- 1Department of Agricultural Sciences, University of Helsinki, Helsinki, Finland
- 2Department of Food and Nutrition, University of Helsinki, Helsinki, Finland
Lettuce (Lactuca sativa L.) may accumulate high amounts of nitrate
Introduction
Lettuce (Lactuca sativa L.) contains essential vitamins, minerals, and health-promoting phytochemicals, such as carotenoids and polyphenols (Kimura and Rodriguez-Amaya, 2003; Kim et al., 2016). However, when lettuce is consumed in large quantities, the consumers’ recommended acceptable daily intake (ADI) of may be exceeded (European Food Safety Authority, 2008). Since is classified as a harmful food additive in the EU, the European Commission has set a maximum content for fresh lettuce in regulation 194/97 and requires the Member States to monitor the content of commercial lettuce. If the content in lettuce exceeds the set maximum the product will be rejected by traders. Thus, methods for controlling the content in lettuce leaves remain a major issue in commercial greenhouse production.
Commercial greenhouse-grown lettuce is commonly produced hydroponically in a continuous process, using the Nutrient Film Technique (NFT). In the NFT system, seedlings grow in small plugs of a growth substrate that are placed in narrow channels in which the nutrient solution flows continuously. Continuous production means a system in which the growth channels are set on a long table and continuously moved along the table until the plants reach commercial size. In this method, the plants have unlimited access to all the nutrients they need. Hence, lettuce may accumulate high amounts of . Lettuce takes up by roots from the nutrient solution. In the plant cell, the is reduced to nitrite and further assimilated into amino acids and proteins or stored in the cells divided into the vacuole and cytoplasm, as reviewed by Tischner (2000). Growing conditions, plant age, and fertilization affect the rate of assimilation (Blom-Zandstra, 1989; Santamaria et al., 1998; Zhang et al., 2018; Yi et al., 2020), and if uptake exceeds the rate of assimilation, accumulates in the vacuoles, resulting in high content in the leaves.
Many have expressed great interest in the means used to regulate the content of leafy vegetables. Especially the various fertilization strategies (Santamaria et al., 1998; Tabaglio et al., 2020). The effects of light intensity and spectrum on lettuce assimilation and content have also been of interest to researchers (Liu et al., 2016; Zhang et al., 2018; Yi et al., 2020). Reducing the concentration in the nutrient solution several days before harvest, replacing in part with ammonium ( ) at the end of the growing period, and removing the oldest leaves during the harvest, are methods previously tested in the commercial scale production of leafy vegetables (Santamaria et al., 1998, 2001). However, the use of in the nutrient solution has been associated with stunted growth and reduced accumulation of fresh weight (FW) and leaf area (Santamaria et al., 1998; Hoque et al., 2008). The may also damage leaves and roots (Hoque et al., 2008). Moreover, removing the oldest leaves during the harvest increases the workload and causes crop losses throughout the production chain. Light promotes photosynthesis and affects lettuce growth and metabolism. While growing lettuce indoors under a controlled climate and light-emitting-diode (LED) light, regulation of the light spectrum and photoperiod can be used to control lettuce quality (Viršilė et al., 2019). However, when growing lettuce in a greenhouse, especially in the Nordic countries, the proper light conditions may become unattainable, and therefore an additional precise method for regulating product quality is needed.
Osmolyte applications provide a novel approach to controlling lettuce content (Jokinen et al., 2022). Plants accumulate osmolytes (e.g., sugars, sugar alcohols, and compounds) to maintain cell osmotic potential (Burns et al., 2010).The taken up by roots can replace these assimilates if photosynthesis is limited, which partly explains the increase in lettuce content under un-optimal growing conditions (Blom-Zandstra, 1989; Burns et al., 2010). Exogenous application of osmolytes, such as glucose and sucrose decreased uptake in pak choi (Brassica rapa var. chinensis L.) (Ma et al., 2018). Furthermore, Jokinen et al. (2022) found that lettuce takes up root-applied glycinebetaine (GB), while GB treatment reduced content up to 40%, increased dry matter (DM) content, and affected the mineral -and amino acid profile in lettuce. In addition, Jokinen et al. (2022) reported that GB was stored in lettuce leaves in a concentration-dependent manner, while the leaf GB content was negatively correlated with leaf content.
Glycinebetaine (N, N′, N″ – trimethylglycine) is a quaternary ammonium compound that is endogenously accumulated in many higher plants (Wyn Jones and Storey, 1981; Rhodes and Hanson, 1993), and thus GB is a natural part of the human diet. However, lettuce does not accumulate endogenous GB (Jokinen et al., 2022). Glycinebetaine is considered a health-promoting compound in the human diet (Hayes et al., 2003; Craig, 2004). A dietary supplement GB reduced the plasma homocysteine concentration (Schwab et al., 2002), which is involved in a lower risk of cardiovascular diseases (Cho et al., 2006). Glycinebetaine also positively affects the gut microbiota (Koistinen et al., 2019).
Here we investigated the effect of GB on lettuce content and nutritional quality in commercial-scale hydroponic cultivation. We further focused the impact of GB on lettuce growth and adjusted the GB application to fit that of a commercial-scale continuous NFT-cultivation system. We hypothesized that lettuce accumulates GB from the nutrient solution reducing its growth while the edible quality (i.e., higher amino acid-and mineral content and lower content) of lettuce may be improved. The experiments were conducted in Finland in a commercial greenhouse during the winter and spring of 2020. This study consisted of three experiments, using the same greenhouse facilities and experimental design.
Materials and methods
Cultivation
Lettuce (cv. ‘Danstar’ or ‘Frillice’) seeds were sown in plastic pots (Ø 5 cm 0,08 L, PR30, VEFI EUROPE, (Skierniewice, Poland) VEFI Drammen, Norway) filled with fine peat (pH 6.0, electrical conductivity (EC) 0.21 S/m, Kekkilä Professional, VHM 620 R8060 + iron (Fe), Kekkilä Oy, Vantaa, Finland) at a rate of one seed per pot. The seeds were germinated in a climate-controlled dark room at 19°C for 1 day and transferred into a seedling line in a greenhouse under automatically controlled (Priva Connex; De Lier, Netherlands) climatic conditions. The day/night temperature was 20–24°C/17–18°C, and the relative humidity (RH %) fluctuated between 48 and 77%. In the first two experiments, conducted in January and February (2020), artificial high-pressure sodium (HPS) lights (average intensity 160 μmol m−2 s−1) were used, and the day/night light period was set to 20/4 h. Artificial lights were not used in the third experiment conducted in May 2020, because there was already enough sunlight available, and the day length in Finland was over 16 h.
For seedlings over the first 15 days, the nutrient solution was prepared by mixing a 2.5% stock solution of Professional Superex Farm Edition (Nitrogen-Phosphorus-Potassium (N-P-K) 10–3-30, Mg 1.7, Kekkilä Professional, Kekkilä Oy, Vantaa, Finland) and 2.5% stock solution of calcium nitrate (Ca (NO3)215.5–26.3, Van Iperen, International B.V.,Westmaas, The Netherlands). The stock solutions were mixed in a 1:1 ratio and automatically added to the irrigation water according to the demand for EC, which was set to 0.11 S/m. The seedlings were watered with an irrigation ramp and spray nozzles. After the 15-day growing period in the seedling line, well-developed seedlings were transplanted to the NFT-channel tables for channel cultivation. The channel tables were 100 m long and 4 m wide. Each channel had 32 pots and the plant density was 25.6 plants m−2. The seedlings were set in and harvested at the opposite ends of the channel table at the rate of 10 channels per day, hence the channels were moved forward along the table once per day. In general, the growing period from seeding to harvest fluctuated between 40 and 43 days.
Two fertigation stock solutions were manually prepared in separate tanks for channel cultivation. Solution A contained 100 kg 1,000 L−1 Kekkilä Professional Superex Farm Edition, and solution B 25 kg 1,000 L−1 Ca (NO3). Stock solutions A and B were automatically added in equal amounts to the circulating nutrient solution reaching the EC demanded. The automatically regulated irrigation term took place after every 45 min. The EC of the circulating nutrient solution was set to 0.21 S/m in the first two experiments, and 0.18 S/m in the third experiment. The concentration of nitrate nitrogen (NO3 –N) in the nutrient solution fluctuated between 180 and 200 mg L−1. After every irrigation pulse, the returning water was filtered through sand (3–5 mm) and rock wool biofilters and led to a basin; the basin water was re-used. During the irrigation pulse, the greenhouse automatic (Vialux Hd/UV system, Priva, De Lier, Netherlands) adjusted the EC in the nutrient solution by adding fertilizer stock solutions or pure water disinfected with an ultraviolet (UV) filter.
