- 1Tecnológico Nacional de México/ I.T. Mérida, Departamento de Ingeniería Química y Bioquímica, Mérida, México
- 2Universidad Autónoma de Yucatán, Facultad de Ingeniería Química, Laboratorio de Biotecnología, Mérida, México
- 3Tecnológico Nacional de México/ I.T. Zacatepec, Departamento de Ingeniería Química y Bioquímica, Zacatepec, México
The wastes generated by the shrimp industry are approximately between 50 and 60% of the catch volume. These residues such as head, viscera, and shell are potential pollutants if they are not treated for proper disposal. One way to solve this problem is to use the residues as functional food ingredients. In this regard, shrimp residues are rich in chitin, the second most abundant biopolymer on the planet after cellulose. Chitin is composed of N-acetyl glucosamine, a molecule used as a sweetener in the food industry and as an aid in the treatment of coronary diseases and gonarthrosis. N-acetyl glucosamine can be obtained by the hydrolysis of colloidal chitin using chemical or enzymatic methods; however, chemical methods are associated with pollution. In this study, we determined the hydrolysis conditions of shrimp colloidal chitin for obtaining N-acetyl glucosamine, using the extracellular enzymes produced by a marine bacterium isolated in the coastal zone of Progreso, Yucatan, Mexico. The best N-acetyl glucosamine yield obtained was 2.65%, using 10 mg/mL colloidal chitin, at 60°C, and pH 8.9 with 3.5% NaCl.
1. Introduction
In Mexico, the shrimp industry ranks second in the national fishing production and first from an economic perspective. The historical production of shrimp in Mexico from 2011 to 2020, as presented in Table 1, indicates that the average production is 1,67,617 tons per year (Anuario Estadístico de Acuacultura y Pesca, 2020). Considering that the amount of waste is approximately between 40 and 66% of the total weight (50% average) (Xu et al., 2013), the potential volume of solid waste generated during shrimp processing is 1,01,020 tons per year. Solid shrimp wastes contain between 15 and 40% chitinous fraction (Yan and Chen, 2015); therefore, the estimated amount of chitin ranges from 15,153 to 40,408 tons per year.
Despite the large volume of solid waste generated from shrimp, these are not fully exploited, and only a small proportion is dried and added to agricultural soils to improve consistency or added to balanced animal food (Kandra et al., 2012).
Diverse materials of industrial interest can be recovered from these residues (Figure 1). Among these compounds, there are β-carotenes, which can be used in the food industry as a pigment or as an antioxidant agent (Liñan-Cabello et al., 2002; Sachindra and Bhaskar, 2008; Pereira da Costa and Campos Miranda-Filho, 2020). The muscle proteins that remain attached to the cephalothorax can be recovered by enzymatic hydrolysis and used as a food supplement or in the formulation of culture media (Pereira et al., 2022). Chitin can be used as a healing agent or in soil improvement, as well as in the production of chitosan, N-acetyl-glucosamine (GlcNAc), or glucosamine. Structurally, chitin is a polymer with unbranched chains of GlcNAc units linked by β1–4 glycosidic bonds. It is present in the cuticle of insects and in the shell of crustaceans such as shrimp, lobster, and crab (Nagpure et al., 2014).
Chitinases are glycosyl hydrolases grouped in families 18, 19, and 20. Family 18 includes enzymes from a wide variety of prokaryotes and eukaryotes, while family 19 holds enzymes from higher plants. Gram-positive bacteria such as Streptomyces, and some of viral origin to produce free GlcNAc. Family 20 includes chitobiases responsible for the hydrolysis of GlcNAc dimers (Oyeleye and Normi, 2018).
GlcNAc can be obtained by chemical hydrolysis; however, this method requires a purification process to remove unwanted compounds generated during the hydrolysis. The cost of chemical hydrolysis is high, and the yield is low. In this sense, enzymatic hydrolysis becomes relevant as an environmentally friendly method (Cardozo et al., 2019).
The total enzymatic hydrolysis of chitin to GlcNAc is carried out by a chitinolytic system that acts synergistically and consecutively (Patil et al., 2000). This enzymatic system comprises endochitinases that produce GlcNAc multimers, exochitinases, which catalyze the progressive release of chitobiose (low molecular weight soluble dimer), and chitobiases responsible for the hydrolysis of chitobiose to the chitin monomer, GlcNAc (Souza et al., 2003).
Enzymatic methods reported to produce GlcNAc are presented in Table 2. Despite the fact that different substrates were used in these studies, the use of colloidal chitin is promising because the amorphous structure facilitates the access of the enzymes, increasing the rate of hydrolysis.
