- 1Department of Dairy, Food Science and Technology, Egerton University, Nakuru, Kenya
- 2Department of Human Nutrition, Egerton University, Nakuru, Kenya
- 3Alliance of Bioversity International and International Centre for Tropical Agriculture (CIAT), Nairobi, Kenya
- 4School of Food Technology, Nutrition, and Bio-Engineering, Makerere University, Kampala, Uganda
- 5Alliance of Bioversity International and International Centre for Tropical Agricultural, Kampala, Uganda
Introduction: Current research on micronutrient deficiency indicates positive outcomes associated with utilizing biofortified beans in mitigating nutrition-related iron and zinc deficiencies, primarily in Sub-Saharan Africa. The common bean is however not palatable in its raw state and requires cooking before consumption. Existing research on the cooking qualities and nutrient retention of these novel biofortified common bean varieties is nonetheless scant, notably in Burundi.
Materials and methods: This study determined water absorption capacity, cooking yield, cooking time, and iron and zinc retention of 9 biofortified common bean varieties with Kinure, a traditional non-biofortified variety, as the control. The study employed a 10 × 2 completely randomized design in a factorial arrangement. The first factor was bean varieties with ten levels, and the second was pre-treatment with two levels, soaking and not soaking. Standard methods were used to analyze cooking properties and mineral retention, and experiments replicated thrice. Data analysis was conducted at a 95% confidence level and included Analysis of Variance and t-test comparison studies. Post-hoc analysis was performed using Tukey's Honestly Significant Difference.
Results and discussion: Cooking properties and mineral retention varied significantly between soaked and unsoaked bean varieties (P < 0.05) and in comparison, to Kinure (P < 0.05 and P < 0.001). Water absorption capacity varied from 64.51% to 116.3%. Soaked beans recorded half the total cooking time needed to cook unsoaked beans (77.62 versus 156.86 minutes), had higher cooking yields (289.77% versus 259.82%), and reduced Fe (121.58 versus 167.8%) and Zn (127.74% versus 145.15%) retention. Compared to Kinure, soaking resulted in longer cooking times, lower cooking yields in the biofortified common beans, and an insignificant effect on their zinc retention. A significant correlation between iron and zinc retention (r = 0.445), water absorption capacity and iron retention (r = −0.0425) and water absorption capacity and zinc retention (r = −0.477) were recorded. Three clusters were also observed; iron and zinc retention, cooking yields, and water absorption capacity, and cooking time. It was observed that, soaking common beans before cooking reduces cooking time and increases cooking yield in both biofortified and traditional common bean varieties. Moreover, biofortified common beans are a good source of iron and zinc and could considerably alleviate micronutrient deficiencies, particularly in developing countries.
1. Introduction
Hidden hunger, which refers to a deficiency in essential micronutrients (vitamins and minerals) such as iron, zinc, calcium, iodine, vitamins A and D, remains a public health challenge in Sub-Saharan Africa (FAO, 2019). Iron and zinc deficiencies affect an estimated 2 billion people globally, with a fifth of the population at risk of zinc deficiency and 30% anemic primarily owing to iron deficiency (FAO, 2019). About two-thirds of the people in Burundi suffer from health complications related to inadequate intake of these micronutrients, with the situation worsening among vulnerable populations (MSPLS, 2017; FAO, 2019). Despite the decline in prevalence of anemia caused by iron deficiency around the world, the proportion of children under 5 years suffering from anemia in Burundi increased from 45% in 2010 to 61% in 2017, significantly hampering the country's efforts to develop human capital (MSPLS, 2017). In low-income nations like Burundi, micronutrient deficiencies are frequently ignored and given lower priority when it comes to treatment until a widespread medical issue linked to the deficiency develops.
Therefore, nutrition-sensitive agricultural interventions such as micronutrient biofortification are needed to mitigate micronutrient malnutrition and its related health complications. Common bean plays a crucial role in meeting the dietary needs of people in Burundi (Mbonankira et al., 2016; Fungo et al., 2020). Common beans are affordable and accessible to vulnerable populations in Burundi which has a current average bean consumption of 31.5 kg per person per year (FAO, 2019). In addition, common beans are a significant source of cash and food for more than 90% of smallholder farmers in the country providing up to 20 and 50% of the required calories and protein needs, respectively (Katungi et al., 2020). Common beans can also be consumed in various forms, including fresh grains, edible bean leaves, pods, and dried bean grains, and processed into other food products such as bean flour (Katungi et al., 2020). The common bean crop is thus vital in offering a staggering food supply for most Burundi households. For these reasons, the common bean provides a key entry point to curbing the problems of poverty, food and nutrition security for the people in Burundi.
The genetic bean breeding programs in Burundi have resulted in development of various bean varieties which differ in appearance, yield, and disease resistance (Gilligan, 2012). Further research to develop biofortified common beans has been underway, focusing on bean varieties high in iron and zinc content (Buruchara et al., 2011; Buruchara, 2019). Micronutrient biofortification comprises genetic or agricultural modification of staple crops, including maize, rice, cassava, sweet potatoes, and legumes, to improve their nutritional composition (Moloto et al., 2018). Biofortification also improves other factors, such as disease resistance, and increased yields (Gilligan, 2012; Katungi et al., 2020). The availability of biofortified common beans thus provides consumers with a wide range of foods to select from when preparing household meals at home and at industrial scale (Katungi et al., 2009), which can result in reduced food prices (Bouis et al., 2011).
Using biofortified common beans is one of the strategies for mitigating micronutrient malnutrition and its associated complications, particularly in poor rural communities that can hardly access health care facilities (Moloto et al., 2018; Buruchara, 2019). Biofortification can go a long way in complementing other nutrition programs, such as fortification and supplementation (Bechoff et al., 2017; Buruchara, 2019). For example, studies on high iron beans released in Rwanda revealed that the beans provided about 30% of the estimated average requirements of iron in women and preschool children in nearly 250,000 households (Bouis et al., 2013; Ngozi, 2013).
The common bean is however unpalatable in its raw state and must be cooked or processed before eating. Moreover, discarding the soaking water is a practice that scientists unanimously encourage as it partially removes antinutritional factors such as oligassacharides, raffinose and staychose that are involved in gas production leading to flatulence (Fernandes et al., 2010). Cooking degrades a toxic compound called lectin phytohemagglutinin, which can cause severe gastric upsets (Carvalho et al., 2012a). Cooking legumes also improves their nutritional value by reducing antinutrients factors, such as phytic acid and tannins, while at the same time imparting desirable sensory properties (Díaz-Gómez et al., 2017; Samtiya et al., 2020). Cooking processes including soaking beans and discarding soaking water before cooking, while critical, might result in nutrient loss through the leaching of micronutrients in the broth, exuberating micronutrient deficiencies even in regions with higher consumption of these foods (Carvalho et al., 2012a; Bechoff, 2017). According to Díaz-Gómez et al. (2017), soaking beans reduces micronutrients and phytic acid via solubilization and leaching in the soaking water. As a result, there is a need to ensure sufficient nutrient retention during post-harvest handling, processing steps such as storage, and cooking (Zamindar et al., 2013; Bechoff, 2017). Furthermore, any biofortification program's effectiveness depends on how well the crop is accepted and consumed.
Numerous aspects, such as the culinary qualities, affect the extent to which people consume biofortified common beans (Gathu et al., 2012; Buzera et al., 2018). Variations in these qualities between bean varieties are attributed to intrinsic factors (genotype) and external factors such as storage conditions, agronomic techniques, climate, and technological factors (Buzera et al., 2018; Wainaina et al., 2021). Cooking time is also an essential consideration in customer acceptance of these biofortified common bean varieties. The seed coat's quality determines the bean grains' ability to absorb water and, consequently, their cooking time (Santos et al., 2016). Consumers highly desire beans with a quick hydration capacity, shorter cooking time, production of thick broth, pleasant flavor, soft texture, thin skin, stable color, and moderately cracked (Gathu et al., 2012; Wainaina et al., 2021). Additionally, on an industrial scale, a bean variety with a short cooking time indicates a lower cost to acquire the final product.
