- 1Department of Soil Science, University of Eldoret, Eldoret, Kenya
- 2Department of Seed Crops and Horticultural Sciences, University of Eldoret, Eldoret, Kenya
- 3Department of Agricultural Economics and Rural Development, University of Eldoret, Eldoret, Kenya
Soil erosion threatens the sustainable intensification of food systems among smallholder farmers in arid and semi-arid lands (ASALs). Intensifying adoption of soil mitigation and rehabilitation measures is thus needed urgently in these ASALs, but scaling up these measures depends on scientific evidence of their contributions to key components of sustainable intensification such as soil organic carbon. However, there is no information on how existing mitigation and rehabilitation measures influence soil carbon fractions and carbon management indices in ASALs. This study evaluated the influence of soil erosion mitigation and rehabilitation measures on soil carbon fractions and management indices in Arenic Lixisols of semi-arid environments in West Pokot County, Kenya. We evaluated different vegetation types (maize-beans intercrop and pastures) with and without two locally developed terrace designs for soil conservation (Fanya Juu and Fanya Chini). Combining terracing with annual cropping significantly increased total organic carbon (TOC). The highest TOC (13 g C kg−1) was recorded in pasturelands with terraces while degraded land with no intervention was found to have the lowest TOC (6.0 g C kg−1). Terraced farms with longer residence time (>4 years old) had significantly higher organic carbon than (<4 years old). Other soil properties remained stable with terrace age (1–5 years). Labile SOC and non-labile SOC differed significantly within and across vegetation types with or without terraces (p < 0.05). Pasture and crop systems with terraces had high labile SOC content of 5.9 g C kg−1 and 7.2 g C kg−1, respectively. Labile SOC followed the TOC trend with terrace age, i.e., increasing from 1 year to 5 years old. Combined pasture and terraces had a significantly higher carbon management index (CMI) of 161.7, or 14 times the CMI found in degraded systems with no interventions and 1.5 times the combined crop system with terraces. CMI was also directly correlated with residence time terraces had stayed in the crop system, increasing from 1 year to 5 years old. Contrary to CMI and other indices, the weighted enrichment ratio was found to inversely correlate with age of terrace. Improvement of carbon content and CMI resulted from restorative measures and likely improved soil quality and ecosystem functions. Although terraces play a significant role in the restoration of degraded soils as indicated by the above-mentioned changes, they are most beneficial when used in combination with croplands because of the high level of disturbance and flows of both inputs and outputs of carbon for these croplands.
1. Introduction
Global increases in human populations have increased pressure on natural resources as demand for food, fiber, fuel, pasture, and alternative livelihood intensifies (Ovuka, 2000; Muñoz-Rojas et al., 2020). Most often this induces severe soil erosion, where conservation is not prioritized (Hassen and Bantider, 2020). Soil erosion is more pronounced in sub-humid, semi-arid, and arid environments due to their fragile ecosystems as characterized by low vegetation cover, slow growth rate of vegetation recovering from disturbances, highly erodible soils, and resource-poor smallholder farmers (Valentin et al., 2005; Jinger et al., 2021). Severe soil erosion in these regions compromises their resilience by exacerbating soil, nutrients, and organic carbon losses (Jin et al., 2021), hence accelerating aridification and desertification (Gomez et al., 2003; Avni, 2005).
Soil organic carbon (SOC) plays critical ecosystem functions that control chemical and biophysical processes in the soil (Johnston et al., 2009; Murphy, 2014; Wiesmeier et al., 2019). These functions include: the formation of soil structure, nutrient and water retention, support of soil biodiversity, and control of greenhouse gas emissions (Murphy, 2014; Bradford et al., 2016; Chen et al., 2021). Thus SOC is a key indicator of soil quality (Aravindh et al., 2020; Or et al., 2021), and is vital to sustainable ecosystems. For instance, labile organic carbon fraction, which is a more oxidizable fraction of SOC, has been shown to be sensitive to land management practices (Haynes, 2000; Aravindh et al., 2020), and can be used to assess the occurrence of land degradation or the impact of rehabilitation measures on the restoration (Rui et al., 2011; Ghosh et al., 2016).
With increased soil erosion in the drylands various rehabilitation practices and techniques have been proposed and adopted to restore degraded lands and intensify sustainable food, fodder, fiber, and fuel production (Thondhlana and Muchapondwa, 2014; Muturi, 2016). Some of the prominent land rehabilitation measures embraced by small-holder farmers in the drylands target reduction of surface runoff and protection of topsoil against the raindrop effect (Wolka et al., 2018). This is achieved through terracing and the establishment of vegetation cover such as cover crops and pastures (Mati, 2010; Nyberg et al., 2015; Matere et al., 2016; Saiz et al., 2016; Strassburg et al., 2019).
Adoption and integration of indigenous terraces (Fanya Juu and Fanya Chini) in soil conservation has enabled sustainable farming of high-sloped lands in arid and semi-arid regions (ASALs) of Kenya (Kassie et al., 2009; Wolka et al., 2018), thus making them prominent mechanical soil erosion management practices (SEMPs) (Critchley, 2010; Matere et al., 2016; de Trincheria Gomez et al., 2018). Their contribution to crop yield and soil health is widely recognized, for instance; Saiz et al. (2016) reported that use of Fanya Juu terraces in drylands increases yield by more than 25% and soil organic matter by a third. Fanya Juu and Fanya Chini are often used synergistically and are constructed by digging a ditch along the contours and mounting excavated soil either upslope or downslope, respectively (Mati, 2010; White, 2016; Adimassu et al., 2017) as shown in Figure 2. Besides reducing soil erosion, the dykes accompanying them capture surface runoff and promote slow infiltration after rains (Mati, 2010; Saiz et al., 2016) enabling successful farming in the face of low rainfall.
Despite the benefits associated with integration of Fanya Juu and Fanya Chini terraces in different systems such as cropping and pasture, there exist no documented studies on how these restoration measures contribute to building different soil organic carbon fractions, which are key indicators of restoration progress. Thus, this study sought to evaluate the effect of integrating Fanya Juu and Fanya Chini terraces with different soil vegetation cover practices on soil carbon fractions and carbon management indices in semi-arid environments managed by smallholders, as a key aspect of sustainable intensification of these smallholder systems.