Chitinolytic enzymes are widely distributed in nature and play a key role in the degradation of chitin (Nagpure et al., 2014; Oyeleye and Normi, 2018). Therefore, chitinases are a viable option for the transformation of solid shrimp waste into compounds of pharmaceutical or food interest, such as low molecular weight oligosaccharides and GlcNAc (Suresh, 2012).
GlcNAc is a biocompatible, innocuous, and biodegradable compound used in the pharmaceutical and food industries (Table 3) (Das et al., 2019; Mojumdar et al., 2019; Shintani, 2019). This molecule can be used in the treatment of diseases such as osteoarthritis and Bowell's inflammatory disease and as a potential prebiotic and sweetener (Bode, 2012; Cardozo et al., 2019; Verspecht et al., 2021).
In this sense, our group has been working in the use of the extracellular chitinolytic enzymes produced by a halophilic moderate bacterium PRO-III 115, isolated in the coastal area of the Progreso Port, Yucatan, México (21° 16' 14” N; 89° 41' 55”). The objective of this study was to establish the conditions to produce GlcNAc using the colloidal chitin, produced from solid shrimp waste, as a substrate and the extracellular enzymes produced by PRO-III 115.
2. Materials and methods
2.1. Microorganism
Moderate halophilic bacteria PRO-III-115 isolated in the port of Progreso, Yucatan, México was used.
2.2. Chitin obtention
The shrimp waste, used for the chitin recovery and later for the colloidal chitin production, was collected in Lerma, Campeche, México.
Shrimp wastes were dried in the sun for 5 days, ground using a hammer mill, and sieved through a 20-mesh screen (particle size: 0.9 mm). The retained material was used for the demineralization, deproteinization, depigmentation, and bleaching process (No et al., 1989).
2.2.1. Demineralization
The removal of minerals associated with the chitin matrix was performed by soaking the shrimp shell fraction retained in the 20 meshes, in a 1.0 N HCl solution for 30 min at room temperature, in a proportion 1:15 (w/v). At the end of this time, the material was washed with demineralized water to eliminate the excess acid, left in deionized water for 24 h, filtered, and dried at 70°C for 24 h.
2.2.2. Deproteinization
Deproteinization of the demineralized material was carried out using a 3.5% NaOH solution for two h at 65°C. The solid:aqueous ratio used was 1:10 (w/v). The obtained material was subjected to successive washings using demineralized water to eliminate the excess alkali.
2.2.3. Depigmentation
The pigments were extracted by the Soxhlet method using acetone as the solvent. At the end of the process, the material was dried at an environmental temperature in an extraction hood.
2.2.4. Bleaching
The material was bleached using sodium hypochlorite solution at 0.315% and the solid:solution ratio of 1:10 (w/v) for 5 min. The material obtained was subjected to successive washings with demineralized water to eliminate residual chlorine.
2.3. Colloidal chitin preparation
Colloidal chitin was prepared according to Monreal and Reese (1969) using phosphoric acid at 85% with a ratio of 1:10 (chitin/phosphoric acid) under refrigerated conditions for 48 h. After this time, the colloidal chitin was recovered by precipitation with an excess of demineralized water and subjected to successive washings until a neutral pH was achieved. The excess water was drained to obtain colloidal chitin. Finally, the moisture was determined, and the material was kept refrigerated until use.
2.4. Culture medium
The culture medium used for the conservation and propagation of the PRO-III-115 strain was composed of bacteriological agar (2%), yeast extract (0.5%), NaCl (3.5%), and colloidal chitin (1.0%) at pH 7.9 (Carroad and Tom, 1978). The same culture medium, without the addition of agar, was used for the growth and production of chitinolytic activity.
2.5. Inoculum preparation
The inoculum used to produce chitinolytic enzymes was prepared propagating the PRO-III-115 bacteria in Petri dishes with the propagation medium and incubated at 30°C for 15 days. After this time, a hydrolysis zone of colloidal chitin became clear. Cells obtained were suspended in a NaCl (3.554%) solution, and the cell suspension had an optical density of 0.5 at 360 nm.
2.6. Fermentation system for the extracellular chitinolytic enzymes production
The free cell supernatant with chitinolytic activity was produced in 250-mL Erlenmeyer flasks with 50 mL of the medium. Each flask was inoculated with 1.0 mL of the PRO-II-115 cell suspension. The flasks were incubated at 30°C and 200 rpm for 7 days. After this time, the culture medium was centrifuged at 12,000 rpm for 7 min at room temperature to remove the cells and residual colloidal chitin. The obtained free cell supernatant was stored at 4°C until use.
2.7. Chitinolytic assay
The chitinolytic activity of the free cell supernatant was determined according to Monreal and Reese (1969). Reaction mixtures containing 0.5 mL of the cell-free supernatant and 1 mL of 1% colloidal chitin suspension in Trizma-base buffer (0.5 M, pH 7.9) were added to 18 × 175 mm tubes. All the assays were incubated for 1 h at 50°C.