Current research in Burundi has focused on farmer acceptance of biofortified common beans and other aspects of the crop's value chain, including production, distribution, and value-added bean-based products (Mbonankira et al., 2016; Fungo et al., 2020; Katungi et al., 2020). These studies used nine biofortified bean varieties released in the country, including RWV1129, RWV1272, MAC44, and MAC70, released in 2015; RWR2245 and RWR2154, released in 2016; and NUV30, NUV91 and NUV130 released in 2018 (Buruchara, 2019). These bean varieties have been a game changer for Burundi's and Zimbabwe's bean sectors. The beans have been utilized to promote food and income security through cultivation, consumption and value addition, in addition to contributing to gender-equitable access to higher-yielding bean varieties in the two countries (Buruchara, 2019). However, there is a scarcity of studies in Burundi that detail the cooking properties and nutrient retention, especially of the recently released biofortified common bean varieties. This study aimed to emulate a home cooking preparation environment for common beans varieties and determined water absorption capacity, cooking yield, cooking time, and iron and zinc retention of 10 common bean varieties in Burundi. Findings from this study will inform bean breeders and policymakers in the biofortified common bean value chain on various opportunities and gaps that need further improvement and provide a reference for future bean studies.
2. Materials and methods
2.1. Study sites
The study was conducted at the Guildford Dairy Institute, Department of Dairy, Food Science and Technology, Egerton University, and Kawanda Research Center, Kampala, Uganda. Table 1 gives the names of the bean varieties used in the study and their key nutritional traits. These bean varieties were released from the International Center of Tropical Agriculture (CIAT), Colombia, grown by farmers in Burundi during the long and short cropping seasons in 2019. The bean samples were randomly sourced from reputable retailers in Bujumbura, Burundi. Bean breeders purposively selected mature, dry common bean varieties in Burundi due to their high concentration of iron and zinc and low concentration of phytic acid. The Institute of Agricultural Science of Burundi (ISABU) issued a phytosanitary certificate, and the Kenya Health Inspectorate Service (KEPHIS) issued a plant importation permit with permit number KEPHIS/PIP/000248226. The samples were packaged in weights of 2 kgs and subsequently delivered to Kenya by Dalsey, Hillblom, and Lynn (DHL) services in well-labeled transparent zip lock sample bags and packaged in one opaque carton box. The beans were stored at room temperature in transparent sterilized airtight plastic containers at the Egerton University's Food Chemistry Laboratory until further analyses.
2.2. Experimental design
Dry common bean samples, nine biofortified and one traditional non-biofortified, Kinure, were utilized for the study. Kinure bean variety, was used as the control. The study employed a 10 × 2 completely randomized design (CRD) in a factorial arrangement. The first factor was bean varieties with 10 levels, while the second was pretreatment with two levels, soaking and not soaking. The sample size was 20 common beans before replication. Ten bean samples were soaked and cooked by boiling, while the other 10 were boiled without prior soaking. All experiments were carried out in triplicates. The cooking duration was measured in minutes, while water absorption capacity, cooking yield, iron, and zinc retention were computed as percentages.
2.3. Sample preparation
2.3.1. Raw beans
Samples of different common bean varieties were prepared by sorting and grading according to Biama et al. (2020). A microphyte lab disintegrator model Fz102 (Tianjin, China), fitted with 500-μm, was used to mill 150 g of matured bean grains for each bean variety. The ground beans were then sieved to give whole grain flour and stored at a temperature of 8°C for use in iron and zinc retention studies (pre-cooking analyses).
2.3.2. Cooked beans preparation for laboratory analysis
Grains of various soaked and unsoaked (dry) mature common bean varieties were cooked as described by Biama et al. (2020) with modifications whereby granite pots with glass lids were used in place of aluminum pots without lids, and two pretreatments (soaking and not soaking) employed. Two cooking methods were used: dry for unsoaked bean grains and wet for soaked bean grains. The cooking process was done using non-stick granite pots with a thickness of 0.5 inches, and distilled water was used to clean the pots and glass lids. The cooking pots and glass lids were used to prevent contamination that may interfere with iron and zinc concentration in the different bean varieties. Regular pot cooking was conducted with glass lids half-open at temperatures of 93.5°C (the boiling temperature of water in Njoro) on hot charcoal stoves. Hot distilled water was added to compensate for evaporation loss and reduce frothing and spillage. For the dry cooking process, 150 g of mature, dry raw bean grains from each variety were cooked directly in 1,000 ml of distilled water. For the wet cooking method, 150 g of mature bean grains of each bean variety were first soaked overnight (16 h) in distilled water at room temperature. Soaking water was discarded, and soaked common beans were cooked as described above until the cooking broth was fully absorbed. Bean grains were hand-squeezed for tenderness after dry and wet cooking to ensure complete cooking. Cooked common bean samples were then collected, ice-cooled, and placed in a refrigerator for 24 h at 4°C before analysis awaiting cooking yield, iron, and zinc determination. Cooking and laboratory analysis were done in triplicates for every common bean variety and treatment.
2.4. Cooking properties
2.4.1. Water absorption capacity
The water absorption capacity of various common bean varieties was determined using the soaking method developed by McWatters et al. (2002) and modified by Shafaei et al. (2016). A sub-sample of roughly 200 mature bean grains of each variety were randomly picked, placed in a 6.5 × 14 cm envelope, and stored at room temperature (Biama et al., 2020). Before cooking, samples were stored in a hermetic storage cabinet within a controlled environment (Storage Control Systems, Inc. Sparta, MI, USA) to equilibrate the moisture content of the common bean grains. After achieving a moisture content of 10–14%, 30 common bean grains were selected per entry, weighed, and soaked overnight in a 1:4 (w/v) ratio (bean weight to distilled water). The experiment was done in triplicates. The bean grains were drained and reweighed to assess the percentage of water uptake during soaking Shafaei et al. (2016). The equation used was as follows:
2.4.2. Determination of cooking time
Studies were conducted to determine the cooking time of the different common bean varieties at Kawanda Research Center, Kampala, Uganda. Cooking time was measured for 25 soaked and unsoaked mature common bean grains using a Mattson cooker (Customized Machining and Hydraulics Co., Winnipeg, Canada) in boiling distilled water, according to Mughi (2017). The Mattson cooker is a stand-alone machine monitored by a computer that automatically records the test results on the computer plate. The cooker comprises a plate with 25 wells for holding individual seeds and a metal pin on top of each well (Wang and Daun, 2005). The Mattson cooker is then immersed in a 3-liter beaker and placed on a hot plate with water boiling at about 98°C, and the cooking time is instantly recorded. Hot distilled water was gradually added and maintained at the 1,000 millilitre mark. Cooking time was calculated as the duration it took a 2mm−85g stainless steel rod to fully punch 80% of the bean grains. This proportion is equivalent to when the 20th of the 25 pins of the cooker penetrates the bean grain of the various bean types (Wang and Daun, 2005). Cooking time experiments were determined in triplicates.
2.4.3. Cooking yield
Cooked bean grains of the different common bean varieties from both dry and wet cooking processes were weighed, and the weights were recorded. The cooking yield was then calculated using the formula stated in the United States Department of Agriculture (USDA) Table of Nutrient Retention Factors Release 6 (Haytowitz et al., 2009). The equation is illustrated below.
2.5. Iron and Zinc analyses
Iron and zinc concentrations in raw, dry-cooked, and wet-cooked mature bean grains of several bean types were determined using the method described by Mughi (2017). About 45–50 grams of the bean samples (raw, dry cooked, and wet cooked) were oven-dried at 60°C for at least 12 hrs and then ground using a Sunbeam Conical Burr Mill EM0480 Grinder (Sunbeam, Australia) at a course (Gathu et al., 2012; Zamindar et al., 2013; Santos et al., 2016; Buzera et al., 2018; Biama et al., 2020; Wainaina et al., 2021) setting and subsequently a finer (0–5) setting. The grinder was cleaned in between samples. Ground beans of the different bean varieties were stored in 6.5 × 14 cm envelopes and kept at room temperature for analysis at the Kawanda Research Station-Kampala. An energy-dispersive X-ray fluorescence spectrometer machine (ARL QUANT'X EDXRF Analyser) was used to analyse for Fe and Zn. One set of 10 reference samples was sourced from Flinders University, Australia and used to calibrate the EDXRF machine. The data was validated using a different set of validation samples analyzed via an Inductively Coupled Plasma-Optical Emission Spectroscopy (ICP-OES). These analyses was replicated six times. Data was autogenerated and the relationship between fluorescence intensity (counts/sec) and reference values concentrations (mg/kg) was then established using the EDXRF “calibrate” function. Correlation between EDXRF and reference values were also examined along with standard errors. Limits of quantification were established as 3.4 ± 1.6 and 8.4± mg/kg for Fe and Zn respectively. Five grams of ground bean samples were then transferred into separate sample cups. Iron and zinc concentrations were assessed using spectrophotometry, with each ground sample examined in triplicates for 100 s.