Experimental design
The experimental area consisted of two adjacent NFT-channel tables in the middle of the block greenhouse. Table A was set up as the control table and table B was set up for GB treatment. Table A was connected to the automatic irrigation system generally used in the greenhouse. The channel table used for GB treatment was split into two water recycling sections (Figure 1). The first section was connected to the greenhouse’s general irrigation system, and the plants were grown in it for approx. 20 days. Thereafter, the plants reached the treatment area where they were grown for 6 days before the harvest. The GB-treatment section consisted of 144 channels totaling 4,608 plants. A water tank (3,000 L) containing nutrient solution was connected to the GB-treatment section for automatic irrigation (Siemens Logo 8, Siemens AG, Munich, Germany), and the irrigation pulse was set at 45 min intervals corresponding to the irrigation interval used for the control plants.
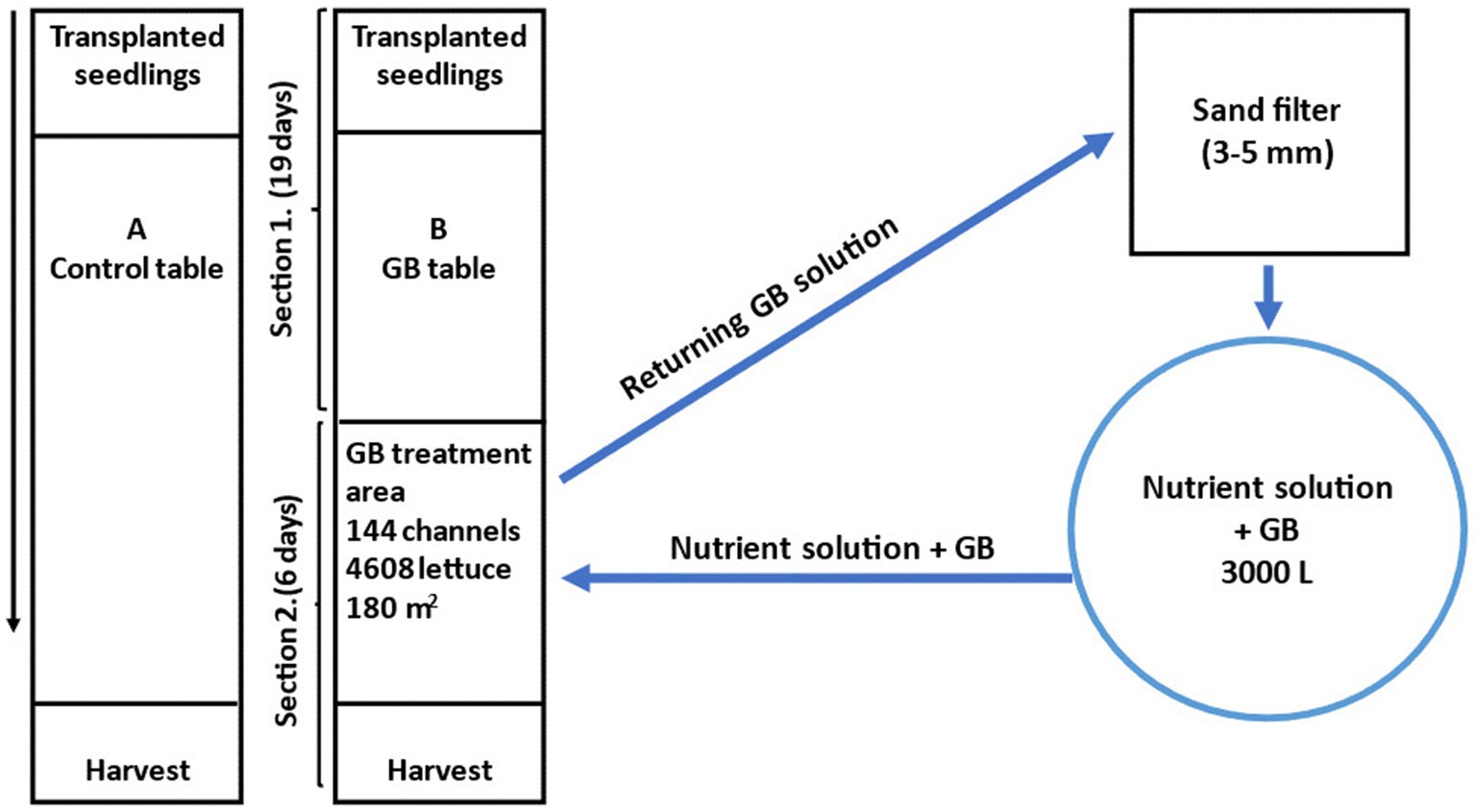
Figure 1. Floor plan of the glycinebetaine (GB) experiment. Lettuce (Lactuca sativa L.) seedlings were transplanted in NFT-channel cultivation at 15 DAS and let grow until harvest size (> 100 g) was reached. The channels were moved along the tables continuously; every day 10 channels of lettuce were harvested, and new seedlings were put in. The GB solution was let into the treatment area through a separate water recycling system. The plants reached the treatment area approx. 34 DAS and were harvested at 40–43 DAS. DAS = days after seeding. NFT = Nutrient Film Technique.
At the beginning of the experiments, the water tank (3,000 L) was filled with the EC-adjusted nutrient solution. In the first two experiments, the EC was set to 0.21 S/m, and in the third experiment to 0.18 S/m. Glycinebetaine (Nutristim pure, Finnfeeds Finland Oy, Naantali, Finland) was applied in the tank by first dissolving it in small batches in 10 L of nutrient solution. The GB-containing nutrient solution was then mixed with an underwater pump (Grundfos Unilit KP 250, Grundfos Holding A/S, Bjerringbro, Denmark) for 1 h before it was led to the channels. Water consumption and the EC of the tank solution were monitored daily (Senmatic EC Mesur hand instrument, Senmatic A/S Industrivej, Søndersø, Denmark). The fertilizer stock solutions were manually added to the tank to maintain EC demand. In the third experiment, the GB application was repeated 4 days after the first application, for which that the tank was refilled with fresh nutrient solution and GB was applied as described above.
Glycinebetaine treatment
In the first two experiments, the GB was applied once in the nutrient solution followed by an experimental period of 6 days. The GB concentration was set to 4 mM in the first experiment, and 7.5 mM in the second. In the third experiment, two GB applications (double-application) were used: the first dose of GB resulting in a10 mM GB concentration in the solution was applied at the beginning of the experiment, and a second 10 mM dose of GB was applied 4 days later to continuously maintain the GB available for the plants (Figure 2). During the second GB application, the tank was refilled with fresh nutrient solution. The third experiment lasted 14 days. Thus, the channels were moved daily for the harvest, and the GB concentration in the nutrient solution was reduced over time, plants arriving at the treatment area were exposed to GB at different concentrations and times.
