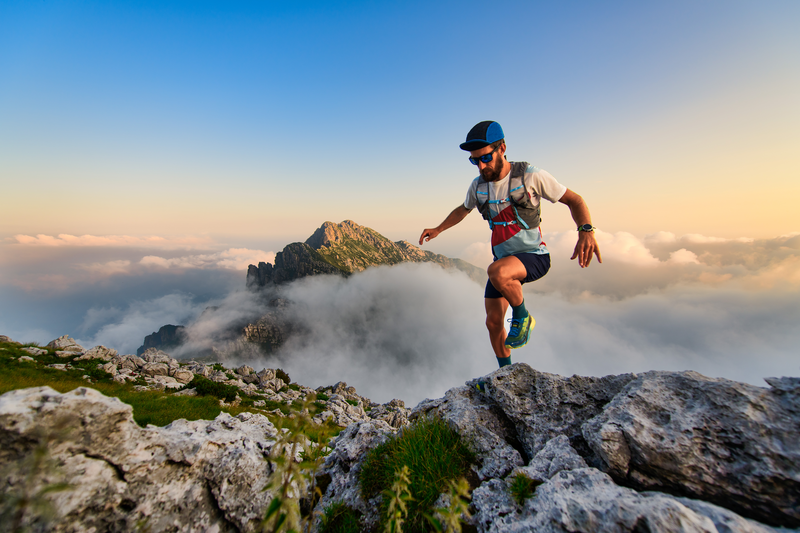
94% of researchers rate our articles as excellent or good
Learn more about the work of our research integrity team to safeguard the quality of each article we publish.
Find out more
ORIGINAL RESEARCH article
Front. Sustain. Food Syst. , 31 July 2023
Sec. Land, Livelihoods and Food Security
Volume 7 - 2023 | https://doi.org/10.3389/fsufs.2023.1094290
This article is part of the Research Topic Sustainable Intensification of Smallholder Farming Systems in Sub-Saharan Africa and South Asia View all 16 articles
In sub-Saharan Africa (SSA), food security is a significant challenge due to unreliable rainfall and depleting soil fertility. Most of the soil resource in the sub-region which constitutes majority of the fields of smallholder farmers is degraded. Hence, there is a need to identify suitable soils for sustainable intensification. The objectives of this study were to: (i) evaluate the suitability and fertility constraints of soils and (ii) discuss the influence of soil properties on maize production in the Nkoranza (north and south) district. A total of sixty (60) soil samples were sampled from smallholder farms under careful consideration of topography and the spatial pattern of land use systems. The evaluation of soil suitability was carried out using climate (temperature and rainfall) and physico-chemical characteristics of soils for maize (Zea mays) production. The results indicated that soil texture varied from sandy loam to sandy clay loam. Soil organic carbon concentration (SOC) ranged between 0.55 and 2.02%. Total nitrogen (TN) and SOC were low in all soil types except in the Bediesi series (Haplic Luvisol). Base cations (Ca2+, Mg2+, Na+, and K+) were low and varied between soil types. Although climatic factors and physical properties were highly suitable (S1), more than half of the pedons were moderately suitable (S2). The soils functioned at a moderate capacity for maize production. The major limitations identified were sub-optimal and related to soil fertility (CEC). Pearson correlation revealed a relationship between parametric actual index (PAI) and parametric potential index (PPI; r = 0.940, p < 0.003) and between soil resilience index (SRI; r = 0.768, p < 0.037) and the relationship between these variables is a perfect correlation. Soil management is required to increase maize yield in the study area. Soil erosion prevention measures such as cover crops, mulching, organic manure (poultry), and mineral fertilizer application are recommended to improve soil fertility in the Nkoranza (north and south) district. This study can inform policies and interventions geared toward sustainable agricultural intensification. Land and soil are heterogeneous and any decision on intensification in this study accounted for the prevailing local conditions of the study area. Therefore, indexing soil suitability using climate (rainfall and temperature), physical land characteristics (topography, drainage) and chemical properties (pH, SOM, SOC, TN, Av. P, Av. K, etc.) of soil resources for sustainable intensification of maize is proposed for smallholder farming communities of Nkoranza (north and south) districts in the Forest-Savannah Transition Zone of Ghana.
Agriculture is the backbone of African countries south of the Sahara Desert and employs more than 60% of its population (AGRA, 2014). Intensification of crop production, which is mainly based on rainfall has not matched population growth (Pradhan et al., 2015; Giller et al., 2021). Yields have stagnated over the past half century [Food and Agriculture Organization (FAO) of the United Nations, 2020a] rendering small farm owners financially poor to afford recommended inputs. According to Food and Agriculture Organization (FAO) of the United Nations (2020b), cereal production is low with an average yield of 1.6 t ha−1 compared to the global average of 3.9 t ha−1. Also, the per capita income deficit for cereals increased from 1.5 million tons in 1967 to more than 20 million tons in 2015 (Sanchez, 2002; van Ittersum et al., 2016). These projections indicate that the sub-region requires about 35 million tons of cereal imports to satisfy the demands of the growing population [Food and Agriculture Organization (FAO) of the United Nations, 2015].
Sustainable supply of food, feed and fiber to a growing world population estimated at more than 9 billion by 2050 is a global concern (Foley et al., 2011; van Bussel et al., 2015). Therefore, the conservation of natural resources require an increase in yield on each hectare (Iizumi et al., 2018) of arable land suitable for intensification. Thus, increasing food production on existing farmlands is an important component of the sustainable intensification (SI) concept. Hence, the need to develop a robust indexing method to identify soils suitable for sustainable intensification (Claessens et al., 2013; Panel, 2013). This means intensifying food production and ensuring that the natural resources that sustain and improve agriculture for future generations are maintained and improved (Pretty and Bharucha, 2014). Sustainable intensification of agriculture paradigm was introduced by the British Royal Society of London (BRSL) in 2009 (Baulcombe et al., 2009). The BRSL defined SI as an agricultural production system that increases yield without adverse environmental impacts.
Maize importation is not economically feasible to eradicate food shortages. Sustainably intensifying the area under cultivation can increase domestic food production and this forms the basis for achieving Sustainable Development Goals #1 (zero poverty), #2 (zero hunger), #3 (enhance good health and wellbeing), #12 (responsible contribution and production), #13 (mitigate climate change), and #15 (sustenance of life on land, reduce soil degradation, and support biodiversity) (United Nations, 2016). Maize production is mostly subsistence, and most smallholder farmers use little or no soil amendments (Fening et al., 2005, 2009; Bationo et al., 2018). Nutrient depletion ranges from 40 to 60 kg of nitrogen (N), potassium (P) and phosphorous (K) ha yr−1 (AQUASTAT and FAO, 2005; Bationo et al., 2018), are among the highest in SSA. This is due to a decline in soil fertility as a result of continuous cropping without replenishment (Sanchez et al., 1997; Sanchez, 2002), as well as low and erratic rainfall are among biophysical constraints (International Center for Soil Fertility and Agricultural Development, 2007).
However, low maize yield is further attributed to the inability of farmers to apply even the blanket fertilizer recommendation [Ministry of Food and Agriculture (MoFA), 2011]. Also, the soil in the study area is depleted and does not meet the food demand of the growing population via area expansion-based crop production by small farm holders (Awoonor et al., 2021). Therefore, sustainable intensification is the only way for farmers to access high quality and affordable fertilizers that increase yield and profit for farmers in the rural communities. Also, intercropping is a form of a sustainable intensification cropping system that uses mutually beneficial ecological relationships that arise when two or more crops are cultivated, either as mixtures or in rotations (e.g., mixed cropping, rotations etc.). The low productivity from small farm holdings is due to biophysical factors related to declining soil fertility, pest and diseases, limited use of external input, unfavorable government policies, markets, institutional arrangements, as well as the effects of climate change in recent years. Much effort must be incorporated into soil management to ensure agricultural sustainability (Asiamah, 2008; Gelaw et al., 2015; Vanlauwe et al., 2015).
Farming activities are semi-intensive due to the peculiar nature of the native vegetation. The sustainability of an agricultural production system measures how the qualities of a land unit match the requirement of a particular form of land use [Food and Agriculture Organization (FAO) of the United Nations, 1976, 1985]. Some farming practices are not sustainable (Stoorvogel et al., 1993; Nandwa, 2001). These have resulted in environmental consequences, such as frequent changes in temperature, potential evapotranspiration, and changes in rainfall patterns with a pronounced decline throughout the transition zone. These challenges, if not addressed, will further reduce agricultural productivity, which adversely affects food security and rural livelihoods (Lal, 2006; Frelat et al., 2015). These challenges resulted in a relatively low annual yield despite the high potential for improvement. In spite of these challenges, an increase in organic matter can improve soil nutrients retention and release, improve soil water holding capacity, as well as control and reduce soil erosion on small farmlands. Several authors (Mbagwu et al., 1984; Lal, 1987, 1997) stressed that at the field and plot level, soil erosion can cause a yield reduction of about 30-90% in some shallow root-restrictive soils in the tropics and subtropics.
