- 1Department of Plant Sciences, University of California, Davis, Davis, CA, United States
- 2Department of Agronomy, Iowa State University, Ames, IA, United States
- 3Department of Industrial and Manufacturing Systems Engineering, Iowa State University, Ames, IA, United States
Introduction: In Midwestern maize (Zea-mays L.)-based systems, planting an over-wintering cover crop such as rye (Secale cereale L.) following fall harvests of summer crops maintains continuous soil cover, offering numerous environmental advantages. However, while adoption of cover crops has increased over the past decade, on a landscape-scale it remains low. Identifying where agronomic research could be most impactful in increasing adoption is therefore a useful exercise. Decision analysis (DA) is a tool for clarifying decision trade-offs, quantifying risk, and identifying optimal decisions. Several fields regularly utilize DA frameworks including the military, industrial engineering, business strategy, and economics, but it is not yet widely applied in agriculture.
Methods: Here we apply DA to a maize-soybean [Glycine max (L.) Merr.] rotation using publicly available weather, management, and economic data from central Iowa.
Results: In this region, planting a cover crop following maize (preceding soybean) poses less risk to the producer compared to planting following soybean, meaning it may be a more palatable entry point for producers. Furthermore, the risk of reduced maize yields when planting less than 14 days following rye termination substantially contributes to the overall risk cover crops pose to producers, but also has significant potential to be addressed through agronomic research.
Discussion: In addition to identifying research priorities, DA provided clarity to a complex problem, was performed using publicly available data, and by incorporating risk it better estimated true costs to the producer compared to using input costs alone. We believe DA is a valuable and underutilized tool in agronomy and could aid in increasing adoption of cover crops in the Midwest.
1. Introduction
Many cropping systems in the United States (US) have undergone simplifications, now being composed of only a few, often annual, crops (Aguilar et al., 2015; Hijmans et al., 2016; Crossley et al., 2021). These systems frequently leave the soil fallow for some period of time, presenting notable environmental challenges including but not limited to increased risk of soil erosion and an increased potential for nutrient loss (Mitsch et al., 2001; Hatfield et al., 2009; Syswerda et al., 2012). The notion of “continuous living cover” has been used to encourage creative solutions to these issues by focusing cropping system re-design on eliminating these environmentally-challenging fallow periods. Planting cover crops to reduce fallow periods is one such tactic that could at least partially address many of the environmental problems presented by annual cropping systems.
The US produces approximately one-third of the word's maize (Zea mays L.) and soybean [Glycine max (L.) Merr.] [Food and Agriculture Organization (FAO), 2020], with five states in the Midwestern region contributing over half of that production [Feyereisen et al., 2006]. It follows that large amounts of agricultural land in the Midwestern US are dedicated to cropping systems that grow only maize and soybean [Boryan et al., 2011; USDA National Agricultural Statistics Service Cropland Data Layer (USDA NASS CDL), 2021]. Utilizing over-wintering cover crops in these systems has been shown to reduce soil erosion and nitrate leaching (Kaspar et al., 2007, 2012; Chen et al., 2022), is associated with a reduction in crop insurance losses due to drought, excess heat, and excess moisture (Aglasan and Rejesus, 2021), and possibly offer numerous other context-specific benefits such as increased soil infiltration rates, higher soil water-holding capacity, or increased soil organic matter content (Moore et al., 2014; Basche and DeLonge, 2017; Krupek et al., 2022; Nichols et al., 2022). However, the Midwestern maize-soybean systems present challenges to cover crop adoption. In some regions of the US, cover crop adoption on annual cropland is above 25% and growing (Hamilton et al., 2017). Meanwhile, states comprising the Midwestern US exhibit some of the lowest adoption rates, with most states well below 10% adoption (Hamilton et al., 2017; Rundquist and Carlson, 2017; Seifert et al., 2018).
Low adoption rates within the Midwest have been the subject of numerous studies, and it is clearly a complex issue involving economics, climate constraints, field operations, management, equipment, culture, and technical knowledge (Lee et al., 2018; Church et al., 2020; Nichols et al., 2020a; Thompson et al., 2021; Yoder et al., 2021; Park et al., 2022). One barrier we believe merits more attention is that of risk. Risk incorporates two components, uncertainty and negative consequences, and is frequently measured with probabilities describing the potential severity of consequences (Kaplan and Garrick, 1981; Bedford and Cooke, 2001; Hubbard, 2020). Cover crops present both direct, and indirect risks. Managerially, maize and soybean are both are planted in the late spring (April, May) and harvested in the fall (September, October, November). Producers typically fit over-wintering cover crops into these systems by planting a cover crop in the fall after the cash crop harvest, and terminating the cover crop in the spring before the next cash crop is planted [Sustainable Agriculture Research and Education (SARE), 2020]. Therefore, both the planting and termination of an over-wintering cover crop such as rye (Secale cereale L.) can conflict with cash crop management. As such, using a cover crop requires complex decision-making that balances risk and rewards in uncertain conditions. While perceived risks associated with cover cropping are often cited as barriers to adoption (Arbuckle and Roesch-McNally, 2015), quantifying those risks in economic terms is challenging (e.g., Bergtold et al., 2019; Plastina et al., 2020). Furthermore, while lists of cover crop research priorities have been proposed (e.g., Carlson and Stockwell, 2013; Basche and Roesch-McNally, 2017), a tool for ranking priorities would be useful. By quantifying the risk associated with each decision point for producers, research priorities can be set to address points posing the highest risk. The use of risk as a ranking tool would also help researchers and funding organizations assess how resources can be used most impactfully. Furthermore, understanding how uncertainties around weather conditions elevate risks of profit loss is important for understanding both the mechanisms for delivering incentives, and the amount producers may require for meaningful participation.
Decision analysis is an interdisciplinary tool that can be applied to analyze decision-making under uncertain conditions (Howard, 1988; Clemen and Reilly, 2013; Howard and Abbas, 2015). It can leverage both quantitative information and expert knowledge, incorporate different degrees of risk aversion, and through sensitivity analyses can allow exploration of the decision space (Cegan et al., 2017; Shackelford et al., 2019). It is a recognized tool for coping with risk in agriculture (Hardaker et al., 2015) and has been applied to a range of agronomic-related topics including agroforestry adoption risks, nitrate pollution loading, cover crop species selection, optimal cropping system choices, and promoting sustainable agricultural practices (Almasri and Kaluarachchi, 2005; Gandorfer et al., 2011; Ramírez-García et al., 2015; Talukder et al., 2017; Do et al., 2020). However, to our knowledge decision analysis frameworks have had limited application regarding management decisions related to cover crops in the maize/soybean systems of the Midwestern US. Therefore, the objectives of this study were two-fold:
1) Provide a case study using publicly available data to demonstrate the process and utility of applying decision analysis to cover crop systems.
2) Use a basic analysis to suggest research priorities for cover crops in Central Iowa.
We chose to use Central Iowa as a case study because it has large areas in maize/soybean systems that are broadly representative of the US Midwest [USDA National Agricultural Statistics Service Cropland Data Layer (USDA NASS CDL), 2021], and currently demonstrates a moderate amount of cover crop adoption (Rundquist and Carlson, 2017). Furthermore, Iowa's land grant institution, Iowa State University, as well as the United States Department of Agriculture (USDA) National Laboratory for Agriculture and the Environment (NLAE) are located in Central Iowa and support a strong infrastructure for publicly funded agronomic research trials in this region that provide rich sources of public data.
2. Methods and materials
2.1. Decision set
We used cereal rye (Secale cereale L.) as our “model” over-wintering cover crop because it is the most used cover crop in Iowa and is one of the most widely used cover crop species in the Midwest (Singer, 2008). Assuming a producer has both the maize and soybean phase of a maize-soybean rotation growing at a given time, there are two scenarios for cover crop integration, each including three decision alternatives with unique benefits and challenges (Table 1). Concomitant benefits and challenges of each decision alternative highlights the need to use a quantitative approach to decision optimization, which can be achieved using decision analysis frameworks.
