- Microbiology Research Laboratory, Department of Basic Sciences, Dr. Y. S. Parmar University of Horticulture and Forestry, Solan, Himachal Pradesh, India
Introduction: The current need of the food industry is to develop a safe packaging system that maintains the quality of food and prevents its spoilage. Food safety techniques improvised using functional nanoparticles minimize the chances of spoilage by maintaining moisture stability, mechanical strength, and durability and ensuring product safety. In the present study, we synthesized silver nanoparticles using purified bacteriocins obtained from probiotics. Bacteriocin-synthesized AgNPs are eco-friendly and secure packaging solutions that can be utilized in the packaging industry for the storage of food products.
Methods: Crude, partially purified and purified bacteriocin was obtained from three potential probiotic isolates, i.e., Lactobacillus pentosus S6 (KU92122), Lactobacillus crustorum F11 (KT865221) and Lactobacillus spicheri G2 (JX481912). The antimicrobial efficacy of bacteriocin was tested against two food-borne spoilage-causing pathogens, i.e., Bacillus cereus and Staphylococcus aureus. The purified bacteriocin obtained was used for the synthesis of AgNPs. The synthesized AgNPs were characterized using UV-vis spectroscopy, TEM, and SEM techniques. The AgNPs were used for coating cellulose paper. The coated paper was characterized using SEM and was used for the storage of tomato fruit.
Results and discussion: The purified bacteriocin obtained was used for the synthesis of AgNPs. The formation of AgNPs was confirmed by using UV-vis spectroscopy, which showed maximum absorption at 450 nm. Furthermore, we confirm shape and morphology by using Scanning Electron Microscopy (SEM). Transmission Electron Microscopy (TEM) analysis showed the mean size of synthesized AgNPs in the range of 5–20 nm. Bacteriocin-synthesized AgNPs were then used for the coating of cellulose paper with the main motive to avoid spoilage and enhance the shelf stability of tomato fruit during storage. SEM analysis confirmed the coating of AgNPs in the cellulose paper. The enhanced antimicrobial efficacy of different treatments coated paper was observed against B. cereus and S. aureus. Out of all, F11 AgNPs coated paper showed maximum inhibition of 24 mm for S. aureus and 22 mm for B. cereus. The coated paper from three different bacteriocin-synthesized AgNPs, along with silver nitrate (AgNO3) coated and uncoated paper, was used for the storage of tomato fruit for a period of 10 days at room temperature. Changes during storage were determined by analyzing morphological and color changes. Compared to AgNO3 coated and uncoated paper, tomato fruit preserved in F11 AgNPs coated paper maintained and held its appearance and firmness, thereby confirming their effectiveness in the preservation of tomatoes.
Introduction
Research in the field of nanotechnology offers a plethora of opportunities in various sectors of science and technology. In pharmaceuticals, agriculture, and the automobile industry, nanotechnology is expanding its reach optimistically. In the food industry, nanotechnology is becoming a boon with its significance in food packaging, food delivery, nanoencapsulation, and enhancement of the shelf life of food (De Azeredo, 2009; Sharma et al., 2022a,b). Packaging is a multi-billion-dollar industry facing limitations due to early spoilage of stored food products. Nanotechnology introduces novel food packaging techniques, that by using nanoparticles improves foods physicochemical and antimicrobial properties (Anvar et al., 2021). Food safety techniques improvised using functional nanoparticles minimize the chances of spoilage by maintaining moisture stability, mechanical strength, durability and ensure product safety and quality (PrimoŽič et al., 2021). Development of secure packaging systems is a need that can be met using nanoparticles. Antimicrobial packaging is necessary to increase the food's shelf life and hence avoid deterioration because commercially accessible food items are susceptible to the appearance of numerous microorganisms (Sharma et al., 2017; Chaudhary et al., 2020). Attributed to a larger surface-to-volume ratio, broad-spectrum antimicrobial efficacy nanoparticles could be considered a feasible solution to overcome various challenges in food manufacturing companies (Sharma et al., 2022a). Antimicrobial nanoparticles are currently being used in packaging, and there are several uses for them in food packaging and preservation (Mihindukulasuriya and Lim, 2014).
Silver nanoparticles (AgNPs) are one of the most promising nanoparticles, possessing advanced functional properties, making them a suitable candidate for the food sector. Silver nanoparticles synthesized using various biological sources (plants and microorganisms) are extensively employed for their strong antimicrobial potential against different pathogens. Biologically synthesized silver nanoparticles using microorganisms are ecofriendly, less toxic, and cost-effective as compared to chemically synthesized nanoparticles (Simbine et al., 2019; Zorraquín-Peña et al., 2020; Wang et al., 2022). Their use in active packaging materials could be pivotal in increasing the shelf life of food products. This in turn eliminates toxic spoilage microbes, ensuring safer or more protective packaging when scaled against conventional preservation techniques (Carbone et al., 2016). Multiple studies cited in the literature mention the use of healthy food microorganisms (probiotics and bacteriocins) in the synthesis of silver nanoparticles (Ghosh et al., 2022).
Bacteriocins are peptides or proteins produced by microorganisms that have a proven record as antibacterial agents and hence have been commercially approved for application in the food industry due to their GRAS status. Bacteriocins are produced by wide variety of gram-positive and gram-negative bacteria and are secreted extracellularly. The fusion of nanoparticles with bacteriocins has shown a positive effect on improving bacteriocin yield, protect them from destruction by proteolytic enzymes and increased their survival rate (Sulthana and Archer, 2021). Furthermore, bacteriocins' antibacterial characteristics are improved by combining them with nanoparticles, increasing their potency for usage in many food industry applications (Fahim et al., 2016; Sidhu and Nehra, 2020).
Therefore, the idea of fortifying the antimicrobial effect of silver nanoparticles has been perceived in the present study because of their barrier properties and structural integrity which help in the reduction of spoilage or pathogenic microbial growth in food (Anvar et al., 2021). The bacteriocins integration with silver nanoparticles strengthens them as well as broadens the antagonistic action. The use of bacteriocin in packaging is a new concept and can be used to prevent spoilage and hence increase the stability of food products for longer periods (Chikindas et al., 2018; Silva et al., 2018). We have already utilized probiotics to synthesize silver nanoparticles and determined their antimicrobial potential in our previous study (Sharma et al., 2022b). In the present study, we synthesized silver nanoparticles using purified bacteriocins obtained from three different in-house potential probiotics. Using probiotics for bacteriocin production is a safe approach as probiotics are embedded with several nutritional attributes that benefit human health (Sharma and Sharma, 2021). In order to develop an eco-friendly and secure packaging solution that can be utilized in the storage of food goods and consequently increase their shelf life, the application of bacteriocin-produced silver nanoparticles was examined in the coating of cellulose paper.
Materials and methods
Materials
Three in-house probiotic cultures procured from the Microbiology Laboratory, Department of Basic Sciences, UHF Nauni, Solan, H.P., India, were used for bacteriocin production. The Lactobacillus MRS agar was purchased from HIMEDIA laboratories (Mumbai, India). The bacterial strain Bacillus cereus was purchased from CRI (Kasauli, H.P., India) and Staphylococcus aureus was purchased from IGMC (Shimla, H.P., India). A cellulose paper bag along with fresh tomato fruit was procured from the local market in Solan, Himachal Pradesh. All the chemicals used were of analytical grade.
