- 1Institute of Plant Protection, Shandong Academy of Agricultural Sciences, Jinan, China
- 2College of Plant Protection, Shandong Agricultural University, Tai'an, Shandong, China
The population of Osmia excavata, an important pollinator in commercial orchards, has been in serious decline over recent years. To evaluate the risk of high-temperature stress on O. excavata, we evaluated the high-temperature tolerance and potential physiological and biochemical responses of O. excavata after a series of high-temperature stresses. The results showed that the mortality rates of O. excavata increased gradually with increases in temperature and duration of stress (R2 = 0.88–0.99; p < 0.05). The larvae of O. excavata were more sensitive to temperature stress than adults, and the median lethal time (LT50) value of the former was smaller than the latter in the acute response test. By comparing the results of acute and chronic responses of O. excavata to high-temperature stress, we found that the LT50 values of natural cocoon-break adults at slightly higher temperatures (35°C and 40°C) were smaller than those of artificial cocoon-break adults, but the LT50 values were similar under extreme high-temperature stress (45°C). Furthermore, the acute and chronic responses on the fat content of adult Osmia obtained by artificial and natural cocoon-break methods were significantly different (F = 5.03; p < 0.05). Additionally, the mortalities of the young larvae and artificial cocoon-break adults were both significantly and positively correlated with trehalose content (r = 0.78–0.82, p < 0.05). However, the mortality of the natural cocoon-break adults was negatively related to the acetylcholinesterase activity (r = −0.93, p < 0.001). Overall, these results suggested that O. excavata has a low tolerance to high-temperature stress and provide evidence of causes that could be contributing to the population decline of O. excavata.
Introduction
Osmia excavata (Hymenoptera: Megachilidae) is a univoltine pollinator that spends its entire life inside a bee tube until it emerges the following spring (Men et al., 2018). This insect is used widely to pollinate apple, pear, peach, cherry, and other commonly planted fruit trees in China because of several advantages, including a better pollination efficiency than that of bees and artificial pollination, resistance to low temperature in winter, low take-off temperature (i.e., about 13°C), long daily pollination activity, fast frequency of visiting flowers, and simple feeding and management (Shu et al., 2002; He and Zhou, 2009; Sgolastra et al., 2015). Known as the king of pollinators (Li, 1992), O. excavata has been used for more than 30 years in China (Lu et al., 1992). However, the population of O. excavata has been in serious decline in recent years, which has impeded its function in ecological pollination (Cao et al., 2017; Liu et al., 2018). Many studies have found that the lethal factors in the decline of O. excavata have included residual pesticide in pollen, egg abortion, predation by parasitic mite wasps, and natural death (Yu, 1999; Zhai et al., 2016; Song et al., 2021), while Liu et al. (2018) believed that natural death was the main cause among the other factors. Cao et al. (2017) also reported that nearly 80% of O. excavata would die due to unsuitable growing conditions. However, relevant research is still very limited.
According to investigations, farmers in China usually hang the collected bee tubes with O. excavata in a ventilated outdoor environment, and occasionally put them under the eaves (Yu, 2014). However, this method of storing O. excavata can increase the risk of high temperatures in the following ways. First, the temperature outside is approximately 3.8°C higher than that in the ambient conditions (Zhou et al., 2018). Second, an outdoor storage environment is vulnerable to short periods of direct sunlight, which in turn creates a greenhouse effect inside the cocoon. Finally, global warming is already causing extreme high temperatures and heat waves in many parts of the world, and this phenomenon is posing a serious threat to biodiversity (García-Robledo et al., 2016; IPCC, 2021). It is reported that high-temperature events have increased by 40% over the past 60 years and the duration, frequency, and intensity of heat waves are predicted to increase with a 90%–99% probability (Ju et al., 2013).
Although insects are ectotherms, the growth and development of insects including O. excavata need to be carried out in an optimal temperature range (Zhang et al., 2020; Kuczyk et al., 2021). Otherwise, the fitness of the insect would be severely decreased. Chen et al. (2018) reported that the lethal temperatures for most insects are usually between 40°C and 50°C, depending on the species and life stage. Some insects are even at risk of extinction at current projected rates of global warming (García-Robledo et al., 2016; Abou-Shaara et al., 2017; Wang et al., 2017). For example, when the growth temperatures are higher than 36°C, a colony of honey bees is likely to be exposed to superheated temperatures, which would impact the adult brain (Abou-Shaara et al., 2017); short-term high-temperature stress can also decrease oviposition in Bactrocera cucurbitae and Carposina sasakii (Zeng et al., 2019; Zhang et al., 2020), and increase the instantaneous death risk of Ostrinia furnacalis (Zhou et al., 2018). However, few relevant studies on how O. excavata respond to high-temperature stress exist due to their long life history and the difficulty in observing their development status in the cocoon.
Thus, the objective of this research was to evaluate the high-temperature tolerance and potential physiological and biochemical responses of O. excavata after a series of high-temperature stresses. We speculated that (i) the higher is the stress temperature and longer the stress period, the greater the risk of O. excavata mortality; (ii) the acute and chronic responses of O. excavata to high-temperature stress may be different and; (iii) the physiological and biochemical substances of O. excavata and resisting high-temperature stress may be disturbed under high-temperature stress.
Materials and methods
Insects
The population of O. excavata was commercial, and acquired from Yantai Bifeng Agricultural Technology Co. Ltd., China. It has been continuously mass-reared for more than 10 generations in fruit orchards in Shandong Province, China. No pesticides were sprayed 20 days before flowering and throughout the flowering period.
High-temperature stress
The effects of high-temperature stress on O. excavata refer to previous research methods with minor modifications (Wang et al., 2017; Zhang et al., 2020). The tubes containing O. excavata were placed in growth climatic chambers (RXZ-600C, Ningbo Jiangnan, China) at different temperatures (30°C, 35°C, 40°C, and 45°C) during the young (2nd instar) larval, mature (5th instar) larval, and adult stages of O. excavata. After a certain period of temperature stress treatment, the larval and adult of O. excavata were taken out for life index detection.
Measurement of mortality
In the acute response test, the young and mature larvae and artificial cocoon-break adults of O. excavata were measured. Bee tubes were opened by hand and cocoons were artificially and carefully dissected with scissors after different periods of high-temperature stress and the mortality of O. excavata was observed according to the method of Song et al. (2021). There were three tubes per replicate (i.e., 18–24 larvae or cocoons per replicate) and three repeats (i.e., 54–72 larvae or cocoons per treatment) every sample date. The number of cocoons in each tube (6–8 cocoons per tube) was determined according to the egg-laying situation of maternal O. excavata. Mortality was observed at 0.25 h intervals, the longest observation period was 2.5 h for larvae and 24 h for adults.