2.8. Factorial design 33 for the determination of hydrolysis conditions of colloidal chitin to obtain N-acetyl-glucosamine
Colloidal chitin hydrolysis conditions, using the free cell supernatant produced by the PRO-III-115 strain, were determined using colloidal chitin (1%), and a 33 factorial design was based on the fixed effects model, as shown in Table 4. The central points used were 50°C, pH 7.9, and 0.05 M of Trizma-base buffer. To evaluate if NaCl was an essential factor for the hydrolysis, a second experiment was conducted with the same factorial design, evaluating the effect of pH, temperature, buffer molarity, and suppressing NaCl.
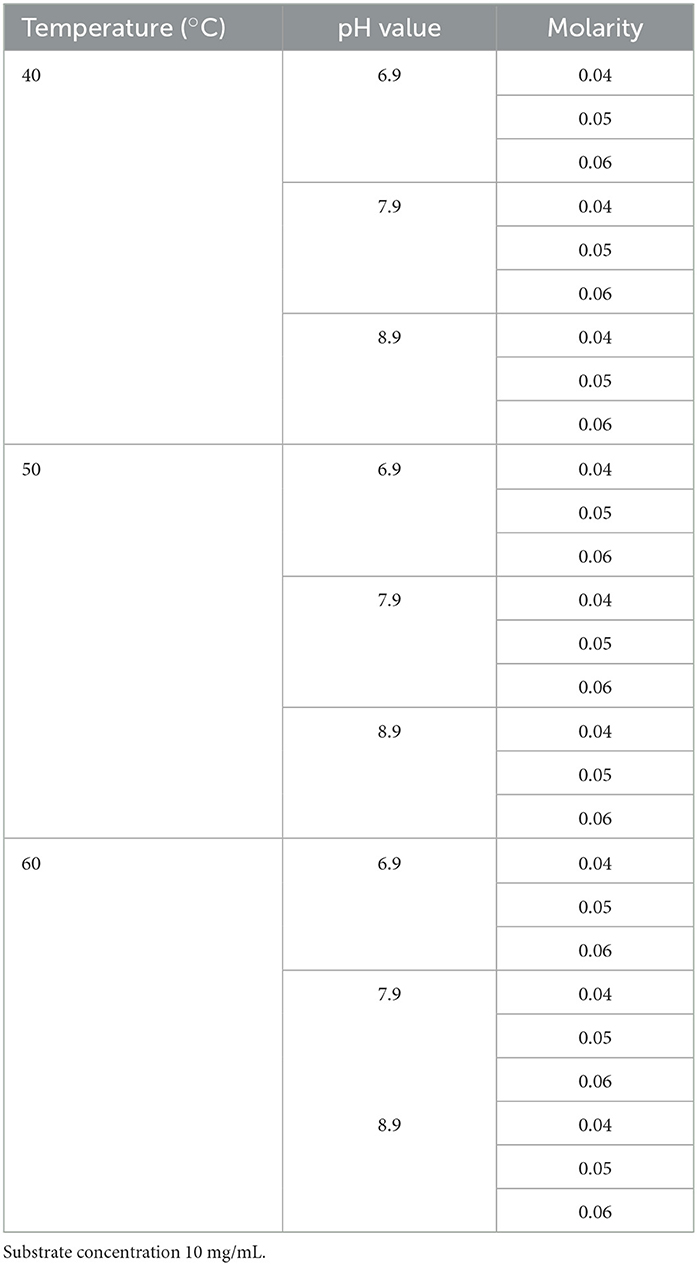
Table 4. Factorial design 33 to evaluate conditions for colloidal chitin hydrolysis to obtain N-Acetyl-glucosamine.
2.9. Reducing sugar quantification
The GlcNAc released was quantified as reducing sugar according to Miller (1959). For each assay, 3.0 mL of DNS reagent (3,5-dinitrosalicylic acid) was added, and the tubes were boiled in a water bath for 5 min. Subsequently, reactions were cooled and diluted to obtain a final volume of 20.0 mL. The optical density was determined at 550 nm, and the concentration was calculated using a GlcNAc concentrations standard curve.
2.10. Statistical analysis
Statistical analysis was performed with SPSS software (IBM, 2007) using the analysis of variance (ANOVA) technique. All experiments were conducted in duplication using two replicas. The p-value was 0.05.
3. Results
3.1. Colloidal chitin hydrolysis conditions in the presence of NaCl
Figure 2 shows the effect of the evaluated factors on GlcNAc production in the presence of NaCl. The highest GlcNAc concentration obtained was 265 μg/mL in the system with a temperature of 60°C, pH value of 8.9, and 0.04 M Trizma-base buffer. An increment in the concentration of the buffer (0.05 M) decreased the production of GlcNAc obtaining 216.7 μg /mL. Using a more acidic pH (6.9) and higher concentration of the buffer (0.06 M) increased the amount of GlcNAc hydrolyzed (218.3 μg/mL); however, this value was below the highest obtained. In addition, the yield associated with the highest concentration of GlcNAc were calculated, being 0.027 mg GlcNAc/mg with a productivity of 0.265 mg/mL*h, respectively.