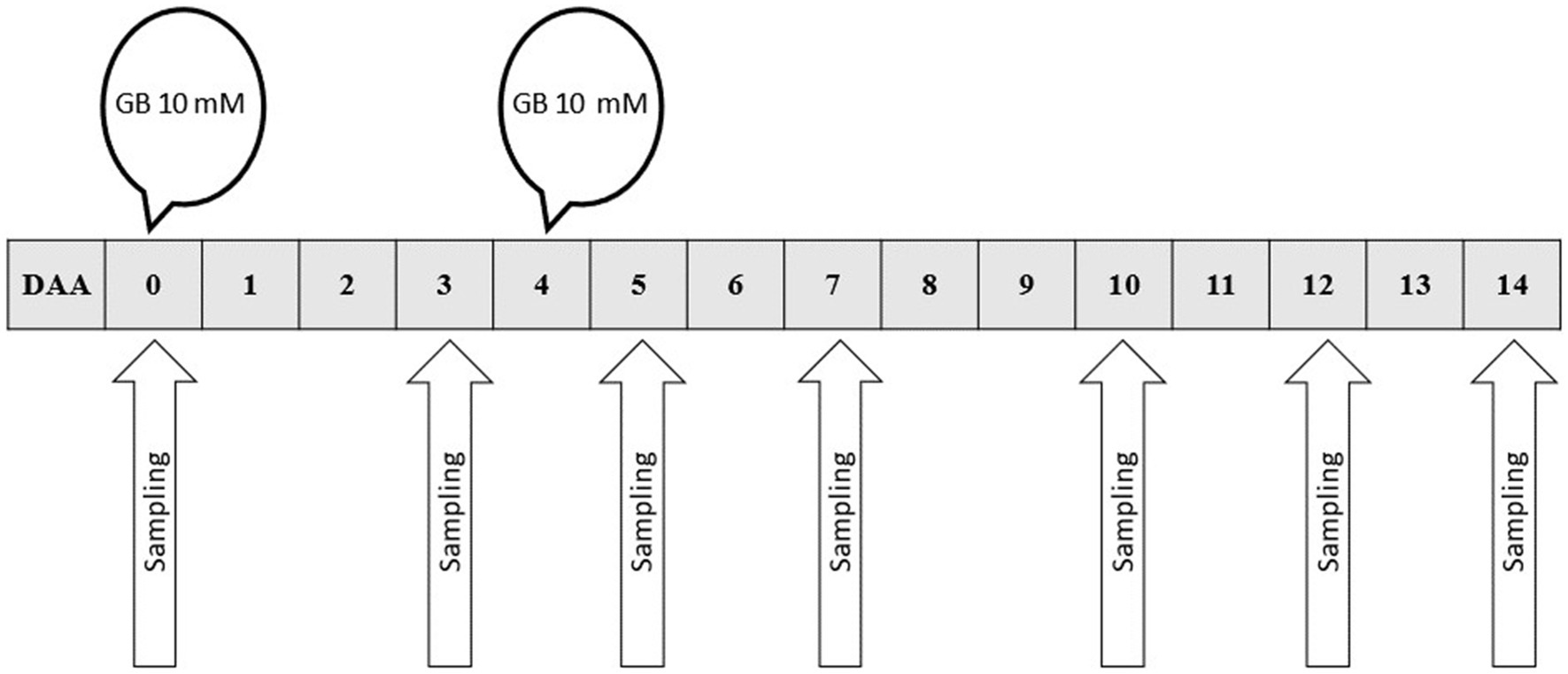
Figure 2. Experimental protocol with GB double-applications (10 + 10 mM). The plants were sampled at different times (DAA) and were grown in GB-containing system as follows; 0, no GB; 3, one GB dose 3 days before harvest (DBH); 5, GB dose five and one DBH; 7, GB dose seven and three DBH; 10, GB dose 10 and 6 DBH; 12, GB dose 12 and 8 DBH; 14, GB dose 14 and 10 DBH. DAA = days after application. GB = glycinebetaine.
Plant sampling
In the first two experiments, the sampling was conducted daily over the 0–5 days after GB application (0–5 DAA). In the third experiment, the sampling was conducted at 0, 3, 5, 7, 10, 12, and 14 DAA. The plants were harvested at 40–43 days after seeding (DAS) when they had reached 100–200 g FW. At each sampling time at 10:00 am, one plant from the middle of each of the 10 channels was collected totaling 10 plants from the GB growing table and 10 plants from the control table. The plants were cut on the surface of the peat, and the lettuce head was weighed, and frozen for at least 2 s at −20°C. The nutrient solution was sampled simultaneously with plant samples from both lines and frozen for 24 h at −20°C.
Nitrate content in lettuce leaves
The content in the lettuce leaves was measured with a Horiba LAQUA Twin 741 crops meter (Horiba Advanced Techno Co. Ltd., Kyoto, Japan). In brief, six frozen samples of each of the sampling times were allowed to thaw at 5°C overnight followed by at least 2 h at room temperature. The thawed samples were squeezed with a potato press. The content was measured four times from each liquid sample and the average of measurements was multiplied by a correction factor of 0.77 according to Näkkilä et al. (2015). The meter was calibrated after every fourth measurement using a two-point calibration procedure with Y042 300 parts per million (ppm) and Y041 5,000 ppm Nitrate Ion Standard Solutions (Horiba).
Chemical analyses
All the chemical analyses conducted in this study were earlier used and described in Jokinen et al. (2022). In brief, the remaining frozen plants from the third experiment (4 GB-treated and untreated plants in each of the sampling times) were freeze-dried, weighed, ground (1 mm sieve, Retsch Grindomix GM 200; Retsch GmbH, Haan, Germany), and stored at −20°C until further analysis. The ground dry samples were used for chemical analyses. The GB content was analyzed with ultrahigh-performance liquid chromatography (UHPLC) (Water Acquity Ultra Performance LC; Waters Corp. Singapore) equipped with a light-scattering detector using a Cortecs hydrophilic interaction liquid chromatography (HILIC) column (2.1 × 150 mm, 2.7 μm; Waters). Glycinebetaine concentration in the fertigation solutions was analyzed using the same method. For the analyses, samples of nutrient solution were diluted 1:5 with acetonitrile (acetonitrile: water (9:1 v/v)). The total nitrogen (N) and carbon (C) contents were analyzed with Duma’s combustion method (CN 828, Leco Corp., St. Joseph, MN), and the other mineral contents (Ca, Fe, K, magnesium Mg, manganese Mn, sodium Na, P, sulfur S, and zink Zn) with inductively coupled plasma-optical emission spectrometry (ICP-OES, iCAP 6,200; Thermo Fisher Scientific, Cambridge, United Kingdom). The amino acids were analyzed with an ACQUITY UPLC system (Waters Milford, MA, United States) consisting of an Acquity photodiode array (PDA) optical detection system. A Waters BEH C18 column (100 mm × 2.1 mm i.d., 1.7 μm; Waters Milford USA) was used. Empower 2 (Waters, USA) software was used for system control and data acquisition.
Data analysis
Data on the growth and contents of , GB, minerals, and amino acids were analyzed with the IBM SPSS data editor (Version 26, Armonk, NY, USA). A two-way analysis of variance (ANOVA) was performed to show the effects of the treatment (Trmt), the effect of time after the first GB application (DAA), and their interaction. Significant differences were considered when the p-values were < 0.05. If a significant difference within the DAA x Trmt interaction was observed, the means in every sampling time were compared, using Tukey’s multiple range test.
Results
Glycinebetaine in the fertigation solution and in leaves
The GB was detectable in the nutrient solution over 3 DAA after 4 mM application (Figure 3A), and 4 DAA after 7.5 mM application (Figure 3B). In the third experiment, the GB concentration detected in the fertigation solution was 4 mM after the first application, even though the targeted concentration was 10 mM (Figure 3C). However, the GB concentration was below the detection limit 3 DAA. After the second application, the GB concentration in the fertigation solution reached a peak value of 6.6 mM at 7 DAA and was again below the detection limit at 11 DAA until the end of the experiment. Samples of the fertigation solution were not collected at 2, 8, and 10 DAA.
