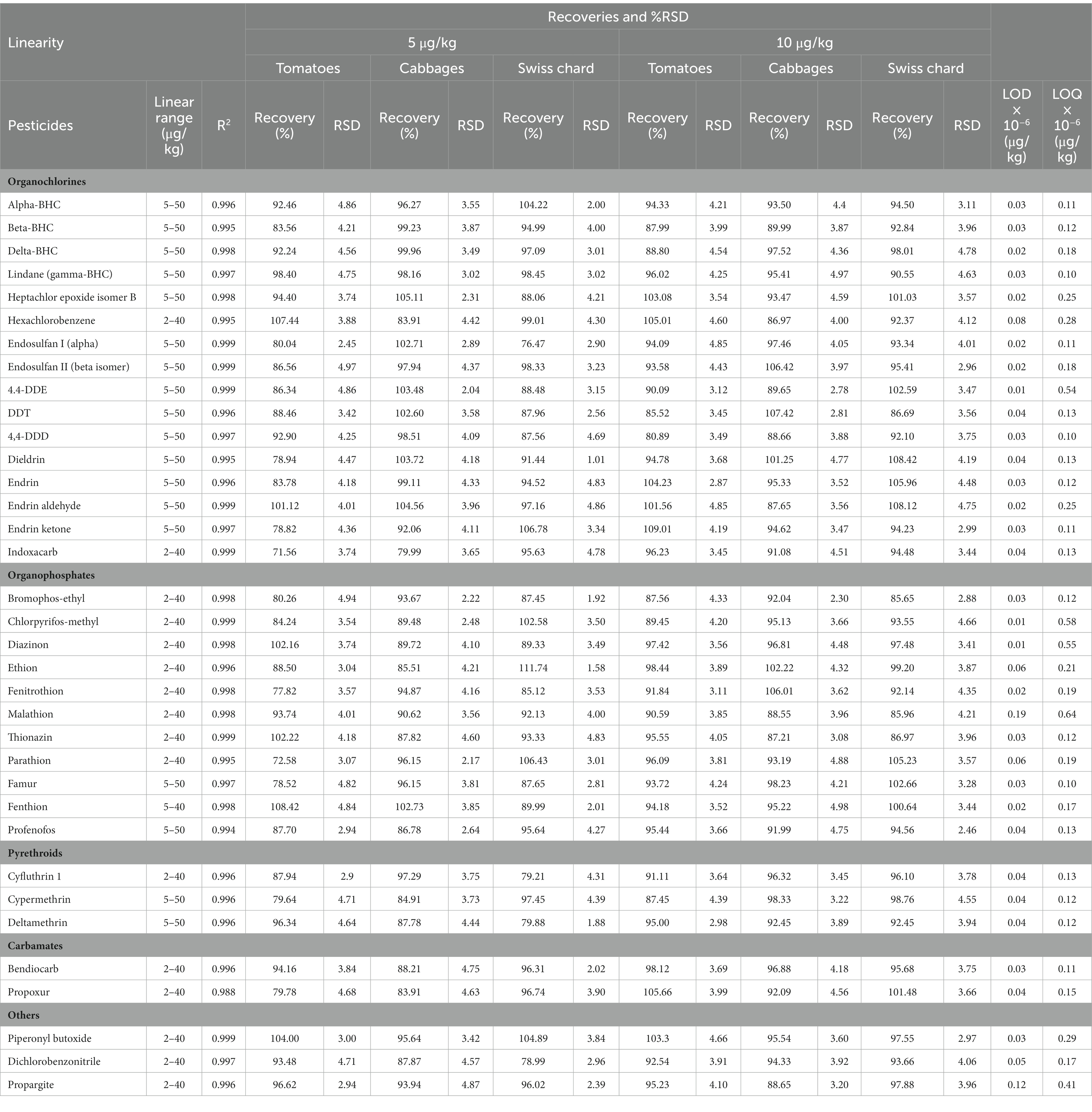
Table 1. Method validation data at 5 and 10 μg/kg: linearity, recovery (n = 5), %RSD (n = 5), LOD and LOQ of 35 pesticides in 3 commodities.
3.2. Pesticide prevalence and concentration
Out of the 35 agrochemicals tested, organophosphates (diazinon, profenofos), organochlorines (endrin), carbamates (bendiocarb) and other agrochemicals (piperonyl butoxide, propargite) were detected. Diazinon was the most prevalent residue, detected in 33% (76/232, 95% CI: 27%–39%) of the vegetable samples.
Of the 232 total vegetable samples analyzed, 48% (112/232, 95% CI: 42%–55%) contained at least one type of detectable pesticide residue: 60% (21/35, 95% CI: 42%–76%) of Swiss chard, 47% (50/106, 95% CI: 37%–57%) of cabbages and 45% (41/91, 95% CI: 34%–55%) of tomatoes. But there was weak evidence for pesticide residue prevalence variation between vegetable types (p = 0.3). Pesticide contamination prevalence was similar for vegetables from markets 51% (61/119, 95% CI: 41%–61%) and fields 45% (51/113, 95% CI: 45%–64%; p = 0.42) (Table 2).