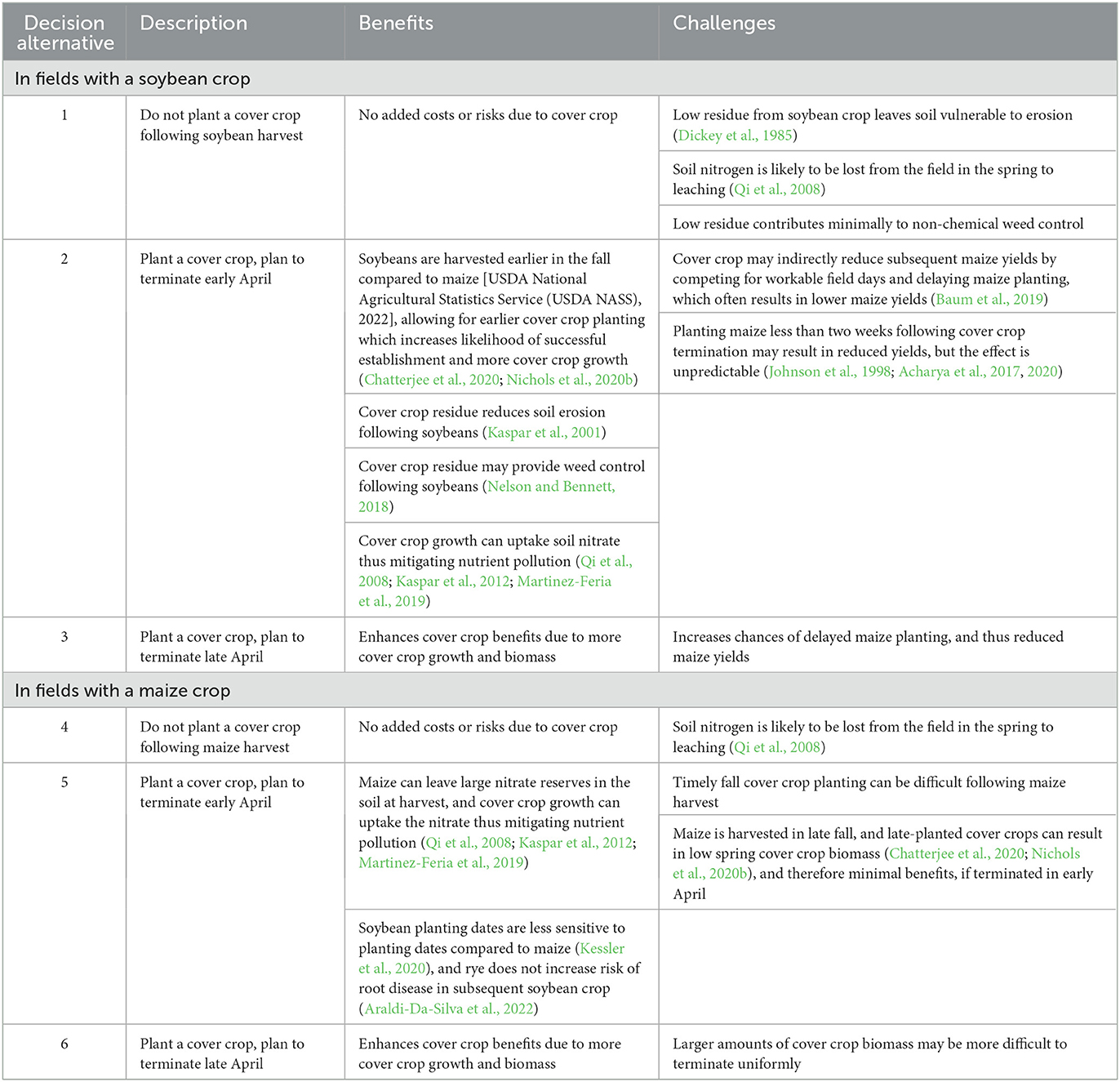
Table 1. Two scenarios each including three decision alternatives related to cover cropping in a maize/soybean rotation with various benefits and challenges associated with each alternative.
2.2. Decision structure
The decision set was translated into decision models with known states, uncertainties, and values, each described below.
2.2.1. Fall weather uncertainties
Cover crops are most often planted following cash crop harvests [Sustainable Agriculture Research and Education (SARE), 2020]. Soybean crops in Central Iowa are harvested in September or October, and maize in October or November [USDA National Agricultural Statistics Service (USDA NASS), 2022]. Planting cover crops into standing crops before harvest can increase the probability of establishment (Wilson et al., 2014) but requires specialized equipment that is not yet widely available. We therefore assume cover crop planting occurs after cash crop harvest.
Seeds require precipitation to germinate, and heat units to establish such that the plants emerge and survive the winter. Failure of a cover crop to germinate or establish in the fall results in wasted seed, wasted fuel, and possible weed problems the following spring. While the amount of precipitation needed for rye to germinate depends on soil moisture conditions at planting, crop advisors and producers often assume 1.27 cm (0.5 inches) is needed (Sarrantonio, 1994), which is consistent with field studies (Fisher et al., 2011; Wilson et al., 2013) and simulation model assumptions (Feyereisen et al., 2006; Marcillo et al., 2019). While we assumed 1.27 cm was needed for our baseline analysis, this assumption was tested through a sensitivity analysis (see Section 2.3.2).
Growing degree days (GDDs) represent an estimation of the number of heat units accumulated above a threshold temperature specific to a crop. For rye the threshold is 0 or 1°C (Feyereisen et al., 2006). We acknowledge the number of GDDs required for rye to successfully over-winter will depend on several additional factors including soil texture and snow cover. A study in Minnesota suggested rye required at least 100 GDDs in the fall to produce biomass in the spring (Kantar and Porter, 2014). We therefore estimated rye requires 100 GDDs to successfully establish before winter, but tested the sensitivity of this assumption (see Section 2.3.2).
To estimate the probability of successful rye establishment, we used 30 years of historical weather data (1988–2019) collected at the AMES-8-WSW station from the Iowa Environmental Mesonet (IEM) (2022). We chose this dataset because it had previously undergone an extensive quality check (Archontoulis et al., 2020). Using 30 years of weather data, we calculated (i) the probability the site received 1.27 cm of rainfall during an allotted timeframe, and (ii) the probability of achieving 100 GDDs in the allotted timeframe. The timeframes differed by decision alternative to account for the generally earlier harvest dates for soybean compared to maize [USDA National Agricultural Statistics Service (USDA NASS), 2022]. The precipitation timeframes were 15-Oct through 30-Nov and 1-Nov through 30-Nov for rye following soybean and rye following maize, respectively. The GDD accumulation timeframes were 15-Oct through 1-Dec and 1-Nov through 1-Dec for rye following soybean and rye following maize, respectively. We chose to calculate the precipitation and GDD probabilities separately rather than as a joint probability to aid in assessing how breeding efforts could increase changes of establishment. We recognize this model for establishment is a simplification of the complex interactions between weather, soil, and management considerations. While more sophisticated modeling approaches have been utilized for predicting cover crop establishment (Baker and Griffis, 2009; Marcillo et al., 2019; Nichols et al., 2020b), they require specialized skillsets and a significant time commitment. Our goal in this exercise is to demonstrate how insights can be obtained using publicly available data and approachable methodologies.
2.2.2. Spring weather uncertainties
Iowa has a humid continental climate wherein a significant amount of precipitation occurs during the spring months. In addition to the direct constraints on management that precipitation exerts, performing field operations in wet soils can result in undesirable outcomes including long-term soil compaction and equipment malfunctions. The USDA National Agricultural Statistics Service (NASS) surveys producers to determine the number of days suitable for fieldwork (workable-field day; WFD) for each week throughout the year [USDA National Agricultural Statistics Service (USDA NASS), 2018]. A “suitable” day is defined as one in which weather and field conditions allow producers to work in fields the majority of a given day. Determining whether a day is a “suitable” is subjective, but provides valuable information about the progress and constraints of agricultural production on a landscape level.
Historical data shows that in Iowa, the number of WFDs during the spring can severely restrict field activities (Urban et al., 2015; Edwards, 2020). To comply with governmental crop insurance cost-share policies, cover crops must be terminated before the cash crop is planted [Bergtold et al., 2019; USDA Risk Management Agency (USDA RMA), 2019]. Therefore, the presence of a living cover crop that must be terminated before the cash crop can be planted can potentially add to the spring workload for a producer. While this depends on whether producers typically have a pre-plant or pre-emergent herbicide pass, the operation is much less crucial when the goal is simply to eliminate weeds around cash crop planting compared to killing a live cover crop to comply with federal crop insurance requirements. To account for the increased importance of timely cover crop termination, in this exercise we assumed cover crop termination requires an additional set of field working-days compared to systems without a cover crop. However, because many producers do a pre-plant or pre-emergent herbicide pass in systems without cover crops, we did not assume extra herbicide or fuel costs associated with terminating the cover crop. In short, we assumed producers who plant a rye cover crop require two more spring WFDs than those who do not. This fact introduces an important component of risk that is often not accounted for explicitly in economic analyses.
The decision of cover crop termination timing will also affect WFDs, and therefore may indirectly affect cash crop yields. If a producer has WFDs in early April, the producer must choose whether to utilize them to terminate the cover crop, or wait in order to accrue more benefits from prolonged cover crop growth (Table 1). Societal-level benefits such as reduced nitrate leaching, as well as farm-level benefits such as the potential to off-set weed control costs, increase as spring cover crop termination dates are delayed and cover crop biomass increases (Finney et al., 2016; Thapa et al., 2018; Nichols et al., 2020b). However, by choosing not to utilize early April WFDs, the producer risks not having sufficient WFDs in late April to terminate the cover crop or plant the cash crop, resulting in delayed cash crop planting and a possible concomitant reduction in yields. Therefore, understanding the uncertainty around WFDs in the spring is an important component in assessing optimal decision alternatives.
In this analysis we only include the uncertainties associated with WFDs. In years with very low spring precipitation, delaying cover crop termination can also result in decreased cash crop yields due to the cover crop's use of stored soil water needed for cash crop production. While this risk is possible, due to climatic patterns it is not common in Central Iowa (Daigh et al., 2014; Martinez-Feria et al., 2016). Therefore, the risk of cover crops inducing drought-related yield reductions in the following cash crop is not considered in this exercise.
Workable field days are estimated by surveying farmers about how many days in the previous week were field-workable. The data is therefore reported as a number of days within a 7-day calendar period, with this period being inconsistent between years. For the purposes of this exercise, we chose to take the total WFDs over the 7-day reporting period and divide the total by seven to assign a number of WFDs to each calendar day the reporting week included. We then created five spring categories (early April, late April, early May, and late May, June). Workable field day values were then summed within these spring calendar categories. More details, including R code, concerning this procedure can be found in Supplementary material. We assumed cover crop termination would require two WFDs within a spring category, and cash crop planting would likewise require two WFDs. Therefore, cover crop termination and cash crop planting within a given window would require four WFDs. The probability of two and four WFDs being reported in a given spring category was calculated using 30 years of historical data (1988–2019).