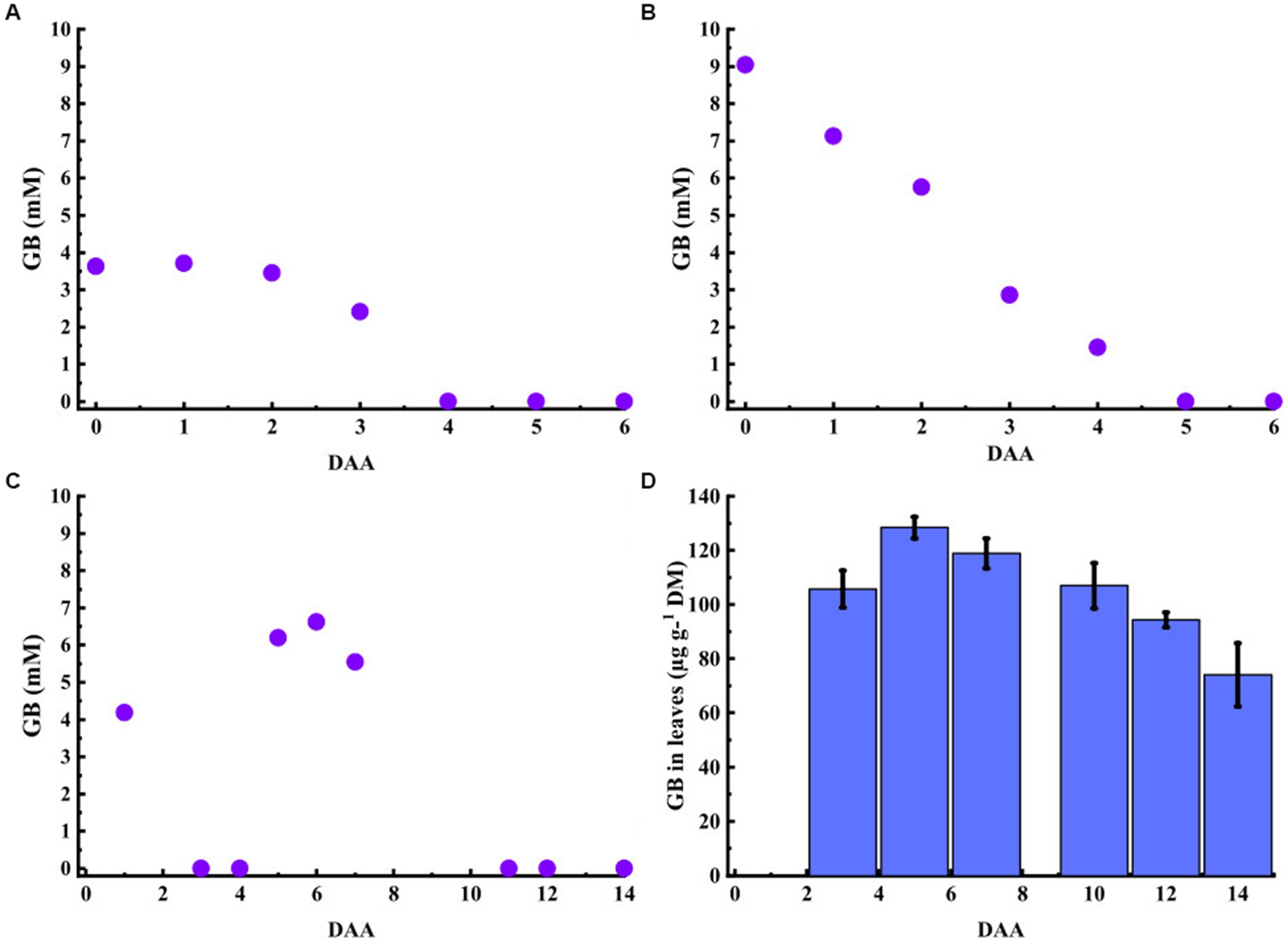
Figure 3. Glycinebetaine (GB) concentrations in the nutrient solutions (A-C) and lettuce leaves (D). (A) 4-mM of GB were applied in the solution (at 0 DAA), and the compound was detectable in the solution over 3 days. (B) 7.5-mM of GB were applied to the solution (at 0 DAA); the solution’s GB concentration decreased continuously after the application and was detectable until 5 DAA. (C) A 10-mM dose of GB was applied in the solution at 0 DAA and 4 DAA. The GB was detectable in the solution for 2 DAA after the first and second applications. The samples were not collected at 2 or 8–10 DAA. (D) Lettuce-accumulated GB of the nutrient solution and the GB content in leaf DM were maximal at 128 μg g−1. (D) Data shown are means± SE (N = 4). DAA = days after application; DM = dry matter.
In the third experiment, the lettuce accumulated root-applied GB in its leaves. Following the first 10 mM GB application, the leaf GB content was 106 μg g−1 DM at 3 DAA (Figure 3D). The GB content in the leaves increased further after the second GB application attaining maximal value of 128 μg g−1 DM at 5 DAA.
Nitrate content
The plant content varied over time in the treated and untreated plants. Following the 4 mM GB application, no clear response was observed (Figure 4A). The content in lettuce leaves was reduced, following the 7.5 mM GB application when the plants were exposed to GB over 3 days (Figure 4B). In the double-application GB treatment (10 + 10 mM GB), the content of the lettuce leaves decreased, and a significant interaction (p < 0.05) between DAA and Trmt was observed. The content in the GB-treated plants remained lower in comparison to the untreated plants from 6 DAA onwards (Figure 4C). The difference in the content between the GB-treated and untreated plants peaked at 12 DAA when the content in the GB-treated plants was 2,447 mg kg−1 FW and in untreated plants 3,465 mg kg−1 FW. Hence, double-application GB treatment of 10 mM + 10 mM reduced leaf content by more than 29% in comparison to untreated plants.
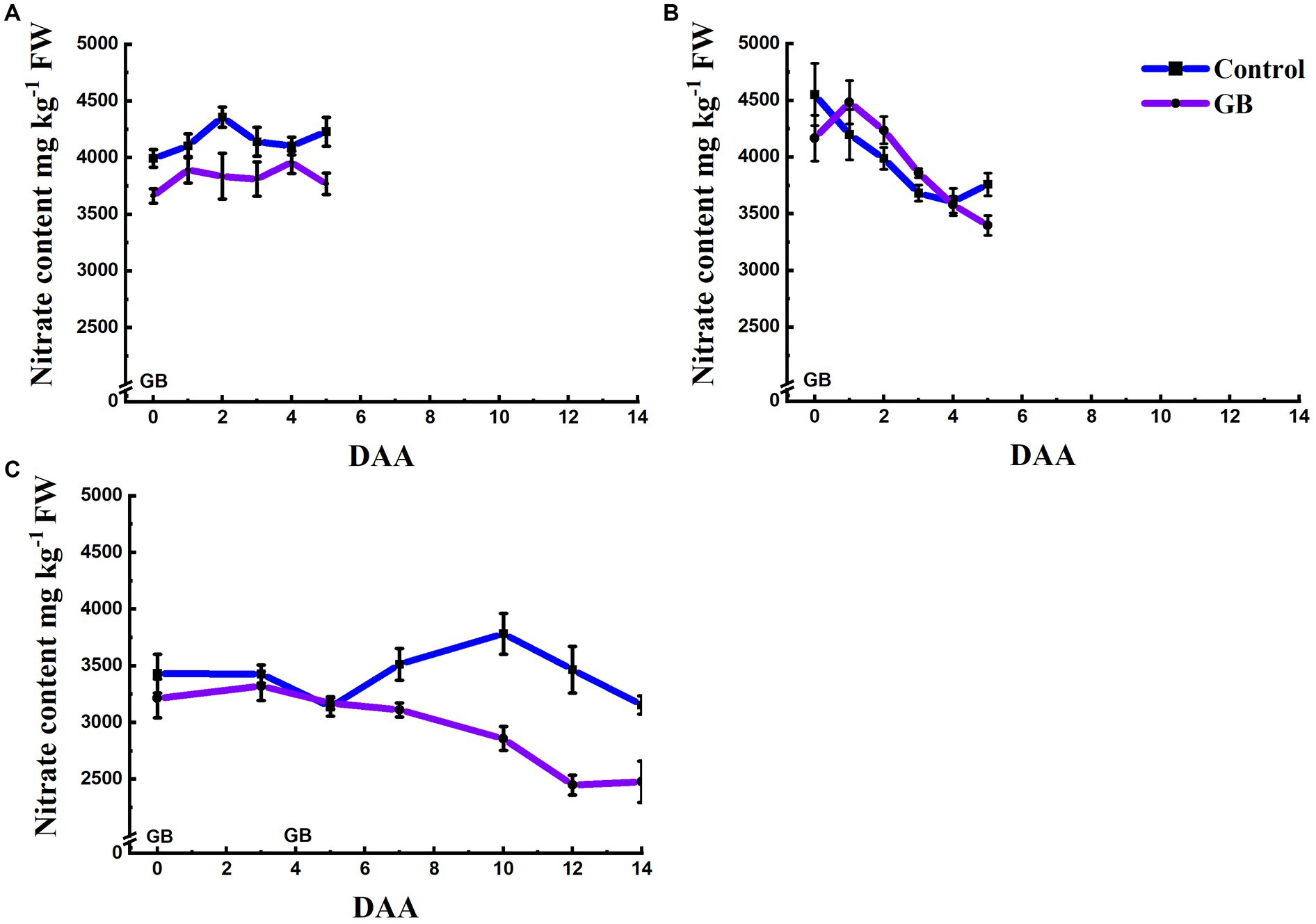
Figure 4. Effect of glycinebetaine (GB) on lettuce (cv. A,B = ‘Danstar’, C = ‘Frillice’) nitrate content. (A) The GB (4 mM) was applied into the nutrient solution at 0 DAA. (B) The GB (7.5 mM) was applied into the nutrient solution at 0 DAA. (C) The first dose of GB (10 mM) was applied into the nutrient solution at 0 DAA, and the second dose of GB (10 mM) was applied at 4 DAA. Each plant entered the treatment area 6 days before harvest. Data shown are means ± SE (A,B : N = 10; C: N = 6). FW = fresh weight; DAA = days after application.
Lettuce growth
The double-application GB treatment (10 + 10 mM) resulted in significantly lower (p = 0.014) FW values in comparison to untreated plants only at a sampling time of 12 DAA (Figure 5A). Furthermore, the double-application of GB increased the DM accumulation in lettuce, while the leaf DM content was significantly (p < 0.05) higher in GB-treated plants at 5, 7, 12, and 14 DAA in comparison to untreated plants (Figure 5B).