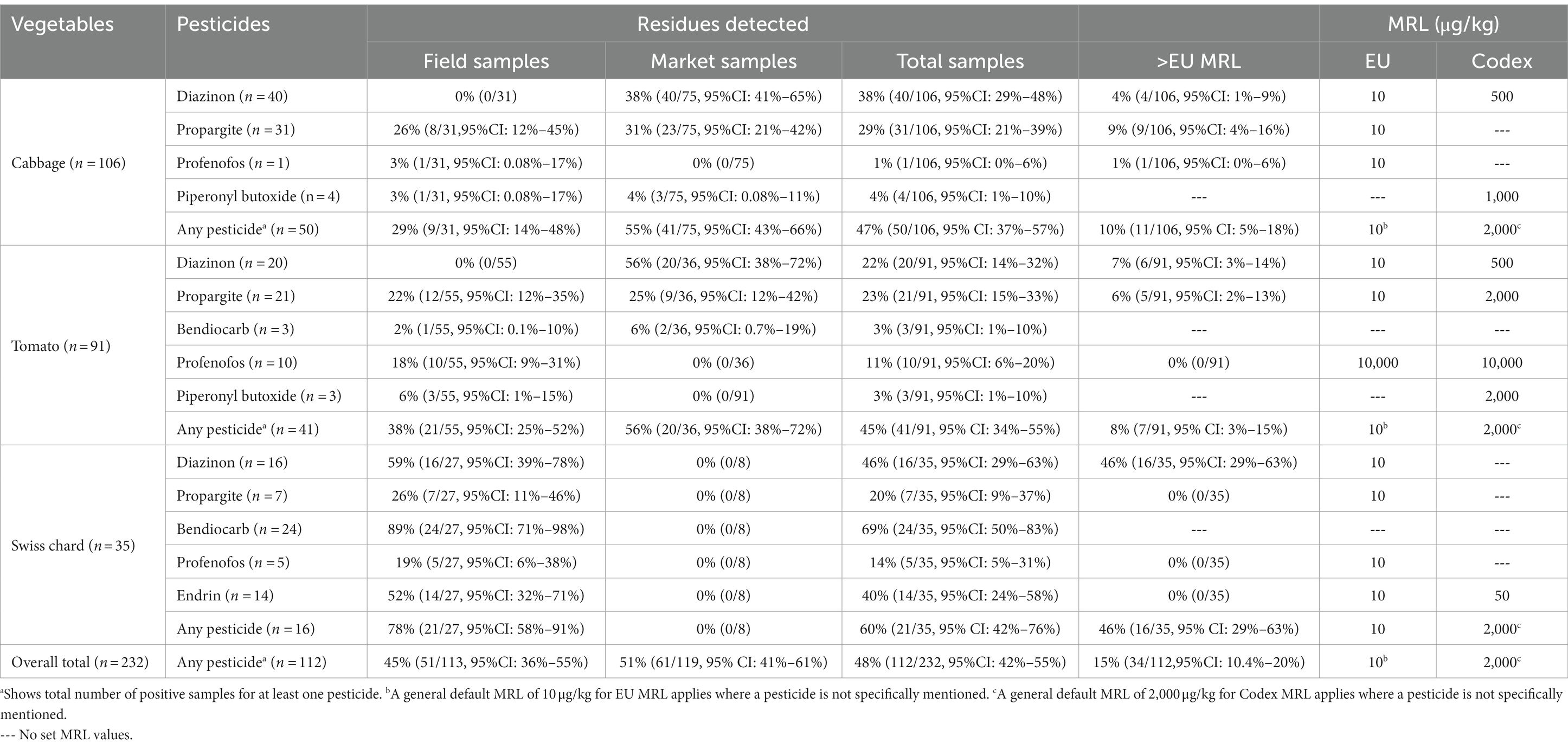
Table 2. Number and percentage of cabbage, tomato, and Swiss chard samples from fields and markets with pesticide residues detected and those with residue levels above EU MRLs (all pesticides had MRLs below Codex MRLs).
Of all the detected pesticides, diazinon had the highest overall mean concentration (0.97(0.73,1.21) μg/kg). Mean concentrations of pesticides sometimes varied between the vegetable types (p < 0.001). Mean concentrations of bendiocarb (p < 0.001), endrin (p < 0.001) profenofos (p < 0.001) and propargite (p = 0.02) varied between field and market samples but diazinon (p = 0.84) and piperonyl butoxide (p = 0.28) had similar mean concentration in field and market samples (Figure 2).
