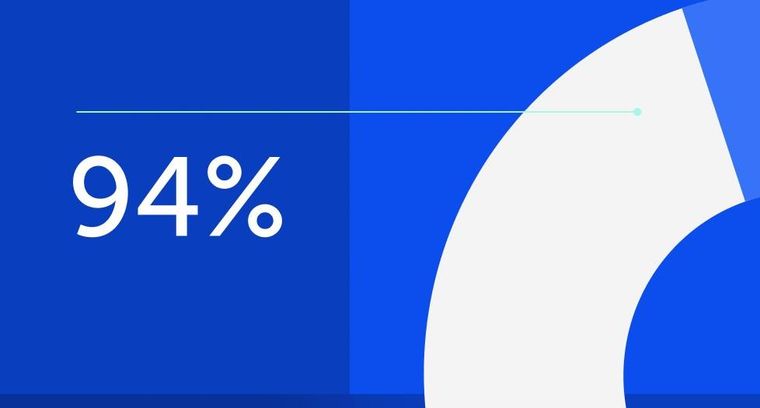
94% of researchers rate our articles as excellent or good
Learn more about the work of our research integrity team to safeguard the quality of each article we publish.
Find out more
ORIGINAL RESEARCH article
Front. Sustain. Food Syst., 02 October 2023
Sec. Agro-Food Safety
Volume 7 - 2023 | https://doi.org/10.3389/fsufs.2023.1057123
This article is part of the Research TopicFood Safety in Low and Middle Income CountriesView all 25 articles
Poor energy and nutrient-dense complementary food for infants have resulted in malnutrition and poor growth. Some processes are known to improve the nutritional value while reducing the antinutrient factors in food formulations. Maize-soybean-based composite flours from nixtamalized maize and heat-treated soybean were used to formulate six composite flours (CFs). The proximate composition, mineral content, antinutritional effect, mineral molar ratios, and mycotoxin level were investigated. The nixtamalization of maize and the heat treatment of soybean substantially improved the nutritional properties of the CFs. The pH value varied with the nixtamalization process, from 6.82 ± 0.7 to 9.32 ± 0.4. The energy content of the complementary foods was in the range of 354.77 ± 3.2 to 429.56 ± 4 kcal, meeting the minimum standard set for a cereal-based CF, which is 400 kcal. The protein values of the CFs (9.48 ± 0.3 to 13.92 ± 1.92%) on a dry weight basis were slightly below 15% of the recommended levels set by Codex. The antinutrient levels were reduced with nixtamalization and heat treatment. The molar ratio of phytate to minerals (calcium, zinc, and magnesium) was lower than the reported critical value, except for phytate to iron, where only whole maize, nixtamalized maize, and composite flour 3 (CF3) had a molar ratio that exceeded the reported critical value of 1. Although all composite flours were contaminated with mycotoxin, aflatoxin content in CFs ranged from 1.35 ± 0.67 to 13.8 ± 0.29 g/kg and from 0.3 ± 0.07 to 0.63 ± 0.015 mg/kg for fumonisin. Only the control and CFs made from the untreated maize did not meet the EU regulatory threshold (4.0 g/kg) for total aflatoxins and total fumonisin (4 mg/kg). The soybeans were not contaminated with the mycotoxins. The composite flour 5 (Composite flour 25% HS + 75% NixM) can be considered the best composite flour with regard to its nutritional properties, mineral, antinutrient, and mycotoxin content. These findings have shown that nixtamalization and heat treatment can improve the nutritional properties and food safety of composite flours.
Malnutrition is a situation in which there is a deficiency or excess of nutrients in the population’s diet, causing the deaths of more than half of the children in the world (FAO, 2022). Although the entire world population suffers from various nutritional deficiencies, people with low incomes are the most affected, particularly in developing countries where the majority have to compromise on the nutritional quality of the food they consume as well as reduce consumption (Erokhin et al., 2021).
When there is a lack of nutrients like protein, carbohydrates, and vitamins, malnutrition can also impede intellectual development and reduce working capacity in adults (Arimond et al., 2008; UNICEF, 2020). Regarding the statistics in the Democratic Republic of Congo (DRC), from 2006 to 2016, Kuamba (2016) reported that the proportion of children who suffer from stunting is constant and affects at least 42% of children in the country. The most affected regions are South Kivu and Kasai provinces, with a rate of 48 and 52% malnourished children, respectively (Kuamba, 2016). Only three crops—wheat, rice, and corn—provide half of the world’s calories, but these grains are also deficient in protein and numerous minerals (Campbell et al., 2016). In diets, legumes supplement cereals; they provide significant protein, necessary amino acids, and minerals and can be used in place of meat when it is unavailable or pricey (Singh et al., 2022). Due to its high protein content, soybean is a common legume used for enriching porridges served at breakfast for the whole family and as a complementary food for infants and young children in sub-Saharan Africa (Flax et al., 2010; Mangani et al., 2015). However, the uncooked soybean flavors are often described as “painful and unpleasant” and make the legume unpalatable (Wibke et al., 2017). Soybeans are usually roasted or extruded with maize to get rid of antinutritional factors and unpleasant tastes. This helps reduce the time it takes to make porridge (Kalumbi et al., 2019). In sub-Saharan Africa, people eat maize-based porridges that are mostly flat and tasteless, and any change from this norm is likely to be rejected (Stevens and Winter-Nelson, 2008; Khumalo et al., 2011; Pillay et al., 2011; De Groote and Kimenju, 2012). Similarly, it is also impractical to use a raw corn-soybean meal mixture, as it takes a long time to cook and this form of preparation retains unpleasant flavors (Kalumbi et al., 2019).
Maize is a staple food and has the most significant calorie contribution in South Kivu (Smale et al., 2013; CTA, 2015). However, it is one of the commodities that is prone to contamination by mycotoxins. The studies conducted by Matendo et al. (2022) and Udomkun et al. (2018) show that the prevalence of aflatoxin in maize in South Kivu was very high, with a range between 3.1–2,806.5 μg/kg. Aflatoxins are toxins that occur naturally due to Aspergillus spp. metabolism (Ayo et al., 2018). Therefore, aflatoxin-contaminated maize poses a serious problem, rendering it unsuitable for human and livestock consumption (Zain, 2011). Soybean has been reported to be less contaminated by mycotoxins. Niyibituronsa et al. (2018) reported an aflatoxin prevalence of 0.023% in soybean in Rwanda, with only 0.003% of samples above the most stringent EU maximum permitted limit of 4 μg/kg. Even when contaminated with A. flavus, previous research has shown that soybeans are not a good substrate for aflatoxin production. This means that soybeans can be promoted as a healthy and aflatoxin-free food (Niyibituronsa et al., 2018).
The relationship between aflatoxins and the childhood disease kwashiorkor is unclear. Although kwashiorkor is widely thought to be a form of protein-energy malnutrition, there are hypotheses suggesting that some characteristic features of the disease are known to be among the pathological effects caused by aflatoxins in animals (Williams et al., 2004, 2010). Also, specific antinutritional factors present in foods limit the bioavailability of micronutrients (Zimmermann and Hurrell, 2007). These antinutrients tend to limit mineral delivery by interfering with intake, digestion, and absorption. Of particular concern are the relatively heat-stable phytates and tannins (Popova and Mihaylova, 2019).
Soybeans and maize can be subjected to technologies that could improve their nutritional value and acceptability. Nixtamalization is a traditional maize preparation process in which dried maize is cooked and steeped in an alkaline solution, usually water and food-grade lime (calcium hydroxide) (Odukoya et al., 2021). This process is used to prepare some maize-based products like tortillas, masa, and snacks. This technique can cause several physicochemical modifications to maize kernels, contribute to flavor, and reduce mycotoxins in the final product (Odukoya et al., 2021).
