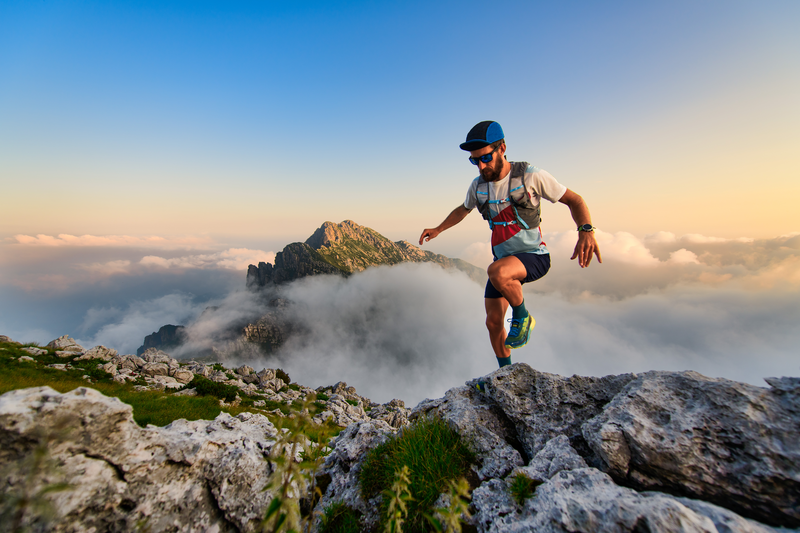
95% of researchers rate our articles as excellent or good
Learn more about the work of our research integrity team to safeguard the quality of each article we publish.
Find out more
ORIGINAL RESEARCH article
Front. Sustain. Food Syst. , 03 April 2023
Sec. Climate-Smart Food Systems
Volume 7 - 2023 | https://doi.org/10.3389/fsufs.2023.1101717
Abiotic stresses (drought, heat) are one of the major impediments to enhancing the maize productivity of marginal farmers in the facet of climate change. The present study attempts to investigate the impact of heat-tolerant maize hybrid on yield and income in the Terai region of Nepal. This study uses cross-sectional farm household-level data collected in August 2021 from a randomly selected sample of 404 rural households. We used a doubly robust inverse probability weighted regression adjustment method to obtain reliable impact estimates. Adoption of heat-tolerant hybrid increases yields by 16% and income by 44% in the spring season (a stress condition). Overall, yield increases by 12%, net income by 31%, saving of 40% in seed costs, and per capita food expenditure increases by 8.50%. Hence a conducive environment must be created for scaling up heat-tolerant maize varieties to increase productivity, minimize risk, and transform of the maize sector.
Maize is cultivated on nearly 202 million hectares globally and contributes approximately 44% (1,162 million tons) to global grain production. As a result of the research and development of breeding technologies, world maize productivity increased by 33% over the last two decades (2000–2020) (FAOSTAT, 2022). It is an important staple food in many countries in the world. As maize has multiple uses—food, feed, fuel, and for industrial use –potential demand for it rises throughout the year globally. Maize is used by industries in Asia thus: 65–70% for the feed industry, 20% for industrial applications, 6–8% in the food industry, and 2–5% for seed and other uses (FICCI, 2015). Concerning Nepal, maize is the second most important cereal crop cultivated on 0.96 million hectares, with a total production of 2.84 million tons with a productivity of 2.96 tons/ha in 2020 (FAOSTAT, 2022). It is widely grown in all three agroeconomic zones of Nepal: Terai and Inner Terai (below 900 msl), the mid-hills (900–1,800 msl), and high hills (above 1,800 msl). Per capita consumption of maize is 98 g per day in Nepal, which is the highest among South Asian countries (Ranum et al., 2014). Hybrids and open-pollinated varieties (OPVs) occupy 12–15%, and 85–88% of the total maize area in the country, respectively (Koirala et al., 2020a,b). Low seed replacement rate and wide yield gap between actual (2.84 t ha-1) and potential yield (6.50 t ha-1) of maize at the farmer level (Koirala et al., 2020c) are the major reasons for lower production and productivity of the crop. The seed replacement rate of maize is 15.56% in 2018–19 (MoALD, 2021) which should be increased to 33% by 2025 (SQCC, 2013).
Agricultural output is a collective action of climatic conditions over a period. We have been hearing the words “climate change” for the last two decades but now we are actually experiencing the effect of climate change on the agricultural ecosystem. As an effect of climate change, abiotic stresses such as drought, heat stress, flood, long dry spells, become major impediments to maize production in maize-based agro-ecologies, including Nepal. Preceding studies on Nepal show that no change in the total amount of annual precipitation but the unreliable pattern of fewer rainy days with high-intensity of rainfall (Shrestha et al., 1999; Baidya and Karmacharya, 2007) causing prolonged drought and flood-like situations. The winter season of 2006–2008 was usually reported as dry. More than 150 droughts were reported in a period of 36 years (1971–2007) affecting more than 330,000 ha of agricultural land in Terai and Western hills/mountains of Nepal (NSET, 2009). Furthermore, there has been a substantial rise in the incidence of hot nights and a substantial decrease in the incidence of cold days and nights. Hot nights have surged by 2.5% (McSweeney et al., 2008). Mean temperature across Nepal is projected to increase by 0.5 to 2° C by the 2030s, 1.7–4.1°C by the 2060s, and 3.0–6.3°C by the 2090s (NCVST, 2009; Bhusal, 2019). The frequency of “hot days” (the hottest 5% of days in the period 1970–1999) in the pre-monsoon period are projected to increase by 15–55% by the 2060s, and 26–69% by the 2090s (NCVST, 2009).