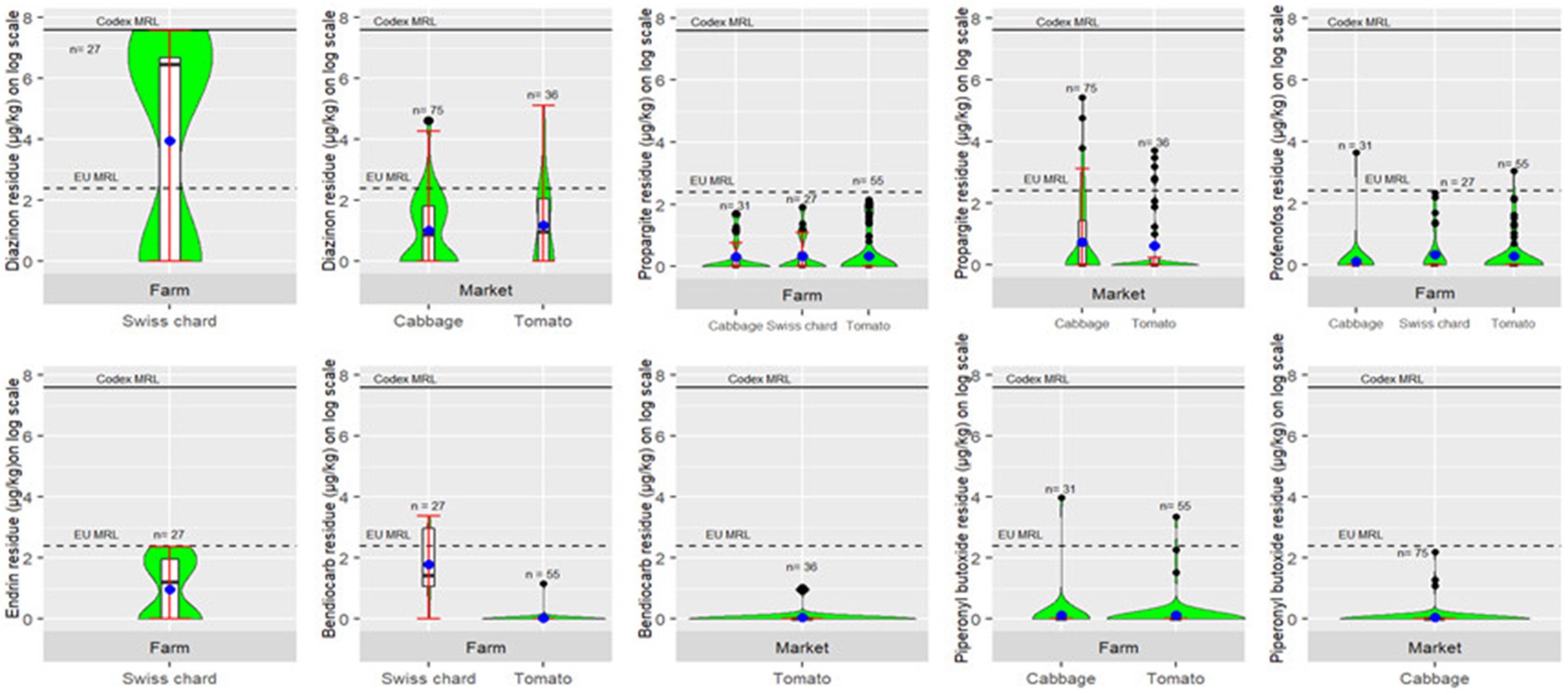
Figure 2. Violin and boxplots showing comparison of pesticide residue levels (μg/kg) on log(x + 1) scale in field and market vegetable samples for diazinon, propargite, profenofos, endrin, bendiocarb, and piperonyl butoxide. Blue points show mean concentration of pesticide residues in vegetables and black points show outliers. Horizontal dashed and solid lines indicate EU and Codex MRLs (μg/kg) on log(x + 1) scale, respectively. NB, we used a general default MRL of 10 μg/kg for EU MRL and 2,000 μg/kg for Codex MRL where a pesticide is not specifically mentioned.
About 15% (34/232, 95% CI: 10%–20%) of the total samples had a pesticide residue level of greater than the EU MRLs (mostly diazinon, but also propargite and one sample with profenofos), and an additional 34% (78/232) had pesticides detected but at less than or equal to the EU MRLs. About 46% (16/35, 95% CI: 29%–63%) of Swiss chard, 10% (11/106, 95% CI: 5%–18%) of cabbages and 8% (7/91, 95% CI: 3%–15%) of tomatoes had a pesticide residue level of greater than the EU MRL with variation between vegetable types (p < 0.001). MRL exceedance was similar in field samples, 15% (17/113, 95% CI: 9%–23%) and market samples, 14% (17/119, 95% CI: 9%–22%; p = 0.10; Table 2).
We detected many samples contaminated with multiple pesticide residues; up to five in a single sample. Of all samples tested, 22% (52/232, 95% CI: 14–36%) of them had two or more pesticides residues. More than half [54%(19/35, 95% CI: 37%–71%)] of Swiss chard, 20% (21/106, 95% CI: 13%–29%) of cabbages and 13% (12/91, 95% CI: 7%–22%) of tomatoes samples were contaminated with two or more pesticide residues (Figure 3).