2.6. Determination of nutrient retention
True iron and zinc retention was assessed for various dry and wet cooked mature common bean grains. True nutrient retention (iron and zinc) was then calculated according to Bechoff (2017). The formula used for calculation was as follows:
Where: Fe or Zn = Fe or Zn content of food (μg. g-1) after cooking, Feo or Zno = Fe or Zn content of food (μg. g-1) before cooking, m = mass of food (g) after cooking, m0 = initial mass of food (g).
2.7. Statistical analysis
Prior to data analyses, data on cooking properties and nutrient retention were validated and then analyzed using SAS® software version 9.4 and MINITAB® software version 21. Tests for goodness of fit (Kolmogorov-Smirnov and Cramer-von Mises) were conducted to check for normal distribution of the data. Levene's test and Welch's ANOVA was used to test for homogeneity and ANOVA of squared deviations from grouped means, respectively. ANOVA was conducted to test for hypothesis using PROCGLM procedures. PROC TTEST procedures were used to perform t-tests to determine the effect of pretreatment on cooking properties and nutrient retention for each common bean variety. Multinomial logistics regression was performed using PROC LOGISTICS procedures to quantify the effect of pretreatment on biofortified bean varieties compared to the control (Kinure). Pairwise Pearson's correlation was performed to establish if there was a relationship between response variables for the pretreated common bean varieties. Clustering was done to group response variables with similar behavior. Data were analyzed at a 95% confidence level, and post-hoc analysis was performed using Tukey's Honestly Significant Difference (HSD).
3. Results
3.1. Cooking properties
Figure 1 presents the biofortified bean varieties' water absorption capacity (WAC). Bean grains absorbed water before cooking, with variations from 64.51 to 116.3%. MAC44 and NUV91 bean variety had the highest and lowest WAC, respectively (P < 0.05). In comparison, NUV91 bean variety displayed a lower WAC than the control Kinure (82.03%).
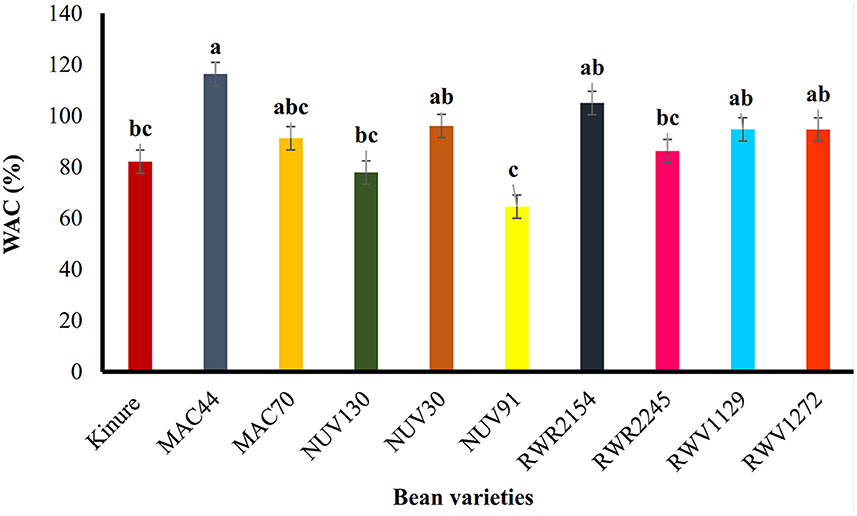
Figure 1. Water absorption capacity (WAC) of the biofortified common bean varieties. Error Bars with the same letters did not differ significantly and those with different letters differed significantly (P < 0.05).
Results presented in Figure 2 indicate that soaking significantly (P < 0.05) reduced cooking time in all bean varieties with soaked beans taking less than half of the total cooking time required to cook unsoaked beans (77.62 vs. 156.86 min, respectively).
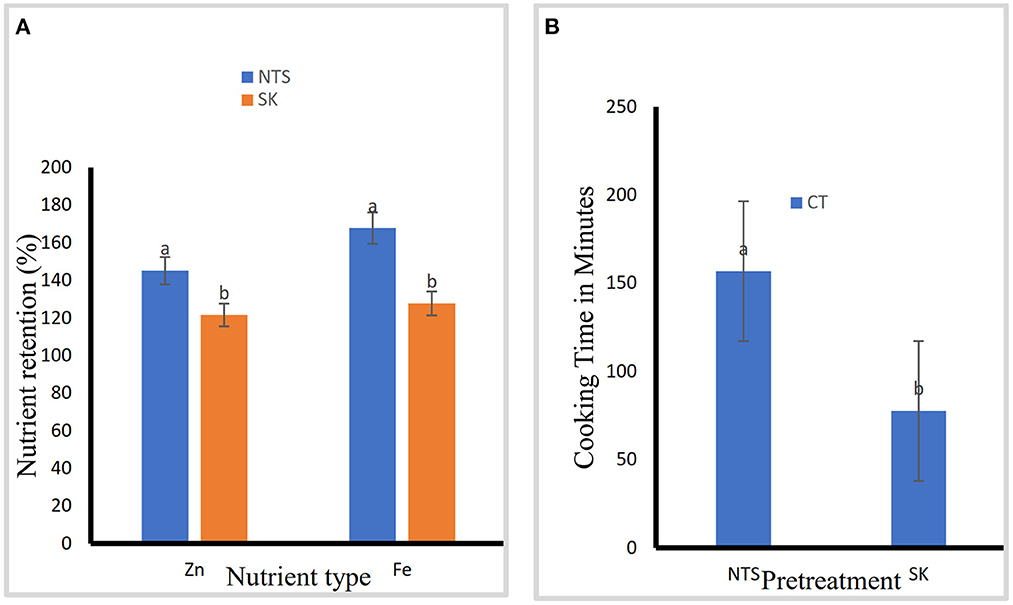
Figure 2. Cooking time, Fe, and Zn retention as affected by soaking. (A) Effect of pre-treatment on nutrient retention. (B) Effect of pre-treatment on cooking time. Key: Values are mean. The letters along the bar are mean separation showing a significant difference at P < 0.05; bars with the same letter are not significantly different. Pre-treatment (SK, soaked; NTS, not soaked; CT, cooking time).
Table 2 depicts the cooking properties of the different bean varieties when cooked without soaking (dry cooking) and after soaking (wet soaking). Cooking time was significantly reduced in all the bean varieties after soaking (P < 0.05). The cooking time was longest for MAC70 (238.95 min) and shortest for NUV130 (121.33 min) for dry-cooked beans. MAC44 had the longest cooking time (106.58 min), while NUV30 and RWR1129 had the shortest cooking time recorded at 61.06 and 61.83 min, respectively, in wet-cooked common bean varieties.
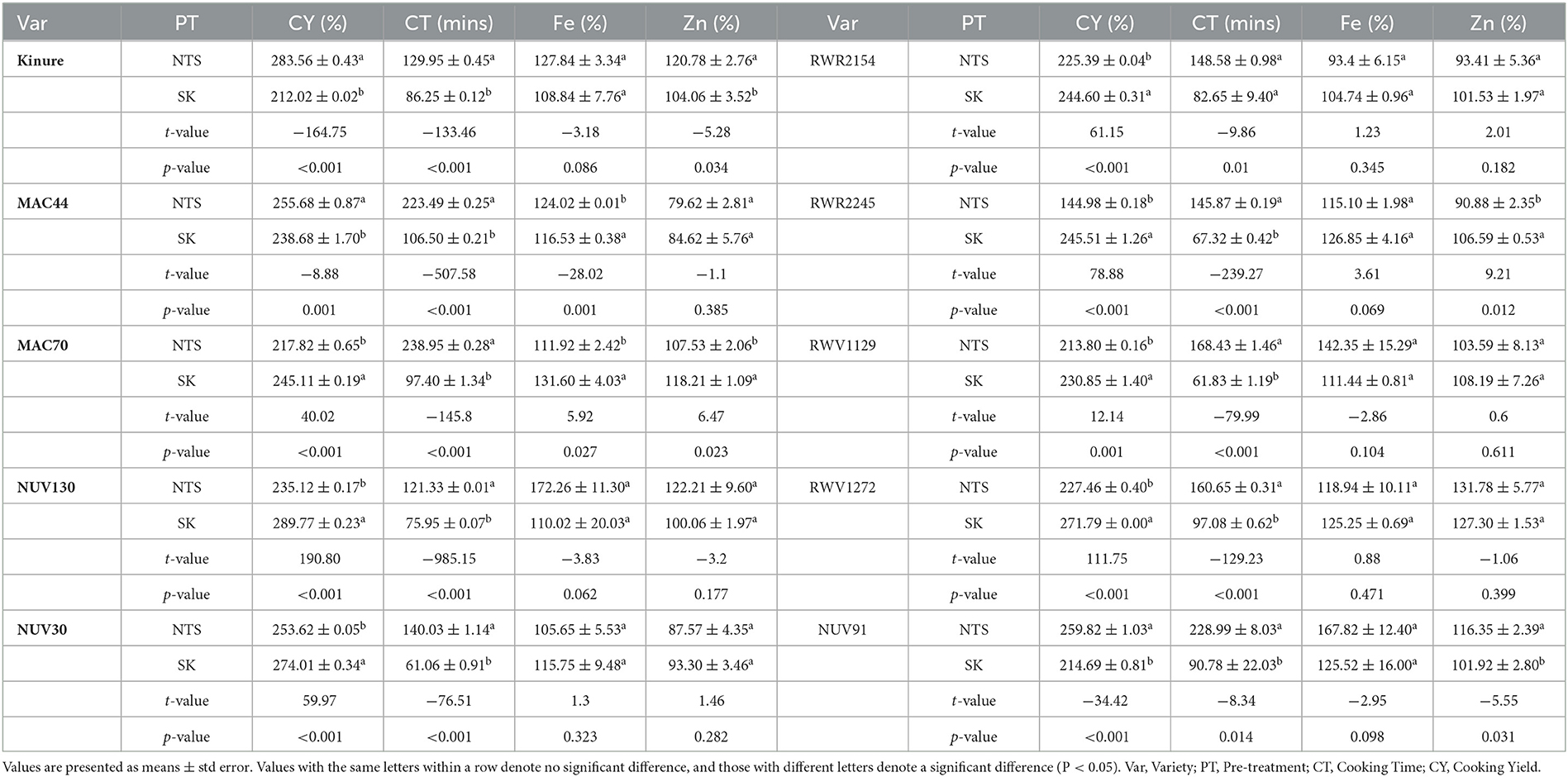
Table 2. Effect of soaking selected biofortified common bean varieties on their cooking properties and mineral retention.