3.2. Colloidal chitin hydrolysis conditions in the absence of NaCl
The effect of pH, temperature, and buffer molarity without NaCl is presented in Figure 3. The GlcNAc concentrations were lower than that obtained in the presence of NaCl. The conditions that favor the chitin hydrolysis with NaCl were also favorable without NaCl. In the system with 60°C, a pH of 8.9 and a 0.04 M Trizma-base buffer were the highest values of GlcNAc that were obtained (178.3 μg/mL). Increasing the buffer concentration to 0.06 M of Trizma-base buffer negatively affected the hydrolysis of chitin (170 μg of GlcNAc/mL). A temperature decrement (50°C) with a higher concentration of buffer of 0.05 M decreased the concentration to a value of 166.7 μg GlcNAc/mL.
3.3. ANOVA analysis
ANOVA with 95% confidence indicated that temperature was the factor that had a significant influence on chitin hydrolysis. In this analysis, it was also found that no double interaction, temperature–pH, temperature–molarity, and pH–molarity had an influence on the GlcNAc concentration obtained. Similarly, the interaction between the three factors had no significant influence on the enzymatic hydrolysis system.
4. Discussion
Table 5 shows the concentration of GlcNAc reported by different authors using enzymes from different microbial origins. The yield and productivity of GlcNAc were calculated to compare with the highest data obtained in this study.
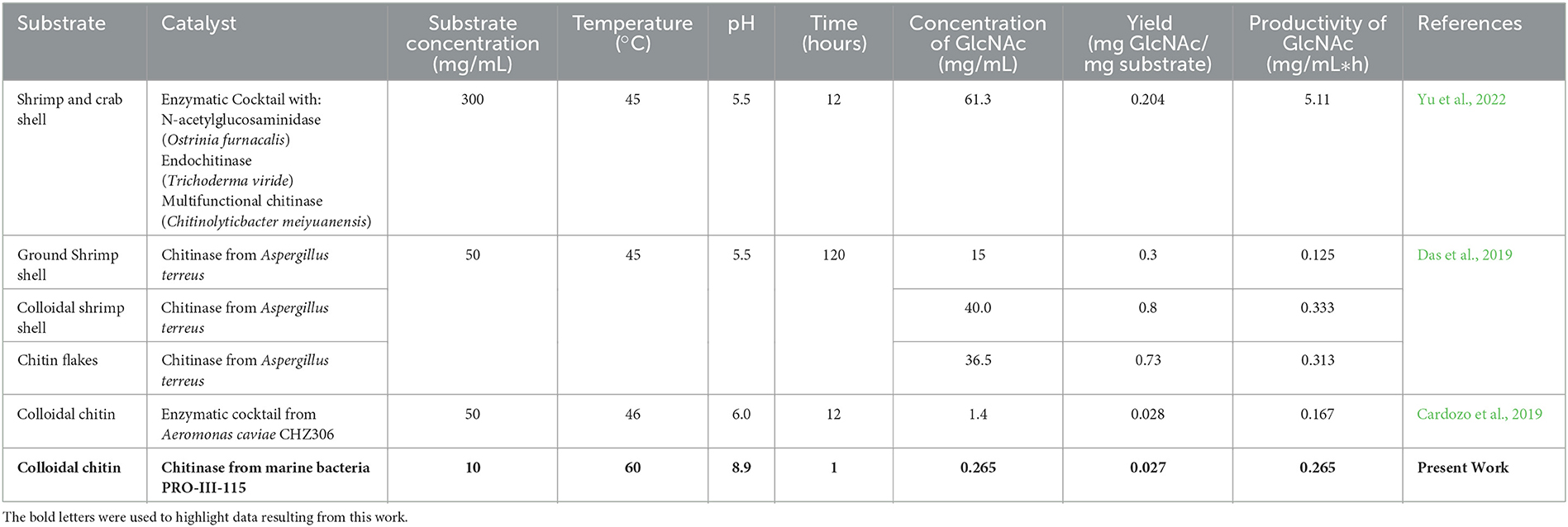
Table 5. N-Acetyl-glucosamine production by enzymatic process, using the crustacean shell or derivates from them.