Land suitability assessment is conducted to manage soil resources sustainably to determine which type of soil resource is most suitable for a particular crop (Jones et al., 2013). The evaluation of land suitability involves identification and measurement of land quality and its assessment for alternative uses. The principles of land evaluation involve comparing the quality of land with the requirements of specific crops (Ranst et al., 1996; Ande, 2011). It involves the limiting factors of a particular crop for production [Food and Agriculture Organization (FAO) of the United Nations, 1976; Young, 1980]. The goal of this evaluation process is to increase productivity of soils for the specified crop. Crop suitability is a measure of the climatic and other biological properties of an area that sustains the production cycle of crops in order to meet current or expected targets. The farmer's challenge is often associated with or dependent on the suitability of soils for an intended purpose. The mismatch of crops with land use requirements has not ensured a reliable supply of food produced in the FSTZ. Therefore, soil suitability assessment identifies areas where adaptation measures are generally required to prevent the consequences of the climatic suitability of a crop.
In Ghana, maize is grown on small holdings by low-income farmers in rural communities. Hence, the need to evaluate the suitability of soils to increase maize production without depleting soil fertility. Climate and soil are the main environmental factors that determine crop yield. Furthermore, decreased soil fertility, increased soil erosion, erratic rainfall, emerging diseases, insect and pest infestations, and poor management of natural resources and land use have exposed the Nkoranza district (north and south) to food and nutrition insecurity. Additionally, land is becoming a scarce resource due to population growth. The associated economic pressures have exerted pressure on the limited natural resources available, resulting in a reduction in agricultural productivity per hectare.
According to Ogunkunle (1993, 2016) and Kihoro et al. (2013), the need to evaluate farmlands is because the soil classification system, with its maps and accompanying legends, does not meet the current needs and aspirations of most smallholder farmers and other land users. The impact of mismanagement of land resources and the lack of land utilization regarding land's potential suitability remain a challenge in developing countries such as Ghana. A more comprehensive land suitability evaluation for maize production was adopted, as suggested by Sys (1985). The need to identify the suitability of areas suitable for specific or selected crops improves the productivity and resilience of smallholder farms (Debesa et al., 2020).
Furthermore, limited attention has been paid to soil suitability assessment impacts on smallholder agricultural options for diversification of maize production systems in the study area. To the best of our knowledge, there are few publications that assess crop suitability at the national or local level to guide smallholder farmers select the most suitable crop for their farming communities to build resilience (Janzen et al., 2011). The adoption of appropriate land use management based on technological input enhance soil resilience. The use of proven scientific inputs (e.g., ISFM) results in a synergistic and positive effect on inherent soil properties, terrain, landscape and climatic factors (Lal, 1997). Again, the current food crisis after the COVID-19 pandemic has resulted in increased food prices. Hence, the need to feed an increasing population of 30 million in a changing world climate is a major challenge facing the government of Ghana.
Therefore, there is the need to identify suitable soils for sustainable intensification. The term suitable in this study refers to soils that support resource-efficient and cost-effective responses to agronomic interventions without physical, chemical, and biological degradation when subjected to intensification (Claessens et al., 2013). Intensification is producing more unit of output per units of all inputs and through new combinations of inputs and related innovations (Panel, 2013). Also, sustainable intensification involves improving the physical input-output relations to increase the overall efficiency of production. Thus, maize production on existing small-holder farms forms an important component of the sustainable intensification paradigm indicated by Claessens et al. (2013). This study assessed the importance of climate (temperature and rainfall) and soil (physical and chemical) properties on the suitability of land for maize production in smallholder farms in the study area. The main objectives were to assess the morphological, physical, and chemical properties of soils and their suitability for growing maize on sustainable bases in the Nkoranza (north and south) district of Ghana.
The study area falls within Ghana's Forest-Savannah Transition Zone (FSTZ). The geographic location lies between longitude 1° 10′ & 1° 55′ West and latitude 7° 20′ & 7° 55′ North, covering ~2,592.09 km2 (see Figure 1).
Soil moisture and temperature regime of Nkoranza's north and south districts is udic and hyperthermic (Wambeke, 1974). According to the Koppen-Geiger climate classification, the area experiences the tropical savannah climatic regime (Aw) (Peel et al., 2007). The study area has distinct climates (McSweeney et al., 2010). Annual rainfall, although high, varies between 1,200 and 1,600 mm year−1 (Table 1). Temperatures are high throughout the year (Kasei, 1993; AQUASTAT and FAO, 2005), with a mean monthly temperature range between 24 and 30°C (Dickson and Benneh, 1995). Annual potential evaporation is about 1400 mm and relative humidity varies from 90 to 95% in the rainy season to 75 to 80% in the dry season (Christiansen and Awadzi, 2008). The study area is a major producer of maize (Dickson and Benneh, 1995).
The FSTZ is characterized by deep, well-drained soils with sandy clay loam texture, strongly weathered, highly leached and acidic (pH: 5.1–6.5) (Adjei-Gyapong and Asiamah, 2002). These soils are easily eroded once the forest or savannah vegetation has been removed [Food and Agriculture Organization (FAO) of the United Nations, 2015, 2020a]. The soils contain a small amount of plant-available nutrients, particularly nitrogen (N), phosphorus (P), and potassium (K), with low cation exchange capacity (<10 cmol kg−1). Drainage is related to topography. Poorly drained, flood-prone clay to loamy sand textured gleysols are found in valley bottoms (Annan-Afful et al., 2004, 2005; Owusu-Bennoah et al., 2008). Six (6) soil types (Table 1), namely: Kpelesawgu (Dystric Plinthosol), Changnalili (Gleyic Plinthosol), Damongo (Rhodic Luvisol), Murugu (Haplic Luvisol), Bediesi (Dystric Nitisol), and Sutawa (Thapto-Plinthic Luvisol) was identified and classified using the Ghana Interim Soil Classification System (GISCS) (Phillips and Wills, 1963; Adjei-Gyapong and Asiamah, 2002). The soils were reclassified using the FAO/WRB classification [IUSS Working Group (WRB), 2015]. Most of these soils were developed from hydrological conditions along slopes. These geomorphological processes resulted in different soils from uplands (north-west and south-west) to lowlands (north-east and south-east sections of the Nkoranza district), resulting in various formations of soil associations. Bediesi-Sutawa association was associated with upland soils, Damongo-Murugu was attributed to middle slope soils, while Kpelesawgu-changnalili association were ascribed to lowland soils (Adu and Mensah-Ansah, 1995; Agyili, 2003) Soils of the district are generally deep on the upper, middle, and lower slopes.
A systematic stratified sampling procedure was used to distribute sampling points throughout the study area under careful consideration of topography and the spatial pattern of smallholder land use systems. For morphological description of benchmark soils, soil pits (2 m L × 1.5 m W × 1 m D) were dug and described according to the recommendations of IUSS Working Group (WRB) (2022). Sixty (60) soil samples were collected at a depth of 0 – 20 cm, air-dried and passed through a 2-mm sieve. Soil color was determined using the Munsell Color Chart. Soil particle size (sand, silt, and clay) was determined with standard Bouyoucos hydrometer method (Bouyoucos, 1962). Bulk density (BD g cm−3) was determined using the core method (Blake and Hartage, 1986). The soil reaction (pH) and electrical conductivity (EC) were measured in distilled water in a soil: water ratio of 1:2.5 w/v soil/water suspension (Olsen et al., 1982; Thomas et al., 1996; Rhoades, 2018). Total nitrogen (TN) was determined using the Kjeldahl method (Soil Survey Staff, 2006), available phosphorus (Av. P) determined colorimetrically after extraction with Bray's No.1 solution (Bray and Kurtz, 1945), available potassium (Av. K) by flame photometry (Motsara, 2015), and cation exchange capacity (CEC) was determined by ethylenediamine tetraacetic acid (EDTA) titrimetric method after extraction with 1 M ammonium acetate solution buffered at pH 7 (Thomas, 1983). Soil organic carbon (SOC) was determined with the Walkley and Black wet combustion method as described by Jackson (1973). Base saturation (BS) was determined as the ratio of basic cations in CEC.
In terms of agriculture, land suitability evaluates land to meet the agro-ecological requirements of a given crop to increase yield (Kawy and El-Magd, 2012). Land suitability assessment assesses relevant land characteristics such as soil, climate, and topography [Food and Agriculture Organization (FAO) of the United Nations, 1976; Young, 1980] to the requirements of a particular crop. These were regrouped into three thematic indicators. These indicators, as adopted from Baroudy (2016), and Diallo et al. (2016) are soil fertility, chemical, and physical quality indices (Equation 1):
The above equation was used to calculate land suitability for maize in the study area. From equation 1, LS is the land suitability factor, FQI is the soil fertility quality index, CQI is the soil chemical quality index, and PQI is the soil physical quality index. Soil fertility index (Equation 2) was calculated as:
where SSOC, STN, SAv.P, SK, and SCEC are parameters that express factors for soil organic carbon, total nitrogen, phosphorus, potassium, and cation exchange capacity content. The chemical quality index was calculated using Equation 3;
where SNa, SpH, and SEC are parameters that express factors for sodium, soil pH, and electrical conductivity. The physical quality index was calculated using Equation 4
where St, Ss, Scf, Sd, Sw, and Sg are parameters that express factors for texture, structure, coarse fragment, depth, drainage, and slope (gradient). The parameters or factors for the evaluation process were rated (Sys, 1985; Sys et al., 1993). For this evaluation exercise, rates were assigned to the elements of each particular parameter with valid scores ranging from 0, the worst condition, to 100, rated as the best condition (Baroudy, 2016). For each class, a weighted index score was calculated according to the importance of its role in the land evaluation process. The suitability ratings were as follows: S1-highly suitable, S2-moderately suitable, S3-marginally suitable, and, N-unsuitable. The values of the final results were compared with two parametric methods, the square root and the Storie method (Storie et al., 1976).