Production and purification of bacteriocin from bacteriocinogenic lactic acid bacteria
Lactobacillus pentosus S6 (KU92122), Lactobacillus crustorum F11 (KT865221), and Lactobacillus spicheri G2 (JX481912) probiotic cultures were seeded @10% (A = 1.0 at 540 nm) in MRS broth (1,000 ml) and incubated at 37°C for 36 h. After incubation, cultures were centrifuged at 8,000 g for 30 min, and the supernatant was collected. Partial purification was done by adding ammonium sulfate at 50 % of saturation level for L. pentosus S6 and L. crustorum F11 and 30% for L. spicheri G2, followed by dialysis for 12 h. The pellet was collected after centrifugation, dissolved in phosphate buffer (0.1 M, pH 7.0) and purified by a column chromatography technique on a sephadex G-75 column. The bacteriocin-positive fractions were combined and kept at 4°C pending use (Gautam and Sharma, 2015).
Antimicrobial efficacy of crude, partially purified and purified bacteriocins
The antimicrobial assay of crude, partially purified, and purified bacteriocin was assessed against two bacterial test indicators, i.e., B. cereus and S. aureus, using the well diffusion method (Kimura et al., 1998). One ml inoculum of each indicator bacteria (1.0 OD) was swabbed properly on prepoured sterilized nutrient agar plates with the help of sterilized cotton buds. The swabbing was done in such a way that indicator culture covers the whole surface of the nutrient agar plate. The crude, partially purified and purified bacteriocin from L pentosus S6, L crustorum F11 and L spicheri G2 was poured into the wells cut into these swabbed plates. The plates were then incubated at 37°C for 24 h and the zones of inhibition formed around the wells were measured.
Biosynthesis of AgNPs using purified bacteriocin
The purified bacteriocin obtained was mixed with silver nitrate (AgNO3) solution (0.1 mM) for the biosynthesis of AgNPs. The prepared solutions were incubated at 30°C for 24 h. The primary detection of synthesized silver nanoparticles was determined by observing the color change in the solution. The production of AgNPs by bacteriocins, their characterization, and their use in the food sector are schematically depicted in Figure 1.
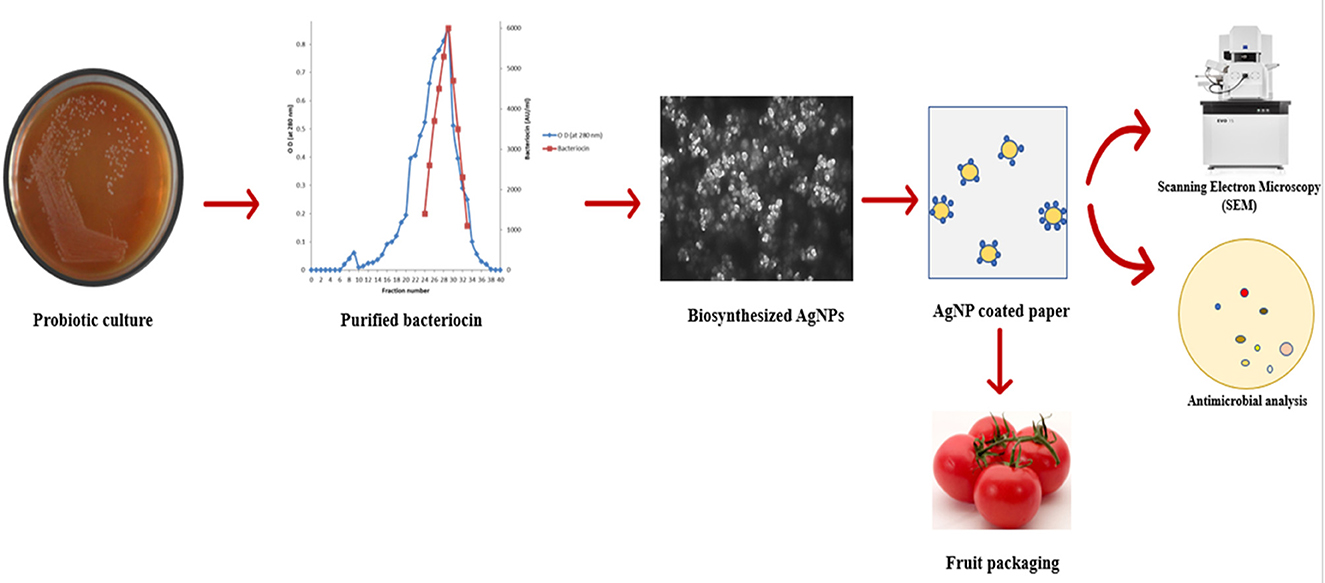
Figure 1. Schematic representation of synthesis of AgNPs by bacteriocins and their application in food packaging.
Characterization of silver nanoparticles
UV-visible spectroscopy analysis
UV-Vis spectroscopy was used to confirm the synthesis of AgNPs. Using the UV-Vis spectrophotometer UV-2450, the absorbance of synthetic silver nanoparticles was measured over the wavelength range of 350 to 650 nm.
Scanning electron microscopy
SEM was used to determine the morphology and structure of synthesized AgNPs. SEM images were taken on a JEOL JSM−6610LV instrument at an accelerating voltage of 20 KeV. An AgNPs sample was prepared on an aluminum plate and was dried overnight to take images the following day.
Transmission electron microscopy
Transmission electron microscopy (TEM) (FP 5022/22-Tecnai G2 20 S-TWIN) was used to analyze the size of synthesized AgNPs. The AgNPs suspension was deposited on a copper grid with a carbon coating, and water was allowed to evaporate for 1 h within a vacuum dryer (Sarvamangala et al., 2013).
Preparation of silver nanoparticle coated paper
Bacteriocin synthesized AgNPs were further used for the coating of cellulose paper. The market-available brown cellulose paper bag (20 × 25 cm) (Hindustan Paper Mill) having a thickness of 0.20 mm was immersed in 0.1 M of 50 ml of prepared silver nanoparticle solution for about 30 min. The paper was then rinsed with ethanol (1 min) to eliminate the unabsorbed AgNPs. The paper was then washed with water for about 30 min, and then dried in the hot air oven for about 2 h (Peungsamran and Namwong, 2016). As controls, cellulose paper that had not been treated and paper that had simply been dipped in AgNO3 solution were employed.
Characterization of silver nanoparticle coated paper
Scanning electron microscopy
A JEOL model JSM-6610LV equipment was used to conduct a SEM study of cellulose paper at a 20 KeV accelerating voltage. SEM analysis of AgNPs coated paper along with AgNO3 coated and uncoated paper was determined.
Antibacterial efficacy of AgNPs coated paper
Spot on lawn preparation
The antibacterial efficacy of the coated paper was observed against two challenging pathogens, i.e., S. aureus and B. cereus. In the spot method, the sample was applied as a spot on the respective plate containing a selective medium for their growth (Schillinger and Lucke, 1989). The paper sample from different treatments was spotted with the help of sterilized forceps on the plate. The plates were then incubated at 37°C for 24 h. The diameter of the zone formed was measured as its zone size.