Heat stress can be defined as temperatures above a threshold level that results in irreversible damage to crop growth and development and is a function of intensity, duration, and the temperature increase rate (Larkindale et al., 2005; Zaidi et al., 2016). In maize, the favorable soil temperature for seedling growth is 26°C, and when this temperature rises, root and shoot mass both decline by 10% for each degree of increase until 35°C when growth is severely retarded (Walker, 1969). The most critical stages for heat stress are from tassel emergence to early grain filling (Nejad et al., 2017; Thayil et al., 2020). At elevated temperatures, the number of fertilized ovules that develop into grain decreases (Schoper et al., 1987a,b). Pollen produced under elevated temperatures has reduced viability (Herrero and Johnson, 1980; Schoper et al., 1986, 1987a,b; Dupuis and Dumas, 1990; Lizaso et al., 2018; Prasanna et al., 2021) leading to poor grain setting. For every day with temperature above 30° C grain yield was reduced by 1% and 1.7% under optimal rainfed and drought conditions, respectively (Lobell et al., 2011). Lobell and Burke (2010) suggested that a 2°C increase in temperature would result in a greater decrease in maize yields than a 20% decrease in precipitation. Spring maize, grown in the maize-rice system is estimated to account for about 15.5% of the total maize in Nepal. Yield loss of spring and early summer maize reaches up to 75% due to heat stress (Koirala et al., 2017) which causes leaf scorch, silk damage, and panicle shock, which relies upon at the crop's stage, severity, and duration of stress (Monsanto, 2012). These reports highlight the need to incorporate tolerance to heat stress into the maize germplasm. The development of climatic-resilient maize hybrid is an appropriate option to deal with such climatic uncertainties (Acharya and Bhatta, 2013). In recent years, plant breeding techniques focus on the development of heat-tolerant crop varieties intended to help marginal farmers to mitigate weather stress. Studies conducted by Simtowea et al. (2019) found that the adoption of drought-tolerant maize varieties (DTMVs) increased yield by 15% over non-adopters in Uganda. Wossen et al. (2017) conducted a study in Nigeria and found that adoption of DTMVs increased maize yields by 13.3% and reduced the level of variance by 53%. Lunduka et al. (2019) found that adopters received 617 kg/ha more yield as compared to non-adopters and a change in varieties to drought-tolerant maize seeds give an extra income of USD 240/ha or more than 9 months of food at no additional cost. Manda et al. (2018) used Inverse Probability Weighted Regression Adjusted (IPWRA) methods to estimate the treatment effects and it showed that per capita food expenditure and the probability of food security increased by ZMK 127,000 (USD 24) and 21 percent with improved maize adoption, respectively in Eastern Zambia. Most of the adoption and impact of abiotic stress-resilient maize varieties studies were carried out in Africa, very few were observed in Asia (Kulkarni et al., 2022). To reduce the observed literature gap, the study has been conducted in Nepal where seeds of Heat-Tolerant Maize Hybrid (HTMH) are deployed by seed companies. HTMHs have been developed in a collaborative effort by the International Maize and Wheat Improvement Center (CIMMYT), National Maize Research Program (NMRP) and deployed with the help of private seed companies in Nepal.
The main objectives of this paper are twofold: (1) to investigate the determinants of adoption of heat-tolerant maize hybrid; and (2) to assess the effect of adoption of HTMH on yield and profitability in the Terai region of Nepal. The paper is constructed as follows. The demand for hybrid maize is discussed in Section 2. Data collection methods and analytical framework are summarized in Section 3. Section 4 discusses the descriptive and econometric results. The last section (Section 5) concludes the study and offers policy recommendations.
In the border districts adjacent to India, farmers started growing maize hybrids in the 1980s by importing seeds from India (Thapa, 2013). Demand for maize is continuously increasing as it is being used as food, feed, fodder, and industrial raw materials. OPVs are the result of natural (uncontrolled) pollination between plants, and their genetic diversity can be limited. As a result, it may not have the desired traits that make them responsive to increased inputs such as fertilizers, water, and pesticides. As a result, the yield of OPVs cannot be increased beyond a certain limit even though applying high inputs (Koirala et al., 2021). To increase productivity, hybrid maize research was commenced officially in 1987 (Koirala et al., 2002). The National Maize Research Program (NMRP) released the first maize hybrid (Gaurav hybrid) in 2003 (SQCC, 2013) but “Gaurav” could not reach farmers' fields due to the non-synchronization of male and female parents hindering F1 seed production (NMRP Annual Report 2018). Since then, NMRP has released and registered seven single cross-hybrids in collaboration with the International Maize and Wheat Improvement Center (CIMMYT). Hybrids, namely Rampur Hybrid-2 (2012), Khumal Hybrid-2 (2014), Rampur Hybrid-4 (2016), Rampur Hybrid-6 (2016), Rampur Hybrid-8 (2018) and Rampur Hybrid-10 (RH-10) (2018) are available in the market SQCC (2022). Out of these, Rampur Hybrid-8 and Rampur Hybrid-10 are heat-tolerant single cross hybrids. The area covered under hybrid maize seed was only 150,000 ha up to 2019, (National Seed Vision (NSV): 2013–2025, 2020). NMRP and CIMMYT have licensed RH-8 and RH-10 to local seed companies to speed up the pace of hybrid seed production and distribution in the country. A total of 59 hybrids are registered between 2010 and 2018, of which seven were released and registered for NMRP Rampur release and 52 are hybrids of multinational companies (Kandel, 2021).
Even though seven hybrid varieties have been released, hybrid seed production is in the nascent stage compared to country demand. Nepal relies heavily on imported hybrid seeds to fulfill local demand. Demand for maize hybrid seed increased from 20 tons in 2008 (Paudel et al., 2022) to 27,754 tons in 2019–20, which created a trade deficit of USD 9.76 million (MoALD, 2021). Moreover, importing hybrid seed increases the price, and restrain farmers from adopting hybrid seeds. In Nepal, the growth rate of the livestock population enhanced from 0.73 to 1.23% per annum for the 20-year period from 1994/95 to 2014/15 (Upadhyay et al., 2017), which increased the demand for maize for animal feed production. Out of the total maize used for feed production, 87% was imported from India each year by feed industries (Gairhe et al., 2021). Nepal imported 421 million tons of maize which constitutes a value of USD 116 million (MoALD, 2021). To enhance the adoption of hybrids, the Government of Nepal, implemented a subsidized hybrid maize seed distribution program through the Prime Minister Agricultural Modernization Project (PMAMP) and the Climate Smart Agriculture Project implemented by the Lumbini provincial government. To meet the demand for hybrid maize, NSV (2013–25) foreseen the development and promotion of 12 and five maize hybrids by the public and private sectors, respectively, by 2025.