To observe the long-term effects of high-temperature stress on the adults, the chronic response for natural cocoon-break adults was tested. The cocoons containing O. excavata that have been exposed to heat stress for a certain length of time were transferred to their optimal growing environment, that is a darkroom with 65%–75% relative humidity and 25°C ± 2°C temperature (Song et al., 2021). Then the mortality of O. excavata was observed after a natural cocoon break.
Physiological and biochemical indexes
The young and mature larvae, natural cocoon-break, and artificial cocoon-break adults of O. excavata were both used to measure the physiological and biochemical indexes after high-temperature stress exposure. There were three tubes per replicate and three repeats.
The trehalose content in each O. excavata was assayed using a trehalose quantification kit (Suzhou Keming Biotechnology Co., Ltd., China). In brief, each O. excavata was weighted using an electronic balance (AL104; Mettler-Toledo, China). The lapping liquid of O. excavata and extracting solution was left standing at room temperature for 45 min and oscillated 3–5 times. After cooling, the sample was centrifuged for 10 min at 8,000 rpm and 25°C to obtain the supernatant. The value was recorded spectrophotometrically at 620 nm.
Acetylcholinesterase (AchE) activity was measured using a kit provided by Suzhou Keming Biotechnology Co., Ltd. The O. excavata and extracting solution were ground to homogenate under ice bath conditions. The abrasive liquid was centrifuged for 10 min at 8,000 rpm and 4°C. Then, the activity of AchE was evaluated spectrophotometrically at 412 nm.
The fat and free water were also assayed. First, the fresh weight of O. excavata was determined using an electronic balance. Then the body was dried for 48 h at 60°C and the dry weight was determined. The free water was obtained by calculating the difference between the wet and dry weight of O. excavata. Then dried O. excavata was added to 2 ml of chloroform:methanol (2:1), and fully ground until homogenized. Afterward, the homogenate was centrifuged for 10 min at 2,600 rpm and the supernatant was discarded. Another 2 mL of the mixture was added to the residue, the centrifugation was repeated once, and the supernatant was discarded. The remaining residue was dried for 72 h at 60°C to constant weight. Finally, the fat was calculated according to a method described previously (Colinet et al., 2007).
Data analysis
Before statistical analysis, data were transformed to log10, arcsine, or square-root when necessary to evaluate data normality and homogeneity. The median lethal time (LT50) and analysis of variance were determined using SPSS v.21.0 (SPSS Inc., Chicago, IL; Abbott, 1925). One-way analysis of variance (ANOVA) was used to estimate the impact of different duration of high-temperature stress on the mortality and the physiological and biochemical indexes of O. excavata. Two-way ANOVAs were performed to determine the impact of insect stages (or response mode) and high-temperature stress on the physiological and biochemical indexes of O. excavata. Means were compared by using Tukey’s LSD test at p < 0.05. Pearson’s correlation was used to analyze the relationships between the mortality and the physiological and biochemical indexes of O. excavata at different insect stages under high-temperature stress.
Results
Acute responses of Osmia excavata to high-temperature stress
The mortality of O. excavata significantly increased with an increase in the duration of high-temperature stress (p < 0.05; Figure 1) and increased with an increase in temperature (R2 = 0.92–0.99; Table 1).
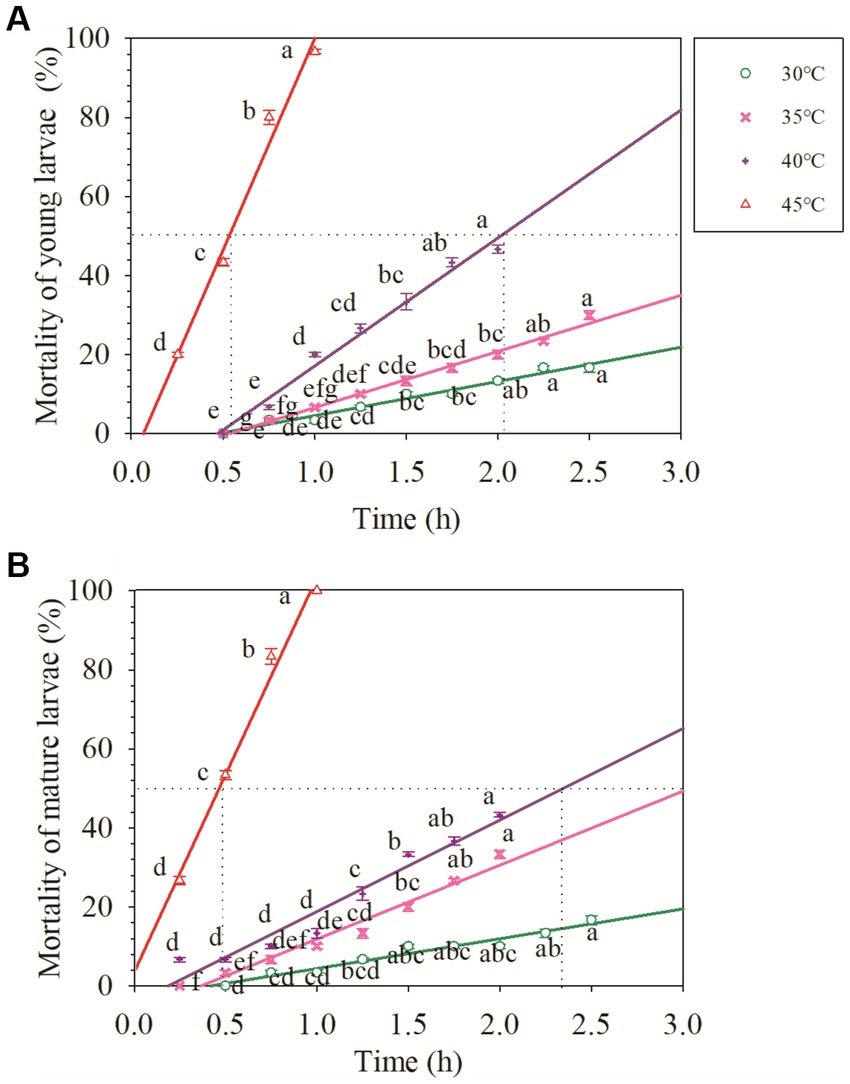
Figure 1. Acute response of the young and mature larvae of Osmia excavata to different durations of high-temperature stress (different lowercase letters indicate significant differences between different stress times at the same temperature according to Tukey’s LSD test at p < 0.05).