For the non-parametric approach, soils were placed in suitability classes by matching these climatic characteristics (rainfall, temperature, humidity) with the agronomic requirements of the maize crop (chemical elements: SOC, TN, SOM, Av. P, Ex. K, etc.). An optimal soil property value for a crop must have no limitation. However, if the attribute is unfavorable to the crop, there is a limitation. These limitations range from 0 (no limitation) to 4 (very severe limitation). A value of 100 is assigned when the characteristics of the soil are optimal for the intended use. However, if the characteristic is not helpful, a low value is assigned, reflecting the degree of limitation for climate (c), topography (t), soil water conditions (w), soil physical characteristics (s) and soil chemical characteristics (f). The product of these parametric values gives the soil suitability index as described in Food and Agriculture Organization (FAO) of the United Nations (1976), Ogunkunle (1993). The Storie method was used in the calculation of the land index (I) (equation 5):
where I is the suitability index. A is the rating of the surface texture parameter, and B, C, and D are the rating values for other parameters. A score ranging from 0 to 100% was determined for each factor and the scores were multiplied to generate an index rating (Storie et al., 1976). The square root method uses the formula (Equation 6):
where I is the square root index, Rmin is the minimum rating, and A, B, C, and D are the remaining rating values (Khiddir, 1986).
The rating of each criterion is derived from field observation and laboratory analysis of each land characteristic, and the comparison of these measures with a specific crop (maize) requirement (Table 2). After rating the measurements with threshold values, a rating of 0–100 is assigned to each criterion. Also, a score of the land unit is given a rate of 0–100 by calculation as described by the three methods discussed above. The method of maximum limitation involves the selection of the most restricting rating and/or considering it as the total score for a land unit. Soil series were first classified for their suitability by matching their characteristics with the FAO requirements in Table 2. The suitability class of the soil was indicated by its most limiting characteristics. For the parametric method, each limiting characteristic was rated.
Table 2. Land requirements suitability classes for maize (Sys et al., 1993).
Furthermore, the productivity index for each soil series (pedon) was calculated using Equation 1 above. In all, five (5) land quality factors, thus climate (c), topography (t), soil physical properties (s), wetness (w), and fertility (f), were used for the assessment. Only a member of each group was used to calculate the overall suitability index because there was a strong correlation among variables of the same group (e.g., texture and structure) (Ogunkunle, 1993). All the lowest characteristics for rating each land quality group were substituted into the suitability equation for the actual suitability index calculation formulae. Regarding the potential suitability index, the observed corrective limitation was no longer a constraint. Finally, the suitability indices were assigned to land suitability classes following the Sys ratings (Sys, 1985; Sys et al., 1991, 1993) (Tables 3, 4).
The Munsell Color Chart, a standard system for soil color description was used. The Munsell color notations are systematic, numerical and letter designations of three parameters (hue, value and chroma). A small piece of soil was compared with the standard color chips in the soil color book. Each color chip was described by three components: hue, value and chroma. Thus, hue represents the dominant spectral color which refers to the redness or yellowness in the soil. The value refers to the relative lightness or darkness of a color (amount of reflected light), a value of zero (0) denotes black. Chroma signifies the purity of the dominant color (strength of the color), and a chroma of zero (0) being neutral gray. For example, the notation of 2.5 YR5/6 means a hue of 2.5 YR, value of 5 and a chroma of 6. The equivalent soil color name is “red.”
In order to quantify SI, six (6) land and soil characteristics indicating the resilience and performance of land were used. For each land unit the measured values for the indicators were derived from the field and analyzed according to defined threshold values (Tables 5, 6). By summation of all scores, a minimum value of 6 and a maximum value of 20 (four points for SOC, Clay + Silt content, and three points each for pH, CEC, soil depth, and slope) could be attributed to a land unit. The total points were grouped into four different categories of SI potential. The land with the lowest quality has only a final score between 6 and 10 (category 1). This implies that the soil has intrinsic properties which cannot support sustainable intensification. Land in category 2 can show medium or good conditions (score > 10), but one or even more indicators are in a poor condition. Therefore, intensification is possible only at a high risk. A score between 11 and 15 represents medium (category 3). This implies a poor potential for SI. This means that intensification should be done with much caution. For category 4 a total score between 16 and 20, represents soils which can compensate environmental impacts through agricultural production. This implies that soils of this nature can be recommended for intensive agriculture under the precondition that it can be managed sustainably.
All statistical analyses were performed with Excel and SPSS version 20.
Moist soil surface color differed among soil types. The average moist color reading ranged between 2.5 YR 3/4 (Bediesi series) to 10YR 6/3 (Changnalili series) for the A horizon (Table 7). For B horizon, soil color ranged from 2.5YR 4/6 in Bediesi and Damongo series to 10 YR 5/6 in Kpelesawgu series (Table 7).
The soils are deep (>100 cm), well-drained and gravel-free. The results from textural analysis indicate that these soils ranged from sandy loam to sandy clay loam (Table 8). The percentage of sand varied between 60% (Changnalili series) and 81% (Damongo series) for 0 to 20 cm. For percentage silt, it ranged between 15.2% (Damongo series) and 36% (Changnalili series) for the 0 to 20 cm. Also, the percentage of clay varied between 2.5% (Murugu series) and 6% (Sutawa series).
Chemically, soil pH ranged from 5.20 to 6.60 (Table 9). The percentage of SOC ranged from 0.55% to 2.02%. The concentration of Ex. K ranged from 0.05 to 1.41 to 86.06 mg kg−1. For Ex. K, Sutawa series had the highest (1.41 cmol(+) kg−1), followed by Bediesi (0.30 cmol(+) kg−1), Kpelesawgu (0.19 cmol(+) kg−1) and Murugu (0.15 cmol(+) kg−1). However, Changnalili and Damongo series recorded the lowest (0.05}. The concentration of available phosphorus (Av. P) ranged from 2.18 to 12.32 mg kg−1. Within soil series, Av. P followed the order: Bediesi (12.32 mg kg−1), Kpelesawgu (7.65 mg kg−1), Sutawa (6.79 mg kg−1), Changnalili (6.50 mgkg−1), Murugu (3.12 mg kg−1), and Damongo series (2.18 mg kg−1). Base saturation ranged from 67.0 and 98.89%. For soil types, base saturation followed the order: Sutawa > Damongo > Bediesi > Murugu > Kpelesawgu > Changnalili series (Table 9). Also, CEC ranged from 3.63 to 12.63 cmol(+) kg−1. Micronutrient cations (Ca2+, Mg2+, Na+, and K+) varied in all soil series. Bediesi series had the highest concentration of these cations. Also, we observed that cation exchange capacity varied between 3.61 cmol(+) kg−1 in Changnalili series and 12.63 cmol(+) kg−1 in Bediesi series. Within the soil series, mean values recorded were in the order: Bediesi > Sutawa > Kpelesawgu > Damongo > Murugu > Changnalili series. These results stressed a high potential for soil nutrient leaching in all soil series except Bediesi series. With an EC of > 4 dS m−1 (Table 9), all soils encountered had negligible effects of salinity.
Pearson correlation values >0.70 between physico-chemical properties indicate that most of the indicators positively correlated with each other (Table 10). Organic carbon positively correlated with TN (0.94**). Also, CEC positively correlated with pH (0.74**), Ex. Ca (0.96**), Ex. Mg (0.89**), ECEC (0.99**) and negatively correlated with Ex. Acidity (−0.73**). With respect to percentage BS, it correlated with pH (0.93**), Ex. Ca (0.82**), Ex. Mg (0.79**), CEC (0.85**), ECEC (0.82**) and negatively correlated with Ex. Acidity (−0.90**). Sand recorded a negative correlation between Silt (−0.92**) and Clay (−0.72**). From the above, soil physico-chemical processes tend to function simultaneously. However, the trends are broad and high with a correlation coefficient ranging between 0.01 and 0.99 and −0.02 and −0.92 (Table 10).
The rating of land and/or soil characteristics with the requirements of maize (Tables 2, 7) produced suitability classes for maize (Table 11). Most of the soil series encountered were marginally suitable (S3) for parametric and non-parametric methods (Tables 12, 13). The major limitations observed were related to soil texture and structure. These two physical properties of the soil directly affect water-holding capacity, permeability, and other physical properties for the non-parametric (potential) rating (Table 13). Other soil limiting factors were drainage, soil fertility (as measured by CEC, soil organic carbon, organic matter, and total nitrogen content) for the non-parametric (actual) rating.
Table 13. Suitability classifications with rankings of soils of Nkoranza (north and south) district for maize cultivation.
Soil suitability for maize cultivation was based on several indices for the six major soil series identified in the Nkoranza (north and south) district. The climatic index (CI) for Bediesi, Sutawa, Kpelesawgu, Changnalili, Damongo, and Murugu series had a value of S1 (80) (Table 12). A pedological index consisting of soil texture, structure, coarse fragment, and depth for various soil series computed had a value of S2 (71).
