- 1Center of Agricultural and Agri-Food Biotechnology From Alentejo, Centro de Biotecnologia Agrícola e Agro-Alimentar do Alentejo (CEBAL), Beja, Portugal
- 2Unit of Microbiology, Department of Biology, Federal University of Lavras, Lavras, Brazil
- 3Unit of Agrotechnology, Department of Agriculture, Federal University of Lavras, Lavras, Brazil
- 4Department of Agriculture, São Paulo State University, Jaboticabal, Brazil
Introduction: The bad management of organic waste negatively affects environmental quality and composting has been a viable recycling alternative. Microorganisms are responsible for waste degradation during the composting process and, consequently, for transforming this waste into natural fertilizer. This work aimed to analyze and identify the biodiversity of yeasts and filamentous fungi throughout a composting process based on organic residues under different treatments (commercial inoculum, non-commercial inoculum, and control treatment) and to investigate the enzymatic activity of these microorganisms.
Methods: Microorganisms were isolated and identified from samples at 0, 5, 10, 20, 40, 60, and 120 days. Filamentous fungi were identified according to their macroscopic and microscopic characteristics, and yeasts were identified by sequencing the 18S rDNA region. All identified strains were evaluated for ligninolytic, cellulolytic, hemicellulolytic, amylolytic, pectinolytic, proteolytic, lipolytic, and ammonification. During the composting phases, the filamentous fungi were higher than the yeast population.
Results and discussion: At the beginning of the process, a higher species diversity was observed, and the population of yeasts and filamentous fungi was, on average, 6.50 log CFU g−1. The microbial communities were similar throughout the process in the two inoculated treatments, which showed more significant microbial activity, diversity, and efficiency in the transformation of organic matter, and consequently, advantages in terms of the final product quality compared to the control treatment. The yeasts Pichia kudriavzevii, Pichia farinosa, Issatchenkia orientalis, and the filamentous fungi of the genus Aspergillus spp. proved to have high biotechnological value and could be used as starter cultures to accelerate the composting process.
1. Introduction
In the last few decades, the generation of different types of waste has been growing at an accelerated and exponential rate, with an emphasis on solid organic waste, which for the most part is the result of the waste of restaurants, especially those located in large urban centers (Zhang and Sun, 2019). This disposal implies several problems both at a social level–related to public health and world hunger–and an environmental if done improperly (Huang et al., 2022).
The proper treatment and disposal of this waste are essential due to the environmental problems that it can generate (Palaniveloo et al., 2020). Numerous research and studies have been carried out worldwide, aiming to find new methods of treatment or disposal of this waste (Kim et al., 2021; Torrijos et al., 2021; Huang et al., 2022). The composting technologies have stood out as a highly viable and practical approach to treating organic waste since it is a low-cost process whose scope is environmental sustainability (Rempel et al., 2018; Huzairi et al., 2022). Composting involves the transformation of organic matter through the action of various microorganisms and results in natural fertilizers, closing a sustainable cycle (Zhang and Sun, 2019; Huang et al., 2022). Composting is a degradation process that, in addition to microbiological activity, it involves other parameters such as C:N ratio, aeration, and moisture, which determine the proper development of specific groups of microorganisms, which ensure the quality of compost (Chen et al., 2021; Wang et al., 2021). Therefore, everything must be harmonious and proportioned to produce efficient and quality fertilizer (Fan et al., 2018; Torrijos et al., 2021).