Yu et al. (2022) used a mechano-enzymatic treatment for the hydrolysis of shrimp and crab shells. The milling process, associated with a fungal enzymatic cocktail, produced the highest concentration of GlcNAc (61.3 mg/ml) and productivity (5.11 mg/mL*h) among the analyzed studies. This finding suggests that the particle size of the chitinous material could have a strong impact on the hydrolytic process since it generates a larger contact surface between the colloidal chitin and enzymes. However, the yield obtained was low reaching 0.204 mg GlcNAc/mg substrate.
Das et al. (2019) evaluated three different substrates: ground shrimp shell, colloidal shrimp shell, and chitin flakes. By using the ground shrimp shell, 15 mg/mL of GlcNAc was obtained, and this behavior may be associated with the fact that this substrate contains calcium carbonate (CaCO3) and has a highly crystalline structure (Kurita, 2006; Joseph et al., 2020) that results in a poor release of GlcNAc since both conditions hinder the diffusion of enzymes through the matrix of the material. On the other hand, by using the colloidal shrimp shell as a substrate, it managed to release the highest concentration of GlcNAc, i.e., 40 mg/mL, and this may be caused due to this material having an amorphous structure (Sampath et al., 2022) which facilitates the access of the enzymes to the material, causing more efficient hydrolysis. Finally, when chitin flakes were used as a substrate, 36.5 mg/mL of GlcNAc was released, and this may be caused because this material no longer contains CaCO3 its crystalline structure is not entirely accessible as in the case of colloidal chitin (Joseph et al., 2020). These results suggest that the pretreatment of chitinous material has a strong impact on the release of GlcNAc. With regard to productivity, the obtained values were 0.125, 0.333, and 0.313 GlcNAc mg/mL*h, respectively, using a hydrolysis time of 120 h.
In this study, 0.265 mg/mL of GlcNAc was produced with a yield of 0.027 mg GlcNAc/mg substrate and a productivity of 0.265 mg/mL*h of GlcNAc using colloidal chitin as the substrate in 1 h (Cardozo et al., 2019). Using the same substrate, we obtained a concentration of 1.4 mg/ml of GlcNAc but a yield (0.028 mg GlcNAc/mg substrate) and productivity (0.167 mg/mL*h of GlcNAc) values. The differences can be attributed to the amount of substrate used by Cardozo et al. (2019), which was five times higher than our system.
The enzymatic production of GlcNAc from colloidal chitin suggests that the strain PRO-III-115 produces extracellular endochitinase, exochitinase, and chitobiose hydrolase enzymes. Furthermore, the highest concentration of GlcNAc was obtained in 1 h of processing, using a salinity close to marine water at alkaline pH (8.9) and 60°C. The NaCl presence incremented the GlcNAc production, suggesting that it may affect both the enzyme and the colloidal chitin structure. NaCl may affect the surface charges of chitin (Gonzalez-Davila and Millero, 1990), favoring the attachment of the enzymes; in addition, it has been found that the modification of the conditions of pH, temperature, ionic strength, and the speed of agitation has a strong impact on the adsorption capacity of these ions (Anastopoulos et al., 2017). This could explain why the marine bacterium PRO-III-115 and its extracellular enzymes are capable of catalyzing reactions under conditions of high ionic strength, alkaline pH, and high temperature. Furthermore, these conditions could prevent the proliferation of unwanted microorganisms during aseptic GlcNAc production.
Regarding the results obtained in this study, it should be considered that shrimp wastes used as the raw material for the production of colloidal chitin were collected in a shrimp processing plant; therefore, this material is a mixture of shrimp shells of different species and chemical composition. Given the chitin recovery process was carried out in a single condition, it is possible that the colloidal chitin produced contains particles with random chemical composition (particularly CaCO3). This factor is likely to have a steric impediment during the hydrolysis process, and it is possible that the values of the standard deviations in Figures 2, 3 are associated with this condition.
5. Conclusion
According to the ANOVA analysis, the factor that significantly changed the sugar-free titer was the temperature at 60°C with which the highest activity titer was obtained, i.e., 265 μg of GlcNAc/mL. The variance analysis also points out that the pH and the buffer's molarity did not significantly influence the chitinolytic activity present in the cell-free supernatant obtained with the bacteria PRO-III-115.
Since the filtrates with enzymatic activity were produced by a moderate halophilic marine bacterium, it is logical that the presence of NaCl in the hydrolysis system is essential for the chitinolytic enzymes produced by the PRO-III-115 strain to work more efficiently. This is reinforced by the fact that the chitinolytic activity in the absence of NaCl decreased by 33% in the system in which a temperature of 60°C, pH 8.90, and a 0.04 M Trizma-base buffer were used.
The aforementioned characteristics allow us to think about the possibility that the hydrolysis of colloidal chitin occurs under aseptic conditions, without the need to sterilize the substrate. These results, allow us to think about the possibility to design an enzymatic process in which colloidal chitin, obtained from solid shrimp waste, will be oriented to the production of GlcNAc.
