- 1School of Biosciences, University of Nottingham Malaysia, Semenyih, Selangor, Malaysia
- 2The UWA Institute of Agriculture, The University of Western Australia, Perth, WA, Australia
- 3Accelerated Crop Improvement, International Cros Research Institute for the Semi-Arid Tropics, Hyderabad, India
- 4School of Biological Sciences, The University of Western Australia, Perth, WA, Australia
- 5Centre for Applied Bioinformatics, The University of Western Australia, Perth, WA, Australia
- 6Centre for Transformative Agricultural and Food Systems, School of Agricultural, Earth and Environmental Science, University of KwaZulu-Natal, Pietermaritzburg, South Africa
- 7International Water Management Institute, Pretoria, South Africa
- 8School of Science, Monash University Malaysia, Bandar Sunway, Selangor, Malaysia
The recent Russia–Ukraine conflict has raised significant concerns about global food security, leaving many countries with restricted access to imported staple food crops, particularly wheat and sunflower oil, sending food prices soaring with other adverse consequences in the food supply chain. This detrimental effect is particularly prominent for low-income countries relying on grain imports, with record-high food prices and inflation affecting their livelihoods. This review discusses the role of Russia and Ukraine in the global food system and the impact of the Russia–Ukraine conflict on food security. It also highlights how diversifying four areas of agrifood systems—markets, production, crops, and technology can contribute to achieving food supply chain resilience for future food security and sustainability.
1. Introduction
In the past 2 years, global issues such as climate change, the COVID-19 pandemic, and the Russia–Ukraine conflict have taken the spotlight, with reports and discussions around these events flooding the media. This has led to intense pressure on socioeconomics, healthcare, and food systems in a short time. Confronting us are direct consequences of these global crises on the agrifood systems, particularly from the Russia–Ukraine conflict, throwing food security issues into the limelight. Ukraine and Russia are in the top three global exporters of various staple foods, including wheat, barley, maize, rapeseed, and sunflower (FAO, 2022a). Disruptions to their exports are having strong ripple effects on the global food market and concerns for food security (Behnassi and El Haiba, 2022).
The Russia–Ukraine conflict directly impacts global agrifood systems through trade, production, and prices (FAO et al., 2022). The FAO estimates that the shortfall in grain and sunflower seeds exports from Ukraine and Russia will increase international wheat prices by between 8.75% (moderate case) and 21.5% (severe case) in 2022/23. Countries hardest hit by the reduction in Ukraine and Russia's food exports in terms of physical supply are those relying heavily on wheat and wheat products imports—the Middle East and North Africa, developing countries in Europe, and sub-Saharan Africa (World Bank Group, 2022), therefore impeding the efforts to eradicate malnutrition and hunger by 2030 which is the focus in the second goal of the United Nation's 17 Sustainable Development Goals (SDG Target 2, Zero Hunger). This will be pronounced in net food-importing developing countries (Figure 1), i.e., the world's poorest countries (UNCTAD, 2022), concentrated in sub-Saharan African nations that imported 85% of national food requirements in 2016–2018. The Russia–Ukraine conflict has caused a shortfall of at least 30 million tons of food in sub-Saharan African nations (African Development Bank Group, 2022), as well as other regions (South Asia, the Middle East, North Africa, Latin America and the Caribbean, and East Asia) that were already facing food security problems (UNCTAD, 2021).
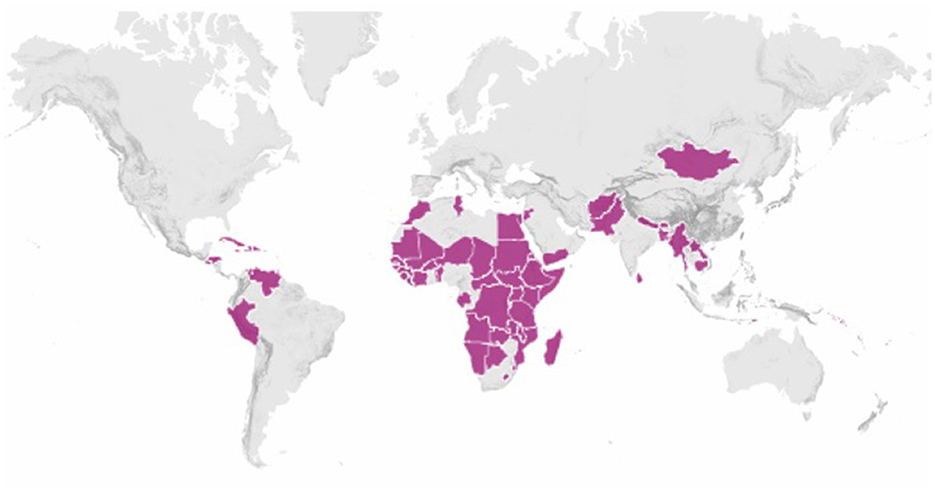
Figure 1. Net Food Importing Developing Countries (NFIC) vulnerable to food system disruption due to exogenous events such as the Russia–Ukraine conflict including sub-Saharan Africa, South Asia, the Middle East, North Africa, Latin America and the Caribbean, and East Asia (FAO, 2022b) (Figure re-produced with permission from FAO Copyright Office).
This food crisis at a global scale was not unexpected. Global food crises have occurred in the past, incurred by: (1) high oil prices in 2007–2008, (2) increased food demand in emerging economies (Mittal, 2009; Headey and Fan, 2010), (3) extreme weather incidents such as drought, floods and storms in 2010–2011 in many grain-producing countries (e.g., China, Australia, Argentina, Russia, and Ukraine) (Johnstone and Mazo, 2011; Werrell et al., 2013), and more recently, (4) the COVID-19 pandemic causing global disruptions in the food supply chains (Hart et al., 2022; Pauw and Thurlow, 2022). If past global food crises have taught us anything, agrifood systems need to be restructured to make them more resilient and strategies put in place to manage such crises (Pellegrini and Fernández, 2018).
To this end, this review highlights the impact of the current international crisis on agrifood systems. We first discuss the role of Russia and Ukraine as the main exporters of cereal grains and oilseed crops, followed by a discussion of the impact of the conflict on global agrifood systems. We then highlight the over-reliance on a few countries for a large portion of our major food staples and its potential consequences for global food security. Lastly, we offer suggestions on several aspects of diversification within the agrifood systems to enhance global food security and nutrition in this challenging time.
2. Agrifood systems
Agrifood systems comprise all players and components in producing agricultural food products, such as crops, livestock, fisheries, forestry, and aquaculture, and non-agricultural food products, such as synthetic meat. The production, storage, processing, distribution, consumption, and disposal of these products support human food consumption and sustain life (FAO, 2021; United Nations 2021 Food Systems Summit, 2021). Agrifood systems also encompass non-food products that are agriculturally based, such as textiles, biofuels, fiberglass, and cosmetics, but these are outside the context of our current discussion. The 2020 FAO statistics record shows that the total gross value of agricultural production (crop and livestock) worldwide equated to USD 4.79 trillion (FAOSTAT, 2022). The main players in the global agrifood systems are those that influence international food prices and demand through various agrifood sectors. These include major exporters of food commodities, agriculture companies involved in agrochemicals, commercial seed suppliers, farm equipment suppliers, food processors, retailers, and others (ETC Group, 2022). We discuss how we should not over-rely on major food exporters and propose diversification as a sustainable way to achieve agrifood system resilience.
3. Role of the Russian federation and Ukraine in the agrifood systems
Russia and Ukraine are ranked 11 and 55 in the world economy in terms of GDP (US$), with their top export commodities being petroleum and agriculture, respectively (OEC, 2022). Both countries are global contributors to a substantial share of agricultural commodities, including raw materials for fertilizers, and are key exporters of cereal and oilseed food crops (World Bank Group, 2022). In 2020, Russia ranked number one in wheat exports, and Ukraine ranked number one in seed oil exports (OEC, 2022). Figure 2 illustrates the economic contributions of exported commodities by Russia and Ukraine in 2021 (UN Comtrade Database Intelligence, 2021).
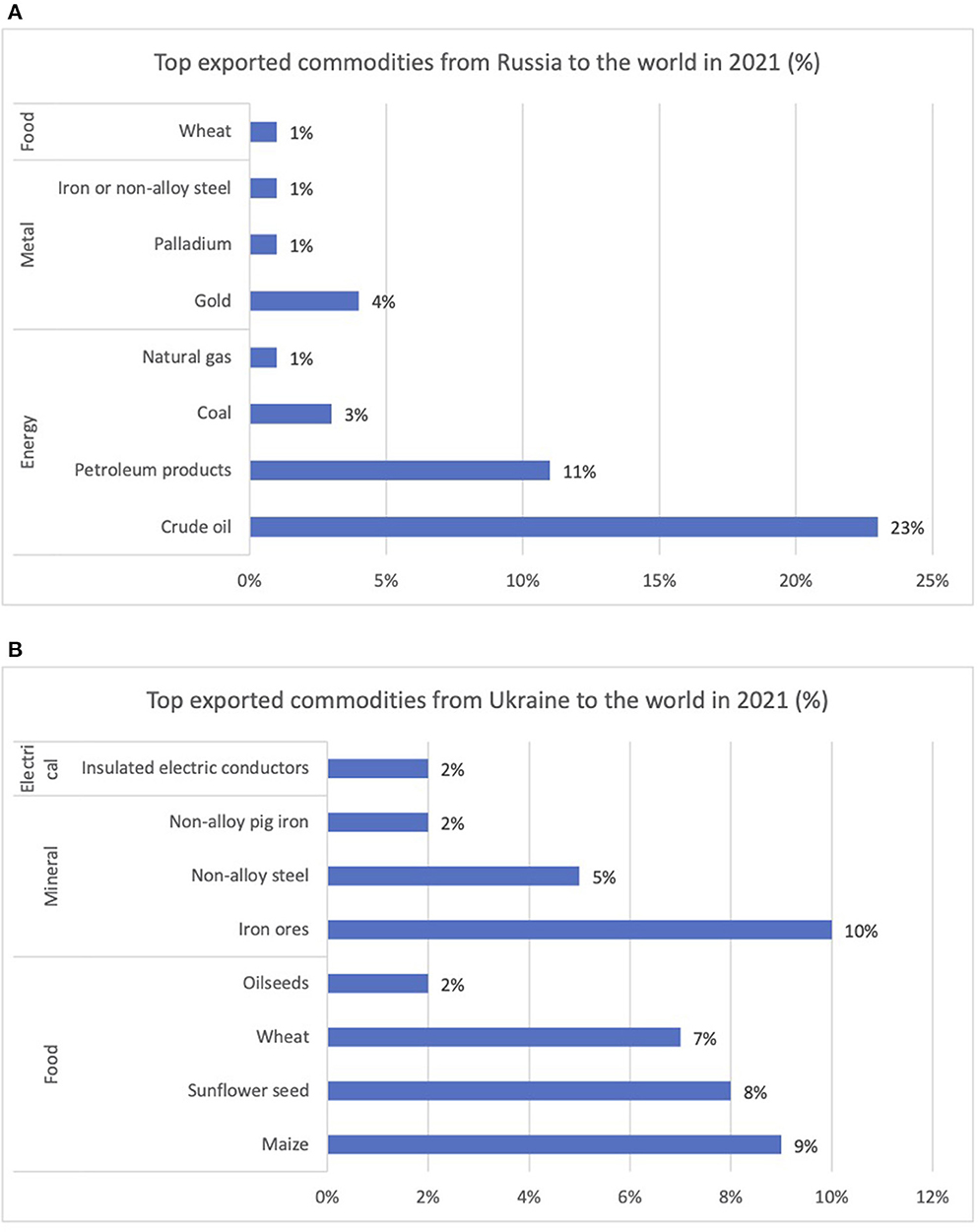
Figure 2. Top exported commodities in (A) Russia, and (B) Ukraine in 2021 (UN Comtrade Database Intelligence, 2021).
4. Impact of the Russia–Ukraine conflict on agrifood systems
4.1. Food export bans and restrictions
The Russia–Ukraine conflict has produced shocks in the commodity markets for food, feed, fertilizers, and energy (OECD, 2022a) and impacted agrifood systems in many areas. As of early April 2022, 16 countries had imposed food commodities export bans following the escalation of the Russia–Ukraine conflict, equating to about 17% of total calories traded globally (Glauber et al., 2022). This is to ensure a sufficient supply of food within their own countries. With the closure of main Ukrainian ports at the Black Sea and the effects of multiple global economic sanctions imposed on Russia, both countries have evidently contributed to the largest percentage of food exports restrictions, equating to 42% of total calories (European Parliament Think Tank, 2022; Glauber et al., 2022). Other countries introduced export bans on various agricultural products, such as palm oil from Indonesia (Office of Assistant to Deputy Cabinet Secretary for State Documents and Translation, 2022) and grain products from Turkey, Kyrgyzstan, and Kazakhstan (CGIAR, 2022; Donley, 2022).
While some of these export bans were temporary and have been lifted, this exercise could potentially generate a domino effect with other exporting countries then implementing their own import/export restrictions in response to deviations in food prices. This will further destabilize the food supply in global markets with almost immediate inflation of food prices, severely affecting Central Asian countries and North African countries which rely on imports of wheat and maize, while India, Pakistan, and Bangladesh rely on imports of vegetable oils (Giordani et al., 2016; Glauber et al., 2022).
4.2. Food price hike
The rise in food prices is not solely a direct consequence of the Russia–Ukraine conflict, as an increasing trend has been apparent since 2020, but 2022 saw a “giant leap” in the food price index (Figure 3) (FAO, 2022c; Weersink and von Massow, 2022).