Composting occurs with natural microbiota, including bacteria, actinobacteria, yeasts, and filamentous fungi, which are usually involved in the degradation of primary components during aerobic composting (Huzairi et al., 2022). These microorganisms, including yeasts (and fungi), are beneficial in the outcome of compost (Kuznetsova et al., 2022) and knowledge of these at the species level becomes important, as many within these groups have a decisive impact on plant growth promotion and disease suppression (Corato, 2020). The diversity of the microorganisms present throughout the process interferes with compost quality (Palaniveloo et al., 2020; Kim et al., 2021). Therefore, it is essential to monitor and identify the microbial species present in the different phases related to temperature, namely: mesophilic (around 40°C), thermophilic (around 70°C), maturation (around 30°C), and cooling (>20°C) (López-González et al., 2013; Oviedo-Ocaña et al., 2019). The evolution of the microbial population is related to temperature variations and other physical-chemical and biological parameters, such as aeration, humidity, and C: N ratio (Bohacz, 2016; Puspitaloka et al., 2022). The addition of selected inoculants can contribute significantly to the improvement in the degradation of recalcitrant residues (Awasthi et al., 2020) and, consequently, reduce the final time with the acceleration of the process (Onwosi et al., 2017; Liu et al., 2018).
Microorganisms actively degrade residues (Bohacz, 2016; Zhao et al., 2022), and some genera of microorganisms are commonly found in various composting processes, such as Candida, Aspergillus, Bacillus, and Fusarium (Di Piazza et al., 2020; Du et al., 2021; Wang Y. et al., 2022). However, the microbial species changes due to several factors and depends on the material to be composted, process conditions, the use or not of starter cultures, and reactors (Niu et al., 2019). Thus, composting is considered an auspicious process at an environmental and biotechnological level since isolated and identified microorganisms can have industrial applications for use as inoculants and have valuable and interesting metabolites and enzymes (López-González et al., 2015a; Zhang and Sun, 2019).
This study aimed to analyze and identify the biodiversity of yeasts and filamentous fungi isolated during restaurant waste-based composting conducted with different treatments [commercial inoculum (CI), non-commercial inoculum (EM) and control treatment] and to investigate the enzymatic activity and biotechnological potential of these microorganisms.
2. Materials and methods
2.1. Composting and sampling
Composting was performed by mixing organic waste from the University Restaurant (UR) and gardening waste from the Federal University of Lavras–UFLA, Lavras, Minas Gerais, Brazil. The research was conducted from 2017 to 2018 in Lavras, Minas Gerais, Brazil.
The initial physicochemical characterization of both residues was carried out so that the initial C: N ratio was calculated (Table 1) and, consequently, the ideal proportion (30:1) of each residue to be composted to start the process in an ideal and satisfactory way (Pisa et al., 2020). The waste from the restaurant is composed of leftover rice, beans, vegetables, lettuce leaves, purple cabbage, carrots, and zucchini pieces, among others in smaller quantities. Residues from the landscaping management of the university campus such as leaves from the trees that are swept and residue from tree and lawn pruning were also used to balance de C: N ratio.
The experiment was carried out using two different inoculants: a non-commercial (EM) and a commercial (CI) biological product composed of Lactobacillus plantarum 104 CFU/mL, Saccharomyces cerevisiae 103 CFU/mL, sugarcane molasses and water. EM was prepared according to Sharma et al. (2017) by cooking 700 g of unsalted rice in distilled water as a non-specific substrate for capturing different microorganisms in native forests, rich in beneficial microorganisms. After cooking, the rice was distributed in small amounts in a disposable plastic container (500 g), covered with perforated plastic, and placed under the burlap in the forest. Five disposable plastic bottles were distributed in the forest in a radius of 20 meters., for the growth of natural microorganisms, for 15 days, where the temperature and humidity were on average 19°C and 80%, respectively. Then, the microorganisms were deposited in a 20-liter bottle, homogenized with 1 liter of sterile sugar cane juice as a carbon source, and later, the volume of the bottle was filled with distilled water in a ratio of 20:1. The bottle was stored in a ventilated and cool place for 20 days, a period sufficient for multiplication and fermentation of the natural microbiota (Kwon et al., 2011) to obtain the inoculum EM. We did not identify the microbiota present in the inoculum since there was no growth in the culture medium.