2.2.3. Subsequent maize yield uncertainties
On average, winter cover crops such as rye have been shown to have a neutral effect on subsequent maize and soybean yields (Marcillo and Miguez, 2017). However, numerous studies have shown that under certain conditions, planting maize <10–14 days following cover crop termination can result in lower maize yields (Johnson et al., 1998; Pantoja et al., 2015; Acharya et al., 2017; Hirsh et al., 2021; Quinn et al., 2021). We assumed a producer would plant their maize crop as early as possible, regardless of the penalty that would be incurred due to the <14 day window. We made this assumption because conversations with producers confirmed that while they were aware there may be a yield penalty from a small termination-planting window, it was inconsistent and may not occur at all, and they were therefore more concerned with timely maize planting. We therefore assumed if there were four WFDs in a given spring category, the producer would plant maize but there would be a 50% chance of a 10% decrease in maize yield. We acknowledge that in our scenarios, the 10% yield penalty from the small termination-planting window is larger than the penalty incurred for delaying planting until late May, but we believe our decision structure captures the uncertainty currently associated with whether that yield penalty will be incurred. Soybeans are not impacted by the time between rye termination and soybean planting (Acharya et al., 2020), so no yield penalty was assigned in those circumstances.
2.2.4. Value
The main contributors to decision value were estimated using partial budgets and included the costs from planting a cover crop, the savings from planting a cover crop, and the income from the subsequent cash crop. Extension publications, farming group publications, and peer-reviewed literature were used to guide each estimation. Sensitivity analyses were performed on assumed values (Section 2.3.2), and instances where conclusions were overly sensitive to assumptions were noted.
To estimate the direct costs associated with planting and terminating a cover crop we used Iowa State University's “Economics of Cover Crops” decision tool (Iowa State University Extension, 2018). While these prices will fluctuate depending on the price of fuel and labor, we feel they are sufficiently representative for this exercise (Table 2).
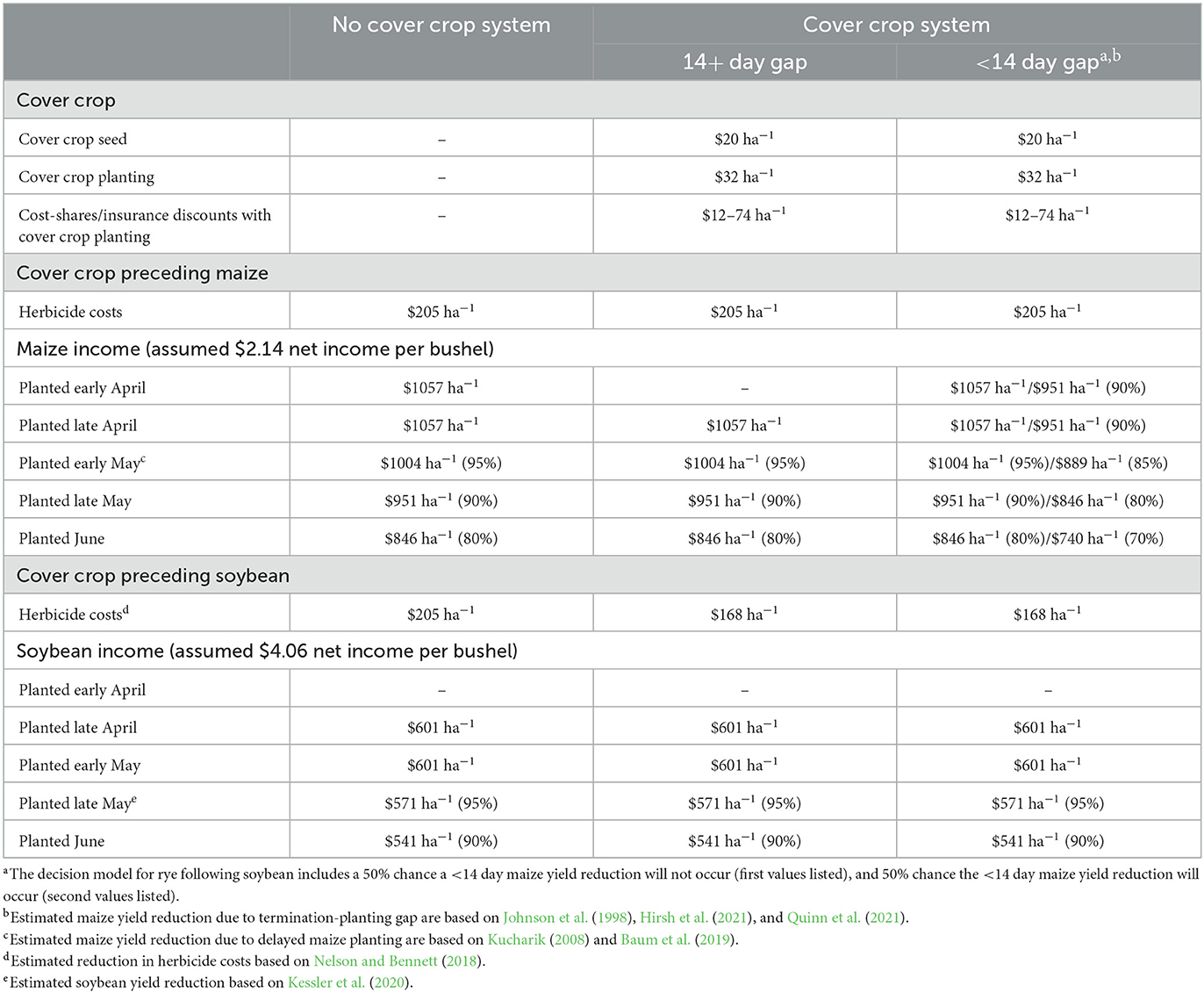
Table 2. Summary of economic assumptions for each scenario with relative cash crop yield assumptions provided in parentheses.
In order to account for the effect of cover cropping on income from crop yields, we needed to estimate the net revenue a producer expects per unit crop yield. The net revenue from a crop will depend on producer costs of production as well as market prices, both of which vary significantly across years. To overcome this variability, we looked at production costs (Iowa State University Extension, 2022) and market prices [USDA National Agricultural Statistics Service (USDA NASS), 2022) from 2013 to 2021, calculated the net revenue per unit crop yield for each year, then took the year with the maximum net revenue for each crop. By calculating the net revenue in this manner, when a rye cover crop negatively impacted cash crop yields our analyses represented the highest potential costs of those effects. All prices and calculations are available in Supplementary material.
Maize was assumed to have a maximum yield of 10.7 dry Mg ha−1 (200 bu ac−1) and soybean a yield of 1.4 dry Mg ha−1 (60 bu ac−1), which are representative of the state average yields in Iowa [USDA National Agricultural Statistics Service (USDA NASS), 2022]. Maize yield is sensitive to planting date, with later planting dates being associated with lower yields (Kucharik, 2008; Baum et al., 2019). We therefore assume a graduated yield penalty increasing 5–20% as maize planting occurs past April (Supplementary Table S2). In summary, the decision of whether to terminate the cover crop early or late impacts the available WFDs (Table 3), which impact whether the producer incurs a termination-planting penalty or a late-planting penalty, both of which impact the value of the decision.
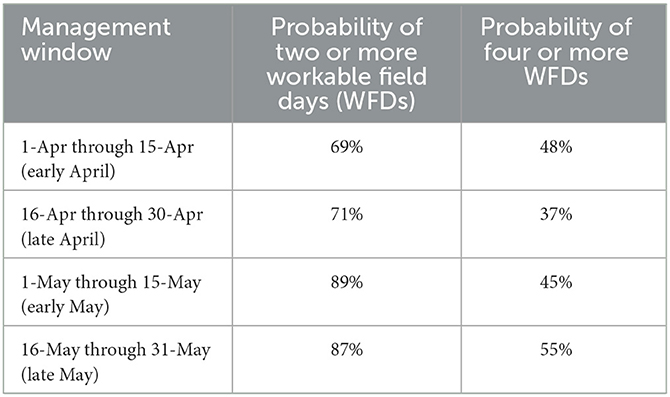
Table 3. Summary of probabilities of workable field days (WFDs) in a given timeframe based on 30 years of NASS survey data [USDA National Agricultural Statistics Service (USDA NASS), 2022].
Soybean yields are less sensitive to planting dates compared to maize (Kessler et al., 2020) and therefore was assumed to have a less severe graduated penalty as planting was delayed (5–10%; Table 2).
When the cover crop was followed by a maize crop (decision alternatives 1–3), we assumed herbicide costs were equal in the cover crop and no-cover alternatives ($205 ha−1). When the cover crop was followed by a soybean crop (decision alternatives 4–6), we utilized information from on-farm experiments showing producers reduced herbicide costs due to the mulch provided by a late-terminated cover crop. Therefore, in the decision alternative where the cover crop was terminated in late April or later followed by soybean planting (decision alternative 6), a $37 ha−1 savings in herbicides was applied (Nelson and Bennett, 2018).
There are currently no payments available to farmers in Iowa for the societal benefits reaped from delaying cover crop termination. However, other areas in the US have implemented payment structures that reward late termination due to the societal benefits gained from late termination (Maryland Department of Agriculture, 2022), so the potential for this payment in decision alternatives 3 and 6 was included in sensitivity analyses.