Storage of food items in AgNPs coated paper
To check the effect of coated paper on food shelf life, we tried to store fresh tomato fruit (Solanum lycopersicum) in the package. The effect of AgNPs coated paper was compared with AgNO3 coated paper (without nanoparticles) (control 1) and uncoated paper (control 2). Five different sets of paper were used to store fresh tomato fruit purchased from the local market. The paper was punched with the help of a puncture to allow the passage of air uniformly to the food item and then kept for storage at room temperature (18–20°C). For each of the numbers 0, 3, 5, 7, and 10, the storage stability was examined, and the impact of coated paper was noted.
Changes in morphological characteristics during storage
The morphological changes observed in tomatoes during the storage period were determined. Changes in appearance and firmness were analyzed.
Determination of color change during storage
The Royal Horticulture Society of London's color cards were used to compare tomatoes' changing colors, and the accompanying card numbers were mentioned along with the color to track changes over the course of storage (http://rhscf.orgfree.com/).
Results and discussion
Bacteriocin production
Bacteriocin production was performed by the addition of ammonium sulfate to the culture supernatant at different saturation levels (Supplementary Table S1). The partially purified bacteriocin from L. pentosus S6 exhibited a higher activity unit of 6 × 103 AU/ml as compared to 2 × 103 AU/ml for its crude preparation. Complete purification of bacteriocin was achieved after gel exclusion column chromatography, where 12–30 fractions were pooled together and the activity unit of purified bacteriocin finally increased to 8 × 103 AU/ml. For L. crustorum F11, the activity unit is 4 × 103 AU/ml for partially purified bacteriocin, 2 × 103 AU/ml for crude bacteriocin, and 6 × 103 AU/ml for partially purified bacteriocin. Increased activity units in concentrate form are desirable for increasing the antibacterial effects against spoilage-causing food-borne pathogens. Handa and Sharma (2016) reported the bacteriocin production of L. crustorum F11, which has been utilized in this study to synthesize AgNPs, has good potential for use in food preservation due to its antagonism against various challenging food-borne and spoilage-causing pathogens. For L. spicheri G2, there was an increase in the activity units from 2 × 103 AU/ml for culture supernatant, 6 × 103 AU/ml for partially purified bacteriocin to 8 × 103 AU/ml (Gautam and Sharma, 2015) for purified bacteriocin, respectively. Purified bacteriocin was used to synthesize AgNPs, creating a more effective and environmentally acceptable food packaging material.
Antimicrobial efficacy of crude, partially purified, and purified bacteriocin
The antimicrobial potential of crude, partially purified, and purified bacteriocins was analyzed against S. aureus and B. cereus before their usage in the biosynthesis of AgNPs. The antibacterial impact of bacteriocins derived from three distinct probiotic isolates followed a similar pattern in that it was greatest for purified bacteriocin, then partially purified bacteriocin, and crude bacteriocin (Figure 2, Table 1). Therefore, purified bacteriocin was chosen for AgNPs synthesis due to its high antimicrobial activity, to ensure its positive role in preventing food spoilage by inhibiting the growth of spoilage pathogens. Leslie et al. (2021) observed strong antimicrobial activity of bacteriocins against Bacillus pumilus, Bacillus amyloliquefaciens, Staphylococcus aureus, and Listeria monocytogenes. The strong antibacterial activity of bacteriocins against microorganisms that cause food degradation makes them an excellent choice for usage in the food industry.
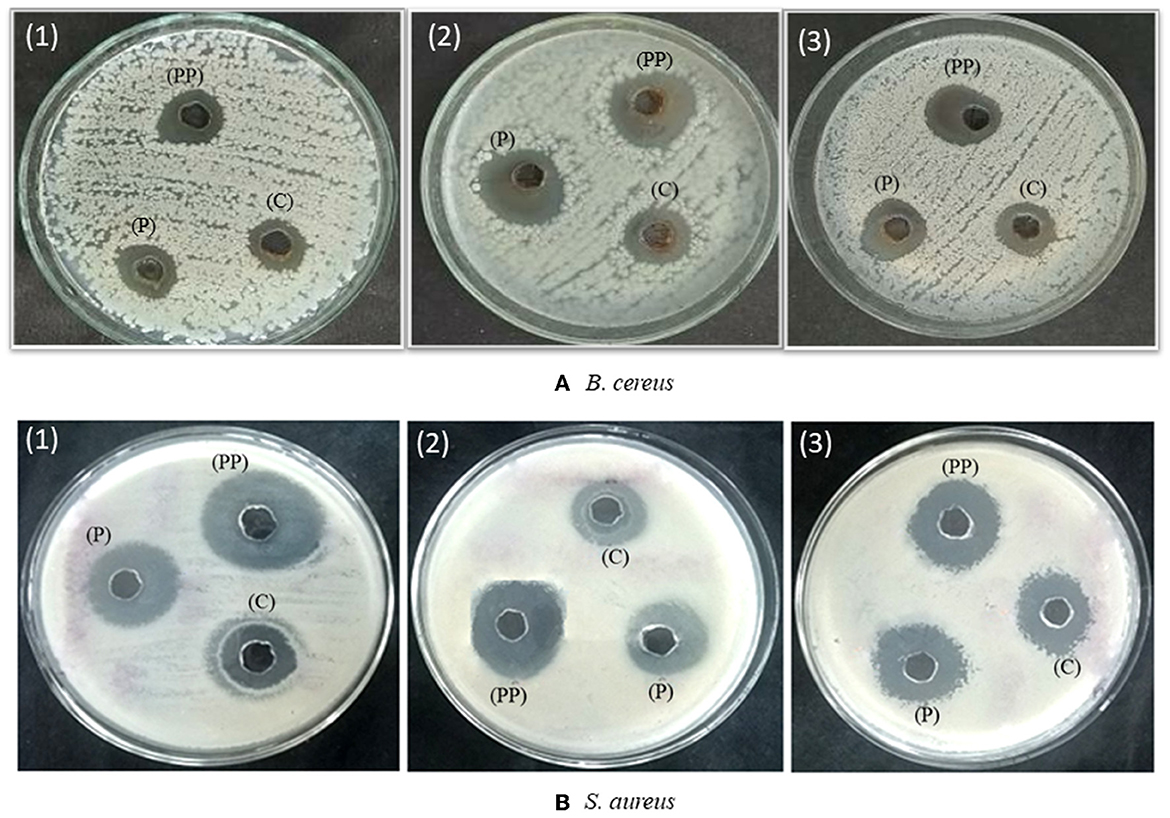
Figure 2. Antimicrobial activity of crude (c), partially purified (PP), and purified bacteriocin (P) of probiotics (1) L. crustorum F11 (2) L. spicheri G2 (3) L. pentosus S6 against (A) Bacillus cereus and (B) Staphylococcus aureus.

Table 1. Antimicrobial activity of crude, partially purified and purified bacteriocin against different pathogens of selected probiotics.