2. Materials and methods
2.1. Description of the study area
The study was undertaken in Chepareria ward of West Pokot County located between 35° 7′ and 35° 27′ latitude and 01°15′ and 01° 55′ longitude. The area is mainly comprised of plains with altitudinal range of 1,100–1,600 m above sea level surrounded by hills and ridges with elevations of up to 3,000 m as shown in Figure 1. The surrounding hills and ridges collect large amounts of surface run-off that descends at high speeds contributing to extreme soil erosions (Mutio et al., 2021). The area experiences a temperature range between 10°C and 30°C and receives an average of 600 mm of annual precipitation (Nyberg et al., 2015). The location is dominated by four major soil types which are; Lixisols, Leptosols, Cambisols, and Luvisols. Lixisols, Leptosols, and Cambisols have weak structures with a high sand content of more than 65% (Schad et al., 2014). The study was conducted on Arenic Lixisols which is the soil type that dominates the study area selected for testing rehabilitation measures and because of its inherent weak properties that make it highly erodible and with visible signs of erosion over substantial areas in the region (Muturi, 2016), as illustrated in Figure 2.
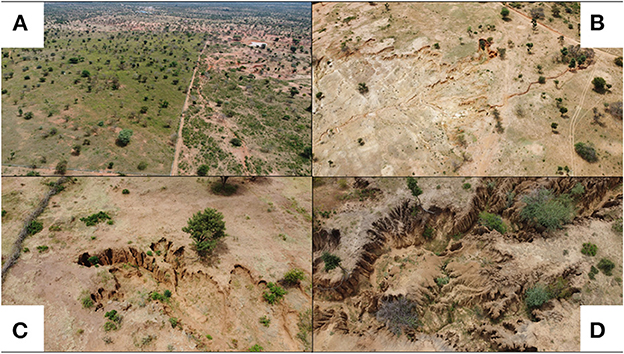
Figure 2. Images showing the high erodibility of Arenic Lixisol soils in the study area. (A) A range land undergoing degradation. (B) A sample section of degraded land with visible rill channels. (C) Growing gullies. (D) Severely degraded sections with deep and wide gullies.
2.2. Selection of land rehabilitation measure
The study scope was limited to two soil vegetation cover practices widely adopted in the area; maize-bean intercrop and pasture farms. A mechanical measure, primarily locally developed terraces (Fanya Juu and Fanya Chini) are integrated into the practices to arrest and capture surface run-off for soil and water conservation. To evaluate additive influence of vegetation cover practices and terraces, three farms with each practice (Maize-bean intercrop + terraces, and pasture + terraces) were selected as treatments. A similar number of the selected vegetation cover without terraces were also included in the sampling. Three replicate farms were selected based on similar soil fertility management practices over 5 years per each soil conservation measures combination, resulting in a total of 12 farms. In addition, three severely degraded rangeland with no management were also selected as a negative comparative treatment, and finally, to evaluate terrace age on SOC fractions in cropping system, soil samples were taken from the maize-bean intercropped farms that had hosted the terraces for five, four, three, two, and one year as shown in Figure 1. For the determination of Carbon Management (CMI, see Methods below) and related indices, a reference samples were obtained from a shrubland that had not been under cultivation of pasture for more than 15 years. Sampling of reference soil samples followed the same technique used in the selected farms.
2.3. Sample design and soil sampling
Soil samples were taken at a depth of 0–30 cm by augering method along three diagonal line transects at 10 m interval resulting in 9 samples per each of the selected farm's horizontal interval (HI). HI is the farmable distance between two terraces as shown in Figure 3, and in each of selected farms had at least three. Collected samples were thoroughly mixed and a composite sample obtained for laboratory analysis. Three intact core samples were also collected for bulk density determination in each of the selected treatment.
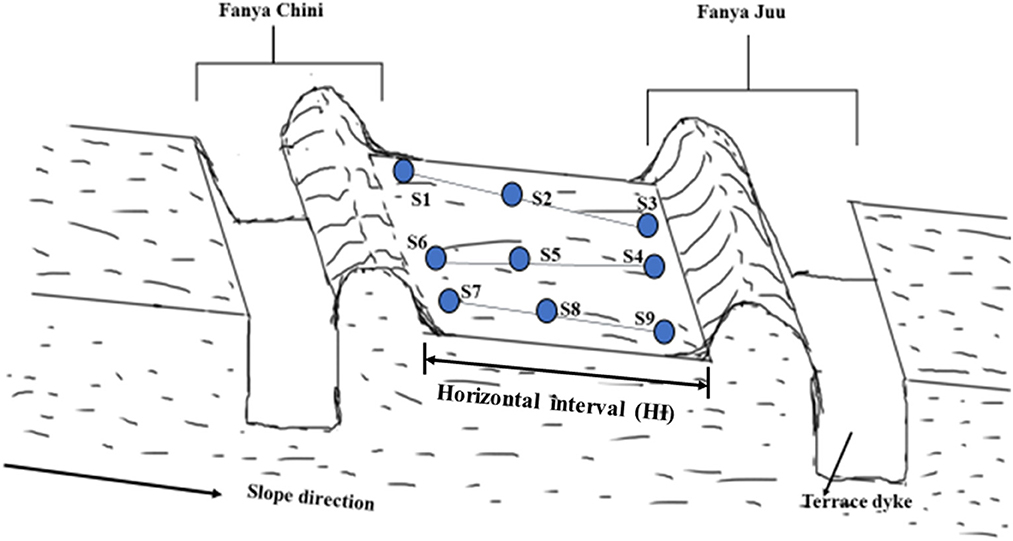
Figure 3. Sampling design in complementary Fanya Chini and Fanya Juu terrace designs. These are terrace designs widely adopted in dry lands of Kenya for soil and water conservation.
2.4. Soil characterization across the selected soil erosion mitigation and rehabilitation measures
Soil texture, pH water (2.5:1), total organic carbon (TOC), total nitrogen, and carbon-to-nitrogen ratio were determined across all the treatments. The soil particle sizes were determined through the hydrometer method (Bouyoucos, 1962), and TOC was determined by the wet ashing method (Walkley and Black, 1934). TOC was converted to soil organic matter by multiplying values by 1.72. Total soil nitrogen (TN) was determined through the Kjeldahl method (Okalebo et al., 2002).