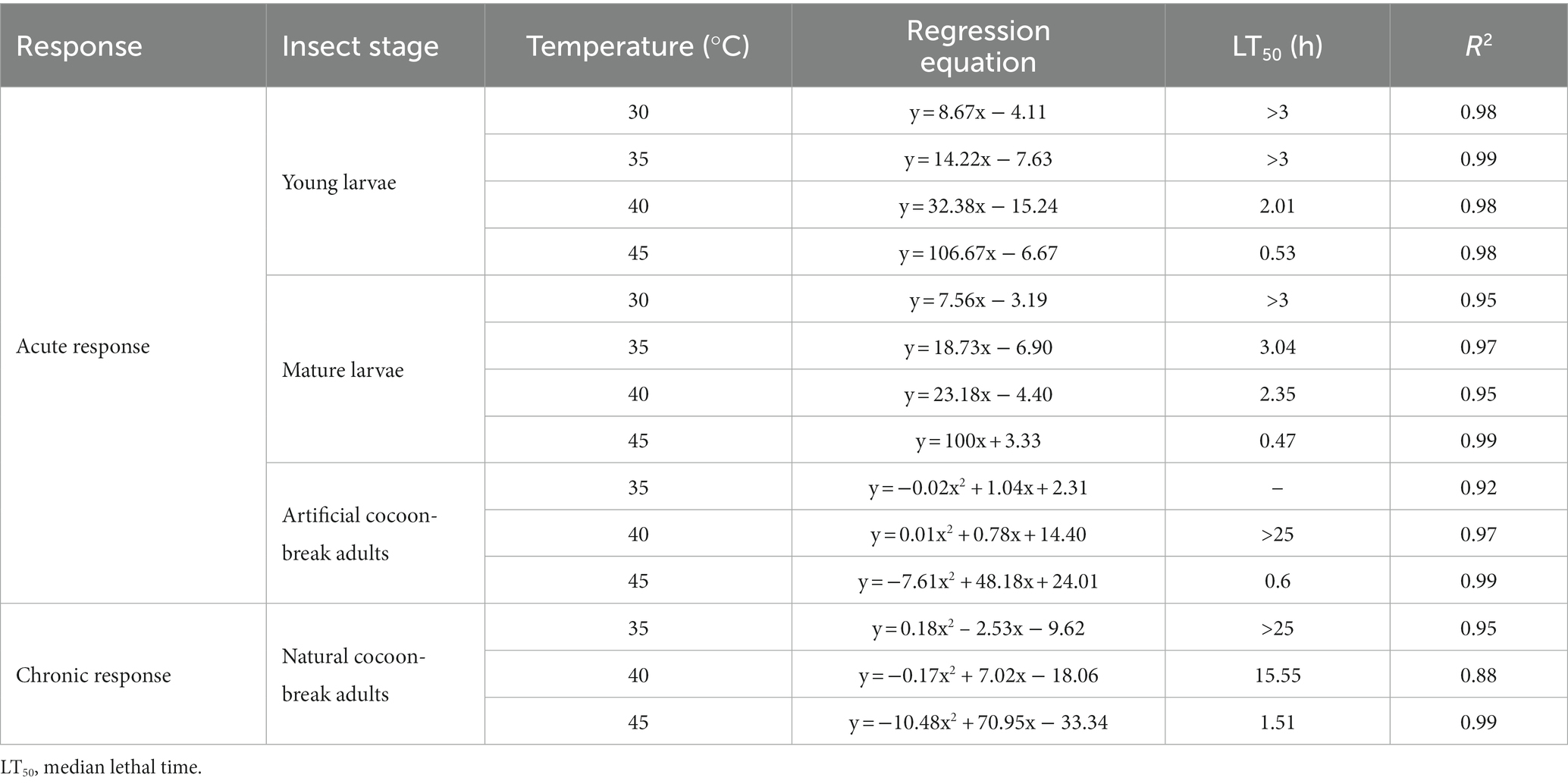
Table 1. Effects of high-temperature stress on the time of death in Osmia excavata at different stages of development, including young larvae, mature larvae, artificial cocoon-break adults, and natural cocoon-break adults.
For the young larvae of O. excavata, the mortality at 30°C for 2.5 h was about 15%, and that at 35°C for 2.5 h was about 30%, but those were significantly higher than those at 30°C and 35°C for less than 1.75 h (+66.7% and +79.96%), respectively (p < 0.05). The mortality of young larvae at 40°C for 1.75–2.0 h was more than 40%, and both were markedly higher than those at 40°C for less than 1.25 h (p < 0.05). The mortality of the young larvae at 45°C for 1.0 h reached 96.67%, and significantly higher than that at 45°C for 0.75 h (+20.84%; p < 0.05). The young larvae did not die when the temperature was lower than 40°C for 0.5 h, but the mortality reached 20% after 0.25 h at 45°C (Figure 1). Additionally, the LT50 of the young larvae decreased with an increase in temperature (Table 1). The LT50 of the young larvae at 30°C and 35°C were over 3 h, and at 40°C and 45°C, they were 2.01 and 0.53 h, respectively (R2 = 0.98–0.99; Table 1; Figure 1).
For the mature larvae of O. excavata, the mortality at 30°C for 2.25 h was about 13% and significantly higher than that at 30°C for less than 1.25 h (+100%; p < 0.05). The mortalities of the mature larvae at 35°C and 40°C for 2.0 h were markedly higher than those for 1.5 h, respectively (+66.65% and + 30%; p < 0.05). The mortality of the mature larvae at 45°C for 1.0 h reached 100%, and significantly higher than that at 45°C for 0.75 h (+20%; p < 0.05; Figure 1). The LT50 of the mature larvae at 40°C and 45°C were 2.35 h and 0.47 h, respectively (R2 = 0.95–0.99; Table 1; Figure 1).
For the artificial cocoon-break adults, the mortality increased slowly with time at 35°C and 40°C, but rapidly at 45°C (Figure 2). The mortality of the artificial cocoon-break adults at 45°C for 0.5 h was nearly 50% and markedly increased to 90% after 2 h (+107.14%; p < 0.05). The LT50 of the artificial cocoon-break adults at 40°C and 45°C were more than 25 and 0.6 h, respectively (R2 = 0.92–0.99; Table 1; Figure 2).