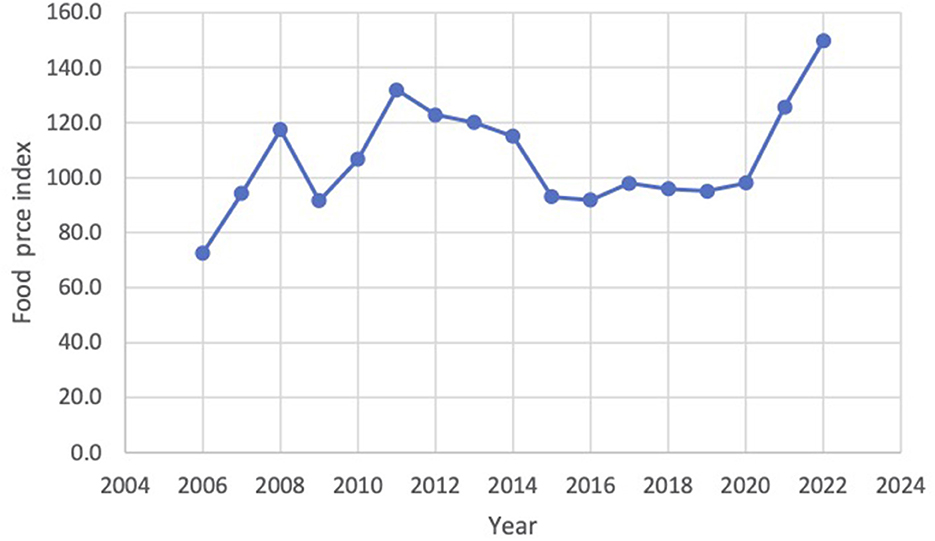
Figure 3. Annual FAO food price indices from 2006–2022 (FAO, 2022c).
The increasing trend in food prices from 2020 to early 2022 is mainly shaped by the COVID-19 pandemic, with travel restrictions and lockdowns within and outside countries causing farm production to slow down, creating bottlenecks in the food supply chain [OECD Policy Responses to Coronavirus (COVID-19), 2020]. Other causes of food price hikes include the upward shift in food, feed, and fuel demand as the world gradually recovered from the pandemic and some crop production losses due to unfavorable weather patterns in Brazil and the USA (Sova and Man, 2021). The conflict in Ukraine during the first quarter of 2022 held back the reduction of food and energy inflation, thus increasing the global commodity prices in 2022–2023 (OECD, 2022b).
In the longer term, food prices are predicted to continue to increase even after the Russia–Ukraine conflict ends, as we continue to face reduced crop production due to volatile weather patterns induced by climate change (Cowling et al., 2019; Sultan et al., 2019; Anderson et al., 2020), such as the recent extreme heat, drought and flood conditions in Europe, the USA, the Horn of Africa and China, among others (Lodewick, 2022; WFP, 2022). Models have also predicted yield declines of up to 21.5% in maize on the US Great Plains due to erratic climate patterns (Irmak et al., 2022) and a reduction of up to 12.5% in wheat production in South Africa due to extreme heat (Shew et al., 2020). Moreover, studies have shown that extreme weather events are likely to occur more frequently and will be more intense in the future, directly and indirectly impacting crop yields and further threatening global agrifood systems and supplies with long-term implications for increasing food prices (Diffenbaugh et al., 2018).
4.3. Threat to food security
According to the Bill and Melinda Gates Foundation (BMGF) Goalkeepers Report (Bill and Melinda Gates Foundation Goalkeepers Report 2022, 2022), achieving SDG target 2 (Zero Hunger) by 2030 is too ambitious. Yet understanding the current status of global agrifood systems and food security is critical in facilitating the eradication of hunger (FAO et al., 2022).
In 2021, ~193 million people across 53 countries faced acute food insecurity at crisis level or worse (IPC/CH Phase 3 or above; 1 = minimal/non, 5 = catastrophe/famine) (Integrated Food Security Phase Classification, 2022), almost doubling from 2016 levels, with a concerning figure of 570,000 people categorized as in catastrophe conditions (IPC/CH Phase 5) in Ethiopia, South Sudan, southern Madagascar, Yemen, Afghanistan, and Somalia, where conflict is the primary driver of severe food insecurity (Global Report on Food Crises, 2022; WFP and FAO, 2022) because it disrupted agriculture-based livelihoods, restricted food access, impeded humanitarian operations and displaced populations (mainly smallholder farmers).
Even before the conflict in Russia–Ukraine, ongoing social unrest in several countries concentrated in Eurasia, namely Afghanistan, Bangladesh, Iraq, the Syrian Arab Republic, Yemen, and Ukraine, resulted in as many as 52.8 million people experiencing food insecurity at a crisis level (IPC Phase 3 or above) in 2021, up from 30.9 million in 2016, with nearly 16.8 million people displaced due to conflict (Global Report on Food Crises, 2022). That this is a continuous issue is supported by the findings that in 2014 food insecurity across sub-Saharan Africa, particularly in South Sudan and Nigeria, was driven by conflict (Anderson et al., 2021).
Based on FAO estimations, the Russia–Ukraine conflict will increase the number of undernourished people globally by 13.1 million in a worst-case scenario, added to the current 733.9 million undernourished people not impacted by the onset of the conflict (FAO et al., 2022). Sub-Saharan Africa, the Middle East, and North Africa are the most vulnerable population groups impacted by the Ukraine conflict (FAO et al., 2022). Adding to the malnutrition problem, food insecurity positively correlates with social unrest, particularly in developing countries, leading to further insecurity (Arezki and Bruckner, 2011; Weinberg and Bakker, 2015; Soffiantini, 2020).
4.4. Livelihood struggle among the rural poor
The Russia–Ukraine crisis has impacted poor and rural households due to fuel, food, and fertilizer price hikes. Countries such as Kenya (Breisinger et al., 2022), Egypt (Abay et al., 2022), Bangladesh (Diao et al., 2022b), and Ethiopia (Diao et al., 2022a) have witnessed declines in food consumption and diet quality. The increase in fertilizer prices also caused a heavy toll on local farmers in these countries, whose livelihoods depend on agriculture. If farmers reduce fertilizer use due to price hikes, their production often declines, impacting rural household incomes.
Typically, most farmers in developing countries in East Asia and the Pacific, South Asia, and sub-Saharan Africa are smallholders (farm owners operating agricultural land areas < 2 ha). They account for 84% of global farms, contributing about 35% of the world's food (Ricciardi et al., 2018; Lowder et al., 2021). These smallholder farmers often live in remote areas and produce a wide variety of foods in addition to the main crop, but often with low productivity (Rapsomanikis, 2015). They are vulnerable to food insecurity due to sociodemographic factors (e.g., family size, education level of family members, farming experience) and have low resilience to changing circumstances, such as weather shocks and pest and disease outbreaks, as reflected in household surveys conducted in Central America (Guatemala and Honduras) (Alpízar et al., 2020) and Africa (Madagascar) (Harvey et al., 2014).
Using satellite data images of croplands in Ukraine, studying the impact of the conflict on winter wheat production across 2019–2021, Deininger et al. (2022) revealed that smallholder farmers are the most vulnerable group. They own 60% of the agricultural land and have experienced the most severe output losses, perpetuated by agricultural resource shortages such as labor, seed, fertilizers, and pesticides.
5. Over-reliance on major food exporters/importers and inequalities in agrifood business
Although the Russia–Ukraine conflict is not the sole cause of the current global food crisis, it has highlighted deeply inequitable agrifood systems (Hawkes et al., 2022). One of the inequities is the over-reliance on major food exporters, driven by the high global demand and reliance on a few major cereal crops, such as wheat, maize, and rice, much of which are consumed by populations in developing countries, and maize is also highly used for feed and biofuel applications (Figure 4).
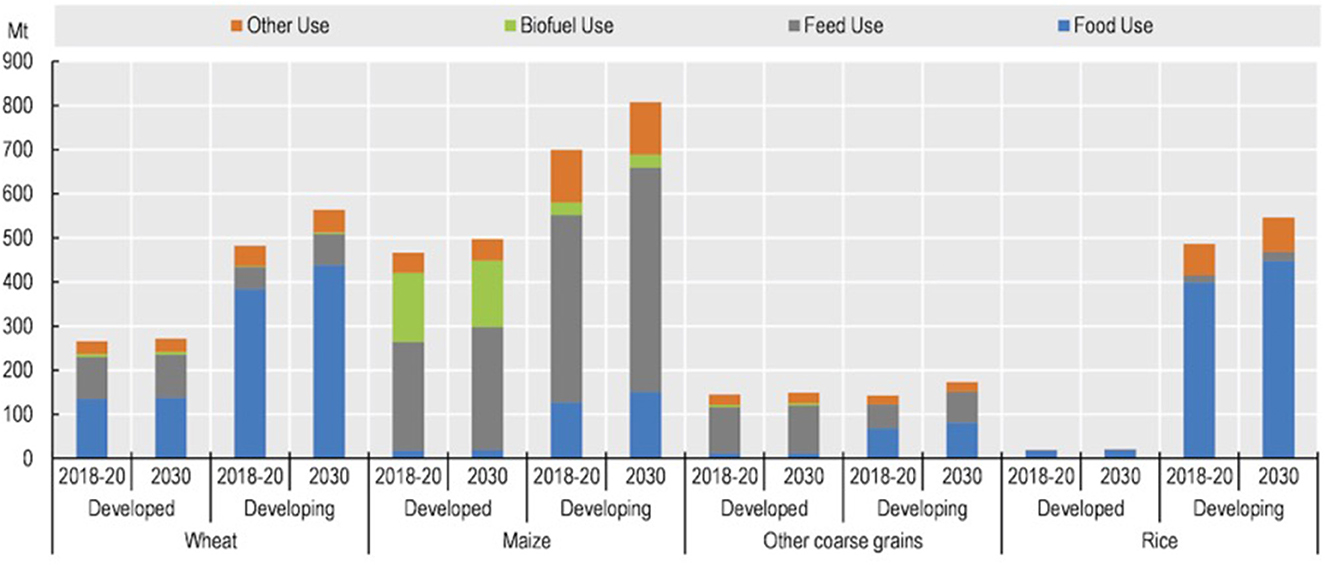
Figure 4. Cereal use in developed and developing countries (OECD/FAO, 2019) (Figure re-produced with permission from UHViUmlnaHRzQG9lY2Qub3Jn).
Often, global staple food producers are concentrated in a small number of countries. For example, for the top staple commodities based on gross production value—rice, maize, wheat, and soybean—the biggest net producers and exporters are China, India, the USA, Brazil, and Russia (FAOSTAT, 2022) (Figure 5). The top rice-producing countries are China and India, producing 50% of global rice production. The top maize-producing countries are the USA, China, and Brazil, producing 60% of global maize production. China, India, Russia, and the USA produce 49.6% of the world's wheat, while Brazil and the USA produce 66.3% of the world's soybean (FAOSTAT, 2022). The Middle Eastern, North African, and East Asian regions are the largest net importers of these commodities, yet also have the highest rates of food insecurity (OECD/FAO, 2022b).
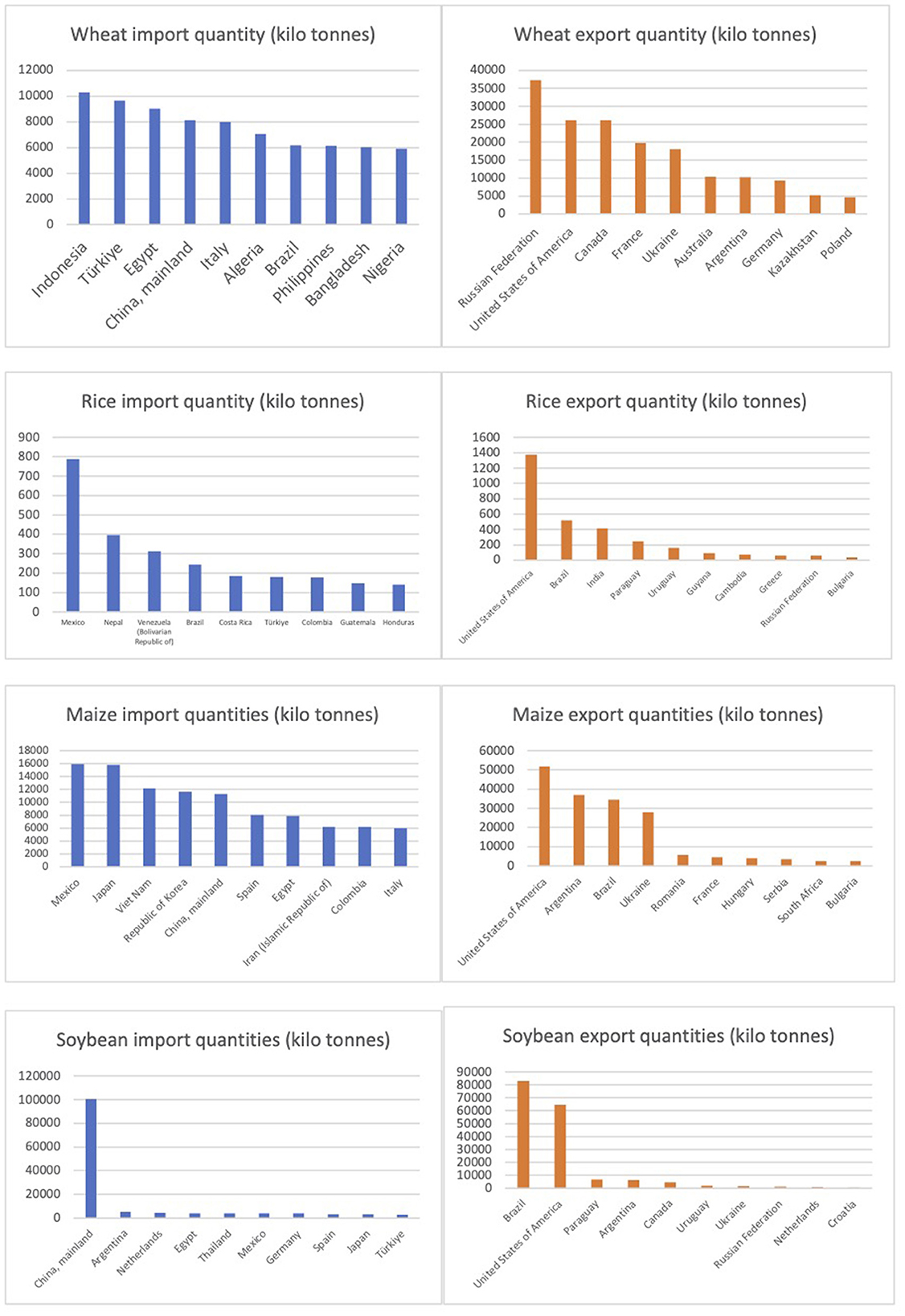
Figure 5. Import and export quantities (kilo tons) for the four major staple crops (wheat, rice, maize, and soybean) (FAOSTAT, 2022).