The commercial inoculum was prepared and activated according to the manufacturer's instructions technical sheet. The recommended amount of distilled water and commercial sugar was added to the product, and the fermentation took place for 20 days in a conditioned place at room temperature (21–26°C). The compost without the addition of inoculants was used as a control treatment.
The waste was crushed separately into small particles (1–2.5 cm) with the help of a shredder. After being crushed, the organic waste from the UR was added directly to the gardening waste in a proportion of 1:2.5, respectively. Then the wastes were mixed with the help of a tractor, resulting in a homogeneous mixture. The present work used a bioreactor (1 m3) designed with an automatic air injection system and built with masonry blocks (Supplementary Figure 1). After homogenization, microbial inoculation was applied once, at the beginning of the experiment. In each experimental unit, 5 liters of inoculum (EM or CI) and 15 liters of water were used and properly identified. In the control treatment, only water was added. In the control treatment, only water was added.
Samples were collected based on the changes in the composting phases, which were monitored by temperature measurements at intervals of 0, 5, 10, 20, 40, 60, and 120 days, as seen in Table 2. To ensure a representative sample, subsamples were taken from nine distinct locations of each composter, being 3 surface samples, 3 interior samples, and 3 depth samples. The subsamples were mixed in equal amounts for each treatment to obtain a final sample weight of ~500 grams.
2.2. Isolation and identification of yeasts and filamentous fungi
Twenty-five g of each sample was added to 225 ml of sterile peptone water on an orbital shaker at 120 revolutions per minute (rpm), for 30 min, at room temperature, on average of 25°C (López-González et al., 2013). The samples were mixed in a stomacher at an average speed of the 60's, and 10-fold dilutions were prepared. The microbial population was evaluated using four different culture media. YEPG (Yeast Extract-Peptone-Dextrose) and DRBC (Dichloran Rose Bengal Chloramphenicol) media at 28°C for 48 h for isolating yeasts (Nathaniel et al., 2020) and PDA (Potato Dextrose Agar) and DG18 (Dicloran Glycerol Agar) media at 25°C for 7 days for isolating filamentous fungi (Rempel et al., 2018).
For morphological analysis and grouping of filamentous fungi, the following macroscopic characteristics were observed: (1) color of the colony; (2) mycelium color; (3) color of the back of the petri dish, and (4) absence or presence of streaks. After grouping based on macroscopic characteristics, the fungi were identified based on microscopic characteristics, such as (1) septation of hyphae; (2) shape and size of vesicles and conidia, and (3) length and texture of the stipe observed on fresh slides stained with methylene blue and observed under a microscope. Subsequently, these fungi were picked on specific media according to the genera found. The fungi identified as belonging to the genus Cladosporium were picked in MEA medium (in g L-1: 20 g malt extract, 1 g bacteriological peptone, 20 g glucose, 20 g agar) and incubated at 25°C for 7 days. The fungi identified as the genera Aspergillus and Penicillium were picked in CYA medium (in g L-1: 1g K2HPO4, 10mL, Czapek concentrate, 5 g yeast extract, 1 mL metallic solution, 20 g agar) and incubated with MEA at 25°C and 37°C for 7 days.
2.3. Phenotypic identification of filamentous fungi
The identification based on the microscopic characteristics of the filamentous fungi of the Aspergillus, Penicillium, and Cladosporium species was based on the keys proposed by Klich (2002), Bensch et al. (2012), and Visagie et al. (2014), respectively, and the analyzes were conducted in the laboratory of mycology and mycotoxins of the Department of Food Science of UFLA.
After incubation, the isolates were further classified according to their macroscopic and microscopic characteristics. For taxonomic identification at the species level, identification keys for the genera Aspergillus and Penicillium and the descriptions of the genus Cladosporium by Bensch et al. (2012) were used.