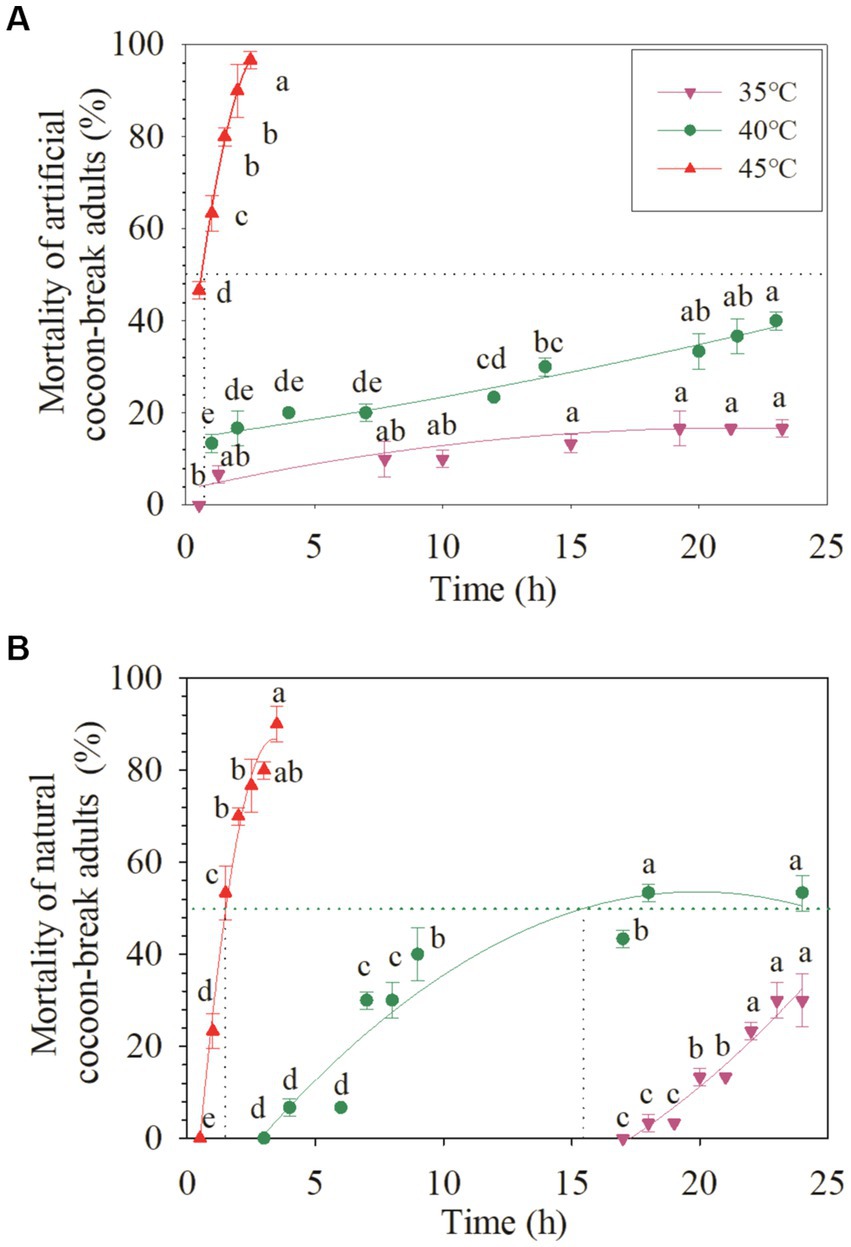
Figure 2. Acute response of the artificial cocoon-break adults and chronic response of the cocoon-break adults of Osmia excavata to different durations of high-temperature stress (different lowercase letters indicate significant differences between different stress times at the same temperature according to Tukey’s LSD test at p < 0.05).
Chronic responses of Osmia excavata to high-temperature stress
In the natural cocoon-break adults of O. excavata, no death occurred under 35°C stress after 17 h, but the mortality increased significantly to 30% after 24 h (p < 0.05; Figure 2). The mortality of natural cocoon-break adults increased faster with time at 40°C than that at 35°C, but slower than that at 45°C (p < 0.05). The mortality of natural cocoon-break adults at 45°C for 1 h was nearly 23.33% and markedly increased to 90% after 3.5 h at 45°C (+285.77%; p < 0.05). The LT50 of natural cocoon-break adults at 40°C and 45°C were 15.55 and 1.51 h, respectively (R2 = 0.88–0.99; Table 1; Figure 2).
Physiological and biochemical indexes of Osmia excavata under high-temperature stress
Although the acute response of the physiological and biochemical indexes of O. excavata were significantly different between young larvae, mature larvae, and artificial cocoon-break adult stages (F = 5.52–653.19; p < 0.01), the high-temperature stress had no significant effect on the acute response of the physiological and biochemical indexes (F = 0.36–0.88; p > 0.05; Table 2). There were significant interactions of the fat content between temperature stress and insect stage (F = 3.38; p < 0.01). Additionally, the trehalose content of the mature larvae at 35°C was significantly increased compared with that at 30°C (+126.20%; p < 0.05; Figure 3). The fat content of the artificial cocoon-break adults at 45°C was markedly higher than that at 35°C (+32.12%; p < 0.05). The free water content was significantly decreased compared with that at 35°C (−9.21%; p < 0.05; Figure 3).

Table 2. Effects of high-temperature stress on the acute response of the physiological and biochemical indexes of Osmia excavata at different stages of development, including young larvae, mature larvae, and artificial cocoon-break adults (F value).