2.5. Physical fractionation and determination of labile and non-labile organic carbon
Granulometric carbon fractions reflecting more stable vs. more labile properties of soil carbon based on particle size were determined using wet sieving (Cambardella and Elliott, 1992; Vieira et al., 2007). Each 20 g sample of air-dried soil was passed through a 2 mm sieve and mixed with 70 ml of sodium hexametaphosphate (HMP) solution. The mixtures were shaken for 16 h on an end-to-end shaker. Mixtures of soil were then passed through 250 μm sieve and then through a 53 μm sieve. Carbon in the fraction passing the 53 μm sieve was defined as non-labile, and that which had a size between 53 μm and 250 μm was defined as labile, in accordance with other research developing rapid assessments of such carbon fractions (Cambardella and Elliott, 1992; Vieira et al., 2007; van Wesemael et al., 2019; Ouédraogo et al., 2020). Both soil fractions were oven-dried at 50°C for 48 h. Fractions were ground using a pestle and mortar, and organic carbon in each fraction was determined by wet ashing method (Walkley and Black, 1934) as per Okalebo et al. (2002).
2.6. Carbon management index
Several CMI related indicators (Vieira et al., 2007) were determined by using an undisturbed shrubland as a reference. This shrubland has been under enclosure for more than 15 years with relatively continuous inputs of organic litter from shrubs and forbs.
2.6.1. Carbon stock
Carbon stocks (CS) for each fraction (TOC, labile SOC, and non-labile SOC) were calculated following Equation 1 while the CMI calculated using Equation 2 as shown below:
Where,
ρb = Soil bulk density (g cm−3).
Depth-soil depth = 0.3 m.
The carbon management index (CMI) was determined according to Equation 2 through 5 following (Vieira et al., 2007).
Where;
A weighted enrichment ratio (WER) was adopted to model the labile SOC change over the years after terrace construction. This was calculated as a ratio to the total organic carbon in the system.
Where; YAC is years after construction indicating the number of years terrace structures have been functional in a farm from the time of their construction to the time of the experiment.
2.7. Statistical analysis
Data were curated in Excel 2016, and Shapiro-Wilk test of normality conducted on each parameter at p = 0.05. Data that were not normally distributed were subjected to log transformation upon which normality was achieved. Linear mixed models were used to compare the SOC fractions and CMI under different conservation measures. One way analysis of variance (ANOVA) was used to analyze SOC fractions, CMI, and weighted enrichment ratio mean comparisons across the selected time intervals of years after terrace construction in maize-bean intercrop. A Tukey's HSD post-hoc test was performed when significant differences between means was detected at p < 0.05. Data analyses were conducted using JMP Version 16.0. All results from transformed variables were back transformed by subjecting them to anti-log before reporting.
3. Results
3.1. Soil physico-chemical properties across selected conservation measures
The soils for the selected management practices were found to have high sand content of more than 70%, hence classified as loamy Sand except for degraded rangeland which was classified as sand clay loam. TOC and TN were high in lands with soil management practices as compared to degraded rangeland without any management practice. pH of the soil was found to slightly acidic with degraded rangeland having the lowest (5.61), as illustrated in Table 1.
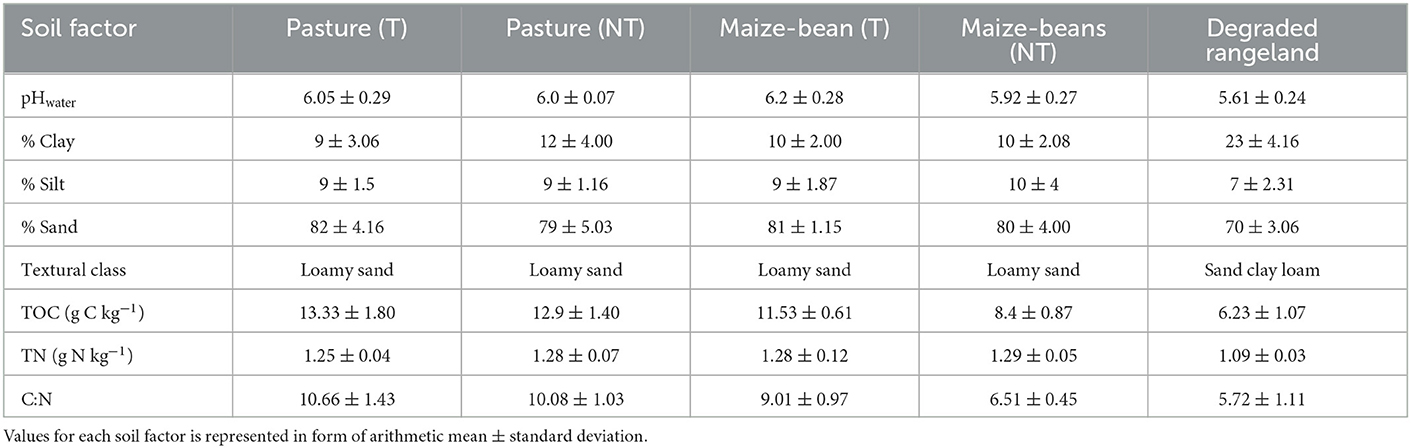
Table 1. Physico-chemical soil properties across selected soil conservation measures (T-with terrace, NT-without terraces).
3.2. Effect of land rehabilitation on carbon fractions
Vegetation cover and terracing had a significant effect on total organic carbon, labile carbon, non-labile carbon, and soil organic matter at p < 0.05 (Figure 4). Pasture significantly increased TOC. On average farms with pasture as a vegetation cover had 13.2 g C kg−1. This was significantly higher than values obtained in degraded land and maize farms of 6.2 and 9.9 g C kg−1, respectively (Figure 4i). Terracing significantly increased TOC by 3.1 g C kg−1 when combined with a maize-bean cover crop. However, it did not significantly increase TOC when combined with pastures as illustrated by Figure 4i. Pastures and maize-bean intercrop were found to significantly improve labile organic fraction by 5.0 and 3.1 g C kg−1, respectively over degraded lands. Similarly, to TOC, terracing significantly increased labile carbon only in croplands by 3.1 g C kg−1 as indicated in Figure 4iii. Non-labile carbon was significantly higher in pastureland than in degraded lands by 2.2 g C kg−1 as shown in Figure 4iv. Unlike in other fractions of carbon, terracing reduced significantly the non-labile carbon fractions in pasturelands by 0.9 g C kg−1.