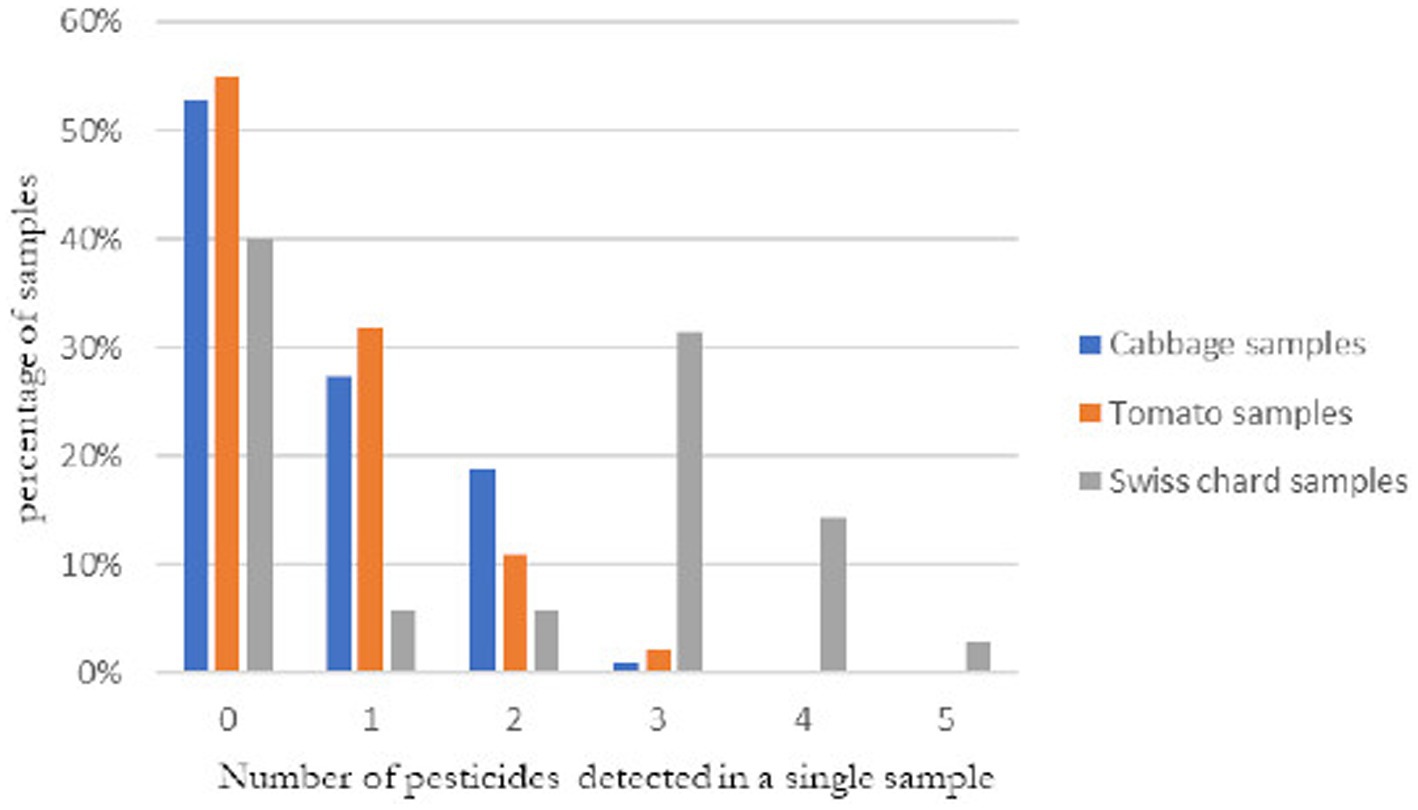
Figure 3. Number of pesticides found in a single sample of cabbage (n = 106), tomato (n = 91) and Swiss chard (n = 35) and percentage of the samples with the detected number of pesticides.
4. Discussion
The prevalence of pesticide contamination of vegetables in our study is comparable with pesticide residue prevalence reported in vegetables elsewhere in Sub-Saharan Africa: 46% in Tanzania (Kapeleka et al., 2020) and 54% in Kenya (Nakhungu et al., 2017), with higher values sometimes reported: 63% in Zambia (Mwanja et al., 2017), 92% in Sudan (Ali et al., 2020) and 96% in Tanzania (Mahugija et al., 2017). However, in Ethiopia, pesticide residues in vegetables has seldom been assessed (Loha et al., 2020), with a few studies assessing the residues in other commodities such as khat (Daba et al., 2011; Atnafie et al., 2021), wheat (Daba et al., 2011) and tea (Siraj et al., 2021).
EU MRL exceedance of pesticide residues concentration in vegetables observed in this study was relatively lower compared with findings in other developing countries where 21%–28% were reported (Lozowicka et al., 2016; Jallow et al., 2017; Ramadan et al., 2020). Considering inadequate pesticide legislation enforcement in Ethiopia, higher pesticide concentration is anticipated in the vegetables than the current findings, though, various factors could contribute to pesticide concentration levels in vegetables. Fields samples are expected to have higher residue levels than market samples as pesticides evaporate and degrade after application, also traders may wash the vegetables especially cabbages and Swiss chard to keep them fresh, which could reduce pesticide concentration (Inonda et al., 2015; El-Saeid and Selim, 2016). Our current results also showed slightly higher pesticide residues concentration in fields samples than market samples, though, the evidence for the variation between the sample types was weak. In addition, in this study, more than half of the samples were market samples which might contribute to reduced concentration levels. Pesticide concentration in vegetables can also vary with seasons of study (Inonda et al., 2015), with our samples collected at the post-harvest stage which might contribute to reduced concentration as frequency of pesticide application is greatly reduced at this stage. Crop types can affect the levels of pesticide volatilization-a process by which pesticides dissipate-determining pesticide residue concentration in them (Gamalero et al., 2003; Inonda et al., 2015). Consistent with this, we found in our analysis that pesticide residue concentration varied among vegetable types. Lack of adherence to pre-harvest interval between application and harvest is also a common cause of high pesticide residues (Osei et al., 2015). Farmers’ knowledge level and practices in the safe use of pesticides can further influence pesticide concentration levels in vegetables (Horna, 2008).