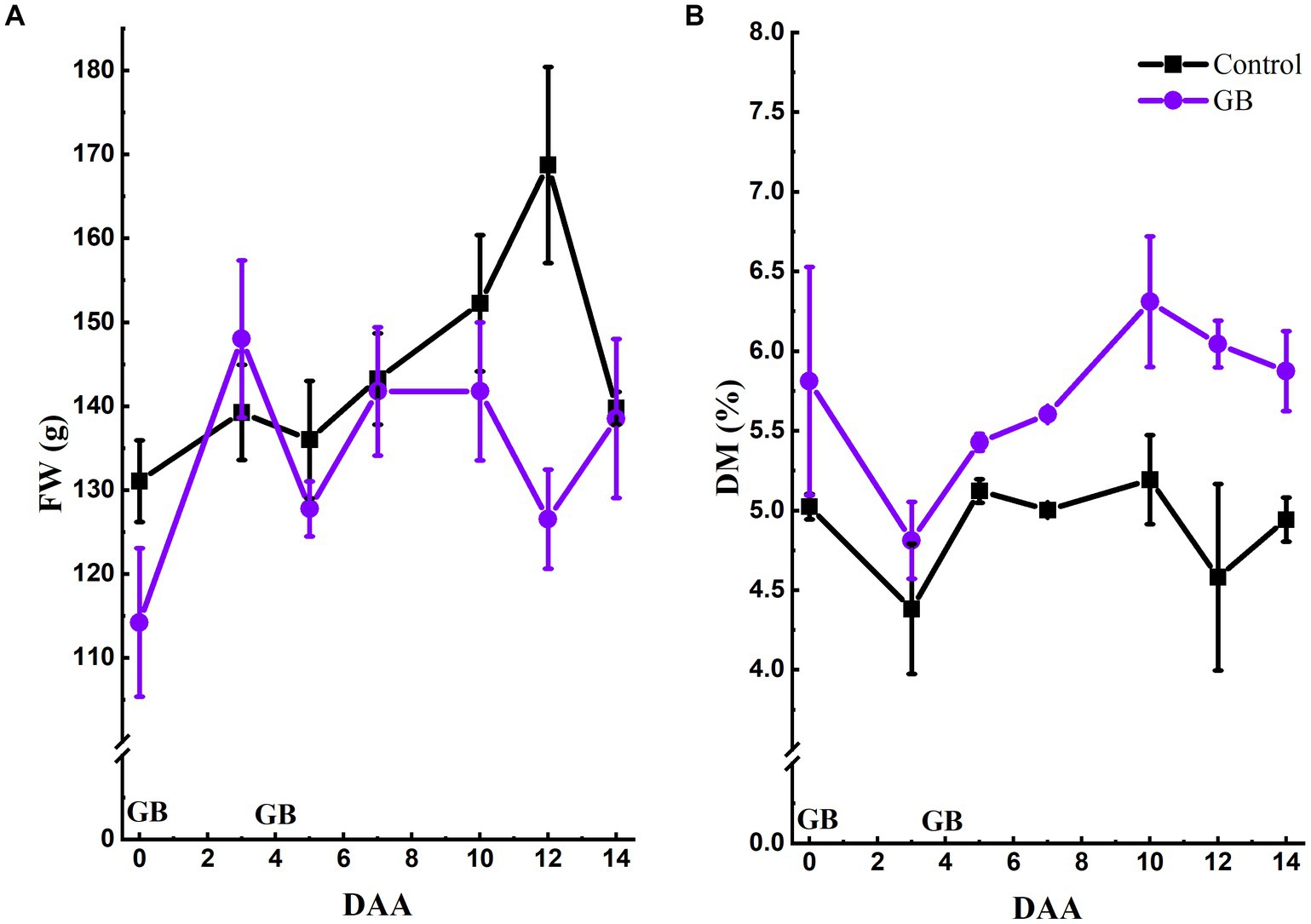
Figure 5. Effect of root-applied GB on lettuce fresh weight and dry matter content (A) Root-applied GB (10 + 10 mM) reduced lettuce (cv. ‘Frillice’) fresh weight (FW) accumulation in comparison to untreated plants. (B) Root-applied GB increased the dry matter (DM) content of lettuce leaves. The application time of GB is marked above the X-axis. Data shown are means ± SE (A: N = 10; A: N = 4). GB = glycinebetaine; DAA = days after application.
Amino acid and mineral contents in lettuce
The root-applied GB affected the content and composition of amino acids in the lettuce DM (Table 1). There was significant interaction between time (DAA) and treatment (p < 0.05) in the contents of cysteine, glycine, aspartic acid, glutamic acid, leucine, and tryptophan, as well as the total content of amino acids, which increased following the GB treatment (Table 1). The difference between the GB-treated and untreated plants was highest at 10 DAA when the GB plants contained amino acids at 148 g kg−1 DM and untreated plants 133 g kg−1 DM.
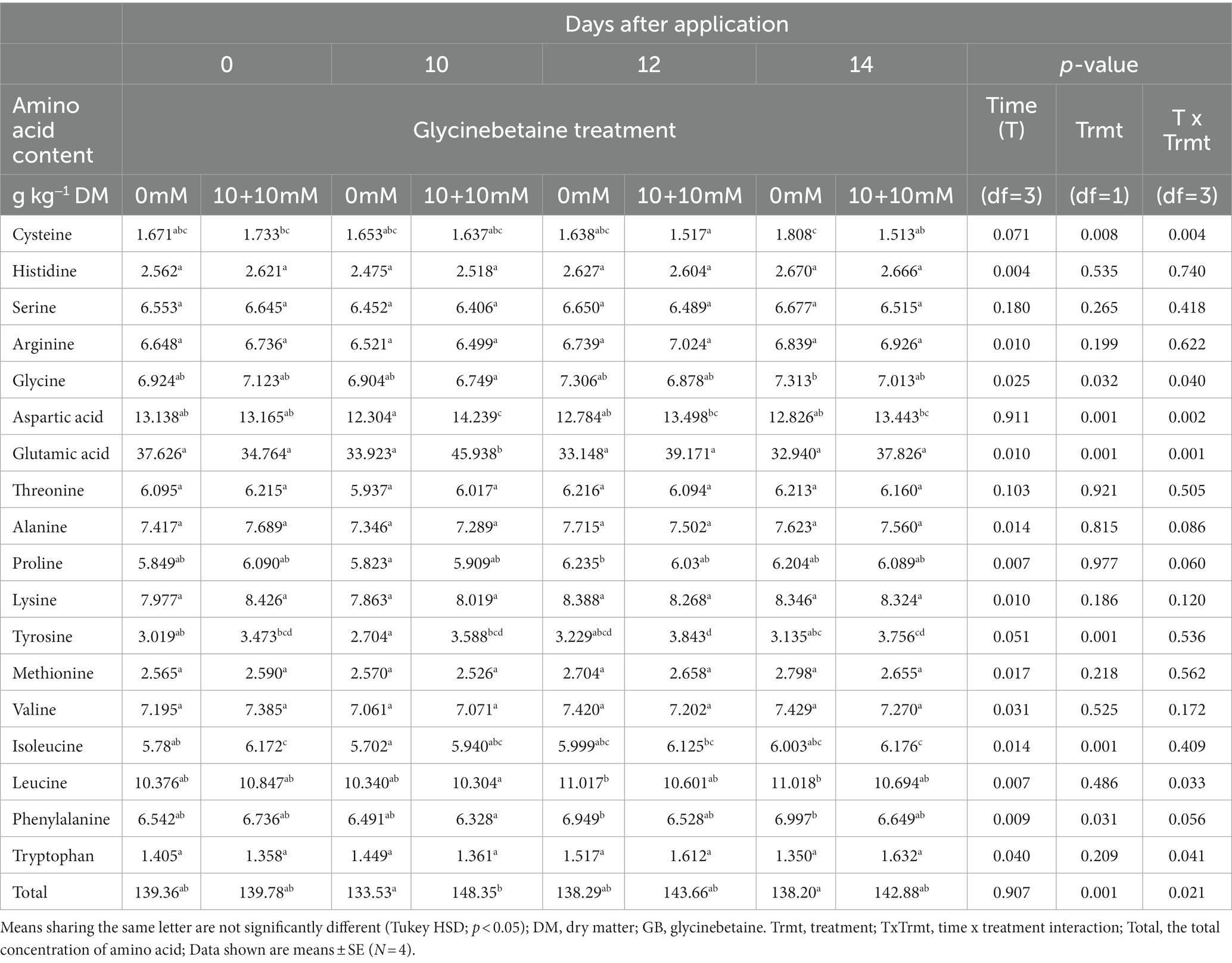
Table 1. Effect of root-applied glycinebetaine (GB 10 mM + 10 mM) on amino acids in lettuce leaves over time.