Synthesis of silver nanoparticles using bacteriocin
The purified bacteriocin obtained was further used for synthesizing silver nanoparticles (AgNPs). The primary confirmation observed was the color change in the reaction mixture after incubation (Figure 3A). The color of bacteriocin synthesized AgNPs turned dark brown, whereas no change was observed in the control, which indicated the synthesis of AgNPs. Other authors have also reported color change as the initial confirmation for the synthesized AgNPs (Naseer et al., 2021; Wang et al., 2022). Further, we used UV-Vis spectroscopy analysis for confirmation of their synthesis, which showed maximum absorbance at 450 nm (Figure 3B), which falls in the same range as suggested by others (Nithya and Ragunathan, 2012). Bacteriocin conjugated AgNPs were used in this study, keeping in view the strong antagonistic action of bacteriocin against spoilage-causing microorganisms. This potential of bacteriocin was further strengthened with silver metal ions due to the well-known antagonistic function of silver against bacteria and fungi (Moodley et al., 2018). Different studies have been documented in the literature about the utilization of probiotics or bacteriocin to synthesize AgNPs. Amer et al. (2021) utilized bacteriocin plantaricin to synthesize AgNPs to enhance the antimicrobial potential of plantaricin. In another study, the probiotic strain of Lactococcus lactis was used to synthesize AgNPs (Saravanan et al., 2017). Three different bacteriocin isolates were selected in this study to synthesize AgNPs, as we also want to compare the difference in the size of synthesized AgNPs and their application in preserving food products. We used SEM analysis, which revealed the shape of bacteriocin synthesized AgNPs as round or spherical and present as a single form or in association with others (Figure 4A). TEM was used to determine the size of synthesized AgNPs. The micrograph (Figure 4B) revealed that the mean size of synthesized AgNPs was found to be 20 nm for G2 AgNPs, 10 nm for S6 AgNPs, and 5 nm for F11 AgNPs, respectively. Esmail et al. (2022) found the size of synthesized AgNPs to be < 25 nm. Because of their small size, AgNPs can more efficiently target harmful pathogens by releasing silver ions only where they are needed (Zhang et al., 2016).
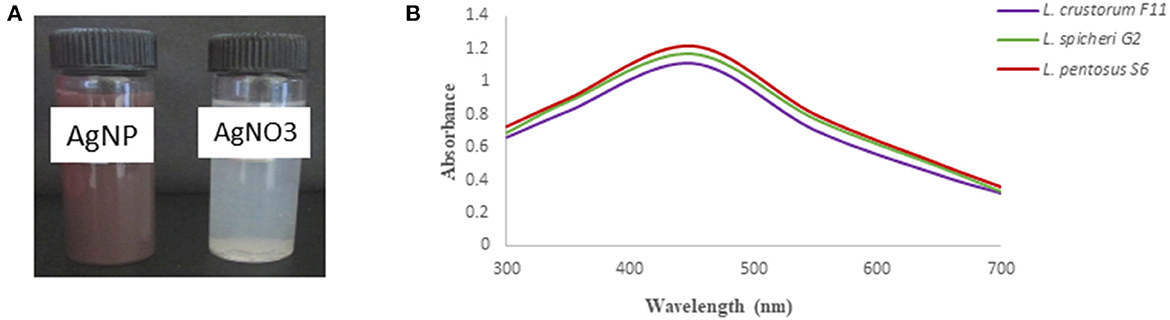
Figure 3. (A) synthesis of AgNPs was confirmed by color change in the solution after incubation. (B) UV-vis spectroscopy analysis of synthesized AgNPs using different bacteriocins.
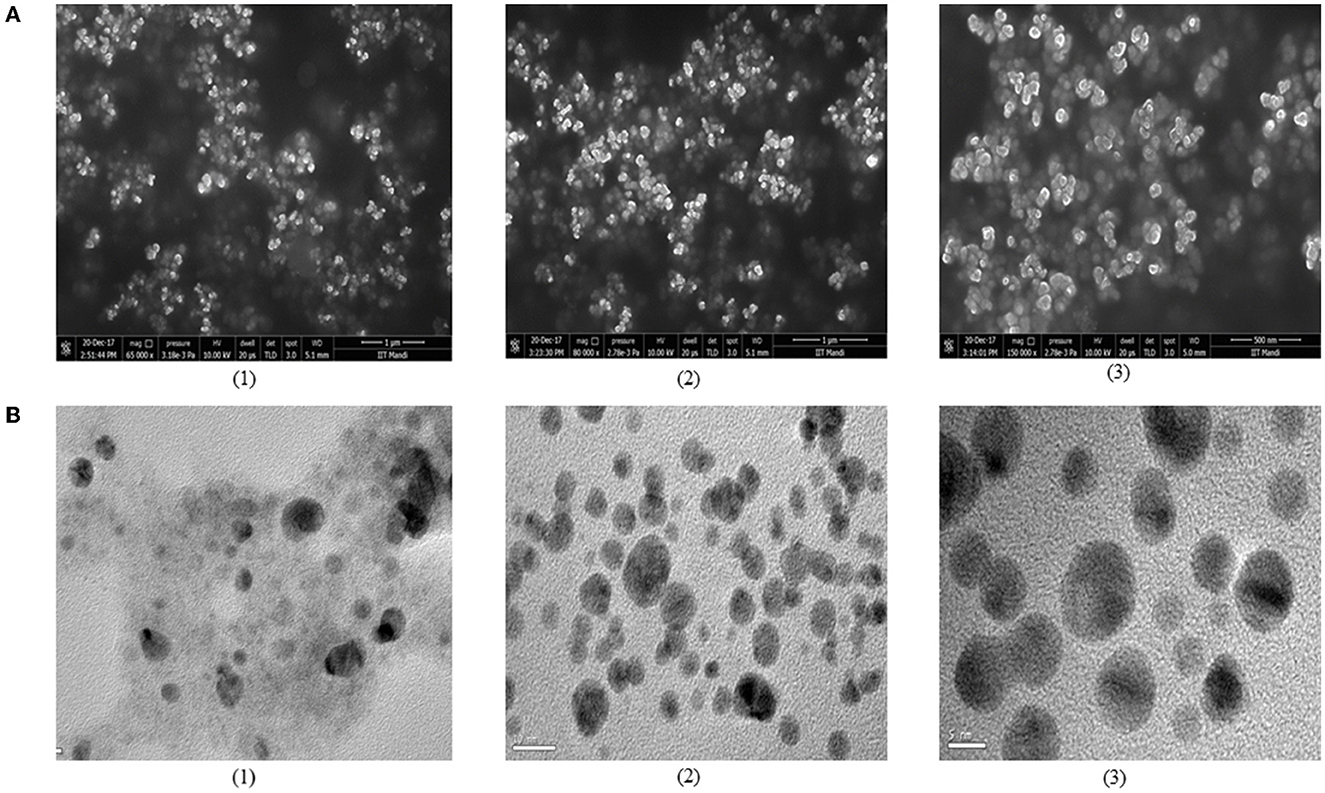
Figure 4. Determination of morphology and structure of biosynthesized AgNPs using (A) SEM and (B) Tem techniques. Micrograph represents bacteriocin synthesized AgNPs from (1) L. spicheri G2 (2) L. pentosus S6 (3) L. crustorum F11.