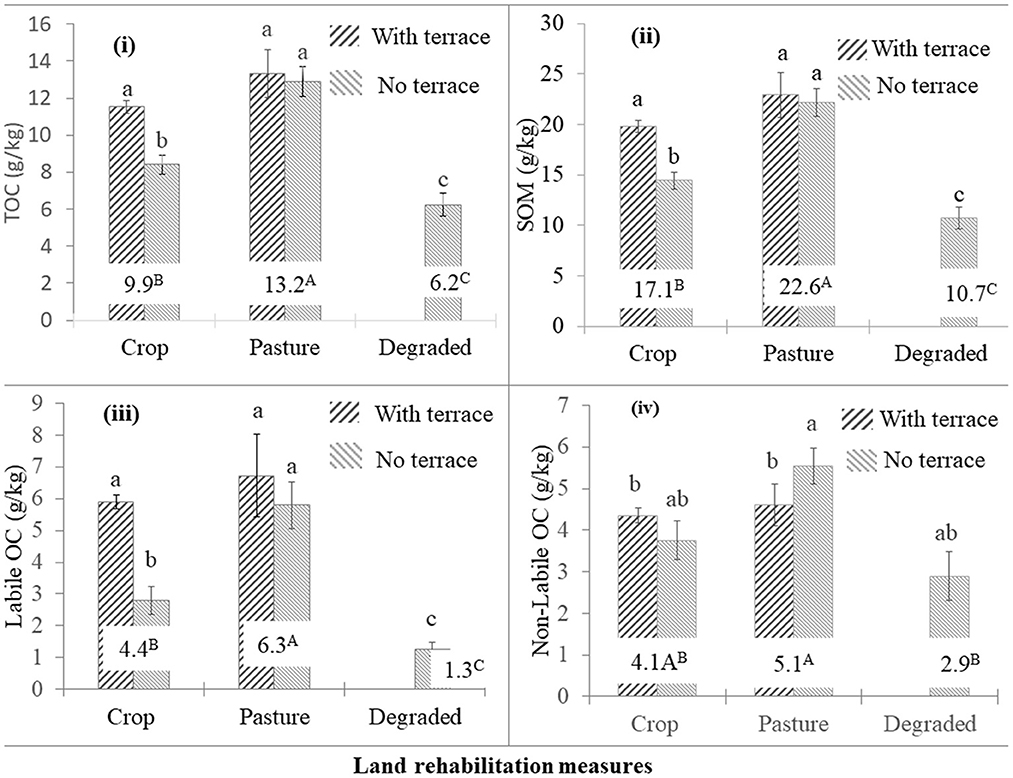
Figure 4. Influence of rehabilitation measures on soil total organic carbon (i), organic matter (ii), labile (iii), and non-labile carbon fractions (iv). Different lower case letters indicate significant difference within the same type of land use between the terraced and un-terraced systems at p = 0.05. Different upper case letters indicate significant difference across different land uses at p = 0.05.
3.3. Effect of age of terraces on carbon fractions
Terrace age had a significant effect on TOC, labile, and non-labile organic carbon fractions. For example, TOC and the labile organic carbon fraction significantly increased over 5 years in terraced maize-bean intercrop with increases of 4.6 and 3.0 g C kg−1, respectively over those aged 1 year. For non-labile organic carbon fraction had a fluctuating trend, peaking at 3YAC and dropping after and henceforth with terraces, aged 1 and 2 years had significantly lower as illustrated by Figure 5.
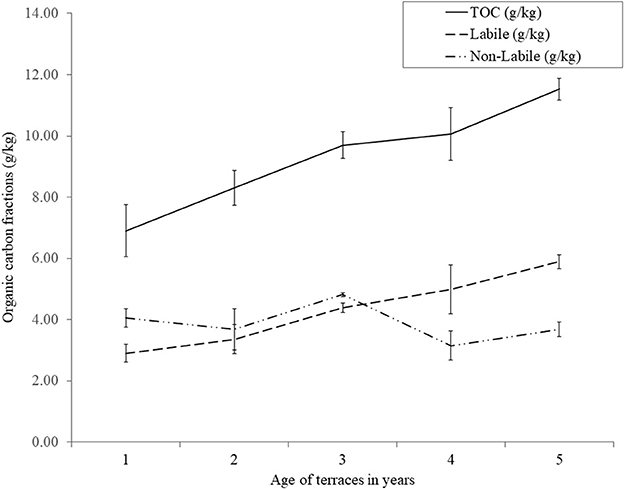
Figure 5. Effect of age of terraces (Fanya Juu and Fanya Chini) on organic carbon fractions in cropping system of maize-bean intercrop. The line shows means trends of each variable while error bars indicate the standard deviation.
3.4. Influence of rehabilitation practices on carbon stock index, lability index, and carbon management index
The introduction of terraces in pastures and croplands significantly increased all test indices vs. non-terraced land and degraded land, particularly in the case of maize-bean intercropping Terracing in pasture and maize-bean intercrops increased LI by 0.48 and 0.31 over the practices without terraces, respectively. In contrast, the introduction of terraces led to strong significant increase in CSI in maize-bean intercropping but not in pastures, while CMI is reversed being only strongly significantly different for pastures. Combining terrace with vegetation practices were found to significantly increase CMI (p < 0.05) compared to both practices without terraces and the degraded land. For instance, terraces improved CMI four and about three times in pasture and maize-beans intercrops, respectively compared to vegetative practices without terraces. Leaving the land degraded without any intervention was found to significantly lower its CMI as illustrated by Table 2.
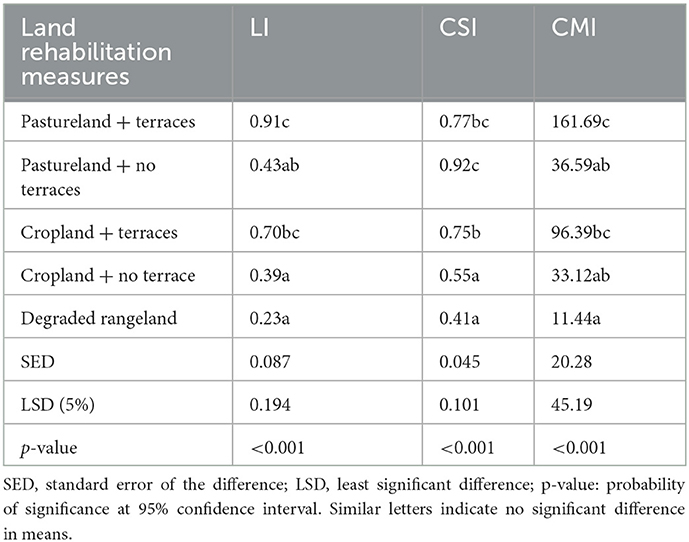
Table 2. Influence of terrace practice on carbon management index (CMI), lability index (LI), and carbon stock index (CSI) across soil conservation practices.
