- 1State Key Laboratory of Urban and Regional Ecology, Research Center for Eco-Environmental Sciences, Chinese Academy of Sciences, Beijing, China
- 2College of Resource and Environment, University of Chinese Academy of Sciences, Beijing, China
- 3Key Lab of Urban Environment and Health, Institute of Urban Environment, Chinese Academy of Sciences, Xiamen, China
- 4Xiamen Key Lab of Urban Metabolism, Institute of Urban Environment, Chinese Academy of Sciences, Xiamen, China
Landscape structures affect pests, depending on compositional heterogeneity (the number and proportions of different habitats), configurational heterogeneity (spatial arrangement of habitats), and spatial scales. However, there is limited information on the relative effects of compositional and configurational heterogeneity on invasive pests and their associates (species that can benefit from invasive pests), and how they vary across spatial scales. In this study, we assayed the invasive pest Bactrocera dorsalis (Hendel) and its associated fly Drosophila melanogaster in 15 landscapes centered on mango orchards. We calculated landscape composition (forest percentage, mango percentage, and Shannon's diversity) and configuration (edge density) using two methods: spatial distance scales and combined scales. Spatial distance scales included buffer rings with radii of 0.5, 1.0, and 1.5 km, and combined scales referred to cutting or not cutting a smaller ring from larger ones. Our results shown that compositional heterogeneity positively affected B. dorsalis and D. melanogaster due to forest cover percentage, whereas configurational heterogeneity with high edge density negative effect on B. dorsalis. Forest cover had less of an effect on B. dorsalis than configurational heterogeneity, but the opposite effect was observed for D. melanogaster. Importantly, the direction and strength of forest cover and configurational heterogeneity to species did not vary with spatial distance scales or spatial combined scales. Thus, compositional and configurational heterogeneity exhibit differential effects on this invasive pest and its associator, and revealed that the relative effects of landscape structures are consistent across multiple scales. These results provide new insights into landscape effects on interconnected species using a diverse spatial-scale approach.
1. Introduction
Land use change and intensification have led to habitat destruction and fragmented landscapes, potentially exacerbating biodiversity loss and pest outbreaks (Sirami et al., 2019; Tougeron et al., 2022). Landscape heterogeneity, constituted by the types of habitats surrounding agricultural lands and their spatial arrangement, has been recognized as important for pest control (Clemente-Orta et al., 2020; Paredes et al., 2021). However, previous studies have focused on the impact of landscape structures on native pests while paying little attention to invasive pests and their effects on interconnected insects (e.g., commensalism) (Schmidt et al., 2019; Shi et al., 2021). Understanding of how landscape heterogeneity regulates pests and their associates (species with interactions with pests) remains a core topic in revealing landscape effects (Gagic et al., 2021; Shi et al., 2021) and an urgent need for developing conservation strategies (Rios et al., 2021; Ratto et al., 2022).
Landscape effects on pests depend on compositional heterogeneity and configurational heterogeneity (Fahrig et al., 2011; Kheirodin et al., 2020; Ouyang et al., 2020; Zhang et al., 2021), but there is no consensus on their relative contributions for pest control (Rybicki et al., 2020; Metzger et al., 2021; Saura, 2021), perhaps the lack of consensus is due to the functional trait and different needs of the species studied (Aristizábal and Metzger, 2018; Martin et al., 2019). The habitat amount hypothesis states that the habitat amount in the landscape surrounding a sample site determines species abundance (Fahrig, 2013; Rios et al., 2021; Malagnini et al., 2022). Several studies support this hypothesis; for example, coffee coverage in agricultural landscapes is positively correlated with coffee berry borer (Aristizábal and Metzger, 2018), as more resources are available to the pest. In addition, the island biogeography theory argues that landscape configurations with high habitat fragmentation are critical to species (Rybicki et al., 2020; Saura, 2021). Generally, habitat fragmentation is detrimental to individual species abundance (Heidrich et al., 2020; Rybicki et al., 2020), as it forms small isolated islands that support smaller communities, increasing the probability of inbreeding and extinction (MacDonald et al., 2018). The negative correlation between habitat fragmentation (e.g., edge density) and pests observed in previous studies supports this theory (Bosem Baillod et al., 2017); however, contradictory results have shown that habitat fragmentation is positively related to species abundance (Fahrig, 2017; Fletcher et al., 2018), which is attributable to, but not limited to, habitat type diversity and spatial scale of effect (Aristizábal and Metzger, 2018; Fletcher et al., 2018; MacDonald et al., 2018).
Differences in spatial scale may significantly affect the impact of habitat cover and fragmentation on pests (Aristizábal and Metzger, 2018; Da Silva Carneiro et al., 2022). Landscape structures may even have opposite effects on pests at different spatial distance scales (the radius of the distance from the sampling site forms different spatial buffer rings, Figure 1B), as the dispersal ability of pests is limited due to their functional traits. For example, the correlation between forest cover and coffee berry borers changes from positive to negative as the spatial distance changes from 300 m to 2 km (Aristizábal and Metzger, 2018). In addition to the spatial distance scale, the spatial combined scale formed by the small distance buffer rings (e.g., 0.5 km radius) nested within the large distance buffer rings (e.g., 1.5 km radius, Figure 1B) may affect the species distribution (Da Silva Carneiro et al., 2022). Delineating the scope of the landscape by spatial distance is insufficient to elucidate the landscape effects, because the surrounding landscape effects is not clarified by removing small nested circles. Moreover, the expansion of spatial distance potentially increases new habitat types and spatial arrangements (Da Silva Carneiro et al., 2022), and it is difficult to elucidate the landscape effects of these new elements without decoupling spatial scales (removing the small buffer ring from the large one). Unfortunately, almost all previous studies have focused on the spatial distance scale while ignoring the spatial decoupled of effect (Aristizábal and Metzger, 2018; Kheirodin et al., 2020), which limits the understanding of how landscape affects pests.