Data used in this study come from a farm-household survey conducted in August 2021, just after 15 days of lifting the restrictions of the second COVID lockdown in villages of the Terai region of Nepal. CIMMYT Asia conducted the survey in collaboration with NMRP, Rampur, Nepal, under the project Heat Tolerant Maize for Asia (HTMA). Trained researchers using structured questionnaires created through computer-assisted personal interviewing (CAPI) software with validation to minimize data entry errors and save time for face-to-face interviews. The questionnaire generated information on household demographics, socio-economic status, agricultural landholding, agricultural input use for maize cultivation and outputs, maize varieties cultivated, and sources of income and expenditure. The data was collected from two districts in the Terai region of Nepal, namely Banke and Dang, which were intentionally selected based on the deployment of RH-10 hybrid by Nepali seed companies and the maize area under cultivation (Figure 1). Seed companies distributed seeds to farmers of RH-10 through the Prime Minister Agricultural Modernization Project (PMAMP) and Climate Smart Agriculture Programs via co-operative societies. From Banke (Nepalgunj) district, Khajura and Duduwa rural municipalities were selected, whereas from Dang district, Rapti, Gadawa, Rajpur rural municipalities, and Lamahi municipality were selected purposely based on seed deployment of RH-10 through co-operatives societies. Adopter farmers and non-adopter farmers were selected randomly from the same and nearby villages for the study. From Banke district 146 maize growing farm households (71 adopters and 75 non-adopters), and from Dang district 258 maize growing farm households (121 adopters and 137 non-adopters) were selected which forms the full sample size of 404 (192 adopters and 212 non-adopters) farmers for the study.
The major objective of this study is to analyze the impact of the adoption of heat-stress tolerant maize hybrid (RH-10) on yield and income. We used the average treatment effect. It is defined as the change in an outcome affected from one subject (often an individual) receiving one treatment instead of another. The causal effect is measured by the average treatment effect (ATE), which is the average difference in outcomes of households adopting improved maize with and without the technology (Takahashi and Barrett, 2013). The potential outcome is the outcomes we would observe under each possible treatment option. The potential outcome is what we would observe if we set treatment to certain values, such as treated vs. untreated.
Where E–Expected values
Y1-Potential outcome if population treated with treatment (A) = 1
Y0-Potential outcome if population treated with treatment (A) = 0.
The expected value of this difference in the potential outcome is a causal effect because we are comparing the same population of farmers. The only different factor is treatment. So, in one case we give everyone treatment A = 0, in the other case, we give everybody A=1. So, we have isolated the treatment effect in that sense. We use the IPWRA estimation method proposed by Wooldridge (2010) as our primary estimator. IPWRA combines inverse probability weighting (IPW) and regression adjustment (RA) methods to estimate the treatment effect. IPW weights the observations by the inverse of their propensity score, which is the probability of receiving the treatment given observed covariates. RA involves fitting a regression model to the outcome variable, controlling for the observed covariates. By combining these two methods, IPWRA aims to balance the covariates between the treated and untreated groups and estimate the treatment effect. Doubly robust estimators, on the other hand, use both the outcome modeling strategy of RA and the treatment modeling strategy of IPW. These estimators are called doubly robust because they provide consistent estimates of the treatment effect if either the outcome model or the propensity score model is correctly specified. This property makes doubly robust estimators more robust to model misspecification than either IPW or RA alone. Overall, both IPWRA and doubly robust estimators are useful methods for estimating causal effects in observational studies where randomization is not possible.
In the IPW method, for subjects who did receive treatment, the weight is equal to the reciprocal of the predicted probability of treatment. For subjects who did not receive treatment, the weight is equal to the reciprocal of the predicted probability of not receiving treatment; the probability of not receiving treatment is just one minus the probability of receiving treatment:
A sample of data from n farmers with independent individual covariates (Xi) and identically distributed treatment indices (Ti) i 1, …n. Propensity scores are unknown and estimated based on observed covariates and treatment allocation. Let πi be the estimated propensity score and let T() be the treatment indicator function, if the condition is met, the value is 1 and otherwise 0. The inverse probability-weighted estimate of treatment-specific effect is given by Wooldridge (2010) with the following estimating equation:
Where,
n = number of people in the population
Ti=1 if treated, otherwise 0
Yi= Outcome variable
πi(Propensity score) = P(T = 1∣Xi)
Any type of estimator using the propensity score requires three assumptions: consistency, exchangeability, and positivity. Consistency means that the treatment assignment should not be affected by any unobserved or unknown factors that may influence both the treatment assignment and the outcome of interest. Exchangeability, means that the treatment assignment is random or as-if random, and there are no confounding factors that are related to both the treatment assignment and the outcome. Positivity is the assumption that all subjects have a non-zero probability of receiving each treatment: 0 <Pr (A = 1) <1.
Table 1 presented descriptive statistics of some explanatory variables used in the study disaggregated by adopters' households' status. About 92% of the households were male-headed. The average age of the household head was 50.53 years, with adopting households reported as being 2.66 years significantly older than non-adopters. An average household comprised of 6.86 persons, with adopting households reporting larger households (7.03 persons) as compared to the non-adopters (6.71 persons). The average landholding was 0.92 ha in the sampled households. Per capita per person landholding size was 0.15 ha and adopting households have significantly larger landholding than non-adopters. Household size and landholding indicated over-dependency on agriculture. The average land allocated to maize crops was 0.27 ha. Only 11% of households used tractor-drawn seed drills for sowing, whereas 69.80% of households used dibbling method of sowing. A significant difference was observed with respect to formal group membership; among the adopters, 93% of households were members of a co-operative society/SHG whereas it was 79% of households among non-adopters. Significant differences were observed in sources of agriculture information; adopter farmers had more access to agricultural co-operative societies and agriculture extension offices whereas non-adopters accessed agrovets, friends/relatives. Average maize seed cost was NPR 7,353/ha, with an adopting household reporting significantly lower seed cost (NPR 5,366/ha) compared to non-adopters (NPR 9,153/ha). Seed price of RH-10 hybrid was NPR 3,787/ha lower than non-adopter maize hybrid. With respect to inputs used, no significant differences were observed in fertilizer, manure and number of irrigations given. Average maize grain yield reported was 42.35q/ha and adopter households had a significantly higher yield (44.25 q/ha) compared to non-adopters (40.63 q/ha). Adopter farmers received 3.62 q/ha—a significantly higher yield than non-adopters. As adopter farmers were located closer to the input dealer and government extension offices as compared to non-adopters, a significant difference was observed in years of awareness of new hybrids in them as compared to non-adopters. Out of the total sample households, 59% of farmers grew maize in the spring season, with adopters showing a significant 66% as compared to 53% in non-adopter farmers. The nature of the soil in the Dang district is sandy loam soil whereas the Banke district site represents clay type of soil with poor drainage. Farmers prefere good soil depth in plots for new varieties as compared to conventional maize hybrid. Adopting households had a 7.69% higher per capita food expenditure (PCFE) (NPR 27,953 or USD 238.18) compared to non-adopter farmers (NPR 25,953 or USD 221.14).