3.5. Effect of age of terraces on carbon stock index and carbon management index
The lability index in croplands with terraces increased from 1YAC to 5YAC although the increase between 4 YAC and 5 YAC was significantly lower than the difference between 4 YAC and other test years. Similar to LI, the Carbon stock index (CSI) steadily increased from 1 YAC to 5 YAC. The carbon management index followed an increasing trend similar to that of LI. The highest CMI in croplands that had hosted terraces for 5 years was found to be more than triple what was recorded in the 1 year after terrace construction. Contrary to trends of all other indices, the weighted enrichment ratio depicted a dropping trend from 1 to 5 YAC. The former had the highest weighted enrichment ratio of 0.42 compared to the lowest 0.10 recorded in 5 YAC farmlands (Table 3).
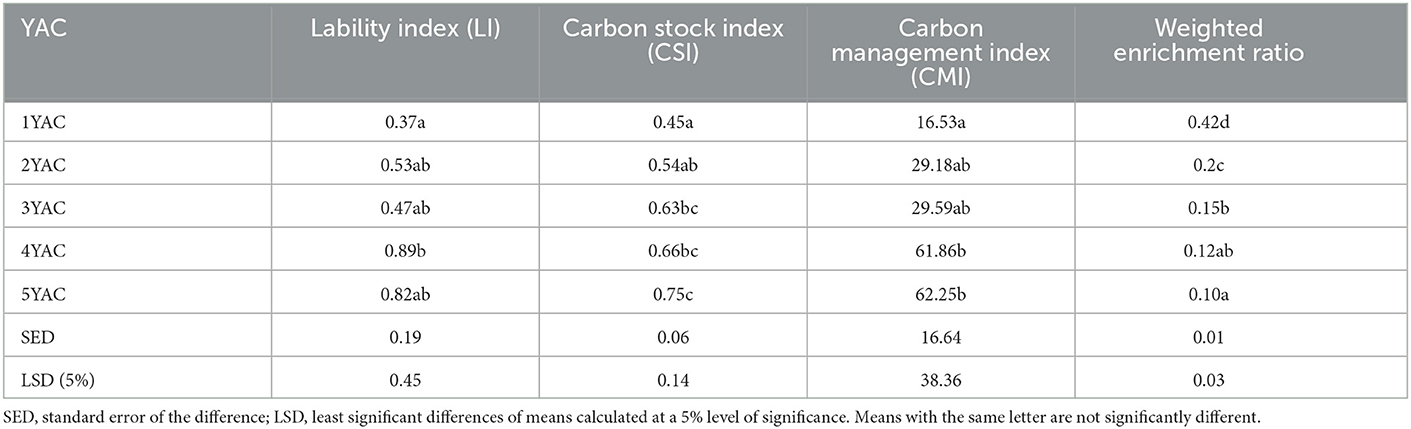
Table 3. Lability index, carbon stock index, carbon management index, and weighted enrichment ratio in cropping system of maize-bean intercrop as influenced by age of terrace (YAC).
4. Discussion
4.1. Soil properties change across vegetation cover practices and years after terrace construction
High sand content of >70% was found in all the soils. This is an inherent characteristic and is the basis on which the soils were sub-classified as Arenic Lixisol (Schad et al., 2014). Contrary to both pasturelands and farmlands, clay content was high in severely degraded rangeland, indicating argic properties of sub-soil as reported in other studies (Schad et al., 2014; Blum et al., 2018). Our study established that erosion in the degraded rangeland was so severe that the A horizon had been partially or wholly washed away. Observed changes in the soil profile instigated by erosion explain the variation of pH among the vegetation cover practices and along YAC. Similar findings were reported by Hailu et al. (2012), who found that the use of soil and erosion management practices (SEMPs) in intervening erosion supervenes the rise of pH. According to the authors, Jones (2003) and Dessalegn et al. (2014), uncontrolled erosion causes the removal of basic cations like magnesium and calcium and leaves behind unbalanced acidic cations, resulting in a decline in pH. Low pH in a degraded system lowers the restoration rates and reduces carbon sequestration (Rengel, 2003; Naorem et al., 2022).
Variation of soil TOC, TN, and C:N across the vegetation cover practices, indicated by the findings were attributive to the benefits of the terraces as a conservation measure, particularly in highly disturbed systems like farmlands. Although, on average, the TOC and TN were low compared to results reported by Sainepo et al. (2018), who found an average of 22.6 g C kg−1 in shrublands. TOC and TN also declined with YAC. The terraced farms with 5 YAC had a comparable high TOC than 1 YAC. The buildup of SOC is interplayed by several factors, including rainfall amount and land use management, as reported by Dessalegn et al. (2014).
4.2. Organic carbon fractions change across vegetation cover practices and YAC
Among the three vegetation cover practices, pasturelands were observed to have a stable distribution of organic carbon fractions. However, the terraced pasturelands, on average, recorded high labile SOC content compared to pasturelands without terraces. The findings are in agreement with the results of Kalambukattu et al. (2013) and Shi et al. (2020), who reported that less disturbed vegetation cover practices have less fluctuations in their SOC than any other system. Like shrublands, pasturelands are relatively less disturbed, and their organic matter source is relatively stable throughout the seasons. In addition, the soils of such systems are less eroded because of the trees, shrubs, grass, and litter, which form a natural mulch. This is consistent with, Jourgholami et al. (2019), who found that an increase in surface litter inversely correlates to surface runoff, hence, reducing erosion. In addition, permanent root systems in pastures hold soil in stable aggregates that tend to promote infiltration and reduce erosion (Gyssels et al., 2005).
