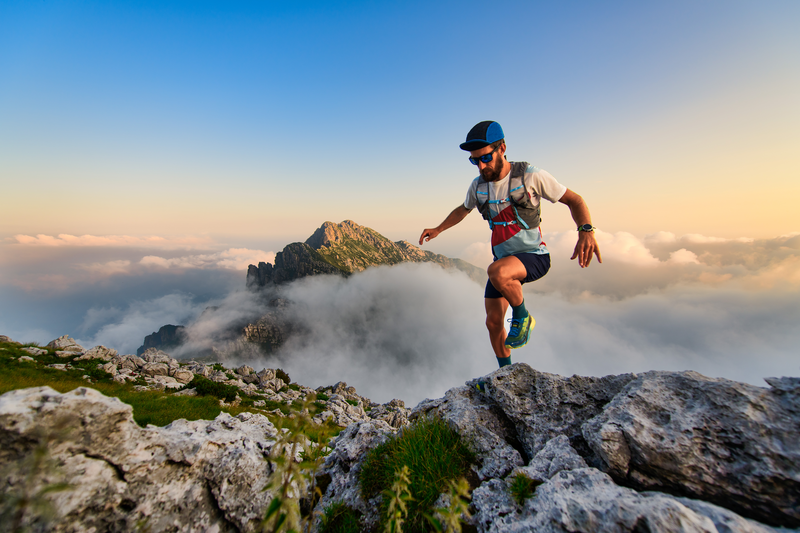
95% of researchers rate our articles as excellent or good
Learn more about the work of our research integrity team to safeguard the quality of each article we publish.
Find out more
ORIGINAL RESEARCH article
Front. Sustain. Food Syst. , 28 February 2023
Sec. Agroecology and Ecosystem Services
Volume 7 - 2023 | https://doi.org/10.3389/fsufs.2023.1143431
This article is part of the Research Topic Optimising Management Practices to Secure Grassland Agroecosystems' Sustainability View all 18 articles
Livestock intensification improves production efficiency and enhances the demand for quality forage to feed ruminants. Novel combinations of forage plants, especially including Gramineae and Leguminous plants, benefit both ruminant animals and contribute to a sustainable environment. This study explored an oat-pea mixed seeding strategy as an approach to improving silage quality. Before ensialing, lactic acid bacteria (Lactobacillus plantarum and Lactobacillus brucelli) were added to forage from five different mixed seeding proportions of oats (O) and peas (P) (10:0, 8:2, 7:3, 5:5, and 0:10 oat to pea ratio) at two harvesting periods (the early flowering stage and the milk ripening stage for the oats). The results showed that mixed seeding changed the soil quality parameters. Moreover, the silage from the O5P5 (5:5 oat to pea ratio) group showed the lowest pH values (4.16) and highest LA contents (7.74% DM) after ensiling for 7d (p < 0.05) in early flowering stage. Also, the O5P5 group increased the number of tillers/branches and produced silage with the highest CP content (13.14–14.06) after ensiling for 7d in early flowering stage and both 7d and 30d in early flowering stage and milk ripening stage (p < 0.05). In conclusion, this study found that the selection of oat-pea mixed seeding as O5P5 and harvesting at the milk ripening stage of oat is recommended as a desirable oat-pea mixed seeding strategy for producing high quality silage.
With increasing demands for livestock products, there is increasing emphasis on the need for good quality animal feeds and forages. This emphasis differs in different parts of the world and in the Qinghai-Tibetan Plateau, modern animal husbandry practices include an emphasis on the requirements for high quality seasonal and local forage products (Zhuang and Li, 2017) that are produced under conditions of the ecological benefit. Many different plant species are used to make forages for ruminants and an emerging area of potential is the silage production following mixed seeding of different plant species. The purpose of this approach is to (a) utilize the characteristics of stratification of different forage plants that may utilize water and nutrients separately and (b) to produce nutritious high-quality silage. Thus, the planting combination of Gramineous plants (such as cereal crops or grass) and Leguminous plants (such as peas or beans) shows great potential (Cui et al., 2014).
Oat (Avena sativa) is an annual forage crop and a main source of supplementary livestock feed in the cold season in alpine regions. It has strong cold resistance, high yield, and good quality (Dong et al., 2007). Peas (Pisum sativum) fix atmospheric nitrogen, are high-yielding and produce high-quality, high nutritional value, animal feeds, and human foods. Peas are extremely suitable for inclusion in ruminant diets in high-altitude plateau regions because of the excellent planting and growth conditions in the continental monsoon climate zone with long sunshine hours, high atmospheric transparency, strong solar radiation, and abundant light energy resources (Pflueger et al., 2020).