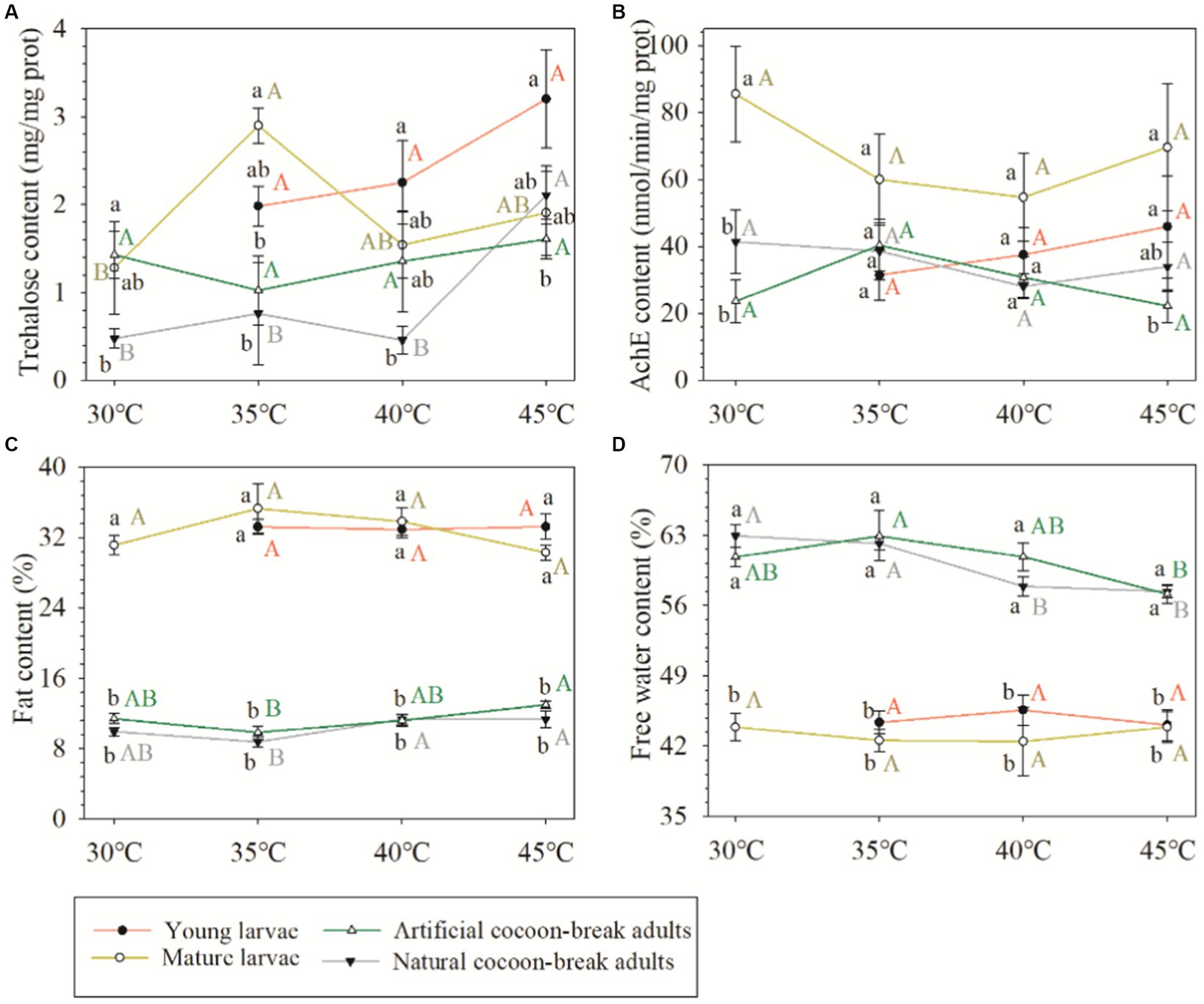
Figure 3. Effects of high-temperature stress on the physiological and biochemical indexes of Osmia excavata at different stages of development, including young larvae, mature larvae, artificial cocoon-break adults, and natural cocoon-break adults (AchE, acetylcholinesterase; different lowercase letters indicate significant differences between different insect stages at the same temperature stresses according to Tukey’s LSD test at p < 0.05; different capital letters indicate significant differences between different temperature stresses in the same insect stage at p < 0.05).
There was no significant difference between the acute and chronic responses on the physiological and biochemical indexes of adult Osmia obtained by either artificial or natural cocoon-break methods (F = 1.42–2.76; p > 0.05), except the fat contents (F = 5.03; p < 0.05; Table 3). The high-temperature stress had a significant effect on the contents of trehalose and fat (F = 4.38–6.86; p < 0.05). The trehalose content of adult Osmia obtained by the natural cocoon-break method at 45°C was significantly higher than that at 30°C (+359.31%; p < 0.05), 35°C (+177.17%; p < 0.05), and 40°C (+342.15%; p < 0.05). The fat content of adults by the natural cocoon-break method at 45°C was markedly higher than that at 35°C (+29.59%; p < 0.05; Figure 3).

Table 3. Physiological and biochemical indexes of the acute and chronic responses of adult Osmia obtained by artificial and natural cocoon-break methods to high-temperature stress (F value).
Correlation analysis between the mortality and physiological and biochemical indexes of Osmia excavata
The mortality of the young larvae was significantly and positively correlated with the trehalose content (r = 0.78, p < 0.05) and AchE activity (r = 0.67, p < 0.05; Figure 4). The mortality of the artificial cocoon-break adults of O. excavata was also significantly and positively related to the trehalose content (r = 0.82, p < 0.01). However, the mortality of the natural cocoon-break adults was negatively related to the AchE activity (r = −0.93, p < 0.001). The mortality of the mature larvae was not significantly correlated with physiological and biochemical indexes [r = (−0.10) − 0.11, p > 0.05; Figure 4].
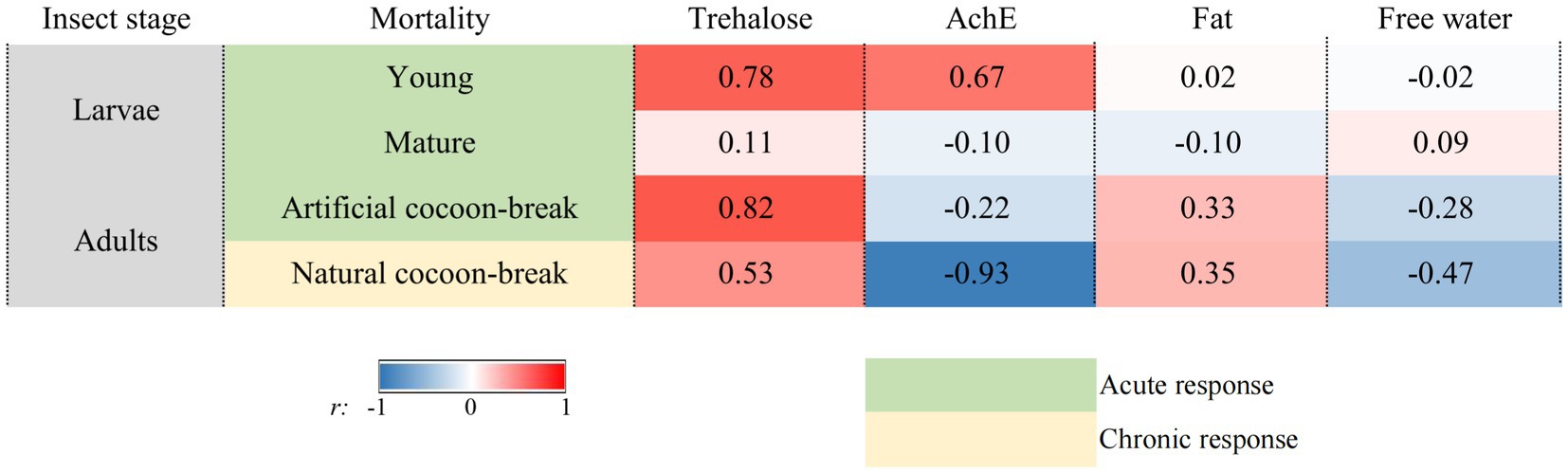
Figure 4. Pearson’s correlation between the mortality and the physiological and biochemical indexes of the young larvae, mature larvae, artificial cocoon-break adults, and natural cocoon-break adults of Osmia excavata under high-temperature stress (r value). The darker the blue or red, the stronger the negative or positive correlation, respectively.