Suitable climatic conditions and cultural practices can lead to attaining the potential yield of the maize crop. But climatic conditions beyond control leads to low yield and financial loss. In rainfed agriculture, long dry spells, moisture stress, high temperature at critical stages increase the yield loss of farmers. Even in optimal climatic conditions during the winter season, differences in day maximum-night minimum temperature increases are one of the important climatic factors that influence increasing the average yield. With respect to crop diversity and water availability, after harvesting mustard-potato, in the study locations, farmers prefer maize in the spring season as compared to other crops. In the spring season, sowing takes place in January and February months whereas pollination happens in the March-April months. <33°C is the optimal temperature for plant growth, 33°C−36°C is referred as a moderate stress condition, 36°C−40°C is a stress condition and >40°C is severe stress condition, drastically affecting most of the physiological process of plant growth (personal communication with P.H. Zaidi). In general, spring maize segment in South Asia comprises >37°C temperature leads to heat-stress (Thayil et al., 2020). Day maximum-minimum temperature data was collected for January 2020–April 2021 from the Directorate of Agricultural Research, Khajura, Banke (Nepalgunj) district at the time of the survey, and given in Figure 2. The graph (below) reveals that, at the time of pollination, which overlaps in March-April months, the maximum temperature was 33°C in March and 38°C in April 2020, whereas in 2021 it was 37.40° C in March and 40.40° C in April, which is beyond the critical limit for pollen viability, stigma receptivity, formation of the pollen tube and for crop development – thus drastically affecting fertilization and early grain filling. In the spring season, the average temperature ranges from 15°C to 25°C whereas the maximum temperature ranges from 33°C to 40.40°C. The previous climatic study showed that the physiological functions of plants are affected adversely when the temperature goes beyond 35°C (Walker, 1969; Traore et al., 2000; Chaubey et al., 2016), and here the need for heat-stress resilient maize hybrid comes into action. Therefore, the development and deployment of heat-resilient varieties is an appropriate option or risk mitigation strategy to deal with the negative impacts of climate change (Acharya and Bhatta, 2013).
Figure 2. Maximum, minimum, and average temperature in the study location. Source: directorate of Agricultural Research, Khajura, Banke, 2021.
Cost of cultivation disaggregated by heat stress resilient maize hybrid adopters and non-adopters are presented in Table 2. Low landholding and more family members dependent on agriculture leads to most of the agricultural work being done through family labor alone. A significant difference has been observed in the seed cost of adopters and non-adopters. The seed cost of the heat-tolerant hybrid was NPR 3,787/ha lower than the cost of the conventional hybrid seed. This is because the production and deployment of heat-tolerant maize hybrid (RH-10) is done by Nepali local seed companies. As RH-10 hybrid seed produced by local Nepali seed companies has their own seed processing unit in core maize production districts in Nepal and this enables a price advantage to farmers by way of low transportation and marketing costs as compared to conventional hybrid seed which is mostly produced by multi-national companies (MNCs). MNCs' hybrid maize seed coming from India involved huge transportation, storage, import, and other taxes which increases the seed cost to farmers. But being local seed companies can give a price advantage to farmers. We didn't find any noticeable difference in fertilizer cost. It is observed that the use of weedicide is negligible in the study location. Only 39% of farmers used threshers for threshing maize, indicating that 61% of households still do manual threshing because of the availability of family labor, which increases the total labor cost.
Paid-out cost of adopters was significantly less by NPR 4,032/ha than of non-adopters because of reasonably low locally produced hybrid seed sold by Nepali seed companies. The family labor cost of adopter farmers was slightly more because more labor is required for harvesting and threshing the additional yield, but the difference is not significant. Total cost of maize cultivation for adopters was NPR 76,397 whereas for non-adopters it was NPR 78,284/ha which matches with the earlier studies of Paudel et al. (2022). The total cost of adopters was less by NPR 1,887/ha as compared to non-adopters. Adopter farmers received 3.62 quintals extra which is a significant rise (at 5%) in yield/ha as compared to non-adopter farmers. A significant notable difference was also observed in dry fodder and grain income. Adopter farmers received an additional net income of NPR 13,716/ha because of the two-way benefit that is saved in seed cost and from additional yield.
Partial budgeting is a financial analysis tool used in business and agriculture to evaluate the potential impact of a specific change in a farm business operation or production process. In our case, the change is the adoption of the RH-10 heat-tolerant maize hybrid, while other things stay the same. Given the one change of adopting RH-10 by farmers, what will be the effect on cost and returns? This will be answered through partial budgeting. With respect to full sample size, adopter farmers get 3.62 q/ha additional yield, and two more laborers are also needed as more operations are needed for the extra yield. Because of the RH-10 hybrid, adopter farmers saved NPR 3,787 in seed cost (Table 3). Adopter farmers received NPR 12,541.5 additional income over non-adopters. With respect to seasons, adopter farmers received NPR 17,379.75 additional income in the spring season (Table 4), whereas in the winter season adopter farmers received at par yield, even though adopters received positive income (NPR 2,232 [USD 14.68]) because of farmer savings in seed cost of RH-10 (Table 5).
Table 3. Scenario 1: partial budget of RH-10 for full sample size (regardless of location and season).
The maximum likelihood of estimates of the logit model of adoption of heat tolerance maize hybrid in Nepal's Terai region is presented in Table 6. It indicates the driving forces behind the farmer's decision to adopt agricultural technologies. Household size or active family labor force positively affected the adoption of heat-tolerant maize hybrid in the study location. This reflects the importance of family labor (as evidenced by the number of worker family members) in cultivating the new heat-tolerant maize varieties. Labor cost in Nepal is higher because of the higher migration rate to other countries. But the family members working in nearby districts come back to work on farms at the time of agricultural practices such as sowing (dibbling), weeding, harvesting, and threshing. During the peak time of sowing and harvesting, families with small household sizes could face labor shortages and will have to pay more wages for hired labor to perform timely agricultural practices. The positive effect of household size is also in harmony with Gebremedhin et al. (2009) and Manda et al. (2018) for improved maize varieties.