Data availability statement
The original contributions presented in the study are included in the article/supplementary material, further inquiries can be directed to the corresponding author.
Author contributions
All authors listed have made a substantial, direct, and intellectual contribution to the work and approved it for publication.
Conflict of interest
The authors declare that the research was conducted in the absence of any commercial or financial relationships that could be construed as a potential conflict of interest.
Publisher's note
All claims expressed in this article are solely those of the authors and do not necessarily represent those of their affiliated organizations, or those of the publisher, the editors and the reviewers. Any product that may be evaluated in this article, or claim that may be made by its manufacturer, is not guaranteed or endorsed by the publisher.
References
Anastopoulos, I., Bhatnagar, A., Bikiaris, D., and Kyzas, G. (2017). Chitin adsorbents for toxic metals: A review. Int. J. Mol. Sci. 18, 114. doi: 10.3390/ijms18010114
Anderson, T., Zhou, S., Bergerud, B., Fosdick, L. E., Han, T., Vasina, J., et al. (2012). Glucosamine and N-acetylglucosamine compositions and methods of making the same fungal biomass. U.S. Patent 8,222,232.
Anuario Estadístico de Acuacultura y Pesca (2020). Anuario Estadístico de Acuacultura y Pesca. Available online at: https://www.gob.mx/conapesca/documentos/anuario-estadistico-de-acuacultura-y-pesca (accessed October 5, 2022).
Bode, L. (2012). Human milk oligosaccharides: every baby needs a sugar mama. Glycobiology 22, 1147–1162. doi: 10.1093/glycob/cws074
Cardozo, F. A., Facchinatto, W. M., Colnago, L. A., Campana-Filho, S. P., and Pessoa, A. (2019). Bioproduction of N-acetyl-glucosamine from colloidal α-chitin using an enzyme cocktail produced by Aeromonas caviae CHZ306. World J. Microbiol. Biotechnol. 35, 114. doi: 10.1007/s11274-019-2694-x
Carroad, P. A., and Tom, R. A. (1978). Bioconversion of shellfish chitin wastes: process conception and selection of microorganims. J. Food Sci. 43, 1158–1161. doi: 10.1111/j.1365-2621.1978.tb15259.x
Chen, J.-K., Shen, C.-R., Yeh, C.-H., Fang, B.-S., Huang, T.-L., and Liu, C.-L. (2011). N-acetyl glucosamine obtained from chitin by chitin degrading factors in Chitinbacter tainanesis. Int. J. Mol. Sci. 12, 1187–1195. doi: 10.3390/ijms12021187
Das, S., Dey, P., Roy, D., Maiti, M. K., and Sen, R. (2019). N-Acetyl-d-glucosamine production by a chitinase of marine fungal origin: a case study of potential industrial significance for valorization of waste chitins. Appl. Biochem. Biotechnol. 187, 407–423. doi: 10.1007/s12010-018-2822-3
Frisbie, D. D., Wayne McIlwraith, C., Kawcak, C. E., and Werpy, N. M. (2016). Efficacy of intravenous administration of hyaluronan, sodium chondroitin sulfate, and N-acetyl-d-glucosamine for prevention or treatment of osteoarthritis in horses. Am. J. Vet. Res. 77, 1064–1070. doi: 10.2460/ajvr.77.10.1064
Gonzalez-Davila, M., and Millero, F. J. (1990). The adsorption of copper to chitin in seawater. Geochim. Cosmochim. Acta 54, 761–768. doi: 10.1016/0016-7037(90)90371-Q
György, P., Villanova, P., Kuhn, R., and Zilliken, F. (1955). Food products containing glucosides of N-Acetyl-D-glucosamine. US Patent Office.