Major agrifood players are key in ensuring global food security through international trade. However, models of the relationships between population growth, food production, and trade dynamics have shown that over-dependence on food imports impairs global food security (Suweis et al., 2015). Low-income food-deficit regions, such as Africa, the Middle East, and Latin America, are most vulnerable to price shocks in food trade and least able to protect themselves against domestic food production shocks (Grassia et al., 2022). Worse still, the UN International Labor Organization said in its 2021 report that people in these countries live with much lower rates of social welfare protection. For example, Africa has the lowest level, with 17.4% of the population having access to social welfare protection, compared to Europe and Central Asia, where 84.0% of the population have access to social welfare protection (International Labour Organization, 2021). Consumers in low-income countries spend relatively more on food (40% of spending in sub-Saharan Africa) than high-income countries (17% in advanced economies) (Bogmans et al., 2022), such that food price variabilities potentially result in poor people being unable to afford enough, presenting a conundrum for these affected countries because they increasingly need to import food to meet the needs of rapid population growth (van Berkum, 2021).
The Russia–Ukraine conflict has exposed inequalities in the international agribusiness sector. In the last decades, there has been a trend toward consolidation of corporations that supply food and agricultural inputs through large-scale mergers such as Kraft and Heinz, Dow and Dupont, Bayer and Monsanto, with each company's worth reaching over US$60–100 billion after merging (Clapp, 2022). These companies play a crucial role in global food supply, at the same time influencing the volatility of the international price of food commodities. In the agrochemical industry, the top four companies—Syngenta Group (China), Bayer (Germany), BASF (Germany), and Corteva (USA) dominate 62% of the global agrochemical market, where Bayer and Corteva also control 40% of the global seed market (ETC Group, 2022).
6. Role of diversification in four aspects of agrifood systems
6.1. Market diversification
The projected increase in global food demand of 1.4% per annum over the coming decade, stimulated by a large growth in the world's population and per capita income (OECD/FAO, 2022a), will place tremendous pressure on major food exporters to ensure consistent agricultural outputs and to develop high resilience to various shocks. The main exporters of wheat and other staple products are sometimes major importers of the same crop. For example, Asia's top five wheat producers (China, India, Pakistan, Turkey, and Iran) contributed 332.94 Mt to global production, compared with 260.23 Mt in Europe in 2019. However, their wheat imports (74.19 Mt) exceeded their exports (6.61 Mt), attributed to the limited countries in Asia that meet the required growth conditions for bread wheat (Sendhil et al., 2022). However, with modern breeding technologies, many cereal crop varieties can withstand adverse climatic patterns, providing breakthroughs that allow crops to be grown outside their typical environments. For instance, high-yielding, heat-tolerant wheat varieties have been developed from large-scale field trials and germplasm screening in the Senegal River, a joint effort by ICARDA and the Swedish University of Agriculture (Bassi, 2017; Sall et al., 2018). Abandoned land in many developing countries could be used to cultivate staple food crops that fit the region's ecology, despite originating from other geographic regions.
To minimize the global impact of the food crisis, it is critical to diversify the import/export sources of food crops. For example, New Zealand has recently diversified its avocado export markets from Australia, where it previously shipped 79% of its total exports in the last 5 years, to Asian countries such as Singapore, Hong Kong, and South Korea after a decline in Australia's import demand (F+B Tech, 2022; Piggott, 2022). This is a win-win situation for establishing food resilience where the exporting country retains its role as the food supplier while the importing country benefits from securing food products to meet domestic food demands. Singapore imports 90% of its food from more than 170 countries, with food items coming from geographically diverse regions; the country continues to identify new food sources as part of its strategy to ensure market diversification with constant food supply (Teng, 2020; Singapore's Food Supply, 2022). The FAO measurement of countries with multiple food trade partners to ensure food security and nutrition—using the dietary sourcing flexibility index (DSFI)—is an attractive approach to market diversification and minimizing the adverse impact of a food crisis. The DSFI index measures food supply diversity based on multiple sourcing pathways of food commodities, imports, and available stocks. Countries with high DSFI values, such as Israel, Lebanon, Norway, and the UK, have a high capacity to absorb shocks with multiple options for food suppliers coming from diverse trade partners and multiple commodities. This contrasts with countries such as Indonesia, Madagascar, Pakistan and the Republic of Moldova with limited trade partners and fewer food commodity options (FAO, 2021).
Food market diversification on the international front requires trade agreement policies between nations, with transparent, updated information provided to all nations regarding agricultural markets. For example, the Agricultural Market Information System (AMIS), comprising G20 members with additional major exporters and importers of agricultural commodities, makes concerted efforts to enhance international food trade policy using real-time data of food markets to prevent price hikes (Hertel et al., 2021; Agricultural Market Information System, 2022). At the regional level, for example, the project initiative “Agricultural Transformation and Market Integration in the ASEAN Region: Responding to Food Security and Inclusiveness Concerns (ATMI-ASEAN),” supported by the International Food Policy Research Institute (IFPRI), addresses food security issues within the ASEAN region to increase smallholder farmer competitiveness in regional agrifood markets by identifying diverse crops with high impact value, with cooperation between agricultural stakeholders in member countries—Cambodia, Lao PDR, Myanmar, Viet Nam and the Philippines (Southeast Asian Regional Center for Graduate Study and Research in Agriculture, 2017). In addition, market diversification involves shifting part of the demand and supply for staples to non-staple foods such as fruits, vegetables, and pulses, considered high in protein and micronutrients, entering such food markets for a more balanced diet and nutritional outcomes (Pingali, 2015).
Another way to diversify food markets is to promote the production and commercialization of alternative foods sourced sustainably (e.g., plant-based foods). This approach has good health effects and diversity of flavors, tastes, and textures, increasing consumers' menu options and making food markets more resilient to price shocks. For example, consumers are receptive to blending wheat flour with cassava flour, indicating the potential use of minor crops as alternative foods (Owusu et al., 2017). Governments could provide funds to start-up companies involved in “ecologically friendly” food production or businesses involved in agricultural technologies to stimulate the demand for alternative crops, boosting the country's economy and supporting food security and healthy living.
Domesticating indigenous fruit tree species (IFTs), and herbaceous species within the broad traditional foods and medicinal plants can also be a good option to diversify food markets (Leakey, 2014; Leakey et al., 2022). We can achieve this by extending the food market and value chain to include nutrient-dense foods that fall under the radar of public attention, including neglected and underutilized species (NUS) and indigenous fruit tree species, such as Strychnos madagascariensis (fruit processed as flour) from Africa countries (Chemane et al., 2022) and Garcinia andamanica King (fruit suitable as a food additive) from Andaman Islands, India (Bohra et al., 2021). To increase the marketability of these alternative foods, attention should be drawn toward strengthening independent local and regional food systems. This can be achieved by providing a support network to farmers selling through a direct-to-consumer channel or in short food supply chains (SFSC) markets (Jarzebowski et al., 2020; Schreiber et al., 2022). For instance, policymakers ought to put into action the delivery of high-quality workflow for the value-added processing, storage and distribution of fresh products to local communities that can help farmers retain a larger portion of the retail value of food, and enhance resilience to shocks in the international supply chain (Enthoven and Van den Broeck, 2021).
6.2. Production diversification
Due to soaring costs, the Russia–Ukraine conflict has put immense pressure on farmers and supply chains relying on fertilizer, feed, and fuel. How we produce food crops has to be diversified as part of food sustainability strategies. Intensive agriculture, driven by the Green Revolution in the mid-20th Century, emphasized monoculture, crop hybridization, and reliance on fertilizers and other agrochemicals that increased yield. However, these developments also resulted in increased reliance on international supply chains and had unintended impacts on natural resources, such as water and land, due to the heavy use of fertilizers and other agrochemicals (United Nations, 2004; Rosa et al., 2021; Soria-Lopez et al., 2022). In contrast, sustainable intensification in the 21st Century seeks to leverage on least agricultural inputs to achieve equal if not higher crop yield without incurring the environmental impact and further expansion of cropland (Pretty and Bharucha, 2014; Campanhola and Pandey, 2019). This involves using improved crops, regenerative agricultural methods, and diversified production and distribution systems. The concept of sustainable intensification was incepted by FAO in 2011 under the “Save and Grow” model, aiming to achieve food security through balancing increasing productivity and positive outcomes for the environment (Collette et al., 2011).
Diversification of agricultural practices, such as intercropping spatially or temporally, mixed farming with crop and livestock, adding organic material or beneficial microbes in the soil, and reduced tillage, has contributed to biodiversity conservation, improved soil fertility, enhanced nutrient cycling and water regulation, and improved pest control, decreasing the environmental burden without compromising crop yield (Tamburini et al., 2020; Yan et al., 2022). For example, intercropping systems with grain legumes such as pigeonpea (Cajanus cajan) or leguminous tree species such as fliricidia (Gliricidia sepium) in smallholder maize farming in Tanzania, East Africa, has shown that the grain yield, caloric and protein yields were not inferior to monoculture maize. The other benefit is the maize-pigeonpea intercrop requires a reduced land area to produce the same grain yields under drought and well-watered conditions (Renwick et al., 2020). Agroforestry tree and shrub species in semi-arid West African parklands in which forests areas were cleared for agriculture farming purposes have been shown to restore both soil fertility and health by providing rich organic biomass through the burial of ramial wood or small branches during forest management (Félix et al., 2018). Depending on the type of tree and shrub species, for example, legume tree Leucaena leucocephala and berry fruit tree Phalsa (Grewia asiatica), when utilized as an intercrop or rotational crop with different food crops including cereals, leguminous and oilseeds, would not only improve crop yield (Sileshi et al., 2011; Rathore et al., 2022) but provide far-reaching benefits for the farmers, environment and food security. Farm diversification among smallholder farmers in Bangladesh and Central Malawi, including alternative crops, livestock and aquaculture farming, has seen a significant shift toward diversified diets and high nutrient assimilation as these farmers consume their farm produce and improve their household income and food security (Mango et al., 2018; Rehan et al., 2021).
Simulation and modeling studies of sustainable intensification in the Brazilian Amazon basin have shown that, adding soybean cultivation to livestock production areas, has multiple environmental and yield benefits, increasing soybean production from 2022/2023 forecasted 144–162 Mt without deforestation and decreasing climate warming by up to 58% (Marin et al., 2022; USDA FAS, 2022). Cropping system diversification, for example, rotational sequences of cereals/grasses such as rice and maize with legumes such as peas and mungbean in China, India, and Bangladesh, or mixtures of pea, oilseeds, and wheat in the Boreo-Nemoral region (Scandinavian or Northern Europe countries) have improved system productivity through higher crop resource use efficiencies with great potential for increasing nutrient levels and reducing carbon footprints (Lizarazo et al., 2020; Chai et al., 2021; Emran et al., 2022; Gora et al., 2022).
Integrated pest management (IPM) for crop protection also taps into diversified strategies to prevent, avoid or reduce pests using ecologically sound methods such as crop rotation, natural and biological controls, and host plant pest resistance or tolerance, and synergies between these strategies. One example is biofumigation by burying into the soil the natural glucosinolate sourced from plant members within the Brassicaceae family to suppress the pests (Richard et al., 2022). Another example of IPM is the control of the moth Tuta absoluta. This South American tomato pinworm pest severely destroys tomato production worldwide, using biological agents such as bacteria, fungi, nematodes, and predatory arthropods (Desneux et al., 2022). Recently, IPM technology (IPMT) has been proposed, which expands from IPM that incorporates the components of genetically engineered cultivar and biotechnology approaches that are relevant to today's agricultural setting with the focus on monitoring, identification, assessment, and prevention of pests with minimal environmental impacts using data-driven tools (Tanda, 2022). For example, the Intelligent and Integrated Pest and Disease Management (I2PDM) system was developed to capture the presence of plant pests such as thrips and whiteflies under a controlled environment setting, which greatly enhance the efficiency of plant disease monitoring (Rustia et al., 2022).
Smallholder farmers play an important role in supplying food globally (Terlau et al., 2019). All the positive outcomes of diversification described above cannot reach their full potential impacts in addressing global or regional food security without providing strong socio-economic support to smallholder farmers and ensuring that farming activities are enriching (Giller et al., 2021). There will also need to be a transformation in agronomy knowledge whereby on top of the hard sciences, integration of social science disciplines such as sociology, economics, and policy studies is embraced for future knowledge-based sustainable farming (Struik and Kuyper, 2017; Klerkx et al., 2019). Understanding farmers' difficulties in farm practices, with strong support from government agriculture agencies offering attractive subsidies, zero- to low-interest micro-financing, proper training and guidance, and close monitoring of agricultural outputs is essential (Epule, 2019). On the national front, strong support from the government through incentives, allowances, and subsidies to smallholder farmers to reinforce crop cultivation is an effective measure for household food security.