Foods with residue levels below MRLs can be considered safe for consumers (WHO, FAO, 2010) but MRL is not a safety limit as foods with residues above MRL may still be safe for consumption (Keikotlhaile and Spanoghe, 2011). However, in addition to health and ecological impacts, MRL exceedance can restrict access to international export markets (Horna, 2008) with governing authorities required to monitor and enforce MRL compliance (WHO, FAO, 2010). Reflecting cultural differences in risk perception, the European Union has set more stringent MRLs (typically 0.01 mg/kg) than the Codex Alimentarius Commission (typically 2.0 mg/kg; Codex, 2021; European Commission, 2021; Food Business Africa, 2021), reflecting the need for exporting countries to establish and enforce national pesticide regulations to meet these standards.
Farmers in Ethiopia have poor knowledge about the safe use of pesticides, contributing to MRL exceedance (Gesesew et al., 2016; Negatu et al., 2016; Mergia et al., 2021). Although GAP allows productive farming with lower amounts of agrochemicals (Kılıç et al., 2020) contributing to reduced pesticide residues in produce (Inonda et al., 2015), it is rarely practiced in the country’s agricultural activities. Ethiopia currently exports vegetables to only non-European countries. One of the constraints for EU market access is an inability to prove that pesticide use is compliant with GAP and that the MRLs are within the required limits (CBI, 2020).
This study found widespread presence of pesticide residues on vegetables with nearly half of the vegetable samples contaminated with one or more types of pesticide, mostly organophosphates. We also detected multiple pesticide residues in a single vegetable sample. Pesticide concentration varied between vegetables and, field and market samples representing the need to adhere to specific GAP while using pesticides for different vegetable types. About 15% of vegetable samples had pesticide residue concentrations above EU MRL but none were above Codex MRLs. But it is unlikely that we capture the margins of the distribution of pesticide contamination and rare, higher exposures are anticipated. Nonetheless, the study results still raise food safety and economic risk concerns needing improved monitoring and regulation of agricultural pesticides, with improved farmer education on the safe use of pesticides and the risks they pose to producers, consumers, and the environment.
Data availability statement
The data used in this study are available in ILRI Datasets Portal (https://hdl.handle.net/20.500.11766.1/FK2/ZFUA66), containing pesticide residues in the study vegetables (Data file.pesticide residues).
Ethics statement
Ethics approval was granted for this study by ILRI’s Institutional Research Ethics Committee (approval number: ILRI-IREC2019-36/1; ILRI, 2021). While collecting vegetable samples from retailer markets, we did not collect information on vegetable stalls that could identify them and raise privacy issues. We obtained oral consent from vegetable farmers to collect vegetable samples from their farms, analyzing the survey data anonymously.
Author contributions
TJDK designed, supervised and managed the project and received funding for the study. LG, SG, and WB collected the study data. GD curated the data and created data visualization. GD and SMF performed formal data analysis. GD wrote original draft of the manuscript. TJDK, WB, MFS, RS, RR, DG, and HG reviewed and edited the manuscript. All authors contributed to the article and approved the submitted version.
Funding
This work was supported by the Bill & Melinda Gates Foundation [INV-008430 (OPP1195588)]. Under the grant conditions of the Bill & Melinda Gates Foundation, a Creative Commons Attribution 4.0 Generic License has already been assigned to the Author Accepted Manuscript version that might arise from this submission. The work was also supported by the German Federal Ministry for Economic Cooperation and Development (BMZ) through the One Health Research, Education and Outreach Centre in Africa (OHRECA) led by ILRI. Additional support was received from CGIAR Research Program on Agriculture for Nutrition and Health (A4NH). Support was also provided by United Kingdom aid from the UK Foreign, Commonwealth and Development Office.
Acknowledgments
This activity is part of the urban food markets in Africa: incentivizing food safety using a pull-push approach which is supported by the Bill & Melinda Gates Foundation, German Federal Ministry for Economic Cooperation and Development (BMZ) through the One Health Research, Education and Outreach Centre in Africa (OHRECA) led by ILRI, the CGIAR Research Program on Agriculture for Nutrition and Health (A4NH) led by the International Food Policy Research Institute and the Foreign, Commonwealth and Development Office (FCDO) of the UK Government. We also acknowledge the CGIAR Fund Donors (https://www.cgiar.org/ funders). Our special thanks go to the people who participated in the study in data collection and in sharing their expertise and experience with us. We would like to extend our acknowledgements to Bless Agri Food Laboratory Services staff for their collaboration during laboratory analysis of our study samples.