The interaction between time (DAA) and treatment was significant (p < 0.05) in the total content of free amino acids, and especially in the contents of free histidine, asparagine, arginine, glutamine, glycine, and tyrosine (Table 2). The content of free amino acids in the GB-treated plants was lowest at 0 DAA (28 g kg−1 DM) and highest at 10 DAA (38 g kg−1 DM), whereas in untreated plants it was the lowest at 14 DAA (25 g kg−1 DM) and highest at 0 DAA (32 g kg−1 DM).
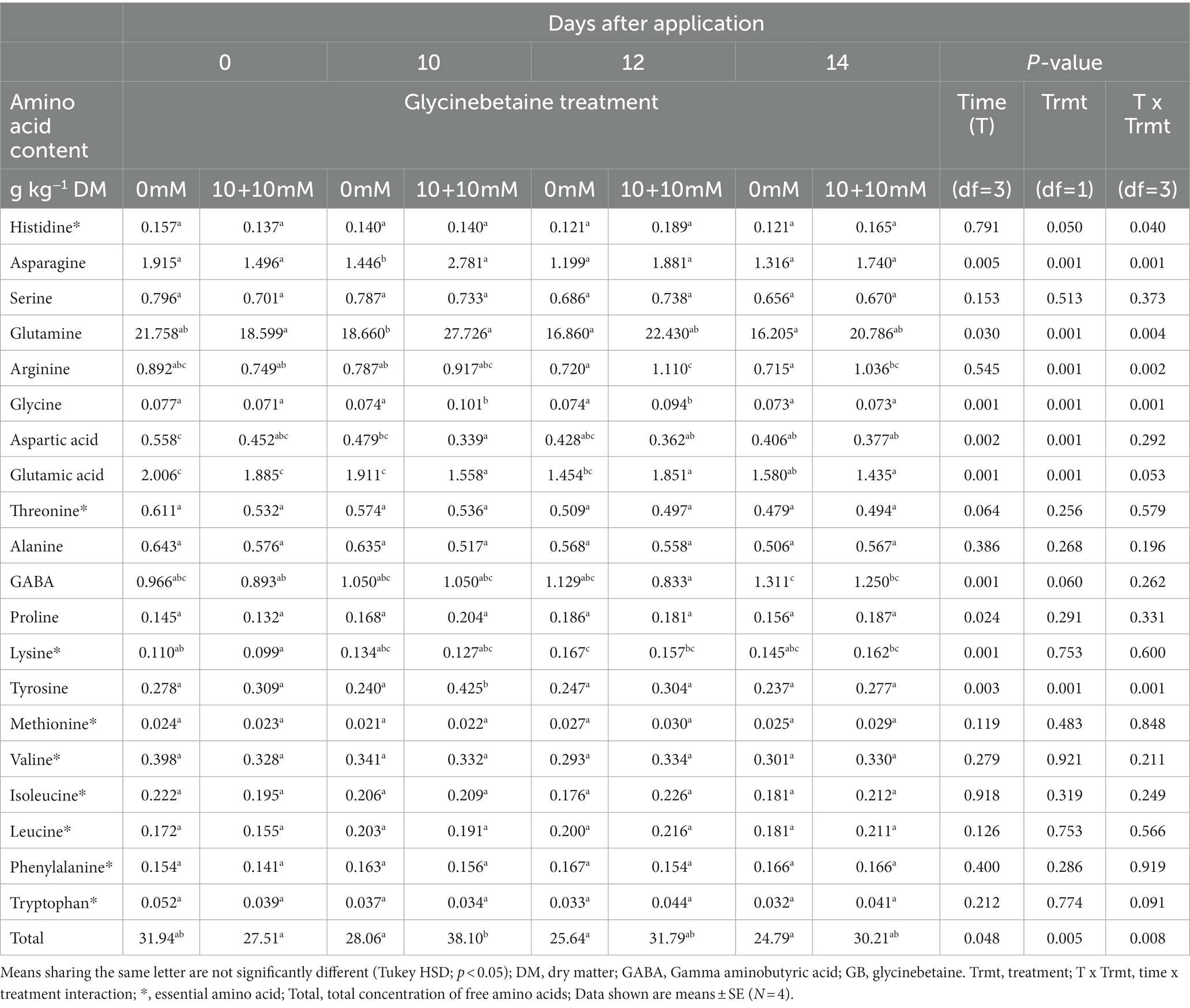
Table 2. Effect of root applied glycinebetaine (GB 10 mM + 10 mM) on free amino acids in lettuce leaves over time.
In general, the contents of minerals in the lettuce DM decreased after the GB application. Significant (p < 0.05) interaction between DAA and Trmt was observed in Ca, K, Mg, Mn, Na, P, and S contents, while the contents of Fe, Zn, N, and C were not significantly altered (Table 3). The most marked change occurred in the content of K, which decreased over 43% following the double application (10 + 10 mM) GB treatment.
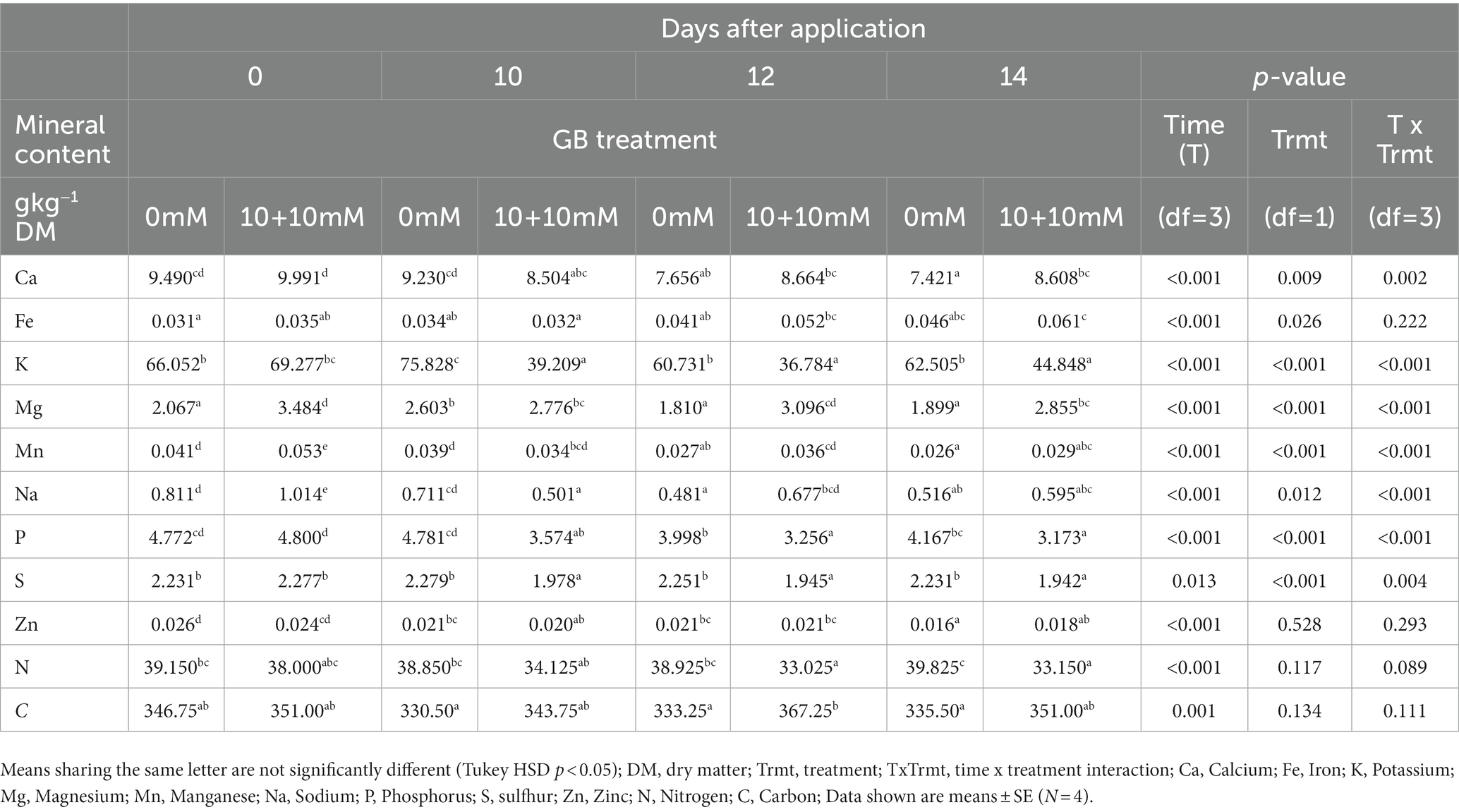
Table 3. Effect of root applied glycinebetaine (GB 10 mM + 10 mM) on mineral contents in lettuce leaves.