Further results from Table 2 indicate soaking significantly increased cooking yield in other bean varieties except for Kinure (P < 0.001), NUV91 (P < 0.001), and MAC44 (P = 0.001). Kinure had the highest cooking yield for dry-cooked common beans (283.56%) but the lowest for wet-cooked common beans (212.02%). RWR2245 had the lowest cooking yield of 144.98% in dry-cooked common beans, while NUV130 recorded the highest cooking yield (289.77%) in wet-cooked common bean varieties.
Table 3 shows the effect of soaking on cooking properties among the biofortified common bean varieties with reference to Kinure (control). Soaking significantly resulted in lower cooking yield in other biofortified common bean varieties apart from NUV91 by 19.8–65.8% (P < 0.001). Additionally, soaking resulted in longer cooking times in six biofortified common bean varieties, MAC44 and RWR2245 (P < 0.05) and MAC70, NUV30, NUV91, and RWR1129 (P < 0.001), unlike Kinure, by 39.7–82.9%.
3.2. Iron and Zinc retention
Average retentions of Zn and Fe as influenced by soaking are illustrated in Figure 2. Soaking reduced Fe and Zn retention significantly (P < 0.05). Unsoaked beans had averages of 145.15 and 167.8% of Zn, and Fe retention, respectively, after cooking, while soaked beans had averages of 121.58 and 127.74% of Fe and Zn retention, respectively after cooking.
Table 2 further depicts mineral (Fe and Zn) retention of the different common bean varieties when cooked without soaking (dry cooking) and when cooked after soaking (wet soaking). Mineral retention varied significantly (P < 0.05) within the common bean varieties. NUV130 and RWR1272 showed the highest Fe and Zn retention when cooked without soaking, at 172.26 and 131.78%, respectively. RWR2154 had the lowest Fe retention at 93.4%, while MAC44 had the lowest Zn retention at 79.62%. On the other hand, MAC70 and RWR1272 had the highest Fe and Zn retention at 131.60 and 93.4%, respectively, while RWR2154 and MAC44 had the lowest Fe and Zn at 82.65 and 84.62 % sequentially, for wet-cooked common beans.
Table 2 further shows that only five bean varieties exhibited significant variations in nutrient retention upon soaking (P < 0.05). MAC44 variety had a significantly (P = 0.001) higher Fe content when cooked without soaking than with soaking. In contrast, MAC70 retained more Fe after soaking (P = 0.027). Zn retention was on the other hand, significantly reduced (P = 0.034) in Kinure and NUV91 (P = 0.031) but increased significantly in RWR2245 (P = 0.012) and MAC70 (P = 0.023) after wet cooking.
Table 3 shows the effect of soaking on nutrient retention among the biofortified bean varieties with reference to Kinure (control). Soaking increased Fe retention by 33.7% only in NUV130 and decreased Fe retention in MAC70 and RWR2245 by 27.60 and 22.50%, respectively. Regarding zinc retention, soaking reduced Zn retention in variety RWR2245 only by 26.40% (P < 0.05). However, compared to the control, soaking had an insignificant effect on zinc retention (P < 0.05 and P < 0.001) in the other biofortified common bean varieties, apart from NUV130, which had an insignificant increase in Zn retention of 5.3% (P > 0.05).
3.3. Relationship between cooking properties and nutrient retention
Table 4 illustrates the pairwise correlation between the bean varieties' cooking properties and nutrient retention. There was a significant (P < 0.05) correlation between Fe and Zn retention (r = 0.445), WAC and Fe (r = −0.425), and WAC Zn retention (r = −0.477).
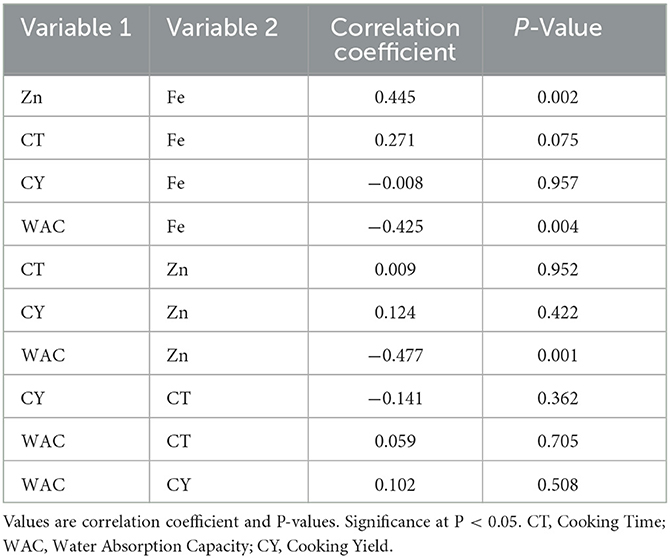
Table 4. Pairwise correlation comparison between cooking properties and nutrient retention of selected soaked common beans.
3.4. Clustering of variables
Figure 3 presents results with regard to clustering of cooking properties and nutrient retention of biofortified common bean varieties based on their correlation coefficient. The similarity in these properties resulted from pretreatment (soaking and not soaking). There was a similarity between iron and zinc (cluster 1), cooking yields and water absorption capacity (Cluster 2), and finally cooking time (Cluster 3).
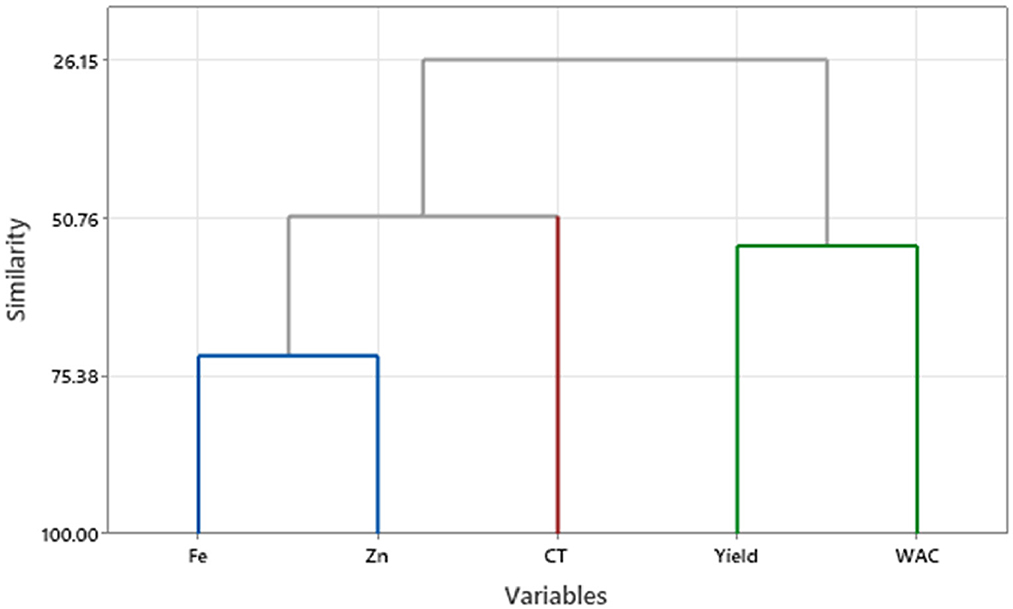
Figure 3. Dendrogram showing clustering of cooking properties and nutrient retention in selected biofortified common bean varieties based on their correlation coefficients.