Conflict of interest
The authors declare that the research was conducted in the absence of any commercial or financial relationships that could be construed as a potential conflict of interest.
Publisher’s note
All claims expressed in this article are solely those of the authors and do not necessarily represent those of their affiliated organizations, or those of the publisher, the editors and the reviewers. Any product that may be evaluated in this article, or claim that may be made by its manufacturer, is not guaranteed or endorsed by the publisher.
Footnotes
1. ^Pull-Push Project: urban food markets in Africa—incentivizing food safety using a pull-push approach.
References
Ali, S. E. A., Aziz, M. E. A., and Mohamed, S. E. (2020). Determination of pesticides residues in eggplant and tomatoes from central marked in Khartoum state using Quechers method and gas liquid chromatography-mass spectrometry. Biomed J Sci Tech Res. 24, 18165–18173. doi: 10.26717/BJSTR.2020.24.004035
Amenu, K., Bedasa, M., Wamile, M., Worku, H., Kasim, K., Taha, M., et al. (2021). Qualitative assessment of chicken and vegetable value chains in Harar and Dire Dawa, Ethiopia: Food safety perspectives. ILRI Research Report 82. ILRI Research Report. Nairobi, Kenya: ILRI.
Association of Official Analytical Chemists International. (2007). AOAC official method 2007.01. Pesticide residues in foods by acetonitrile extraction and partitioning with magnesium sulfate gas chromatography/mass spectrometry and liquid chromatography/tandem mass spectrometry first action 2007. Rockville, Maryland, USA. Available at: https://www.aoac.org/about-aoac-international/ (Accessed 16 October 2021).
Atnafie, S. A., Muluneh, N. Y., Getahun, K. A., Tsegaw Woredekal, A., and Kahaliw, W. (2021). Pesticide residue analysis of Khat leaves and health risks among Khat chewers in the Amhara region, northwestern Ethiopia. Yabe J, editor. J. Environ. Public Health 2021:4680573. doi: 10.1155/2021/4680573
CBI. (2020). Netherlands Ministry of Foreign Affairs. The Centre for the Promotion of imports from developing countries (CBI). EU market research—Ethiopia fresh fruit and vegetables. Available at:
Codex. (2021). Pesticides|CODEX ALIMENTARIUS FAO-WHO. Available at: http://www.fao.org/fao-who-codexalimentarius/codex-texts/dbs/pestres/pesticides/en/ (Accessed 8 September 2021).
Daba, D., Hymete, A., and Bekhit, A. A. (2011). Multi residue analysis of pesticides in wheat and Khat collected from different regions of Ethiopia. Bull. Environ. Contam. Toxicol. 86, 336–341. doi: 10.1007/s00128-011-0207-1
Darko, G., and Akoto, O. (2008). Dietary intake of organophosphorus pesticide residues through vegetables from Kumasi, Ghana. Food Chem. Toxicol. 46, 3703–3706. doi: 10.1016/j.fct.2008.09.049
De Roos, A. J., Zahm, S. H., Cantor, K. P., Weisenburger, D. D., Holmes, F. F., Burmeister, L. F., et al. (2003). Integrative assessment of multiple pesticides as risk factors for non-Hodgkin’s lymphoma among men. Occup. Environ. Med. 60, 1–9. doi: 10.1371/journal.pone.0232258
El-Saeid, M., and Selim, M. (2016). Effect of food processing on reduction of pesticide residues in vegetables. J Appl Life Sci Int. 8, 1–6. doi: 10.9734/JALSI/2016/26801
Eskenazi, B., Marks, A. R., Bradman, A., Harley, K., Barr, D. B., Johnson, C., et al. (2007). Organophosphate pesticide exposure and neurodevelopment in young Mexican-American children. Environ. Health Perspect. 115, 792–798. doi: 10.1289/ehp.9828
ESRI. (2020). Environmental Systems Research Institute. ArcGIS Desktop: Release 10. 2020. Redlands, CA. Available at: https://www.esri.com/en-us/arcgis/products/arcgis-online/overview (Accessed 16 October 2021).
European Commission. (2021). EU pesticides database (v.2.2) search products. Available at:
Ferré, D. M., Quero, A. A. M., Hernández, A. F., Hynes, V., Tornello, M. J., Lüders, C., et al. (2018). Potential risks of dietary exposure to chlorpyrifos and cypermethrin from their use in fruit/vegetable crops and beef cattle productions. Environ. Monit. Assess. 190:292. doi: 10.1007/s10661-018-6647-x
Fikadu, Z. (2020). Pesticides use, practice and its effect on honeybee in Ethiopia: a review. Int J Trop Insect Sci 40, 473–481. doi: 10.1007/s42690-020-00114-x
Food Business Africa. (2021). Food Business Africa EU lowers the maximum residue level for several pesticides allowable on horticultural imports from Kenya. Available at: https://www.foodbusinessafrica.com/eu-lowers-the-maximum-residue-level-for-several-pesticides-allowable-on-horticultural-imports-from-kenya/ (Accessed 18 September 2021).