2.3. Decision analysis
2.3.1. Building decision trees
Decisions can be visualized and modeled using decision tree notation (Clemen and Reilly, 2013; Howard and Abbas, 2015). The full decision model is available in Supplementary material and consists of building out a branch for each unique decision node and uncertainty outcome with probabilities, then assigning a value to each branch. We assume a risk-neutral decision maker which means that the decision maker should choose the alternative that maximizes his or her expected value. A square in the decision tree represents a choice between two or more alternatives, and a circle represents an uncertainty where each branch stemming from the uncertainty is assigned a probability. The first decision for the producer is whether or not to plant a cover crop in the fall (Figure 1). If the producers choose to plant a cover crop, there is an uncertainty about whether or not sufficient precipitation occurs followed by a second uncertainty about whether or not sufficient GDDs are accumulated. If sufficient precipitation and sufficient GDDs occur, the producer makes a second decision about whether or not to terminate in early April (Supplementary Figure S1). This decision is followed by uncertainties in the number of WFDs available in a given time frame, and whether there is a penalty when maize is planted in the same spring category as cover crop termination. The decision tree is solved using a “rollback” procedure starting from the right-hand side of the tree. If a decision (square) node is encountered, the alternative with the largest expected monetary value is selected. If an uncertainty (circle) node is encountered, the expected monetary value is calculated using the probabilities on the branches as weights. This procedure results in identifying the alternative for a given decision (e.g., whether or not to plant a cover crop in the fall) that maximizes the producer's expected monetary value.
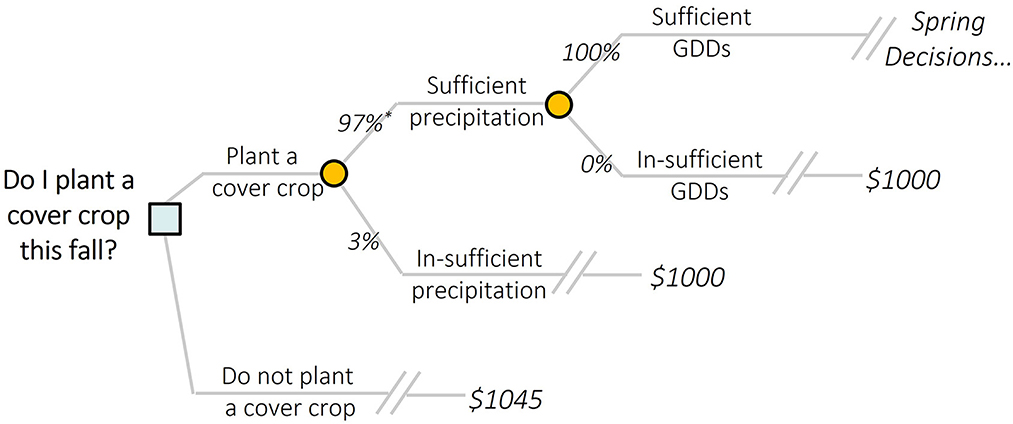
Figure 1. Decision tree visualization for planting a cover crop following a soybean crop. The first decision (light blue square) is whether or not to plant a cover crop. If the producer chooses to plant a cover crop, there is uncertainty about precipitation and growing degree days (GDDs); if the cover crop is successfully established the producer will have to decide whether to terminate the cover crop in early April, or to wait until late-April. Each decision branch has a monetary value. *See section 2.2 for calculation of these probabilities.
2.3.2. Sensitivity analyses
Sensitivity analysis on the uncertainty and parameter assumptions can provide insight into the criticality and importance of an assumption or variable to the decision. The sensitivity of outcomes was assessed for the precipitation required for rye germination (ranging from 0 to 3.5 cm in 1 mm increments), the number of GDDs needed for rye to over-winter (ranging from 0 to 300 in 5 GDD increments), the potential relative reduction in maize yields when maize was planted <14 days following rye termination (ranging from 0 to 20% in 5% increments), the incentive payments offered to plant rye (ranging from $0 to 200 ha−1 in $1 increments), and the incentive payments offered to delay termination of rye (ranging from $0 to 200 ha−1 in $1 increments). Additionally, sensitivity analyses were performed on the assumed revenues and costs associated with each scenario to ensure conclusions were not overly sensitive to these assumptions (see Supplementary material; Gupta, 2022 for details).
2.3.3. Value of information
In our decision model, if a producer has two WFDs within 14 days following cover crop termination, they have a 50% of incurring a 10% maize yield reduction if they choose to plant. This uncertainty is due to research gaps—we do not yet have sufficient information to provide a producer to help them determine whether this reduction will occur. By estimating the value of the decision if the producer knows whether the yield reduction will occur, one can estimate the “value of perfect information” (Repo, 1989). This provides an estimate of what that information would be worth to producers, thus allowing researchers to assess how impactful such research would be. We therefore estimated the value of knowing when there would not be a reduction in maize yields when planting <14 days after cover crop termination.
3. Results and discussion
3.1. Optimal decisions
Assuming there is no cost-share available for planting a cover crop and long-term or societal economic benefits are not accounted for, the overall expected monetary value of not planting a cover crop is greater than the expected monetary value of planting a cover crop, regardless of the sequencing scenario (Figure 2). This analysis shows that in addition to the cost of seed and fuel to plant the cover crop ($52 ha−1), when rye precedes maize there is an additional $40–70 ha−1 cost associated with the risk that the spring management of the cover crop will result in reduced maize yields (either through delayed maize planting due to insufficient WFDs or <14 day gap penalties). When rye precedes soybeans, the costs of planting the cover crop and risks of reduced yields due to delayed planting are partially compensated by through reduced herbicide costs. Within the decision sets that include the alternative of planting a cover crop, the value of the decision is always maximized if the cover crop is terminated in early vs. late April.
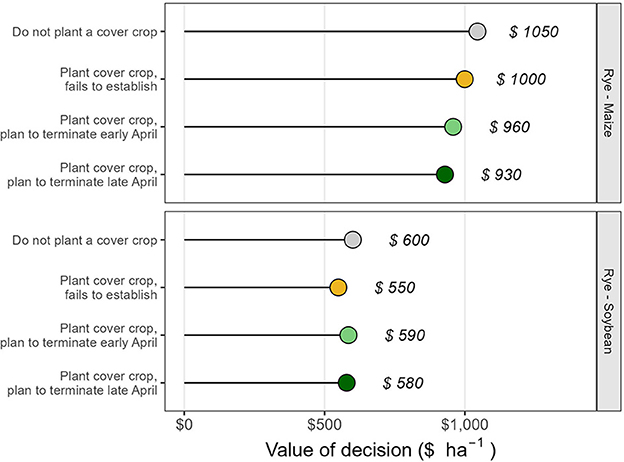
Figure 2. Value of each decision alternative (rounded to the nearest $10) assuming no incentive/cost share payments for planting a cover crop.
Many of the benefits reaped from planting cover crops (e.g., reduced soil erosion, reduced nitrate leaching, non-chemical weed control) are directly related to the amount of biomass the cover crop produces (Finney et al., 2016; Thapa et al., 2018; Nichols et al., 2020b). However, in areas that lack incentives for delaying cover crop termination to allow the cover crop to grow, our analyses show the optimal decision is to terminate the cover crop as soon as possible, even when there might be cost savings from reduced herbicide use (Rye-Soybean scenario in Figure 2). Notably, the termination decision differential is highest when the cover crop precedes maize, meaning the sequencing where society may benefit the most (higher mitigation of erosion and nitrate leaching, Table 1) would also require the highest incentives to render late April termination the optimal decision. The US state of Maryland has created a tiered incentive system wherein producers are compensated more for early planting and late termination of cover crops (Maryland Department of Agriculture, 2022). Our analysis indicates having compensation rates differ by cropping sequence may also be an approach worth considering.
Our analyses also expose a potential moral hazard. If a producer chooses to plant a cover crop preceding a maize crop and receives a cost-share or incentive for doing so, failed cover crop establishment will lead to a better financial result than successful establishment (Rye-Maize scenario in Figure 2). It is important to provide support for producers as they learn to manage cover crops, and often cover crop establishment is out of a producer's control, but our analyses demonstrate the complexity in determining the best payment structures, and the need to include the risks the cover crop may pose to the subsequent crop yields.
3.2. Sensitivity to cost-share/incentives
If there are no cost-shares or incentive programs, the overall expected monetary value of not planting any cover crop is greater than the expected value of planting a cover crop, regardless of the sequencing scenario (in the top panel of Figure 3, this is seen from the “do not plant rye” alternative having a greater value when the cost share or incentive is $0 on the horizontal axis). However, current incentive programs may be enough to make planting a cover crop preceding a soybean cash crop (“Rye-Soybean”) the optimal decision. If the incentive is greater than $30 ha−1, the expected monetary value of planting rye prior to soybeans is greater than not planting rye.