6.3. Crop diversification
For the past 60 years, human food sources that provide macronutrients such as energy, protein, and fat have primarily come from 50 crop commodities, including wheat, rice, maize, barley, potatoes, and general vegetable and fruit commodities comprising < 100 plant species (Khoury et al., 2014; Massawe et al., 2016; Shelef et al., 2017). With 7,039 known edible plant species recorded across 2,319 genera (Kew Gardens, 2020), there is still a large portion of edible plants that we could use for food diversification, including neglected and underutilized food crops (NUS) (Sogbohossou et al., 2018; Mustafa et al., 2019).
NUS currently have limited production and market values compared to major staples (Khoury et al., 2022). They have been nurtured as native crops agriculturally suited to local or regional climates and grown in domesticated forms or introduced centuries ago and are now established in their local environments. NUS are breeding candidates for advanced food crops with great economic potential (Mabhaudhi et al., 2019; Tirnaz et al., 2022). NUS cover a wide range of crop types, including cereals, roots and tubers, legumes, fruits and vegetables, seeds and spices, and were often traditionally grown by indigenous people and smallholder farmers as food for home consumption or a source of income at local markets (Padulosi et al., 2019; Heindorf et al., 2021). NUS can be found across various agroclimatic regions and landscapes suitable for agriculture. For example, 77 NUS in the form of leafy vegetables, tuber, edible wild fruits, and legumes have been found in Vietnam highlands (Vu and Nguyen, 2017).
Research into NUS has gained momentum over the years, with a focus on diversifying food crops to fight hunger and provide agricultural resilience (Li and Siddique, 2020; Mustafa et al., 2021; Siddique et al., 2021), and some could be mainstreamed into the food system, particularly those rich in protein and minerals or medicinal properties (Mudau et al., 2022). Bambara groundnut (Vigna subterranea) (Mayes et al., 2019; Tan et al., 2020), winged bean (Psophocarpus tetragonolobus) (Adegboyega et al., 2019; Tanzi et al., 2019) and amaranth (Amaranthus spp.) (Sarker et al., 2020; Jamalluddin et al., 2021) have high fiber content and nutrients, such as protein, carbohydrates, unsaturated fatty acids, and essential minerals. They are thus good candidates to supplement food systems to overcome malnourishment in adults and children (Talabi et al., 2022). Breeding programs have been developed to produce improved varieties of NUS crop species by initially characterizing germplasm. The African Orphan Crops Consortium (AOCC), in global partnership with biotechnology industries and research institutions, has initiated plans to assemble the genome sequence of 101 traditional African food crops for genetic improvement (Jamnadass et al., 2020; African Orphan Crops Consortium, 2022). To date, the genomes of at least 29 orphan crops in nine families have been sequenced, including Fonio (Digitaria exilis) (Abrouk et al., 2020), African eggplant (Solanum aethiopicum), breadfruit (Artocarpus altilis), apple-ring acacia (Faidherbia albida), Bambara groundnut (Vigna subterranea), jackfruit (Artocarpus heterophyllus), lablab (Lablab purpureus), marula (Sclerocarya birrea) and moringa (Moringa oleifera) (Chapman et al., 2022).
Indigenous fruit tree species (IFTs), which also falls under underutilized food crop, should be given equal emphasis as candidates for crop diversification. Tree commodities play a crucial role in diversifying crop production through agroforestry practices, which contribute to sustainable development goals in Africa (Mbow et al., 2014; Minang et al., 2021). Domesticated IFTs have two main benefits, the first being naturally nitrogen-fixing tree species such as leguminous type can help restore the land and improve soil nutrient availability. Second, fruit trees offer nutritious foods, with a surplus, can also be turned into a source of income for farmers from fruit selling, on top of fuel wood and timber trades (Leakey, 2014, 2020). Both benefits of agroforestry practices have translated into overall farmers' wellbeing, as shown from interviews and discussions with subsistence farmers in western Kenya (Thorlakson and Neufeldt, 2012). IFTs are valuable for their edible fruits/nuts and most of their plant parts. For example, Garcinia livingstonei (African mangosteen)'s stem bark has a skin lightening effect on human skin (Mulholland et al., 2013), and its fruit contains high levels of macro and micro elements (Joseph et al., 2017). At least 29 IFT species within the South African provinces have been identified as potentials for commercialization (Nkosi et al., 2020). Domesticating IFTs through species diversity characterization could potentially close the yield gap, thereby increasing the harvest yield (Sulieman and Mariod, 2019).
Crop wild relatives and their associated weedy forms of agricultural crops are great genetic resources for trait improvement in cultivated species, including tolerance to a range of abiotic and biotic stresses, with many carrying out the C4 photosynthesis and thus adaptable to warm and dry environmental conditions (Ye and Fan, 2021). With good breeding models, prediction tools for the optimal selection of useful alleles and their introgression into elite pools with minimal linkage drag are possible (Cowling, 2013; Cowling et al., 2017). An initiative to collect wild, weedy, or landrace materials representing 29 target crops sourced from their country of origin and other exotic places was carried out by the Crop Trust Crop Wild Relatives Project, with expertise from 170 worldwide research institutes and breeding programs in 70 countries to conserve valuable wild germplasm in ex-situ collections, for plant breeders, researchers and farmers (Khoury et al., 2010; Crop Trust Annual Report 2021, 2022). Output from the project includes the collection of eggplant Solanum genus wild relatives from partners in 12 countries, 17 samples of wild Bambara groundnut from Nigeria not found in genebanks previously, and pre-breeding lines derived from crop wild relatives and landraces of grasspea and finger millet, with more than 14,000 pre-bred lines from 19 crops available to crop researchers and farmers (Crop Trust, 2019; Crop Trust Annual Report 2021, 2022).
6.4. Technology diversification
Advances in agricultural practices and plant breeding methods are key to securing food for the future. Collaborative networks between farmers, private and government research institutions, agronomists, researchers, and industry professionals in breeding programs drive knowledge and technology transfer through active dialogues and participatory research. Such an example includes the sorghum network in Mali involving ICRISAT and farmer organizations to strengthen the seed system (Rattunde et al., 2021). Supported by reliable international, regional, or local agricultural research funding bodies and expertise, integrating farmers' indigenous in-farm knowledge with modern scientific knowledge in plant breeding will stimulate innovation and real-life application to boost sustainable agriculture productivity (Dawoe et al., 2012; Pagliarino et al., 2020). Farmer field school offers an experiential learning approach where a group of farmers works together with the facilitator, discussing regularly the various situations encountered in the field and coming up with solutions of empowering farmers' capability in agrosystem management and expand their knowledge base (Bakker et al., 2021, 2022).
Cutting-edge biotechnology tools, such as genome sequencing, pangenomics, SNP marker discovery, and genotyping, genome-wide association studies, and genomic selection approaches, have revolutionized crop breeding with smarter and more targeted ways to improve crop productivity (Bayer et al., 2021; Marsh et al., 2021), as seen by the advances in many major crop species and application in NUS crops (Tay Fernandez et al., 2022). These tools will benefit crop breeding, provided sufficient plant material is available that covers a wide genetic diversity for each crop species (major and NUS) (Khan et al., 2020; Mohd Saad et al., 2021).
Viable plant materials from the world's food crops are well conserved in genebanks worldwide. Online resources such as Genesys, containing the genebank database, make it convenient for researchers to perform research and studies (Genesys, 2022). For example, the current wheat germplasm collection contains more than 800,000 accessions held in 80 different collections, with CIMMYT hosting the largest wheat collection worldwide (Crop Wild Relative Diversity, 2022). This large pool of genetic resources, with as many as 80,000 wheat accessions sourced from tetraploid species, wild relatives, and landraces, has been studied for its genetic diversity using DArTseq and SilicoDArT approaches (Sansaloni et al., 2020). The global genetic yield gap for wheat is estimated at 51% due to suboptimal crop and soil management, indicating that there is room to improve crop productivity through genetic improvements by tapping into the large wheat germplasm resources (Senapati et al., 2022). Another example is the genotyping of the entire collection of barley accessions from the German ex-situ collection, with 22,626 accessions, using genotyping-by-sequencing to differentiate and track redundant material in the genebank (Milner et al., 2019). Combining high-throughput genotyping with high-throughput phenotyping (Danilevicz et al., 2021) using machine learning (Khotimah et al., 2020) makes it possible to accurately identify the diversity underlying agronomic traits.
Maize, wheat, and soybean productivity can be improved to reduce import requirements by combining various modern breeding techniques, such as genomics-assisted breeding, phenomics, artificial intelligence, and gene editing (Naqvi et al., 2022), to overcome the limitations of climate-dependent food crops by developing tropical varieties. Besides, investing in the latest breeding technologies would facilitate knowledge transfer from lab to the field, potentially improving crop productivity. For example, Japan, Korea, and China are the origin sites of domestication for soybean; on-site plant materials collection and data analysis supported by genomics facilities means cost-saving in terms of time and resources at the same time, translating research outputs into improving soybean production in their own countries (Li et al., 2020). The International Maize and Wheat Improvement Center (CIMMYT) developed maize hybrids acclimated to South and South East Asia climate zones (CIMMYT, 2019).
Crop pangenomics resources are useful for plant breeding and improvement, revealing valuable information for breeders to tap into from the large diversity of a species (Golicz et al., 2016; Bayer et al., 2020, 2022a; Zanini et al., 2022). For example, the soybean pangenome from the USDA collection revealed more genes with lower frequencies, such as those controlling plant architecture and seed composition, than those with higher frequencies, such as those controlling flowering time and stress tolerance traits; this gives breeders an idea of which genes to select from which accession during the selective breeding process (“smart” breeding) (Bayer et al., 2022b). In addition to genomes and pangenomes being developed for major crops, they are also being constructed for crops important in developing countries, such as banana (Rijzaani et al., 2022), yam bean (Tay Fernandez et al., 2021), sorghum (Ruperao et al., 2021), chickpea (Varshney et al., 2013) and pigeonpea (Zhao et al., 2020). Ongoing efforts have been made to build pangenomics resources for developing climate-ready crops (Marsh et al., 2021; Petereit et al., 2022).
Crop mutation breeding, a plant breeding technique that uses induced mutation in plants for better adaptability to the environment, generates genetic variation for crop improvement (Forster and Shu, 2012). The Plant Mutation Breeding Network for Asia and the Pacific established by the Joint FAO/IAEA Center successfully released improved varieties of cowpea in Zimbabwe using the irradiation method, with improved drought tolerance and insect resistance and increasing yields by 10–20% (Dixit and Slavchev, 2018). With the development of genome editing techniques, it is possible to generate precise modifications in genomes. When this technology is combined with the knowledge of pangenomes and associated traits, it is possible to accelerate the production of climate-ready crops to support future food security (Mohd Saad et al., 2021; Varshney et al., 2021a,b; Derbyshire et al., 2022).
In summary, by considering the diversification in four aspects of agrifood systems, we believe the goal of attaining a more sustainable, shock-resilient, and improved global food security is not far-reaching (Figure 6).
7. Future perspectives and conclusion
The Russia–Ukraine conflict has highlighted the vulnerability of global food security and underscored the critical need to achieve food resilience by transforming and diversifying agrifood systems. We propose diversification in food markets, production, crop, and technology to secure global food supply and build resilience toward future shocks. Specifically, there should be stronger support for increasing market demand for alternative foods. It is important to consider sustainable intensification in producing sufficient food to feed the growing global population with minimal risks to the natural ecosystems. Concerted efforts at the global, regional, national, and local levels are needed to fulfill the mandate of global food security through policymaking, increased consumer awareness, knowledge of food markets, adding value with NUS crops, and research investments using advanced biotechnology tools to enhance crop productivity. At the smallholder farmers' level, the efficiency and productivity of production and processing markets for food crops, including indigenous fruit trees, could be improved by creating platforms for farmers to distribute their fresh harvest directly to consumers. Food system experts indicate that food resilience research should focus on prediction tools and high-quality, local-scale on-the-ground data collection to determine the impact of extreme events on food security (Mehrabi et al., 2022). Within the context of long-term food crop trials, practical implementation via participatory on-farm research using real-time farming challenges could provide solutions for NGOs, consumers, academia, policymakers, and value chain experts (Riar and Bhullar, 2020). Incorporating all these roles, carried out by diverse players in the agri-food industry, should combat hunger by 2030 and fight the global food crisis.
Author contributions
TN and FM conceptualized the study. TN wrote the first draft of the manuscript. BKS wrote sections of the manuscript. All authors contributed to manuscript revision, read, and approved the submitted version.
Funding
The authors acknowledge funding support from the Future Food Beacon of Excellence, University of Nottingham, and the Monash University Malaysia (MUM)-ASEAN Sustainable Development Research Grant (ASEAN-2019-01-SCI).
Acknowledgments
We thank Professor Wallace Cowling from The University of Western Australia for providing valuable insights into the study.
Conflict of interest
The authors declare that the research was conducted in the absence of any commercial or financial relationships that could be construed as a potential conflict of interest.
The reviewer RL declared a past co-authorship with the author TM to the handling editor.
Publisher's note
All claims expressed in this article are solely those of the authors and do not necessarily represent those of their affiliated organizations, or those of the publisher, the editors and the reviewers. Any product that may be evaluated in this article, or claim that may be made by its manufacturer, is not guaranteed or endorsed by the publisher.