Oat and pea seeding mixtures have been shown to have high productivity (Pflueger et al., 2020) and produce silage, which is a usual low-cost forage processing technology (Muck, 2010), that shows great potential. However, to our knowledge, few studies have used oat-pea mixed forage with different seeding proportions to make silage in the Qinghai-Tibetan Plateau. Therefore, our study aimed to examine the effect of five sowing treatments differing in the seeding proportion of oats and peas and the two maturity stages at harvest, on soil properties and silage quality on the Qinghai-Tibetan plateau.
The study was conducted on 13.5 ha (each plot size was greater than 1 ha, see Figure 1A) of land on a farm in Qinghai Province, China, in the northwest of an area known as the Qinghai-Tibetan plateau. (36.65N, 101.20E, elevation 2,930 m, average temperature 1.0 °C, average annual precipitation 450 mm). The land area was divided into 10 plots, each receiving 150 kg/ha of diammonium phosphate and urea based fertilizer (diammonium phosphate: urea, 1:1). The based fertilizer was turned into the soil before sowing. Plots were randomly assigned to the five sowing treatments of different mixing ratios (10:0, 8:2, 7:3, 5:5, and 0:10 oat to pea ratio) according to the weight of expected forages wet yield of Gramineae and Leguminous plants (Oat, Qingtian No. 1 and Pea, Qingjian No. 1). Oats (O) and Peas (P) in different farm plot were seeded at the corresponding weight (Table 1) from May 12 to 13, 2021. Each plot of land was seeded with a seeder after the seeds were mixed according to the predetermined sowing plan (seed vigor above 95%), with the seeding depth of 6–10 cm, and a roller was used to flatten after sowing (Figure 1C). Five sowing proportions compound two forages harvest periods for oats (at the early flowering stage, from September 7 to 11, S1; the milk ripening stage, from October 11 to 13, S2), resulting in 10 different combinations (Table 1, Figure 1).
Figure 1. Explanations of the 10 plots and photos of the schematic diagram of the experiment. (A) Farm plot (Different number represents different treatment. 1. O0P10 at early flowering stage; 2. O10P0 at the early flowering stage; 3. O0P10 at the milk ripening stage; 4. O10P0 at the milk ripening stage; 5. O5P5 at the early flowering stage; 6. O8P2 at the early flowering stage; 7. O7P3 at the early flowering stage; 8. O5P5 at the milk ripening stage; 9. O8P2 at the milk ripening stage; 10. O7P3 at milk ripening stage); (B) ploughing the soil; (C) seeding; (D) sampling quadrats (large blue 5 × 5 m squares arranges across the plot in a W shape, and small yellow 0.5 × 0.5 m squares). (E) Harvesting; (F) bale silage.
In each plot five 5 × 5 m large quadrats were chosen at the place with the same growth trend according to the “W” shape. Furthermore, five 0.5 × 0.5 m sampling quadrats were set in each large quadrat according to the 5-point sampling method (Figure 1D).
Twenty-five sampling quadrats (Figure 1D) were collected for each farm plot, yielding 125 quadrats for all five farm plots at each harvest time. The stubble on the ground was 15 cm, and all quadrats were harvested manually while the rest were with a combine harvester. Agronomic traits of oats and peas (wet weight yield, dry weight yield, the number of tillers/branches, stalk length of oat/stem length of pea, and natural height of pea) were determined immediately at the sampling time according to Guo et al. (2022).
Before planting and at two harvest times in the autumn of 2021, soil samples from each large quadrat were collected from a depth of 0–30 cm, sieved (< 2 mm) to remove visible fine roots and plant residues, stored at 4°C, and then analyzed according to the procedure described by (Jia Y. et al., 2022). Soil pH and Electro Conductibility (EC) were measured in water (1:2.5 w/v). The organic matter (OM) and cation exchange capacity (CEC) were measured according to Fan et al. (2020) and (Jia R. et al., 2022). Total nitrogen (TN) was measured by the Kjeldahl digestion (FOSS KjeltecTM 2300, FOSS NIR Systems Inc, Denmark). Total potassium (TK), total phosphorus (TP), and alkali-hydro nitrogen (AN), rapidly-available phosphorus (AP) and rapidly-available potassium (AK) were measured according to Shao et al. (2020). The concentrations of exchangeable Na (ANa), Ca (ACa), and Mg (AMg) were tested following the hot block acid digestion protocol (Huang and Schulte, 1985). NH-N and NO3-N were determined according to Fan et al. (2020) using microplate spectrophotometer (Thermo1510, MULTISKAN GO, Thermo Fisher, USA). To calculate the soil quality index (SQI), each soil indicator was converted to a value between 0 and 1, following the procedure described by Zeraatpisheh et al. (2020), Zhou et al. (2020). The overall SQI score was estimated using an SQI-area approach according to the area of a radar diagram yielded by all standard soil indicators (Kuzyakov et al., 2020).