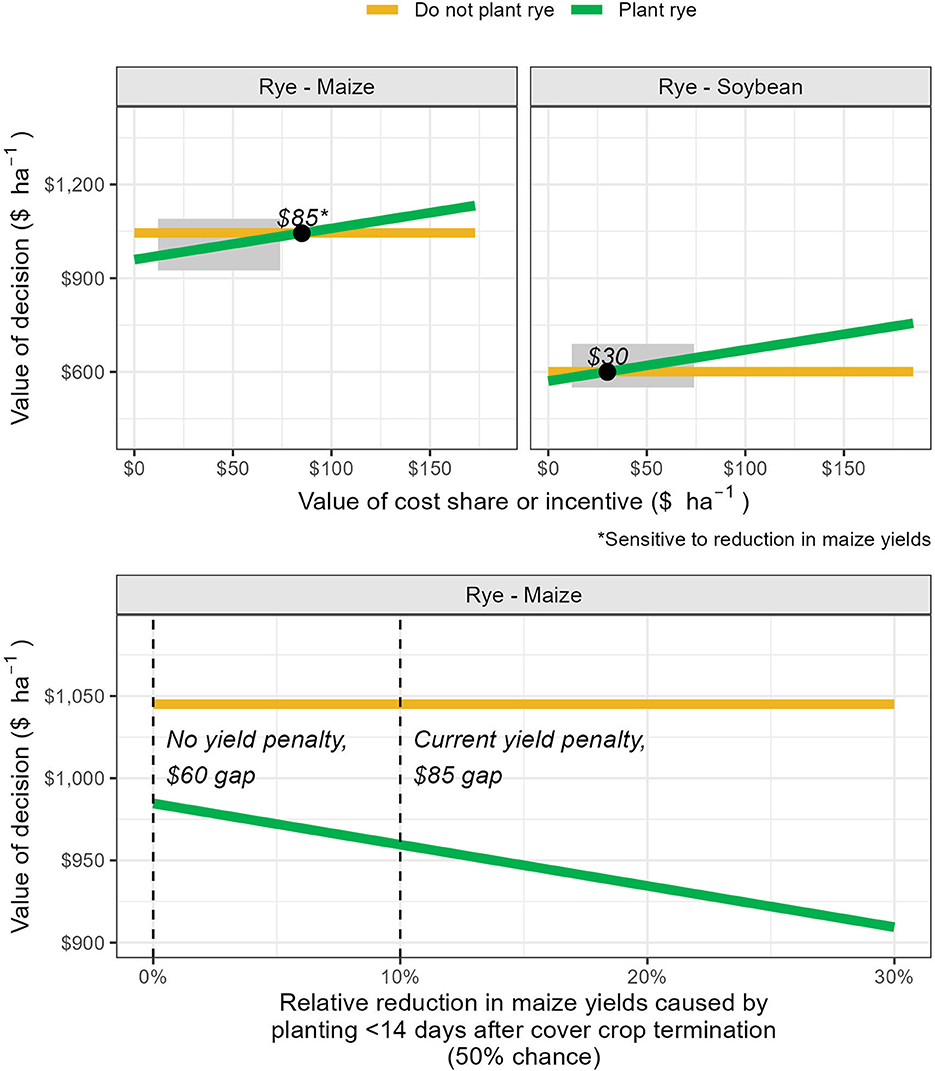
Figure 3. (Top) Planting a rye cover crop (green line) required $85 ha−1 and $30 cost shares/incentives, respectively for a soybean-rye-maize and maize-rye-soybean scenario to make decision values equal to not planting a cover crop (gold line); gray box represents range of current incentive values. (Bottom) Current estimates show maize yields can be reduced by approximately 10% when maize is planted <14 days after terminating a cover crop; if agronomic research efforts were able to eliminate this yield reduction the difference in decision values would be within the range of current incentive programs.
When a cover crop precedes a maize crop (“Rye-Maize” in top panel of Figure 3), within the current range of incentives the optimal decision is to not plant a cover crop. However, this recommendation is sensitive to the reduction in maize yield due to planting <14 days following cover crop termination (bottom panel of Figure 3). If the potential reduction in yield were eliminated, the difference between the value of not planting a cover crop and planting a cover crop could be reduced from $85 ha−1 to $60 ha−1, bringing the difference into the range of current incentive programs in this area ($12–74 ha−1).
The exact causes of the reduced yield in maize are not yet clear and it is currently not possible to predict when they will manifest (e.g., Patel et al., 2019; Quinn et al., 2021). The value of perfect information is worth $20–25 depending on the planned cover crop timing, which is roughly equal to the increased value from eliminating the yield penalty. This indicates that research that allows producers to accurately predict when the yield penalty will occur is equally as valuable as eliminating the yield penalty. Potential mechanisms include altered nutrient dynamics, disease pressure, allelopathy, rye stands that are not fully terminated, changes in soil temperature and/or moisture in a rye cover crop system. A meta-analysis of studies may aid in identifying factors that drive the variation in the effect. Our analyses demonstrate that this phenomenon poses a significant risk to producers, and a better understanding of the drivers and identification of ways to predict when yield declines are likely would greatly reduce the financial risk associated with planting a rye cover crop in these systems.
3.3. Sensitivity to weather
On average, Central Iowa received 7.4 and 4.2 cm of rain from 15-Oct and 1-Nov through 30-Nov, respectively. This equated to a high probability (>80%) of the rye cover crop receiving sufficient precipitation for germination (>1.27 cm) in both sequences (Figure 4, Supplementary Table S3). This result was robust against uncertainty in our assumptions; even if rye required almost double the assumed precipitation, the probability of receiving that amount of rainfall did not drop below 80% for either planting scenario (Figure 4). While the probability of accumulating sufficient GDDs (100) was 100% when the rye was planted following soybeans (15-Oct planting date), it dropped to 71% chance of success when planted following maize (1-Nov planting date; Supplementary Table S3). The probability of establishment was very sensitive to the sequencing (rye following soybeans or rye following maize). For the 1-Nov planting date, the results are very sensitive to the assumed GDDs required for establishment.
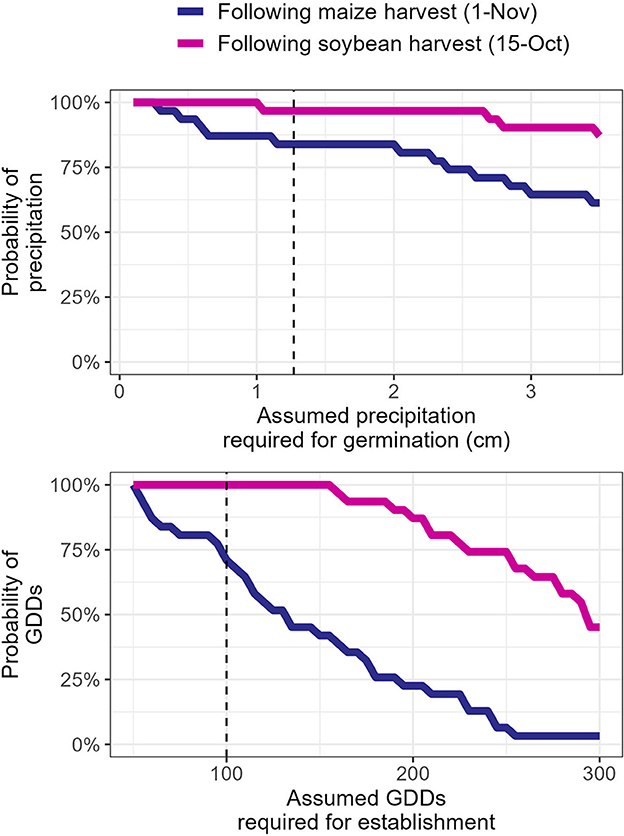
Figure 4. Sensitivity of outcomes to assumption of fall weather for rye planted following maize (dark blue; 1-Nov planting date) and following soybeans (pink; 15-Oct planting date). (Top) The probability of receiving sufficient precipitation is not sensitive to sequencing nor the assumed amount required for germination. (Bottom) The probability of accumulating sufficient GDDs for rye establishment is very sensitive to both the sequencing and the number of GDDs required.
These results can be used to guide research efforts. Our analysis demonstrates that in most cases, precipitation is not the limiting factor for cover crop establishment in Central Iowa. Breeding varieties that require less precipitation to germinate would likely involve breeding for smaller seeds, which carries inherent tradeoffs (e.g., Carleton and Cooper, 1972; Mohler et al., 2009). A study done in Minnesota showed precipitation accounts for the highest amount of variation in rye establishment, followed by temperature (Wilson et al., 2013), demonstrating the value of evaluating weather-related risks locally. While our results do not account for how the precipitation is distributed across time and how that may impact germination, our results suggest this area of Iowa can support larger precipitation requirements for cover crops without experiencing a significant reduction in the probability of cover crop germination.
Our results also show when planting after soybean harvest, the cover crop is almost guaranteed to gain 100 GDDs in the fall (Figure 4). Conversely, after maize harvest the probability is very sensitive to how many GDDs are assumed to be needed. Our analyses highlight the need to better understand conditions that lead to successful establishment, particularly in the later months of the year. Additionally, research focused on identifying management tactics that allow for earlier cover crop planting may be most effective in increasing the probability of successful cover crop establishment in Central Iowa. For example, some producers report switching to earlier maturing soybean and maize varieties when adopting cover crops in order to plant the cover crop earlier (Plastina et al., 2020). Some areas have organized blocks of producers who share in aerial seeding costs, and custom seeding equipment/services that allows for seeding into a standing crop are becoming more common. Our analyses indicate these types of activities are well-suited to reducing the risk associated with planting a cover crop in Central Iowa.
In the spring, the number of WFDs presented a great deal of uncertainty (Table 3). Averaged over the entire spring period (1-Apr through 31-May), there was a 79% probability of two or more WFDs in a given 2-week period, and only a 46% probability of four or more WFDs. We assumed two or more WFDs were needed to successfully complete a cover crop termination activity, and two additional WFDs were needed to complete cash crop planting activities. Therefore, producers wishing to terminate and plant within a 2-week period may not have sufficient WFDs to do so. The probability of two or more WFDs was higher in May compared to April, indicating paying producers to delay cover crop termination may also increase the chances the producer can terminate in their planned timeframe.