4. Discussions
The common bean varieties evaluated showed a notable diversity in cooking properties and nutrient retention. Soaking legume is a pretreatment explored and implemented before cooking experiments to reduce cooking time. Soaking tends to affect the food products' morphological and crystalline structure, affecting the cooking properties at higher temperatures and reducing cooking time in the process (Zhu et al., 2019). Cooking time is an ideal quality in legumes and is described as the time required for beans to attain a cooked condition with a desired texture acceptable to customers (Marques Corrêa et al., 2010; Mughi, 2017).
Nutrient retention indicates the amount of nutrients retained after preparation and cooking and the amount available for absorption after intake. The approaches used to calculate nutrient retention are apparent retention (AR) and true retention (TR). The current study, however, used TR because it is more accurate than AR, which tends to overestimate nutrient retention (Bechoff, 2017; Hummel et al., 2020). During soaking, metabolism intensifies as bean grains respire, causing leaching (Santos et al., 2016), hence the retention of lesser nutrients. Soaking water was discarded in this study to eliminate or reduce the concentration of antinutrients such as phytic acid associated with reduced mineral retention and flatulence (Carvalho et al., 2012b).
4.1. Water absorption capacity
Soaking common bean varieties overnight is a simulated home pretreatment technique to ensure beans achieve their maximum water-holding capacity of 11–14 h of soaking (Marques Corrêa et al., 2010). Bean varieties with a high Zn and Fe trait had higher water absorption capacity than other varieties. A study by Buzera et al. (2018) revealed a considerably greater water absorption capacity on soaked biofortified beans in Congo of 199.73% in HM21_7 and 203.75% Namulenga, owing to higher quantities of leached solutes in variety Namulenga than in HM21_7s. The micronutrient biofortification procedure resulted in increased concentrations of Fe and Zn in the bean grains (Buruchara et al., 2011) and therefore, the osmotic potential of the bean grains may have improved (Soltani et al., 2021). In addition, zinc and iron are water-insoluble. However, the minerals have an affinity for water molecules, enhancing the absorption process (Waraich et al., 2011). According to Waraich et al. (2011) and Soltani et al. (2021), the leached solids in the soaking water increase the concentration of the solution, which impacts the water absorption rate. Solute leakage, on the other hand, may reduce water affinity and retaining capacity, according to osmotic principles. Another study on bean cultivars grown in comparable conditions in Brazil found a WAC of 102.33–120.33% (Marques Corrêa et al., 2010). The authors contended that an inverse relationship existed between water absorption capacity, polyphenols, lignin concentration, and peroxide activity (Esteves et al., 2002). This finding indicated that chemical characteristics and endogenous enzymatic activity could influence water absorption. Different grain tegument characteristics, such as thickness, weight, cotyledon adhesion, flexibility, porosity, and colloidal properties, have also been associated with changes in legume water absorption (Aghkhani et al., 2012; Buzera et al., 2018). Considering the important role WAC plays in cooking time, cooking yield, and consumer acceptability, there is a need to further understand the role of micronutrient biofortification on WAC of biofortified common beans.
The present study discerned water absorption of 64.51–116.30%, which corroborates the WAC of 63–137% exhibited in improved beans from seven East African countries, including Burundi (Mughi, 2017). The WAC values in both studies are attributable to the bean grain's moisture content. The hard seed coat mechanism controls water absorption in bean grains (Soltani et al., 2021). In instances where beans have a moisture content of 9%, the hard seed becomes a problem, unlike in grains with a moisture content of 12%, which tend to have a similar water absorption capacity (Soltani et al., 2021). The present study was conducted at 10–14% moisture content, whereas Mughi (2017) studied at 12–13%. This reason explains the similarities in water absorption capacities in both studies associated with the texture of the seed coat. Even though this study did not consider the growth habitat for the various bean varieties, the agronomic environment of different common bean varieties impacts their water-retaining capacities (Mughi, 2017).
In contrast to the current study, greater WAC values have been recorded in improved cowpeas lines in Kenya (104.43–114.17%) owing to the lignin content, which impacts water diffusion into the seed (Biama et al., 2020). The percentage moisture content of these cowpeas was 12.78%, explaining the close range in the WAC in both studies. Seed coat thickness and seed size have been demonstrated to alter the water absorption capacity of legumes, which could also explain the discrepancies in WAC in the two studies. Biofortified common bean varieties utilized in this study are of different sizes with different characteristics. Therefore, in order to understand this discrepancy, further studies on the effect of seed coat and seed size on WAC should be conducted.
4.2. The effect of soaking on cooking time
It is worth noting that soaking reduced the cooking time needed to cook unsoaked beans by half, implying that soaking beans before cooking saves on time and energy needed in bean preparation, whether biofortified or non-biofortified. In the current study, a reduction in cooking time after soaking is also linked to the moisture level of the bean grains, which impacted the hydration capacity of the bean grains, resulting in shorter cooking durations.
In this study, soaking of biofortified bean varieties resulted in significantly longer cooking times in some varieties, such as MAC44, MAC70, RWR1272, and NUV91, than in the control (Kinure). Biofortification introduced Fe and Zn in the bean seeds, and these bean varieties are characterized by a nutrition trait of high iron and zinc content, apart from NUV91. Schoeninger et al. (2014) attributed the increased cooking time to the formation of insoluble iron and zinc pectates on cell walls, restricting dissolution, separation of the cell, and thus the longer cooking time. This observation paves the way for common bean researchers on the possible mechanisms involved in longer cooking time for biofortified beans, unlike traditional non-biofortified bean varieties. Considering Kinure was a negative control (different variety and non-biofortified), studies using a control of a similar non-biofortified bean variety to compare cooking times could enlighten on the elevated cooking times in biofortified common bean varieties. Bean consumers and processors prefer fast-cooking beans because they save energy and time during preparation (Gathu et al., 2012; Wainaina et al., 2021). According to this study, NUV30 and RWR1129 bean varieties which had the shortest cooking times of 61.06 and 61.83 min, are ideal for saving energy and preparation time, and could be more desirable to common bean consumers both at the household and industrial levels. Additionally, the possibilities of adoption and utilization in the production of other common bean products of these two varieties by local farmers and bean consumers could be higher and more sustainable than the other biofortified common bean varieties, which had longer cooking times.
The long cooking time observed in this study for unsoaked beans compares relatively well with cooking times of unsoaked improved common beans, 95 min for RWV2872 to 180 min for Mac9 grown in Rwanda (Vasanthakaalam et al., 2020). Lack of pretreatment (soaking) before cooking slowed down all processes that favor shorter cooking time. Moisture transfer is the rate-limiting factor during the initial stages of cooking unsoaked beans because the bean tissues need to be hydrated to ensure sufficient moisture for processes such as starch gelatinization and protein denaturation (Wainaina et al., 2021) that favor a reduction in the cooking time (Waraich et al., 2011). Furthermore, soaking bean grains increases cell wall enzymatic activities, resulting in changes in the degree of polymerization of pectin, making it more extractable (Soltani et al., 2021). The middle lamella of bean grains composes of a native protopectin. During the cooking process, the protopectin forms soluble pectin that depolymerizes due to heat, causing water to flow rapidly through the cotyledonary cells (Zhu et al., 2019; Soltani et al., 2021). These processes consequently result in a high state of hydration and heating and hence the cookability of the bean seeds. As a result of these modifications, cell wall rigidity decreases, intercellular adhesion decreases, and bean cooking time decreases (Martínez-Manrique et al., 2011).
Other factors that may have resulted in lengthier cooking times include longer storage time under high temperatures and humidity, either on the farm or on retailing shelves leading to the development of the hard-to-cook phenomenon (Soltani et al., 2021). In this study, we observe that the variations in moisture content, water absorption capacity, and softening rate differed among the biofortified common bean varieties. More research on the hard-to-cook phenomenon and cultivation conditions for these particular biofortified common bean varieties can be conducted to aid in understanding the longer cooking times. The Pan African Bean Research Alliance (PABRA) bean program seeks to benefit local bean farmers, and consumers across Africa by utilizing these beans for improved food and income security. The inclusion of education outreaches on effective handling and storage practices of biofortified common beans to counteract such an effect could benefit bean consumers and producers.