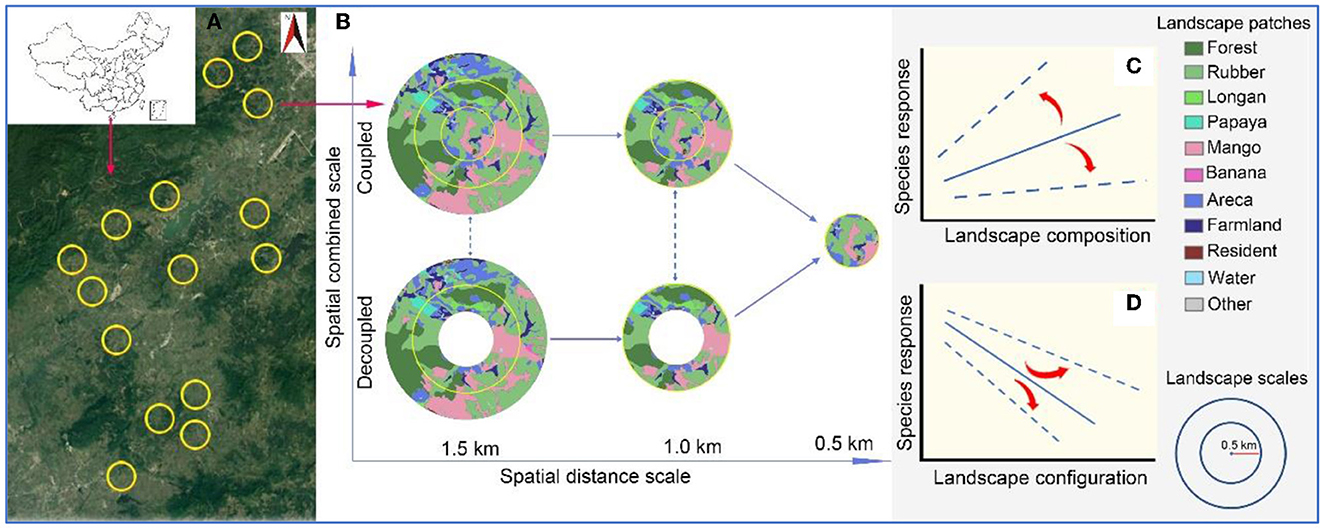
Figure 1. Schematic diagram of the study area and landscape settings. The yellow circles (1.5 km radius) in the left panel (A) indicate the spatial distribution of the 15 landscapes. The right panel (B) shows the processing of the landscape at spatial distance and combined scale. Distance scales refer to buffer rings with 0.5, 1, and 1.5 km radius around the central mango patch, respectively [x-coordinate, (B)], and the combined scale refers to whether to remove the ring with 0.5 km [y-coordinate, (B)]. (C, D) Indicate the potential impact of spatial scale on landscape heterogeneity-species relationships.
In this study, we explored the relative effects of landscape composition and configuration on pests at multiple spatial scales. We targeted a pest fruit fly, Bactrocera dorsalis (Hendel) (Diptera: Tephritidae), in mango (Mangifera indica Linn) orchards. Mangoes are a popular fruit worldwide, and B. dorsalis is a serious threat to mango yield and quality (Grechi et al., 2022). In African countries invaded by B. dorsalis, the proportion of damaged mango fruits that have been recorded can be as high as 78%, causing serious economic losses to local small farmers (Cugala et al., 2020). As an invasive pest in China and other regions (Liu et al., 2019; Grechi et al., 2022), B. dorsalis females pierce the skin of fruit during oviposition. Larvae that develop inside the fruit tend to feed on the most nutritious part of the pulp, which leads to fruit damage. Larvae drop from the host and burrow into soil to form pupae. Adults feed mostly on nectar, but some also draw nutrients from pollen and rotting fruit (Liu et al., 2019). Owing to its strong ability to survive and reproduce, B. dorsalis inevitably affects native species. As another fruit fly, Drosophila melanogaster (Diptera: Drosophilidae) can benefit from B. dorsalis because D. melanogaster can obtain food and reproduce from mango fruits infested by B. dorsalis. Therefore, D. melanogaster is defined as an associator of B. dorsalis.
We selected 15 landscapes with mango orchards at their center on Hainan Island (Figure 1), the main mango fruit-producing area in China, which has been invaded by B. dorsalis (Liu et al., 2019). We measured B. dorsalis and D. melanogaster abundances in each mango orchard and its surrounding habitats. The compositional and configurational heterogeneity in each landscape were characterized on a spatial distance scale and spatial combined (coupled and decoupled) scales. We hypothesized that the impact of landscape heterogeneity on an invasive pest and its associator varies with spatial scale and examined the following questions: (1) How do compositional and configurational heterogeneity differ in their impact on the invasive pest and its associator? (2) Do landscape variables in relation to the invasive pest and its associator vary with spatial distance scales, and (3) spatial combined scales?
2. Materials and methods
2.1. Study site and landscape settings
This study was conducted in the southwestern region of Hainan Island, China (18°45′ N, 109°17′ E). The area has a tropical marine monsoon climate. The average annual temperature is 19–26°C. The mean annual precipitation is 1,400–1,800 mm. Land-use patches are dominated by smallholders, forming a highly heterogeneous mosaic landscape mainly comprised of forest, rubber (Hevea brasiliensis), mango, longan (Dimocarpus longan), papaya (Chaenomeles sinensis), areca (Areca catechu L.), and farmland (rice and vegetables) patches. Mango orchards, which are managed similarly and are the main income source for many smallholders in the region, have been affected by the invasive pest B. dorsalis for many years. B. dorsalis is thought to have invaded Hainan Island as early as 1934 (Liu et al., 2019). Smallholders would use insecticides to exterminate B. dorsalis 1–2 times a year during the near-ripening period of mangoes.
Taking the mango patch as the center, we set 15 landscapes as a gradient based on forest cover, with a radius of 1.5 km surrounding the mangoes (Figure 1A). This landscape radius was chosen based on previous studies that observed traces of multiple pest activity (Gardiner et al., 2009; González et al., 2020). Among the selected landscapes, forest cover percentage varied from 0 to 30%, with varying degrees of landscape fragmentation.
2.2. Compositional and configurational heterogeneity
First, we obtained a high-resolution geographic image map for each landscape based on their coordinates using ArcGIS 10.2 software. We divided the landscape patches into 11 categories [forest, mango, rubber, longan, areca, banana, papaya, farmland, water area, residential land, and others (Figure 1B)] and then used ArcGIS 10.2 software to outline each patch. The patches were identified by combining high-resolution satellite images (0.83 m) and data from field surveys conducted in June–August 2021, after which the vector graphics were converted into raster maps to calculate compositional and configurational heterogeneity metrics. We used FRAGSTATS 4.2 software (McGarigal et al., 2012) to calculate the landscape indices.
Since longan, papaya, farmland and banana have a small percentage of area in the landscape, while forest, mango, rubber and areca are the main land types that make up the composition of the landscape, the compositional heterogeneity indicators included mango cover percentage (%), forest%, rubber%, areca%, and Shannon's diversity index. Configurational heterogeneity was indicated by edge density, which can reflect the degree of landscape fragmentation (Martin et al., 2019).
2.3. Landscape spatial scales
Two spatial scales were set: spatial distance scale and spatial combined scale. The spatial distance scale refers to the circle of buffer rings centered on the mango patch with radii of 0.5, 1, and 1.5 km, respectively (Figure 1B), which can clarify the effect of landscape variables with spatial distance.
The spatial combined scales were defined based on whether the buffer ring with a radius of 0.5 km was cut off from the larger 1 and 1.5 km rings. The nested landscape formed without being cut off was called the coupled (Figure 1B), and the central blank landscape formed by cutting off was called a decoupled. Thus, the spatial combined scale contains two categories of coupled and decoupled (Figure 1B). Combined scales were used to explore the effects of surrounding habitat composition and configuration at different distances.