Our analyses indicate in Central Iowa, there is generally a high probability the fall conditions will foster cover crop establishment, and that the majority of risk occurs due to the potential for the additional management required in the spring to delay cash crop planting. A Midwestern focus group found some producers had been switching to winterkill cover crop varieties because of the difficulties associated with killing the cover crop and planting a cash crop in a timely manner in the spring (Plastina et al., 2020). For this analysis we assumed the rye cover crop could be terminated at any point, but the stage of rye growth will affect how easy it is to terminate, particularly when using mechanical termination (Creamer and Dabney, 2002; Mirsky et al., 2009). Decision support tools that help producers decide if early termination is the best choice could be beneficial in helping producers manage this risk.
4. Conclusions
Using publicly available data and reasonable assumptions, we were able to gain significant insight into localized priorities for cover crop research. Using historical weather data, NASS surveys on WFDs, extension publications, and a partial budget for cover crop economics we were able to build a single-attribute decision model, and model decision values assuming a risk-neutral producer. Our analysis does not include possible long-term impacts such as the maintenance of productivity, long-term impacts on weeds or insects, or changes in yield stability over time, which could be incorporated in future applications of this framework. We found including only the costs of seed and fuel in cover crop economics underestimates the additional financial risk producers assume due to the extra spring work cover crops might entail in areas with limited numbers of WFDs during that time. We found there is minimal information on the number of GDDs required for a rye cover crop to successfully overwinter, and that this may have a large impact on risks associated with planting cover crops in Central Iowa. In Central Iowa, identifying ways to ensure early cover crop planting and managements that render maize yields less sensitive to rye cover crop termination timing, or that allow that reduction to be more predictable, could significantly help reduce the financial risk of planting cover crops. Furthermore, flat payments for planting cover crops may result in a moral hazard, wherein the decision value for planting a cover crop preceding a maize crop is maximized when the cover crop fails to establish in the fall. Policies that promote tiered payment structures could rectify this while still providing support for producers as they learn to manage cover crops.
Data availability statement
Publicly available datasets were analyzed in this study. The data and R code for this study are available in a public github repository: https://github.com/vanichols/Nichols_Frontiers_CoverCropRisk.
Author contributions
GN conceived of and designed the analyses, collected the data, performed the analyses, and wrote the first draft of the manuscript. CM designed the analyses and edited the manuscript. All authors contributed to the article and approved the submitted version.
Funding
This material is based upon work supported in part by theNational Science Foundation (Grant No. DGE-1828942). The open access publication fees for this article were covered by the Iowa State University Library.
Acknowledgments
We acknowledge Dr. Sarah Ryan for organizing a workshop for the NSF DataFEWSion program that sparked this collaboration, Dr. Raj Raman for his helpful feedback, and two reviewers for their insightful comments and suggestions that greatly improved the quality of this manuscript. We also thank Dr. Sotirios Archontoulis and Isaiah Huber for providing cleaned versions of the publicly available weather and workable field days data.
Conflict of interest
The authors declare that the research was conducted in the absence of any commercial or financial relationships that could be construed as a potential conflict of interest.
Publisher's note
All claims expressed in this article are solely those of the authors and do not necessarily represent those of their affiliated organizations, or those of the publisher, the editors and the reviewers. Any product that may be evaluated in this article, or claim that may be made by its manufacturer, is not guaranteed or endorsed by the publisher.
Supplementary material
The Supplementary Material for this article can be found online at: https://www.frontiersin.org/articles/10.3389/fsufs.2023.1040927/full#supplementary-material
References
Acharya, J., Bakker, M. G., Moorman, T. B., Kaspar, T. C., Lenssen, A. W., and Robertson, A. E. (2017). Time interval between cover crop termination and planting influences corn seedling disease, plant growth, and yield. Plant Dis. 101, 591–600. doi: 10.1094/PDIS-07-16-0975-RE
Acharya, J., Moorman, T. B., Kaspar, T. C., Lenssen, A. W., and Robertson, A. E. (2020). Cover crop rotation effects on growth and development, seedling disease, and yield of corn and soybean. Plant Dis. 104, 677–687. doi: 10.1094/PDIS-09-19-1904-RE
Aglasan, S., and Rejesus, R. M. (2021). “An analysis of crop insurance losses, cover crops, and weather in US Crop Production,” in 2021 Agricultural and Applied Economics Association Annual Meeting (Austin, TX).
Aguilar, J., Gramig, G. G., Hendrickson, J. R., Archer, D. W., Forcella, F., et al. (2015). Crop species diversity changes in the United States: 1978–2012. PLoS ONE 10, e0136580. doi: 10.1371/JOURNAL.PONE.0136580
Almasri, M. N., and Kaluarachchi, J. J. (2005). Multi-criteria decision analysis for the optimal management of nitrate contamination of aquifers. J. Environ. Manage. 74, 365–381. doi: 10.1016/j.jenvman.2004.10.006
Araldi-Da-Silva, G., Kandel, Y. R., Han, G., Mueller, D. S., Helmers, M. J., Kaspar, T. C., et al. (2022). Field studies on the effect of rye cover crop on soybean root disease and productivity. PhytoFrontiers. 2, 192–201. Available online at: https://apsjournals.apsnet.org/doi/epdf/10.1094/PHYTOFR-05-21-0038-R
Arbuckle, J. G., and Roesch-McNally, G. (2015). Cover crop adoption in Iowa: The role of perceived practice characteristics. J. Soil Water Conserv. 70, 418–429. doi: 10.2489/jswc.70.6.418
Archontoulis, S. V., Castellano, M. J., Licht, M. A., Nichols, V., Baum, M., Huber, I., et al. (2020). Predicting crop yields and soil-plant nitrogen dynamics in the US Corn Belt. Crop Sci. 60, 721–738. doi: 10.1002/csc2.20039
Baker, J. M., and Griffis, T. J. (2009). Evaluating the potential use of winter cover crops in corn-soybean systems for sustainable co-production of food and fuel. Agric. For. Meteorol. 149, 2120–2132. doi: 10.1016/j.agrformet.2009.05.017
Basche, A., and DeLonge, M. (2017). The impact of continuous living cover on soil hydrologic properties: a meta-analysis. Soil Sci. Soc. Am. J. 81, 1179–1190. doi: 10.2136/sssaj2017.03.0077
Basche, A. D., and Roesch-McNally, G. E. (2017). Research topics to scale up cover crop use: Reflections from innovative Iowa farmers. J. Soil Water Conserv. 72, 59A−63A. doi: 10.2489/jswc.72.3.59A
Baum, M. E., Archontoulis, S. V., and Licht, M. A. (2019). Planting date, hybrid maturity, and weather effects on maize yield and crop stage. Agron. J. 111, 303–313. doi: 10.2134/agronj2018.04.0297
Bedford, T., and Cooke, R. (2001). Probabilistic Risk Analysis: Foundations and Methods. Cambridge: Cambridge University Press. doi: 10.1017/CBO9780511813597
Bergtold, J. S., Ramsey, S., Maddy, L., and Williams, J. R. (2019). A review of economic considerations for cover crops as a conservation practice. Renew. Agric. Food Syst. 34, 62–76. doi: 10.1017/S1742170517000278
Boryan, C., Yang, Z., Mueller, R., and Craig, M. (2011). Monitoring US agriculture: the US Department of Agriculture, National Agricultural Statistics Service, Cropland Data Layer Program. Geocarto Int. 26, 341–358. doi: 10.1080/10106049.2011.562309
Carleton, A. E., and Cooper, C. S. (1972). Seed size effects upon seedling vigor of three forage legumes 1. Crop Sci. 12, 183–186. doi: 10.2135/CROPSCI1972.0011183X001200020008X
Carlson, S., and Stockwell, R. (2013). Research priorities for advancing adoption of cover crops in agriculture-intensive regions. J. Agric. Food Syst. Commun. Develop. 3, 125–129. doi: 10.5304/jafscd.2013.034.017
Cegan, J. C., Filion, A. M., Keisler, J. M., and Linkov, I. (2017). Trends and applications of multi-criteria decision analysis in environmental sciences: literature review. Environ. Syst. Decis. 37, 123–133. doi: 10.1007/s10669-017-9642-9
Chatterjee, N., Archontoulis, S. V., Bastidas, A., Proctor, C. A., Elmore, R. W., and Basche, A. D. (2020). Simulating winter rye cover crop production under alternative management in a corn-soybean rotation. Agron. J. 112, 4648–4665. doi: 10.1002/agj2.20377
Chen, L., Rejesus, R. M., Aglasan, S., Hagen, S. C., and Salas, W. (2022). The of cover on soil erosion in the US Midwest. J. Environ. Manage. 324. doi: 10.1016/j.jenvman.2022.116168
Church, S. P., Lu, J., Ranjan, P., Reimer, A. P., and Prokopy, L. S. (2020). The role of systems thinking in cover crop adoption: implications for conservation communication. Land Use Policy 94, 104508. doi: 10.1016/j.landusepol.2020.104508
Clemen, R. T., and Reilly, T. (2013). Making hard decisions with DecisionTools. Beijing: Cengage Learning.