Results indicated that the soil fertility index (SFI) for maize varied between 30 (S3) and 95 (S1). Thus, soils were moderately suitable due to a slight climatic (80%) and pedological limitation (71%). However, the fertility index (SFI) for Bediesi, Sutawa, Murugu, Damongo, Changnalili and Kpelesawgu were 95%, 82%, 66%, 64%, 39%, and 30%, respectively (Table 12). Concerning salinity, determined by electrical conductivity, Bediesi and Sutawa series had a slight salinity index of 85 and 95%, respectively. However, Kpelesawgu, Changnalili, Damongo, and Murugu series had no salinity limitation.
Potential fertility includes cation exchange capacity, base saturation, pH, and organic matter. These chemical properties were altered during tillage. All soil series had a suitability rating of N1 (40) for CEC except Bediesi series, which had a high suitability rating (S1 (95). Bediesi, Sutawa, Damongo, and Murugu series had an S1 (100) suitability rating for soil pH. Changnalili and Kpelesawgu series recorded S3 (60), S2 (85), and N1 (40), respectively (see Tables 11, 13). For organic matter, all soil series in Table 13 recorded a high suitability rating of S1 (100), S1 (95), S1 (95), and S2 (85) for the Bediesi, Sutawa, Kpelesawgu, Murugu, and Damongo series, respectively, except Changnalili series S3 (60).
Tables 11, 12 indicate that the fertility rating for CEC varied between S1 (95) for Bediesi series and N1 (40) for Sutawa, Kpelesawgu, Changnalili, Damongo, and Murugu series. All soil series encountered had S1 (100). However, the overall potential suitability (non-parametric) evaluation remains moderately suitable, with topography (t), wetness (w), and soil characteristics (s) as limitations that cannot be easily alleviated (Table 5). Bediesi and Sutawa series had topography (t) as a limitation factor, and Kpelesawgu and Changnalili series had wetness (w) as a limiting factor. On the contrary, Damongo and Murugu series had soil characteristics as a constraint. Regarding potential suitability (parametric), Sutawa, Kpelesawgu, Changnalili, Damongo, and Murugu series were moderately suitable (S2), except that Bediesi series was rated as highly suitable (S1). The potential suitability could be moderately suitable for soils with water conservation, moisture harvesting structures with fertilizer, lime, and organic matter management.
Current soil fertility includes all soil properties easily influenced by soil management practices. These are Av. P, Ex. K, Ex. Ca, Ex. Mg, Mg:K ratio, and Exchangeable bases (Ca2+, Mg2+, K+). When subjected to tillage, soil chemical properties were easily altered in the soil medium. All soil series had high exchangeable cation values. The Mg: K ratio is adequate in all soil series. The concentration of SOC, TN, and Av. P was considered to be low in all soils except Bediesi series. All soil series had varied exchangeable cations (Ca2+, Mg2+, Na+, and K+). Bediesi series had the highest cation concentration in calcium, magnesium, and potassium with S1 (100) soil suitability ratings. Bediesi series recorded the highest concentration of these cations with values above 8.68 cmol(+) kg−1for Ex. Ca, 0.30 cmol(+) kg−1 for Ex. K and 2.80 cmol(+) kg−1 for Ex. Mg and 0.85 cmol(+) kg−1 for Ex. Na. Also, CEC varied between 3.61 cmol(+) kg−1 (Changnalili series) and 22.4 cmol(+) kg−1 for Bediesi series. These results stress that there has been an increase in nutrient leaching in all soil series sampled except Bediesi series. The overall rating for the non-parametric (actual/current) evaluation for maize is moderately suitable, with fertility (f) and soil physical properties (s) (thus texture and structure) as the limitations. Hence, soil fertility (f) can be amended. Also, for parametric (actual/current) suitability evaluation: Sutawa, Kpelesawgu, Changnalili, Damongo, and Murugu series were marginally suitable (S3). Bediesi series recorded a high suitability rating of S1. Adopting good farm management practices (eg. organic manure, mulching, etc.), can improve the CEC of Sutawa, Kpelesawgu, Changnalili, Damongo, and Murugu series.
Soil resilience was calculated for bench mark soils (Bediesi, Sutawa, Kpelesawgu, Changnalili, Damongo and Murugu series) of the study area. The summation of all indicator scores resulted in a score ranging between 12 and 15 representing a moderate SI potential (category 3; Tables 14, 15). This means the soil resources of the study area possess a low (poor) potential for SI thus, intensification could be done with caution. The most limiting soil indicators were SOC and CEC and this could be managed sustainably to increase SI in the study area. From our study, the soils of Nkoranza (north and south) can be recommended for intensive agriculture (SI) under the precondition that it can be managed sustainably.
Table 15. Distribution of soil resilience (SR) classes in the Nkoranza (north and south) districts can be recommended for SI.
From Table 16, parametric potential index (PPI), and parametric actual index (PPI) were significant and strongly correlated (r = 0.94, p < 0.003). This indicates that as soil suitability (parametric potential index) increases, soil quality and/or health improves. Also, soil resilience index (SRI) and the parametric potential index (PAI) had a significantly high correlation (r = 0.76, p < 0.037, p being < 0.05), and this means that as soil resilience increases, soil suitability in terms of productivity (yield) for maize increases. This shows that the relationship between PPI and PAI, as well as SRI and PAI are a perfect correlation (Table 16). Hence, it can be deduced that PAI affects the extent of PPI and SRI. However, an insignificant moderate correlation was observed between soil resilience and parametric potential index (r = 0.59, p < 0.104, p being > 0.05).
Table 16. Correlation analysis between parametric actual index (pai), parametric potential index (ppi), and soil resilience index (SRI).
Soil color readings differed among soil management zones due to cropping and land-use systems practiced by smallholder farmers. Soil color was influenced by the mineralogy and chemical composition of the soil. The presence of manganese oxides imparted the black color to the soil. Also, organic matter in the soil imparted dark brown to black color to the soils sampled. From the above, observed soil colors may have contributed to low organic matter content (Table 7). The abundance of oxidized Fe in highly weathered tropical soils (Young, 1980), may have resulted in the low organic matter. The high Fe-MnO2 concretions may have accounted for the red to reddish-brown colors in Bediesi series. Several research findings observed higher chroma values of red and associated these soils with low fertility (Desbiez et al., 2004; Laekemariam et al., 2016; Laekemariam and Kibret, 2020). The presence of iron compounds imparted the red, and/or brown color. The red or brown color is mostly related to the extent of oxidation, hydration and diffusion of iron oxides in the soil medium (Foth, 1990).
Most farmers consider dark soil colors fertile compared to reddish hues in the Savannah ecological zone (Desbiez et al., 2004; FARM-Africa, 2005; Haileslassie et al., 2006). The results of this study indicated that soil color is influenced by SOM content. The dark color in the A-horizon decreased with depth. Soils found on slopes not saturated with water had reddish and brownish subsoil colors. Thus, soils of this nature are well-drained and aerated. Soils sampled at poorly drained locations had gray-colored B-horizons (Foth, 1990). Erosion often removed the topsoil layer from the shoulder/back slope of high slopes. This left behind thin and light-colored soils with low organic matter compared to soils of foot-slopes or toe-slopes. High organic matter content was associated with thick A-horizons (Table 7) as observed by Mulugeta and Sheleme (2011) in southern Ethiopia.
Soil pH ranges from (slightly acidic) to (neutral) for Kpelesawgu and Damongo series. According to Food and Agriculture Organization (FAO) of the United Nations (1985) and Diallo et al. (2016), this range mainly corresponds with productive soils in the humid tropics. Achieving optimal production of maize requires a moderate acidic to slightly basic soil with a pH range of 5.5–7.50 [Food and Agriculture Organization (FAO) of the United Nations, 1976, 1985] and well-distributed rainfall (800–1,200 mm) throughout its growing season. However, although the physical conditions and environmental factors are favorable for maize production, SOM can be improved with fertilizers and other organic resources (e.g., poultry manure). Based on expert opinion, the selected soil properties (Tables 1, 2, 5) (Mandal et al., 2017) can be improved. Increasing the suitability of soils can improve maize yield in the short and long term in the study area. Soil pH affects soil biological activity, and the availability of nitrogen to plants can also serve as an essential chemical indicator for soil fertility (Diallo et al., 2016). Soil pH influences plant growth and increases the bioavailability of soil nutrients and the activity of soil microbes (Diallo et al., 2016).
The CEC levels were in the order: Bediesi > Sutawa > Kpelesawgu > Damongo > Murugu > Changnalili series. Bediesi series had a higher CEC compared to the other soil types due to high surface organic matter. Thus, CEC of a soil is controlled by organic matter and clay content. A CEC of <12 cmol(+) kg−1 is low due to the low availability of organic matter. The variability of CEC within soil series explains the differences in the ability of these soils to hold positively charged ions affecting the stability of soil structure, nutrient availability, soil pH, and the response of these soils to fertilizer when applied (Crewett and Korf, 2008). This finding is in line with McAlister et al. (1998), who stressed that CEC varies with a change in percentage of clay, type of clay, pH, and the amount of SOM among soil types. Base saturation followed the order: Sutawa > Damongo > Bediesi > Murugu > Kpelesawgu > Changnalili series. Soil pH is a major factor in determining the percentage of base saturation of soils. According to Brady et al. (2008), soils of the tropics have a variable charge system, and this charge on the exchange complex gives the soil the strength to attract positively charged basic ions.