Discussion
High-temperature stress poses a serious risk to Osmia excavata
Temperature is one of the most important external conditions affecting the life activities of insects (Dongmo et al., 2021; Gaytán et al., 2022), but an abnormal high-temperature environment has a serious impact on growth and development, and even causes the risk of extinction in some insects (García-Robledo et al., 2016; Abou-Shaara et al., 2017; Wang et al., 2017). Populations of O. excavata are more easily affected by adverse factors because they only have one generation a year (Men et al., 2018). Our results demonstrated that the higher the temperature, the faster the death rate of O. excavata, which was consistent with our hypothesis (i). Similar results have been obtained in Carposina nipponensis (Zhang et al., 2020), Ophraella communa (Chen et al., 2018), and Corythucha ciliata (Ju et al., 2013). In addition, this study found that under a slightly higher temperature (35°C and 40°C), the LT50 value of adults was higher than that of larvae. Thus, we speculated that the harmful effects of high temperatures on O. excavata depend not only on the intensity and duration of stress but also on the developmental stage (Enriquez and Colinet, 2017). As the adults of O. excavata will naturally emerge from the cocoon with the rising temperature in the spring (Men et al., 2018), this may be the reason for a higher temperature tolerance in adults than in larvae. By comparing the results of acute and chronic responses of O. excavata to high-temperature stress, it was found that the LT50 values of natural cocoon-break adults at 35°C and 40°C were smaller than those of artificial cocoon-break adults, but similar LT50 values were observed at 45°C. This may occur for three reasons: (a) the physiological and biochemical substances that maintain the normal growth and development of O. excavata were destroyed after slightly higher temperature stress, even if they did not die immediately, but it was not enough to support their survival for long; (b) the functions related to cocoon breaking were severely damaged and failed to successfully break the cocoon; (c) extreme high-temperature stress (45°C) may cause O. excavata to die instantly. Considering the high mortality we found in larvae and adult Osmia, apiarists should store bee tubes in a cool ventilated place out of direct sunlight to maintain an adequate population of O. excavata.
Metabolites in larvae of Osmia excavata are more susceptible to high temperature stress than those in adults
Trehalose is an important blood sugar in insects, not only can it be stored as an energy source and carbohydrate reserve but also as a compatible solute adapted to various stresses, such as heat, cold, osmotic stress, and drought (Xin et al., 2013; Qin et al., 2015). There was no significant difference in the trehalose content in larvae and artificial cocoon-break adults at 30°C, but the trehalose content in the former increased faster than the latter with an increase in temperature, especially when the temperature reached 40°C and 45°C, the content and growth rate of trehalose in the young larvae were both higher than that of the mature larvae. These results showed that the smaller the insect stage under high-temperature stress, the more drastic the change of trehalose content in O. excavata. This may be due to the poor ability of the younger larvae to resist an adverse environment. Jiang et al. (2016) also believed that the body surface of younger larvae was soft and its cuticle was thin, but the body surface of adult insects was relatively hard and the cuticle layer was thicker, which can reduce stress and retain normal life activities of adults.
Fat plays an important role in the energy storage and metabolism of insects (Wu et al., 2019). At the same time, insects cannot live without the participation of free water, which is a good solvent in the cell, can participate in the cellular biochemical reaction, and transport nutrients and waste produced by metabolism. Adults of O. excavata were found to have less fat and more free water than larvae. The temperature in the larval stage of O. excavata is relatively low under natural conditions, so the larvae would resist the cold climate by storing more fat and reducing free water content; as temperatures rise in the adult stage, the O. excavata would break diapause, cocoon breaking, flight, etc., therefore, they need to use more energy and free water to speed up metabolism (Sgolastra et al., 2015; Men et al., 2018). It was also found that the contents of fat and free water in larvae were not significantly affected after extreme heat stress, whereas the contents in adults were more affected, indicating that the fat and free water contents in larvae of O. excavata were less sensitive to high-temperature stress than that in adults. This may be because the larvae of O. excavata have fewer activities and slower metabolism than adults in the cocoon, but further research is needed.
Special metabolism in adult Osmia excavata after high-temperature stress
In the chronic response test, the trehalose content of adult Osmia was significantly increased after extreme high-temperature stress compared to slightly higher temperature stress. We speculated that there may be two reasons: (a) trehalase was inactivated in insects under extreme high-temperature stress, so trehalose could not be hydrolyzed, and more trehalose content was accumulated (Qin et al., 2015); (b) a special protective membrane is formed on the cell surface by increasing trehalose content under harsh conditions, such as extreme high temperature, to prevent the structure of biomolecules from being disrupted (Ma et al., 2018). These results were similar to previous studies on Monolepta hieroglyphica and Gomphocerus sibiricus and suggested that this was a manifestation of insect adaptation to high-temperature stress (Li et al., 2014; Ma et al., 2018). However, in the acute response test, the trehalose contents of larvae or artificial cocoon-break adults were not markedly changed after being subjected to high temperatures. We believed that although the trehalose content in O. excavata increased with an increase in temperature stress at each insect stage, it did not increase to a significant level in a short time.