Forages were harvested at S1 (at the early flowering stage of oats, from September 7 to 11) and S2 (the milk ripening stage of oats, from October 11 to 13) stage from each of the eight plots containing Oats, and were chopped by a combine harvester (GR80, LOVOL, China, Figure 1E) into pieces of about 2 cm immediately during harvesting. Lactic acid bacteria (LAB), commercial strains, were purchased from an animal husbandry market (Gansu Pro-Bicon Biotech. Co., Ltd., Lanzhou, Gansu, China) and the forage was added to the water containing LAB (1.0 × 105 colony forming unit/g on a fresh matter basis of forage) after chopping. Then the raw materials were mixed and packed as bales (38 × 40 × 68 cm) at a density of approximately 483.75 kg fresh weight (FW)/m3 and put into the silage bags with the same specification. Wrapping and anaerobic sealing were carried out in the local common way shown in Figure 1F. Triplicate silage bags for each treatment were stored at ambient temperature (1–22°C) and opened for analysis after 7 and 30 days of ensiling.
All fresh silages from each bag were taken out by a forage sampler and then immediately mixed to obtain samples of about 500g by quartering. And among each silage sample, 20g subsamples were blended with sterilized distilled water (w:v = 1:9) for 1 min and filtered through three layers of qualitative filter paper. The filtrate was collected to measure pH. The NH3-N level was measured using the phenol-hypochlorite method (Broderick and Kang, 1980) expressing in proportion to total nitrogen (%TN). Organic acids, such as lactic acid (LA), acetic acid (AA), propionic acid (PA), and butyric acid (BA), were evaluated using a high-performance liquid chromatography method, adjusted from those previously described (column, Shodex RS Pak KC-811; Showa Denko K.K., Kawasaki, Japan; detector, DAD, 210 nm, SPD-20A; Shimadzu Co., Ltd., Kyoto, Japan; eluent, 3 mmol L−1 HClO4; flow speed, 1.0 ml min−1; column oven temperature, 50°C) (Ohmomo, 1993). Dry matter (DM) was measured by oven-drying at 105°C for 3 h (Porter and Murray, 2010). All dried samples were ground in a hammer mill and filtered through a 1 mm screen for other analyses. Crude protein (CP) was measured according to the Association of Official Analytical Chemists (AOAC) International procedures (method 976.05; AOAC., 2000). Contents of neutral detergent fiber (NDF) and acid detergent fiber (ADF) (Van Soest et al., 1991) were measured using an Ankom 2000i fiber analyzer (Ankom Technology Corp., Fairport, NY). Heat-stable α-amylase and sodium sulfite were used in the determination of NDF. Water soluble carbohydrate (WSC) content was determined using the improved anthrone-sulfuric acid assay (Webster, 1992) whereas Ether Extract (EE) was analyzed using an automatic Fat Analyzer (ANKOM XT15i, ANKOM Inc, USA) (AOAC., 2000).
The data were analyzed in a completely randomized 2x5 or 2 × 4 factorial design for Tables 2, 3, or for Table 4, using the Proc MIXED method of SAS (Statistical Analysis System, version 9.3) to determine significant differences among treatments. Mean values were compared using Tukey's test and the level of statistical significance was set to P ≤ 0.05, a very significantly difference was set if P ≤ 0.01. Soil physicochemical properties were ordinated by principal component analysis (PCA) using the R package FactoMineR v1.0.7 (Le et al., 2008).
Table 3. The effect of seeding ratios and maturity stages at harvest on the chemical composition of oats and peas in mixed seeding plantations (n = 5).
Table 4. The effect of ensiling time on fermentation quality and chemical composition of silage made from oats and peas planted in mixed seeding plantations (n = 3).