Even though hydrothermal deactivation of lipoxygenase is used in the soymilk industry, there is no report on evaluating the hydrothermal treatment of soybean in the preparation of odorless products for the enrichment of protein-deficient maize slurry treated by nixtamalization techniques to reduce mycotoxin incidence and antinutrient factors. Therefore, the objective of this study was to evaluate the nutrient, antinutrient, mineral molar ratios, and mycotoxin contents of cereal-legume-based composite flours that undergo nixtamalization and heat treatment.
The soybeans (variety SB24) and maize (Bambou) were obtained at INERA (Institut National d’Etude et de Recherche Agronomique/Mulungu station). Fifteen kilograms of the maize sample were packed in a one-kilogram polyethylene plastic bag (biohazard peligroso) and labeled with the following information: day and month, source, and district. For soybeans, only five kilograms were bought from INERA. The samples were transported and kept at room temperature prior to processing. Before processing, the grains were first sorted to remove impurities. A total of eight experimental flours were prepared from maize and maize-soybean mixtures, following a 3×2 factorial design involving two factors, namely soybean type (roasted, torrefied, and hydrothermal soybean) and maize flour type (whole maize and nixtamalized maize), with two controls as summarized in Figure 1.
Figure 1. Diagram of the preparation of composite flours of flour preparation. W.M.: Whole maize. NixM: Nixtamalized Maize. R.S.: Roasted Soybean. H.S.: Hydrothermal soybean T.S.: Torrefied Soybean. CF1: (Composite flour 25%RS + 75%WM), CF2: (Composite flour 2: 25%HS + 75%WM). CF3: (Composite flour 3: 25%TS + 75%WM),CF4:(Composite flour 25%RS +75% NixM) CF5:(Composite flour 25%HS + 75% NixM), CF6: (Composite flour 25%TS + 75% NixM).
After sorting, soybeans were heated to remove the bean and grass taste, as follow:
1. For hydrothermal treatment, soybeans were washed, then the clean soybeans were gradually introduced into boiling water and held for 1 h (one part soybean to three parts water). After cooling the soybean in water, the hulls were removed manually by rubbing the seeds between the palms of the hands. The shelled soybeans were then washed and let dry.
2. For roasted soybean: Sorted soybean was maintained at 100°C for 15 min in an oven according to the methods described by WFP (WFP, 2004).
3. For torrefied or toasted soybeans: Sorted soybeans were maintained at 180°C in a roaster for 10 min.
Nixtamalized maize: 400 g of maize kernels were boiled or cooked in 2.000 mL of a 1% calcium hydroxide solution for 40 min at 92°C. After steeping the cooked maize for 16 h at room temperature, the cooking liquor was collected, and the nixtamal was rinsed. Wet nixtamal was dried for 24 h at 65°C using an air oven, then mixed with different soybean types and ground to a fine flour. In order to ensure homogeneity of the maize meal mixtures, the dried and heat-treated soybeans were carefully mixed with the maize portion (whole maize or nixtamalized maize) before milling. Figure 1 shows a diagram of the preparation of different composite flours. From the (NixM) and non-nixtamalized maize (W.M.) and different types of soybean (Roasted, hydrothermal, and torrefied). We formulated six different types of composite flour (CF). The composite flour 1 was a mixture of 25% roasted soybean and 75% whole maize; the composite flour 2 was a combination of 25% hydrothermal soybean and 75% whole maize; and the composite flour 3 was a mixture of 25% roasted soybean and 75% whole maize. The composite flour 4 was a mixture of 25% roasted soybean and 75% nixtamalized maize; the composite flour 5 was a mixture of 25% hydrothermal soybean and 75% nixtamalized maize; and finally, the composite flour 6 was a mixture of 25% torrefied soybean and 75% nixtamalized maize.
The moisture content was determined using the method [Association of Official Analytical Chemists; AOAC 952.08, (AOAC, 2016)]. About 2 grams of each sample were kept overnight in an air oven (model UF55, Memmert Oven, Germany) at 105°C for 16 h and weighed. The loss in weight was regarded as a measure of moisture content. The crude protein was determined by the micro Kjeldahl method (AOAC 992.23, 2016) and the total protein was calculated by conversion from the total nitrogen. A 6.25 value was used as the conversion factor.
For ash content, the method AOAC 930.30, (AOAC, 2016) was used. A clean, dry crucible was weighed, and about 2 grams of sample were weighed into it. The crucibles were placed on a hot plate under a fume hood, and the temperature increased slowly until smoking stopped and the samples were thoroughly charred. They were then put in a muffle furnace, where the temperature increased gradually to 250°C and was heated for an hour. The temperature was increased to 550°C and incinerated for about 5 h in a VULCAN™ furnace (model 3–1750, Cole- Parmer, Illinoi, United States). The temperature was then decreased to 300°C, and the crucibles were removed and cooled to room temperature.
The fat content was determined by the AOAC 948.15, 2016 method; approximately two grams of moisture-free samples were placed in a Soxhlet cartridge and covered tightly with oil-free cotton. After extraction using petroleum ether at 60–80°C in a Soxhlet apparatus (model FOSS Soxtec™ extraction, Sweden) for about 6–8 h, The extract was evaporated in a pre-weighed beaker. The increase in weight of the beaker gave the crude fat content of the samples, and the petroleum ether was collected in a separate balloon. The crude fiber content was determined using fiber extraction equipment (model FOSS Fibertec™ 2010). The percentage of carbohydrate was calculated using the formula: 100 – (percentage of ash + percentage of moisture + percentage of fat + percentage of protein). The pH was determined using a digital pH meter (Nahita Model 903, Auxilab S.L., Beriáin, Spain) equipped with a glass electrode (XS Sensor Food S7, XS Instruments, Carpi, Italy). Energy was calculated using the Atwater’s calorie conversion factors: 4 kcal/g for crude protein, 9 kcal/g for crude fat and 4 kcal/g for available carbohydrate and adding up the values. Each sample was measured in triplicate.
Mineral analysis were determined according to AOAC 985.35, 2016 for calcium while for iron the AOAC 984.27, 2016 was used. Analyses were conducted using dry ashing and an atomic absorption spectrophotometer (AAS). The ash was transferred quantitatively to a 100-ml beaker using 20 mL of 1 N HCl, then heated at 80°C on a hot plate for 5 min. This was then transferred to a 100-ml volumetric flask and filled to the mark using 0.5 N HNO3. Insoluble matter was filtered, and the filtrate was kept in a labeled polyethylene bottle. Minerals were quantified using an atomic absorption spectrophotometer (AAS) (Shimadzu AA-7000 series, Japan). The AAS was equipped with an automatic atomizer changer, a graphite furnace atomizer, an auto-sampler, and a dual atomizer system. Mineral determinations were undertaken in triplicate for each composite flour. The various mineral standards were also prepared for the calibration curve.
The high-performance liquid chromatography (HPLC) method of phytic acid was used to analyze phytates (Ochanda et al., 2010). Approximately 0.5 g of sample was accurately weighed using an analytical balance and placed into a 100-ml Erlenmeyer flask. Samples were extracted with 10 mL of 3% H2S04 shaken and centrifuged at 1500 rpm at 25°C for 30 min. Contents were filtered using a filter paper of the Whatman series, and 50 mL of the filtrate was transferred into centrifuge tubes and placed in a boiling water bath for 5 min, followed by the addition of 3 mL of a FeCl3 solution containing 6 mg ferric iron per ml in 3% H2S04.