Creamer, N. G., and Dabney, S. M. (2002). Killing cover crops mechanically: review of recent literature and assessment of new research results. Am. J. Altern. Agric. 17, 32–40. doi: 10.1079/AJAA200204
Crossley, M. S., Burke, K. D., Schoville, S. D., and Radeloff, V. C. (2021). Recent collapse of crop belts and declining diversity of US agriculture since 1840. Glob. Chang. Biol. 27, 151–164. doi: 10.1111/gcb.15396
Daigh, A. L., Helmers, M. J., Kladivko, E., Zhou, X., Goeken, R., Cavdini, J., et al. (2014). Soil water during the drought of 2012 as affected by rye cover crops in fields in Iowa and Indiana. J. Soil Water Conserv. 69, 564–573. doi: 10.2489/jswc.69.6.564
Dickey, E. C., Shelton, D. P., Jasa, P. J., and Peterson, T. R. (1985). Soil erosion from tillage systems used in soybean and corn residues. Trans. ASAE 28, 1124–1130. doi: 10.13031/2013.32399
Do, H., Luedeling, E., and Whitney, C. (2020). Decision analysis of agroforestry options reveals adoption risks for resource-poor farmers. Agron. Sustain. Dev. 40, 1–12. doi: 10.1007/s13593-020-00624-5
Edwards, W. (2020). The Number of Days Suitable for Fieldwork in Iowa is Shrinking. Iowa Staet University Extension and Outreach. Available online at: https://www.extension.iastate.edu/agdm/articles/edwards/EdwMar20.html (accessed September 2, 2022).
Feyereisen, G. W., Wilson, B. N., Sands, G. R., Strock, J. S., and Porter, P. M. (2006). Potential for a rye cover crop to reduce nitrate loss in southwestern Minnesota. Agron. J. 98, 1416–1426. doi: 10.2134/agronj2005.0134
Finney, D. M., White, C. M., and Kaye, J. P. (2016). Biomass production and carbon/nitrogen ratio influence ecosystem services from cover crop mixtures. Agron. J. 108, 39–52. doi: 10.2134/agronj15.0182
Fisher, K. A., Momen, B., and Kratochvil, R. J. (2011). Is broadcasting seed an effective winter cover crop planting method? Agron. J. 103, 472–478. doi: 10.2134/agronj2010.0318
Food Agriculture Organization (FAO) (2020). FAOSTAT Crops and Livestock Products. Available online at: https://www.fao.org/faostat/en/#data/QCL (accessed September 1, 2022).
Gandorfer, M., Pannell, D., and Meyer-Aurich, A. (2011). Analyzing the effects of risk and uncertainty on optimal tillage and nitrogen fertilizer intensity for field crops in Germany. Agric. Syst. 104, 615–622. doi: 10.1016/j.agsy.2011.06.004
Gupta, S. (2022). Sensitivity Analysis in Excel. One and Two Variable Data Table. Available online at: https://www.wallstreetmojo.com/sensitivity-analysis-in-excel/ (accessed September 1, 2022).
Hamilton, A. V., Mortensen, D. A., and Allen, M. K. (2017). The state of the cover crop nation and how to set realistic future goals for the popular conservation practice. J. Soil Water Conserv. 72, 111A−115A. doi: 10.2489/jswc.72.5.111A
Hardaker, J. B., Lien, G., Anderson, J. R., and Huirne, R. B. (2015). Coping With Risk in Agriculture: Applied Decision Analysis. Wallingford: CABI Publishing. doi: 10.1079/9781780645742.0000
Hatfield, J. L., McMullen, L. D., and Jones, C. S. (2009). Nitrate-nitrogen patterns in the Raccoon River Basin related to agricultural practices. J. Soil Water Conserv. 64, 190–199. doi: 10.2489/jswc.64.3.190
Hijmans, R. J., Choe, H., and Perlman, J. (2016). Spatiotemporal patterns of field crop diversity in the United States, 1870–2012. Agric. Environ. Lett. 1, 160022. doi: 10.2134/ael2016.05.0022
Hirsh, S. M., Duiker, S. W., Graybill, J., Nichols, K., and Weil, R. R. (2021). Scavenging and recycling deep soil nitrogen using cover crops on mid-Atlantic, USA farms. Agric. Ecosyst. Environ. 309, 107274. doi: 10.1016/j.agee.2020.107274
Howard, R., and Abbas, A. E. (2015). Foundations of Decision Analysis. Pearson. Available online at: https://www.amazon.com/Foundations-Decision-Analysis-Ronald-Howard/dp/0132336243
Howard, R. A. (1988). Decision analysis: practice and promise. Manage. Sci. 34, 679–695. doi: 10.1287/mnsc.34.6.679
Hubbard, D. W. (2020). The Failure of Risk Management: Why It's Broken and How to Fix It. New York: John Wiley and Sons. doi: 10.1002/9781119521914
Iowa Environmental Mesonet (IEM) (2022). Available online at: https://mesonet.agron.iastate.edu (accessed September 1, 2022).
Iowa State University Extension (2018). Economics of Cover Crops Worksheets. Available online at: https://www.extension.iastate.edu/agdm/crops/html/a1-91.html (accessed September 1, 2022).
Iowa State University Extension (2022). Esimated Costs of Crop Production. Available online at: https://www.extension.iastate.edu/agdm/crops/html/a1-20.html (accessed September 1, 2022).
Johnson, T. J., Kaspar, T. C., Kohler, K. A., Corak, S. J., and Logsdon, S. D. (1998). Oat and rye overseeded into soybean as fall cover crops in the upper Midwest. J. Soil Water Conserv. 53, 276–279.
Kantar, M., and Porter, P. (2014). Relationship between planting date, growing degree days and the winter rye (Secale cereale L.) variety “Rymin” in Minnesota. Crop Manage. 13, 1–9. doi: 10.2134/CM-2013-0096-RS
Kaplan, S., and Garrick, B. J. (1981). On the quantitative definition of risk. Risk Anal. 1, 11–27. doi: 10.1111/j.1539-6924.1981.tb01350.x
Kaspar, T. C., Jaynes, D. B., Parkin, T. B., and Moorman, T. B. (2007). Rye cover crop and gamagrass strip effects on NO3 concentration and load in tile drainage. J. Environ. Qual. 36, 1503–1511. doi: 10.2134/jeq2006.0468
Kaspar, T. C., Jaynes, D. B., Parkin, T. B., Moorman, T. B., and Singer, J. W. (2012). Effectiveness of oat and rye cover crops in reducing nitrate losses in drainage water. Agric. Water Manage. 110, 25–33. doi: 10.1016/j.agwat.2012.03.010
Kaspar, T. C., Radke, J. K., and Laflen, J. M. (2001). Small grain cover crops and wheel traffic effects on infiltration, runoff, and erosion. J. Soil Water Conserv. 56, 160–164. Available online at: https://www.jswconline.org/content/56/2/160
Kessler, A., Archontoulis, S. V., and Licht, M. A. (2020). Soybean yield and crop stage response to planting date and cultivar maturity in Iowa, USA. Agron. J. 112, 382–394. doi: 10.1002/agj2.20053
Krupek, F. S., Mizero, S. M., Redfearn, D., and Basche, A. (2022). Assessing how cover crops close the soil health gap in on-farm experiments. Agric. Environ. Lett. 7, e20088. doi: 10.1002/ael2.20088
Kucharik, C. J. (2008). Contribution of planting date trends to increased maize yields in the central United States. Agron. J. 100, 328–336. doi: 10.2134/agronj2007.0145
Lee, D., Arbuckle, J. G., Zhu, Z., and Nowatzke, L. (2018). Conditional causal mediation analysis of factors associated with cover crop adoption in Iowa, USA. Water Resour. Res. 54, 9566–9584. doi: 10.1029/2017WR022385
Marcillo, G. S., Carlson, S., Filbert, M., Kaspar, T., Plastina, A., and Miguez, F. E. (2019). Maize system impacts of cover crop management decisions: a simulation analysis of rye biomass response to planting populations in Iowa, USA. Agric. Syst. 176, 102651. doi: 10.1016/j.agsy.2019.102651
Marcillo, G. S., and Miguez, F. E. (2017). Corn yield response to winter cover crops: an updated meta-analysis. J. Soil Water Conserv. 72, 226–239. doi: 10.2489/jswc.72.3.226
Martinez-Feria, R., Nichols, V., Basso, B., and Archontoulis, S. (2019). Can multi-strategy management stabilize nitrate leaching under increasing rainfall? Environ. Res. Lett. 14, 124079. doi: 10.1088/1748-9326/ab5ca8
Martinez-Feria, R. A., Dietzel, R., Liebman, M., Helmers, M. J., and Archontoulis, S. V. (2016). Rye cover crop effects on maize: a system-level analysis. Field Crops Res. 196, 145–159. doi: 10.1016/j.fcr.2016.06.016
Maryland Department of Agriculture (2022). Maryland's 2022–2023 Cover Crop Program. Available online at: https://mda.maryland.gov/resource_conservation/Pages/cover_crop.aspx (accessed September 1, 2022).