Preparation of bacteriocin-capped silver nanoparticle coated paper
The application of biosynthesized AgNPs was analyzed in the coating of cellulose paper to provide their antimicrobial action to the paper. This AgNPs coated paper prepared in this study could be used for the preservation of food products for a longer duration of time, thereby eliminating the growth of food spoilage microorganisms. Ionic silver has long been known as a strong antimicrobial agent. Therefore, utilization of its potential in synthesizing AgNPs could provide an alternate to fight various challenges in the food industry in an economical way. In AgNPs coated paper, the accumulation of ionic silver is released slowly from the surface to the bulk to prevent food spoilage. The integration of bacteriocins with AgNPs was done in this study to multiply their antimicrobial action in fighting spoilage-causing microorganisms. The bacteriocin coated paper prepared was compared with AgNO3 coated paper and uncoated paper to check their effectiveness.
Bacteriocin from B. borstelensis AG1 demonstrated antimicrobial activity against the most difficult and dangerous food-borne pathogens and served as an alluring food bio preservative, according to Sharma et al. (2014). This high antagonistic potential of bacteriocins makes them desirable for the preservation of food items in the food processing and packaging industries.
Characterization of coated paper
SEM analysis was carried out to investigate the presence and dispersion of bacteriocin synthesized AgNPs in the paper. SEM analysis of AgNP coated paper from three bacteriocin AgNPs, i.e., S6 AgNPs, F11 AgNPs, and G2 AgNPs, along with two controls, i.e., one coated only with AgNO3 and one uncoated paper, was presented in Figures 5A–E. The AgNPs coated paper from three bacteriocins AgNPs exhibited a smooth appearance, indicating a clear coating over the paper substrate. The adsorbed bacteriocins AgNPs appeared to be spherical/ square in shape, present in bunches or alone, and were well-distributed on the surface of the coated paper (Figures 5A–C). Control group with AgNO3 coating also showed the adsorption, but it was not appeared to be well distributed (Figure 5D), while nothing was visualized in the uncoated paper (Figure 5E). Others have also reported the appearance of spherical-shaped nanoparticles on AgNPs coated paper, when observed through SEM analysis (Peungsamran and Namwong, 2016; Jung et al., 2018).
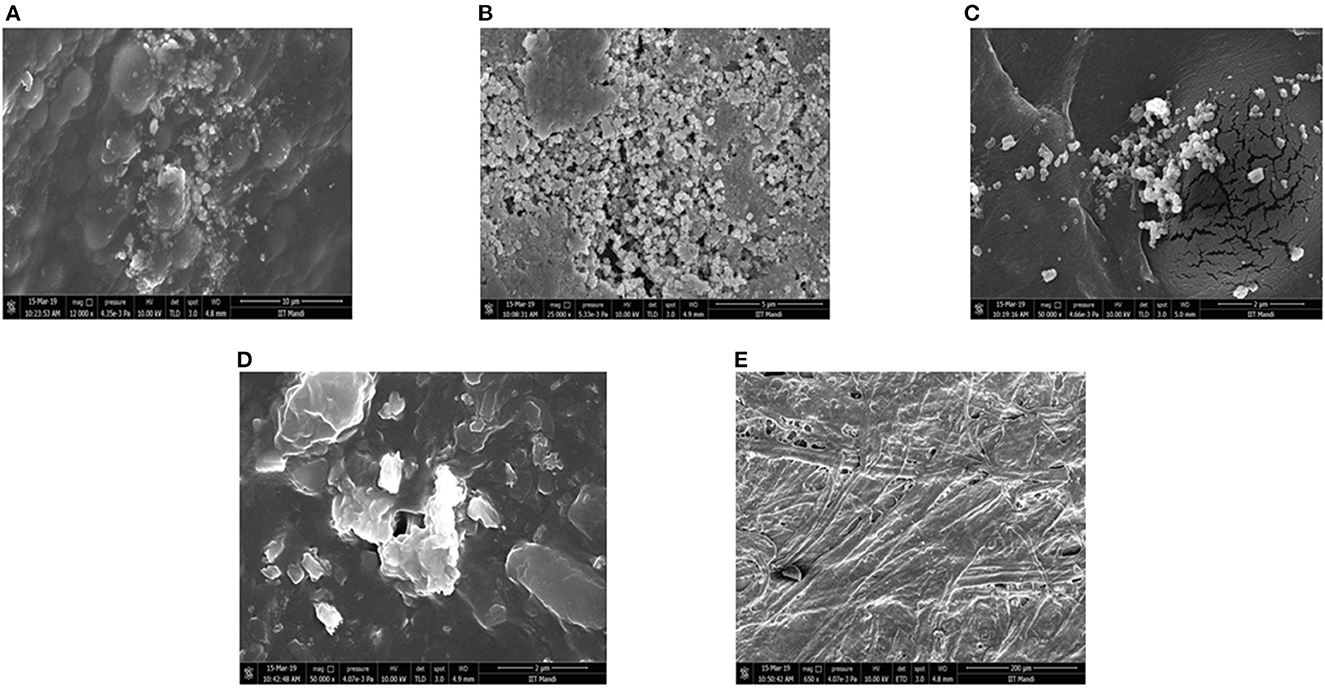
Figure 5. SEM micrograph of different treatments coated cellulose paper (A) L. pentosus S6 AgNP (B) L. crustorum F11 AgNP (C) L. spicheri G2 AgNP (D) AgNO3 coated paper (E) untreated paper.
Antagonistic efficacy of silver nanoparticles coated paper
The antibacterial efficacy of coated paper was determined against S. aureus and B. cereus using the spot method (Table 2, Figure 6). F11 AgNPs coated paper showed the highest antimicrobial against the two pathogens as compared to AgNO3 coated paper. For S. aureus, its antimicrobial activity was 24 mm as compared to 15 mm for AgNO3, whereas for B. cereus, it was 22 mm for its coated paper and 15 mm for AgNO3 coated paper (Figure 6, Table 2). The rest of the two isolates i.e., S6 AgNPs and G2 AgNPs also showed strong antimicrobial potential against the two pathogens as compared to AgNO3 coated paper. The uncoated paper (without treatment) showed no zones around the pathogens, thus indicating no antibacterial activity. This antimicrobial activity exhibited by all the three bacteriocin coated papers confirms their role in the prevention of food spoilage microorganisms, thereby their utilization by the food industry to store food products thereby maintaining its nutritional quality and shelf life. AgNPs high surface-to-volume ratio promotes a wide range of antibacterial action against pathogenic microbes and has numerous applications in food processing and packaging (Gutierrez et al., 2010). Jung et al. (2018) reported the antibacterial activity of AgNPs coated paper, which showed an inhibition zone of 2.2 mm for E. coli and 1.8 mm for S. aureus. Tsai et al. (2017) reported the antibacterial activity of cellulose paper coated with silver-coated gold nanoparticles. The coated paper showed a 15 mm zone of inhibition against E. coli.