References
Abay, K. A., Abdelradi, F., Breisinger, C., Diao, X., Dorosh, P. A., Pauw, K., et al. (2022). Egypt: Impacts of the Ukraine and Global Crises on Poverty and Food Security. Washington, DC: IFPRI. doi: 10.2499/p15738coll2.136321
Abrouk, M., Ahmed, H. I., Cubry, P., Šimoníková, D., Cauet, S., Pailles, Y., et al. (2020). Fonio millet genome unlocks African orphan crop diversity for agriculture in a changing climate. Nat. Commun. 11, 4488. doi: 10.1038/s41467-020-18329-4
Adegboyega, T. T., Abberton, M. T., AbdelGadir, A. H., Dianda, M., Maziya-Dixon, B., Oyatomi, O. A., et al. (2019). Nutrient and antinutrient composition of winged bean (Psophocarpus tetragonolobus (L.) DC.) seeds and tubers. J. Food Qual. 2019, 3075208. doi: 10.1155/2019/3075208
African Development Bank Group (2022). African Development Bank Group Board Approves More Than $1 Billion for Emergency Food Production Plan. Abidjan: African Development Bank Group. Available online at: https://www.afdb.org/en/news-and-events/press-releases/african-development-bank-group-board-approves-more-1-billion-emergency-food-production-plan-53584 (accessed August 26, 2022).
African Orphan Crops Consortium (2022). African Orphan Crops Consortium. Nairobi: AOCC. Available online at: https://africanorphancrops.org (accessed September 26, 2022).
Agricultural Market Information System (2022). About AMIS. New York, NY: AMIS. Available online at: http://www.amis-outlook.org/amis-about/en/ (accessed September 19, 2022).
Alpízar, F., Saborío-Rodríguez, M., Martínez-Rodríguez, M. R., Viguera, B., Vignola, R., Capitán, T., et al. (2020). Determinants of food insecurity among smallholder farmer households in Central America: recurrent vs. extreme weather-driven events. Reg. Environ. Change 20, 22. doi: 10.1007/s10113-020-01592-y
Anderson, R., Bayer, P. E., and Edwards, D. (2020). Climate change and the need for agricultural adaptation. Curr. Opin. Plant Biol. 56, 197–202. doi: 10.1016/j.pbi.2019.12.006
Anderson, W., Taylor, C., McDermid, S., Ilboudo-Nébié, E., Seager, R., Schlenker, W., et al. (2021). Violent conflict exacerbated drought-related food insecurity between 2009 and 2019 in sub-Saharan Africa. Nat. Food 2, 603–615. doi: 10.1038/s43016-021-00327-4
Arezki, R., and Bruckner, M. (2011). Food prices and political instability. IMF Work. Papers 2011, A001. doi: 10.5089/9781455221066.001
Bakker, T., Dugué, P., and de Tourdonnet, S. (2021). Assessing the effects of Farmer Field Schools on farmers' trajectories of change in practices. Agron. Sustain. Dev. 41, 18. doi: 10.1007/s13593-021-00667-2
Bakker, T., Dugué, P., Roesch, K., Phillips, S., and Poisot, A. S. (2022). How Can the Farmer Field School Approach be Used to Support Agroecological Transitions in Family Farming in the Global South? Recommendations for Farmer Field School Facilitators, Agricultural Development Project Designers and Managers. Rome: FAO. Available: https://www.fao.org/documents/card/en/c/CB9920EN/ (accessed Feburary 13, 2023).
Bassi, F. M. (2017). ICARDA (Beirut: International Center for Agricultural Research in the Dry Areas (ICARDA)). Available online at: https://www.icarda.org/media/blog/adapting-durum-wheat-varieties-senegal-river-basin (accessed October 8, 2022).
Bayer, P. E., Golicz, A. A., Scheben, A., Batley, J., and Edwards, D. (2020). Plant pan-genomes are the new reference. Nat. Plants 6, 914–920. doi: 10.1038/s41477-020-0733-0
Bayer, P. E., Petereit, J., Danilevicz, M. F., Anderson, R., Batley, J., and Edwards, D. (2021). The application of pangenomics and machine learning in genomic selection in plants. Plant Genome 14, e20112. doi: 10.1002/tpg2.20112
Bayer, P. E., Petereit, J., Durant, É., Monat, C., Rouard, M., Hu, H., et al. (2022a). Wheat Panache: a pangenome graph database representing presence/absence variation across 16 bread wheat genomes. Plant Genome 15, e20221. doi: 10.1101/2022.02.23.481560
Bayer, P. E., Valliyodan, B., Hu, H., Marsh, J. I., Yuan, Y., Vuong, T. D., et al. (2022b). Sequencing the USDA core soybean collection reveals gene loss during domestication and breeding. Plant Genome 15, e20109. doi: 10.1002/tpg2.20109
Behnassi, M., and El Haiba, M. (2022). Implications of the Russia–Ukraine war for global food security. Nat. Hum. Behav. 6, 754–755. doi: 10.1038/s41562-022-01391-x
Bill Melinda Gates Foundation Goalkeepers Report 2022 (2022). The Future of Progress. Seattle: Bill and Melinda Gates Foundation. Available online at: https://www.gatesfoundation.org/goalkeepers/report/2022-report/?download=false (accessed December 9, 2022).
Bogmans, C., Kearns, J., Pescatori, A., and Prifti, E. (2022). War-Fueled Surge in Food Prices to Hit Poorer Nations Hardest. Washington, DC: International Monetary Fund. Available online at: https://blogs.imf.org/2022/03/16/war-fueled-surge-in-food-prices-to-hit-poorer-nations-hardest/ (accessed August 30, 2022).
Bohra, P., Waman, A. A., and Giri, N. A. (2021). Garcinia andamanica King (Clusiaceae): an important horticultural genetic resource from Indian islands. Genet. Resour. Crop Evol. 68, 1675–1689. doi: 10.1007/s10722-021-01136-1
Breisinger, C., Diao, X., Dorosh, P. A., Mbuthia, J., Omune, L., Oseko, E. O., et al (2022). Kenya: Impacts of the Ukraine and Global Crises on Poverty and Food Security. Washington, DC: FAO. Available online at: https://ebrary.ifpri.org/utils/getfile/collection/p15738coll2/id/135942/filename/136153.pdf (accessed October 28, 2022).
Campanhola, C., and Pandey, S., (eds.). (2019). “Chapter 10: Context for sustainable intensification of agriculture,” in Sustainable Food and Agriculture, (New York, NY: Academic Press), 171–172.
CGIAR (2022). More Than 20 Countries Issue a “Ban” on Grain Exports, Will Food Prices Rise Further? (eNorth). France: CGIAR. Available online at: https://www.cgiar.org/news-events/news/more-than-20-countries-issue-a-ban-on-grain-exports-will-food-prices-rise-further-enorth/ (accessed February 30, 2023).
Chai, Q., Nemecek, T., Liang, C., Zhao, C., Yu, A., Coulter, J. A., et al. (2021). Integrated farming with intercropping increases food production while reducing environmental footprint. Proc. Natl. Acad. Sci. 118, e2106382118. doi: 10.1073/pnas.2106382118
Chapman, M. A., He, Y., and Zhou, M. (2022). Beyond a reference genome: pangenomes and population genomics of underutilized and orphan crops for future food and nutrition security. New Phytol. 234, 1583–1597. doi: 10.1111/nph.18021
Chemane, S. S. I., Ribeiro, M., Pinto, E., Pinho, S. C. M., Martins, Z. S., Almeida, A., et al. (2022). Nutritional characterization of strychnos madagascariensis fruit flour produced by mozambican communities and evaluation of its contribution to nutrient adequacy. Foods 11, 616. doi: 10.3390/foods11040616
CIMMYT (2019). New CIMMYT Pre-Commercial Hybrids Available from Asia Maize Breeding Programs (International Maize and Wheat Improvement Center (CIMMYT)). Available online at: https://www.cimmyt.org/news/new-cimmyt-pre-commercial-hybrids-available-from-asia-maize-breeding-programs/ (accessed September 19, 2022).
Clapp, J. (2022). The Rise of Big Food and Agriculture: Corporate Influence in the Food System (Edward Elgar Publishing Ltd). 45–66. doi: 10.4337/9781800880269.00011
Collette, L., Hodgkin, T., Kassam, A., Kenmore, P., Lipper, L., Nolte, C., et al (2011). Save and Grow: A policymaker's Guide to the Sustainable Intensification of Smallholder Crop Production. Rome: Food and Agriculture Organization of the United Nations (FAO). Available online at: https://www.fao.org/3/I2215E/i2215e.pdf (accessed February 12, 2023).
Cowling, W. A., Li, L., Siddique, K. H. M., Banks, R. G., and Kinghorn, B. P. (2019). Modeling crop breeding for global food security during climate change. Food Energy Sec. 8, e00157. doi: 10.1002/fes3.157
Cowling, W. A., Li, L., Siddique, K. H. M., Henryon, M., Berg, P., Banks, R. G., et al. (2017). Evolving gene banks: improving diverse populations of crop and exotic germplasm with optimal contribution selection. J. Exp. Bot. 68, 1927–1939. doi: 10.1093/jxb/erw406
Crop Trust (2019). A Global Rescue: Safeguarding The World's Crop Wild Relatives. Deerfield: G.C.D. Trust. Available online at: https://www.croptrust.org/fileadmin/uploads/croptrust/Documents/A-Global-Rescue-Safeguarding-the-Worlds-Crop-Wild-Relatives.pdf (accessed September 15, 2022).
Crop Trust Annual Report 2021 (2022). 2021 Annual Report and Financial Statement. Germany: Crop Trust. Available online at: https://www.croptrust.org/resources/2021-annual-report-financial-statement/ (accessed September 15, 2022).
Crop Wild Relative Diversity (2022). Wheat (Bonn: Global Crop Diversity Trust). Available online at: https://www.cwrdiversity.org/crop/wheat/ (accessed September 28, 2022).
Danilevicz, M. F., Bayer, P. E., Boussaid, F., Bennamoun, M., and Edwards, D. (2021). Maize yield prediction at an early developmental stage using multispectral images and genotype data for preliminary hybrid selection. Remot. Sens. 13, 3976. doi: 10.3390/rs13193976
Dawoe, E. K., Quashie-Sam, J., Isaac, M. E., and Oppong, S. K. (2012). Exploring farmers' local knowledge and perceptions of soil fertility and management in the Ashanti Region of Ghana. Geoderma 179–180, 96–103. doi: 10.1016/j.geoderma.2012.02.015
Deininger, K., Ali, D. A., Kussul, N., Shelestov, A., Lemoine, G., and Yailimova, H. (2022). Quantifying War-Induced Crop Losses in Ukraine in Near Real Time to Strengthen Local and Global Food Security. Washington, DC: FAO. Available online at: https://openknowledge.worldbank.org/handle/10986/37665 (accessed September 10, 2022).
Derbyshire, M. C., Batley, J., and Edwards, D. (2022). Use of multiple ‘omics techniques to accelerate the breeding of abiotic stress tolerant crops. Curr. Plant Biol. 32, 100262. doi: 10.1016/j.cpb.2022.100262
Desneux, N., Han, P., Mansour, R., Arnó, J., Brévault, T., Campos, M. R., et al. (2022). Integrated pest management of Tuta absoluta: practical implementations across different world regions. J. Pest Sci. 95, 17–39. doi: 10.1007/s10340-021-01442-8
Diao, X., Dorosh, P. A., Kedir Jemal, M., Smart, J., Taffesse, A. S., and Thurlow, J. (2022a). Ethiopia: Impacts of the Ukraine and Global Crises on Poverty and Food Security (International Food Policy Research Institute (IFPRI)). Available online at: https://ebrary.ifpri.org/utils/getfile/collection/p15738coll2/id/135948/filename/136155.pdf (accessed October 20, 2022).
Diao, X., Dorosh, P. A., Smart, J., and Thurlow, J. (2022b). Bangladesh: Impacts of the Ukraine and Global Crises on Poverty and Food Security (International Food Policy Research Institute (IFPRI)). Available online at: https://ebrary.ifpri.org/utils/getfile/collection/p15738coll2/id/135947/filename/136158.pdf (accessed October 20, 2022).
Diffenbaugh, N. S., Singh, D., and Mankin, J. S. (2018). Unprecedented climate events: historical changes, aspirational targets, and national commitments. Sci. Adv. 4, eaao3354. doi: 10.1126/sciadv.aao3354
Dixit, A., and Slavchev, S. (2018). New Mutant Cowpea Variety Helps Zimbabwe's Farmers in Drought Prone Areas. Vienna: International Atomic Energy Agency. Available online at: https://www.iaea.org/newscenter/news/new-mutant-cowpea-variety-helps-zimbabwes-farmers-in-drought-prone-areas (accessed September 19, 2022).
Donley, A. (2022). List of Countries Restricting Grain Exports Growing. New York, NY: World-Grain.com. Available online at: https://www.world-grain.com/articles/16917-list-of-countries-restricting-grain-exports-growing (accessed August 29, 2022).
Emran, S.-A., Krupnik, T. J., Aravindakshan, S., Kumar, V., and Pittelkow, C. M. (2022). Impact of cropping system diversification on productivity and resource use efficiencies of smallholder farmers in south-central Bangladesh: a multi-criteria analysis. Agron. Sustain. Dev. 42, 78. doi: 10.1007/s13593-022-00795-3
Enthoven, L., and Van den Broeck, G. (2021). Local food systems: reviewing two decades of research. Agric. Syst. 193, 103226. doi: 10.1016/j.agsy.2021.103226
Epule, T. E. (2019). “Chapter 1: Contribution of Organic Farming Towards Global Food Security: An Overview,” in Organic Farming, eds S. Chandran, M.R. Unni, and S. Thomas (Sawston: Woodhead Publishing), 1–16. doi: 10.1016/B978-0-12-813272-2.00001-X
ETC Group (2022). Food Barons 2022: Crisis Profiteering, Digitalization and Shifting Power. Springfield: ETC Group. Available online at: https://etcgroup.org/content/food-barons-2022 (accessed December 7, 2022).