2.4. Identification of yeasts by sequencing of the 18S rDNA region
For the extraction of yeast DNA, colonies were suspended in 500 μL of sterile ultrapure water, and the DNA was extracted using the QIAamp DNA Micro Kit (Qiagen, Germany). For amplification, PCR was performed in a final reaction volume of 25 μL, which contained: 17.2 μL of sterile MilliQ water, 5 μL of MasterMix 5X buffer (Promega), 0.5 μL of dNTP, 0.5 μL of each primer: ITS1 (5' TCCGTAGGTGAACCTGCGG 3') and ITS4 (5' TCCTCCGCTTATTGATATGC 3'), 0.1 μL Taq DNA polymerase (Invitrogen), 0.25 μL MgCl2 and 1 μL DNA. The thermal cycler was programmed for an initial denaturation of 5 min at 95°C, followed by 35 steps of denaturation at 95°C for 1 minute, primer annealing at 55°C for 1 min, and DNA strand extension at 72°C for 1 min. The final extension took place at 72°C for 7 min and the storage temperature was 4°C.
PCR products were analyzed for purity and subjected to denaturing gradient gel electrophoresis (DGGE) using the vertical DCode system (Bio-Rad). The PCR products were applied in 10 μL aliquots, added with 10 μL of running dye (2% bromophenol blue, 2% xylene cyanol, glycerol, and MilliQ water) on a polyacrylamide gel (8% w/v) in TAE buffer (0.5X) with denaturation gradient ranging from 40 to 60% (100% corresponding to 7 M urea and 40% (v/v) formamide). Electrophoresis was carried out at a constant voltage of 150 V for 4 hours, with a constant temperature of 60°C. After electrophoresis, the gels were stained with SYBR-Green I (Molecular Probes1:10,000 v/v), and the image was captured using a transilluminator. Sequencing was performed based on the DGGE bands, where the different and constant bands were excised with the aid of a sterile blade, and the DNA was eluted in sterile ultrapure water. The DNA was reamplified in PCR under the same conditions as above. The products of the second PCR were sequenced by Macrogen and the sequences obtained were aligned with the GenBank database, with the aid of the BLAST software (National Center for Biotechnology Information, Maryland, USA). Multiple sequence alignments were performed using the MEGA-X software with the Clustal-W package. The Hasegawa-Kisinho-Yano nucleotide substitution method was used with a gamma distribution. The maximum Likelihood Tree test and the bootstrap method with 500 bootstrap replications were used for the phylogenetic analysis.
2.5. Enzymatic activity analyses
A total of 318 yeast and 41 filamentous fungus isolates were used in the assay for the activity of eight different enzymes, specifically ligninolytic (Widiastuti and Wulaningtyas, 2008), cellulolytic and hemicellulolytic (Kasana et al., 2008), amylolytic (Rempel et al., 2018), and pectinolytic (Ferreira et al., 2013), proteolytic (Scatamburlo et al., 2015), lipolytic (Fuciños et al., 2005) and ammonification (Jana and Roy, 1985) activities. The tests were performed on Petri dishes with solid media containing the appropriate substrate for each enzyme. Each yeast isolate (incubated in YEPG medium at 28°C for 48 hours) was normalized to a concentration of 107 cells/mL in 3 mL of sterile saline (0.9% NaCl), according to the McFarland scale. Petri dishes for each enzyme to be evaluated were inoculated with 10 μL aliquots of the yeast cells at 107. For the filamentous fungi, 6-mm diameter disks from a colony grown for 3 days on MA medium were placed in the center of the Petri dishes. The inoculated media were then incubated at 28 and 25°C for the yeasts and filamentous fungi.
Iodine solution was used to confirm the cellulolytic, hemicellulolytic amylolytic, and the results were classified based on the presence or absence of a clear halo around the yeast and filamentous fungi colonies. Discoloration around the colonies in the presence of Azure-B was used for assessing ligninolytic activity. A solution of 1% hexadecyltrimethylammonium bromide was used for the pectinolytic activity. The lipolytic activity was evaluated by forming a clear, crystallized halo around the colonies, and ammonification activity was assessed based on the formation of a pink halo. Extracellular protease production (Strauss et al., 2001) was determined after 5 days at 30°C in a YPD medium by the presence of a clear zone around the colony.