Therefore, the higher the soil pH (8.5), the higher the negative charges created and the higher the basic cations absorbed by the soil. The more cations absorbed, the higher percentage of base saturation. Damongo, Sutawa, and Bediesi series had a relatively higher pH than Murugu, Changnalili, and Kpelesawgu series. This could be due to leaching of basic cations in the latter, giving rise to a relatively lower soil pH resulting in a lower base saturation in Changnalili series. Damongo, Sutawa, and Bediesi series had enough vegetation cover to check the leaching of basic cations, and this in the long-term increased soil pH and base saturation. The concentration of soil organic matter and exchangeable cations was low, indicating that the soils are inherently low in fertility. This implies that soil pH influences the efficiency of plant growth. Soil organic matter and exchangeable cations represent actual and/or potential soil fertility (PSF) (Diallo et al., 2016). When soil is mismanaged, it loses its fertility after years of cultivation. In a similar research, Lal (1996) observed a rapid decline in SOM after intensive cultivation. The low soil fertility could be attributed to a decrease in soil pH due to absorption of nutrients by plants and leaching of basic cations beyond plant roots.
Land suitability assessment focus on crop requirements, soil type, and landscape attributes that influence cultivation. For the parametric evaluation process (potential) for maize, the soils of the study site showed moderate suitability for maize production (Tables 11–13). However, except Bediesi series, all other pedons were rated severe for the actual parametric evaluation. The limitation can be corrected (see Tables 12, 13). The parametric and non-parametric methods classified land units as S2 (highly suitable) and S3 (marginally suitable). The chemical characteristics of the soils were the dominant limiting factors that affected the suitability of land for maize farming in the Nkoranza district.
Concerning pedogenesis and the addition of organic inputs, organic matter is low (Wang et al., 2014). As a result, these soils hardly store organic matter in the form of organic carbon. Also, inappropriate farming practices depleted soil nutrients in the identified soil series. Therefore, application of poultry manure which is abundant in Nkoranza district can be used to restore soil nutrient stocks, and improve soil quality (Yimer et al., 2007). From the above discussion, the main limitation (see Table 13) is soil nutrient deficiency, not climate or topography, because the water need for maize is not greater than the edaphic conditions of the surrounding environment (Diallo et al., 2016). With the significant limitation identified as chemical degradation, these soils would be highly suitable for maize production, if much attention is paid to soil management. Also, these smallholders can use poultry manure from the vast poultry industry in the Nkoranza district to enrich soil fertility on their farms. The use of integrated soil fertility management practices can restore the chemical limitation of these soils.
Table 11 indicates land suitability evaluation for maize cultivation. Potential soil fertility based on cation exchange capacity, base saturation, organic carbon, and soil pH indicated that these soils have a limitation. The soils in Tables 7, 9 had different levels of fertility limitations due to different parent materials. Sandstone had more fertile soils and was richer in plant nutrients than those found in old sediments due to abundant weatherable primary minerals in these soils. These physical properties influence soil fertility by controlling the release and supply of nutrients. Soil physico-chemical parameters, as influenced by parent material, directly influence soil fertility for maize production in the transition zone of Ghana. Maize growth and development depend on the potential of the soil parent material to supply nutrients. Climate is not a significant limitation to maize production because mean annual maximum temperature promotes favorable soil moisture conditions for plant growth and development. As a result, good soil management techniques are required to increase soils suitability ratings for maize production. Water is important during the growth and development stages of maize because a deficit or excess can result in a low yield. Several studies indicated that poor drainage reduces maize yield by affecting net photosynthesis, stomata conductance, and transpiration. Areas with high clay content can be managed by establishing drainage systems that remove excess water to promote maize root development.
Soil physical characteristics had no significant limitations except for sites with very high clay content. Maize grows well in soils with sandy clay loam texture with an optimal water table depth (50–60 cm). The low values of Ex. K in these soils are due to low CEC and high amounts of rainfall that promote the leaching of basic cations. Also, the farmers are smallholders who engage in crop production in a fragile environment with little or no resources for good agronomic practices. In recent years, soil degradation through inappropriate farming practices such as uncontrolled grazing and bush burning with low fertilizer use has increased. Appropriate soil fertility management for K fertilization is required to improve yield. Potassium is the most important nutritional factor that determines maize yield because K plays a major role in the proper functioning of the stomata and prevents droughty conditions. Also, its availability promotes the transportation of assimilates from photosynthesis, assists in enzyme activation, and makes the maize plant disease resistant. Soil fertility evaluation results indicated a low Mg:K ratio due to the relatively low Ex. Mg and a high Ex. K values. This means excess K ions depress the uptake of other cations such as Mg2+ ions. Low P availability was a major constraint to maize production in the study area. The application of phosphorus and magnesium amendments could increase maize yield. Understanding the nature and variability of soil properties with respect to soil fertility and maize are critical issues to consider in soils formed on sandstone and clay shale parent materials in the transition zone of Ghana.
This study reveals how soil can be included in discussions on where to find land to produce more food to feed the growing population with the adoption of soil resilience principles (Schiefer et al., 2016). The maize fields sampled had soils with moderate soil resilience (Table 15). The soils intrinsic characteristics are favorable for intensification if and only the main limiting factor thus SOC and CEC are managed carefully to avoid causing low soil resilience. The relationship between CEC and other indicators (pH, Ex. Ca, Ex. Mg, and Ex Acidity) as well as between SOC and Sand, Silt and Clay confirms the fact that these physico-chemical properties function simultaneously (Table 10) and at a moderate capacity (Tables 14, 15). Thus, CEC is the soils capacity to retain organic and inorganic positively charged compounds in the soil medium.
Inappropriate land use management has resulted in the degradation of soils hence rejuvenating the negative effects of poor parent material, terrain characteristics and in a changing climate has affected soil resilience (Lal, 1997). The processes of soil resilience involve the mechanisms that influence the soils' ability and rate to recover after disturbance. Good soil management results in the provision of other ecosystem services such as biodiversity, water storage, carbon storage, flood and/or drought regulation etc. According to van Ittersum et al. (2013), the evaluation of the potential of land for SI is important because this could serve as a guide to evaluate yield gap potential including the differences associated with climatic conditions and cropping systems on farmer's fields locally and regionally. This can assist in the prediction of the future potential of sustainable intensification of smallholder agriculture in Ghana.
From the above discussion, the integration of organic and inorganic resources into soil fertility management options (compost, manure, plant residue, etc.) and organic residues can be use by farmers (Bationo et al., 2007; Bekunda et al., 2010) during the beginning of the major and minor farming seasons to increase maize yield from the current 1.75 t/ha in the Nkoranza (North and South District). Also, site specific fertilizer application (Tetteh et al., 2017, 2018) compared to the blanket fertilizer application [mostly two (2) bags of 50 kg NPK and 1 bag of 50 kg of Urea or Sulfate of Ammonia per ha] is recommended for small farm holders in the Nkoranza (north and south) districts. Thus, the relevance of mineral fertilizer use in the intensification of food production on small farms cannot be under estimated especially in areas with soils deficient in nutrients.
According to Tetteh et al. (2018), combined application of organic fertilizer (poultry manure at 2.5 T ha−1) with 60 kg N ha−1 of mineral fertilizer yielded the same yield as the application of sole 90 kg N ha−1. Hence, the application of 90 kg N ha−1 yielded optimum economic returns in the Forest-Savannah Transition Zone (FSTZ). Thus, the current fertilizer recommendation for maize with respect to NPK recommendation is N-P2O5-K2O:90-60-60+1.7 Zn and for recommended blends per hectare is N-P2O5-K2O:15-20-20+0.7 Zn (8 bags/ha + 2bags/ha urea) for the FSTZ of Ghana. Also, the recommended fertilizer formulae blend is N-P2O5-K2O:15-20-20 +0.7 Zn (6 bags/ha + 2 bags/ha urea) (Tetteh et al., 2017, 2018). The mean yield from field validations of the new fertilizer recommendations using the new blends or formulae yielded a mean of 6.0 t/ha. Thus, on a fertile soil with recommended fertilizer application and planting at the onset of the major and minor rainy season, a grain yield ranging between 5 to 8t/ha with high returns is expected compared to the current yield of 1.75 t/ha for Nkoranza (North and South) district and 1.6t/ha of national average yield for Ghana. This would assist improve the efficiency and profitability of fertilizer use on smallholder maize farms in the Nkoranza (north and south) districts in the Forest Transition Agro-ecological Zone of Ghana.
The creation of an enabling environment through the formulation of policies and institutions to facilitate the intensification process in smallholder agricultural production systems should be a national priority. Also, investments into agricultural research as documented in the Maputo declaration that at least 10% of national budgetary allocations should be invested in agriculture and rural development (African Union, 2003) could strengthen innovative systems that increase productivity on small farms. These investments should include the provision of credit (loans, subsidies etc.), input (fertilizers, herbicides, and pesticides) and the creation of markets for agricultural produce. These according to Schut et al. (2016) could address 70% of the constraints associated with the sustainable intensification process at the local, regional and national level in Ghana.