Farmlands with terraces significantly surpassed those without terraces with more than twice the labile SOC fraction. Non-labile SOC fraction between farms with and without the two types of indigenous terraces had no significant difference. Labile SOC is the fraction of carbon considered critical to the agriculture system (Zhang et al., 2020), and is also the most affected by either SEMPs or soil erosion. Zhang et al. (2020) indicate further that farming at a slope greater than 10% exacerbates erosion, and increases organic carbon loss, particularly the labile fraction. The complementary effect of Fanya Juu and Fanya Chini significantly reduces soil erosion as reported by Mati (2010) and Saiz et al. (2016), which simultaneously reduce loss of SOC. However, the benefits and increment in SOC accrues over time, which is why we observed an increase in Labile SOC from 1 YAC to 5 YAC.
4.3. Carbon management index
Terraced systems (pasturelands and farmlands) had higher carbon management index (CMI) than those without terraces. The difference in CMI between maize-bean intercrop systems with and without terrace was higher compared to difference found between pasturelands with and without terraces. CMI also increased with YAC, indicating that time is essential for significant increases or maintenance in carbon stocks as influenced by SEMPs, particularly in degraded systems. The results found by this study are highly comparable to past studies in the similar environments, as reported by Sainepo et al. (2018). Their study established a CMI range of 22–100 in different vegetation cover practices. Similarly, CMI values reported by Tang et al. (2018) from rice plantations indicated a range of 90–184. Weighted enrichment ratio decreased with an increase in YAC, indicating that as more organic matter accumulates on the land, carbon stability continues increasing, balancing between the inflow and outflows, as also reported by Chowdhury et al. (2021).
5. Conclusion
Indigenous Fanya Juu and Fanya Chini terraces for soil conservation are more beneficial when used in maize-bean intercropped farmland systems. Terraces are labor-intensive and costly to construct and maintain, particularly on a large scale. However, their benefits greatly increase if they are placed in farming systems. The increase of carbon stocks in terraced farmlands falls on the backdrop of terrace functionality and efficiency. Given that the construction and maintenance of Fanya Juu and Fanya Chini terraces are expensive, placing them in pasturelands may not result in substantial soil benefits that justify this expense. Placement of the terraces in farmlands, particularly in degraded high terrains, is most beneficial and has more significant impacts on both carbon sequestration and carbon management indices.
Data availability statement
The raw data supporting the conclusions of this article will be made available by the authors, without undue reservation.
Author contributions
JM conceived the study, collected the data, analyzed the data, and wrote the first manuscript draft. SK designed data collection and methodology and reviewed the manuscript. RN reviewed and developed the manuscript. HC conceived the study, wrote, and developed the manuscript. WN sourced for research funds, proof reading, and development of manuscript. BA contributed to manuscript proof reading and development of data collection tools. FW contributed to manuscript proof reading. DM contributed in data collection and proof reading of the manuscript. All authors contributed to the article and approved the submitted version.
Funding
The study was funded by the Drylands FRN grant from the McKnight Foundation Collaborative Crop Research Program (CCRP), in collaboration with the University of Eldoret.
Acknowledgments
Authors wish to acknowledge Steven Vanek of Colorado State University, USA, for his invaluable inputs to this paper.
Conflict of interest
The authors declare that the research was conducted in the absence of any commercial or financial relationships that could be construed as a potential conflict of interest.
Publisher's note
All claims expressed in this article are solely those of the authors and do not necessarily represent those of their affiliated organizations, or those of the publisher, the editors and the reviewers. Any product that may be evaluated in this article, or claim that may be made by its manufacturer, is not guaranteed or endorsed by the publisher.
References
Adimassu, Z., Langan, S., Johnston, R., Mekuria, W., and Amede, T. (2017). Impacts of soil and water conservation practices on crop yield, run-off, soil loss and nutrient loss in Ethiopia: review and synthesis. Environ. Manage. 59, 87–101. doi: 10.1007/s00267-016-0776-1
Aravindh, S., Chinnadurai, C., and Balachandar, D. (2020.). Development of a soil biological quality index for soils of semi-arid tropics. Soil 6, 483–497. doi: 10.5194/soil-6-483-2020
Avni, Y. (2005). Gully incision as a key factor in desertification in an arid environment, the Negev highlands, Israel. CATENA 63, 185–220. doi: 10.1016/j.catena.2005.06.004
Blum, W. E. H., Schad, P., and Nortcliff, S. (2018). Essentials of Soil Science Soil Formation, Functions, Use and Classification (World Reference Base, WRB). Stuttgart: Borntraeger Science Publishers.
Bouyoucos, G. J. (1962). Hydrometer method improved for making particle size analyses of soils. Agron. J. 54, 464–465. doi: 10.2134/agronj1962.00021962005400050028x
Bradford, M. A., Wieder, W. R., Bonan, G. B., Fierer, N., Raymond, P. A., and Crowther, T. W. (2016). Managing uncertainty in soil carbon feedbacks to climate change. Nat. Clim. Chang. 6, 751–758. doi: 10.1038/nclimate3071
Cambardella, C. A., and Elliott, E. T. (1992). Particulate soil organic-matter changes across a grassland cultivation sequence. Soil Sci. Soc. Am. J. 56, 777–783. doi: 10.2136/sssaj1992.03615995005600030017x
Chen, L., Fang, K., Wei, B., Qin, S., Feng, X., Hu, T., et al. (2021). Soil carbon persistence governed by plant input and mineral protection at regional and global scales. Ecol. Lett. 24, 1018–1028. doi: 10.1111/ele.13723
Chowdhury, S., Bolan, N., Farrell, M., Sarkar, B., Sarker, J. R., Kirkham, M. B., et al. (2021). “Role of cultural and nutrient management practices in carbon sequestration in agricultural soil,” in Advances in Agronomy (vol. 166, pp. 131–196), ed. D. L. Spark. New York: Academic Press Inc.
Critchley, W. (2010). Climate Change Adaptation technology: Fanya Juu Terraces. In J. Rodgers (Producer). Kenya: IFAD and IIED.
de Trincheria Gomez, J., Dawit, D., Famba, S., Filho, W. L., Malesu, M., Mussera, P. V., et al. (2018). “Using rainwater for off-season small-scale irrigation in arid and semi-arid areas of sub-saharan Africa: key working principles and best practices,” in Smart Agriculture in Arid and Semi-Arid Areas: Fostering the Use of Rainwater for Food Security, Poverty Alleviation, Landscape Restoration and Climate Resilience (pp. 9–36): Springer, Cham.