2.4. Species sampling
In September 2021, the newly grown leaves of mango trees in the current year were in the mature stage. The invasive pest (B. dorsalis) and its associator (D. melanogaster) were identified in mango patches at the center of each landscape and other surrounding patches (such as forest and rubber) using yellow sticky traps (length × width: 20 × 25 cm) and yellow funnel traps (attractant: methyl eugenol). Three yellow sticky traps and three funnel traps were set at a height of ~1–2 m above the ground and were displayed at least 3 m apart within each patch. All traps were placed at least 6 m from the edge of each patch to reduce edge effects. Except for 15 mango patches at the center of the landscape, other surrounding patches were randomly selected in each landscape to determine whether the land cover types were habitats for B. dorsalis and D. melanogaster. In total, 75 patches were investigated in 15 landscapes, including 4 forest, 15 mango, 15 areca, 13 rubber, 9 farmland, 9 longan, 7 banana, and 3 papaya patches; thus, we placed 225 yellow sticky traps, and funnel traps, respectively. After 3 days, we collected all yellow sticky and funnel traps and counted the number of each species.
During the flowering (October 2021), young fruit (November 2021), and near-ripe stages (March 2022) of mangoes, we measured the invasive pest and its associator in central mango patches using two yellow traps methods by the same sampling method as in September 2021. Therefore, the numbers of each species in mango patches were determined four times. The species abundance of each patch measured each time was obtained by summing the two methods, following previous study (Perrot et al., 2022).
2.5. Statistical analysis
At the patch level, we confirmed the presence of both species in the selected habitat types, and then analyzed the differences in B. dorsalis and D. melanogaster abundances among habitats using ANOVA. Multiple comparisons were then made using the Tamhane's T2 due to the heterogeneity of variance in the species abundance data. We explored the correlation between B. dorsalis and D. melanogaster abundance using linear regression analysis across all patches. Species abundance was log-transformed before doing the regression analysis, as were the following regressions and correlation analysis.
At the landscape level, we first removed the covariance between landscape variables in two steps. (a) Pearson's correlation analysis was performed on the landscape variables at each spatial scale (Supplementary Figure 1), and only those variables with weak correlations (r < 0.65) were retained (Da Silva Carneiro et al., 2022); (b) The retained landscape variables were subjected to multiple regression analysis to log-transformed species abundance, and the landscape variables were further filtered by VIF<2 (Perrot et al., 2022). The landscape variables that were thus screened were edge density, forest cover percentage (%), mango%, and Shannon's diversity (Supplementary Table 1). The general linear model was then used to analyze the relationship between each landscape variable and species abundance, with landscape variables as fixed effects, species abundance as response variables, and sampling site and month as covariates in the spatial distance and combined scales.
To explore the effects of spatial distance and combined scales on the landscape variable-species abundance relationship, we explored differences in linearly fitted relationships between landscape variables (Supplementary Table 1) and species abundance using grouped regression. The spatial distance scale varies from 0.5 to 1 km and then to 1.5 km. Spatial combined scales for coupled and decoupled were compared at distance scales of 1 and 1.5 km, respectively. Differences in linearly fitted relationships were checked using the Chow test (Zeileis et al., 2002; Wen et al., 2022).
To reveal the optimal landscape variables and spatial scales, we subjected all landscape variables (Supplementary Table 1) and species (B. dorsalis and D. melanogaster) abundance to multiple linear regression analysis in a stepwise approach, with landscape variables as independent variables and species abundance as dependent variables. Standardized regression coefficients were used to compare the relative effects of landscape variables on species. All of the above data analyses were conducted using IBM SPSS software (version 21.0).
3. Results
A total of 40 594 Bactrocera dorsalis and 10 438 Drosophila melanogaster specimens were collected during the sampling period. Both species were captured in the selected habitat types (Figure 2A). B. dorsalis abundance in forests was significantly higher than that in mango orchards (P < 0.05, Figure 2A), but there was no significant difference between mangoes and other habitats (P < 0.05, Figure 2A). There were no significant differences in D. melanogaster abundance among the habitats (P > 0.05, Figure 2B). B. dorsalis and D. melanogaster abundances showed a significant positive correlation across the landscape patches (P < 0.05, Figure 2C).
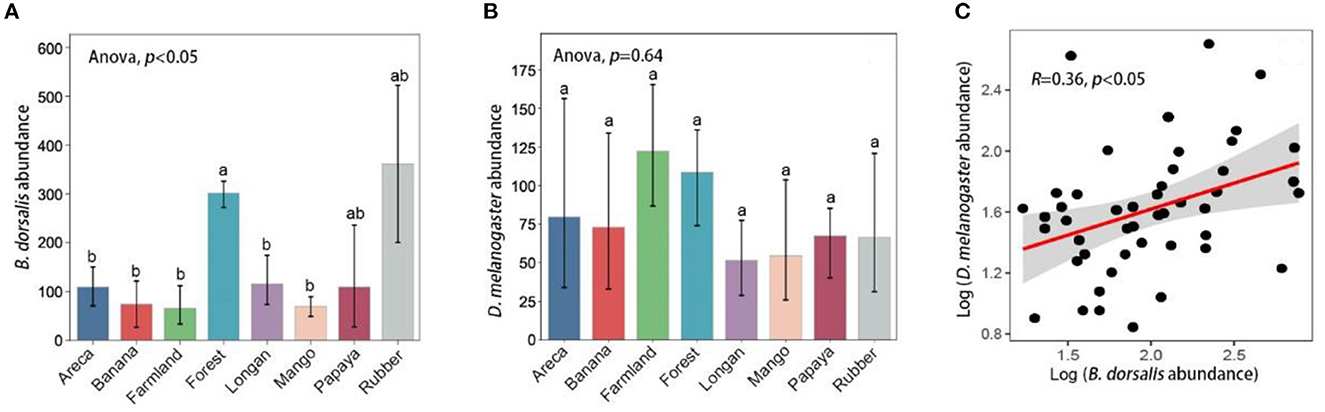
Figure 2. Species abundance differences among habitats. (A) Indicates Bactrocera dorsalis abundance, (B) indicates Drosophila melanogaster abundance, and different lowercase letters on the histogram indicate significant differences. (C) Indicates the correlation between B. dorsalis and D. melanogaster abundance by correlation analysis.
3.1. Impact of landscape heterogeneity on pests
Configurational heterogeneity (edge density) was negatively correlated with B. dorsalis abundance (P < 0.05, Figure 3A, Supplementary Figure 2A), but the correlation with D. melanogaster was insignificant (P > 0.05, Figure 3D, Supplementary Figure 2D). In contrast, compositional heterogeneity was positively correlated with B. dorsalis and D. melanogaster abundance owing to an increase in the percentage of forest cover (P < 0.05, Figures 3B, E, Supplementary Figures 2B, E). The percentage of mango cover was not significantly associated with either species at 1 and 1.5 km scales (P > 0.05, Figures 3C, F).