The soil physicochemical properties that were measured were not affected (p > 0.05) by the seeding ratio (Table 2) while harvesting stage significantly affected soil pH, EC, TP, TK, AN, and NO3-N (p < 0.05). The overall soil pH and TP were higher at the early flowering harvest stage (S1) whereas EC, TK, AN, and NO3-N were higher at the milk ripening harvest stage (S2). Seeding ratio by harvesting stage interaction effects were detected in exchangeable Na (Ana) and exchangeable Mg (Amg) with the highest and lowest Ana in O8P2 at S2 and O7P3 at S1, respectively (p = 0.025) and highest and lowest Amg in O5P5 at S2 and O8P2 at S1, respectively (p = 0.036). This is also shown in the PCA plots for the difference between harvest stages (Figure 2) and among seeding ratios within the harvest stage (Figure 3).
Figure 2. Principal component analysis (PCA) based on soil physicochemical properties as variables. The samples of the same harvest stage were outlined and grouped in different colors.
Figure 3. Principal component analysis (PCA) based on soil physicochemical properties as variables. The samples of the same mixed seeding ratio from the early flowering stage (A) and milk ripening stage (B) were outlined and grouped in different colors. Only Peas: O0P10; Only Oats: O10P0.
At the milk ripening stage, SQI comparison (Figure 4) between different mixed seeding ratios showed that the O5P5 group had higher soil quality parameters than others (although not significant).
Figure 4. The radar graphs show the relative responses of soil properties to mixed seeding ratios at the early flowering stage and milk ripening stage. Only Peas: O0P10; Only Oats: O10P0.
Table 3 shows that the yield and chemical composition of mixed seeding forage was affected to varying degrees by different seeding ratios, harvesting stage, and seeding ratio by harvesting stage interaction. The highest wet weight yield was in O10P0 at S1 and the lowest was in O5P5 at S1 for Oats whereas O0P10 had the highest wet yield of Peas in S1 and S2. There was no significant difference in the dry weight yield of peas at S1 and oats at S2 of mixed seeding treatment groups. However, the highest dry weight yield for oats was shown at S2 in the single seeding group (O10P0). For the CP content at S2, the O7P3 group had the highest protein content in both oats and peas. In each planting pattern, the EE contents of both peas and oats were higher at S2 than at S1; the opposite was detected for NDF and ADF. The WSC content of oats decreased significantly (p < 0.05) with increasing ratio of pea seeding both at S1 and S2. In addition, the O5P5 group at S1 showed the highest ash content in all samples (p < 0.05).
The agronomic traits of mixed seeding forage are in Table 3. Except for single seeding (O10P0 and O0P10), the maximum number of tillers/branches of peas was in O5P5 at S1, while it was in O8P2 at S2 for oats. As far as the AH and NH were concerned, the O5P5 group also got the best results in mixed groups, and the S2 was better than S1. The seeding rate by stage of harvest interaction effects showed that O5P5 at S1 had the highest Ash contents of oats and peas and ADF content of peas, compared to all groups. O5P5 at S2 had the highest NDF and AH contents of peas. O7P3 at S1 had the highest NDF and ADF contents of oats and DM and NDF contents of peas (p < 0.05).
After ensiling, all fermentation quality indicators (pH value, contents of LA, AA, PA, LA/AA ratio, and NH3-N) and fermentation quality and chemical composition (DM, CP, NDF, ADF, WSC, and ash) were affected by varying degrees by different treatments (Table 4). After storage for 7 days (d), both mixed seeding ratio and harvest stage caused very significant differences (p < 0.01) in all the contents of LA, PA, DM, CP, NDF, WSC, ash, and LA/AA ratio, while very significant interaction effects occurred in the pH value, contents of LA, NDF, WSC, and ash (p < 0.01). However, for 30 d silage samples, only the AA and ash contents were very significantly affected (p < 0.01) by mixed seeding ratio and harvest stage among all fermentation quality and chemical composition parameters. For the four organic acids, PA was not detected in some treatment groups, but there were significant differences (p < 0.05) in the contents of LA, AA, and PA. Interestingly, O8P2 and O5P5 groups at S1 rather than O10P0 had the highest LA content in both storage times (p < 0.05). The O8P2 and O5P5 groups at S1 groups also resulted in higher AA content (especially greater than 3.6% DM at storing for 30 d). Overall, the LA/AA ratio of S2 samples was less than 2.00. At 7 and 30 d of ensiling, the O10P0 group at S1 showed the lowest NH3-N content (p < 0.05), while the treatment at S2 after 7d was abnormally high.