Cooking time for beans ranges from 45 to 230 min, depending on the variety, cooking method, and cooking medium (Arruda et al., 2012). Current findings on cooking time are consistent with studies that reported cooking times ranging from 81 to 101 min for common bean varieties in Malawi (Marques Corrêa et al., 2010) and 35 to 122 min in improved common beans in Eastern Africa regions (Mughi, 2017). These researchers attributed the observed cooking durations to differences in bean varieties, bean seed characteristics such as the bean's skin, grain thickness, flatness, and physicochemical parameters related to the cotyledons (Santos et al., 2016).
According to Schoeninger et al. (2014), cooking time depends mainly on the genotype and the soaking time. Considering that the soaking duration and conditions were similar for all common bean varieties in this study, discrepancies in cooking time could be a result of bean variety. Additionally, the high WAC observed could have resulted from more imbibed water acting as a catalyst for cooking and thus softening the cells and allowing for cell separation. A previous study varied the soaking times in legumes to diversify further soaking intervals and cooking times and their role in the utilization of beans by consumers and producers at household and industrial scales (Zamindar et al., 2013).
4.3. The effect of soaking on cooking yield
The present study's cooking yield was similar between soaked and unsoaked beans. The increased cooking yield associated with soaking could imply an increase in retained water in the common bean grain. Beans with a high WAC tend to increase or yield more after cooking hence more appealing to consumers both at a household and industrial scale (Biama et al., 2020). From the present study, all bean varieties have a good cooking yield since the percentage yield between dry and wet cooked common beans did not change considerably. The lower cooking yield observed in all biofortified beans compared to Kinure implies that the soaking effect on cooking yield was thus less in the biofortified beans than in the control variety.
4.4. The effect of soaking on Fe and Zn retention
The question of whether to discard soaking water used in bean preparation before cooking remains debatable. Despite soaking and boiling, most common bean varieties exhibited Fe retention values above 100%, and earlier studies report similar findings (Wang et al., 2010). The modest increase in minerals could be linked to the fact that some soluble solids are lost during cooking, leading to an increased concentration of the minerals in the bean grains. Although the soaking water was discarded before boiling, cooking was done under controlled conditions with similar cooking temperatures and constant water levels of boiling water. These conditions allowed for reduced frothing and little to no spillage of the bean broth; hence, a possible explanation for the retention values above 100% observed in most cooked common bean varieties. For varieties with < 100% Fe retention, we attribute this observation to the diversity of the bean varieties. On the contrary, Granito et al. (2007) observed that soaking and cooking without the soaking water resulted in a considerable loss of minerals. However, utilizing these beans with the soaking water and cooking broth led to significant mineral retention (Huma et al., 2008).
Overall, zinc retention was lower than that of iron between soaked and unsoaked beans and when compared to the control, Kinure. A study by Carvalho et al. (2012a) revealed that the zinc level in cooked bean grains and broth did not differ. Although our study did not test for zinc concentration in the broth, we concluded that most of the zinc was retained during boiling and concentrated in the cooked bean. Furthermore, the insignificant increase in zinc could have resulted in less leaching of the mineral since zinc is insoluble in water (Wainaina et al., 2021).
Results from another study by Hummel et al. (2020) indicated that soaking led to a substantial decrease in Zn, but no significant differences were found for Fe (P > 0.05). This observation is linked to cooking affecting the extractability of micronutrients such as zinc, while fermentation increases the extractability of iron (Carvalho et al., 2012a). Therefore, besides soaking as a pretreatment before cooking common beans, other pretreatments, such as fermentation, can be performed, and their effect on nutrient retention of these biofortified common bean varieties further explored. On the contrary, Huma et al. (2008) reported that soaking and cooking significantly reduced the concentration of minerals. During traditional cooking, especially in Burundi and other African countries, cooking conditions are not controlled and the spillage of froth is higher. Minerals are lost through the process and hence less retention. It is therefore, important to educate the population to adopt improved bean preparation procedures with minimal loss of bean broth or until the broth is fully absorbed by the bean grains to allow for less mineral loss and more mineral concentration in the common bean grains. Research incorporating the mineral content of both the biofortified common bean broth and the bean grains can also be conducted to compare their concentrations.
4.5. The Relationship between cooking properties and nutrient retention
In this study, Fe and Zn retention showed a significant positive correlation, meaning a unit increase in either iron or zinc would result in a 0.45 increase in either mineral. However, we contend that the relationship is weak. These results are explained by Izquierdo et al. (2018), who found many closely linked quantitative trait loci in common beans within the same interval as a major contributor to this trait. Furthermore, the highly significant positive association between these micronutrient concentrations in bean seeds depicts that Fe and Zn concentration-increasing genetic factors co-segregate (Mughi, 2017; de Lima et al., 2021). As a result, for any biofortification bean breeding program, selecting beans with a high concentration of either Fe or Zn could help concentrate both elements positively.
These findings confirm those of Nchimbi-Msolla and Tryphone (2010), Mukamuhirwa et al. (2015), Mughi (2017) and de Lima et al. (2021) that indicated a significant positive correlation (P < 0.05) between Fe and Zn. The differences in the common bean genotypes in these studies contributed to the observed association. In this work, however, indirect selection for high Fe beans can be done by improving the Zn content since its retention was insignificant and quite similar to that of the control variety, Kinure. This observation is important as it confirms earlier possibilities of developing beans with a concentration of both minerals and hence a supply of more micronutrients to the population. Zinc is still a micronutrient of major public health concerns, especially in Eastern Africa. However, an earlier study observed the possibility of accumulating zinc concentration only (Gelin et al., 2007). We conclude that the need for increasing the concentration of either Fe and Zn or both minerals solely depends on the objective of a particular common bean breeding program.
The significant negative correlation between WAC and Fe and WAC and Zn indicates an inverse relationship between the variables. A unit increase in either variable could cause a 0.43 and a 0.48 decrease, respectively. This observation can result from the biofortification process and hence a need for in-depth research on water absorption mechanisms for biofortified and improved common bean varieties. This observation additionally implies that the hydration capacity of these biofortified common bean varieties may be indirectly used when selecting biofortified common bean varieties with high Fe and Zn retention.
5. Conclusion and recommendations
Conclusively, the micronutrient biofortification process has achieved its goal of first improving iron and zinc concentration since these two minerals had the greatest similarity in terms of behavior. The process also impacted more on water absorption capacity, and cooking yield, and least on cooking times.
In the present study, soaking biofortified common bean varieties resulted in shorter cooking times than in unsoaked beans due to hydration facilitating the softening process. The mineral content of biofortified common bean significantly impacted nutrient retention, explaining mineral retention of more than 100% in some common bean varieties despite soaking as a pretreatment. This observation is possibly due to increased WAC, expedited leaching, or higher mineral concentrations causing greater diffusive flux. Biofortified common beans however, recorded longer cooking times than the traditional non-biofortified Kinure. The higher Fe and Zn retention recorded in the biofortified common bean varieties, particularly MAC70 and RWR1272, confirms that the biofortified common bean varieties are a good source of iron and zinc. Biofortified common beans can therefore be utilized in daily diets as a source of both iron and zinc while simultaneously saving on the time and energy needed in preparation.
Considering this is the first study incorporating the nine biofortified common beans released in Burundi under the country's current Pan African Bean Research Alliance bean breeding program, there is need for comprehensive research standardizing the mechanisms of water absorption capacity and nutrient retention among these bean varieties. Moreover, there is a need for further studies to investigate the concentration of Fe and Zn in the soaking water discarded before cooking such beans. Factors such as altitudes, growth environment, and storage conditions after harvesting also need to be well-understood before interpreting cooking properties and nutrient retention, as these components greatly impact on these parameters. In conclusion, the RWR1129 bean variety can be utilized both at the household level and industrial scale due to its significant Fe and Zn retention even after soaking, cooking yield, and considerably shorter cooking time. These traits confirm that common bean biofortification programs can concurrently produce varieties with desirable cooking characteristics and nutritional properties in terms of iron and zinc retention. Consequently, utilizing these biofortified common bean varieties in diets would contribute to the alleviation of nutrition-related anemia and other health complications associated with both iron and zinc deficiency among vulnerable populations in low-income developing countries.
Data availability statement
The original contributions presented in the study are included in the article/Supplementary material, further inquiries can be directed to the corresponding author/s.
Author contributions
MM designed and conducted the study. The manuscript was written by MM and reviewed by LW, RF, SM, and AK. All authors contributed to the article and approved the submitted version.