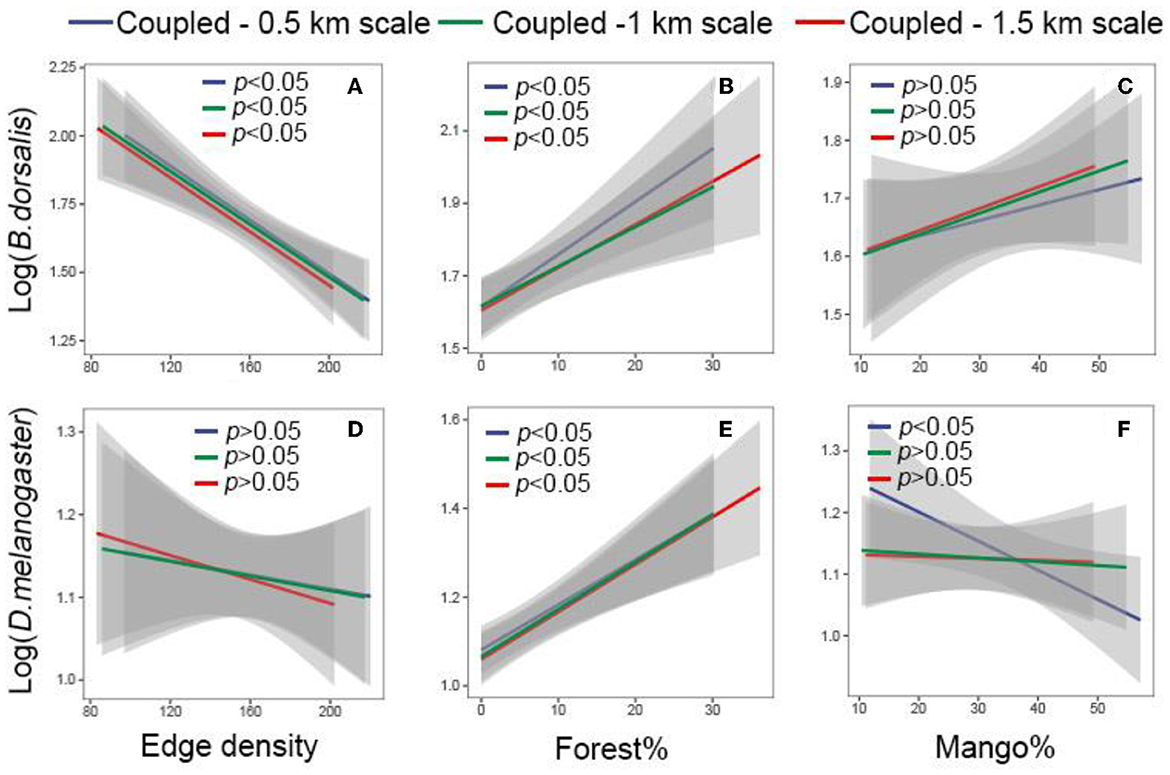
Figure 3. Linear relationships between landscape heterogeneity and species at spatial distance scales (0.5, 1, and 1.5 km). (A–C) Indicate log-transformed Bactrocera dorsalis abundance; (D–F) indicated log-transformed Drosophila melanogaster abundance. The blue, green, and red lines represent the spatial distance scales of 0.5, 1, and 1.5 km, respectively. The coupled results are shown, and the decoupled results can be known from Supplementary Figure 2.
3.2. Spatial distance scale effects of landscape heterogeneity on pests
Negative relationships between edge density with B. dorsalis abundance was observed across spatial distance scales of 0.5, 1, and 1.5 km (Figure 3A, Figure 2A). In addition, there were no significant changes in the slopes of these fitted lines (Figure 3A), implying that variation in the strength of these indicator effects is limited. Positive correlation between forest cover percentage with B. dorsalis and D. melanogaster abundances did not change with spatial distance scale from 0.5, 1 to 1.5 km (Figures 3B, E). The slope of the fitted lines between forest cover and species abundance did not change significantly at the scales of 0.5,1, and 1.5 km (Figures 3B, E), indicating that the effect of forest cover had limited variation in intensity. Overall, spatial distance scales did not alter the direction and intensity of compositional and configurational heterogeneity affecting the species (Figure 3).
3.3. Spatial combined scale effects of landscape heterogeneity on pests
At spatial coupled and decoupled, the direction of linear relationships between edge density with B. dorsalis abundance was consistent, regardless of 1 or 1.5 km space distances (Figure 3). In addition, there was no difference in the strength of the linear relationship, as the differences in the regression coefficients of the fitted straight lines were insignificant (P > 0.05, Table 1, Supplementary Table 2). Similarly, the direction and strength of the correlation between forest percentage with B. dorsalis and D. melanogaster abundance did not change from spatial coupled (Figures 3B, E) to decoupled, regardless of 1 km (Table 1) or 1.5 km space distances (Supplementary Table 2). Therefore, spatially combined scales had limited effects on compositional and configurational heterogeneity effects.
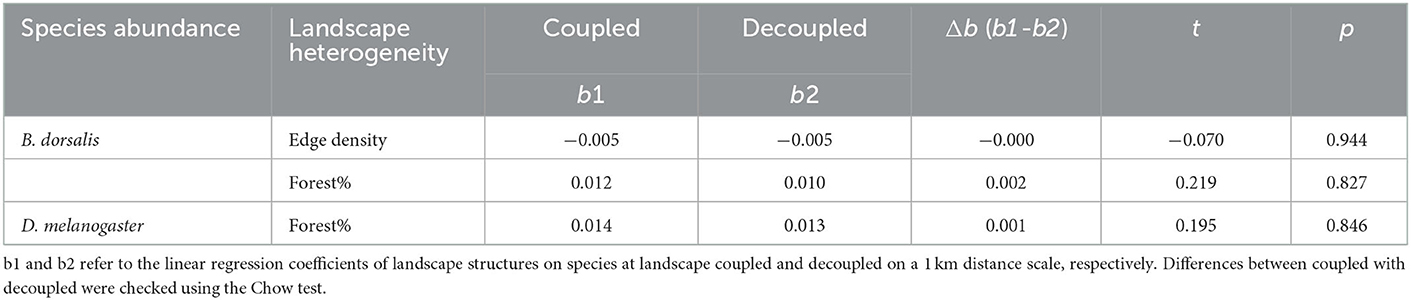
Table 1. Grouped regression results show differences in the fitting linear relationship between landscape heterogeneity with pests from spatial coupled to decoupled.
Considering all landscape variables in spatial distance and combined scales, edge density at coupled 1 km scale and forest cover at coupled 0.5 km scale were important factors for B. dorsalis, and the importance of the former (absolute value of the standardized coefficient) was higher than the latter (Table 2). For D. melanogaster, the proportion of forest cover at coupled 1 km scale was more important than edge density at coupled 1 km scale, although the latter had a significant effect (Table 2).