The O10P0 group had the highest DM content in all treatments at 7 and 30 d, and O5P5 showed the highest CP content (except ensiling for 30 days in S1), which indicated the CP content of oat-pea silage generally increased with the proportion of peas in mixed seeding at the same harvest time. As far as cellulose was concerned, the NDF and ADF contents in silages seem mainly contributed by oats. Single seeding oats at S1 had higher NDF and ADF contents than at S2, which was also observed in most groups with the same mixed seeding ratio after storage for 7 or 30 d. There was lower WSC content after ensiling for 30 d than for 7 d (except O8P2 and O7P3 groups S1).
Seeding ratio by stage of harvest interaction effects were detected in all the fermentation quality and chemical composition indicators except in PA and DM after 7 days of ensiling, whereas after 30 days of ensiling, interaction effects were detected only in AA, WSC, and Ash contents (p<0.05). After 7 days of ensiling, O5P5 at S1 had the highest LA, AA, LA/AA, and CP contents compared to all groups. O10P0 at S1 had the highest contents of NDF, ADF, and Ash while O10P0 at S2 had the highest contents of NH3-N and WSC. After 30 d of ensiling, O5P5 at S2 had the highest contents of AA whereas O5P5 at S1 and O7P3 at S1 had the highest Ash and WSC contents, respectively.
The present study explored the silage production from mixed planting of two forage species, Leguminous and Gramineous, suitable for cultivation on the Qinghai-Tibetan Plateau. The results demonstrated that, using oats and peas, nutritional quality of silage and utilization potential can be improved by varying the mixed seeding ratio and the harvest time, and that they are suitable for producing high-quality silages for livestock.
Mixed sowing of Leguminous and Gramineous plants is considered an effective approach to improving total biomass and soil fertility (Berdahl et al., 2001; Tekeli and Ates, 2005; Salama et al., 2020). Our results indicated that although mixed seeding could alter the availability of some nutrients, it could not comprehensively change the soil quality. By scaling at the mixed seeding ratio, the O8P2 group obtained the highest total wet weight yield among the mixed seeding groups. Previous studies have found that the mixed sowing of peas and oats is a successful model (Lauk and Lauk, 2008). Similar to the results of our study, the maximum yield per unit area comes from pure crop (single seeding) plots but mixing with peas decreased the amount of additional nitrogen required. Specifically, they found that the addition of peas to oats resulted in a significant increase in protein content in cereals. Moreover, mixed seeding promoted tillering of oats, which may be due to reduced competition from grass weeds (Arlauskiene et al., 2021). Due to peas' chemical composition, the mixture was superior to single grain oat in protein yield (Soufan and Al-Suhaibani, 2021). Furthermore, the co-sowing of peas and oats has also been shown to have application potential in organic agriculture due to a relatively good harvest and high protein yield in the soil without fertilizer (Candelaria-Morales et al., 2022). This might provide a possible feed source for the organic production of dairy and meat products.
Several factors may be attributable to the characteristics of co-sowing pea-oat silages in our study. Nitrogen ammoniation during silage due to high moisture content was of concern. Zhang et al. (2020) showed that the NH3-N/TN (NH3-N concentration expressed in TN%) of silage had a strong negative correlation with the DM intake of livestock. Thus, the content of ammonia nitrogen directly determined the feeding value of the silage. In the O5P5 group, regardless of harvesting and ensiling at S1 or S2, the DM content was always lower than in other treatments. At the same time, NH3-N concentration was at a worrisome high level in these silages unexpectedly, even though the samples had low pH values and high LA content, that may explain the higher AA content of O8P2 and O5P5 groups at S1 groups. Silage with low moisture content delays the growth of anaerobic microorganisms (Mariotti et al., 2020), thus reducing the speed and ability of sugar to convert into organic acids (Zhang et al., 2014). When the moisture content of the silage was high, Clostridium spp. would multiply, and butyric acid, ammonia nitrogen, and other harmful substances would produce in large quantities, thus affecting the quality of silage fermentation (Zheng et al., 2018; Du et al., 2022). Therefore, successful ensiling fermentation by lactic acid bacteria can only be obtained if the water content is appropriate. Weinberg and Ashbell (2003) demonstrated that when the DM content of silage raw materials is high (the moisture content is low), the inhibitory effect on the activity of lactic acid bacteria and other microorganisms increases so that the silage cannot quickly reach a stable state.