The evaluation methods used for soil suitability assessment indicated that climate, soil texture, and topography were suitable for maize cultivation. All pedons were moderately suitable (S2) for the parametric and marginally suitable (S3) for the non-parametric method. The limitations relating to texture and structure directly affected soil water holding capacity and soil permeability. The primary soil fertility constraints were CEC, organic matter, and available P, varied across soil types. The maize fields sampled had soils with moderate soil resilience. The relationship between CEC and other indicators (pH, Ex. Ca, Ex. Mg, and Ex Acidity) as well as between SOC and Sand, Silt and Clay confirms that these physico-chemical properties function simultaneously and at a moderate capacity. Also, pearson correlation revealed a strong significant relationship between parametric actual index (PAI) and parametric potential index and between soil resilience (SRI). This suggests that soil physico-chemical properties can be used to quantify the productivity of soils. Therefore, emphasis on soil management techniques to enhance soil nutrient and moisture-holding capacity can be improved to increase productivity levels of maize. Farm management techniques (e.g., soil and water conservation, moisture harvesting, organic and inorganic fertilizers) are recommended to improve soil nutrient levels. Soil suitability indexing can enable soil quality monitoring in relation to sustainable intensification of agricultural land use so that threats to soil resources and opportunities for sustainable intensification could be identified in the Nkoranza district in the Forest-Savanna Transition Zone of Ghana.
The original contributions presented in the study are included in the article/supplementary material, further inquiries can be directed to the corresponding author.
JA designed the research, analyzed all data, and wrote the manuscript. JA and BD did field survey and carried out the laboratory experiments. GQ checked for accuracy of data and reviewed the manuscript. All authors contributed to the article and approved the submitted version.
We highly acknowledge the reviewers of this paper.
The authors declare that the research was conducted in the absence of any commercial or financial relationships that could be construed as a potential conflict of interest.
All claims expressed in this article are solely those of the authors and do not necessarily represent those of their affiliated organizations, or those of the publisher, the editors and the reviewers. Any product that may be evaluated in this article, or claim that may be made by its manufacturer, is not guaranteed or endorsed by the publisher.
Adjei-Gyapong, T., and Asiamah, R. D. (2002). The Interim Ghana Soil Classification System and its Relation with the World Reference Base for Soil Resources. Quatorzieme Reunion de Sous-Comite oust et centre Africain de Correlation des Sols. Kumasi: Soil Research Institute.
Adu, S., and Mensah-Ansah, J. (1995). Soils of the Afram Basin, Ashanti and Eastern Regions, Ghana. Kumasi: Soil Research Institute.
AGRA (2014). Africa Agriculture Status Report: Climate Change and Smallholder Agriculture in Sub-Saharan Africa. Nairobi: Alliance for a Green Revolution in Africa (AGRA).
Agyili, R. (2003). Soils of the Pru River Basin. Ashanti and Brong Ahafo Regions Ghana. Tech. rep. Kumasi: CSIR-Soil Research Institute.
Ande, O. T. (2011). Soil suitability evaluation and management for cassava production in the derived savanna area of Southwestern Nigeria. Int. J. Soil Sci. 6, 142–149. doi: 10.3923/ijss.2011.142.149
Annan-Afful, E., Iwashima, N., Otoo, E., Asubonteng, K. O., Kubota, D., Kamidohzono, A., et al. (2004). Nutrient and bulk density characteristics of soil profiles in six land use systems along topo-sequences in inland valley watersheds of Ashanti region, Ghana. Soil Sci. Plant Nutr. 50, 649–664. doi: 10.1080/00380768.2004.10408522
Annan-Afful, E., Masunaga, T., and Wakatsuki, T. (2005). Soil properties along the toposequence of an inland valley watershed under different land uses in the Ashanti Region of Ghana. J. Plant Nutr. 28, 141–150. doi: 10.1081/PLN-200042199
AQUASTAT and FAO (2005). Irrigation in Africa in figures: AQUASTAT Survey. FAO Water Report. Rome: FAO.
Asiamah, R. D. (2008). “Soil resources of Ghana,” in Synthesis of Soil, Water and Nutrient Management Research in the Volta Basin, eds. A. Bationo, R. Tabo, B. Waswa, J. Okeyo, J. Kihara, M. Fosu, and S. Kabore (Nairobi: Ecomedia Ltd), 25–41.
Awoonor, J. K., Yeboah, E., Dogbey, B. F., and Adiyah, F. (2021). Sustainability assessment of smallholder farms in the savannah transition agro-ecological zone of Ghana. Agric. Sci. 12, 1185–1214. doi: 10.4236/as.2021.1211076
Baroudy, A. A. E. (2016). Mapping and evaluating land suitability using a GIS-based model. CATENA 140, 96–104. doi: 10.1016/j.catena.2015.12.010
Bationo, A., Fening, J. O., and Kwaw, A. (2018). “Assessment of soil fertility status and integrated soil fertility management in Ghana,” in Improving the Profitability, Sustainability and Efficiency of Nutrients Through Site Specific Fertilizer Recommendations in West Africa Agro-Ecosystems, eds A. Bationo, D. Ngaradoum, and S. Youl (Berllin: Springer International Publishing), 93–138. doi: 10.1007/978-3-319-58789-9_7
Bationo, A., Kihara, J., Vanlauwe, B., Waswa, B., and Kimetu, J. (2007). Soil organic carbon dynamics, functions and management in West African agro-ecosystems. Agric. Syst. 94, 13–25. doi: 10.1016/j.agsy.2005.08.011
Baulcombe, D., Crute, I., Davies, B., Dunwell, J., Gale, M., Jones, J., et al. (2009). Reaping the Benefits: Science and the Sustainable Intensification of Global Agriculture. London: The Royal Society.
Bekunda, M., Sanginga, N., and Woomer, P. L. (2010). “Restoring soil fertility in sub-Sahara Africa,” in Advances in Agronomy, ed D. O. Sparks (Amsterdam: Elsevier), 183–236. doi: 10.1016/S0065-2113(10)08004-1
Blake, G., and Hartage, H. (1986). Methods of soil analysis 2d ed., pt. 1; physical and mineralogical methods. Soil Sci. 146, 138. doi: 10.1097/00010694-198808000-00014
Bouyoucos, G. J. (1962). Hydrometer method improved for making particle size analyses of soils. Agron. J. 54, 464–465. doi: 10.2134/agronj1962.00021962005400050028x
Brady, N. C., Weil, R. R., and Weil, R. R. (2008). The Nature and Properties of Soils, Vol. 13. Upper Saddle River, NJ: Prentice Hall.
Bray, R. H., and Kurtz, L. T. (1945). Determination of total, organic, and available forms of phosphorus in soils. Soil Sci. 59, 39–46. doi: 10.1097/00010694-194501000-00006
Christiansen, E., and Awadzi, T. W. (2008). Water balance in a moist semi-deciduous forest in Ghana. West Afr. J. Appl. Ecol. 1, 11–22. doi: 10.4314/wajae.v1i1.40566
Claessens, L., Vanlauwe, B., Cassman, K. G., van Wart, J. P., Grassini, P., Yang, H., et al. (2013). “Soil suitability for sustainable intensification in smallholder systems in Sub-Saharan Africa,” in Proceedings of the First International Conference on Global Food Security held in Noordwijkerhout, Netherlands (Noordwijkerhout). Available online at: http://www.agmip.org/wp-content/uploads/2013/11/Poster-Claessens-et-al.pdf
Crewett, W., and Korf, B. (2008). Ethiopia: reforming land tenure. Rev. Afr. Polit. Econ. 35, 203–220. doi: 10.1080/03056240802193911
Debesa, G., Gebre, S. L., Melese, A., Regassa, A., and Teka, S. (2020). GIS and remote sensing-based physical land suitability analysis for major cereal crops in Dabo Hana district, south-west Ethiopia. Cogent Food Agric. 6, 1780100. doi: 10.1080/23311932.2020.1780100
Desbiez, A., Matthews, R., Tripathi, B., and Ellis-Jones, J. (2004). Perceptions and assessment of soil fertility by farmers in the mid-hills of Nepal. Agric. Ecosyst. Environ. 103, 191–206. doi: 10.1016/j.agee.2003.10.003
Diallo, M. D., Wood, S. A., Diallo, A., Mahatma-Saleh, M., Ndiaye, O., Tine, A. K., et al. (2016). Soil suitability for the production of rice, groundnut, and cassava in the peri-urban niayes zone, Senegal. Soil Tillage Res. 155, 412–420. doi: 10.1016/j.still.2015.09.009
FARM-Africa (2005). Soil Fertility Practices in Wolaita Zone, southern Ethiopia: Learning from Farmers. Policy and Research. Southampton: FARM-Africa.
Fening, J. O., Adjei-Gyapong, T., Yeboah, E., Ampontuah, E. O., Quansah, G., Danso, S. K. A., et al. (2005). Soil fertility status and potential organic inputs for improving small holder crop production in the interior savanna zone of Ghana. J. Sustain. Agric. 25, 69–92. doi: 10.1300/J064v25n04_07
Fening, J. O., Yeboah, E., Adjei-Gyapong, T., and Gaizie, E. (2009). On farm evaluation of the contribution of three green manures to maize yield in the semi - deciduous forest zone of Ghana. Afr. J. Environ. Sci. Technol. 3, 234–238.