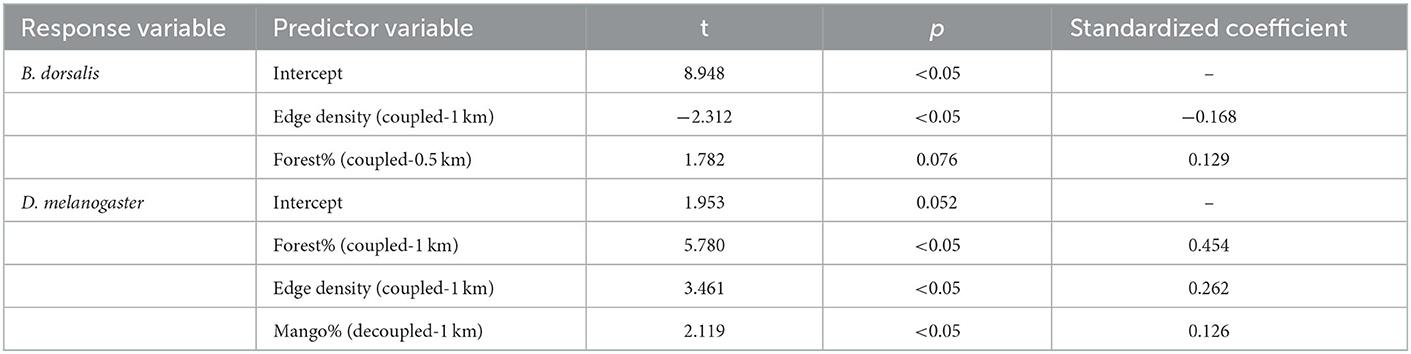
Table 2. Multiple linear regression screening of landscape heterogeneity variables explaining species variation integrating spatial coupled and decoupled.
4. Discussion
This study aimed to explore at multiple spatial scales how landscape heterogeneity affects an invasive pest (B. dorsalis) and an associated fly insect (D. melanogaster, hereafter “associator”) that could benefit from the invasive pest. The results showed that the effects of landscape heterogeneity on the invasive pest were opposite in compositional and configurational heterogeneity, with the former having positive and the latter having negative effects. Compared with compositional heterogeneity, configurational heterogeneity had higher and lower relative contributions to the invasive pest and associator, respectively, but did not vary with diverse spatial scales. Thus, landscape effects are species-dependent, but not spatial scale-dependent.
4.1. Effects of compositional and configurational heterogeneity
Compositional heterogeneity was positively correlated with the invasive pest and associator dependent on forest cover rather than other habitats, which differs from the results of previous studies showing that forest cover is often thought to reduce pest abundance (Medeiros et al., 2019; González et al., 2020). For example, forest cover is negatively associated with a coffee leaf pest (Leucoptera coffeella) (Medeiros et al., 2019) and fruit pest (coffee berry borer) (Aristizábal and Metzger, 2018). This conflicting result may be attributed to the differences in the ability of forests to control native and invasive pests. For native pests, various factors exist in the forests that limit their populations, such as competitors and natural predators (Henri et al., 2015; Aristizábal and Metzger, 2018). For invasive pests, however, there is not only a lack of competitors and natural enemies, but also sufficient alternative food sources, overwintering sites, and refuges provided by forests with high plant diversity (Tscharntke et al., 2016; Gurr et al., 2017; Tamburini et al., 2020). This is potentially conducive to the survival and reproduction of invasive pests, thereby increasing the number of invasive pests in agricultural land adjacent to forests. Similarly, in addition to obtaining resources from surrounding forests, the associator can better maintain populations by following the invasive pest, as shown by the significant positive correlation between the abundance of the invasive pest and the associator.
In contrast, configurational heterogeneity was negatively correlated with invasive pest abundance. Both negative and positive effects of landscape configuration on insects have been reported in previous studies (Fahrig, 2017; Martin et al., 2019). For pests, configurational heterogeneity with high edge density may reduce crop cover available as a food resource, echoing the resource concentration hypothesis (Tscharntke et al., 2016), while high edge density resulting from fragmentation promoted pest transfer from crop patches to surrounding habitat due to dispersal effects (Martin et al., 2019; Haan et al., 2020). Fragmentation increases new habitats that are more suitable for pests to survive, as in the present study, and rubber plantations are more suitable for B. dorsalis than mango orchards, accelerating the outward spread of B. dorsalis, thereby making configurational heterogeneity negatively related to this invasive pest. However, the associator was insignificantly associated with configurational heterogeneity, which may be attributed to the insignificant differences in associator abundance across habitat types (Figure 2B), that is, the number per unit area remained unchanged regardless of configurational heterogeneity. This indicates that species respond differently to landscape changes (Martin et al., 2019), even though the two species are closely related.
Notably, compositional heterogeneity contributed less to invasive pest abundance than configurational heterogeneity; however, the opposite was true for the associator. Although landscape composition with high forest cover promoted the invasive pest, invasive pest overflow from forests would be buffered by configurational heterogeneity with high edge density through dilution effects (Haan et al., 2020), similar to that observed in other insects (Martin et al., 2019; Souza et al., 2020). Other habitats (e.g., areca and banana) did not differ significantly from mango orchards in attracting invasive pests (Figure 2A), but some habitats (e.g., rubber) were more suitable for the survival of invasive pests than mango orchards. This also facilitates the escape of invasive pests in response to strong disturbances in mango orchards due to habitat fragmentation with high edge density, which provides a longer common boundary (Souza et al., 2020; Moore et al., 2022). These factors make configurational heterogeneity more important for invasive pests, supporting the habitat diversity hypothesis (MacDonald et al., 2018). In contrast, compositional heterogeneity had a greater effect on the associator, mainly because it was positively affected by forest cover, whereas edge density showed limited effects. However, at the landscape level, the relative effects of landscape variables on specific species remain elusive, as species respond to landscape heterogeneity, which varies widely across diverse landscape contexts (Martin et al., 2019; Moore et al., 2022).
4.2. Effects of spatial distance and combined scales
The results showed that the strength and direction of the relationships between compositional and configurational heterogeneity with the invasive pest and its associator did not vary with spatial distance. Some studies suggest that the effects of landscape variables on species vary with spatial distance scales (Aristizábal and Metzger, 2018; Redlich et al., 2018), which contradicts our results. This may be due to differences between species with different functional traits and dispersal abilities. Winged insects (such as B. dorsalis and D. melanogaster) can travel faster and farther than other insects because of their ability to fly (Miguet et al., 2016; Zhang et al., 2021), and generalist pests (such as B. dorsalis) spread farther than specialists because of extensive habitat adaptation (Miguet et al., 2016; Pan et al., 2022). In particular, invasive pests can survive in a variety of habitats and spread easily among patches owing to the formation of patch connectivity. Furthermore, the spatially coupled scales formed by small distances (e.g., 1 km) nesting within a large distance (e.g., 1.5 km) make it difficult to separate the effects from each other (Martin et al., 2016), thus making the direction and intensity of landscape variable effects constant with spatial distance.