The contents were heated for 45 min to complete the precipitation of the ferric phytate complex. They were then centrifuged at 2500 rpm for 10 min, and the supernatant was discarded. The precipitate was washed with 30 mL of distilled water, centrifuged, and the supernatant discarded. 3 mL of 1.5 N NaOH was added to the residues, and the volume was brought to 30 mL with distilled water. The contents were heated for 30 min in a boiling water bath to precipitate the ferric hydroxide. Cooled samples were centrifuged, and the supernatant was transferred into a 50-ml volumetric flask. The precipitate was rinsed with 10 mL of distilled water, centrifuged, and the supernatant added to the contents of the volumetric flask. This was microfiltered (with the microfilter of 0.45 μm porosity) and kept awaiting HPLC analysis. HPLC analysis was done using a Shimadzu Refractive Index Detector (RID-10A). The standards were serial dilutions prepared from a stock solution of 10 mg/mL of sodium phytate (inositol hexaphosphoric acid C6H6 (OPO3Na2) 6H2O) in distilled water. The mobile phase had a flow rate of 1 mL/min at 30°C and contained 0.005 N sodium acetate. The column used was C18 ODS, 5 μM size, 250x46mm. The analysis was carried out in triplicate for each composite flour.
The vanillin-HCl method of Ochanda et al. (2010) served to estimate tannin quantification. The first extraction was performed from approximately 0.5 g of the composite flours for the extraction of tannins by using acidic methanol. Ten mL of 4% HCl in methanol was pipetted into each flask, shaken for 20 min, using a shaker (Labortechnik KS 250b, Germany), then separation done using a refrigerator cemtrifuge (Kokusan, Type H-2000C, Japan) at 4500 rpm and 25°C for 10 min. The supernatant was then transferred to 25-ml flasks. Then, the second extraction was done by adding 5 mL of 1% HCl in methanol to the residue of the first extraction. The supernatants from both extractions were combined and made up to 25 mL using methanol. The standards were serial dilutions prepared from a stock solution of catechin. 1 mL of the suitably diluted extract was taken in a test tube, and 5 mL of freshly prepared vanillin-HCl reagent was slowly added to the samples extracts and standards. The absorbance of the standards, CFs, and blanks was read in a UV–VIS spectrophotometer (Shimadzu, UV mini 1,240, Japan) at 500 nm. The quantification of tannins was expressed in mg of catechin equivalent per gram of sample. All measurements were carried out in triplicate. The measurement was recorded in an Excel sheet, and the content of tannin was calculated as percent catechin equivalent (CE) using the standard calibration curve.
To predict mineral bioavailability in vitro, the molar ratio between antinutrients and minerals has been used (Zhang et al., 2020). The study has explored four to two antinutrient-to-mineral molar ratio categories. The molar ratios of phytate and tannins to iron, calcium, zinc, and magnesium were calculated by dividing the weight of those chemicals by their atomic weight. The molar mass ratio between antinutrient and mineral was obtained by dividing the mole of the antinutrient by the mole of the mineral, as demonstrated in the equation below.
With the following values for the molar masses: phytic acid = 660.04 g/mol, tannins = 636.5 g/mol, iron = 55.845 g/mol, zinc = 65.38 g/mol, Mg =24.305 g/mol and Calcium =40.078 g/mole (Proietti et al., 2013).
Aflatoxins and fumonisins analyses were done at the IITA Kalambo laboratory. The samples were analyzed using Neogen Reveal® Q+ kits to determine mycotoxins. A 10 g subsample was weighed with a balance and transferred into a 100 mL beaker. Then 100 mL of 65% ethanol was added. The mixture was stirred for 3 min using an orbital shaker at 200 rpm. After that, the mixture rest for 3 min before filtering with Whatman No. 1 filter paper. Five hundred microliters of sample diluent for aflatoxin and 400 μL for fumonisin were transferred to a sample dish, and 100 μL of sample extract for aflatoxin and 200 μL for fumonisin were added. Finally, a 400 μL aliquot of sample extract and diluent was added to the Neogen Raptor cartridge. The reading was done on the Neogen Raptor® display screen after 6 min. Aflatoxin concentration was quantified in parts per billion (ppb; μg/kg), while fumonisin concentration was quantified in parts per million (ppm; mg/kg). The Reveal Q + assay for aflatoxin is a quantitative assay for total aflatoxins. The linear range of detection is 2–150 μg/Kg. The Reveal Q + assay for fumonisin is also a quantitative assay for the quantification of B1 plus B2. The linear range of detection for B1 plus B2 is 1–7 mg/kg. Maize-soybean composite flour samples were analyzed in triplicate.
The data was recorded using Microsoft Office Excel, and R software was used for the analysis. A two-way ANOVA was used to test the main effects and interactions among the independent variables (maize-soybean composite flour). Post-hoc mean separations were performed using Tukey’s honestly significant difference test at a significance level of 5%.
The proximate analysis of the composite flour is shown in Table 1 and Figure 2. Results show that heat treatment and nixtamalization processing influence the proximate composition of different flours. The moisture content did not vary significantly with heat treatment of soybean. Meanwhile, hydrothermal treatment of soybean led to a slightly increase of moisture. The total protein and carbohydrate contents ranged from 74.8 to 78.68% dry weight (dwt) and 12.19 to 13.57% dwt, respectively. The total carbohydrate content did vary significantly with the heat treatment of soybean with p < 0.04. The heat treatment also influences the protein content (p < 0.05), with the hydrothermal soybean flours generally exhibiting higher protein content than roasted and toasted. The heat inactivation of the trypsin inhibitors and the heat denaturation of soyabean globulins, makes them more susceptible to proteolysis, thereby improving soy protein bioavailability for human.
The CF’s moisture content was in the range of 3.8 to 5.6% on a dry weight basis; this is thought to be contributed by the moisture content of the ingredient flours. Among the proximate values, moisture content is an indicator of quality and an important factor that impacts the storage and safety of food (Gemede, 2020; Ibeabuchi et al., 2020). When the moisture is low, we assume an extended shelf life. Furthermore, the pH value varied with the nixtamalization process, from 6.82 to 9.32.
This increase is due to the presence and absorption of calcium ions (Ca2+). Protein content was slightly reduced after nixtamalization. Protein content in raw maize was 9.48% on a dry weight basis, and when nixtamalized, the protein was reduced to 9.01% on a dry weight basis. However, in the composite flour, the protein content increased due to the fortification of maize flour with soybean. CF2 and CF5 had the highest protein content. This indicates that in an environment where maize and maize products are often consumed and where protein deficiencies prevail, enrichment of maize by foods rich in protein is required. Additionally, the energy values obtained from CFs were in accordance with the recommendations. For carbohydrate content values in the range of (74.43–78.86%) and gross energy value (354.1–429.56 kcal/100 g), when we compare the codex requirement for baby’s flours, some of the CFs did not meet the requirement of 400 Kcal/100 g, suggesting that there is a need to fortify maize so that it can provide the required energy.
The lipid content ranged from 2.57 to 8.4%. The fiber value varies from 0.23 to 1.86%, with nixtamalized maize having the highest-fiber value. The main component of nixtamalized flour is carbohydrates. The difference between maize is owing to maize variety. Pigmented maize generally has a soft endosperm, whereas crystalline endosperm is present in white maize. The ash content also presented significant differences among treatments.
The mineral and antinutrient contents of CFs of maize-soybean based flours are shown in Table 2 and Figure 3. Generally, significant differences (p < 0.05) existed among the CFs. The mineral content increased with the use of technology in making the CFs. The calcium content obtained ranged between 1383.07 and 1949.64 mg/100 g. The highest value was found in the sample containing the nixtamalized maize and the roasted soybean, while the least value occurred in the sample with 100% untreated maize. The contents of magnesium were in the range of 100.66–142.92 mg/100 g and iron (3.32–9.63 mg/100 g). The highest value was found (p < 0.05) in CFs made of nixtamalized flour. Among composite flours, the lowest amount of magnesium (100.66 mg/100 g) was found in maize flour; whereas the highest content of iron was found in CF4, CF5, and CF6. The content of iron in CFs with nixtamalized maize led to a significant increase in iron content. CF5 contained the highest amount of zinc (8.78 mg/100 g).