European Parliament Think Tank (2022). Russia's War on Ukraine: Maritime Logistics and Connectivity. Strasbourg: European Parliament. Available online at: https://www.europarl.europa.eu/thinktank/en/document/EPRS_ATA(2022)733603 (accessed November 8, 2022).
F+B Tech (2022). Avocado Industry: Diversification is Critical (Auckland: Review Publishing Co Ltd). Available online at: https://www.fbtech.co.nz/2022/08/01/avocado-industry-diversification-is-critical/ (accessed September 1, 2022).
FAO (2021). “Agrifood systems' resilience at national and subnational levels,” in The State of Food and Agriculture 2021. Making Agrifood Systems More Resilient to Shocks and Stresses, ed FAO (Rome: FAO), 21–45.
FAO (2022a). Information Note: The Importance of Ukraine and the Russian Federation for Global Agricultural Markets and the Risks Associated with the War in Ukraine. Rome: FAO. Available online at: https://www.fao.org/3/cb9013en/cb9013en.pdf (accessed October 13, 2022).
FAO (2022b). Net Food Importing Developing Countries (NFIC). FAO Hand-in-Hand Geospatial Platform. Rome: FAO. Available online at: https://data.apps.fao.org/catalog/dataset/special-country-groups/resource/56ac7f70-6286-426d-8579-555390927bc3 (accessed October 13, 2022).
FAO (2022c). World Food Situation: FAO Food Price Index. Rome: FAO. Available online at: https://www.fao.org/worldfoodsituation/foodpricesindex/en/ (accessed October 13, 2022).
FAO IFAD UNICEF, WFP, and WHO. (2022). The State of Food Security and Nutrition in the World 2022. Repurposing Food and Agricultural Policies to Make Healthy Diets More Affordable. Rome: FAO. Available online at: https://www.fao.org/documents/card/en/c/cc0639en (accessed October 13, 2022).
Félix, G. F., Scholberg, J. M. S., Clermont-Dauphin, C., Cournac, L., and Tittonell, P. (2018). Enhancing agroecosystem productivity with woody perennials in semi-arid West Africa. A meta-analysis. Agron. Sustain. Dev. 38, 57. doi: 10.1007/s13593-018-0533-3
Forster, B. P., and Shu, Q. Y. (2012). “Plant mutagenesis in crop improvement: basic terms and applications,” in Plant Mutation Breeding and Biotechnology, eds Q.Y. Shu, B.P. Forster, and H. Nakagawa (Wallingford: CABI), 9–20. doi: 10.1079/9781780640853.0009
Genesys (2022). Genesys (Bonn: Global Crop Diversity Trust). Available online at: https://www.genesys-pgr.org (accessed September 28, 2022).
Giller, K. E., Delaune, T., Silva, J. V., Descheemaeker, K., van de Ven, G., Schut, A. G. T., et al. (2021). The future of farming: Who will produce our food? Food Sec. 13, 1073–1099. doi: 10.1007/s12571-021-01184-6
Giordani, P. E., Rocha, N., and Ruta, M. (2016). Food prices and the multiplier effect of trade policy. J. Int. Econ. 101, 102–122. doi: 10.1016/j.jinteco.2016.04.001
Glauber, J., Laborde, D., and Mamun, A. (2022). From Bad to Worse: How Russia–Ukraine War-Related Export Restrictions Exacerbate Global Food Insecurity. Available online at: https://www.ifpri.org/blog/bad-worse-how-export-restrictions-exacerbate-global-food-security (accessed October 11, 2022).
Global Report on Food Crises (2022). Global Report on Food Crises: Acute Food Insecurity Hits New Highs. Rome: FAO. Available online at: https://www.fao.org/newsroom/detail/global-report-on-food-crises-acute-food-insecurity-hits-n;ew-highs/en (accessed September 28, 2022).
Golicz, A. A., Bayer, P. E., Barker, G. C., Edger, P. P., Kim, H., Martinez, P. A., et al. (2016). The pangenome of an agronomically important crop plant Brassica oleracea. Nat. Commun. 7, 13390. doi: 10.1038/ncomms13390
Gora, M. K., Kumar, S., Jat, H. S., Kakraliya, S. K., Choudhary, M., Dhaka, A. K., et al. (2022). Scalable diversification options delivers sustainable and nutritious food in Indo-Gangetic plains. Sci. Rep. 12, 14371. doi: 10.1038/s41598-022-18156-1
Grassia, M., Mangioni, G., Schiavo, S., and Traverso, S. (2022). Insights into countries' exposure and vulnerability to food trade shocks from network-based simulations. Sci. Rep. 12, 4644. doi: 10.1038/s41598-022-08419-2
Hart, T. G. B., Davids, Y. D., Rule, S., Tirivanhu, P., and Mtyingizane, S. (2022). The COVID-19 pandemic reveals an unprecedented rise in hunger: the South African Government was ill-prepared to meet the challenge. Sci. Afr. 16, e01169. doi: 10.1016/j.sciaf.2022.e01169
Harvey, C. A., Rakotobe, Z. L., Rao, N. S., Dave, R., Razafimahatratra, H., Rabarijohn, R. H., et al. (2014). Extreme vulnerability of smallholder farmers to agricultural risks and climate change in Madagascar. Philos. Trans. Roy. Soc. B Biol. Sci. 369, 20130089. doi: 10.1098/rstb.2013.0089
Hawkes, C., Ambikapathi, R., Anastasiou, K., Brock, J., Castronuovo, L., Fallon, N., et al. (2022). From food price crisis to an equitable food system. Lancet 400, 413–416. doi: 10.1016/S0140-6736(22)01348-4
Headey, D., and Fan, S. (2010). Reflections on the Global Food Crisis. How Did It Happen? How Has It Hurt? And How Can We Prevent the Next One? Washington, DC: IFPR Institute.
Heindorf, C., Reyes-Agüero, J. A., and van't Hooft, A. (2021). Local markets: agrobiodiversity reservoirs and access points for farmers' plant propagation materials. Front. Sustain. Food Syst. 5, 822. doi: 10.3389/fsufs.2021.597822
Hertel, T., Elouafi, I., Tanticharoen, M., and Ewert, F. (2021). Diversification for enhanced food systems resilience. Nat. Food 2, 832–834. doi: 10.1038/s43016-021-00403-9
Integrated Food Security Phase Classification (2022). IPC Acute Food Insecurity Classification. Rome: FAO. Available online at: https://www.ipcinfo.org/ipcinfo-website/contacts/en/ (accessed August 12, 2022).
International Labour Organization (2021). World Social Protection Report 2020–2022: Social Protection at the Crossroads in Pursuit of a Better Future. Geneva: International Labour Organization. Available online at: https://www.ilo.org/global/publications/books/WCMS_817572/lang–en/index.htm (accessed December 18, 2022).
Irmak, S., Sandhu, R., and Kukal, M. S. (2022). Multi-model projections of trade-offs between irrigated and rainfed maize yields under changing climate and future emission scenarios. Agric. Water Manag. 261, 107344. doi: 10.1016/j.agwat.2021.107344
Jamalluddin, N., Symonds, R. C., Mayes, S., Ho, W. K., and Massawe, F. (2021). “Chapter 6: Diversifying crops for food and nutrition security: A case of vegetable amaranth, an ancient climate-smart crop,” in Food Security and Nutrition, ed C. M. Galanakis (New York, NY:Academic Press), 125–146. doi: 10.1016/B978-0-12-820521-1.00006-X
Jamnadass, R., Mumm, R. H., Hale, I., Hendre, P., Muchugi, A., Dawson, I. K., et al. (2020). Enhancing African orphan crops with genomics. Nat. Genet. 52, 356–360. doi: 10.1038/s41588-020-0601-x
Jarzebowski, S., Bourlakis, M., and Bezat-Jarzebowska, A. (2020). Short food supply chains (SFSC) as local and sustainable systems. Sustainability 12, 4715. doi: 10.3390/su12114715
Johnstone, S., and Mazo, J. (2011). Global warming and the arab spring. Survival 53, 11–17. doi: 10.1080/00396338.2011.571006
Joseph, K. S., Bolla, S., Joshi, K., Bhat, M., Naik, K., Patil, S., et al. (2017). Determination of chemical composition and nutritive value with fatty acid compositions of African Mangosteen (Garcinia Livingstonei). Erwerbs-Obstbau 59, 195–202. doi: 10.1007/s10341-016-0311-9
Kew Gardens (2020). State of the World's Plants and Fungi. London: K. Gardens. Available online at: https://www.kew.org/science/state-of-the-worlds-plants-and-fungi (accessed September 2, 2022).
Khan, A. W., Garg, V., Roorkiwal, M., Golicz, A. A., Edwards, D., and Varshney, R. K. (2020). Super-pangenome by integrating the wild side of a species for accelerated crop improvement. Trends Plant Sci. 25, 148–158. doi: 10.1016/j.tplants.2019.10.012
Khotimah, W. N., Bennamoun, M., Boussaid, F., Sohel, F., and Edwards, D. (2020). A high-performance spectral-spatial residual network for hyperspectral image classification with small training data. Remot. Sens. 12, 3137. doi: 10.3390/rs12193137
Khoury, C., Laliberté, B., and Guarino, L. (2010). Trends in ex situ conservation of plant genetic resources: a review of global crop and regional conservation strategies. Genet. Resour. Crop Evolut. 57, 625–639. doi: 10.1007/s10722-010-9534-z
Khoury, C. K., Bjorkman, A. D., Dempewolf, H., Ramirez-Villegas, J., Guarino, L., Jarvis, A., et al. (2014). Increasing homogeneity in global food supplies and the implications for food security. Proc. Natl. Acad. Sci. 111, 4001–4006. doi: 10.1073/pnas.1313490111
Khoury, C. K., Brush, S., Costich, D. E., Curry, H. A., de Haan, S., Engels, J. M. M., et al. (2022). Crop genetic erosion: understanding and responding to loss of crop diversity. New Phytol. 233, 84–118. doi: 10.1111/nph.17733
Klerkx, L., Jakku, E., and Labarthe, P. (2019). A review of social science on digital agriculture, smart farming and agriculture 4.0: New contributions and a future research agenda. NJAS Wageningen J. Life Sci. 90–91, 100315. doi: 10.1016/j.njas.2019.100315
Leakey, R. R. B. (2014). The role of trees in agroecology and sustainable agriculture in the tropics. Ann. Rev. Phytopathol. 52, 113–133. doi: 10.1146/annurev-phyto-102313-045838
Leakey, R. R. B. (2020). A re-boot of tropical agriculture benefits food production, rural economies, health, social justice and the environment. Nat. Food 1, 260–265. doi: 10.1038/s43016-020-0076-z
Leakey, R. R. B., Tientcheu Avana, M.-L., Awazi, N. P., Assogbadjo, A. E., Mabhaudhi, T., Hendre, P. S., et al. (2022). The future of food: domestication and commercialization of indigenous food crops in Africa over the third decade (2012–2021). Sustainability 14, 2355. doi: 10.3390/su14042355
Li, M.-W., Wang, Z., Jiang, B., Kaga, A., Wong, F.-L., Zhang, G., et al. (2020). Impacts of genomic research on soybean improvement in East Asia. Theor. Appl. Genet. 133, 1655–1678. doi: 10.1007/s00122-019-03462-6
Li, X., and Siddique, K. H. M. (2020). Future smart food: Harnessing the potential of neglected and underutilized species for Zero Hunger. Maternal Child Nutr. 16, e13008. doi: 10.1111/mcn.13008
Lizarazo, C. I., Tuulos, A., Jokela, V., and Mäkelä, P. S. A. (2020). Sustainable mixed cropping systems for the boreal-nemoral region. Front. Sustain. Food Syst. 4, 103. doi: 10.3389/fsufs.2020.00103
Lodewick, C. (2022). Extreme Heat in the US, Europe and China is Slamming Economies Around the World—and Making Inflation Worse. Ashburn: Fortune. Available online at: https://fortune.com/2022/08/27/extreme-heat-slamming-economies-inflation-worse-us-china-europe/ (accessed August 29, 2022).