Another novel finding of the present study is that spatially combined (coupled and decoupled) scales did not change the intensity and direction of landscape effects, which provides new insights into the effects of landscapes on pests and associators. Previous results have only shown that landscape structures control pests at spatially coupled (Aristizábal and Metzger, 2018; Kheirodin et al., 2020; Zhang et al., 2021), making it difficult to distinguish the role of surrounding landscape heterogeneity at different spatial distances from the sampled crop patches (Da Silva Carneiro et al., 2022). The comparison of results in the present study revealed that spatial coupling and decoupling did not change the landscape effects, whether the spatial distance was 1 or 1.5 km, indicating that farther and nearer distance habitat heterogeneity has similar effects on species, at least for B. dorsalis and D. melanogaster. This may be because species with a flexible diet and flight ability have an efficient dispersal capability and adaptability regardless of habitat type (Miguet et al., 2016; Zhang et al., 2021), especially for invasive pests, there may also be a lack of natural enemies in geographic space. Furthermore, habitat type and landscape variable changes were limited after spatial decoupling compared to coupling, regardless of distance.
4.3. Implications and prospects
The present findings have implications for habitat conservation and biological control, especially in the context of landscape heterogeneity due to global land-use change (Martin et al., 2019; Zheng et al., 2019). Although the results showed that forest cover was positively associated with an invasive pest, especially at a distance scale of 0.5 km radius, reducing forests to control invasive pests is not recommended. Most studies have found that forests conserve biodiversity, including plants, arthropods, birds, and mammals (Zhang et al., 2017; Aristizábal and Metzger, 2018; González et al., 2020). The benefits of controlling invasive pests by destroying forests are likely to be far less than those of biodiversity conservation by protecting forests, not to mention the fact that forests potentially provide many natural enemies to control agricultural pests (Aristizábal and Metzger, 2018; Varela et al., 2018; Martin et al., 2019). Habitat fragmentation had a negative effect on the invasive pest. Maintaining the diversity of habitat types in the surrounding landscape composition is beneficial for the control of invasive pests and can be attempted in landscape management at the 1 km landscape radius. In addition, the effects of spatial scales were not evident in the present study, it is suggested that spatial distance and combined scale should be combined to elucidate landscape effects in broader regions (Medeiros et al., 2019; Da Silva Carneiro et al., 2022), especially at spatially decoupled, as scale effects may depend on landscape contexts and species functional groups (Martin et al., 2019; Haan et al., 2020).
Pests are not only regulated by landscapes but also affected by natural enemies (Kheirodin et al., 2020; Zhang et al., 2021), which are thought to be closely related to compositional and configurational heterogeneity (Tscharntke et al., 2016; Martin et al., 2019; Kheirodin et al., 2020). Although we used a pest sampling method known to trap parasitic natural enemies (Böckmann et al., 2015), we did not capture parasitoids associated with the invasive pest that have been reported (Liu et al., 2019). It is still necessary to explore the presence or absence of other natural enemies associated with invasive pests and their responses to landscape heterogeneity at multiple spatial scales, which is important for unraveling the mechanisms of landscapes controlling invasive pests. Moreover, the effects of invasive pests on crops need to be assessed in detail, such as fruit damage rates and economic losses to smallholders, which are directly related to policymaking. Taken together, we suggest that a cascading framework of landscape structures, natural predators, invasive pests, and crop loss should be constructed to address the possible impacts of land-use change on sustainable agriculture in the future.
5. Conclusions
Landscape compositional heterogeneity with high forest cover was positively related to an invasive pest and its associator, whereas the negative effect of configurational heterogeneity on the invasive pest mainly depended on edge density. The relative effect of compositional heterogeneity on the invasive pest was lower than that of configurational heterogeneity, but the opposite was true for the associator. The direction of the relationships between landscape variables with the invasive pest and the associator did not change with spatial distance and combined scales, nor did the strengths of these relationships. Possible reasons for this are that species with wings have a high dispersal ability and habitat adaptability, and habitat types and landscape structure have limited variation at spatial scales. We believe that maintaining appropriate landscape fragmentation around crop patches is effective for inhibiting invasive pests, but further elucidation of the relationships between landscape effects, natural enemies, invasive pests, and ecological effects is necessary for developing agricultural conservation strategies in the future.
Data availability statement
The original contributions presented in the study are included in the article/Supplementary material, further inquiries can be directed to the corresponding author.
Author contributions
ZW designed the study, collected and analyzed data, and produced a draft of the manuscript. QY and BH provide technical support and process data. LZ, HZ, YS, YY, ZO, and RL provided comment on various drafts. All authors read and approved the final manuscript.
Funding
This study was supported by the Project funded by the National Natural Science Foundation of China (grant nos. 42101094 and 41925005).
Acknowledgments
We are grateful to Jindong Lin for his help with the research work.
Conflict of interest
The authors declare that the research was conducted in the absence of any commercial or financial relationships that could be construed as a potential conflict of interest.
Publisher's note
All claims expressed in this article are solely those of the authors and do not necessarily represent those of their affiliated organizations, or those of the publisher, the editors and the reviewers. Any product that may be evaluated in this article, or claim that may be made by its manufacturer, is not guaranteed or endorsed by the publisher.
Supplementary material
The Supplementary Material for this article can be found online at: https://www.frontiersin.org/articles/10.3389/fsufs.2023.1114508/full#supplementary-material