Minerals are substances that are used in small amounts in the human body, but their deficiency can lead to the development of some disorders and diseases. A deficiency often happens slowly over time and can be caused by a number of reasons. An increased need for the mineral, a lack of the mineral in the diet, or difficulty absorbing the mineral from food are some of the more common reasons. Mineral deficiencies can lead to a variety of health problems, such as weak bones, fatigue, or a decreased immune system (Aysha et al., 2022).
The phytate content varied from 0.357 to 1.540 mg/g; the treated CF5 exhibited the lowest phytate content, which was 0.357 ± 0.012 mg/g, while whole maize and CF2 had the highest content, which was 1.5 mg/g (Table 2). The result showed that there is a great loss of phytic acid in nixtamalized maize and the CFs formulated by the treated maize. This reduction in phytic acid was comparable to that previously published by different authors, where we found losses of 17.4 to 35% when the maize was nixtamalized with 1.2% lime concentration (Bressani et al., 2004; Rong and Wang, 2009).
Phytic acid is an antinutrient that interacts with minerals and other substances like amino acids to form insoluble complexes that can chelate, decreasing the bioavailability of minerals and amino acids. It has been put forward that the fractional absorption of iron and zinc is much lower in high-phytate diets compared to low phytate diets (Gibson et al., 2010). Consumption of CFs with high phytate content, such as CF1 and CF3, identified in the current work, may be discouraged among populations with a high prevalence of micronutrient deficiencies (Popova and Mihaylova, 2019); although some findings have revealed phytic acid to have anti-carcinogenic and antioxidant properties as it can easily chelate some metal ions.
The tannin content in the CFs ranged from 0.0117 ± 0.001 to 0.066 ± 0.005 mg/g in maize flour (Table 2). These findings are in line with earlier studies in which the ranges of tannin contents reported were 9.4 mg/100 g when the maize was not nixtamalized and were reduced to 2.3 mg/100 g when it was fortified with 20% hydrothermally cooked soybean (Igbua et al., 2020).
In this study, because the concentrations of phytate in some CFs were high, we had to analyze their effect on the bioavailability of some minerals. The molar ratios predicting the bioavailability of calcium, iron, magnesium, and zinc are presented in Table 3.
The phytate to calcium (Phy: Ca), zinc (Phy: Zn), iron (Phy: Fe), and to magnesium (Phy: Mg) was evaluated. Also listed in Table 3 are tannins to iron (Tan: Fe), phytate plus tannins to iron (Phy + Tan: Fe), and phytate multiplied by calcium to zinc molar ratios (MRs) obtained for the different composite flours. The findings of this study indicated that the MRs varied within the different antinutrient to mineral categories. The Phy:Ca MRs ranged from 0.0012 ± 0.0011 in composite flour CF5 to 0.0067 ± 0.004 in whole maize. Interestingly, the Phy:Zn MRs ranged from 0.40 ± 0.34 in CF5, with the highest zinc content, to phytate of 2.7 ± 0.24 in CF2. The average values of Phy: Ca, Phy: Fe, and Phy:Zn MRs were in line with earlier reported values of 0.00049, 0.01, and 0.025, respectively, for composite flour made of maize and soybean (Igbua et al., 2020). It has been established that Phy:Ca, Phy:Fe molar ratios above 1, and Phy:Zn molar ratios above 15 negatively impact the bioavailability of the respective minerals (Castro-Alba et al., 2019). The Phy: Ca, Phy: Fe, and Phy: Zn MRs of the CFs in this study were below the critical limits, which means that the iron and zinc bioavailability was effective. It should be noted that it has been previously demonstrated that different processing techniques, such as nixtamalization and heat treatment, can increase mineral bioavailability. The Tan: Fe MRs ranged from 0.1 in composite flours 5 and 6 to 1.75 ± 0.24 in whole maize, whereas the Phy + Tan: Fe MRs ranged from 0.02 ± 0.003 to 0.56 ± 0.24. To the best of our knowledge, the negative influence of tannic acid, a hydrolysable tannin, on iron bioavailability has been explored, but very little is known on Tan: Fe MRs and Phy + Tan:Fe MRs are associated with condensed tannins, which are the most common tannins in foods (Delimont et al., 2017).
The aflatoxin level in the composite flour varied according to the type of composition in the mixture. Thus, the results show that the high aflatoxin level was obtained from whole maize flour (13.8 μg/kg) while fumonisin and aflatoxin were not detected in soybean. All composite flours in this study were contaminated with aflatoxins and fumonisin; and levels ranged from 1.36 to 13.8 μg/kg and 0.3 to 0.6 mg/kg, respectively (Table 4).
Although most of the samples met the proposed East Africa regulatory threshold of 10 μg/kg, whole maize, the composite flours 1, 2, and 3 did not meet the EU regulatory threshold for total aflatoxins of 4.0 μg/kg (EU, 2010). In general, aflatoxin contamination in agricultural commodities can be compounded by a wide range of pre- and post-harvest factors such as high temperatures and drought conditions, poor farm practices, a lack of drying facilities, and inappropriate storage practices (Udomkun et al., 2018; Matendo et al., 2022). The highest aflatoxin contamination in this study was found in all samples composed of untreated whole maize. This might be attributed to poor storage of maize as well as poor governmental regulations and legislation on aflatoxin contamination in the country.
In addition, we also observe a variation in fumonisin levels depending on the composition and type of composite flour analyzed. The high fumonisin values of 0.63 mg/kg were obtained for the whole maize. On the other hand, the nixtamalized flour found its aflatoxin and fumonisin content lowered.
There is a high prevalence of malnutrition in South Kivu Province, and a search for sustainable solutions by using available foods to improve the nutrient densities of staple diets in the region is crucial. The study reports on the effect of incorporating nixtamalized maize with different heat-treated soybeans on the nutritional and safety of the consumer. The results have shown that it is possible to enrich nixtamalized maize with a substantial proportion of heat-treated soybeans, thus enhancing the food’s nutritional status.
So, the results of this study suggest that roasting, toasting, and hydrothermal treatment of soybeans add other flavors and get rid of some anti-nutritional factors like phytates, tannins, and mycotoxin that can make some nutrients unavailable and cause diseases.
Moisture is used as an indicator of quality. Food with a low moisture content lasts longer and is less susceptible to microorganism attack. Even the production of mycotoxins requires high moisture content and temperature, as it allows the fungi to grow and produce the secondary metabolite (Gemede, 2020; Ibeabuchi et al., 2020; Valencia-Quintana et al., 2020). Although the composite flour’s moisture content was slightly higher (p < 0.05) than the codex standard’s, other studies recommend the specified limits (14% or less), which means that the moisture of the composite flour in this study was significantly lower (p < 0.05). With low moisture, we expect extended shelf stability (Osuji et al., 2019; Ibeabuchi et al., 2020). The ash content obtained from this study correlates with the findings reported by Gemede (2020) for pea, maize, and anchor flours. Ash is the mineral constituent in flour; a composite flour with a high ash content could meet the minimum requirements of limiting minerals in complementary foods made of locally available commodities. The value of fiber content in composite flours was close to the value of 0.50% reported by Osuji et al. (2019) for rice flour. The fiber value of the whole maize and nixtamalized maize was slightly low compared to the 2.51% reported by Gemede (2020) for maize flours. The nixtamalization process appears to be the main contributor to fiber content in maize, as the interaction between the ions (Ca2+ and OH−) present in the solution and the different constituents of the maize grains can produce some non-digestible products and thus increase the fiber content during the process. A higher amount of insoluble dietary fiber was found in nixtamalized flours (Liu et al., 2015). Fiber is known to cleanse the digestive tract (Emebu and Anyika, 2011) and facilitate food’s passage quickly through the gut, which helps prevent constipation (Igwe et al., 2015; Odimegwu et al., 2019). In vivo and in vitro studies have demonstrated the ability of insoluble dietary fiber to absorb carcinogens (Naumann et al., 2019).