Gamalero, E., Lingua, G., Berta, G., and Lemanceau, P. (2003). Methods for studying root colonization by introduced beneficial bacteria. Agronomie 23, 407–418. doi: 10.1051/agro:2003014
Gesesew, H. A., Woldemichael, K., Massa, D., and Mwanri, L. (2016). Farmers knowledge, attitudes, practices and health problems associated with pesticide use in rural irrigation villages, Southwest Ethiopia. PLoS One 11, 1–13. doi: 10.1371/journal.pone.0162527
Hanssen, V. M., Nigatu, A. W., Zeleke, Z. K., Moen, B. E., and Bråtveit, M. (2015). High prevalence of respiratory and dermal symptoms among Ethiopian flower farm workers. Arch. Environ. Occup. Health 70, 204–213. doi: 10.1080/19338244.2013.853645
Horna, D. (2008). Selected paper prepared for presentation at the American agricultural economics association annual Meeting,Orlando, FL. Orlando, FL.
ILRI. (2021). Institutional Research Ethics Committee (IREC) Operating guidelines. Nairobi, Kenya: ILRI.
Inonda, R., Njage, E., Ngeranwa, J., and Mutai, C. (2015). Determination of pesticide residues in locally consumed vegetables in Kenya. African J Pharmacol Ther 4, 1–6.
Jallow, M. F. A., Awadh, D. G., Albaho, M. S., Devi, V. Y., and Thomas, B. M. (2017). Pesticide risk behaviors and factors influencing pesticide use among farmers in Kuwait. Sci. Total Environ. 574, 490–498. doi: 10.1016/j.scitotenv.2016.09.085
Kapeleka, J. A., Sauli, E., Sadik, O., and Ndakidemi, P. A. (2020). Co-exposure risks of pesticides residues and bacterial contamination in fresh fruits and vegetables under smallholder horticultural production systems in Tanzania. PLoS One 15, e0235345–e0235323. doi: 10.1371/journal.pone.0235345
Keikotlhaile, B. M., and Spanoghe, P. (2011). Pesticide residues in fruits and vegetables. Pesticides—formulations, effects, fate, 243–252.
Kılıç, O., Boz, İ., and Eryılmaz, G. A. (2020). Comparison of conventional and good agricultural practices farms: a socio-economic and technical perspective. J. Clean. Prod. 258:120666. doi: 10.1016/j.jclepro.2020.120666
Loha, K. M., Lamoree, M., and De, J. (2020). Pesticide residue levels in vegetables and surface waters at the central Rift Valley (CRV) of Ethiopia. Environ. Monit. Assess. 192:546. doi: 10.1007/s10661-020-08452-6
Lozowicka, B., Jankowska, M., Hrynko, I., and Kaczynski, P. (2016). Removal of 16 pesticide residues from strawberries by washing with tap and ozone water, ultrasonic cleaning and boiling. Environ. Monit. Assess. 188, 1–19. doi: 10.1007/s10661-015-4850-6
Mahugija, J. A. M., Khamis, F. A., and Lugwisha, E. H. J. (2017). Assessment of pesticide residues in tomatoes and watermelons (fruits) from Markets in Dar es salaam, Tanzania. J. Appl. Sci. Environ. Manag. 21, 497–501. doi: 10.4314/jasem.v21i3.10
Meeker, J. D., Ravi, S. R., Barr, D. B., and Hauser, R. (2008). Circulating estradiol in men is inversely related to urinary metabolites of nonpersistent insecticides. Reprod. Toxicol. 25, 184–191. doi: 10.1016/j.reprotox.2007.12.005
Mekonen, S., Argaw, R., Simanesew, A., Houbraken, M., Senaeve, D., Ambelu, A., et al. (2016). Pesticide residues in drinking water and associated risk to consumers in Ethiopia. Chemosphere 162, 252–260. doi: 10.1016/j.chemosphere.2016.07.096
Mengistie, B. T. (2016). Policy-practice nexus: pesticide registration, distribution and use in Ethiopia. SM J Environ Toxicol. 2, 1–13.