References
Aristizábal, N., and Metzger, J. P. (2018). Landscape structure regulates pest control provided by ants in sun coffee farms. J. Appl. Ecol. 56, 21–30. doi: 10.1111/1365-2664.13283
Böckmann, E., Hommes, M., and Meyhöfer, R. (2015). Yellow traps reloaded: what is the benefit for decision making in practice? J. Pest Sci. 88, 439–449. doi: 10.1007/s10340-014-0601-7
Bosem Baillod, A., Tscharntke, T., Clough, Y., and Batáry, P. (2017). Landscape-scale interactions of spatial and temporal cropland heterogeneity drive biological control of cereal aphids. J. Appl. Ecol. 54, 1804–1813. doi: 10.1111/1365-2664.12910
Clemente-Orta, G., Madeira, F., Batuecas, I., Sossai, S., Juárez-Escario, A., and Albajes, R. (2020). Changes in landscape composition influence the abundance of insects on maize: the role of fruit orchards and alfalfa crops. Agric. Ecosyst. Environ. 291, 106805. doi: 10.1016/j.agee.2019.106805
Cugala, D., Massimiliano, V., Maulid, M., De Meyer, M., and Canhanga, L. (2020). Economic injury level of the Oriental fruit fly, Bactrocera dorsalis (Diptera: Tephritidae), on commercial mango farms in Manica Province, Mozambique. African Entomol. 28, 278–289 doi: 10.4001/003.028.0278
Da Silva Carneiro, L., Ribeiro, M. C., Aguiar, W. M. D., de Fátima Priante, C., Frantine-Silva, W., and Gaglianone, M. C. (2022). Orchid bees respond to landscape composition differently depending on the multiscale approach. Landsc. Ecol. 37, 1587–1601. doi: 10.1007/s10980-022-01442-8
Fahrig, L. (2013). Rethinking patch size and isolation effects: the habitat amount hypothesis. J. Biogeogr. 40, 1649–1663. doi: 10.1111/jbi.12130
Fahrig, L. (2017). Ecological responses to habitat fragmentation per se. Annu. Rev. Ecol. Evol. Syst. 48, 1–23. doi: 10.1146/annurev-ecolsys-110316-022612
Fahrig, L., Baudry, J., Brotons, L., Burel, F. G., Crist, T. O., Fuller, R. J., et al. (2011). Functional landscape heterogeneity and animal biodiversity in agricultural landscapes. Ecol. Lett. 14, 101–112. doi: 10.1111/j.1461-0248.2010.01559.x
Fletcher, R. J., Didham, R. K., Banks-Leite, C., Barlow, J., Ewers, R. M., Rosindell, J., et al. (2018). Is habitat fragmentation good for biodiversity? Biol. Conserv. 226, 9–15. doi: 10.1016/j.biocon.2018.07.022
Gagic, V., Holding, M., Venables, W. N., Hulthen, A. D., and Schellhorn, N. A. (2021). Better outcomes for pest pressure, insecticide use, and yield in less intensive agricultural landscapes. Proc. Nat. Acad. Sci. 118, e2018100118. doi: 10.1073/pnas.2018100118
Gardiner, M. M., Landis, D. A., Gratton, C., DiFonzo, C. D., O'Neal, M., Chacon, J. M., et al. (2009). Landscape diversity enhances biological control of an introduced crop pest in the north-central USA. Ecol. Appl. 19, 143–154. doi: 10.1890/07-1265.1
González, E., Landis, D. A., Knapp, M., and Valladares, G. (2020). Forest cover and proximity decrease herbivory and increase crop yield via enhanced natural enemies in soybean fields. J. Appl. Ecol. 57, 2296–2306. doi: 10.1111/1365-2664.13732
Grechi, I., Preterre, A., Lardenois, M., and Ratnadass, A. (2022). Bactrocera dorsalis invasion increased fruit fly incidence on mango production in Reunion Island. Crop Prot. 161, 106056. doi: 10.1016/j.cropro.2022.106056
Gurr, G. M., Wratten, S. D., Landis, D. A., and You, M. (2017). Habitat management to suppress pest populations: progress and prospects. Annu. Rev. Entomol. 62, 91–109. doi: 10.1146/annurev-ento-031616-035050
Haan, N. L., Zhang, Y., and Landis, D. A. (2020). Predicting landscape configuration effects on agricultural pest suppression. Trends Ecol. Evol. 35, 175–186. doi: 10.1016/j.tree.2019.10.003
Heidrich, L., Bae, S., Levick, S., Seibold, S., Weisser, W., Krzystek, P., et al. (2020). Heterogeneity–diversity relationships differ between and within trophic levels in temperate forests. Nat. Ecol. Evol. 4, 1204–1212. doi: 10.1038/s41559-020-1245-z
Henri, D. C., Jones, O., Tsiattalos, A., Thébault, E., Seymour, C. L., and van Veen, F. J. F. (2015). Natural vegetation benefits synergistic control of the three main insect and pathogen pests of a fruit crop in southern Africa. J. Appl. Ecol. 52, 1092–1101. doi: 10.1111/1365-2664.12465
Kheirodin, A., Cárcamo, H. A., and Costamagna, A. C. (2020). Contrasting effects of host crops and crop diversity on the abundance and parasitism of a specialist herbivore in agricultural landscapes. Landsc. Ecol. 35, 1073–1087. doi: 10.1007/s10980-020-01000-0
Liu, H., Zhang, D., Xu, Y., Wang, L., Cheng, D., Qi, Y., et al. (2019). Invasion, expansion, and control of Bactrocera dorsalis (Hendel) in China. J. Integr. Agric. 18, 771–787. doi: 10.1016/S2095-3119(18)62015-5
MacDonald, Z. G., Anderson, I. D., Acorn, J. H., and Nielsen, S. E. (2018). The theory of island biogeography, the sample-area effect, and the habitat diversity hypothesis: complementarity in a naturally fragmented landscape of lake islands. J. Biogeogr. 45, 2730–2743. doi: 10.1111/jbi.13460
Malagnini, V., Cappellari, A., Marini, L., Zanotelli, L., Zorer, R., Angeli, G., et al. (2022). Seasonality and landscape composition drive the diversity of pollen collected by managed honey bees. Front. Sustain. Food Syst. 6, 865368. doi: 10.3389/fsufs.2022.865368
Martin, E. A., Dainese, M., Clough, Y., Báldi, A., Bommarco, R., Gagic, V., et al. (2019). The interplay of landscape composition and configuration: new pathways to manage functional biodiversity and agroecosystem services across Europe. Ecol. Lett. 22, 1083–1094. doi: 10.1111/ele.13265
Martin, E. A., Seo, B., Park, C., Reineking, B., and Steffan-Dewenter, I. (2016). Scale-dependent effects of landscape composition and configuration on natural enemy diversity, crop herbivory, and yields. Ecol. Appl. 26, 448–462. doi: 10.1890/15-0856
McGarigal, K., Cushman, S. A., and Ene, E. (2012). FRAGSTATS v4: Spatial Pattern Analysis Program for Categorical and Continuous Maps. University of Massachusetts, Amherst, MA, United States. Available online at: http://www.umass.edu/landeco/research/fragstats/fragstats.html
Medeiros, H. R., Grandinete, Y. C., Manning, P., Harper, K. A., Cutler, G. C., Tyedmers, P., et al. (2019). Forest cover enhances natural enemy diversity and biological control services in Brazilian sun coffee plantations. Agron. Sustain. Dev. 39, 50. doi: 10.1007/s13593-019-0600-4
Metzger, J. P., Villarreal-Rosas, J., Suárez-Castro, A. F., López-Cubillos, S., González-Chaves, A., Runting, R. K., et al. (2021). Considering landscape-level processes in ecosystem service assessments. Sci. Total Environ. 796, 149028. doi: 10.1016/j.scitotenv.2021.149028
Miguet, P., Jackson, H. B., Jackson, N. D., Martin, A. E., and Fahrig, L. (2016). What determines the spatial extent of landscape effects on species? Landsc. Ecol. 31, 1177–1194. doi: 10.1007/s10980-015-0314-1