Foley, J. A., Ramankutty, N., Brauman, K. A., Cassidy, E. S., Gerber, J. S., Johnston, M., et al. (2011). Solutions for a cultivated planet. Nature 478, 337–342. doi: 10.1038/nature10452
Food and Agriculture Organization (FAO) of the United Nations (1976). A Framework for Land Evaluation. Rome: Food and Agriculture Organization of the United Nations.
Food and Agriculture Organization (FAO) of the United Nations (1985). Guidelines: Land Evaluation for Irrigated Agriculture. Rome: Food and Agriculture Organization of the United Nations.
Food and Agriculture Organization (FAO) of the United Nations (2020a). The State of Food and Agriculture 2020. Rome: FAO.
Food and Agriculture Organization (FAO) of the United Nations (2020b). World Food and Agriculture - Statistical Yearbook 2020. Rome: FAO.
Foth, H. (1990). Fundamentals of soil science. Soil Sci. 125, 272. doi: 10.1097/00010694-197804000-00021
Frelat, R., Lopez-Ridaura, S., Giller, K. E., Herrero, M., Douxchamps, S., Djurfeldt, A. A., et al. (2015). Drivers of household food availability in sub-Saharan Africa based on big data from small farms. Proc. Nat. Acad. Sci. 113, 458–463. doi: 10.1073/pnas.1518384112
Gelaw, A., Singh, B., and Lal, R. (2015). Soil quality indices for evaluating smallholder agricultural land uses in northern Ethiopia. Sustainability 7, 2322–2337. doi: 10.3390/su7032322
Giller, K. E., Delaune, T., Silva, J. V., van Wijk, M., Hammond, J., Descheemaeker, K., et al. (2021). Small farms and development in sub-Saharan Africa: farming for food, for income or for lack of better options? Food Secur. 13, 1431–1454. doi: 10.1007/s12571-021-01209-0
Hagos, Y. G., Mengie, M. A., Andualem, T. G., Yibeltal, M., Linh, N. T. T., Tenagashaw, D. Y., et al. (2022). Land suitability assessment for surface irrigation development at Ethiopian highlands using geospatial technology. Appl. Water Sci. 12. doi: 10.1007/s13201-022-01618-2
Haileslassie, A., Priess, J. A., Veldkamp, E., and Lesschen, J. P. (2006). Smallholders' soil fertility management in the central highlands of Ethiopia: implications for nutrient stocks, balances and sustainability of agroecosystems. Nutr. Cycl. Agroecosystems 75, 135–146. doi: 10.1007/s10705-006-9017-y
Iizumi, T., Kotoku, M., Kim, W., West, P. C., Gerber, J. S., Brown, M. E., et al. (2018). Uncertainties of potentials and recent changes in global yields of major crops resulting from census- and satellite-based yield datasets at multiple resolutions. PLoS ONE 13, e0203809. doi: 10.1371/journal.pone.0203809
International Center for Soil Fertility and Agricultural Development (2007). Africa Fertilizer Summit Proceedings: June 9-13, 2006. Abuja, Nigeria. Abuja: Special Publication. IFDC (IFDC-An International Center for Soil Fertility and Agricultural Development).
IUSS Working Group (WRB) (2015). “World reference base for soil resources 2014, update 2015 international soil classification system for naming soils and creating legends for soil maps,” in World Soil Resources Reports (Rome: FAO), 106.
IUSS Working Group (WRB) (2022). “World reference base for soil resources,” in International Soil Classification System for Naming Soils and Creating Legends for Soil Maps (Rome: FAO).
Jackson, M. (1973). Soil chemical analysis. J. AOAC Int. 41, 740–740. doi: 10.1182/blood.V41.5.740.740
Janzen, H. H., Fixen, P. E., Franzluebbers, A. J., Hattey, J., Izaurralde, R. C., Ketterings, Q. M., et al. (2011). Global prospects rooted in soil science. Soil Sci. Soc. Am. J. 75, 1–8. doi: 10.2136/sssaj2009.0216
Jones, A., Breuning-Madsen, H., Brossard, M., Dampha, A., Deckers, J., Dewitte, O., et al. (2013). Soil atlas of Africa. Luxembourg: Publications Office of the European Union.
Kasei, C. (1993). “A synopsis on the climate of the north of Ghana,” in Proceedings of Workshop on Improving Farming Systems in the Interior Savannah of Ghana (Nyankpala).
Kawy, W. A. M. A., and El-Magd, I. H. A. (2012). Use of satellite data and GIS for assessing the agricultural potentiality of the soils South Farafra Oasis, Western Desert, Egypt. Arab. J. Geosci. 6, 2299–2311. doi: 10.1007/s12517-012-0518-5
Khiddir, S. M. (1986). A Statistical Approach in the Use of Parametric Systems Applied to the FAO Framework for Land Evaluation [Ph.D. thesis]. Ghent: Ghent University.
Kihoro, J., Bosco, N. J., and Murage, H. (2013). Suitability analysis for rice growing sites using a multicriteria evaluation and GIS approach in great Mwea region, Kenya. Springerplus 2, 265. doi: 10.1186/2193-1801-2-265
Laekemariam, F., and Kibret, K. (2020). Explaining soil fertility heterogeneity in smallholder farms of southern Ethiopia. Appl. Environ. Soil Sci. 2020, 1–16. doi: 10.1155/2020/6161059
Laekemariam, F., Kibret, K., Mamo, T., Karltun, E., and Gebrekidan, H. (2016). Physiographic characteristics of agricultural lands and farmers' soil fertility management practices in Wolaita zone, southern Ethiopia. Environ. Syst. Res. 5, 24. doi: 10.1186/s40068-016-0076-z
Lal, R. (1987). Response of maize (Zea mays) and cassava (Manihot esculenta) to removal of surface soil from an Alfisol in Nigeria. Int. J. Trop. Agric. 5, 77–92.
Lal, R. (1996). Deforestation and land-use effects on soil degradation and rehabilitation in western Nigeria. II. soil chemical properties. Land Degrad. Dev. 7, 87–98. doi: 10.1002/(SICI)1099-145X(199606)7:2<87::AID-LDR219>3.0.CO;2-X
Lal, R. (1997). Degradation and resilience of soils. Philos. Trans. R. Soc. B: Biol. Sci. 352, 997–1010. doi: 10.1098/rstb.1997.0078
Lal, R. (2006). Enhancing crop yields in the developing countries through restoration of the soil organic carbon pool in agricultural lands. Land Degrad. Dev. 17, 197–209. doi: 10.1002/ldr.696
Mandal, U. K., Sharma, K. L., Venkanna, K., Pushpanjali, Adake, R. V., Masane, R. N., et al. (2017). Sustaining soil quality, resilience and critical carbon level under different cropping systems in semi-arid tropical alfisol soils. Curr. Sci. 112, 1882. doi: 10.18520/cs/v112/i09/1882-1895
Mbagwu, J. S. C., Lal, R., and Scott, T. W. (1984). Effects of desurfacing of alfisols and ultisols in southern nigeria: I. Crop performance. Soil Sci. Soc. Am. J. 48, 828–833. doi: 10.2136/sssaj1984.03615995004800040026x
McAlister, J. J., Smith, B. J., and Sanchez, B. (1998). Forest clearance: impact of landuse change on fertility status of soils from the São Francisco area of Niterói, Brazil. Land Degrad. Dev. 9, 425–440. doi: 10.1002/(SICI)1099-145X(199809/10)9:5<425::AID-LDR306>3.0.CO;2-Z
McSweeney, C., New, M., Lizcano, G., and Lu, X. (2010). The UNDP climate change country profiles. Bull. Am. Meteorol. Soc. 91, 157–166. doi: 10.1175/2009BAMS2826.1
Ministry of Food and Agriculture (MoFA) (2011). Agriculture in Ghana. Facts and Figures 2010. Accra: SRID Accra
Motsara, M. (2015). Guide to Laboratory Establishment for Plant Nutrient Analysis. London: Scientific Publishers.
Mulugeta, D., and Sheleme, B. (2011). Characterization and classification of soils along the toposequence of Kindo Koye watershed in Southern Ethiopia. East Afr. J. Sci. 4, 65–77. doi: 10.4314/eajsci.v4i2.71528
Nandwa, S. M. (2001). “Soil organic carbon (SOC) management for sustainable productivity of cropping and agro-forestry systems in Eastern and Southern Africa,” in Managing Organic Matter in Tropical Soils: Scope and Limitations eds C. Martius, H. Tiessen, and P. L. G. Vlek (Berlin: Springer Netherlands), 143–158. doi: 10.1007/978-94-017-2172-1_14
Ogunkunle, A. O. (1993). Soil in land suitability evaluation: an example with oil palm in Nigeria. Soil Use Manage. 9, 35–39. doi: 10.1111/j.1475-2743.1993.tb00925.x
Ogunkunle, A. O. (2016). Management of Nigerian Soil Resources: An Imperative for Sustainable Development. Ibadan: Ibadan University Press.
Olsen, S., Sommers, L., and Page, A. (1982). Methods of Soil Analysis, Part 2. Chemical and Microbiological Properties. 2nd ed. Madison, WI: Am Soc Agron, 403–430.