To adopt newly introduced varieties, farmers need to be aware of the varieties available in the market. Sometimes, the lack of awareness of new technology may lead to hindering the adoption. Results indicated that those farmers whose major source/access to agriculture information are agriculture extension offices and co-operative societies, are more likely to adopt as compared to farmers who get information from agrovets and other sources like friends/relatives. In villages, the agrovet is one of the major sources of agricultural information, but most of the time they promote those technologies which deliver a higher margin to them. The seed producers of RH-10 hybrid have kept seed prices reasonable by using co-operative societies and agriculture extension offices. Farmers who have frequently visited government extension offices and members of social networks (like co-operative societies) experiment more with new maize seeds. This positive effect of sources/access to information is consistent with Shiferaw et al. (2008) for improved pigeon pea varieties in Tanzania, Kristjanson et al. (2005) for cowpea varieties, Kaliba et al. (2000) for maize varieties, and Geberessiliese and Sanders (2006) for sorghum in Ethiopia. Adegbola and Gardebroek (2007) report that extension officers positively correlated with improved maize adoption. Sources of seed purchased are also one of the major factors in the adoption of new varieties. Most of the time source of seed purchase influenced the farmer's decision on the adoption of any variety. Our study revealed that when a farmer purchases seed from a cooperative society he has a higher likelihood of adopting heat-tolerant maize as compared to a farmer who purchased seed from an agrovet while keeping other things constant. Farmers having sheep and goats and good soil depth of maize plot were significantly associated with a higher probability of adopting improved maize. The model is correctly predicted within 82.43% accuracy, which means this percentage of variations were explained by these variables.
The IPWRA model estimates the result by counterfactual effect with and without treatment effect. Results on the impact of adoption of stress-resilient maize hybrids on three outcome variables—yield, gross income and net income—are presented in Table 7. Analysis was done by full sample size and season-wise. In the full sample size model, adoption of the RH-10 hybrid has a significant and positive impact on yield (quintals/ha), gross income, net income (NPR/ha) and per capita food expenditure. It indicates that adoption of heat-tolerant maize hybrid increases the average yield by 4.53 q/ha or 11.59% more than non-adopting households, irrespective to season. The yield impact had been observed more in the spring season. Adopter farmers estimated yield was 41.41 q/ha and non-adopters was 36.85 q/ha in the spring season. In other words, yield of adopters that can be attributed solely to adoption of heat-tolerant maize hybrid was 5.56 q/ha or 15.51% higher than that of non-adopters. In the winter season also, adopters got 2.79 quintal/ha additional yield over non-adopters, but the difference is not significant. Yield is the first important criterion considered by farmers for maize variety selection. So, this criterion is fulfilled by a new hybrid. The result implies that heat-tolerant hybrid adoption increases productivity. Our findings are similar to earlier studies of drought-tolerant hybrids conducted in different locations (Balaji and Kumar, 2016; Wossen et al., 2017; Manda et al., 2018; Amondo et al., 2019; Lunduka et al., 2019; Simtowea et al., 2019), which indicated that adoption of drought-tolerant hybrids deliver yield advantage and cushion farmers in marginal climatic condition. Outcome model estimate (OME) 1 indicates the factors affecting yield for adopters and OME 0 for non-adopters mentioned in Supplementary Table S1 and the SWOT analysis of RH-10 mentioned in Supplementary Table S2. Many of the variables show a significant effect on yield of adopters and non-adopters. Major sources of agricultural information (co-operatives), number of family members migrated, winter season, years of hybrid maize cultivation and distance to extension offices were found to have positively influenced the yield levels whereas proportionate maize area (%) and distance to input dealers negatively affected on yield for adopter farmers. For non-adopter farmers major sources of agriculture information (co-operatives), winter season, number of migrated members, family size, years of hybrid maize cultivation, distance to extension officers and number of irrigations positively influenced yield while caste, ownership status of sheep and goats, source of seed purchased (co-operative) and district were negatively influencing yield level. In the treatment model, all variables were found to be significant, except sources of information received from agrovet.
The added contribution of adopting heat-tolerant maize varieties toward net income were estimated at NPR 12,819/ha, in the full sample model. In other words, the net income of adopters that can be attributed solely to adoption of heat-tolerant maize varieties was 31.25% higher than that of non-adopters. With respect to the spring season, net income impact was estimated as NPR 15,486/ha or 43.77% higher than that of conventional hybrid farmers. In the winter season also, the model estimated a positive net income advantage over non-adopters, but the differences were not significant. Adoption of a heat-tolerant maize hybrid has significant and positive impact on per capita food expenditure and the probability of food security. The added contribution of adopting heat-tolerant maize varieties toward wellbeing indicator, per capita food expenditure, was enhanced by NPR 2,223 (USD 18.94) or 8.50% higher than the non-adopter farmers. The model indicated that the heat-tolerant maize (RH-10) hybrid performed better in all scenarios and was best suited for the study location.
This paper analyzed the impact of heat-tolerant maize variety(ies) on the yield and profitability of smallholder farmers using primary data from the Terai region of Nepal. The study employed an inverse probability weighted regression adjustment approach that produces estimates that are doubly robust against the selection bias model to estimate the overall impact of RH-10 adopters on yield and income. The result indicated–from all the estimation methods used in this study–that the adoption of heat-tolerant maize hybrid leads to a significant positive impact on yield in the study location of Nepal. The IPWRA model suggested that RH-10 delivered significant yield gain and net income advantage in the spring season (heat-stress condition) whereas it performed at par in the winter (optimal condition) season. The model also shows that households that did not adopt would also have benefited significantly had they adopted improved maize varieties. Partial budgeting illustrates that adopter farmers got two-way benefits: one is yield advantage in stress conditions, and the second is saving in seed cost. In the climate change scenario, stress could occur in any crop physiological stage of plants. Risk mitigation technologies, such as climate-resilient hybrid varieties, could be one of the appropriate strategies to diminish the risk effect on yield and livelihoods of smallholder farmers in marginal areas.
By 2020, there were nine seed companies involved in RH-10 seed production and deployment in Nepal (Gairhe et al., 2021). In-country hybrid seed production (RH-10 and RH-8) enables seed companies to deliver high-quality seed at a reasonable price to compete with MNCs by reducing transportation, marketing and import taxes as well as reducing over-dependency on hybrid seed imports from neighboring countries. R&D programs and government policies should create an enabling environment for developing efficient and effective public, community, and private seed-related organizations. In collaboration with partner institutes with a public-private partnership model, in-country seed production strengthens the seed value chain along with employment generation. To promote the adoption of heat-tolerant maize hybrid, the hybrid seed subsidization program should continue for some more years, and later it should apply only to in-house hybrid produced seed only (excluding the seed subsidy on the imported hybrid seed). The significant impact of the heat-tolerant maize hybrid is a remarkable result because maize is the second most produced cereal crop by smallholder farmers in Nepal as it accounts for the second largest share of production by volume, and it is produced by more than 2.5 million farming households in the country. Hence, it can significantly contribute to smallholder farmers' economic and social development.