Soybean flour is a good source of protein (Agbemafle et al., 2020). Previous reports indicate that protein is lost during the nixtamalization process, probably due to the solubilization of some protein fractions (Bello-Pérez et al., 2003). Enrichment of nixtamalized maize with soybeans indicates that incorporating legumes treated by appropriate processes improves the nutritional value of infant flours. Marcel et al. (2022) show that increasing the proportion of soybean, amaranthus, and pumpkin in complementary flours increases their protein content, depending on the treatment. Thus, their utilization and consumption after inactivating the antinutritional component may be used to mitigate protein-energy malnutrition in South Kivu. Importantly, the overall effect on protein quality depends on the ingredients and processing methods used. Some processing methods, like germination and extrusion, are known to improve protein quality and digestibility by lowering the amount of antinutrients in food (Ofoedu et al., 2019, 2020, 2021). The lipid content of different composites was low; these CFs can be enhanced to a higher level by adding a small quantity of fat or oil during the preparation of the porridge (Walker et al., 2003). In this study, the carbohydrate content was high due to starch’s appreciable degradation into simple sugars during processing. During steeping, the intermolecular hydrogen bond breaks, which causes the starch to lose its crystalline structure. This can cause the starch granule to partially gel (Pena-Ryes et al., 2016). However, this is advantageous to infants, as the sugars produced can help minimize the addition of table sugar during the preparation of the porridge. The energy of complementary foods determines the quantity of food needed to meet the energy needs of infants.
Minerals are used in small amounts but play a crucial role during metabolism. Among others, calcium is an essential nutrient that serves a critical role in bone structure, particularly in stages of growth such as infancy and childhood. Inadequate calcium intake during childhood may increase the risk of fractures and rickets and prevent the achievement of maximal peak bone mass later in life. Findings from Udomkun et al. (2019) show that the calcium content varies between 1580.3 and 1623.6 mg/100 g for a partial or full substitution of wheat flour with cassava flour in composite flours, respectively. The findings of this study show that we can easily improve the calcium content of maize by practicing nixtamalization. It’s therefore implying that there is no need to fortify with calcium the maize that undergoes the nixtamalization process. In most studies where the CFs are formulated, they encourage substituting the calcium deficiency with other calcium-rich foodstuffs, probably of animal origin or entomophagy (the utilization of insects as food). However, the challenge would be affordability due to the low-income status of most rural and peri-urban households; and the unreliable option due to the seasonal availability of the insects in diverse global geographical regions. So, the nixtamalization process of maize might be one of the most promising solutions for low-income households. Previous findings show that calcium content significantly increased after the nixtamalization with lime (Bressani et al., 2020).
In terms of zinc content, the results showed that all CFs were within the Dietary Reference Intakes (DRIs) range (3.4–8.78 mg/100 g) set by the World Food Program (2018). However, the zinc content in the CFs was much higher than in the control samples. The CFs developed in this study are providing a good source of zinc as 50% of the zinc DRI requirement is met,which can be considered sufficient (Codex Alimentarius Comission, 2006; Adisetu et al., 2017). Zinc deficiency is associated with stunting, anemia, and higher disease susceptibility (Agbemafle et al., 2020). The magnesium content of formulated diets is evidently due to the raw materials utilized in CFs. Previous studies emphasized that 10% soybeans, in addition to orange-fleshed sweet potatoes, increased the magnesium content, indicating that soybeans are a good source of the mineral. Magnesium is essential as it helps strengthen the bones and maintain the immune system (Ndife et al., 2020). The findings show a high reduction of phytate when we combine the nixtamalization and hydrothermal treatment of soybean; this is in line with the findings of Rong and Wang (2009), who show that processes like nixtamalization degrade phytic acid in crops. Phytic acid should be lower in baby porridge to minimize mineral losses.
The phytate/calcium molar ratios in all the samples were less than 0.24, which is regarded as favorable for calcium absorption; predicting that among all the CFs, the calcium bioavailability could be achieved by using the local available crops like maize and soybean to make a good CF. The finding shows that CFs have phytate/iron molar ratios ranging between 0.3 and 3.92; the critical value of the [phytate]:[iron] molar ratio of <1.0 is accepted as phytate begins to lose its inhibitory effect on iron absorption at this level. The molar ratio of phytate to zinc is within the range of 0.40–7.485; this is lower than the range of the suggested critical level < 15 regarded as favorable for zinc absorption. A significant increase in [phytate]: [zinc] molar ratios was observed in CF2 and WM samples when compared with other CFs, while CF5 showed the lowest values. Research has shown that high dietary calcium impairs zinc absorption in the presence of a high intake of phytate (Gemede et al., 2020). Thus, the molar ratio of [phytate][calcium]/[zinc] might be better used as an indicator of zinc bioavailability than the molar ratio of [phytate]:[zinc] alone. In this study, the molar ratios of [phytate], [calcium], and [zinc] ranged from 0.001–0.031 (which is <0.5).
These results are similar to what Agbetiameh et al. (2018) found, which shows that the high level of aflatoxin in untreated products would be caused by the growth of aflatoxigenic fungi in the seeds while they are being stored. Previous studies have shown that applying the nixtamalization process can reduce aflatoxin contamination by up to 70%. According to Price and Jorgensen (1985), cooking, extended steeping, and washing of nixtamal can help reduce the amount of aflatoxin in nixtamal, masa, and tortillas. When compared to the naturally infected, unprocessed maize (starting aflatoxin level of approximately 140 μg/kg), aflatoxin levels in tortillas were lowered by 50 to 70%.
The alkaline treatment led to the formation of at least two unidentified degradation or transformation products: one of 301.25 Da (molecular formula: C17H16O15) and another of 325.33 Da (molecular formula: C17H18O5) (Moreno-Pedraza et al., 2015). Lower mutagenicity and oxidative stress in vitro were observed in nixtamalized maize products, together with decreased aflatoxin contents (Price and Jorgensen, 1985; Moreno-Pedraza et al., 2015).
Other studies that have analyzed children’s foods in various African countries have found there is a significant risk to children’s health due to high levels of aflatoxins in foods (Kang’ethe et al., 2017). A study by Egal et al. (2005) on the dietary exposure to aflatoxin from maize and groundnut in under-five-year-old children from Benin and Togo concluded that maize was an important source of aflatoxin exposure among the children. This was explained by the fact that maize is consumed more frequently than other types of crops. Maize is the primary ingredient, even in food mixtures with additional ingredients. In South Kivu, the case is similar, where 98% of children consume foods made of grains, roots, and tubers, mostly maize, and 85% of infants consume foods made of legumes (Kambale et al., 2022).
In addition, the reduced levels of total fumonisins indicate that they are water-soluble and are easily washed away from the outer layer of the maize grains (Karlovsky et al., 2016). On the other hand, some findings have shown that fumonisins are soluble and prone to leaching from grains into steeping and cooking solutions (Schaarschmidt and Fauhl-Hassek, 2019). In experimental studies, De La Campa et al. (2004) further investigated the impact of lime concentration and cooking time at different initial FB1 levels using fungal-inoculated maize. In doing so, they found a positive impact of lime concentration (when testing lime solutions of around 0.25–1.6%) on FB1 reduction. Nixtamalization significantly reduced the amount of fumonisin in raw corn.
This work was done to figure out the nutrients, antinutrients, molar ratios of the antinutritional factor to minerals (mineral availability), and mycotoxin content of maize-soybean-based composite flours. Results show that the technologies used lead to CFs rich in some macro- and micronutrients and low in mycotoxin contamination. Also, the level of the antinutrients was reduced, and it was found to influence the bioavailability of minerals, especially zinc, iron, calcium, and magnesium. These CFs are recommended as a practical and sustainable approach for improving macro- and micronutrients and mineral availability for the resource-poor in low-income countries. The findings of this study will play an integral role in promoting the utilization of local crops and enhancing agricultural systems. Thus, we recommend using this process in our environment to obtain well-enriched and healthy infant flour.