Funding
The International Center for Tropical Agriculture (CIAT) and the Center of Excellence in Sustainable Agriculture and Agribusiness Management (CESAAM) funded this research work.
Acknowledgments
The Pan-Africa Bean Research Alliance (PABRA) supported the study under the Improving food security, nutrition, incomes, natural resource base and gender equity for better livelihoods of smallholder households in Sub-Saharan Africa project funded by the Swiss Agency for Development and Cooperation. Thanks to the Institute of Agricultural Science of Burundi (ISABU), Burundi, for helping with the acquisition of common bean varieties, the team at Pan-Africa Bean Research Alliance (PABRA) at the National Agricultural Research Organization-Kawanda station and the Rwanda Agriculture and Animal Resources Development Board (RAB) micronutrients analysis lab for their input in the determination of cooking properties, all reviewers, and my academic supervisors for their constructive comments and feedback, my friends and family for their immense support.
Conflict of interest
The authors declare that the research was conducted in the absence of any commercial or financial relationships that could be construed as a potential conflict of interest.
Publisher's note
All claims expressed in this article are solely those of the authors and do not necessarily represent those of their affiliated organizations, or those of the publisher, the editors and the reviewers. Any product that may be evaluated in this article, or claim that may be made by its manufacturer, is not guaranteed or endorsed by the publisher.
Supplementary material
The Supplementary Material for this article can be found online at: https://www.frontiersin.org/articles/10.3389/fsufs.2023.1062978/full#supplementary-material
Abbreviations
NTS, Not Soaked; SK, Soaked; CT, Cooking Time; CY, Cooking Yield; NT, Nutrient retention; WAC, Water Absorption Capacity; AR, Apparent Retention; TR, True Retention.
References
Aghkhani, M., Ashtiani, S. H., Motie, J., and Abbaspour-Fard, M. (2012). Physical properties of christmas lima bean at different moisture content. Int. Agrophysics. 26, 341–346. doi: 10.2478/v10247-012-0048-0
Arruda, B., Guidolin, A., Coimbra, J. L., and Battilana, J. (2012). Environment is crucial to the cooking time of beans. Food Sci. Technol. 32, 573–578. doi: 10.1590/S0101-20612012005000078
Bechoff, A. (2017). Micronutrient (provitamin A and iron/zinc) retention in biofortified crops. African J. Food Agric. Nutr. Dev. 17, 11893–11904. doi: 10.18697/ajfand.78.HarvestPlus04
Bechoff, A., Taleon, V., Carvalho, L. M., Carvalho, J. L., and Boy, E. (2017). Micronutrient (provitamin A and iron/zinc) retention in biofortified crops. African J. Food Agric. Nutr. Dev. 7, 11893–11904.
Biama, P., Faraj, A., Mutungi, C., Osuga, I., and Kuruma, R. (2020). Nutritional and technological characteristics of new cowpea (Vigna unguiculata) lines and varieties grown in Eastern Kenya. Food Nutr. Sci. 11, 416–430. doi: 10.4236/fns.2020.115030
Bouis, H., Hotz, C., McClafferty, B., Meenakshi, J, and Pfeiffer, W. (2011). Biofortification: a new tool to reduce micronutrient malnutrition. Food Nutr. Bull. 32, S31–40. doi: 10.1177/15648265110321S105
Bouis, H., Low, J., Mcewan, M., and Mcewan, M. (2013). Biofortification: Evidence and Lessons Learned Linking Agriculture and Nutrition. Available online at: http://www.fao.org/publications (accessed September 4, 2022).
Buruchara, R. (2019). PABRA Narrative Cumulative Report 2015-2018. Pan-Africa Bean Research Alliance (PABRA). Available online at: https://cgspace.cgiar.org/bitstream/handle/10568/105583/Technical_report_v5.pdf?sequence=1&isAllowed=y (accessed November 16, 2022).
Buruchara, R., Chirwa, R., Sperling, L., Mukankusi, C., Rubyogo, J, Mutonhi, R., et al. (2011). Development and delivery of bean varieties in Africa: the pan-africa bean research alliance (PABRA) model. African Crop Sci J. 19, 227–245. Available online at: https://www.ajol.info/index.php/acsj/article/view/74168
Buzera, A., Kinyanjui, P., Ishara, J., and Sila, D. (2018). Physical and cooking properties of two varieties of bio-fortified common beans (Phaseolus Vulgaris L.) grown in DR Congo. Food Sci. Qual. Manag. 71, 2225–2557.
Carvalho, A. F., de Sousa, N., Farias, D., da Rocha-Bezerra, L. C., da Silva, R. M., Viana, M., et al. (2012b). Nutritional ranking of 30 Brazilian genotypes of cowpeas including determination of antioxidant capacity and vitamins. J. Food Compos. Anal. 26, 81–88. doi: 10.1016/j.jfca.2012.01.005
Carvalho, L. M. J., Corrêa, M. M., Pereira, E. J., Nutti, M. R., Carvalho, J. L. V., Ribeiro, E. M. G., et al. (2012a). Iron and zinc retention in common beans (Phaseolus vulgaris L.) after home cooking. Food Nutr. Res. 56:15618. doi: 10.3402/fnr.v56i0.15618
de Lima, J., Rivadavea, W., Kavalco, S. A., Junior, A. C., Lopes, A., and da Silva, G. (2021). Chemical and nutritional characterization of bean genotypes (Phaseolus vulgaris L.). AIMS Agric Food. 6, 932–944. doi: 10.3934/agrfood.2021056
Díaz-Gómez, J., Twyman, R., Zhu, C., Farré, G., Serrano, J., Portero-Otin, M., et al. (2017). Biofortification of crops with nutrients: factors affecting utilization and storage. Curr. Opin. Biotechnol. 44, 115–123. doi: 10.1016/j.copbio.2016.12.002
Esteves, A. M., Abreu, C. M. P., Santos, C. D., and Corrêa, A. D. (2002). Chemical and enzimatic comparison of six bean lineages (Phaseolus vulgaris L.). Ciência Agrotecnol. 26, 999–1005. Available online at: https://agris.fao.org/agris-search/search.do?recordID=BR2003001327
FAO, IFAD, UNICEF, WFP, WHO. (2019). The State of Food Security and Nutrition in the World. Safeguarding Against Economic Slowdowns and Downtuns. Rome. FAO: Licence:CC BY-NC-SA 3.0 IGO.
Fernandes, A., Nishida, W., and Da Costa Proença, R. (2010). Influence of soaking on the nutritional quality of common beans (Phaseolus vulgaris L.) cooked with or without the soaking water: a review. Int. J. Food Sci. Technol. 45, 2209–2218. doi: 10.1111/j.1365-2621.2010.02395.x
Fungo, R., Katungi, E., Nduwarugira, E., Ntukumazina, N., Mukankusi, C., Elvira, O., et al. (2020). Biofortified Beans: An Agricultural Investment for Nutrition, Income and Food Security in Burundi. Kampala: SDC Burundi Policy Brief.
Gathu, E., Karuri, E., Njage, P. M. (2012). Physical characterization of new advanced drought tolerant common bean (Phaseolus vulgaris) lines for canning quality. Am. J. Food Technol. 7, 22–28. doi: 10.3923/ajft.2012.22.28
Gelin, J., Forster, S., Grafton, K., McClean, P., and Rojas-Cifuentes, G. (2007). Analysis of seed zinc and other minerals in a recombinant inbred population of navy bean (Phaseolus vulgaris L.). Crop Sci. 47, 1361–1366. doi: 10.2135/cropsci2006.08.0510
Gilligan, D. (2012). Biofortification, agricultural technology adoption, and nutrition policy: some lessons and emerging challenges. CESifo Econ Stud. 58, 405–421. doi: 10.1093/cesifo/ifs020
Granito, M., Brito, Y., and Torres, A. (2007). Chemical composition, antioxidant capacity and functionality of raw and processed Phaseolus lunatus. J. Sci. Food Agric. 87, 2801–2809. doi: 10.1002/jsfa.2926
Haytowitz, D., Lemar, L., and Pehrsson, P. (2009). USDA's nutrient databank system – a tool for handling data from diverse sources. J. Food Compos. Anal. 22, 433–441. doi: 10.1016/j.jfca.2009.01.003
Huma, N., Anjum, M., Sehar, S., Issa Khan, M., and Hussain, S. (2008). Effect of soaking and cooking on nutritional quality and safety of legumes. Nutr Food Sci. 38, 570–577. doi: 10.1108/00346650810920187
Hummel, M., Talsma, E., Taleon, V., Londoño, L., Brychkova, G., Gallego, S., et al. (2020). Iron, zinc and phytic acid retention of biofortified, low phytic acid, and conventional bean varieties when preparing common household recipes. Nutrients. 12, 658. doi: 10.3390/nu12030658
Izquierdo, P., Astudillo, C., Blair, M., Iqbal, A., Raatz, B., and Cichy, K. (2018). Meta-QTL analysis of seed iron and zinc concentration and content in common bean (Phaseolus vulgaris L.). Theor. Appl. Genet. 131, 1645–1658. doi: 10.1007/s00122-018-3104-8
Katungi, E., Farrow, A., Chianu, J., Sperling, L., and Beebe, S. (2009). Common bean in Eastern and Southern 608 Africa: a situation and outlook analysis. Int. Cent. Trop. Agric. 61, 1–609. Available online at: https://www.researchgate.net/publication/228601612_Common_bean_in_Eastern_and_Southern_Africa_a_situation_and_outlook_analysis
Katungi, E., Nduwarigira, E., Nepomuscene, N., Niragira, S., Mutua, M., Kalemera, S., et al (2020). Impacts of improved bean technology adoption among smallholder farmers in Burundi SDC Flagship Project End Line Report 2020. Available online at: https://hdl.handle.net/10568/109119 (accessed September 6, 2022).