The fat contents in natural and artificial cocoon-break adults of O. excavata after extreme high-temperature stress were both significantly higher than those after slightly high-temperature stress, but the change in the trend of free water was opposite to that of the fat content. Zhao et al. (2010) found that low-temperature stress can also lead to an increase in insect fat content. We believe that the reasons for the increase in fat content are different between high and low-temperature stress: the former may be due to the serious loss of free water in the adult body of O. excavata under extreme temperature stress, which hinders normal metabolism and inhibits the decomposition of fat, even causing adults to die faster; the latter may be because insects would reduce their supercooling point in a low-temperature environment by increasing their fat content, which can increase their cold tolerance and resist cold damage (Zhao et al., 2010). Interestingly, Liu et al. (2010) reported that heat-shock stress decreased lipid storage in planarian Dugesia japonica to suppress its development. We also found that the fat content of adults decreased at 35°C compared with 30°C, but did not reach a significant level. This may be because the life activities of O. excavata have not been completely disordered after they endured tolerable temperature stress, and the metabolic ability was enhanced to maintain normal life activities by consuming more energy substances such as fat.
Conclusion
To date, the population of O. excavata has been in serious decline and there is a lack of observation on the sensitivity of O. excavata to high-temperature stress. The current study found that the mortality of O. excavata significantly increased with the increased temperature and duration of high-temperature. Additionally, the larvae of O. excavata were more sensitive to the same temperature stress than adults, and the LT50 value of the former was smaller. Furthermore, there was a significant difference between the acute and chronic responses on the fat content of adult Osmia by both the artificial and natural cocoon-break methods. And the mortalities of the young larvae and artificial cocoon-break adults were both significantly and positively correlated with the trehalose content. Given the potential impacts of high-temperature stress on the cocoon break, flight ability, and fertility of O. excavata, the adverse impact of temperature on the population of O. excavata needs further study.
Data availability statement
The original contributions presented in the study are included in the article/supplementary material, further inquiries can be directed to the corresponding author.
Author contributions
YS, YY, BY, and LLiu participated in the experimental investigation and analyzed the data. HC, WG, SL, and LLi contributed reagents/materials. YS wrote the first draft of the article. XM revised the article. All authors contributed to the article and approved the submitted version.
Funding
This study was supported by the Bee Industry Innovation Team of Shandong Province, National Natural Science Foundation of China (32202316), and Agricultural Science and Technology Innovation Project of Shandong Academy of Agricultural Sciences (CXGC2022E04).
Conflict of interest
The authors declare that the research was conducted in the absence of any commercial or financial relationships that could be construed as a potential conflict of interest.
Publisher’s note
All claims expressed in this article are solely those of the authors and do not necessarily represent those of their affiliated organizations, or those of the publisher, the editors and the reviewers. Any product that may be evaluated in this article, or claim that may be made by its manufacturer, is not guaranteed or endorsed by the publisher.
References
Abbott, W. S. (1925). A method of computing the effectiveness of an insecticide. J. Econ. Entomol. 18, 265–267. doi: 10.1093/jee/18.2.265a
Abou-Shaara, H. F., Owayss, A. A., Ibrahim, Y. Y., and Basuny, N. K. (2017). A review of impacts of temperature and relative humidity on various activities of honey bees. Insect. Soc. 64, 455–463. doi: 10.1007/s00040-017-0573-8
Cao, Y. B., Zhou, X. H., Ye, B. H., Li, L. L., Lu, Z. B., Xu, H., et al. (2017). Factors limiting Osmia excavata Alfken populations in apple orchards of Shandong Province. Chin. J. Appl. Entomol. 54, 652–659. doi: 10.7679/j.issn.2095-1353.2017.080
Chen, H., Zheng, X., Luo, M., Solangi, G. S., Wan, F., and Zhou, Z. (2018). Effect of short-term high-temperature exposure on the life history parameters of Ophraella communa. Sci. Rep. 8:13969. doi: 10.1038/s41598-018-32262-z
Colinet, H., Vernon, P., and Hance, T. (2007). Does thermal-related plasticity in size and fat reserves influence supercooling abilities and cold-tolerance in Aphidius colemani (Hymenoptera: Aphidiinae) mummies? J. Therm. Biol. 32, 374–382. doi: 10.1016/j.jtherbio.2007.03.005
Dongmo, M. A. K., Fiaboe, K. K. M., Kekeunou, S., Nanga, S. N., Kuate, A. F., Tonnang, H. E. Z., et al. (2021). Temperature-based phenology model to predict the development, survival, and reproduction of the oriental fruit fly Bactrocera dorsalis. J. Therm. Biol. 97:102877. doi: 10.1016/j.jtherbio.2021.102877
Enriquez, T., and Colinet, H. (2017). Basal tolerance to heat and cold exposure of the spotted wing drosophila. Drosophila suzukii. PeerJ. 5:e3112. doi: 10.7717/peerj.3112
García-Robledo, C., Kuprewicz, E. K., Staines, C. L., Erwin, T. L., and Kress, W. J. (2016). Limited tolerance by insects to high temperatures across tropical elevational gradients and the implications of global warming for extinction. Proc. Natl. Acad. Sci. U. S. A. 113, 680–685. doi: 10.1073/pnas.1507681113
Gaytán, L., Gotthard, K., and Tack, A. (2022). Strong impact of temperature and resource specialisation on patterns of voltinism within an oak-associated insect community. Ecol. Entomol. 47, 544–552. doi: 10.1111/een.13139
He, W. Z., and Zhou, W. R. (2009). Study on the effect of Osmia excavata Alfken, Italy bee and artificial pollination on apple pollination. Apicult China. 60, 9–11.
IPCC (2021). Climate change widespread, rapid, and intensifying: IPCC. Cambridge: Cambridge University Press.
Jiang, S., Li, S., Zhang, B., Li, H., Wan, F., and Zheng, C. (2016). Effects of extreme high temperature on survival rate, reproduction, trehalose and sorbitol of Frankliniella Occidentalis. Sci. Agr. Sinica. 49, 2310–2321. doi: 10.3864/j.issn.0578-1752.2016.12.007
Ju, R. T., Gao, L., Zhou, X. H., and Li, B. (2013). Tolerance to high temperature extremes in an invasive lace bug, Corythucha ciliata (Hemiptera: Tingidae), in subtropical China. PLoS One 8:e54372. doi: 10.1371/journal.pone.0054372
Kuczyk, J., Raharivololoniaina, A., and Fischer, K. (2021). High temperature and soil moisture reduce host-plant quality for an insect herbivore. Ecol. Entomol. 46, 889–897. doi: 10.1111/een.13025
Li, J., Li, S., Wang, D., and Ji, R. (2014). Changes in the contents of stress resistant substances in Gomphocerus sibiricus (Orthoptera: Acrididae) under high temperature stress. Acta Entomol. Sinica. 57, 1155–1161. doi: 10.16380/j.kcxb.2014.10.002
Liu, L., Li, L. L., Li, C., Yuan, R., Yu, Y., Men, X. Y., et al. (2018). Population investigation and restriction factors analyses of Osmia excavata Alfken in Jiaodong. Apicult China. 69, 68–71.