Previous studies have attributed successful ensiling to the contribution of LAB (Muck, 2010). More studies have proved that LAB is the dominant fermentation in high-quality silage (Dunière et al., 2013). Compared with spontaneous fermentation, the exogenous addition of LAB has become a necessary part of silage production (Kung et al., 2018). In this study, all treatments were applied with the same LAB specie in equal loadings to accommodate subsequent animal production needs. Therefore, the difference in fermentation quality may be mainly due to the available substrates of LAB. Low pH and high LA content were key indexes indicating good silage quality. Results of our study showed that the ratio of forage materials seeding with O8P2 at S1 had higher WSC content than other mixing ratios, resulting in a lower pH value of silage samples in this treatment group. In addition, the lower WSC residuals and higher LA content after storage for 7 and 30 d also suggested that superior LAB had a better utilization effect on WSC during ensiling in this group (Zhang et al., 2017). In addition, the increasing ash content might be due to the chemical composition of raw material peas.
Our study found that the forages of the oat-pea mixed seeding altered the availability of soil nutrients and obtained the best fermentation quality at the O8P2 and O5P5 ratios on the Qinghai-Tibetan Plateau. The O5P5 ratio is further recommended as a desirable oat-pea mixed seeding silage production strategy giving the highest crude protein content.
The raw data supporting the conclusions of this article will be made available by the authors, without undue reservation.
Written informed consent was obtained from the individual(s) for the publication of any identifiable images or data included in this article.
Conceptualization: DB and AE. Methodology, formal analysis, and writing—original draft preparation: WL. Software and visualization: ZL. Validation: LZ and YakC. Investigation: YanC. Resources: LZ. Data curation: YS. Writing—review and editing, supervision, project administration, and funding acquisition: DB. All authors contributed to the article and approved the submitted version.
This research was funded by the National Key Research and Development Program of China (No. 2022YFD1301002), the Agriculture Science and Technology Innovation Program (ASTIP-IAS07-1), and Beijing Innovation Consortium of Livestock Research System (BAIC05-2022).
Thanks to the staff of Qinghai experimental base.
The authors declare that the research was conducted in the absence of any commercial or financial relationships that could be construed as a potential conflict of interest.
All claims expressed in this article are solely those of the authors and do not necessarily represent those of their affiliated organizations, or those of the publisher, the editors and the reviewers. Any product that may be evaluated in this article, or claim that may be made by its manufacturer, is not guaranteed or endorsed by the publisher.
AOAC. (2000). Official Methods of Analysis. Gaithersburg, MD, USA: The Association of Official Analytical Chemists.
Arlauskiene, A., Jablonskyte-Rašče, D., Šarunaite, L., Toleikiene, M., Masilionyte, L., Gecaite, V., et al. (2021). Perennial forage legume cultivation and their above-ground mass management methods for weed suppression in arable organic cropping systems. Chem. Biol. Technol. Agric. 8, 34. doi: 10.1186/s40538-021-00228-5
Berdahl, J. D., Karn, J. F., and Hendrickson, J. R. (2001). Dry matter yields of cool-season grass monocultures and grass–alfalfa binary mixtures. Agron. J. 93, 463–467. doi: 10.2134/agronj2001.932463x
Broderick, G. A., and Kang, J. H. (1980). Automated simultaneous determination of ammonia and total amino acids in ruminal fluid and in vitro media1. J. Dairy Sci. 63, 64–75. doi: 10.3168/jds.S0022-0302(80)82888-8