Owusu-Bennoah, E., Awadzi, T. W., Boateng, E., Krogh, L., Breuning-Madsen, H., Borggaard, O. K., et al. (2008). Soil properties of a toposequence in the moist semi-deciduous forest zone of Ghana. West Afr. J. Appl. Ecol. 1, 1–10. doi: 10.4314/wajae.v1i1.40565
Panel, M. (2013). Sustainable Intensification: A New Paradigm for African Agriculture. London: Agriculture for impact.
Peel, M. C., Finlayson, B. L., and McMahon, T. A. (2007). Updated world map of the köppen-geiger climate classification. Hydrol. Earth Syst. Sci. 11, 1633–1644. doi: 10.5194/hess-11-1633-2007
Phillips, J., and Wills, J. B. (1963). Agriculture and land use in Ghana. Geogr. Rev. 53, 473. doi: 10.2307/212600
Pradhan, P., Fischer, G., van Velthuizen, H., Reusser, D. E., and Kropp, J. P. (2015). Closing yield gaps: how sustainable can we be? PLoS ONE 10, e0129487. doi: 10.1371/journal.pone.0129487
Pretty, J., and Bharucha, Z. P. (2014). Sustainable intensification in agricultural systems. Ann. Bot. 114, 1571–1596. doi: 10.1093/aob/mcu205
Ranst, E. V., Tang, H., Groenemam, R., and Sinthurahat, S. (1996). Application of fuzzy logic to land suitability for rubber production in peninsular Thailand. Geoderma 70, 1–19. doi: 10.1016/0016-7061(95)00061-5
Rhoades, J. D. (2018). “Salinity: electrical conductivity and total dissolved solids,” in SSSA Book Series (Soil Science Society of America, American Society of Agronomy), eds D. L. Sparks, A. L. Page, P. A. Helmke, R. H. Loeppert, P. N. Soltanpour, M. A. Tabatabai, et al. (Madison, WI: American Society of Agronomy), 417–435. doi: 10.2136/sssabookser5.3.c14
Sanchez, P. A. (2002). Soil fertility and hunger in Africa. Science 295, 2019–2020. doi: 10.1126/science.1065256
Sanchez, P. A., Shepherd, K. D., Soule, M. J., Place, F. M., Buresh, R. J., Izac, A.-M. N., et al. (1997). Soil fertility replenishment in Africa: an investment in natural resource capital. Replenishing Soil Fertil. Afr. 51, 1–46. doi: 10.2136/sssaspecpub51.c1
Schiefer, J., Lair, G. J., and Blum, W. E. (2016). Potential and limits of land and soil for sustainable intensification of European agriculture. Agric. Ecosyst. Environ. 230, 283–293. doi: 10.1016/j.agee.2016.06.021
Schut, M., van Asten, P., Okafor, C., Hicintuka, C., Mapatano, S., Nabahungu, N. L., et al. (2016). Sustainable intensification of agricultural systems in the Central African highlands: the need for institutional innovation. Agric. Syst. 145, 165–176. doi: 10.1016/j.agsy.2016.03.005
Soil Survey Staff (2006). Natural Resources Conservation Service, United States Department of Agriculture. Keys to Soil Taxonomy. Dublin, VA: Pocahontas Press Blacksburg.
Soil Survey Staff (2014). Keys to Soil Taxonomy, 12 th ed. Washington, DC: USDA-Natural Resources Conservation Service.
Stoorvogel, J. J., Smaling, E. M. A., and Janssen, B. H. (1993). Calculating soil nutrient balances in Africa at different scales. Fertil. Res. 35, 227–235. doi: 10.1007/BF00750641
Storie, R., Weir, W., and Powers, W. (1976). Discussion of Storie on land classification? Trans. Am. Soc. Civil Eng. 108, 672–675. doi: 10.1061/TACEAT.0005658
Sys, C. (1985). Evaluation of the Physical Environment for Rice Cultivation. IRRI (1985) Soil Physics and Rice. Los Banos: International Rice Research Institute, 31–44.
Sys, C., Van Ranst, E., Debaveye, J., and Beernaert, F. (1991). Land Evaluation: Part I. Principles in Land Evaluation and Crop Production Calculations, Part II. Methods in Land Evaluation, Part III. Crop Requirements. Brussels: General Administration for Development Cooperation.
Sys, C., Van Ranst, E., Debaveye, J., and Beernaert, F. (1993). Land Evaluation. Part III: Crop requirements. Agricultural Publications n° 7, G.A.D.C. Brussels: UGent Publication, 191.
Tetteh, F. M., Ennim, S. A., Issaka, R. N., Buri, M., Ahiabor, B. A. K., Fening, J. O., et al. (2018). “Fertilizer recommendation for maize and cassava within the breadbasket zone of Ghana,” in Improving the Profitability, Sustainability and Efficiency of Nutrients Through Site Specific Fertilizer Recommendations in West Africa Agro-Ecosystems, eds A. Bationo, D. Ngaradoum, and S. Youl (Berlin: Springer International Publishing), 161–184. doi: 10.1007/978-3-319-58792-9_10
Tetteh, F. M., Quansah, G. W., Frempong, S. O., Nurudeen, A. R., Atakora, W. K., Opoku, G., et al. (2017). “Optimizing fertilizer use within the context of integrated soil fertility management in Ghana,” in Fertilizer Use Optimization in Sub-Saharan Africa, eds C. S. Wortmann, and K. R. Sones (Oxfordshire: CABI), 67–81. doi: 10.1079/9781786392046.0067
Thomas, G. (1983). Exchangeable Cations. Madison, WI: American Society of Agronomy, Soil Science Society of America, 159–165. doi: 10.2134/agronmonogr9.2.2ed.c9
Thomas, G., Sparks, D., Page, A., Helmke, P., Loeppert, R., Soltanpour, P., et al. (1996). Soil pH and Soil Acidity. Madison, WI: Soil Science Society of America, American Society of Agronomy, 475–490.
United Nations (2016). Transforming Our World: The 2030 Agenda for Sustainable Development. Tech. rep. New York, NY: Department of Economic and Social Affairs, Sustainable Development, UN.
van Bussel, L. G., Grassini, P., Wart, J. V., Wolf, J., Claessens, L., Yang, H., et al. (2015). From field to atlas: upscaling of location-specific yield gap estimates. Field Crops Res. 177, 98–108. doi: 10.1016/j.fcr.2015.03.005
van Ittersum, M. K., Cassman, K. G., Grassini, P., Wolf, J., Tittonell, P., Hochman, Z., et al. (2013). Yield gap analysis with local to global relevance—a review. Field Crops Res. 143, 4–17. doi: 10.1016/j.fcr.2012.09.009
van Ittersum, M. K., van Bussel, L. G. J., Wolf, J., Grassini, P., van Wart, J., Guilpart, N., et al. (2016). Can sub-Saharan Africa feed itself? Proc. Nat. Acad. Sci. 113, 14964–14969. doi: 10.1073/pnas.1610359113
Vanlauwe, B., Six, J., Sanginga, N., and Adesina, A. A. (2015). Soil fertility decline at the base of rural poverty in sub-Saharan Africa. Nat. Plants 1, 15101. doi: 10.1038/nplants.2015.101
Wambeke, A. V. (1974). Management Properties of Ferralsols (FAO Soils Bulletin; 23). Rome: Food and Agriculture Organization.
Wang, Q., Wang, S., He, T., Liu, L., and Wu, J. (2014). Response of organic carbon mineralization and microbial community to leaf litter and nutrient additions in subtropical forest soils. Soil Biol. Biochem. 71, 13–20. doi: 10.1016/j.soilbio.2014.01.004
Yimer, F., Ledin, S., and Abdelkadir, A. (2007). Changes in soil organic carbon and total nitrogen contents in three adjacent land use types in the Bale mountains, south-eastern highlands of Ethiopia. For. Ecol. Manage. 242, 337–342. doi: 10.1016/j.foreco.2007.01.087
Keywords: land suitability, soil fertility, sustainable intensification, soil resilience, maize, Ghana
Citation: Awoonor JK, Dogbey BF and Quansah GW (2023) Soil suitability assessment for sustainable intensification of maize production in the humid Savannah of Ghana. Front. Sustain. Food Syst. 7:1094290. doi: 10.3389/fsufs.2023.1094290
Received: 09 November 2022; Accepted: 26 June 2023;
Published: 31 July 2023.
Edited by:
Folorunso Mathew Akinseye, International Crops Research Institute for the Semi-Arid Tropics (ICRISAT), KenyaReviewed by:
Luciano Gebler, Brazilian Agricultural Research Corporation (EMBRAPA), BrazilCopyright © 2023 Awoonor, Dogbey and Quansah. This is an open-access article distributed under the terms of the Creative Commons Attribution License (CC BY). The use, distribution or reproduction in other forums is permitted, provided the original author(s) and the copyright owner(s) are credited and that the original publication in this journal is cited, in accordance with accepted academic practice. No use, distribution or reproduction is permitted which does not comply with these terms.
*Correspondence: Johnny Kofi Awoonor, amthd29vbm9yQGNzaXIub3JnLmdo
Disclaimer: All claims expressed in this article are solely those of the authors and do not necessarily represent those of their affiliated organizations, or those of the publisher, the editors and the reviewers. Any product that may be evaluated in this article or claim that may be made by its manufacturer is not guaranteed or endorsed by the publisher.
Research integrity at Frontiers
Learn more about the work of our research integrity team to safeguard the quality of each article we publish.