The raw data supporting the conclusions of this article will be made available by the authors, without undue reservation.
Ethical review and approval was not required for the study on human participants in accordance with the local legislation and institutional requirements. Written informed consent from the participants was not required to participate in this study in accordance with the national legislation and the institutional requirements.
AK: methodology, software, formal analysis, investigation, data curation, writing—original draft, and visualization. MT and SS: resources and writing—review and editing. DG: methodology, investigation, resources, and review. KK: investigation, resources, writing—review and editing, and supervision. MK and DR: investigation, resources, and review. PZ: conceptualization, validation, supervision, and project administration. All authors contributed to the article and approved the submitted version.
This study was conducted as part of the Heat Tolerant Maize for Asia research project at the International Maize and Wheat Improvement Center (CIMMYT), Hyderabad, India and financially supported by the United States Agency for International Development (USAID). Grant number [CGIAR Trust fund–MTO No. 069033 (HTMA)].
The household survey was conducted in collaboration with CIMMYT (Hyderabad) and the National Maize Research Program (NMRP), Rampur, Nepal. The authors are grateful to PMAMP, co-operative societies for sharing the adopters' farmers list and other necessary information. We are thankful to the Directorate of Agricultural Research, Khajura, Banke to provide the weather data. Finally, we are thankful to the maize farmers who participated in the survey.
The authors declare that the research was conducted in the absence of any commercial or financial relationships that could be construed as a potential conflict of interest.
All claims expressed in this article are solely those of the authors and do not necessarily represent those of their affiliated organizations, or those of the publisher, the editors and the reviewers. Any product that may be evaluated in this article, or claim that may be made by its manufacturer, is not guaranteed or endorsed by the publisher.
The Supplementary Material for this article can be found online at: https://www.frontiersin.org/articles/10.3389/fsufs.2023.1101717/full#supplementary-material
Acharya, S. P., and Bhatta, G. R. (2013). Impact of climate change on agricultural growth in Nepal. NRB Econ Rev. 25, 1–16. Available online at: https://www.climatenepal.org.np/sites/default/files/doc_resources/cmihy71qq6q.pdf
Adegbola, P., and Gardebroek, C. (2007). The effect of information sources on technology adoption and modification decisions. Agricultural Econ. 37, 55–65. doi: 10.1111/j.1574-0862.2007.00222.x
Amondo, E., Simtowe, F., Rahut, D. B., and Erenstin, O. (2019). Productivity and production risk effects of adopting drought-tolerant maize varieties in Zambia. Int. J. Clim. Chang. 11, 570–591. doi: 10.1108/IJCCSM-03-2018-0024
Baidya, S., and Karmacharya, J. (2007). “Observed and future climate change in Nepal,” in National Workshop on Climate Change and Human Health: Potential Impact, Vulnerability and Adaptation in Nepal, Meghnath, D. (ed.). Kathmandu, Nepal. p. 19-21.
Balaji, S. J., and Kumar, S. (2016). Constraints in cotton cultivation: cost issues and options for income increments. Indian J. Agric. Econ. 71, 361–373. doi: 10.22004/ag.econ.302221
Bhusal, S. (2019). Agricultural impacts of climate change and the climate change adaptations–a nepalese context review. Acta Scientific Agri. 7, 234–238. doi: 10.31080/ASAG.2019.03.0550
Chaubey, I., Bosch, D. D., Munoz-Carpena, R., Harmel, R. D., Douglas-Mankin, K. R., Nejadhashemi, A. P., et al. (2016). Climate change: a call for adaptation and mitigation strategies. Trans. ASABE. 59, 1709–1713. doi: 10.13031/trans.59.12138
Dupuis, I., and Dumas, C. (1990). Influence of temperature stress on in vitro fertilization and heat shock protein synthesis in maize (Zea mays L.) reproductive tissues. Plant Physiol. 94, 665–670. doi: 10.1104/pp.94.2.665
FAOSTAT. (2022). Available online at: https://www.fao.org/faostat/en/#data/QCL (accessed on 18 January, 2022).
FICCI (2015). India Maize Summit 2015. Available online at: https://ficci.in/events/22310/ISP/Background-paper-India-maize-Summit.pdf (accessed October 17, 2022).
Gairhe, S., Timsina, K. P., Ghimire, Y. N., Lamichhane, J., Subedi, S., and Shrestha, J. (2021). Production and distribution system of maize seed in Nepal. Heliyon. 7, e06775. doi: 10.1016/j.heliyon.e06775
Geberessiliese, N., and Sanders, J. H. (2006). Farm-level adoption of sorghum technologies in Tigray, Ethiopia. Agri. Syst. 91, 122–134. doi: 10.1016/j.agsy.2006.02.002
Gebremedhin, B., Jaleta, M., and Hoekstra, D. (2009). Smallholders, institutional services, and commercial transformation in Ethiopia. Agri. Econ. 40, 773–787. doi: 10.1111/j.1574-0862.2009.00414.x
Herrero, M., and Johnson, R. R. (1980). High temperature stress and pollen viability of maize. Crop Sci. 20, 796–800. doi: 10.2135/cropsci1980.0011183X002000060030x
Kaliba, A. R. M., Verkuijl, H., and Mwangi, W. (2000). Factors affecting adoption of improved maize seeds and use of inorganic fertilizer for maize production in the intermediate and lowland zones of Tanzania. J. Agric. Appl. Econ. 32, 35–47. doi: 10.1017/S1074070800027802
Kandel, B. P. (2021). Status, prospect and problems of hybrid maize (Zea mays L.) in Nepal: a brief review. Genet. Resour. Crop Evol. 68, 1–10. doi: 10.1007/s10722-020-01032-0
Koirala, K. B., Adhikari, B. N., and Tripathi, M. P. (2021). Maize (Zea mays L.) hybrids for Terai ecological belt of Nepal. J. Agri. Res. Adv. 03, 21–28. Available online at: https://jara.org.in/uploads/archivepdf/9519JARA_Vol_03_March_2021_03.pdf
Koirala, K. B., Adhikari, J. B., and Tripathi, M. P. (2020c). On-farm evaluation of hybrid maize in different ecologies of Nepal. Azarian J. Agri. 7, 84–92. doi: 10.52547/azarinj.033
Koirala, K. B., Giri, Y. P., Rijal, T. R., Zaidi, P. H., Sadananda, A. R., and Shrestha, J. (2017). Evaluation of grain yield of heat stress-resilient maize hybrids in Nepal. Int. J. Appl. Sci. Biotechnol. 5, 511–522. doi: 10.3126/ijasbt.v5i4.18774
Koirala, K. B., Gurung, D. B., and Pokharel, B. B. (2002). “A review on hybrid maize research in Nepal,” in Sustainable maize production systems for Nepal: Proceedings of a maize symposium held December 3-5, 2001, Kathmandu, Nepal, Rajbhandari, N. P., Ransom, J. K., Adhikari, K., and Palmer, A. F. E. (eds.). Kathmandu: NARC and CIMMYT. p. 38–45.