Discussion
Glycinebetaine and the edible quality of lettuce
The GB-treated lettuce accumulated root-applied GB in its leaves, whereas the untreated plants contained no GB, suggesting that lettuce does not synthesize endogenous GB as previously observed by Shams et al. (2016) and Jokinen et al. (2022). As the plants accumulated GB, the content in the leaves decreased. The marked decline in the leaf content began when the leaf GB content was 106 μg g−1 DM. Jokinen et al. (2022) observed that leaf GB content interacts negatively with leaf nitrate content, while a GB content of 90 μg g−1 DM was required to decrease the content. Decreased contents of lettuce contribute to consumers’ health effects, and thus GB improves the edible quality of lettuce. Since GB itself is considered a compound with possible health benefits for humans (Schwab et al., 2002; Cho et al., 2006; Koistinen et al., 2019), its accumulation in lettuce leaves also contributes to the nutritional value of lettuce. Based on fresh lettuce, an edible dose of 100 g of fresh lettuce contained 0.7 mg of GB, the highest in this study.
The total content of amino acids in lettuce DM increased following the GB application, which is consistent with previous study by Jokinen et al. (2022). In terms of nutritive value, the nine essential amino acids (histidine, threonine, lysine, methionine, valine, isoleucine, leucine, phenylalanine, and tryptophan), which cannot be synthesized in mammalian cells, are the most valuable nitrogenous compounds for human health (Davies and Jakeman, 2020). In our study the total content of the nine essential amino acids slightly increased in GB treated plants over the experiment period while in untreated plants it decreased. In plants, amino acids are produced through the assimilation process (Tischner, 2000). The increased amino acid content in GB-treated lettuce indicates that GB enhances assimilation and the activity of reductase (NR) and glutamine synthetase (GS) enzymes. Such effect of root-applied osmolytes (glucose and sucrose) were also previously reported in pak choi by Ma et al. (2018).
The contents of the minerals detected were lower in the GB treated plants at 10 DAA than in GB plants at 0 DAA. At a sampling time of 12 DAA, the GB-treated lettuce DM contained more Ca, Fe, Mg, Mn, Na, and C in comparison to untreated plants. However, the impact of GB on lettuce mineral uptake and use is rather unclear. Only the content of K was clearly reduced within GB-treated plants over time and remained a significantly lower level in the GB-treated plants in comparison to untreated plants throughout the experiment. Lettuce contained more DM after the GB application; hence, the reduction in the DM mineral contents did not directly contribute to reduced mineral content in edible fresh lettuce. Based on fresh lettuce, 100 g of fresh GB treated lettuce contained total N 9.7 mg at 0 DAA, and 11 mg at 10 DAA indicating that the reduction observed in content was not due to reduced N uptake. In addition, the content of C was slightly increased in GB-treated plants based on FW. One edible dose of 100 g of fresh GB lettuce contained 2,039 mg C at 0 DAA and 2,220 mg at 12 DAA, whereas the yield of C in untreated plants was 1,742 and 1,527 mg 100 g−1 FW, respectively. Furthermore, the DM content was a positively correlated with lettuce shelf life (Min et al., 2021), and thus our result indicates that GB may extend the shelf life of commercial lettuce.
Glycinebetaine in the commercial NFT-cultivation system
The GB disappeared from the nutrient solution within 3 to 5 days. Therefore, to continuously maintain low content in lettuce also requires repeated GB applications. Based on the results obtained in this study, GB application at 4-day intervals is recommendable. However, the GB uptake rate of lettuce may fluctuate over time and be influenced by environmental factors, plant maturity, and plant FW. In this study, plant responses to single GB applications at 4 mM and 7.5 mM concentration remained weak, whereas the double-application (10 mM+10 mM) resulted in similar leaf reduction previously reported by Jokinen et al. (2022). This result confirms the conclusion by Jokinen et al. (2022) that GB reduces lettuce content in a dose-dependent manner. The number of plants in the treatment area, plant biomass, and plant age are likely significant factors regarding the strength of plant responses to applied GB. Thus, we conclude that the optimal GB concentration is farm-specific and dependent on the targeted reduction in content as well as the technical design of the application system.
Presumably, not all the applied GB was taken up by the plants. For example, it is known that some bacteria take up applied GB (Farwick et al., 1995). The GB applied may be reserved and released by the bacteria over time, which may partly explain why GB was found from leaves at 14 DAA even though it was last applied in the solution 10 days earlier. Regarding the practical use of GB, the sensory method for quick measurement of solution GB concentration would be useful.
In this experiment, the FW of the GB treated plants varied over time being significantly lower compared to control plants only on 12 DAA. Moreover, on 2 DAA the GB treated plants were heavier than control plants. Thus, the impact of GB on lettuce FW can be considered relatively slight in this experiment. In addition, the treatment did not lengthen the production period since all the plants reached the commercial size (100 g). This relatively slight growth retardation was observed probably because the plants were exposed to GB only during the last 6 growing days before harvest. The content was significantly reduced only after a 6-day treatment period with double-application of GB, indicating that a treatment period of 6 days is required in commercial production. The lower concentrations of GB tested in the two first experiments did not reduce leaf content. Assumably the GB concentration required to reach the targeted reduction vary depending on the total plant biomass on the treatment area.
The content in lettuce can also be regulated by reducing the concentration of NO3–N in the nutrient solution before harvest (Santamaria et al., 1998). However, this method may reduce lettuce growth, increase tip burn in leaves, and shorten the shelf-life (Näkkilä et al., 2015). Even though GB can reduce phytomass accumulation, it can delay leaf senescence (Jokinen et al., 2022). Regulation of growth temperature (Santamaria et al., 2001) and optimizing light intensity (Liu et al., 2016; Zhang et al., 2018; Yi et al., 2020) are methods that use practical measures to regulate lettuce content and quality, but the useability of these methods is uncertain in the greenhouse. Accordingly, GB applied in the nutrient solution can be an alternative or supplemental method for lettuce quality control for greenhouse-grown lettuce.
Conclusion
This study demonstrated that GB provides a novel method for improving lettuce nutritive quality in commercial hydroponic NFT lettuce production by reducing leaf content and increasing the content of amino acids. The GB applied in the nutrient solution also increased the DM in lettuce. Lettuce containing more DM is more nutritious for humans. In addition, the increased DM content likely improves the shelf-life of lettuce. Some crop losses involved in high content can be avoided by GB application, which certainly benefits farmers and improves the efficiency throughout the production chain. These avoidable crop losses originated from practical methods for controlling the content by cutting off the oldest lettuce leaves during harvest, and rejection of the plants by traders due to exceeded content. However, the mode of action of root-applied GB on lettuce metabolism and leaf senescence is not yet known. This knowledge is essential, regarding the wider use of the method in commercial production and will therefore be a topic for future research.
Data availability statement
The raw data supporting the conclusions of this article will be made available by the authors, without undue reservation.
Author contributions
JU, KJ, and PM designed the experiments in collaboration with the commercial greenhouse. ME, KJ, IS, PM, and JU designed the chemical analyses conducted. JU conducted the experiments, performed the data work, and wrote the first draft of the manuscript. ME, PM, IS, and KJ provided revisions for the manuscript. All authors contributed to the article and approved the submitted version.
Funding
This work was supported by the Maiju ja Yrjö Rikalan Puutarhasäätiö sr., Kauppapuutarhaliiton puutarhasäätiö sr., Finnish Cultural Foundation sr., and the Niemi Foundation sr.
Acknowledgments
Marjo Kilpinen and Marjut Wallner are acknowledged for their contribution and assistance with chemical analysis. Oksasen Puutarha Oy, Finland, is acknowledged for enabling the experiments conducted in a commercial production system.
Conflict of interest
The authors declare that the research was conducted in the absence of any commercial or financial relationships that could be construed as a potential conflict of interest.
Publisher’s note
All claims expressed in this article are solely those of the authors and do not necessarily represent those of their affiliated organizations, or those of the publisher, the editors and the reviewers. Any product that may be evaluated in this article, or claim that may be made by its manufacturer, is not guaranteed or endorsed by the publisher.