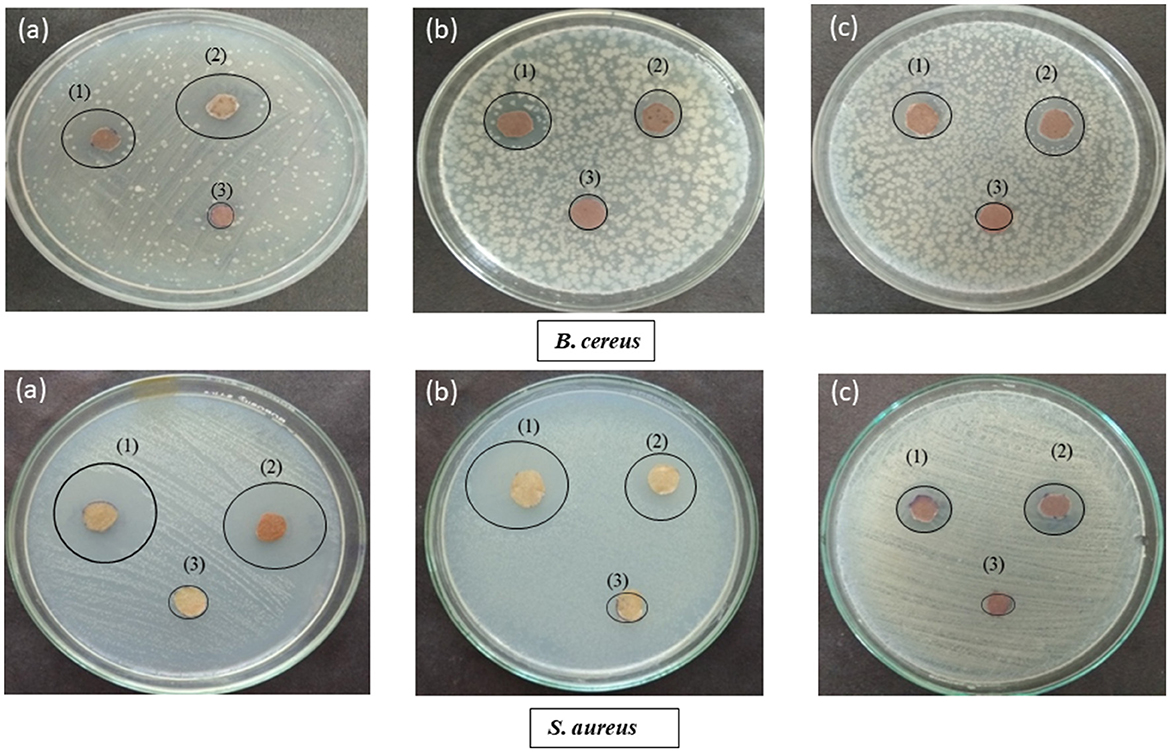
Figure 6. Antibacterial potential of (a) F11 Ba AgNPs (b) G2 Ba AgNPs (c) S6 Ba AgNPs, where (1) represents bacteriocin AgNPs coated paper, (2) AgNO3 coated paper and (3) uncoated paper against two different pathogens i.e., Bacillus cereus and Staphylococcus aureus.
Storage of tomato fruits in bacteriocin synthesized silver nanoparticles coated paper
Five different sets of paper were used to store fresh tomato fruit to check their effect on its storage and shelf life. Table 3 shows the shelf stability of tomato fruit stored at room temperature (18–20°C) for 10 days, as determined by appearance and firmness (Figure 7, Table 3). The shelf life was found to be maximum for L. crustorum F11 AgNPs coated paper (10 days), where the stored tomatoes looked good in appearance and firmness. Minimum shelf life was observed for uncoated paper (Control 1) and AgNO3 coated paper (Control 2) where microbial spoilage and black spots were visualized in the tomato after 10 days of storage, respectively. Tomatoes stored in S6 AgNPs and G2 AgNPs coated paper also become soft and shriveled after 10 days of storage (Table 3). The F11 AgNPs coated paper synthesized using purified bacteriocin from L. crustorum proved to be the best among all other treatments, resulting in enhancing the shelf life of stored fruit. F11 AgNPs potentiation against spoilage-causing bacteria may be ascribed to their short (5 nm) size, which renders them more target-oriented in prevention of spoilage causing microorganisms. In one study, Gao et al. (2017) utilized AgNPs synthesized from tea leaf extract for the preservation of cherry tomato fruit and obtained positive results. Lin et al. (2022) explored the role of AgNPs in the preservation of litchi fruit and the prevention of Peronophythora litchi infection. They applied different concentrations of AgNPs to litchi fruit and observed that AgNPs treatment prolonged the shelf life of litchi fruit and prevented fungal infection.
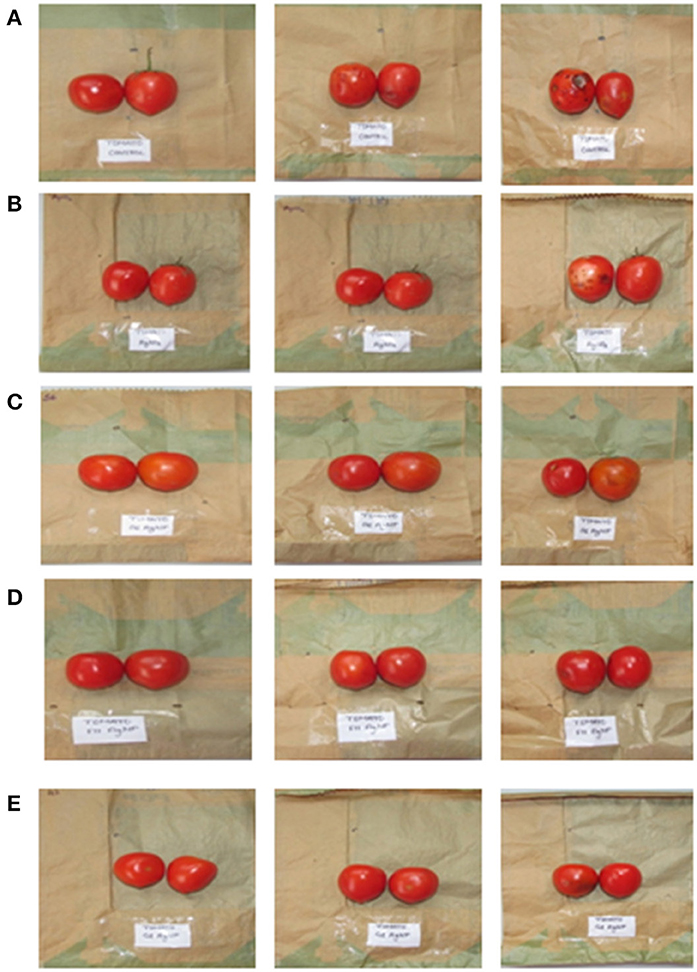
Figure 7. Storage of tomato in cellulose paper coated with different sets of treatment (A) control (untreated) (B) AgNO3 coated paper (C) S6 Ba AgNPs coated paper (D) F11 Ba AgNPs coated paper (E) G2 Ba AgNPs coated paper.
We next determined the change in color of stored tomato fruits during storage. Color is one of the most important and complex attributes of evaluating tomato fruit quality, and hence considerable attention has been given to its characterization and measurement. The color of ripening tomatoes is largely due to the presence of a diverse carotene pigment system. Ripening processes are associated with increasing lycopene content. For the storage of tomatoes, Bacteriocin F11 AgNP coated paper proved to be the best, with the intensity of color retained during 10 days of storage. The color of stored tomato belongs to the Grayed-Red group (180 B) of the Royal Horticultural Society, London color card (Table 4). Whereas, there was significant variation in color observed in tomatoes stored in other treatments.