Koirala, K. B., Rijal, T. R., Kc, G., Katuwal, R. B., Dhami, N. B., Acharya, R., et al. (2020b). Performance evaluation of maize hybrids under rainfed environments across the middle hills of Nepal. Trop. Agroecosyst. 1, 43–49. doi: 10.26480/taec.01.2020.43.49
Koirala, K. B., Rijal, T. R., Kc, G., Khan, S., Mahato, D. N., Manandhar, S., et al. (2020a). Evaluation of maize hybrids in Terai and inner Terai ecological belt of Nepal. J. Agric. For. Environ. 4, 109–116. doi: 10.3126/jafu.v4i1.47055
Kristjanson, P., Okike, I., Tarawali, S., Singh, B. B., and Manyong, V. M. (2005). Farmers' perceptions of benefits and factors affecting the adoption of improved dual-purpose cowpea in the dry savannas of Nigeria. Agric. Econ. 32, 195–210. doi: 10.1111/j.0169-5150.2005.00338.x
Kulkarni, A. P., Kuchanur, P. H., Satihal, D. G., Zaidi, P. H., and Rahut, D. B. (2022). Stress-resilient maize hybrid adoption factors and impact: evidence from rain-fed agroecologies of Karnataka state, India. Front. Sustain. Food Syst. 6, 909588. doi: 10.3389/fsufs.2022.909588
Larkindale, J., Mishkind, M., and Vierling, E. (2005). “Plant Responses to High Temperature” in: Plant Abiotic Stress, Jenk, M. A., and Hasegawa, P. M. (Oxford, Blackwell). p. 100–144, doi: 10.1002/9780470988503.ch5
Lizaso, J. I., Ruiz-Ramos, M., Rodríguez, L., Gabaldon-Leal, C., Oliveira, J. A., Lorite, I. J., et al. (2018). Impact of high temperatures in maize: phenology and yield components. Field Crops Res. 216, 129–140. doi: 10.1016/j.fcr.2017.11.013
Lobell, D. B., Bänziger, M., Magorokosho, C., and Vivek, B. (2011). Nonlinear heat effects on African maize as evidenced by historical yield trials. Nature Clim. Change. 1, 42–45. doi: 10.1038/nclimate1043
Lobell, D. B., and Burke, M. B. (2010). On the use of statistical models to predict crop yield responses to climate change. Agric. Forest Metero. 150, 1443–1452. doi: 10.1016/j.agrformet.2010.07.008
Lunduka, R. W., Mateva, K. I., Magorokosho, C., and Manjeru, P. (2019). Impact of adoption of drought-tolerant maize varieties on total maize production in southeastern Zimbabwe. Clim. Dev. 11, 35–46. doi: 10.1080/17565529.2017.1372269
Manda, J., Gardebroek, C., Kuntashula, E., and Alene, A. D. (2018). Impact of improved maize varieties on food security in Eastern Zambia: a doubly robust analysis. Rev. Dev. Econ. 22, 1709–1728. doi: 10.1111/rode.12516
McSweeney, C., New, M., and Lizcano, G. (2008). UNDP Climate Change Country Profiles: Nepal. Available online at: http://countryprofiles.geog.ox.ac.uk (accessed on March 20, 2022).
MoALD (2021). Statistical information on Nepalese agriculture 2019/20. Government of Nepal, Ministry of Agricultural Development. Singhadurbar, Kathmandu, Nepal: Planning and Development Cooperation Coordination Division. p. 294.
Monsanto. (2012). Heat and Drought Stress Impacts on Corn; Agronomic Alert. Creve Coeur, MO: Monsanto Technology Development and Agronomy (2012). p. 3.
National Seed Vision (NSV): 2013–2025 (2020). Nepal Seed Sector Overview in the Context of the National Seed Vision, 2013–2025: Status, Implementation Gaps and the Way Forward, Ghimire, T. B., Humagain, R., Thapa, M., Khanal, N., Gautam, S., Choudhary, D., et al. Lalitpur, Nepal: CIMMYT South Asia, Regional Office.
NCVST. (2009). Vulnerability Through the Eyes of Vulnerable: Climate Change Induced Uncertainties and Nepal's Development Predicaments. Kathmandu: Institute for Social and Environmental Transition-Nepal (ISET-N, Kathmandu) and Institute for Social and Environmental Transition (ISET, Boulder, Colorado) for Nepal Climate Vulnerability Study Team (NCVST) Kathmandu.
Nejad, S. M. H., Alizadeh, O., Amiri, B., Barzegari, M., and Bayat, M. E. (2017). The effects of drought and heat stress on some physiological and agronomic characteristics of new hybrids of corn in the north of Khuzestan Province (Iran). Eur. Asian Journal of Bio Sci. 11, 32−36.
NSET (2009). Global Assessment of Risk Nepal Country Report Final. Kathmandu, Nepal: United Nations Development Program (UNDP)—Nepal Pulchowk, Lalitpur, Kathmandu, Nepal.