The enzymatic index (EI) was determined using Equation 1 (Eq 1), which is the ratio of the mean diameter of the degradation halo to that of the colony (Hankin and Anagnostakis, 1975).
The isolates exhibiting the highest EI (>2.0) had the highest extracellular enzymatic activity (Oliveira et al., 2012).
2.6. Experimental design
The experimental design was completely randomized; the entire experiment was conducted in triplicate. The efficiency of the composting process was statistically proven and conducted according to an already published article with physicochemical data, by Gaspar et al. (2022).
3. Results and discussion
3.1. Population dynamics of yeast and filamentous fungi
The population of yeasts and filamentous fungi in the inoculum was evaluated before being added to the compost. The number of yeasts in the EM inoculum was approximately 5.53 log CFU g−1, smaller than the 8.09 log CFU g−1 population in the CI inoculum (Figure 1A). However, filamentous fungi were not observed in any inoculum evaluated (Figure 1B). The yeasts found in each inoculum were sequenced, identified, and presented in Table 3, together with their respective quantifications in each inoculum.
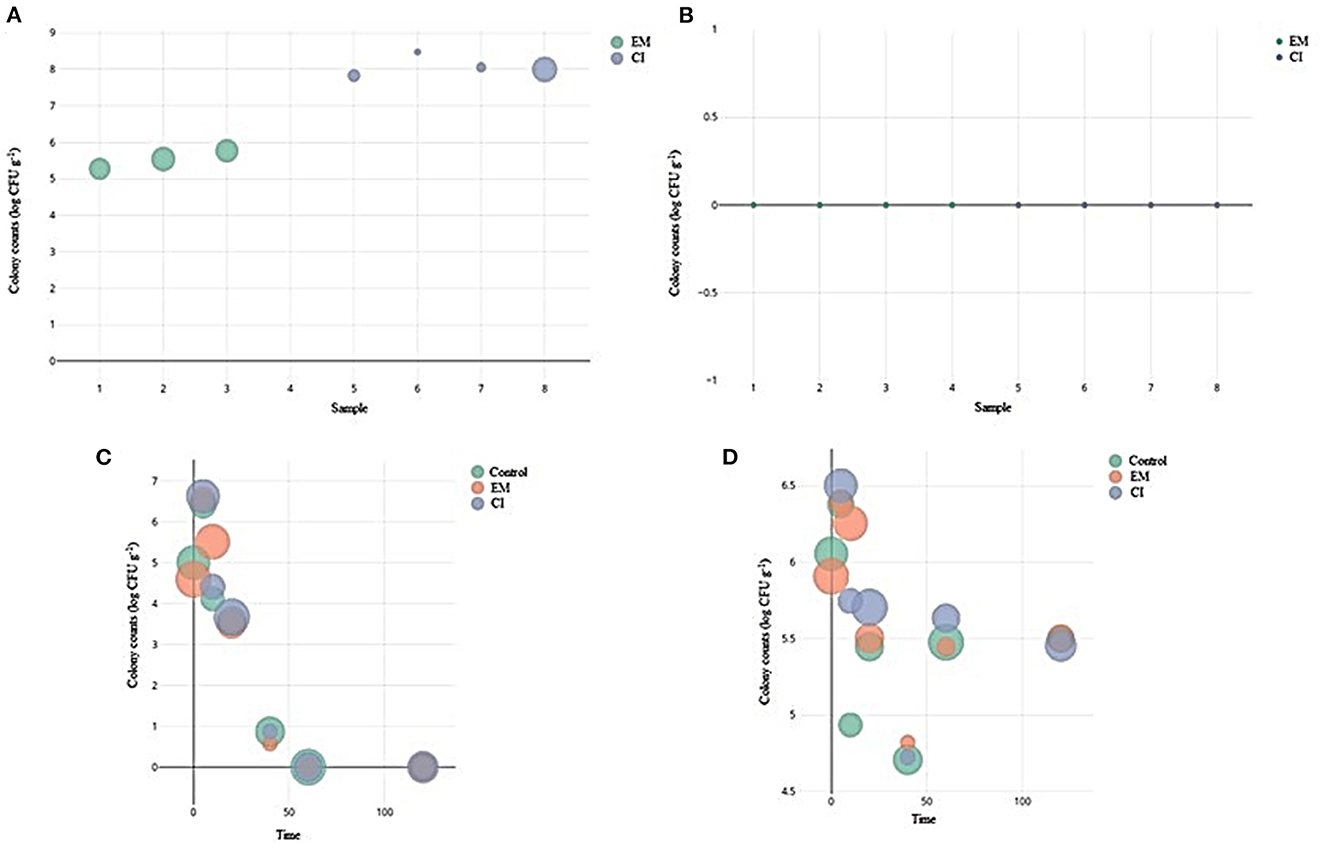
Figure 1. Colony counts (log CFU g−1) and relative abundance [(counts of isolates/total counts of groups) × 100] of the isolates present in each treatment throughout the composting process. Bubble sizes (diameters) represent relative abundance (%); a bubble representing the maximum abundance is included as a reference. Each chart shows the isolated population from each inoculum and the groups of microorganisms analyzed in each treatment throughout the composting process. (A) Yeast population in the inoculum; (B) Filamentous fungi population in the inoculum; (C) Yeast for the composting process; (D) Filamentous fungi for the composting process.