However, as both the right amino acid composition and high digestibility and PDCAAS are required for proteins to meet the requirements of the human body, the ability of dietary proteins to meet these requirements varies widely. Such abilities are often quantitatively expressed in so-called protein quality metrics, which include amino acid composition and digestibility.
Thus, this could be done in further research, as such information would help optimize nutrient use and improve nutrient use efficiency. Finally, it is worth noting that all CFs have different levels of mycotoxin, with the treated CFs having their contamination reduced. Thus, these technologies can be promoted as strategies to manage mycotoxin contamination.
The raw data supporting the conclusions of this article will be made available by the authors, without undue reservation.
RM: conceptualization, data collection, formal analysis, and drafting paper. RM and PU: methodology. All authors review, editing of the manuscript, contributed to the article, and approved the submitted version.
The authors thank the Université Evangélique en Afrique (UEA), Jomo Kenyatta University of Agriculture and Technology (JKUAT), International Institute of Tropical Agriculture IITA/Kalambo and Mawazo Institute. Support for this research was made possible through a capacity building competitive grant Training the next generation of scientists provided by Carnegie Cooperation of New York through the Regional Universities Forum for Capacity Building in Agriculture (RUFORUM) (Grant ID: Grant# R.U./2020/GTA/DRG/017).
The authors declare that the research was conducted in the absence of any commercial or financial relationships that could be construed as a potential conflict of interest.
All claims expressed in this article are solely those of the authors and do not necessarily represent those of their affiliated organizations, or those of the publisher, the editors and the reviewers. Any product that may be evaluated in this article, or claim that may be made by its manufacturer, is not guaranteed or endorsed by the publisher.
Adisetu, P. R., Akonor, P. T., and Bonsi, E. (2017). Orange-fleshed sweet potato based complementary food provides sufficient vitamin A for infants aged6-12 months. Afr. J. Food Sci. 11, 215–222. doi: 10.5897/AJFS2016.1557
Agbemafle, I., Hadzi, D., Amagloh, F. K., Zotor, F. B., and Reddy, M. B. (2020). Nutritional, microbial, and sensory evaluation of complementary foods made from blends of orange-fleshed sweet potato and edible insects. Foods 9, 1225–1237. doi: 10.3390/foods9091225
Agbetiameh, D., Ortega-Beltran, A., Awuah, R. T., Atehnkeng, J., Cotty, P. J., and Bandyopadhyay, R. (2018). Prevalence of aflatoxin contamination in maize and groundnut in Ghana: population structure, distribution, and toxigenicity of the causal agents. Plant Dis. 102, 764–772. doi: 10.1094/PDIS-05-17-0749-RE
AOAC International (2016). Official methods of analysis of AOAC international. (20th Edn.). Gaithersburg, MD, USA: AOAC International. 3172.
Arimond, M., Daelmans, B., and Dewey, K. G. (2008). Indicators for feeding practices in children. Lancet 371, 541–542. doi: 10.1016/S0140-6736(08)60250-0
Ayo, E. M., Matemu, A., and Kimanya, M. E. (2018). Socioeconomic characteristics influencing level of awareness of aflatoxin contamination of feeds among livestock farmers in Meru District of Tanzania. Scientifica 2018, 1–11. doi: 10.1155/2018/3485967
Aysha, K. K., Krist, J. D., Kevin, D., Barbara, A., Valeria, V., Giuseppina, M., et al. (2022). Main nutritional deficiencies. J. Prev. Med. Hyg. 63, E93–E101. doi: 10.15167/2421-4248/jpmh2022.63.2S3.2752
Bello-Pérez, L. A., Osorio-Díaz, P., Agama Acevedo, E., Solorza-Feria, J., Toro-Vázquez, J. F., and Paredes-López, O. (2003). Chemical and physicochemical properties of dried wet masa and dry masa flour. J. Sci. Food and Agric. 83, 408–412. doi: 10.1002/jsfa.1381
Bressani, A. P. P., Martinez, S. J., Sarmento, A. B. I., Borém, F. M., and Schwan, R. F. (2020). Organic acids produced during fermentation and sensory perception in specialty coffee using yeast starter culture. Food Res. Int. 128:108773. doi: 10.1016/j.foodres.2019.108773
Bressani, R., Turcios, J. C., Colmenares de Ruiz, A. S., and De Palomo, P. P. (2004). Effect of processing conditions on phytic acid, calcium, iron and zinc content of lime-cooked maize. J. Agric. Food Chem. 52, 1157, 1157–1162. doi: 10.1021/jf030636k
Campbell, B. M., Vermeulen, S. J., Aggarwal, P. K., Corner-Dollo, C., Girvetz, E., and Loboguerrero, A. M. (2016). Reducing risks to food security from climate change. Glob. Food Security. 11, 34–43. doi: 10.1016/j.gfs.2016.06.002
Castro-Alba, V., Lazarte, C. E., Bergenståhl, B., and Granfeldt, Y. (2019). Phytate, iron, zinc, and calcium content of common Bolivian foods and their estimated mineral bioavailability. Food Sci. Nutr. 7, 2854–2865. doi: 10.1002/fsn3.v7.910.1002/fsn3.1127
Codex Alimentarius Comission (2006). Codex standard for processed cereal-based foods for infants and young children (CODEX STAN 074–1981. REV. 1–2006), 1–9.
CTA. (2015). Building the evidence base on the agricultural nutrition nexus: Rapid country scans for informing policy and practice: Democratic Republic of Congo. Université Evangélique en Afrique, Apia.
De Groote, H., and Kimenju, S. C. (2012). Consumer preferences for maize products in urban Kenya. Food Nutr. Bull. 33, 99–110. doi: 10.1177/156482651203300203
De La Campa, R., Miller, J. D., and Hendricks, K. (2004). Fumonisin in tortillas produced in small-scale facilities and effect of traditional masa production methods on this mycotoxin. J. Agric. Food Chem. 52, 4432–4437. doi: 10.1021/jf035160j
Delimont, N. M., Haub, M. D., and Lindshield, B. L. (2017). The impact of tannin consumption on iron bioavailability and status: a narrative review. Curr. Dev.Nutr. 1, 1–12. doi: 10.3945/cdn.116.000042.1
Erokhin, V., Diao, L., Gao, T., Andrei, J. V., Ivolga, A., and Zong, Y. (2021). The supply of calories, proteins, and fats in low-income countries: a four-decade retrospective study. Int. J. Environ. Res. Public Health 18:7356. doi: 10.3390/ijerph18147356
Emebu, P. K., and Anyika, J. U. (2011). Proximate and mineral composition of kale (Brassica oleracea) grown in Delta state. Nigeria. Pak. J. Nutr. 10, 190–194. doi: 10.3923/pjn.2011.190.194
EU (2010). Commission regulation (EU) no. 165/2010 of 26 February 2010, amending regulation (EC) no. 1881/2006 setting maximum levels for certain contaminants in foodstuffs as regards aflatoxin. Off. J. Eur. Union 50, 8–12.
FAO, IFAD, UNICEF, WFP and WHO (2022). The state of food security and nutrition in the world 2022. Repurposing food and agricultural policies to make healthy diets more affordable. Rome, FAO.