Mirsky, S. B., Curran, W. S., Mortensen, D. A., Ryan, M. R., and Shumway, D. L. (2009). Control of cereal rye with a roller/crimper as influenced by cover crop phenology. Agron. J. 101, 1589–1596. doi: 10.2134/agronj2009.0130
Mitsch, W. J., Day, J. W., Gilliam, J. W., Groffman, P. M., Hey, D. L., Randall, G. W., et al. (2001). Reducing nitrogen loading to the Gulf of Mexico from the Mississippi River Basin: strategies to counter a persistent ecological problem: ecotechnology-the use of natural ecosystems to solve environmental problems-should be a part of efforts to shrink the zone of hypoxia in the Gulf of Mexico. Bioscience 51, 373–388. doi: 10.1641/0006-3568(2001)051(0373:RNLTTG)2.0.CO;2
Mohler, C. L., Liebman, M., and Staver, C. P. (2009). Weed Life History: Identifying Vulnerabilities. Ecological Management of Agricultural Weeds. Cambridge: Cambridge University Press, 40–98. doi: 10.1017/CBO9780511541810
Moore, E. B., Wiedenhoeft, M. H., Kaspar, T. C., and Cambardella, C. A. (2014). Rye cover crop effects on soil quality in no-till corn silage-soybean cropping systems. Soil Sci. Soc. Am. J. 78, 968–976. doi: 10.2136/sssaj2013.09.0401
Nelson, H., and Bennett, S. (2018). Cereal Rye Cover Crop for Reducing Herbicides in Soybeans. Practical Farmers of Iowa Cooperators' Program. Available online at: https://practicalfarmers.org/research/cereal-rye-cover-crop-for-reducing-herbicides-in-soybeans/ (accessed February 2022).
Nichols, V., English, L., Carlson, S., Gailans, S., and Liebman, M. (2020a). Effects of long-term cover cropping on weed seedbanks. Front. Agron. 2, 591091. doi: 10.3389/fagro.2020.591091
Nichols, V., Martinez-Feria, R., Weisberger, D., Carlson, S., Basso, B., and Basche, A. (2020b). Cover crops and weed suppression in the US Midwest: a meta-analysis and modeling study. Agricultural and Environmental Letters 5, e20022. doi: 10.1002/ael2.20022
Nichols, V. A., Moore, E. B., Gailans, S., Kaspar, T. C., and Liebman, M. (2022). Site-specific effects of winter cover crops on soil water storage. Agrosyst. Geosci. Environ. 5, e20238. doi: 10.1002/agg2.20238
Pantoja, J. L., Woli, K. P., Sawyer, J. E., and Barker, D. W. (2015). Corn nitrogen fertilization requirement and corn-soybean productivity with a rye cover crop. Soil Sci. Soc. Am. J. 79, 1482–1495. doi: 10.2136/sssaj2015.02.0084
Park, B., Rejesus, R. M., Aglasan, S., Che, Y., Hagen, S. C., and Salas, W. (2022). Payments from agricultural conservation programs and cover crop adoption. Appl. Econ. Perspect. Policy. doi: 10.1002/aepp.13248
Patel, S., Sawyer, J. E., and Lundvall, J. P. (2019). Can management practices enhance corn productivity in a rye cover crop system? Agron. J. 111, 3161–3171. doi: 10.2134/agronj2019.03.0158
Plastina, A., Liu, F., Miguez, F., and Carlson, S. (2020). Cover crops use in Midwestern US agriculture: perceived benefits and net returns. Renew. Agric. Food Syst. 35, 38–48. doi: 10.1017/S1742170518000194
Qi, Z., Helmers, M. J., and Lawlor, P. A. (2008). “Effect of different land covers on nitrate-nitrogen leaching and nitrogen uptake in Iowa,” in 2008 Providence, Rhode Island, June 29-July 2, 2008 (p. 1). American Society of Agricultural and Biological Engineers.
Quinn, D. J., Poffenbarger, H. J., Leuthold, S. J., and Lee, C. D. (2021). Corn response to in-furrow fertilizer and fungicide across rye cover crop termination timings. Agron. J. 113, 3384–3398. doi: 10.1002/agj2.20723
Ramírez-García, J., Carrillo, J. M., Ruiz, M., Alonso-Ayuso, M., and Quemada, M. (2015). Multicriteria decision analysis applied to cover crop species and cultivars selection. Field Crops Res. 175, 106–115. doi: 10.1016/j.fcr.2015.02.008
Repo, A. J. (1989). The value of information: approaches in economics, accounting, and management science. J. Am. Soc. Inform. Sci. 40, 68–85. doi: 10.1002/(SICI)1097-4571(198903)40:2<68::AID-ASI2>3.0.CO;2-J
Rundquist, S., and Carlson, S. (2017). Mapping Cover Crops on Corn and Soybeans in Illinois, Indiana and Iowa, 2015–2016. Environmental Working Group. Available online at: https://www.ewg.org/research/mapping-cover-crops-corn-and-soybeans-illinois-indiana-and-iowa-2015-2016 (accessed September 1, 2022).
Sarrantonio, M. (1994). Northeast Cover Crop Handbook. Kutztown, PA: Soil Health Series. Rodale Institute.
Seifert, C. A., Azzari, G., and Lobell, D. B. (2018). Satellite detection of cover crops and their effects on crop yield in the Midwestern United States. Environ. Res. Lett. 13, 064033. doi: 10.1088/1748-9326/aac4c8
Shackelford, G. E., Kelsey, R., Sutherland, W. J., Kennedy, C. M., Wood, S. A., Gennet, S., et al. (2019). Evidence synthesis as the basis for decision analysis: a method of selecting the best agricultural practices for multiple ecosystem services. Front. Sustain. Food Systems 3, 83. doi: 10.3389/fsufs.2019.00083
Singer, J. W. (2008). Corn belt assessment of cover crop management and preferences. Agron. J. 100, 1670–1672. doi: 10.2134/agronj2008.0151
Sustainable Agriculture Research Education (SARE) (2020). National Cover Crop Surveys. Available online at: https://www.sare.org/publications/cover-crops/national-cover-crop-surveys/ (accessed September 2, 2022).
Syswerda, S. P., Basso, B., Hamilton, S. K., Tausig, J. B., and Robertson, G. P. (2012). Long-term nitrate loss along an agricultural intensity gradient in the Upper Midwest USA. Agric. Ecosyst. Environ. 149, 10–19. doi: 10.1016/j.agee.2011.12.007
Talukder, B., Blay-Palmer, A., Hipel, K. W., and vanLoon, G. W. (2017). Elimination method of multi-criteria decision analysis (MCDA): a simple methodological approach for assessing agricultural sustainability. Sustainability 9, 287. doi: 10.3390/su9020287
Thapa, R., Mirsky, S. B., and Tully, K. L. (2018). Cover crops reduce nitrate leaching in agroecosystems: A global meta-analysis. J. Environ. Qual. 47, 1400–1411. doi: 10.2134/jeq2018.03.0107
Thompson, N. M., Reeling, C. J., Fleckenstein, M. R., Prokopy, L. S., and Armstrong, S. D. (2021). Examining intensity of conservation practice adoption: evidence from cover crop use on US Midwest farms. Food Policy 101, 102054. doi: 10.1016/j.foodpol.2021.102054
Urban, D. W., Roberts, M. J., Schlenker, W., and Lobell, D. B. (2015). The effects of extremely wet planting conditions on maize and soybean yields. Clim. Change 130, 247–260. doi: 10.1007/s10584-015-1362-x
USDA National Agricultural Statistics Service (USDA NASS) (2018). National Crop Progress—Terms and Definitions. Available online at: https://www.nass.usda.gov/Publications/National_Crop_Progress/Terms_and_Definitions/index.php (accessed September 1, 2022).
USDA National Agricultural Statistics Service (USDA NASS) (2022). Quick Stats. Available online at: https://quickstats.nass.usda.gov (accessed September 1, 2022).
USDA National Agricultural Statistics Service Cropland Data Layer (USDA NASS CDL) (2021). Published Corn Data Layer. Washington, DC: USDA-NASS. Available online at: https://nassgeodata.gmu.edu/CropScape/ (accessed August 10, 2021).
USDA Risk Management Agency (USDA RMA) (2019). 2020 Cover Crops Insurance and NRCS Cover Crop Termination Guidelines. Available online at: https://www.rma.usda.gov/en/News-Room/Frequently-Asked-Questions/2020-Cover-Crops-Insurance-and-NRCS-Cover-Crop-Termination-Guidelines (accessed September 1, 2022).
Wilson, M. L., Allan, D. L., and Baker, J. M. (2014). Aerially seeding cover crops in the northern US Corn Belt: limitations, future research needs, and alternative practices. J. Soil Water Conserv. 69, 67A-72A. doi: 10.2489/jswc.69.3.67A
Wilson, M. L., Baker, J. M., and Allan, D. L. (2013). Factors affecting successful establishment of aerially seeded winter rye. Agron. J. 105, 1868–1877. doi: 10.2134/agronj2013.0133
Keywords: cover crop, soybean, risk, decision analysis (DA), Iowa (USA), maize (Zea mays L.)
Citation: Nichols GA and MacKenzie CA (2023) Identifying research priorities through decision analysis: A case study for cover crops. Front. Sustain. Food Syst. 7:1040927. doi: 10.3389/fsufs.2023.1040927
Received: 09 September 2022; Accepted: 27 February 2023;
Published: 23 March 2023.
Edited by:
Jacob Jungers, University of Minnesota Twin Cities, United StatesReviewed by:
Antonio DiTommaso, Cornell University, United StatesAtique ur Rehman, Bahauddin Zakariya University, Pakistan
Copyright © 2023 Nichols and MacKenzie. This is an open-access article distributed under the terms of the Creative Commons Attribution License (CC BY). The use, distribution or reproduction in other forums is permitted, provided the original author(s) and the copyright owner(s) are credited and that the original publication in this journal is cited, in accordance with accepted academic practice. No use, distribution or reproduction is permitted which does not comply with these terms.
*Correspondence: Cameron A. MacKenzie, Y2FtYWNrZW5AaWFzdGF0ZS5lZHU=