References
Blom-Zandstra, M. (1989). Nitrate accumulation in vegetables and its relationship to quality. Ann. Appl. Biol. 115, 553–561. doi: 10.1111/J.17447348.1989.TB06577.X
Burns, I. G., Zhang, K., Turner, M. K., and Edmondson, R. (2010). Iso-osmotic regulation of nitrate accumulation in lettuce. J. Plant Nutr. 34, 283–313. doi: 10.1080/01904167.2011.533328
Cho, E., Zeisel, S. H., Jacques, P., Selhub, J., Dougherty, L., Colditz, G. A., et al. (2006). Dietary choline and betaine assessed by food-frequency questionnaire in relation to plasma total homocysteine concentration in the Framingham offspring study. Am. J. Clin. Nutr. 83, 905–911. doi: 10.1093/AJCN/83.4.905
Craig, S. A. S. (2004). Betaine in human nutrition. Am. J. Clin. Nutr. 80, 539–549. doi: 10.1093/AJCN/80.3.539
Davies, R. W., and Jakeman, P. M. (2020). Separating the wheat from the chaff: nutritional value of plant proteins and their potential contribution to human health. Nutrients 12:2410. doi: 10.3390/nu12082410
European Food Safety Authority (EFSA) (2008). Nitrate in vegetables -scientific opinion of the panel on contaminants in the food chain. EFSA J. 6:689. doi: 10.2903/j.efsa.2008.689
Farwick, M., Siewe, R. M., and Kramer, R. (1995). Glycine betaine uptake after hyperosmotic shift in Corynebacterium glutamicum. J. Bacteriol. 177, 4690–4695. doi: 10.1128/JB.177.16.4690-4695.1995
Hayes, K. C., Pronczuk, A., Cook, M. W., and Robbins, M. C. (2003). Betaine in sub-acute and sub-chronic rat studies. Food Chem. Toxicol. 41, 1685–1700. doi: 10.1016/S02786915(03)001960
Hoque, M. M., Ajwa, H. A., and Smith, R. (2008). Nitrite and ammonium toxicity on lettuce grown under hydroponics. Comm. Soil Plant Anal. 39, 207–216. doi: 10.1080/00103620701759194
Jokinen, K., Salovaara, A. K., Wasonga, D. O., Edelmann, M., Simpura, I., and Mäkelä, P. S. A. (2022). Root-applied glycinebetaine decreases nitrate accumulation and improves quality in hydroponically grown lettuce. Food Chem. 366:130558. doi: 10.1016/J.FOODCHEM.2021.130558
Kim, M. J., Moon, Y., Tou, J. C., Mou, B., and Waterland, N. L. (2016). Nutritional value, bioactive compounds and health benefits of lettuce (Lactuca sativa L.). J. Food Compos. Anal. 49, 19–34. doi: 10.1016/J.JFCA.2016.03.004
Kimura, M., and Rodriguez-Amaya, D. (2003). Carotenoid composition of hydroponic leafy vegetables. J. Agric. Food Chem. 51, 2603–2607. doi: 10.1021/jf020539b
Koistinen, V. M., Kärkkäinen, O., Borewicz, K., Zarei, I., Jokkala, J., Micard, V., et al. (2019). Contribution of gut microbiota to metabolism of dietary glycine betaine in mice and in vitro colonic fermentation. Microbiome 7, 1–14. doi: 10.1186/S40168-019-0718-2/TABLES/1
Liu, H., Fu, Y., Yu, J., and Liu, H. (2016). Accumulation and primary metabolism of nitrate in lettuce (Lactuca sativa L. var. Youmaicai) grown under three different light sources. Commun. Soil Sci. Plant Anal. 47, 1994–2002. doi: 10.1080/00103624.2016.1225076
Ma, Q., Ma, J., Wang, J., Wang, Q., Feng, Y., and Wu, L. (2018). Glucose and sucrose supply regulates the uptake, transport, and metabolism of nitrate in Pak Choi. Agron. J. 110, 535–544. doi: 10.2134/AGRONJ2017.09.0541
Min, Q., Marcelis, L. F. M., Nicole, C. C. S., and Woltering, E. J. (2021). High light intensity applied shortly before harvest improves lettuce nutritional quality and extends the shelf life. Front. Plant Sci. 12:615355. doi: 10.3389/fpls.2021.615355
Näkkilä, J., Särkkä, L., Jokinen, K., and Kaseva, J. (2015). Kalsiumnitraatin käyttömäärän vaikutus jääsalaatin satoon ja laatuun. Luonnonvarakeskus, Jokioinen, Finland, 19 p.
Rhodes, D., and Hanson, A. D. (1993). Quaternary ammonium and tertiary sulfonium compounds in higher plants. Annu. Rev. Plant Physiol. Plant Mol. Biol. 44, 357–384. doi: 10.1146/annurev.pp.44.060193.002041
Santamaria, P., Elia, A., Gonnella, M., and Parente, A. (2001). Ways of reducing rocket salad nitrate content. Acta Hortic. 548, 529–536. doi: 10.17660/ActaHortic.2001.548.64
Santamaria, P., Elia, A., Parente, A., and Serio, F. (1998). Fertilization strategies for lowering nitrate content in leafy vegetables: chicory and rocket salad cases. J. Plant Nutr. 21, 1791–1803. doi: 10.1080/01904169809365524
Schwab, U., Törrönen, A., Toppinen, L., Alfthan, G., Saarinen, M., Aro, A., et al. (2002). Betaine supplementation decreases plasma homocysteine concentrations but does not affect body weight, body composition, or resting energy expenditure in human subjects. Am. J. Clin. Nutr. 76, 961–967. doi: 10.1093/AJCN/76.5.961
Shams, M., Yildirim, E., Ekinci, M., Turan, M., Dursun, A., Parlakova, F., et al. (2016). Exogenously applied glycine betaine regulates some chemical characteristics and antioxidative defence systems in lettuce under salt stress. Hortic. Environ. Biotechnol. 57, 225–231. doi: 10.1007/S13580-016-0021-0
Tabaglio, V., Boselli, R., Fiorini, A., Ganimede, C., Beccari, P., Santelli, S., et al. (2020). S. Agronomy 10:1208. doi: 10.3390/AGRONOMY10081208
Tischner, R. (2000). Nitrate uptake and reduction in higher and lower plants. Plant Cell Environ. 23, 1005–1024. doi: 10.1046/j.1365-3040.2000.00595.x
Viršilė, A., Brazaitytė, A., Vaštakaitė-Kairienė, V., Miliauskienė, J., Jankauskienė, J., Novičkovas, A., et al. (2019). Lighting intensity and photoperiod serves tailoring nitrate assimilation indices in red and green baby leaf lettuce. J. Sci. Food Agric. 99, 6608–6619. doi: 10.1002/JSFA.9948
Wyn Jones, G., and Storey, R. (1981). “Betaines” in The physiology and biochemistry of drought resistance in plants. eds. L. G. Paleg and D. Aspinall (Sydney, Australia: Academic Press), 172–204.
Yi, Z., Cui, J., Fu, Y., Yu, J., and Liu, H. (2020). Optimization of light intensity and nitrogen concentration in solutions regulating yield, vitamin C, and nitrate content of lettuce. J. Hortic. Sci. Biotechnol. 96, 62–72. doi: 10.1080/14620316.2020.1804468
Keywords: Lactuca sativa L., minerals, amino acids, NFT-cultivation, fertigation
Citation: Uusitupa J, Edelmann M, Simpura I, Jokinen K and Mäkelä PSA (2023) Root-applied glycinebetaine controls nitrate content and edible quality of lettuce in continuous commercial hydroponic greenhouse production. Front. Sustain. Food Syst. 7:1125456. doi: 10.3389/fsufs.2023.1125456
Edited by:
Delia Grace, University of Greenwich, United KingdomReviewed by:
Jingwen Gao, Rutgers, The State University of New Jersey, United StatesMaribela Pestana, University of Algarve, Portugal
Copyright © 2023 Uusitupa, Edelmann, Simpura, Jokinen and Mäkelä. This is an open-access article distributed under the terms of the Creative Commons Attribution License (CC BY). The use, distribution or reproduction in other forums is permitted, provided the original author(s) and the copyright owner(s) are credited and that the original publication in this journal is cited, in accordance with accepted academic practice. No use, distribution or reproduction is permitted which does not comply with these terms.
*Correspondence: Jenni Uusitupa, jenni.uusitupa@helsinki.fi