Marques Corrêa, M., Jaeger de Carvalho, L., Regini Nutti, M., Luiz Viana de Carvalho, J., and Hohn Neto, A, Gomes Ribeiro, E. (2010). Water absorption, hard shell and cooking time of common beans (phaseolus vulgaris l.). Afr. J. Food Sci. Technol. 1, 13–20. Available online at: http://www.alice.cnptia.embrapa.br/alice/handle/doc/867957
Martínez-Manrique, E., Jacinto-Hernández, C., Garza-García, R., Campos, A., Moreno, E., and Bernal-Lugo, I. (2011). Enzymatic changes in pectic polysaccharides related to the beneficial effect of soaking on bean cooking time. J. Sci. Food Agric. 91, 2394–2398. doi: 10.1002/jsfa.4474
Mbonankira, J., Limborta, L., Kissa, C., Luttsa, S., Nizigiyimana, A., Bertin, P., et al (2016). Recherche Agronomique : Amont de l'Agriculture et de l'Elevage au Burundi. Available online at: https://isabu.bi/wp-content/uploads/2021/09/BULLETIN-N%C2%B07.pdf (accessed March 11, 2023).
McWatters, K., Chinnan, M., Phillips, R., Beuchat, L., Reid, L., and Mensa-Wilmot, Y. (2002). Functional, nutritional, mycological, and akara-making properties of stored cowpea meal. J. Food Sci. 67, 2229–2234. doi: 10.1111/j.1365-2621.2002.tb09532.x
Moloto, R., Moremi, L., Soundy, P., and Maseko, S. (2018). Biofortification of common bean as a complementary approach to addressing zinc deficiency in South Africans. Acta. Agric. Sc. Sect. B Soil Plant Sci. 68, 575–584. doi: 10.1080/09064710.2018.1454507
MSPLS (2017). M at the P in charge of GG and P [Burundi] (MPBGP), Ministry of Public Health and the Fight against AIDS [Burundi], Institute of Statistics and Economic Studies of Burundi (ISTEEBU), ICF. Third Demographic and Health Survey. Bujumbura, Burundi: ISTEEBU, MSPLS, and ICF.
Mughi, I. (2017). Inheritance and Characterization of Cooking Time, Seed Iron and Zinc content in Selected African Common Bean Germplasm (Thesis) Master of Science in Plant Breeding and seed systems. Makerere University. Kampala. UG, 74. Available online at: https://hdl.handle.net/10568/96178 (accessed March 11, 2023).
Mukamuhirwa, F., Tusiime, G., Edema, R., Mukankusi, M., Gibson, P. T., and Edema, R. (2015). Characterization and identification of potential sources of high seed iron and zinc content among uganda common bean germplasm. Ambit J Agric. 1, 1–16. Available online at: http://www.ambitjournals.org/ajar
Nchimbi-Msolla, S., and Tryphone, G. (2010). The effects of the environment on iron and zinc concentrations and performance of common bean (Phaseolus vulgaris L.) genotypes. Asian J. Plant Sci. 9, 455–462. doi: 10.3923/ajps.2010.455.462
Ngozi, F. (2013). The role of biofortification in the reduction of micronutrient food insecurity in developing countries. African J. Biotechnol. 12, 5559–5566.
Samtiya, M., Aluko, R., and Dhewa, T. (2020). Plant food anti-nutritional factors and their reduction strategies: an overview. Food Prod. Process Nutr. 2, 6. doi: 10.1186/s43014-020-0020-5
Santos, G., Ribeiro, N., and Maziero, S. (2016). Evaluation of common bean morphological traits identifies grain thickness directly correlated with cooking time. Pesqui Agropecuária Trop. 46, 35–42. doi: 10.1590/1983-40632016v4638191
Schoeninger, V., Coelho, S. R., Christ, D., and Sampaio, S. (2014). Processing parameter optimization for obtaining dry beans with reduced cooking time. LWT Food Sci. Technol. 56, 49–57. doi: 10.1016/j.lwt.2013.11.007
Shafaei, S., Masoumi, A., and Roshan, H. (2016). Analysis of water absorption of bean and chickpea during soaking using Peleg model. J. Saudi Soc. Agric. Sci. 15, 135–144. doi: 10.1016/j.jssas.2014.08.003
Soltani, A., Walter, K., Wiersma, A., Santiago, J., Quiqley, M., Chitwood, D., et al. (2021). The genetics and physiology of seed dormancy, a crucial trait in common bean domestication. BMC Plant Biol. 21, 58. doi: 10.1186/s12870-021-02837-6
Vasanthakaalam, H., and Karayire, A., and Nyagahungu, I. (2020). Hydration and culinary profile of improved common bean (Phaseolus vulgaris L) cultivars. Rwanda J. Agri. Sci. 2, 68–75.
Wainaina, I., Wafula, E., Sila, D., Kyomugasho, C., Grauwet, T., Van Loey, A., et al. (2021). Thermal treatment of common beans (Phaseolus vulgaris L.): Factors determining cooking time and its consequences for sensory and nutritional quality. Comprehen. Rev. Food Sci. Food Saf. 20, 3690–3718. doi: 10.1111/1541-4337.12770
Wang, N., and Daun, J. (2005). Determination of cooking times of pulses using an automated Mattson cooker apparatus. J. Sci. Food Agric. 85, 1631–1635. doi: 10.1002/jsfa.2134
Wang, N., Hatcher, D., Tyler, R., Toews, R., and Gawalko, E. (2010). Effect of cooking on the composition of beans (Phaseolus vulgaris L.) and chickpeas (Cicer arietinum L.). Food Res. Int. 43, 589–594. doi: 10.1016/j.foodres.2009.07.012
Waraich, E. A., Ahmad, R., and Ashraf, M. Y. (2011). Role of mineral nutrition in alleviation of drought stress in plants. Aust. J. Crop Sci. 5, 764–777. doi: 10.3316/informit.282340708899391
Zamindar, N., Baghekhandan, M., Nasirpour, A., and Sheikhzeinoddin, M. (2013). Effect of line, soaking and cooking time on water absorption, texture and splitting of red kidney beans. J. Food Sci. Technol. 50, 108–114. doi: 10.1007/s13197-011-0234-2
Keywords: biofortification, varieties, micronutrient deficiencies, common beans, cooking properties, nutrient retention
Citation: Muroki MW, Waswa LM, Fungo R, Kabwama A and Mahungu SM (2023) Cooking properties and nutrient retention of biofortified common bean (Phaseolus vulgaris) varieties: The case of Burundi. Front. Sustain. Food Syst. 7:1062978. doi: 10.3389/fsufs.2023.1062978
Received: 06 October 2022; Accepted: 27 February 2023;
Published: 31 March 2023.
Edited by:
Rakesh Bhardwaj, National Bureau of Plant Genetic Resources (ICAR), IndiaReviewed by:
Wasiu Awoyale, Kwara State University, NigeriaKarl Kunert, University of Pretoria, South Africa
Copyright © 2023 Muroki, Waswa, Fungo, Kabwama and Mahungu. This is an open-access article distributed under the terms of the Creative Commons Attribution License (CC BY). The use, distribution or reproduction in other forums is permitted, provided the original author(s) and the copyright owner(s) are credited and that the original publication in this journal is cited, in accordance with accepted academic practice. No use, distribution or reproduction is permitted which does not comply with these terms.
*Correspondence: Mary W. Muroki, bWFyeXdhbWJ1aW11cm9raSYjeDAwMDQwO2dtYWlsLmNvbQ==