Lowder, S. K., Sánchez, M. V., and Bertini, R. (2021). Which farms feed the world and has farmland become more concentrated? World Dev. 142, 105455. doi: 10.1016/j.worlddev.2021.105455
Mabhaudhi, T., Chimonyo, V. G. P., Hlahla, S., Massawe, F., Mayes, S., Nhamo, L., et al. (2019). Prospects of orphan crops in climate change. Planta 250, 695–708. doi: 10.1007/s00425-019-03129-y
Mango, N., Makate, C., Mapemba, L., and Sopo, M. (2018). The role of crop diversification in improving household food security in central Malawi. Agric. Food Sec. 7, 7. doi: 10.1186/s40066-018-0160-x
Marin, F. R., Zanon, A. J., Monzon, J. P., Andrade, J. F., Silva, E. H. F. M., Richter, G. L., et al. (2022). Protecting the Amazon forest and reducing global warming via agricultural intensification. Nat. Sustain. 5, 1018–1026. doi: 10.1038/s41893-022-00968-8
Marsh, J. I., Hu, H., Gill, M., Batley, J., and Edwards, D. (2021). Crop breeding for a changing climate: integrating phenomics and genomics with bioinformatics. Theor. Appl. Genet. 134, 1677–1690. doi: 10.1007/s00122-021-03820-3
Massawe, F., Mayes, S., and Cheng, A. (2016). Crop diversity: an unexploited treasure trove for food security. Trends Plant Sci. 21, 365–368. doi: 10.1016/j.tplants.2016.02.006
Mayes, S., Ho, W. K., Chai, H. H., Gao, X., Kundy, A. C., Mateva, K. I., et al. (2019). Bambara groundnut: an exemplar underutilised legume for resilience under climate change. Planta 250, 803–820. doi: 10.1007/s00425-019-03191-6
Mbow, C., van Noordwijk, M., Prabhu, R., and Simons, T. (2014). Knowledge gaps and research needs concerning agroforestry's contribution to Sustainable Development Goals in Africa. Curr. Opin. Environ. Sustain. 6, 162–170. doi: 10.1016/j.cosust.2013.11.030
Mehrabi, Z., Delzeit, R., Ignaciuk, A., Levers, C., Braich, G., Bajaj, K., et al. (2022). Research priorities for global food security under extreme events. One Earth 5, 756–766. doi: 10.1016/j.oneear.2022.06.008
Milner, S. G., Jost, M., Taketa, S., Mazón, E. R., Himmelbach, A., Oppermann, M., et al. (2019). Genebank genomics highlights the diversity of a global barley collection. Nat. Genet. 51, 319–326. doi: 10.1038/s41588-018-0266-x
Minang, P. A., Kamwilu, E., and van Noordwijk, M. (2021). “Pathways to achieving sustainable development goals through tree commodities in Africa,” in Tree Commodities and Resilient Green Economies in Africa, eds M. Pa, D. La, and V. N. Ma (Nairobi:World Agroforestry (ICRAF)).
Mittal, A. (2009). The 2008 Food Price Crisis: Rethinking Food Security Policies. New York and Geneva: United Nations. Available online at: https://unctad.org/system/files/official-document/gdsmdpg2420093_en.pdf (accessed August 26, 2022).
Mohd Saad, N. S., Severn-Ellis, A. A., Pradhan, A., Edwards, D., and Batley, J. (2021). Genomics armed with diversity leads the way in Brassica improvement in a changing global environment. Front. Genet. 12, 110. doi: 10.3389/fgene.2021.600789
Mudau, F. N., Chimonyo, V. G. P., Modi, A. T., and Mabhaudhi, T. (2022). Neglected and underutilised crops: a systematic review of their potential as food and herbal medicinal crops in South Africa. Front. Pharmacol. 12, 4054. doi: 10.3389/fphar.2021.809866
Mulholland, D. A., Mwangi, E. M., Dlova, N. C., Plant, N., Crouch, N. R., and Coombes, P. H. (2013). Non-toxic melanin production inhibitors from Garcinia livingstonei (Clusiaceae). J. Ethnopharmacol. 149, 570–575. doi: 10.1016/j.jep.2013.07.023
Mustafa, M. A., Mabhaudhi, T., and Massawe, F. (2021). Building a resilient and sustainable food system in a changing world: a case for climate-smart and nutrient dense crops. Global Food Sec. 28, 100477. doi: 10.1016/j.gfs.2020.100477
Mustafa, M. A., Mayes, S., and Massawe, F. (2019). “Crop diversification through a wider use of underutilised crops: a strategy to ensure food and nutrition security in the face of climate change,” in Sustainable Solutions for Food Security: Combating Climate Change by Adaptation, eds A. Sarkar, S. R. Sensarma, and G. W. vanLoon (Cham: Springer International Publishing), 125–149. doi: 10.1007/978-3-319-77878-5_7
Naqvi, R. Z., Siddiqui, H. A., Mahmood, M. A., Najeebullah, S., Ehsan, A., Azhar, M., et al. (2022). Smart breeding approaches in post-genomics era for developing climate-resilient food crops. Front. Plant Sci. 13, 164. doi: 10.3389/fpls.2022.972164
Nkosi, N. N., Mostert, T. H. C., Dzikiti, S., and Ntuli, N. R. (2020). Prioritization of indigenous fruit tree species with domestication and commercialization potential in KwaZulu-Natal, South Africa. Genet. Resour. Crop Evol. 67, 1567–1575. doi: 10.1007/s10722-020-00932-5
OEC (2022). The Best Place to Explore Trade Data. Peru: Observatory of Economic Complexity. Available online at: https://oec.world (accessed August 25, 2022).
OECD (2022a). “Executive summary,” in Agricultural Policy Monitoring and Evaluation 2022: Reforming Agricultural Policies for Climate Change Mitigation (Paris: OECD Publishing), 22–26.
OECD (2022b). “General assessment of the macroeconomic situation,” in OECD Economic Outlook (Paris: OECD Publishing), 11–74.
OECD Policy Responses to Coronavirus (COVID-19) (2020). Food Supply Chains and COVID-19: Impacts and Policy Lessons. Paris: OECD. Available online at: https://www.oecd.org/coronavirus/policy-responses/food-supply-chains-and-covid-19-impacts-and-policy-lessons-71b57aea,/ (accessed September 26, 2022).
OECD/FAO (2019). “Cereal use in developed and developing countries,” in OECD-FAO Agricultural Outlook 2019–2028 (Paris: OECD Publishing), 125–140.
OECD/FAO (2022a). “Agricultural and food markets: Trends and prospects,” in OECD-FAO Agricultural Outlook 2022–2031 (Paris: OECD Publishing), 20–84.
OECD/FAO (2022b). “Regional briefs,” in OECD-FAO Agricultural Outlook 2022–2031 (Paris: OECD Publishing), 85–148.
Office of Assistant to Deputy Cabinet Secretary for State Documents Translation (2022). Gov't Bans Palm Oil Export Starting April 28. New Delhi: Cabinet Secretariat of the Republic of Indonesia. Available online at: https://setkab.go.id/en/govt-bans-palm-oil-export-starting-april-28/ (accessed August 29, 2022).
Owusu, V., Owusu-Sekyere, E., Donkor, E., Darkwaah, N. A., and Adomako-Boateng Jr, D. (2017). Consumer perceptions and willingness to pay for cassava-wheat composite bread in Ghana. J. Agribus. Dev. Emerg. Econ. 7, 115–134. doi: 10.1108/JADEE-11-2014-0044
Padulosi, S., Cawthorn, D.-M., Meldrum, G., Flore, R., Halloran, A., and Mattei, F. (2019). “Leveraging neglected and underutilized plant, fungi, and animal species for more nutrition sensitive and sustainable food systems,” in Encyclopedia of Food Security and Sustainability, eds P. Ferranti, E. M. Berry, and J. R. Anderson (Oxford: Elsevier), 361–370. doi: 10.1016/B978-0-08-100596-5.21552-7
Pagliarino, E., Orlando, F., Vaglia, V., Rolfo, S., and Bocchi, S. (2020). Participatory research for sustainable agriculture: the case of the Italian agroecological rice network. Eur. J. Fut. Res. 8, 7. doi: 10.1186/s40309-020-00166-9
Pauw, K., and Thurlow, J. (2022). “COVID-19 impacts on food systems, poverty, and diets: Lessons learned from country-level analyses,” in COVID-19 and Global Food Security: 2 Years Later, eds J. McDermott, and J. Swinnen (International Food Policy Research Institute (IFPRI)). doi: 10.2499/9780896294226_02
Pellegrini, P., and Fernández, R. J. (2018). Crop intensification, land use, and on-farm energy-use efficiency during the worldwide spread of the green revolution. Proc. Natl. Acad. Sci. 115, 2335–2340. doi: 10.1073/pnas.1717072115
Petereit, J., Bayer, P. E., Thomas, W. J. W., Tay Fernandez, C. G., Amas, J., Zhang, Y., et al. (2022). Pangenomics and crop genome adaptation in a changing climate. Plants 11, 1949. doi: 10.3390/plants11151949
Piggott, P. (2022). Australian and New Zealand Avocado Outlook 2022: Navigating Oversupply and Market Turmoil in the Avocado Industry. New Zealand: N. Z. Avocado. Available online at: https://industry.nzavocado.co.nz/download/rabobank-report-australian-new-zealand-avocado-outlook-2022/ (accessed September 1, 2022).
Pingali, P. (2015). Agricultural policy and nutrition outcomes: getting beyond the preoccupation with staple grains. Food Sec. 7, 583–591. doi: 10.1007/s12571-015-0461-x
Pretty, J., and Bharucha, Z. P. (2014). Sustainable intensification in agricultural systems. Ann. Bot. 114, 1571–1596. doi: 10.1093/aob/mcu205
Rapsomanikis, G. (2015). The Economic Lives of Smallholder Farmers. Rome: FAO. Available online at: https://www.fao.org/publications/card/en/c/b09e4a66-2ef0-47bc-b62f-07d182c59e3b/ (accessed October 6, 2022).
Rathore, S. S., Babu, S., El-Sappah, A. H., Shekhawat, K., Singh, V. K., Singh, R. K., et al. (2022). Integrated agroforestry systems improve soil carbon storage, water productivity, and economic returns in the marginal land of the semi-arid region. Saudi J. Biol. Sci. 29, 103427. doi: 10.1016/j.sjbs.2022.103427
Rattunde, F., Weltzien, E., Sidibé, M., Diallo, A., Diallo, B., vom Brocke, K., et al. (2021). Transforming a traditional commons-based seed system through collaborative networks of farmer seed-cooperatives and public breeding programs: the case of sorghum in Mali. Agricult. Hum. Values 38, 561–578. doi: 10.1007/s10460-020-10170-1
Rehan, S. F., Sumelius, J., and Bäckman, S. (2021). Diversification as a means to improve household food security in Bangladesh. J. Dev. Areas 55, 31–44. doi: 10.1353/jda.2021.0030
Renwick, L. L. R., Kimaro, A. A., Hafner, J. M., Rosenstock, T. S., and Gaudin, A. C. M. (2020). Maize-pigeonpea intercropping outperforms monocultures under drought. Front. Sustain. Food Syst. 4, 2663. doi: 10.3389/fsufs.2020.562663
Riar, A., and Bhullar, G. S. (2020). “Chapter I: Long-term experiments in agriculture: stages, challenges, and precautions,” in Long-Term Farming Systems Research, eds G. S. Bhullar, and A. Riar (New York, NY:Academic Press), 3–12. doi: 10.1016/B978-0-12-818186-7.00001-1
Ricciardi, V., Ramankutty, N., Mehrabi, Z., Jarvis, L., and Chookolingo, B. (2018). How much of the world's food do smallholders produce? Glob. Food Sec. 17, 64–72. doi: 10.1016/j.gfs.2018.05.002
Richard, B., Qi, A., and Fitt, B. D. L. (2022). Control of crop diseases through integrated crop management to deliver climate-smart farming systems for low- and high-input crop production. Plant Pathol. 71, 187–206. doi: 10.1111/ppa.13493
Rijzaani, H., Bayer, P. E., Rouard, M., DoleŽel, J., Batley, J., and Edwards, D. (2022). The pangenome of banana highlights differences between genera and genomes. Plant Genome 15, e20100. doi: 10.1002/tpg2.20100
Rosa, L., Rulli, M. C., Ali, S., Chiarelli, D. D., Dell'Angelo, J., Mueller, N. D., et al. (2021). Energy implications of the 21st century agrarian transition. Nat. Commun. 12, 2319. doi: 10.1038/s41467-021-22581-7
Ruperao, P., Thirunavukkarasu, N., Gandham, P., Selvanayagam, S., Govindaraj, M., Nebie, B., et al. (2021). Sorghum pan-genome explores the functional utility for genomic-assisted breeding to accelerate the genetic gain. Front. Plant Sci. 12, 963. doi: 10.3389/fpls.2021.666342
Rustia, D. J. A., Chiu, L.-Y., Lu, C.-Y., Wu, Y.-F., Chen, S.-K., Chung, J.-Y., et al. (2022). Towards intelligent and integrated pest management through an AIoT-based monitoring system. Pest Manag. Sci. 78, 4288–4302. doi: 10.1002/ps.7048
Sall, A. T., Bassi, F. M., Cisse, M., Gueye, H., Ndoye, I., Filali-Maltouf, A., et al. (2018). Durum wheat breeding: in the heat of the senegal river. Agriculture 8, 7. doi: 10.3390/agriculture8070099
Sansaloni, C., Franco, J., Santos, B., Percival-Alwyn, L., Singh, S., Petroli, C., et al. (2020). Diversity analysis of 80,000 wheat accessions reveals consequences and opportunities of selection footprints. Nat. Commun. 11, 4572. doi: 10.1038/s41467-020-18404-w
Sarker, U., Hossain, M. M., and Oba, S. (2020). Nutritional and antioxidant components and antioxidant capacity in green morph Amaranthus leafy vegetable. Sci. Rep. 10, 1336. doi: 10.1038/s41598-020-57687-3
Schreiber, K., Soubry, B., Dove-McFalls, C., and MacDonald, G. K. (2022). Untangling the role of social relationships for overcoming challenges in local food systems: a case study of farmers in Québec, Canada. Agricult. Hum. Values 22, 1–16. doi: 10.1007/s10460-022-10343-0
Senapati, N., Semenov, M. A., Halford, N. G., Hawkesford, M. J., Asseng, S., Cooper, M., et al. (2022). Global wheat production could benefit from closing the genetic yield gap. Nat. Food 3, 532–541. doi: 10.1038/s43016-022-00540-9
Sendhil, R., Kumari, B., Khandoker, S., Jalali, S., Acharya, K. K., Gopalareddy, K., et al. (2022). “Wheat in Asia: Trends, challenges and research priorities,” in New Horizons in Wheat and Barley Research: Global Trends, Breeding and Quality Enhancement, eds P. L. Kashyap, V. Gupta, O. Prakash Gupta, R. Sendhil, K. Gopalareddy, P. Jasrotia, and G. P. Singh (Singapore: Springer Singapore), 33–61. doi: 10.1007/978-981-16-4449-8_3
Shelef, O., Weisberg, P. J., and Provenza, F. D. (2017). The value of native plants and local production in an era of global agriculture. Front. Plant Sci. 8, 2069. doi: 10.3389/fpls.2017.02069
Shew, A. M., Tack, J. B., Nalley, L. L., and Chaminuka, P. (2020). Yield reduction under climate warming varies among wheat cultivars in South Africa. Nat. Commun. 11, 4408. doi: 10.1038/s41467-020-18317-8
Siddique, K. H. M., Li, X., and Gruber, K. (2021). Rediscovering Asia's forgotten crops to fight chronic and hidden hunger. Nat. Plants 7, 116–122. doi: 10.1038/s41477-021-00850-z
Sileshi, G. W., Akinnifesi, F. K., Ajayi, O. C., and Muys, B. (2011). Integration of legume trees in maize-based cropping systems improves rain use efficiency and yield stability under rain-fed agriculture. Agricult. Water Manag. 98, 1364–1372. doi: 10.1016/j.agwat.2011.04.002
Singapore's Food Supply (2022). Meeting Singapore's Food Supply. Singapore: Singapore Food Agency. Available online at: https://www.sfa.gov.sg/food-farming/singapore-food-supply/meeting-singapores-food-supply (accessed September 18, 2022).