Egal, S., Hounsa, A., Gong, Y. Y., Turner, P. C., Wild, C. P., Hall, A. J., et al. (2005). Dietary exposure to aflatoxin from maize and groundnut in young children from Benin and Togo, West Africa. Int. J. Food Microbiol. 104, 215–224. doi: 10.1016/j.ijfoodmicro.2005.03.004
Flax, V. L., Phuka, J., Cheung, Y. B., Ashorn, U., Maleta, K., and Ashorn, P. (2010). Feeding patterns and behaviors during home supplementation of underweight Malawian children with lipid-based nutrient supplements or corn-soy blend. Appetite 54, 504–511. doi: 10.1016/j.appet.2010.02.003
Gemede, H. F. (2020). Nutritional and antinutritional evaluation of complementary foods formulated from maize, pea, and anchote flours. Food Sci. Nutr. 8, 2156–2164. doi: 10.1002/fsn3.1516
Gibson, R. S., Bailey, K. B., Gibbs, M., and Ferguson, E. L. (2010). A review of phytate, iron, zinc and calcium concentrations in plant-based complementary foods used in low-income countries and implications for bioavailability. Food Nutr. Bull. 31, S134–S146. doi: 10.1177/15648265100312S206
Ibeabuchi, J. C., Bede, N. E., Kabuo, N. O., Uzoukwu, A. E., Eluchie, C. N., and Ofoedu, C. E. (2020). Proximate composition, functional properties and oil characterization of "Kpaakpa" (Hildergardia barteri) seed. Res. J. Food Sci. Nutr. 5, 16–29. doi: 10.31248/RJFSN2019.079
Igbua, F. Z., Adejo, S. O., Igoli, N. P., and Daagema, A. A. (2020). Antinutrients and bioavailability of nutrients in maize, cassava and soybeans composite flour. Asian Food Sci. J. 16, 5–12. doi: 10.9734/afsj/2020/v16i230167
Igwe, K., Ofoedu, C. E., Okafor, D. C., Odimegwu, E. N., Agunwah, I. M., and Igwe, V. S. (2015). Comparative proximate analysis of some green leafy vegetables from selected communities of rivers and Imo state Nigeria. Int. J. Basic Appl. Sci. 4, 55–61.
Kalumbi, M., Limbikani, M., Mtimuni, B., Mwangwela, A., and Aggrey, P. G. (2019). Hydrothermally treated soybeans can enrich maize stiff porridge (Africa’s Main staple) without negating sensory acceptability. Foods 8:650. doi: 10.3390/foods8120650
Kang’ethe, E. K., Gatwiri, M., Sirma, A. J., Ouko, E. O., Mburugu-Musoti, C. K., Kitala, P. M., et al. (2017). Exposure of Kenyan population to aflatoxin in foods with reference to Nandi and Makueni counties. Food Qual Safety 1, 131–137. doi: 10.1093/fqsafe/fyx011
Karlovsky, P., Suman, M., Berthiller, F., De Meester, J., Eisenbrand, G., Perrin, I., et al. (2016). Impact of food processing and detoxification treatments on mycotoxin contamination. Mycotoxin Res. 32, 179–205. doi: 10.1007/s12550-016-0257-7
Kambale, M. R., Amani, G. N., Joe, B. K., Sarah, N., Boss, R. C., Armand, B., et al. (2022). Infant and young child feeding practices and nutritional status in two health zones of south Kivu, eastern Democratic Republic of Congo: a community-based study. J. Pediatr. Perinatol. Child. Health. 6, 350–360. doi: 10.26502/jppch.74050118
Khumalo, T. P., Schönfeldt, H. C., and Vermeulen, H. (2011). Consumer acceptability and perceptions of maize meal in Giyani. South Africa. Dev. S. Afr. 28, 271–281. doi: 10.1080/0376835X.2011.570074
Kuamba, P., (2016). Rapports de la recherche sur les aliments locaux à pouvoir nutritionnel dans la réhabilitation des enfants malnutris dans la province du Kasaï central. "2015–2016".
Liu, Y., Chang, C. C. H., Marsh, G. M., and Wu, F. (2015). Population attributable risk of aflatoxin-related liver cancer: systematic review and meta-analysis. Eur. J. Cancer 48, 2125–2136. doi: 10.1016/j.ejca.2012.02.009
Mangani, C., Maleta, K., Phuka, J., Cheung, Y. B., Thakwalakwa, C., Dewey, K., et al. (2015). Effect of complementary feeding with lipid-based nutrient supplements and corn-soy blend on the incidence of stunting and linear growth among 6- to 18-month-old infants and children in rural Malawi. Matern. Child Nutr. 11, 132–143. doi: 10.1111/mcn.12068
Marcel, M. R., Chacha, J. S., and Ofoedu, C. E. (2022). Nutritional evaluation of complementary porridge formulated from orange-fleshed sweet potato, amaranth grain, pumpkin feed, and soybean flours. Food Sci. Nutr. 10, 536–553. doi: 10.1002/fsn3.2675
Matendo, R. E., Imathiu, S., Udomkun, P., Mugumaarhahama, Y., Akullo, J., Ishara, J., et al. (2022). Aflatoxin and fumonisin mycotoxins contamination along the maize value chain in the eastern democratic republic of Congo. Afr. J. Food Agric. Nutr. Dev. 22, 19801–19821. doi: 10.18697/ajfand.108.21695
Moreno-Pedraza, A., Valdés-Santiago, L., Hernández-Valadez, L. J., Rodríguez-Sixtos Higuera, A., Winkler, R., and Guzmán-de Peña, D. L. (2015). Reduction of aflatoxin B1 during tortilla production and identification of degradation by-products by direct-injection electrospray mass spectrometry. Salud Publica Mex. 57, 50–56. doi: 10.21149/spm.v57i1.7402
Naumann, S., Schweiggert-Weisz, U., Eglmeier, J., Haller, D., and Eisner, P. (2019). In vitro interactions of dietary fibre enriched food ingredients with primary and secondary bile acids. Nutrients 11:1424. doi: 10.3390/nu11061424
Ndife, J., Abasiekong, K. S., Nweke, B., Linus-Chibuezeh, A., and Ezeocha, V. C. (2020). Production and comparative quality evaluation of Chin-Chin snacks from maize, soybean and orange fleshed sweet potato flour blends. FUDMA J. Sci. 4, 300–307. doi: 10.33003/fjs2020-0402-220
Niyibituronsa, M., Onyango, N., Gaidashova, S., Imathiu, S. M., Uwizerwa, M., Wanjuki, I., et al. (2018). Evaluation of mycotoxin content in soybean (Glycine max L.) grown in Rwanda. Afr. J. Food Agric. Nutr. Dev. 18, 13808–13824. doi: 10.18697/ajfand.83.17710
Ochanda, S. O., Onyango, C. A., Mwasaru, A. M., Ochieng, J. K., and Mathooko, F. M. (2010). Effects of malting and fermentation treatments on group B-vitamins of red sorghum, white sorghum and pearl millets in Kenya. J. Appl. Biosci. 34, 2128–2134.
Odukoya, J. O., De Saeger, S., De Boevre, M., Adegoke, G. O., Audenaert, K., Croubels, S., et al. (2021). Effect of selected cooking ingredients for nixtamalization on the reduction of fusarium mycotoxins in maize and sorghum. Toxins 13, 1–16. doi: 10.3390/toxins13010027
Odimegwu, E. N., Ubbaonu, C. N., Ofoedu, C. E., Akajiaku, L. O., Njoku, N. E., Agunwah, I. M., et al. (2019). Comparative study on the proximate composition, functional and sensory properties of turmeric (Curcuma longa) and pawpaw (Carica papaya) custard products. Curr. J. Appl. Sci. Technol. 33, 1–11. doi: 10.9734/CJAST/2019/v33i430090
Ofoedu, C. E., Akosim, C. Q., Iwouno, J. O., Obi, C. D., Shortskii, I., and Okpala, C. O. R. (2021). Characteristic changes in malt, wort, and beer produced from different Nigerian rice varieties as influenced by varying malting conditions. Peer J. 9:e10968. doi: 10.7717/peerj.10968
Ofoedu, C. E., Osuji, C. M., and Ojukwu, M. (2019). Sugar profile of syrups from malted and unmalted rice of different varieties. J. Food Sci. 8, 52–59. doi: 10.5539/jfr.v8nlp52
Ofoedu, C. E., Osuji, C. M., Omeire, G. C., Ojukwu, M., Okpala, C. O. R., and Korzeniowska, M. (2020). Functional properties of syrup from malted and unmalted rice of different varieties: a comparative study. J. Food Sci. 85, 3081–3093. doi: 10.1111/1750-3841.15446
Osuji, C. M., Ofoedu, C. E., and Ojukwu, M. (2019). Proximate malting characteristics and grain quality properties of some Nigerian rice of different varieties. Res. J. Chem. Sci. 9, 1–10.