AgNPs synthesized in the present study using bacteriocins produced from healthy probiotic microorganisms can be employed in different sectors of the food industry. Ours is one of the first studies where we used bacteriocin synthesized AgNPs for the coating of food packages that can be used for the storage of tomato fruit. The antimicrobial properties of AgNPs can enhance the antimicrobial spectrum of bacteriocins as they themselves have enormous antibacterial potential and hold promise to target food-borne pathogenic species. As a result, these have been proposed as the best candidates for conjugation with bacteriocins. AgNPs have already been used to create antimicrobial surfaces in a variety of industries, including textiles, fibers, polymers, and metals. The use of AgNPs in the food industry is still not extensive due to some associated limitations. However, a cutting-edge substitute for their manufacture and application in the food sector is the use of bacteriocins and beneficial probiotic microbes.
Conclusion
Biologically synthesized AgNPs have proven to be effective for their application in different fields of food industry. In food packaging, nanoparticles have been utilized to enhanced products stability and quality thereby eliminating the growth of spoilage causing microorganisms. keeping in mind, the importance of silver nanoparticles, we used bacteriocin proteins obtained from healthy probiotic microorganisms to synthesize silver nanoparticles. Bacteriocins are antimicrobial peptides, their fusion with silver nanoparticles increases their antimicrobial potential. The indication of AgNPs formation was confirmed by color change and UV-vis spectroscopy. Further, SEM, and TEM techniques confirmed the shape and size of synthesized AgNPs. These biologically synthesized AgNPs was used for the coating of cellulose paper that was used for food packaging. The impingement of AgNPs in the cellulose paper was determined by SEM and antimicrobial analysis. The AgNPs coated paper along with uncoated and AgNO3 coated paper was used for the storage of tomato fruit. Compared to uncoated paper and AgNO3 coated paper, the bacteriocins AgNPs coated paper proved to be the best for tomato preservation since it enhanced the shelf life of tomato fruit by maintaining storage quality, prolong shelf life and delayed microbiological decomposition.
Data availability statement
The original contributions presented in the study are included in the article/Supplementary material, further inquiries can be directed to the corresponding author.
Author contributions
SS and NS designed the study and experiments. SS and NK performed the experiments. All authors contributed to the article and approved the submitted version.
Acknowledgments
The authors would like to thank Himachal Pradesh Council of Science Technology and Environment (HIMCOSTE) for funding this study.
Conflict of interest
The authors declare that the research was conducted in the absence of any commercial or financial relationships that could be construed as a potential conflict of interest.
Publisher's note
All claims expressed in this article are solely those of the authors and do not necessarily represent those of their affiliated organizations, or those of the publisher, the editors and the reviewers. Any product that may be evaluated in this article, or claim that may be made by its manufacturer, is not guaranteed or endorsed by the publisher.
Supplementary material
The Supplementary Material for this article can be found online at: https://www.frontiersin.org/articles/10.3389/fsufs.2023.1072738/full#supplementary-material
References
Amer, S. A., Abushady, H. M., Refay, R. M., and Mailam, M. A. (2021). Enhancement of the antibacterial potential of plantaricin by incorporation into silver nanoparticles. J. Genet. Eng. Biotechnol. 19, 13. doi: 10.1186/s43141-020-00093-z
Anvar, A. A., Ahari, H., and Ataee, M. (2021). Antimicrobial properties of food nanopackaging: a new focus on foodborne pathogens. Front. Microbiol. 12:690706. doi: 10.3389/fmicb.2021.690706
Carbone, M., Sabbatella, D. T. D. G., and Antiochia, R. (2016). Silver nanoparticles in polymeric matrices for fresh food packaging. J. King Saud Uni. Sci. 28, 273–279. doi: 10.1016/j.jksus.2016.05.004
Chaudhary, P., Fatima, F., and Kumar, A. (2020). Relevance of nanomaterials in food packaging and its advanced future prospects. J. Inorg. Organomet. Polym. 30, 5180–5192. doi: 10.1007/s10904-020-01674-8
Chikindas, M. L., Weeks, R., Drider, D., Chistyakov, V. A., and Dicks, L. M. (2018). Functions and emerging applications of bacteriocins. Curr. Opin. Biotechnol. 49, 23–28. doi: 10.1016/j.copbio.2017.07.011
De Azeredo, H. M. C. (2009). Nanocomposites for food packaging applications. Food Res. Intern. 42, 1240–1253. doi: 10.1016/j.foodres.2009.03.019
Esmail, R., Afshar, A., Morteza, M., Abolfazl, A., and Akhondi, E. (2022). Synthesis of silver nanoparticles with high efficiency and stability by culture supernatant of Bacillus ROM6 isolated from Zarshouran gold mine and evaluating its antibacterial effects. BMC Microbiol. 22, 97 (2022). doi: 10.1186/s12866-022-02490-5
Fahim, H. A., Khairalla, S., and El-Gendy, A. O. (2016). Nanotechnology: a valuable strategy to improve bacteriocin formulations Front in Microbio. 7, 1385–1392. doi: 10.3389/fmicb.2016.01385
Gao, L., Li, Q., Zhao, Y., Wang, H., Liu, Y., Sun, Y., et al. (2017). Silver nanoparticles biologically synthesised using tea leaf extracts and their use for extension of fruit shelf life. IET Nanobiotechnol. 9, 637–643. doi: 10.1049/iet-nbt.2016.0207
Gautam, N., and Sharma, N. (2015). A study on characterization of new bacteriocin produced from a novel strain of Lactobacillus spicheri G2 isolated from Gundruk—A fermented vegetable product of North East India. J. Food Sci. Techn. 52, 5808–5816. doi: 10.1007/s13197-015-1710-x
Ghosh, S., Sarkar, B., Kaushik, A., and Mostafavi, E. (2022). Nanobiotechnological prospects of probiotic microflora: synthesis, mechanism, and applications, Sci. Total Environ. 838, 6212. doi: 10.1016/j.scitotenv.2022.156212
Gutierrez, F. M., Olive, P. L., Banuelos, A., Orrantia, E., Nino, N., Sanchez, E. M., et al. (2010). Synthesis, characterization, and evaluation of antimicrobial and cytotoxic effect of silver and titanium nanoparticles. Nanomedicine 6, 681–688. doi: 10.1016/j.nano.2010.02.001
Handa, S., and Sharma, N. (2016). A Study on bacteriocin produced from a novel strain of Lactobacillus crustorum F11 Isolated from human milk. Proc. Indian Nat. Sci. Acad. 82, 1317–1322. doi: 10.7897/2230-8407.04352
Jung, J., Raghavendra, G. M., Kim, D., and Seo, J. (2018). One-step synthesis of starch-silver nanoparticle solution and its application to antibacterial paper coating. Int. J. Biol. Macromol. 107, 2285–2290. doi: 10.1016/j.ijbiomac.2017.10.108
Kimura, H., Sashihara, T., Matsuki, H., Sonomoto, K., and Ishizzaki, A. (1998). Novel bacteriocin of Pediococcus sp. ISK-1 isolated from well-aged bed of fermented rice bran. Ann. N. Y. Acad. Sci. 864, 345–348.