Mergia, M. T., Weldemariam, E. D., Eklo, O. M., and Yimer, G. T. (2021). Small-scale farmer pesticide knowledge and practice and impacts on the environment and human health in Ethiopia. J Heal Pollut. 11, 1–19. doi: 10.5696/2156-9614-11.30.210607
Mwanja, M., Jacobs, C., Mbewe, A. R., and Munyinda, N. S. (2017). Assessment of pesticide residue levels among locally produced fruits and vegetables in Monze district, Zambia. Int J Food Contam 4, 1–9. doi: 10.1186/s40550-017-0056-8
Nakhungu, M. V., Margaret, N. K., Deborah, A. A., and Peterson, N. W. (2017). Pesticide residues on tomatoes grown and consumed in Mwea irrigation scheme, Kirinyaga County, Kenya. Asian J Agric Hortic Res 2021, 1–11. doi: 10.9734/ajahr/2021/v8i230110,Box PO
Negatu, B., Dugassa, S., and Mekonnen, Y. (2021). Environmental and health risks of pesticide use in Ethiopia. J Heal Pollut. 11, 1–12. doi: 10.5696/2156-9614-11.30.210601
Negatu, B., Kromhout, H., Mekonnen, Y., and Vermeulen, R. (2016). Use of chemical pesticides in Ethiopia: a cross-sectional comparative study Onknowledge, attitude and practice of farmers and farm workers in three farming systems. Ann. Occup. Hyg. 60, 551–566. doi: 10.1093/annhyg/mew004
Nougadère, A., Sirot, V., Cravedi, J. P., Vasseur, P., Feidt, C., Fussell, R. J., et al. (2020). Dietary exposure to pesticide residues and associated health risks in infants and young children – results of the French infant total diet study. Environ. Int. 137:105529. doi: 10.1016/j.envint.2020.105529
Omwenga, I., Kanja, L., Zomer, P., Louisse, J., Rietjens, M. C. M., Mol, H., et al. (2021). Organophosphate and carbamate pesticide residues and accompanying risks in commonly consumed vegetables in Kenya. Food Addit Contam Part B 14, 48–58. doi: 10.1080/19393210.2020.1861661
Osei, A., Sandra, G., Martin, K. A., and Joseph, A. (2015). Estimation of human health risk associated with the consumption of pesticide-contaminated vegetables from Kumasi, Ghana. Environ. Monit. Assess. 2015:187.
Polledri, E., Mercadante, R., Consonni, D., and Fustinoni, S. (2021). Cumulative pesticides exposure of children and their parents living near vineyards by hair analysis. Int. J. Environ. Res. Public Health 18:3723. doi: 10.3390/ijerph18073723
R Core Team. (2021). R: A language and environment for statistical computing. R Foundation for Statistical Computing, Vienna, Austria. R Core TEAM; Available at: https://www.r-project.org/
Ramadan, M. F. A., Abdel-Hamid, M., Altorgoman, M. M. F., AlGaramah, H. A., Alawi, M. A., Shati, A. A., et al. (2020). Evaluation of pesticide residues in vegetables from the Asir region, Saudi Arabia. Molecules 25:205. doi: 10.3390/molecules25010205
Sharma, D. R., Thapa, R. B., Manandhar, H. K., Shrestha, S. M., and Pradhan, S. B. (2012). Use of pesticides in Nepal and impacts on human health and environment. J Agric Environ. 13, 67–74. doi: 10.3126/aej.v13i0.7590
Siraj, J., Mekonen, S., Astatkie, H., and Gure, A. (2021). Organochlorine pesticide residues in tea and their potential risks to consumers in Ethiopia. Heliyon 7:e07667. doi: 10.1016/j.heliyon.2021.e07667
Tarannum, N., Singh, M., and Hawaldar, R. (2019). “Pesticides as an occupational hazard facts and figures” in Handbook of Research on the Adverse Effects of Pesticide Pollution in Aquatic Ecosystems (IGI Global), 201–214.
Teklu, B. M., Hailu, A., Wiegant, D. A., Scholten, B. S., and Van den Brink, P. J. (2018). Impacts of nutrients and pesticides from small-and large-scale agriculture on the water quality of Lake Ziway. Ethiopia. Environ Sci Pollut Res. 25, 13207–13216. doi: 10.1007/s11356-016-6714-1
Teysseire, R., Manangama, G., Baldi, I., Carles, C., Brochard, P., Bedos, C., et al. (2020). Assessment of residential exposures to agricultural pesticides: a scoping review. PLoS One 15, 1–19.
WHO, FAO. (2010). Codex Alimentarius commission: Procedural manual. Rome: Citeseer; Available at: https://www.fao.org/publications/card/en/c/8464e77b-25ee-5e26-8b25-45dd235397b2/
Keywords: Ethiopia, maximum residue level, organochlorines, organophosphates, pesticide residues, vegetables
Citation: Dinede G, Bihon W, Gazu L, Foukmeniok Mbokou S, Girma S, Srinivasan R, Roothaert R, Grace D, Gashaw H and Knight-Jones TJD (2023) Assessment of pesticide residues in vegetables produced in central and eastern Ethiopia. Front. Sustain. Food Syst. 7:1143753. doi: 10.3389/fsufs.2023.1143753
Edited by:
Mohammad Dalower Hossain Prodhan, Bangladesh Agricultural Research Institute, BangladeshReviewed by:
Lauren S. Jackson, United States Food and Drug Administration, United StatesAney Parven, The University of Newcastle, Australia
Copyright © 2023 Dinede, Bihon, Gazu, Foukmeniok Mbokou, Girma, Srinivasan, Roothaert, Grace, Gashaw and Knight-Jones. This is an open-access article distributed under the terms of the Creative Commons Attribution License (CC BY). The use, distribution or reproduction in other forums is permitted, provided the original author(s) and the copyright owner(s) are credited and that the original publication in this journal is cited, in accordance with accepted academic practice. No use, distribution or reproduction is permitted which does not comply with these terms.
*Correspondence: Getachew Dinede, ZGluZWRlZ2VjaEBnbWFpbC5jb20=