Liu, P., Lin, X., and Wang, D. (2010). Heat-shock stress suppresses the development possibly by decreasing lipid and glycogen storage in planarian Dugesia japonica. J. Se U. 29, 36–41. doi: 10.3969/j.issn.1671-6264.2010.01.006
Lu, J. M., Lu, L. N., and Liu, Z. T. (1992). Apple using the local wild bees—pollination experiment of Osmia excavata. J. Deciduous Fruit Trees 1, 14–15.
Ma, X., Zhang, Z., Wang, Z., Zhang, Y., and Chen, J. (2018). Effects of brief exposure to high temperature on free amino acids, total sugars and trehalose of female adult Monolepta hieroglyphica (Motschulsky). Plant Prot. 44, 111–115. doi: 10.16688/j.zwbh.2017120
Men, X. Y., Li, L. L., Lu, Z. B., Ouyang, F., Liu, L., Xu, H., et al. (2018). Biological characteristics and pollination service of Mason bee. Chin. J. Appl. Entomol. 55, 973–983. doi: 10.7679/j.issn.2095-1353.2018.118
Qin, J., Luo, S., He, S., and Wu, J. (2015). Characteristics and functions of trehalose and trehalase in insects. Chin. J. Environ. Entomol. 37, 163–169. doi: 10.3969/j.issn.1674-0858.2015.01.24
Sgolastra, F., Tosi, S., Medrzycki, P., Porrini, C., and Burgio, G. (2015). Toxicity of spirotetramat on solitary bee larvae, Osmia cornuta (Hymenoptera: megachilidae), in laboratory conditions. J Apic. Sci. 59, 73–83. doi: 10.1515/jas-2015-0024
Shu, G. W., Ren, W., Smirle, M. J., and Huan, L. X. (2002). Release of Osmia excavata and Osmia jacoti (Hymenoptera: megachilidae) for apple pollination. Can. Entomol. 134, 369–380. doi: 10.4039/Ent134369-3
Song, Y. Y., Li, L. L., Li, C., Lu, Z., Ouyang, F., Liu, L., et al. (2021). Comparative ecotoxicity of insecticides with different modes of action to Osmia excavata (Hymenoptera: Megachilidae). Ecotox. Environ. Safe. 212:112015. doi: 10.1016/j.ecoenv.2022.114296
Wang, L. H., Shan, D., Zhang, Y. L., Liu, X. D., Sun, Y., Zhang, Z. C., et al. (2017). Effects of high temperature on life history traits and heat shock protein expression in chlorpyrifos-resistant Laodelphax striatella. Pestic. Biochem. Phys. 136, 64–69. doi: 10.1016/j.pestbp.2016.08.002
Wu, Y., Zhou, W., Zhao, S., Jin, D., and Guo, J. (2019). Changes in the fat, carbohydrate and protein content of Aspongopus chinensis during diapause. Chin. J. Appl. Entomol. 56, 1019–1025. doi: 10.7679/j.issn.2095-1353.2019.112
Xin, L., Han, S., Li, L., Simon, G., and Patrick, D. C. (2013). The potential of trehalose to replace insect hemolymph in artificial media for Trichogramma dendrolimi (Hymenoptera: Trichogrammatidae). Insect Sci. 20, 629–636. doi: 10.1111/j.1744-7917.2012.01566.x
Yu, Z. B. (1999). The natural enemy of Osmia excavata: Oligotrichos trichoides. North. Fruit Trees. 5:39.
Yu, X. X. (2014). Some problems in the collection and storage of Osmia excavata. Yantai Fruits. 2, 53–54.
Zeng, B., Zhu, W., Fu, Y., and Zhou, S. (2019). Response mechanism of oviposition and relevant protein expression of Bactrocera cucurbitae (Coquillet) to short-term high-temperature conditions. Neotrop. Entomol. 48, 197–206. doi: 10.1007/s13744-018-0638-z
Zhai, H., Wang, G., Li, X., Xue, X., and Wang, J. (2016). Preliminary report on mite damage to Osmia bee comb tube nest in apple orchard of Weihai. Shandong Agr. Sci. 48, 106–108. doi: 10.14083/j.issn.1001-4942.2016.04.026
Zhang, H. J., Sun, L. N., Yan, W. T., Yue, Q., and Qiu, G. S. (2020). Effects of short-term high temperature stress on the survival and reproduction of adult Peachophagus peachophagus. Fruit Trees South China 49, 133–139. doi: 10.13938/j.issn.1007-1431.20200131
Zhao, J., Cui, N., Zhang, F., Yin, X., and Xu, Y. (2010). Effects of body size and fat content on cold tolerance in adults of Harmonia axyridis (Pallas) (Coleoptera: Coccinellidae). J. Insects. 28, 189–193. doi: 10.1016/S1002-0721(10)60377-8
Keywords: Osmia excavata, high-temperature stress, mortality rate, median lethal time, physiological and biochemical index, ecological risk
Citation: Song Y, Liu L, Cui H, Guo W, Lv S, Ye B, Li L, Yu Y and Men X (2023) Evaluation of Osmia excavata (Hymenoptera: Megachilidae) sensitivity to high-temperature stress. Front. Sustain. Food Syst. 7:1124310. doi: 10.3389/fsufs.2023.1124310
Edited by:
Sadam Hussain, Northwest A&F University, ChinaReviewed by:
Zhaozhi Lu, Qingdao Agricultural University, ChinaMuhammad Hafeez, Zhejiang University, China
Yongsheng Zhang, Hunan Agricultural University, China
Copyright © 2023 Song, Liu, Cui, Guo, Lv, Ye, Li, Yu and Men. This is an open-access article distributed under the terms of the Creative Commons Attribution License (CC BY). The use, distribution or reproduction in other forums is permitted, provided the original author(s) and the copyright owner(s) are credited and that the original publication in this journal is cited, in accordance with accepted academic practice. No use, distribution or reproduction is permitted which does not comply with these terms.
*Correspondence: Xingyuan Men, menxy2000@hotmail.com