Leslie, V. A., Khaloud, M. A., Malaisamy, A., and Balasubramanian, B. (2021). Bacteriocin producing microbes with bactericidal activity against multidrug resistant pathogens. J. Infec. Pub. Health 14, 1802–1809. doi: 10.1016/j.jiph.2021.09.029
Lin, X., Lin, Y., Liao, Z., Niu, X., Wu, Y., Shao, D., et al. (2022). Preservation of litchi fruit with nanosilver composite particles (Ag-NP) and resistance against peronophythora litchi. Foods 11, 2934. doi: 10.3390/foods11192934
Mihindukulasuriya, S. D. F., and Lim, L. T. (2014). Nanotechnology development in food packaging: a review. Trends Food Sci. Techn. 40, 149–167. doi: 10.1016/j.tifs.2014.09.009
Moodley, J. S., Krishna, S. B., and Govender, P. (2018). Green synthesis of silver nanoparticles from Moringa oleifera leaf extracts and its antimicrobial potential. Adv. Nat. Sci. Nanosci. Nanotechnol. 9, 27–37. doi: 10.1155/2022/4136641
Naseer, Q. A., Xue, X., Wang, X., Dang, S., Din, S. U., and Jamil, J. (2021). Synthesis of silver nanoparticles using Lactobacillus bulgaricus and assessment of their antibacterial potential. Braz J. Bio. 82, 82–94. doi: 10.1590/1519-6984.232434
Nithya, R., and Ragunathan, R. (2012). Synthesis of silver nanoparticles using a probiotic microbe and its antibacterial effect against multidrug resistant bacteria. Afr. J. Biotech. 11, 11013–11021. doi: 10.5897/AJB12.439
Peungsamran, N., and Namwong, S. (2016). The coated paper with silver nanoparticles and its antibacterial activity. Key Engineer Mat. 675, 265–268. doi: 10.4028/www.scientific.net/KEM.675-676.265
PrimoŽič, M., Knez, Ž., and Leitgeb, M. (2021). BioNanotechnology in food science—Food packaging. Nanomat 11, 292. doi: 10.3390/nano1102029
Saravanan, C., Rajesh, R., Kaviarasan, T., Muthukumar, K., Kavitake, D., and Shetty, P. H. (2017). Synthesis of silver nanoparticles using bacterial exopolysaccharide and its application for degradation of azo-dyes. Biotechn Rep. 15, 33–40. doi: 10.1016/j.btre.2017.02.006
Sarvamangala, D., Kondala, K., Sivakumar, N., Babu, M. S., and Manga, S. (2013). Synthesis, characterization, and antimicrobial studies of AgNPs using probiotics. Int. Res. J. Pharmacy 4, 240–243.
Schillinger, U., and Lucke, F. K. (1989). Antibacterial activity of Lactobacillus sake isolated from meat. Appl. Environt. Microbio. 55, 1901–1906. doi: 10.1128/aem.55.8.1901-1906.1989
Sharma, C., Dhiman, R., Rokana, N., and Panwar, H. (2017). Nanotechnology: an untapped resource for food packaging. Front. Micro. 8, 1735. doi: 10.3389/fmicb.2017.01735
Sharma, N., Gupta, A., and Gautam, N. (2014). Characterization of bacteriocin like inhibitory substance produced by a new strain Brevibacillus borstelensis AG1 Isolated from “Marcha”. Braz. J. Microbiol. 45, 33. doi: 10.1590/S1517-83822014000300033
Sharma, S., and Sharma, N. (2021). Preparation of probiotic enriched functional beverage of Kodo millet (Paspalum scrobiculatum) a nutritionally enriched absolute new product for commercialization. J. Pharmacogn. Phytochem. 10, 752–758.
Sharma, S., Sharma, N., and Kaushal, N. (2022b). Comparative account of biogenic synthesis of silver nanoparticles using probiotics and their antimicrobial activity against challenging pathogens. Bio. Nano. Sci. 12, 833–840. doi: 10.1007/s12668-022-01004-x
Sharma, S., Sharma, N., and Sharma, P. (2022a). Biosynthesis and characterization of silver nanoparticles using probiotic strain Lactobacillus spicheri G2 and analysis of its antimicrobial potential. Int. J. Nanomat. Mol. Nanotech. 4, 1–6.
Sidhu, P. K., and Nehra, k. (2020). Bacteriocin-capped silver nanoparticles for enhanced antimicrobial efficacy against food pathogens. IET Nanobiotech.14, 245–252. doi: 10.1049/Fiet-nbt.2019.0323
Silva, C. C. G., Silva, S. P. M., and Ribeiro, S. C. (2018). Application of bacteriocins and protective cultures in dairy food preservation. Front in Microbio. 3, 594. doi: 10.3389/fmicb.2018.00594
Simbine, E. O., Rodrigues, L. D. C., Lapa-Guimaraes, J., Kamimura, E. S., Corassin, C. H., and Oliveira, C. A. F. D. (2019). Application of silver nanoparticles in food packages: a review. Food Sci. Technol. 39, 793–802. doi: 10.1590/fst.36318
Sulthana, R., and Archer, A. C. (2021). Bacteriocin nanoconjugates: boon to medical and food industry. J. Appl. Microbiol. 131, 1056–1071. doi: 10.1111/jam.14982
Tsai, T. T., Huang, T. H., Chang, C. J., Yi-Ju Ho, N., Tseng, Y. T., and Chen, C. F. (2017). Antibacterial cellulose paper made with silver-coated gold nanoparticles. Sci. Rep. 7, 1–10. doi: 10.1038/s41598-017-03357-w
Wang, X., Lee, S. Y., Akter, S., and Huq, M. A. (2022). Probiotic-mediated biosynthesis of silver nanoparticles and their antibacterial applications against pathogenic strains of Escherichia coli O157:H7. Poly. 14, 1834. doi: 10.3390/Fpolym14091834
Zhang, X. F., Liu, Z. G., Shen, W., and Gurunathan, S. (2016). Silver nanoparticles: synthesis, characterization, properties, applications, and therapeutic approaches. Int. J. Mol. Sci. 17, 1534. doi: 10.3390/ijms17091534
Keywords: bacteriocins, silver nanoparticles (AgNPs), SEM, food packaging, antimicrobial
Citation: Sharma S, Sharma N and Kaushal N (2023) Utilization of novel bacteriocin synthesized silver nanoparticles (AgNPs) for their application in antimicrobial packaging for preservation of tomato fruit. Front. Sustain. Food Syst. 7:1072738. doi: 10.3389/fsufs.2023.1072738
Received: 17 October 2022; Accepted: 18 January 2023;
Published: 06 February 2023.
Edited by:
Antonio Valero, University of Cordoba, SpainReviewed by:
Swarup Roy, Shoolini University of Biotechnology and Management Sciences, IndiaHaytham M. M. Ibrahim, Egyptian Atomic Energy Authority, Egypt
Copyright © 2023 Sharma, Sharma and Kaushal. This is an open-access article distributed under the terms of the Creative Commons Attribution License (CC BY). The use, distribution or reproduction in other forums is permitted, provided the original author(s) and the copyright owner(s) are credited and that the original publication in this journal is cited, in accordance with accepted academic practice. No use, distribution or reproduction is permitted which does not comply with these terms.
*Correspondence: Shakshi Sharma, c2hha3NoaS5zaGFybWEyMTkzQGdtYWlsLmNvbQ==
†These authors have contributed equally to this work