Dessalegn, D., Beyene, S., Ram, N., Walley, F., and Gala, T. S. (2014). Effects of topography and land use on soil characteristics along the toposequence of Ele watershed in southern Ethiopia. CATENA 115, 47–54. doi: 10.1016/j.catena.2013.11.007
Ghosh, B. N., Meena, V. S., Alam, N. M., Dogra, P., Bhattacharyya, R., Sharma, N. K., et al. (2016). Impact of conservation practices on soil aggregation and the carbon management index after seven years of maize–wheat cropping system in the Indian Himalayas. Agric. Ecosyst. Environ. 216, 247–257. doi: 10.1016/j.agee.2015.09.038
Gomez, B., Banbury, K., Marden, M., Trustrum, N. A., Peacock, D. H., and Hoskin, P. J. (2003). Gully erosion and sediment production: Te Weraroa Stream, New Zealand. Water Resour. Res. 39, 1–7. doi: 10.1029/2002WR001342
Gyssels, G., Poesen, J., Bochet, E., and Li, Y. (2005). Impact of plant roots on the resistance of soils to erosion by water: a review. Prog. Phys. Geogr. 29, 189–217. doi: 10.1191/0309133305pp443ra
Hailu, W., Moges, A., and Yimer, F. (2012). The effects of ‘Fanya juu' soil conservation structure on selected soil physical and chemical properties: the case of Goromti Watershed, Western Ethiopia. Resources and Environment 2, 132–140. doi: 10.5923/j.re.20120204.02
Hassen, G., and Bantider, A. (2020). Assessment of drivers and dynamics of gully erosion in case of Tabota Koromo and Koromo Danshe watersheds, South Central Ethiopia. Geoenviron. Disast. 7, 1–13. doi: 10.1186/s40677-019-0138-4
Haynes, R. J. (2000). Labile organic matter as an indicator of organic matter quality in arable and pastoral soils in New Zealand. Soil Biol. Biochem. 32, 211–219. doi: 10.1016/S0038-0717(99)00148-0
Jin, Q., Peñuelas, J., Sardans, J., Romero, E., Chen, S., Liu, X., et al. (2021). Changes in soil carbon, nitrogen, and phosphorus contents, storages, and stoichiometry during land degradation in jasmine croplands in subtropical China. Exp. Agric. 57, 113–125. doi: 10.1017/S0014479721000089
Jinger, D., Kakade, V., Kaushal, R., Dinesh, D., Bhatnagar, P. R., and Singhal, V. (2021). Rehabilitation of degraded lands through different planting techniques in Melia dubia Cav. Food Sci. Rep. 2, 1–9.
Johnston, A. E., Poulton, P. R., and Coleman, K. (2009). Soil organic matter: its importance in sustainable agriculture and carbon dioxide fluxes. Adv. Agron. 101, 1–57. doi: 10.1016/S0065-2113(08)00801-8
Jones, J. B. (2003). Agronomic Handbook: Management of Crops, Soils, and their Fertility. Boca Raton, FL: CRC Press. p. 450.
Jourgholami, M., Labelle, E. R., and Feghhi, J. (2019). Efficacy of leaf litter mulch to mitigate runoff and sediment yield following mechanized operations in the Hyrcanian mixed forests. J. Soils Sediments 19, 2076–2088. doi: 10.1007/s11368-018-2194-x
Kalambukattu, J. G., Singh, R., Patra, A. K., and Arunkumar, K. (2013). Soil carbon pools and carbon management index under different land use systems in the Central Himalayan region. Acta Agricult. Scand. Sect. B Soil Plant Sci. 63, 200–205. doi: 10.1080/09064710.2012.749940
Kassie, M., Zikhali, P., Manjur, K., and Edwards, S. (2009). Adoption of sustainable agriculture practices: evidence from a semi-arid region of Ethiopia. Nat. Resour. Forum 33, 189–198. doi: 10.1111/j.1477-8947.2009.01224.x
Matere, S. J., Mbatia, L. E., Nzuma, J. M., and Nyamwaro, S. O. (2016). Financial benefitcost analysis of terraces in maize-pigeon pea intercrop in semi-arid areas of Kenya. Agric. Environ. Sci. 5, 140–148.
Mati, B. M. (2010). “Agricultural water management delivers returns on investment in eastern and southern Africa: a regional synthesis,” in Agricultural Water Management Interventions Delivers Returns on Investment in Africa—A Compendium of 18 Case Studies From Six Countries in Eastern and Southern Africa, ed B. Mati (Saarbrücken, Germany: VDM Verlag), p. 1–29.
Muñoz-Rojas, M., Hueso-Gonzalez, P., Branquinho, C., and Baumgartl, T. (2020). Restoration and rehabilitation of degraded land in arid and semiarid environments. Land Degrad. Dev. 2020:1–4. doi: 10.1002/ldr.3640
Murphy, B. W. (2014). Soil Organic Matter and Soil Function—Review of the Literature and Underlying Data. Canberra: Department of Environment.
Mutio J. Njoroge R. Kebeney S. Ng'etich W. Churu H. Alkamoi B. Dynamics of terrace adoption, propagation, benefits & challenges among dryland agro-pastoralist Communities of west pokot. Authorea Preprints. (2021). doi: 10.22541/au.164050501.16730417/v1
Muturi, G. M. (2016). Rehabilitation/Restoration of Degraded Land in Kenya. Paper presented at the Great Green Wall and Drylands Restoration in Eastern Africa, Nairobi, Kenya.
Naorem, A., Jayaraman, S., Dalal, R. C., Patra, A., Rao, C. S., and Lal, R. (2022). Soil inorganic carbon as a potential sink in carbon storage in dryland soils—a review. Agriculture 12, 1256. doi: 10.3390/agriculture12081256
Nyberg, G., Knutsson, P., Ostwald, M., Öborn, I., Wredle, E., Otieno, D. J., et al. (2015). Enclosures in West Pokot, Kenya: transforming land, livestock and livelihoods in drylands. Pastoralism 5, 25. doi: 10.1186/s13570-015-0044-7
Okalebo, J. R., Gathua, K. W., and Woomer, P. L. (2002). Laboratory Methods of Soil and Plant Analysis: A Working Manual (Second Edition ed.). Nairobi, Kenya: TSBF-CIAT and SACRED Africa.