Candelaria-Morales, N. P., Grossman, J., Fernandez, A., and Rogers, M. (2022). Exploring multifunctionality of summer cover crops for organic vegetable farms in the upper midwest. Renew. Agric. Food Syst. 37, 198–205. doi: 10.1017/S1742170521000545
Cui, G. W., Li, H. Y., Sun, T., Wang, Z., and Xi, L. Q. (2014). An experimental study of variety screening, sequential cropping, compaction and mixed cropping techniques for the cultivation of annual forage crops in agro-pastoral area of tibet, china. Int. J. Agric. Biol. 16, 97–103. Available online at: https://www.fspublishers.org/
Dong, S. K., Kang, M. Y., Yun, X. J., Long, R. J., and Hu, Z. Z. (2007). Economic comparison of forage production from annual crops, perennial pasture and native grassland in the alpine region of the qinghai-tibetan plateau, china. Grass Forage Sci. 62, 405–415. doi: 10.1111/j.1365-2494.2007.00594.x
Du, Z., Lin, Y., Sun, L., Yang, F., and Cai, Y. (2022). Microbial community structure, co-occurrence network and fermentation characteristics of woody plant silage. J. Sci. Food Agric. 102, 1193–1204. doi: 10.1002/jsfa.11457
Dunière, L., Sindou, J., Chaucheyras-Durand, F., Chevallier, I., and Thévenot-Sergentet, D. (2013). Silage processing and strategies to prevent persistence of undesirable microorganisms. Animal Feed Sci. Technol. 182, 1–15. doi: 10.1016/j.anifeedsci.2013.04.006
Fan, X., Han, M., Wang, L., Li, X., and Zhou, J. (2020). Analysis of water quality change and its driving factors of the xiaoqing river estuary in recent ten rears. Environ. Sci. 41, 1619–1628. doi: 10.13227/j.hjkx.201909074
Guo, C., Wang, W., Pu, X., Duan, N., Wei, X., Peng, D., et al. (2022). The current situation and prospect of the integration of forage industry, agricultural machinery and agronomy in qinghai province. Acta Agrestia Sinica 30, 2483–2491. doi: 10.13594/j.cnki.mcjgjx.2020.01.005
Huang, C. Y. L., and Schulte, E. E. (1985). Digestion of plant tissue for analysis by icp emission spectroscopy. Commun. Soil Sci Plant Analy. 16, 943–958. doi: 10.1080/00103628509367657
Jia, R., Zhou, J., Chu, J., Shahbaz, M., Yang, Y., Davey, L., et al. (2022). Insights into the associations between soil quality and ecosystem multifunctionality driven by fertilization management: a case study from the north china plain. J. Cleaner Prod. 362, 132265. doi: 10.1016/j.jclepro.2022.132265
Jia, Y., Li, J., Zeng, X., Zhang, N., Wen, J., Liu, J., et al. (2022). The performance and mechanism of cadmium availability mitigation by biochars differ among soils with different ph: hints for the reasonable choice of passivators. J. Environ. Manage. 312, 114903. doi: 10.1016/j.jenvman.2022.114903
Kung, L., Shaver, R. D., Grant, R. J., and Schmidt, R. J. (2018). Silage review: interpretation of chemical, microbial, and organoleptic components of silages. J. Dairy Sci. 101, 4020–4033. doi: 10.3168/jds.2017-13909
Kuzyakov, Y., Gunina, A., Zamanian, K., Tian, J., Luo, Y., Xu, X., et al. (2020). New approaches for evaluation of soil health, sensitivity and resistance to degradation. Front. Agric. Sci. Eng. 7, 282–288. doi: 10.15302/J-FASE-2020338
Lauk, R., and Lauk, E. (2008). Pea-oat intercrops are superior to pea-wheat and pea-barley intercrops. Acta agriculturae Scandinavica. Section B, Soil Plant Sci. 58, 139–144. doi: 10.1080/09064710701412692
Le, S., Josse, J., and Husson, F. (2008). Factominer: an r package for multivariate analysis (article). J. Stat. Softw. 25, 1–18. doi: 10.18637/jss.v025.i01
Mariotti, M., Fratini, F., Cerri, D., Andreuccetti, V., Giglio, R., Angeletti, F. G. S., et al. (2020). Use of fresh scotta whey as an additive for alfalfa silage. Agronomy 10, 365. doi: 10.3390/agronomy10030365
Muck, R. E. (2010). Silage microbiology and its control through additives. Rev. Brasileira De Zootecnia 39, 183–191. doi: 10.1590/S1516-35982010001300021
Ohmomo, S. (1993). Analysis of organic acids in silage by high-performance liquid chromatography. Bull. Natl. Grassl. Res. Inst. 48, 51–56.