Soffiantini, G. (2020). Food insecurity and political instability during the Arab Spring. Glob. Food Sec. 26, 100400. doi: 10.1016/j.gfs.2020.100400
Sogbohossou, E. O. D., Achigan-Dako, E. G., Maundu, P., Solberg, S., Deguenon, E. M. S., Mumm, R. H., et al. (2018). A roadmap for breeding orphan leafy vegetable species: a case study of Gynandropsis gynandra (Cleomaceae). Horticult. Res. 5, 2. doi: 10.1038/s41438-017-0001-2
Soria-Lopez, A., Garcia-Perez, P., Carpena, M., Garcia-Oliveira, P., Otero, P., Fraga-Corral, M., et al. (2022). Challenges for future food systems: From the Green Revolution to food supply chains with a special focus on sustainability. Food Front. 22, 173. doi: 10.1002/fft2.173
Southeast Asian Regional Center for Graduate Study Research in Agriculture (2017). Agricultural Transformation and Market Integration in the ASEAN Region: Responding to Food Security and Inclusiveness Concerns Summary Report on the National Inception Workshop in Cambodia. Los Baños: S.A.R.C.f.G.S.a.R.i.A. (SEARCA) and I.F.P.R.I. (IFPRI). Available online at: https://www.ifpri.org/publication/agricultural-transformation-and-market-integration-asean-region-responding-food-security (accessed October 13, 2022).
Sova, C., and Man, C. (2021). What Is Behind the Recent Rise in Global Food Prices? Washington, DC: Center for Strategic and International Studies. Available online at: https://www.csis.org/analysis/what-behind-recent-rise-global-food-prices (accessed August 29, 2022).
Struik, P. C., and Kuyper, T. W. (2017). Sustainable intensification in agriculture: the richer shade of green: a review. Agron. Sustain. Dev. 37, 39. doi: 10.1007/s13593-017-0445-7
Sulieman, A. M. E., and Mariod, A. A. (2019). “Domestication of indigenous fruit trees,” in Wild Fruits: Composition, Nutritional Value and Products, ed A.A. Mariod (Cham: Springer International Publishing), 59–81. doi: 10.1007/978-3-030-31885-7_6
Sultan, B., Defrance, D., and Iizumi, T. (2019). Evidence of crop production losses in West Africa due to historical global warming in two crop models. Sci. Rep. 9, 12834. doi: 10.1038/s41598-019-49167-0
Suweis, S., Carr, J. A., Maritan, A., Rinaldo, A., and D'Odorico, P. (2015). Resilience and reactivity of global food security. Proc. Natl. Acad. Sci. 112, 6902–6907. doi: 10.1073/pnas.1507366112
Talabi, A. O., Vikram, P., Thushar, S., Rahman, H., Ahmadzai, H., Nhamo, N., et al. (2022). Orphan crops: a best fit for dietary enrichment and diversification in highly deteriorated marginal environments. Front. Plant Sci. 13, 704. doi: 10.3389/fpls.2022.839704
Tamburini, G., Bommarco, R., Wanger, T. C., Kremen, C., van der Heijden, M. G. A., Liebman, M., et al. (2020). Agricultural diversification promotes multiple ecosystem services without compromising yield. Sci. Adv. 6, eaba1715. doi: 10.1126/sciadv.aba1715
Tan, X. L., Azam-Ali, S., Goh, E. V., Mustafa, M., Chai, H. H., Ho, W. K., et al. (2020). Bambara groundnut: an underutilized leguminous crop for global food security and nutrition. Front. Nutr. 7, 1496. doi: 10.3389/fnut.2020.601496
Tanda, A. S. (ed.)., (2022). “Advances in integrated pest management technology systems and going beyond convention,” in Advances in Integrated Pest Management Technology: Innovative and Applied Aspects (Cham: Springer International Publishing), 1–20. doi: 10.1007/978-3-030-94949-5_1
Tanzi, A. S., Eagleton, G. E., Ho, W. K., Wong, Q. N., Mayes, S., and Massawe, F. (2019). Winged bean (Psophocarpus tetragonolobus (L.) DC.) for food and nutritional security: synthesis of past research and future direction. Planta 250, 911–931. doi: 10.1007/s00425-019-03141-2
Tay Fernandez, C. G., Nestor, B. J., Danilevicz, M. F., Gill, M., Petereit, J., Bayer, P. E., et al. (2022). Pangenomes as a resource to accelerate breeding of under-utilised crop species. Int. J. Mol. Sci. 23, 2671. doi: 10.3390/ijms23052671
Tay Fernandez, C. G., Pati, K., Severn-Ellis, A. A., Batley, J., and Edwards, D. (2021). Studying the genetic diversity of yam bean using a new draft genome assembly. Agronomy 11, 953. doi: 10.3390/agronomy11050953
Teng, P. (2020). Assuring food security in Singapore, a small island state facing COVID-19. Food Sec. 12, 801–804. doi: 10.1007/s12571-020-01077-0
Terlau, W., Hirsch, D., and Blanke, M. (2019). Smallholder farmers as a backbone for the implementation of the sustainable development goals. Sustain. Dev. 27, 523–529. doi: 10.1002/sd.1907
Thorlakson, T., and Neufeldt, H. (2012). Reducing subsistence farmers' vulnerability to climate change: evaluating the potential contributions of agroforestry in western Kenya. Agricult. Food Sec. 1, 15. doi: 10.1186/2048-7010-1-15
Tirnaz, S., Zandberg, J., Thomas, W. J. W., Marsh, J., Edwards, D., and Jing, B. (2022). Application of crop wild relatives in modern breeding: an overview of resources, experimental and computational methodologies. Front. Plant Sci,. 13, 1008904. doi: 10.3389/fpls.2022.1008904
UN Comtrade Database Intelligence (2021). Top 10 Exported Products from Russia/Ukraine to the World in 2021. New York, NY: United Nations. Available online at: https://frsabido.shinyapps.io/UNCOMTRADE/ (accessed November 5, 2022).
UNCTAD (2021). Towards a New Trade Agenda for the Right to Food. New York, NY: U. Nations. Available online at: https://unctad.org/webflyer/towards-new-trade-agenda-right-food
UNCTAD (2022). Global Impact of War in Ukraine on Food, Energy and Finance Systems. Geneva: U. Nations. Available online at: https://unctad.org/webflyer/global-impact-war-ukraine-food-energy-and-finance-systems (accessed September 28, 2022).
United Nations (2004). Secretary-general calls for “Uniquely African Green Revolution” in 21st century, to end continent's plague of hunger, in Addis Ababa remarks. New York, NY: United Nations.
United Nations 2021 Food Systems Summit (2021). Science and Innovations for Food Systems Transformation and Summit Actions. New York, NY: U. Nations. Available online at: https://sc-fss2021.org/wp-content/uploads/2021/09/ScGroup_Reader_UNFSS2021.pdf (accessed September 17, 2022).
USDA FAS (2022). Brazil: Oilseeds and Products Update. New York, NY: USDA. Available online at: https://www.fas.usda.gov/data/brazil-oilseeds-and-products-update-30 (accessed November 13, 2022).
van Berkum, S. (2021). How trade can drive inclusive and sustainable food system outcomes in food deficit low-income countries. Food Sec. 13, 1541–1554. doi: 10.1007/s12571-021-01218-z
Varshney, R. K., Bohra, A., Roorkiwal, M., Barmukh, R., Cowling, W., Chitikineni, A., et al. (2021a). Rapid delivery systems for future food security. Nat. Biotechnol. 39, 1179–1181. doi: 10.1038/s41587-021-01079-z
Varshney, R. K., Bohra, A., Roorkiwal, M., Barmukh, R., Cowling, W. A., Chitikineni, A., et al. (2021b). Fast-forward breeding for a food-secure world. Trends Genet. 37, 1124–1136. doi: 10.1016/j.tig.2021.08.002
Varshney, R. K., Song, C., Saxena, R. K., Azam, S., Yu, S., Sharpe, A. G., et al. (2013). Draft genome sequence of chickpea (Cicer arietinum) provides a resource for trait improvement. Nat. Biotechnol. 31, 240–246. doi: 10.1038/nbt.2491
Vu, D. T., and Nguyen, T. A. (2017). The neglected and underutilized species in the Northern mountainous provinces of Vietnam. Genet. Resour. Crop Evol. 64, 1115–1124. doi: 10.1007/s10722-017-0517-1
Weersink, A., and von Massow, M. (2022). How the War in Ukraine Will Affect Food Prices. San Francisco: The Conversation. Available online at: https://theconversation.com/how-the-war-in-ukraine-will-affect-food-prices-178693 (accessed August 25, 2022).
Weinberg, J., and Bakker, R. (2015). Let them eat cake: Food prices, domestic policy and social unrest. Conflict Manag. Peace Sci. 32, 309–326. doi: 10.1177/0738894214532411
Werrell, C. E., Slaughter, A. M., Femia, F., and Climate, C. F. (2013). The Arab Spring and Climate Change: A Climate and Security Correlations Series. New York, NY: Center for American Progress.
WFP (2022). Horn of Africa 'Cannot Wait': WFP Scales Up Assistance as Historic Drought Raises Famine Threat. Rome: World Food Programme. Available online at: https://www.wfp.org/news/horn-africa-cannot-wait-wfp-scales-assistance-historic-drought-raises-famine-threat (accessed August 29, 2022).
WFP FAO (2022). Hunger Hotspots. FAO-WFP Early Warnings on Acute Food Insecurity: June to September 2022 Outlook. Rome: W.F. Programme and F.a.A.O.o.U. Nations. Available online at: https://www.fao.org/3/cc0364en/cc0364en.pdf (accessed September 28, 2022).
World Bank Group (2022). Commodity Markets Outlook: The Impact of the War in Ukraine on Commodity Markets, April 2022. Washington, DC: W. Bank. Available online at: https://openknowledge.worldbank.org/bitstream/handle/10986/37223/CMO-April-2022.pdf?sequence=3andisAllowed=y (accessed August 29, 2022).
Yan, Z., Zhou, J., Yang, L., Gunina, A., Yang, Y., Peixoto, L., et al. (2022). Diversified cropping systems benefit soil carbon and nitrogen stocks by increasing aggregate stability: results of three fractionation methods. Sci. Total Environ. 824, 153878. doi: 10.1016/j.scitotenv.2022.153878
Ye, C.-Y., and Fan, L. (2021). Orphan crops and their wild relatives in the genomic era. Mol. Plant 14, 27–39. doi: 10.1016/j.molp.2020.12.013
Zanini, S. F., Bayer, P. E., Wells, R., Snowdon, R. J., Batley, J., Varshney, R. K., et al. (2022). Pangenomics in crop improvement—from coding structural variations to finding regulatory variants with pangenome graphs. Plant Genome 15, e20177. doi: 10.1002/tpg2.20177
Keywords: Russia–Ukraine conflict, food security, agrifood systems, diversification, sustainable intensification
Citation: Neik TX, Siddique KHM, Mayes S, Edwards D, Batley J, Mabhaudhi T, Song BK and Massawe F (2023) Diversifying agrifood systems to ensure global food security following the Russia–Ukraine crisis. Front. Sustain. Food Syst. 7:1124640. doi: 10.3389/fsufs.2023.1124640
Received: 15 December 2022; Accepted: 22 February 2023;
Published: 15 March 2023.
Edited by:
Surendra K. Dara, Oregon State University, United StatesReviewed by:
Nick Andrews, Oregon State University, United StatesRoger R. B. Leakey, International Tree Foundation, United Kingdom
Copyright © 2023 Neik, Siddique, Mayes, Edwards, Batley, Mabhaudhi, Song and Massawe. This is an open-access article distributed under the terms of the Creative Commons Attribution License (CC BY). The use, distribution or reproduction in other forums is permitted, provided the original author(s) and the copyright owner(s) are credited and that the original publication in this journal is cited, in accordance with accepted academic practice. No use, distribution or reproduction is permitted which does not comply with these terms.
*Correspondence: Ting Xiang Neik, VGluYS5OZWlrQG5vdHRpbmdoYW0uZWR1Lm15; Beng Kah Song, c29uZy5iZW5nLmthaEBtb25hc2guZWR1