Or, D., Keller, T., and Schlesinger, W. H. (2021). Natural and managed soil structure: on the fragile scaffolding for soil functioning. Soil Tillage Res. 208, 1–9. doi: 10.1016/j.still.2020.104912
Ouédraogo, R. A., Chartin, C., Kambiré, F. C., van Wesemael, B., Delvaux, B., Milogo, H., et al. (2020). Short and long-term impact of urban gardening on soil organic carbon fractions in Lixisols (Burkina Faso). Geoderma 362, 1–11. doi: 10.1016/j.geoderma.2019.114110
Ovuka, M. (2000). More people, more erosion? Land use, soil erosion and soil productivity in Muranga District, Kenya. Land Degrad. Dev. 11, 111–124. doi: 10.1002/(SICI)1099-145X(200003/04)11:2<111::AID-LDR371>3.0.CO;2-I
Rui, Y., Wang, S., Xu, Z., Wang, Y., Chen, C., Zhou, X., et al. (2011). Warming and grazing afect soil labile carbon and nitrogen pools diferently in an alpine meadow of the Qinghai-Tibet Plateau in China. J. Soils Sediments 11, 903–914. doi: 10.1007/s11368-011-0388-6
Sainepo, B. M., Gachene, C. K., and Karuma, A. (2018). Assessment of soil organic carbon fractions and carbon management index under different land use types in Olesharo Catchment, Narok County, Kenya. Carbon Balance Manage 13, 1–9. doi: 10.1186/s13021-018-0091-7
Saiz, G., Wandera, F. M., Pelster, D. E., Ngetich, W., Okalebo, J. R., Rufino, M. C., et al. (2016). Long-term assessment of soil and water conservation measures (Fanya-juu terraces) on soil organic matter in South Eastern Kenya. Geoderma 274, 1–9. doi: 10.1016/j.geoderma.2016.03.022
Schad, P., van Huyssteen, C., and Michéli, E. (2014). World Reference Base for Soil Resources 2014: International Soil Classification System for Naming Soils and Creating Legends for Soil Maps. Rome: Food And Agriculture Organization Of The United Nations.
Shi, P., Zhang, Y., Zhang, Y., Yu, Y., Li, P., Li, Z., et al. (2020). Land-use types and slope topography affect the soil labile carbon fractions in the Loess hilly-gully area of Shaanxi, China. Arch. Agron. Soil Sci. 66, 638–650. doi: 10.1080/03650340.2019.1630824
Strassburg, B., Beyer, H., Crouzeilles, R., Iribarrem, A., Barros, F., de Siqueira, M., et al. (2019). Strategic approaches to restoring ecosystems can triple conservation gains and halve costs. Nat. Ecol. Evol. 3, 62–70. doi: 10.1038/s41559-018-0743-8
Tang, H., Xiao, X., Tang, W., Li, C., Wang, K., Li, W., et al. (2018). Long-term effects of NPK fertilizers and organic manures on soil organic carbon and carbon management index under a double-cropping rice system in Southern China. Commun. Soil Sci. Plant Anal. 49, 1976–1989. doi: 10.1080/00103624.2018.1492600
Thondhlana, G., and Muchapondwa, E. (2014). Dependence on environmental resources and implications for household welfare: evidence from the Kalahari drylands, South Africa. Ecol. Econ. 108, 59–67. doi: 10.1016/j.ecolecon.2014.10.003
Valentin, C., Poesen, J., and Li, Y. (2005). Gully erosion: impacts, factors and control. CATENA 63, 132–153. doi: 10.1016/j.catena.2005.06.001
van Wesemael, B., Chartin, C., Wiesmeier, M., von Lützow, M., Hobley, E., Carnol, M., et al. (2019). An indicator for organic matter dynamics in temperate agricultural soils. Agric. Ecosyst. Environ. 274, 62–75. doi: 10.1016/j.agee.2019.01.005
Vieira, F. C. B., Bayer, C., Zanatta, J. A., Dieckow, J., Mielniczuk, J., and He, Z. L. (2007). Carbon management index based on physical fractionation of soil organic matter in an Acrisol under long-term no-till cropping systems. Soil Till. Res. 96, 195–204. doi: 10.1016/j.still.2007.06.007
Walkley, A., and Black, I. A. (1934). An examination of the degtjareff method for determining soil organic matter, and a proposed modification of the chromic acid titration method. Soil Sci. 37, 29–38. doi: 10.1097/00010694-193401000-00003
White, J. (2016). Terracing Practice Increases Food Security and Mitigates Climate Change in East Africa. Available online at: https://ccafs.cgiar.org/news/terracing-practice-increases-food-security-and-mitigates-climate-change-east-africa (accessed October 14, 2022).
Wiesmeier, M., Urbanski, L., Hobley, E., Lang, B., von Lützow, M., Marin-Spiotta, E., et al. (2019). Soil organic carbon storage as a key function of soils—a review of drivers and indicators at various scales. Geoderma 333, 149–162. doi: 10.1016/j.geoderma.2018.07.026
Wolka, K., Mulder, J., and Biazin, B. (2018). Effects of soil and water conservation techniques on crop yield, runoff and soil loss in sub-saharan africa: a review. Agric. Water Manage. 207, 67–79. doi: 10.1016/j.agwat.2018.05.016
Keywords: soil carbon fractions, degraded land, terraces, total organic carbon, carbon management index
Citation: Mutio JM, Kebeney S, Njoroge R, Churu H, Ng'etich W, Mugaa D, Alkamoi B and Wamalwa F (2023) Effect of land rehabilitation measures on soil organic carbon fractions in semi-arid environment. Front. Sustain. Food Syst. 7:1095865. doi: 10.3389/fsufs.2023.1095865
Received: 11 November 2022; Accepted: 23 January 2023;
Published: 15 February 2023.
Edited by:
Robert Hunter Manson, Instituto de Ecología (INECOL), MexicoReviewed by:
Maria Martínez-Mena, Center for Edaphology and Applied Biology of Segura (CSIC), SpainLilit Pogosyan, Lomonosov Moscow State University, Russia
Copyright © 2023 Mutio, Kebeney, Njoroge, Churu, Ng'etich, Mugaa, Alkamoi and Wamalwa. This is an open-access article distributed under the terms of the Creative Commons Attribution License (CC BY). The use, distribution or reproduction in other forums is permitted, provided the original author(s) and the copyright owner(s) are credited and that the original publication in this journal is cited, in accordance with accepted academic practice. No use, distribution or reproduction is permitted which does not comply with these terms.
*Correspondence: James Mumo Mutio, mumomutio@gmail.com