Pflueger, N. P., Redfearn, D. D., Volesky, J. D., Bolze, R., and Stephenson, M. B. (2020). Influence of oat and spring pea mixtures on forage characteristics in different environments. Agronomy J. 112, 1911–1920. doi: 10.1002/agj2.20144
Porter, M. G., and Murray, R. S. (2010). The volatility of components of grass silage on oven drying and the inter-relationship between dry-matter content estimated by different analytical methods. Grass Forage Sci. 56, 405–411. doi: 10.1046/j.1365-2494.2001.00292.x
Salama, H. S. A., Khalil, H. E., and Nawar, A. I. (2020). Utilization of thinned sunflower and soybean intercrops as forage: a useful strategy for small scale farms in intensive agricultural systems. Int. J. Plant Prod. 14, 487–499. doi: 10.1007/s42106-020-00099-0
Shao, T., Zhao, J., Liu, A., Long, X., and Rengel, Z. (2020). Effects of soil physicochemical properties on microbial communities in different ecological niches in coastal area. Appl. Soil Ecol. 150, 103486. doi: 10.1016/j.apsoil.2019.103486
Soufan, W., and Al-Suhaibani, N. A. (2021). Optimizing yield and quality of silage and hay for pea–barley mixtures ratio under irrigated arid environments. Sustainability 13, 13621. doi: 10.3390/su132413621
Tekeli, A. S., and Ates, E. (2005). Yield potential and mineral composition of white clover (trifolium repens l.)-Tall fescue (festuca arundinacea schreb.) Mixtures. J. Central Eur. Agric. 25, 27–34. doi: 10.5513/jcea.v6i1.243
Van Soest, P. J., Robertson, J. B., and Lewis, B. A. (1991). Methods for dietary fiber, neutral detergent fiber, and nonstarch polysaccharides in relation to animal nutrition. J. Dairy Sci. 74, 3583–3597. doi: 10.3168/jds.S0022-0302(91)78551-2
Webster, J. (1992). “The biochemistry of silage (second edition),” in P. Mcdonald, A. R. Henderson and S. J. E. Heron (Marlow, Bucks, UK: Chalcombe Publications) (1991) 340. doi: 10.1017/S0014479700023115
Weinberg, Z. G., and Ashbell, G. (2003). Engineering aspects of ensiling. Biochem. Eng. J. 13, 181–188. doi: 10.1016/S1369-703X(02)00130-4
Zeraatpisheh, M., Bakhshandeh, E., Hosseini, M., and Alavi, S. M. (2020). Assessing the effects of deforestation and intensive agriculture on the soil quality through digital soil mapping. Geoderma 363, 114139. doi: 10.1016/j.geoderma.2019.114139
Zhang, G., Li, Y., Fang, X., Cai, Y., and Zhang, Y. (2020). Lactation performance, nitrogen utilization, and profitability in dairy cows fed fermented total mixed ration containing wet corn gluten feed and corn stover in combination replacing a portion of alfalfa hay. Animal Feed Sci. Technol. 269, 114687. doi: 10.1016/j.anifeedsci.2020.114687
Zhang, Q., Zhao, M., Wang, X., Yu, Z., and Na, R. (2017). Ensiling alfalfa with whole crop corn improves the silage quality and invitro digestibility of the silage mixtures. Grassland Sci. 63, 211–217. doi: 10.1111/grs.12168
Zhang, X. Q., Jin, Y. M., Zhang, Y. J., Yu, Z., and Yan, W. H. (2014). Silage quality and preservation ofurtica cannabina ensiled alone and with additive treatment. Grass Forage Sci. 69, 405–414. doi: 10.1111/gfs.12036
Zheng, M., Niu, D., Zuo, S., Mao, P., Meng, L., and Xu, C. (2018). The effect of cultivar, wilting and storage period on fermentation and the clostridial community of alfalfa silage. Italian J. Animal Sci. 17, 336–346. doi: 10.1080/1828051X.2017.1364984
Zhou, Y., Ma, H., Xie, Y., Jia, X., Su, T., Li, J., et al. (2020). Assessment of soil quality indexes for different land use types in typical steppe in the loess hilly area, china. Ecol. Indic. 118, 106743. doi: 10.1016/j.ecolind.2020.106743
Keywords: oat, pea, mixed seeding, silage, harvest stage
Citation: Liu W, Zhao L, Chen Y, Shen Y, Luo Z, Chen Y, Evans ACO and Bu D (2023) Soil properties and silage quality in response to oat and pea seeding ratios and harvest stage on the Qinghai-Tibetan Plateau. Front. Sustain. Food Syst. 7:1143431. doi: 10.3389/fsufs.2023.1143431
Received: 13 January 2023; Accepted: 10 February 2023;
Published: 28 February 2023.
Edited by:
Yuan Li, Lanzhou University, ChinaReviewed by:
Huang Ding, China Agricultural University, ChinaCopyright © 2023 Liu, Zhao, Chen, Shen, Luo, Chen, Evans and Bu. This is an open-access article distributed under the terms of the Creative Commons Attribution License (CC BY). The use, distribution or reproduction in other forums is permitted, provided the original author(s) and the copyright owner(s) are credited and that the original publication in this journal is cited, in accordance with accepted academic practice. No use, distribution or reproduction is permitted which does not comply with these terms.
*Correspondence: Dengpan Bu, YnVkZW5ncGFuQGNhYXMuY24=
Disclaimer: All claims expressed in this article are solely those of the authors and do not necessarily represent those of their affiliated organizations, or those of the publisher, the editors and the reviewers. Any product that may be evaluated in this article or claim that may be made by its manufacturer is not guaranteed or endorsed by the publisher.
Research integrity at Frontiers
Learn more about the work of our research integrity team to safeguard the quality of each article we publish.