Halim, Y., Devianita, D., Hardoko, H., Handayani, R., and Soedirga, L. C. (2022). Immobilization of providencia stuartii cells in pumice stone and its application for N-acetylglucosamine production. Food Technol. Biotechnol. 60, 4–10. doi: 10.17113/ftb.60.01.22.6994
Jiang, Z., Li, Z., Zhang, W., Yang, Y., Han, B., Liu, W., et al. (2018). Dietary Natural N-Acetyl-d-Glucosamine prevents bone loss in ovariectomized rat model of postmenopausal osteoporosis. Molecules 23, 2302. doi: 10.3390/molecules23092302
Joseph, B., Mavelil Sam, R., Balakrishnan, P.J, Maria, H., Gopi, S., et al. (2020). Extraction of nanochitin from marine resources and fabrication of polymer nanocomposites: recent advances. Polymers (Basel) 12, 1664. doi: 10.3390/polym12081664
Kandra, P., Challa, M. M., and Kalangi Padma Jyothi, H. (2012). Efficient use of shrimp waste: present and future trends. Appl. Microbiol. Biotechnol. 93, 17–29. doi: 10.1007/s00253-011-3651-2
Kim, C., Shores, L., Guo, Q., Aly, A., Jeon, O. H., Kim, D. H., et al. (2016). Electrospun microfiber scaffolds with anti-inflammatory tributanoylated n-acetyl-d-glucosamine promote cartilage regeneration. Tissue Eng. Part A 22, 689–697. doi: 10.1089/ten.tea.2015.0469
Kurita, K. (2006). Chitin and chitosan: functional biopolymers from marine crustaceans. Marine Biotechnol. 8, 203–226. doi: 10.1007/s10126-005-0097-5
Liñan-Cabello, M. A., Paniagua-Michel, J., and Hopkins, P. M. (2002). Bioactive roles of carotenoids and retinoids in crustaceans. Aquac. Nutr. 8, 299–309. doi: 10.1046/j.1365-2095.2002.00221.x
Miller, G. L. (1959). Use of dinitrosalicylic acid reagent for determination of reducing Sugar. Anal. Chem. 31, 426–428. doi: 10.1021/ac60147a030
Mojumdar, A., Upadhyay, A. K., Raina, V., and Ray, L. (2019). A simple and rapid colorimetric method for the estimation of chitosan produced by microbial degradation of chitin waste. J. Microbiol. Methods 158, 66–70. doi: 10.1016/j.mimet.2019.02.001
Monreal, J., and Reese, E. T. (1969). The chitinase of Serratia marcescens. Can J. Microbiol. 15, 689–696. doi: 10.1139/m69-122
Nagpure, A., Choudhary, B., and Gupta, R. K. (2014). Chitinases: in agriculture and human healthcare. Crit. Rev. Biotechnol. 34, 215–232. doi: 10.3109/07388551.2013.790874
Naseem, S., and Konopka, J. B. (2015). N-acetylglucosamine regulates virulence properties in microbial pathogens. PLoS Pathog. 11, e1004947. doi: 10.1371/journal.ppat.1004947
No, H. K., Meyers, S. P., and Lee, K. S. (1989). Isolation and characterization of chitin from crawfish shell waste. J. Agric. Food Chem. 37, 575–579. doi: 10.1021/jf00087a001
Oyeleye, A., and Normi, Y. M. (2018). Chitinase: Diversity, limitations, and trends in Engineering for suitable applications. Biosci. Rep. 38, BSR2018032300. doi: 10.1042/BSR20180323
Panchaphanpong, J., Asawakarn, T., and Pusoonthornthum, R. (2011). Effects of oral administration of N-acetyl-. Am J. Vet. Res. 72, 843–850. doi: 10.2460/ajvr.72.6.843
Patil, R. S., Ghormade, V., and Deshpande, M. V. (2000). Chitinolytic enzymes: an exploration. Enzyme. Microb. Technol. 26, 473–483. doi: 10.1016/S0141-0229(00)00134-4
Pereira da Costa, D., and Campos Miranda-Filho, K. (2020). The use of carotenoid pigments as food additives for aquatic organisms and their functional roles. Rev. Aquac. 12, 1567–1578. doi: 10.1111/raq.12398
Pereira, N. D. L. Á., Fangio, M. F., Rodriguez, Y. E., Bonadero, M. C., Harán, N. S., and Fernández-Gimenez, A. V. (2022). Characterization of liquid protein hydrolysates shrimp industry waste: Analysis of antioxidant and microbiological activity, and shelf life of final product. J. Food Process. Preserv. 46, 15526. doi: 10.1111/jfpp.15526
Richter, J., Capková, K., Hríbalová, V., Vannucci, L., Danyi, I., Malý, M., et al. (2014). Collagen-induced arthritis: severity and immune response attenuation using multivalent N-acetyl glucosamine. Clin. Exp. Immunol. 177, 121–133. doi: 10.1111/cei.12313
Rivlin, M., and Navon, G. (2016). Glucosamine and N-acetyl glucosamine as new CEST MRI agents for molecular imaging of tumors. Sci. Rep. 6, 32648. doi: 10.1038/srep32648
Rogers, B. D., Fosdick, L. E., and Bohlmann, J. A. (2004). N-acetyl-D-glucosamine (NAG) supplemented food products and beverages. United States patent application US 10/533,414.