Paudel, G. P., Krishna, V. V., Rahut, D. B., and McDonald, A. J. (2022). Sustainable intensification under resource constraints: Estimating the heterogeneous effects of hybrid maize adoption in Nepal. J. Crop. Improv. p. 1–26. doi: 10.1080/15427528.2022.2066041
Prasanna, B. M., Cairns, J. E., Zaidi, P. H., Beyene, Y., Makumbi, D., Gowda, M., et al. (2021). Beat the stress: breeding for climate resilience in maize for the tropical rainfed environments. Theoretical Appl. Genet. 134, 1729–1752. doi: 10.1007/s00122-021-03773-7
Ranum, P., Pena-Rosas, J. P., and Garsia-Casal, M. N. (2014). Global maize production, utilization, and consumption. Ann. N. Y. Acad. Sci. 1312:105–112. doi: 10.1111/nyas.12396
Schoper, J. B., Lambert, R. J., and Vasilas, B. L. (1986). Maize pollen viability and ear receptivity underwater and high temperature stress. Crop Sci. 26, 1029–1033. doi: 10.2135/cropsci1986.0011183X002600050038x
Schoper, J. B., Lambert, R. J., and Vasilas, B. L. (1987a). Pollen viability, pollen shedding, and combining ability for tassel heat tolerance in maize. Crop Sci. 27, 27–31. doi: 10.2135/cropsci1987.0011183X002700010007x
Schoper, J. B., Lambert, R. J., Vasilas, B. L., and Westgate, M. E. (1987b). Plant factors controlling seed set in maize: the influence of silk, pollen, and ear-leaf water status and tassel heat treatment at pollination. Plant Physiol. 83, 121–125. doi: 10.1104/pp.83.1.121
Shiferaw, B., Kebede, T.A., and You, L. (2008). Technology adoption under seed access constraints and the economic impacts of improved pigeon pea varieties in Tanzania. Agri. Econ. 39, 309–323. doi: 10.1111/j.1574-0862.2008.00335.x
Shrestha, A. B., Wake, C. P., Mayewski, P. A., and Dibb, J. E. (1999). Maximum temperature trends in the Himalaya and its vicinity: an analysis based on temperature records from Nepal for the period 1971 to 94. J. Climate. 12, 2775–2786.
Simtowea, F., Amondo, E., Marenya, P., Rahut, D., Sonder, K., and Erenstein, O. (2019). Impacts of drought-tolerant maize varieties on productivity, risk, and resource use: evidence from Uganda. Land Use Policy. 88, 104091. doi: 10.1016/j.landusepol.2019.104091
SQCC (2022). Notified and Denotified Crop Varieties in Nepal. Seed Quality Control Centre (SQCC), Ministry of Agricultural and Livestock Development (MoALD), Kathmandu, Nepal.Available online at: https://www.sqcc.gov.np/storage/listies/February2023/Notified%20and%20denotified%20variety%20till%202079-05-30.pdf (accessed November 15, 2022).
SQCC. (2013). National Seed Vision 2013 - 2025 (Seed Sector Development Strategy). Government of Nepal, Ministry of Agricultural Development, National Seed Board, Seed Quality Control Centre, Hariharbhawan, Lalitpur, Nepal. p. 87
Takahashi, K., and Barrett, C. B. (2013). The system of rice intensification and its impacts on household income and child schooling: evidence from rural Indonesia. Amer. J. Agr. Econ. 96, 269–289. doi: 10.1093/ajae/aat086
Thapa, M. (2013). Regulatory framework of GMOs and hybrid seeds in Nepal. Agron J Nepal 3, 128–137. doi: 10.3126/ajn.v3i0.9015
Thayil, V. M., Zaidi, P. H., Seetharam, K., Das, R. R., Viswanadh, S., Ahmed, S., et al. (2020). Genotype-by-environment interaction effects under heat stress in tropical maize. Agronomy. 10, 1998. doi: 10.3390/agronomy10121998
Traore, S. B., Carlson, R. E., Pilcher, C. D., and Rice, M. E. (2000). Bt and non-Bt maize growth and development as affected by temperature and drought stress. Agron. J. 92, 1027–1035. doi: 10.2134/agronj2000.9251027x
Upadhyay, N., Timsina, K. P., Gairhe, S., Sapkota, S., Acharya, Y., and Khadka, S. (2017). “Growth of livestock sector in Nepal: perspective on agricultural perspective plan,” in The Proceeding of 10th National Workshop on Livestock and Fisheries Research in Nepal, Kathmandu, Nepal Held from 5–7 March. p. 364–372.
Walker, J. M. (1969). One degree increments in soil temperatures affect maize seedling behavior. Proc. Soc. Soil Sci. Am. 33, 729–736. doi: 10.2136/sssaj1969.03615995003300050031x
Wooldridge, J. M. (2010). Econometric Analysis of Cross Section and Panel Data (2nd ed.). Cambridge, MA: MIT press.
Wossen, T., Abdoulaye, T., Alene, A., and Feleke, S. (2017). Measuring the impacts of adaptation strategies to drought stress: The case of drought tolerant maize varieties. J. Environ. Manage. 203, 106–113. doi: 10.1016/j.jenvman.2017.06.058
Keywords: heat-stress, heat tolerant maize hybrid, partial budget, IPWRA, yield, Nepal
Citation: Kulkarni AP, Tripathi MP, Gautam D, Koirala KB, Kandel M, Regmi D, Sapkota S and Zaidi PH (2023) Impact of adoption of heat-stress tolerant maize hybrid on yield and profitability: Evidence from Terai region of Nepal. Front. Sustain. Food Syst. 7:1101717. doi: 10.3389/fsufs.2023.1101717
Received: 18 November 2022; Accepted: 13 March 2023;
Published: 03 April 2023.
Edited by:
Stephen Whitfield, University of Leeds, United KingdomReviewed by:
Amadou Sidibé, Agriculture and Agri-Food Canada (AAFC), CanadaCopyright © 2023 Kulkarni, Tripathi, Gautam, Koirala, Kandel, Regmi, Sapkota and Zaidi. This is an open-access article distributed under the terms of the Creative Commons Attribution License (CC BY). The use, distribution or reproduction in other forums is permitted, provided the original author(s) and the copyright owner(s) are credited and that the original publication in this journal is cited, in accordance with accepted academic practice. No use, distribution or reproduction is permitted which does not comply with these terms.
*Correspondence: Atul P. Kulkarni, YS5rdWxrYXJuaUBjZ2lhci5vcmc=
†ORCID: Mahendra P. Tripathi orcid.org/0000-0002-4009-4668
Disclaimer: All claims expressed in this article are solely those of the authors and do not necessarily represent those of their affiliated organizations, or those of the publisher, the editors and the reviewers. Any product that may be evaluated in this article or claim that may be made by its manufacturer is not guaranteed or endorsed by the publisher.
Research integrity at Frontiers
Learn more about the work of our research integrity team to safeguard the quality of each article we publish.