Pena-Ryes, R. N., Ram, R., Fernandez-Perrino, F. J., and Cruz-Guerrero, A. E. (2016). Effect of nixtamalization processing temperature on maize hydration and the texturalproperties of masa and tortillas. J. Food. Pro. Pre. 41. doi: 10.1111/jfpp.13136
Proietti, I., Mantovani, A., Mouquet-rivier, C., and Guyot, J. (2013). Original article modulation of chelating factors, trace minerals and their estimated bioavailability in Italian and African sorghum [Sorghum bicolor (L.) Moench] porridges, 1526–1532. doi: 10.1111/ijfs.12121
Pillay, K., Derera, J., Siwela, M., and Veldman, F. J. (2011). Consumer acceptance of yellow. Provitamin A-biofortified maize in KwaZulu-Natal. S. Afr. J. Clin. Nutr. 24, 186–191. doi: 10.1080/16070658.2011.11734386
Price, R. L., and Jorgensen, K. V. (1985). Effects of processing on aflatoxin levels and on mutagenic potential of tortillas made from naturally contaminated corn. J. Food Sci. 50, 347–349. doi: 10.1111/j.1365-2621.1985.tb13398.x
Popova, A., and Mihaylova, D. (2019). Antinutrients in plant-based foods: a review. Open Biotechnol. J. 13, 68–76. doi: 10.2174/1874070701913010068
Valencia-Quintana, R., Milić, M., Jakšić, D., Šegvić Klarić, M., Tenorio-Arvide, M. G., Pérez-Flores, G. A., et al. (2020). Environment changes, aflatoxins, and health issues, a review. Int. J. Environ. Res. Public Health 17, 1–10. doi: 10.3390/ijerph17217850
Rong, L. I., and Wang, K. N. (2009). Nixtamalization effects on the contents of phytic acid in the varieties of maize and the bioavailability of iron in nixtamalized maize to young pigs. Pak. J. Nutr. 8, 905–909. doi: 10.3923/pjn.2009.905.909
Schaarschmidt, S., and Fauhl-Hassek, C. (2019). Mycotoxins during the processes of nixtamalization and tortilla production. Toxins 11:227. doi: 10.3390/toxins11040227
Smale, M., Byerlee, D., and Jayne, T. (2013). Maize revolutions in sub-Saharan Africa. Afr. Gr. Rev., 165–195. doi: 10.1007/978-94-007-5760-8_8
Stevens, R., and Winter-Nelson, A. (2008). Consumer acceptance of Provitamin A-biofortified maize in Maputo. Mozambique. Food Policy. 33, 341–351. doi: 10.1016/j.foodpol.2007.12.003
Singh, N., Jain, P., Ujinwal, M., and Langyan, S. (2022). Escalate protein plates from legumes for sustainable human nutrition. Front. Nutr. 9:977986. doi: 10.3389/fnut.2022.977986
UNICEF (2020). WHO: World Bank. Levels and trends in child malnutrition: key findings of the 2020 edition of the joint child. Malnutrition Estimates, vol. 24 WHO, 2–10.
Udomkun, P., Tirawattanawanich, C., Ilukor, J., Sridonpai, P., Njukwe, E., Nimbona, P., et al. (2019). Promoting the use of locally produced crops in making cereal-legume-based composite flours: an assessment of nutrient, antinutrient, mineral molar ratios, and aflatoxin content. Food Chem. 286, 651–658. doi: 10.1016/j.foodchem.2019.02.055
Udomkun, P., Wossen, T., Nabahungu, N. L., Mutegi, C., Vanlauwe, B., and Bandyopadhyay, R. (2018). Incidence and farmers' knowledge of aflatoxin contamination and control in eastern Democratic Republic of Congo. Food Sci. Nutr. 6, 1607–1620. doi: 10.1002/fsn3.735
Walker, W. A., Watkins, J. B., and Duggan, C. (2003). Nutrition in pediatrics: basic science and clinical applications. (3rd Edn.). London: BC Decker Inc.; 1121
Wibke, S. U., Laurice, P., Julianne, C., Van de Velde, F., and Peter, M. T. (2017). Flavor aspects of pulse ingredients. Cereal Chem. 94, 58–65. doi: 10.1094/CCHEM-06-16-0161-FI
WFP (2004). Fortified blended food: good manufacturing practice and HACCP principles. A handbook for processors in partnership with the U.N. World Food Programme.
Williams, J. H., Grubb, A. J., Davis, J. W., Wang, V. S., Jolly, P. E., Ankrah, N. A., et al. (2010). HIV and hepatocellular and esophageal carcinomas related to consumption of mycotoxin-prone foods in sab-Saharan Africa. Am. J. Clin. Nutr. 92, 154–160. doi: 10.3945/ajcn.2009.28761
Williams, J. H., Phillips, T. D., Jolly, P. E., Stiles, J. K., Jolly, C. M., and Aggarwal, D. (2004). Human aflatoxicosis in developing countries: a review of toxicology exposure, potential health consequences and interventions. Am. J. Clin. Nutr. 80, 1106–1122. doi: 10.1093/ajcn/80.5.1106
Zain, M. E. (2011). Impact of mycotoxins on humans and animals. J. Saudi Chem. Soc. 15, 129–144. doi: 10.1016/j.jscs.2010.06.006
Zimmermann, M. B., and Hurrell, R. F. (2007). Nutritional iron deficiency. Lancet 370, 511–520. doi: 10.1016/S0140-6736(07)61235-5
Keywords: nixtamalization, heat treatment, mineral availability, antinutrients, composite flour
Citation: Matendo RE, Imathiu S, Udomkun P and Owino WO (2023) Effect of nixtamalization of maize and heat treatment of soybean on the nutrient, antinutrient, and mycotoxin levels of maize-soybean-based composite flour. Front. Sustain. Food Syst. 7:1057123. doi: 10.3389/fsufs.2023.1057123
Received: 29 September 2022; Accepted: 28 August 2023;
Published: 02 October 2023.
Edited by:
Bassirou Bonfoh, Swiss Centre for Scientific Research (Côte d'Ivoire), Côte d'IvoireReviewed by:
Luis Abrunhosa, University of Minho, PortugalCopyright © 2023 Matendo, Imathiu, Udomkun and Owino. This is an open-access article distributed under the terms of the Creative Commons Attribution License (CC BY). The use, distribution or reproduction in other forums is permitted, provided the original author(s) and the copyright owner(s) are credited and that the original publication in this journal is cited, in accordance with accepted academic practice. No use, distribution or reproduction is permitted which does not comply with these terms.
*Correspondence: Rehema Esther Matendo, dmVjb3JkY29uZ29lc3RoZXJAZ21haWwuY29t
†ORCID: Rehema Esther Matendo orcid.org/0000-0002-8492-7166
Disclaimer: All claims expressed in this article are solely those of the authors and do not necessarily represent those of their affiliated organizations, or those of the publisher, the editors and the reviewers. Any product that may be evaluated in this article or claim that may be made by its manufacturer is not guaranteed or endorsed by the publisher.
Research integrity at Frontiers
Learn more about the work of our research integrity team to safeguard the quality of each article we publish.