Sachindra, N. M., and Bhaskar, N. (2008). In vitro antioxidant activity of liquor from fermented shrimp biowaste. Bioresour. Technol. 99, 9013–9016. doi: 10.1016/j.biortech.2008.04.036
Salvatore, S., Heuschkel, R., Tomlin, S., Davies, S. E., Edwards, S., Walker-Smith, J. A., et al. (2000). A pilot study of N-acetyl glucosamine, a nutritional substrate for glycosaminoglycan synthesis, in paediatric chronic inflammatory bowel disease. Aliment Pharmacol. Ther. 14, 1567–1579. doi: 10.1046/j.1365-2036.2000.00883.x
Sampath, L., Ngasotter, S., Layana, P., Balange, A. K., Nayak, B. B., and Xavier, K. A. M. (2022). Effect of chemical treatment duration on physicochemical, rheological, and functional properties of colloidal chitin. Food Hydrocoll. Health 2, 100091. doi: 10.1016/j.fhfh.2022.100091
Shintani, T. (2019). Food industrial production of monosaccharides using microbial, enzymatic, and chemical methods. Fermentation 5, 47. doi: 10.3390/fermentation5020047
Souza, R. F., Gomes, R. C., Coelho, R. R. R., Alviano, C. S., and Soares, R. M. A. (2003). Purification and characterization of an endochitinase produced by Colletotrichum gloeosporioides. FEMS Microbiol. Lett. 222, 45–50. doi: 10.1016/S0378-1097(03)00220-9
Suresh, P. V. (2012). Biodegradation of shrimp processing bio-waste and concomitant production of chitinase enzyme and N-acetyl-D-glucosamine by marine bacteria: production and process optimization. World J. Microbiol. Biotechnol. 28, 2945–2962. doi: 10.1007/s11274-012-1106-2
Tago, K., Naito, Y., Nagata, T., Morimura, T., Furuya, M., Seki, T., et al. (2007). A ninety-day feeding, subchronic toxicity study of oligo-N-acetylglucosamine in Fischer 344 rats. Food Chem. Toxicol. 45, 1186–1193. doi: 10.1016/j.fct.2006.12.027
Temple-Wong, M. M., Raleigh, A. R., Frisbie, D. D., Sah, R. L., and McIlwraith, C. W. (2021). Effects of an articular cartilage lubrication with a viscosupplement in vitro and in vivo following osteochondral fractures in horses. Am. J. Vet. Res. 82, 611–618. doi: 10.2460/ajvr.82.8.611
Verspecht, T., Van Holm, W., Boon, N., Bernaerts, K., Daep, C. A., Zayed, N., et al. (2021). Comparison of the modulatory effects of three structurally similar potential prebiotic substrates on an in vitro multi-species oral biofilm. Sci. Rep. 11, 15033. doi: 10.1038/s41598-021-94510-z
Vordenbäumen, S., Lueking, A., Budde, P., Zucht, H.-D., Goehler, H., Brinks, R., et al. (2016). Sequential high-content profiling of the IgG-autoantibody repertoire reveals novel antigens in rheumatoid arthritis. Arthr. Res. Ther. 18, 235. doi: 10.1186/s13075-016-1135-6
Xu, Y., Bajaj, M., Schneider, R., Grage, S. L., Ulrich, A. S., Winter, J., et al. (2013). Transformation of the matrix structure of shrimp shells during bacterial deproteination and demineralization. Microb. Cell Fact. 12, 90. doi: 10.1186/1475-2859-12-90
Yan, N., and Chen, X. (2015). Sustainability: Don't waste seafood waste. Nature 524, 155–157. doi: 10.1038/524155a
Keywords: chitin, colloidal chitin, N-acetyl glucosamine, hydrolysis, marine bacteria
Citation: Rivera-Solís RA, Granados-Baeza MJ, Solís-Pereira SE, Lizama-Uc G, Magaña-Ortiz D, Sánchez-González MN, Rojas-Herrera RA and Rivera-Muñoz G (2023) Establishment of the colloidal chitin enzymatic hydrolysis conditions to obtain N-acetyl glucosamine. Front. Sustain. Food Syst. 7:1077429. doi: 10.3389/fsufs.2023.1077429
Received: 23 October 2022; Accepted: 08 March 2023;
Published: 31 March 2023.
Edited by:
Nasser Al-Habsi, Sultan Qaboos University, OmanReviewed by:
Yingying Wang, Nanjing Tech University, ChinaPaz Salgado-Cruz, National Polytechnic Institute (IPN), Mexico
Maha Al-Khalili, Sultan Qaboos University, Oman
Copyright © 2023 Rivera-Solís, Granados-Baeza, Solís-Pereira, Lizama-Uc, Magaña-Ortiz, Sánchez-González, Rojas-Herrera and Rivera-Muñoz. This is an open-access article distributed under the terms of the Creative Commons Attribution License (CC BY). The use, distribution or reproduction in other forums is permitted, provided the original author(s) and the copyright owner(s) are credited and that the original publication in this journal is cited, in accordance with accepted academic practice. No use, distribution or reproduction is permitted which does not comply with these terms.
*Correspondence: Gerardo Rivera-Muñoz, Z2VyYXJkby5ybUBtZXJpZGEudGVjbm0ubXg=
†These authors have contributed equally to this work