Moore, H. A., Michael, D. R., Dunlop, J. A., Valentine, L. E., Cowan, M. A., and Nimmo, D. G. (2022). Habitat amount is less important than habitat configuration for a threatened marsupial predator in naturally fragmented landscapes. Landsc. Ecol. 37, 935–949. doi: 10.1007/s10980-022-01411-1
Ouyang, F., Su, W., Zhang, Y., Liu, X., Su, J., Zhang, Q., et al. (2020). Ecological control service of the predatory natural enemy and its maintaining mechanism in rotation-intercropping ecosystem via wheat-maize-cotton. Agric. Ecosyst. Environ. 301, 107024. doi: 10.1016/j.agee.2020.107024
Pan, Y., Hersperger, A. M., Kienast, F., Liao, Z., Ge, G., and Nobis, M. P. (2022). Spatial and temporal scales of landscape structure affect the biodiversity-landscape relationship across ecologically distinct species groups. Landsc. Ecol. 37, 2311–2325. doi: 10.1007/s10980-022-01477-x
Paredes, D., Rosenheim, J. A., Chaplin Kramer, R., Winter, S., and Karp, D. S. (2021). Landscape simplification increases vineyard pest outbreaks and insecticide use. Ecol. Lett. 24, 73–83. doi: 10.1111/ele.13622
Perrot, T., Bretagnolle, V., and Gaba, S. (2022). Environmentally friendly landscape management improves oilseed rape yields by increasing pollinators and reducing pests. J. Appl. Ecol. 59, 1825–1836. doi: 10.1111/1365-2664.14190
Ratto, F., Bruce, T., Chipabika, G., Mwamakamba, S., Mkandawire, R., Khan, Z., et al. (2022). Biological control interventions and botanical pesticides for insect pests of crops in sub-Saharan Africa: a mapping review. Front. Sustain. Food Syst. 6, 883975. doi: 10.3389/fsufs.2022.883975
Redlich, S., Martin, E. A., and Steffan-Dewenter, I. (2018). Landscape-level crop diversity benefits biological pest control. J. Appl. Ecol. 55, 2419–2428. doi: 10.1111/1365-2664.13126
Rios, E., Benchimol, M., Dodonov, P., De Vleeschouwer, K., and Cazetta, E. (2021). Testing the habitat amount hypothesis and fragmentation effects for medium- and large-sized mammals in a biodiversity hotspot. Landsc. Ecol. 36, 1311–1323. doi: 10.1007/s10980-021-01231-9
Rybicki, J., Abrego, N., and Ovaskainen, O. (2020). Habitat fragmentation and species diversity in competitive communities. Ecol. Lett. 23, 506–517. doi: 10.1111/ele.13450
Saura, S. (2021). The habitat amount hypothesis implies negative effects of habitat fragmentation on species richness. J. Biogeogr. 48, 11–22. doi: 10.1111/jbi.13958
Schmidt, J. M., Whitehouse, T. S., Green, K., Krehenwinkel, H., Schmidt-Jeffris, R., and Sial, A. A. (2019). Local and landscape-scale heterogeneity shape spotted wing drosophila (Drosophila suzukii) activity and natural enemy abundance: implications for trophic interactions. Agric. Ecosyst. Environ. 272, 86–94. doi: 10.1016/j.agee.2018.11.014
Shi, X., Xiao, H., Luo, S., Hodgson, J. A., Bianchi, F. J. J. A., He, H., et al. (2021). Can landscape level semi-natural habitat compensate for pollinator biodiversity loss due to farmland consolidation? Agric. Ecosyst. Environ. 319, 107519. doi: 10.1016/j.agee.2021.107519
Sirami, C., Gross, N., Baillod, A. B., Bertrand, C., Carrié, R., Hass, A., et al. (2019). Increasing crop heterogeneity enhances multitrophic diversity across agricultural regions. Proc. Nat. Acad. Sci. 116, 16442–16447. doi: 10.1073/pnas.1906419116
Souza, T. B., França, F. M., Barlow, J., Dodonov, P., Santos, J. S., Faria, D., et al. (2020). The relative influence of different landscape attributes on dung beetle communities in the Brazilian Atlantic forest. Ecol. Indic. 117, 106534. doi: 10.1016/j.ecolind.2020.106534
Tamburini, G., Santoiemma, G. E., O'Rourke, M., Bommarco, R., Chaplin-Kramer, R., et al. (2020). Species traits elucidate crop pest response to landscape composition: a global analysis. Proc. R. Soc. B. 287, 20202116. doi: 10.1098/rspb.2020.2116
Tougeron, K., Couthouis, E., Marrec, R., Barascou, L., Baudry, J., Boussard, H., et al. (2022). Multi-scale approach to biodiversity proxies of biological control service in European farmlands. Sci. Total Environ. 822, 153569. doi: 10.1016/j.scitotenv.2022.153569
Tscharntke, T., Karp, D. S., Chaplin-Kramer, R., Batáry, P., DeClerck, F., Gratton, C., et al. (2016). When natural habitat fails to enhance biological pest control – five hypotheses. Biol. Conserv. 204, 449–458. doi: 10.1016/j.biocon.2016.10.001
Varela, E., Verheyen, K., Valdés, A., Soliño, M., Jacobsen, J. B., De Smedt, P., et al. (2018). Promoting biodiversity values of small forest patches in agricultural landscapes: Ecological drivers and social demand. Sci. Total Environ. 619–620, 1319–1329. doi: 10.1016/j.scitotenv.2017.11.190
Wen, Z., Jiang, Z., Zheng, H., and Ouyang, Z. (2022). Tropical forest strata shifts in plant structural diversity-aboveground carbon relationships along altitudinal gradients. Sci. Total Environ. 838, 155907. doi: 10.1016/j.scitotenv.2022.155907
Zeileis, A., Leisch, F., Hornik, K., and Kleiber, C. (2002). Strucchange: an R package for testing for structural change in linear regression models. J. Stat. Softw. 7, 1–38. doi: 10.18637/jss.v007.i02
Zhang, J., You, S., Niu, D., Gavilanez Guaman, K. G., Wang, A., Saqib, H. S. A., et al. (2021). Landscape composition mediates suppression of major pests by natural enemies in conventional cruciferous vegetables. Agric. Ecosyst. Environ. 316, 107455. doi: 10.1016/j.agee.2021.107455
Zhang, M., Chang, C., and Quan, R. (2017). Natural forest at landscape scale is most important for bird conservation in rubber plantation. Biol. Conserv. 210, 243–252. doi: 10.1016/j.biocon.2017.04.026
Keywords: landscape complexity, landscape fragmentation, landscape effects, invasive species, pest control, multiscale method
Citation: Wen Z, Yang Q, Huang B, Zhang L, Zheng H, Shen Y, Yang Y, Ouyang Z and Li R (2023) Landscape composition and configuration relatively affect invasive pest and its associator across multiple spatial scales. Front. Sustain. Food Syst. 7:1114508. doi: 10.3389/fsufs.2023.1114508
Received: 02 December 2022; Accepted: 12 January 2023;
Published: 27 January 2023.
Edited by:
Wen Xie, Insititute of Vegetables and Flowers (CAAS), ChinaReviewed by:
Xingyuan Men, Shandong Academy of Agricultural Sciences, ChinaShiliang Liu, Beijing Normal University, China
Paul Glaum, Waterborne Environmental, Inc., United States
Copyright © 2023 Wen, Yang, Huang, Zhang, Zheng, Shen, Yang, Ouyang and Li. This is an open-access article distributed under the terms of the Creative Commons Attribution License (CC BY). The use, distribution or reproduction in other forums is permitted, provided the original author(s) and the copyright owner(s) are credited and that the original publication in this journal is cited, in accordance with accepted academic practice. No use, distribution or reproduction is permitted which does not comply with these terms.
*Correspondence: Ruonan Li, cm5saUByY2Vlcy5hYy5jbg==