Monitoring the different microbial populations present throughout the composting process is extremely important to determine the rate of particle biodegradation and the quality of the final product (Jurado M. et al., 2014; Oviedo-Ocaña et al., 2019). After the addition of inoculants in the composters and over the time of the composting process, it was observed that the total yeast population decreased during the process and became null in the cooling and maturation phases, with the highest values of diversity and sizes obtained during the thermophilic phase (Table 5). In the thermophilic phase, the yeast population reached 6.4, 6.56, and 6.63 log CFU g−1 for the control, EM, and CI treatments, respectively (Figure 1C). Different yeast species are typically associated with the beginning of the composting process due to the environmental factors favorable to these microorganisms, such as the high availability of O2 and low pH (Langarica-Fuentes et al., 2014; Nakasaki and Hirai, 2017). The population of filamentous fungi was higher than the yeasts at the beginning of the process, mainly during the thermophilic phase. During this phase, a larger filamentous fungi population with 6.38, 6.39, and 6.50 log CFU g−1 in control, EM, and CI treatments, respectively (Figure 1D), may be present because these microorganisms produce spores, and consequently are more resistant to high temperatures (Nakasaki and Hirai, 2017; Wang Y. et al., 2022). During the cooling and maturation phases, the population of filamentous fungi increased and remained constant, which may result from favorable environmental factors and waste degradation (Meng et al., 2018; Huzairi et al., 2022).
3.2. Population dynamics of microorganisms
Several yeasts and filamentous fungi were identified throughout the composting process in all treatments and are shown in Table 4 and Figure 2.
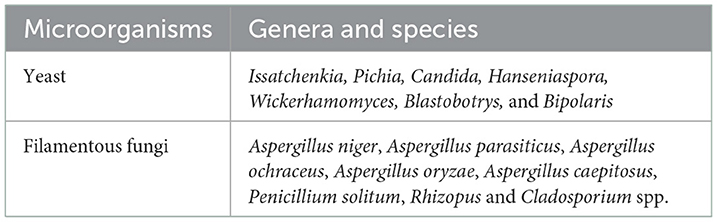
Table 4. Genera and species of microorganisms identified in the composting process in both treatments during the composting process.
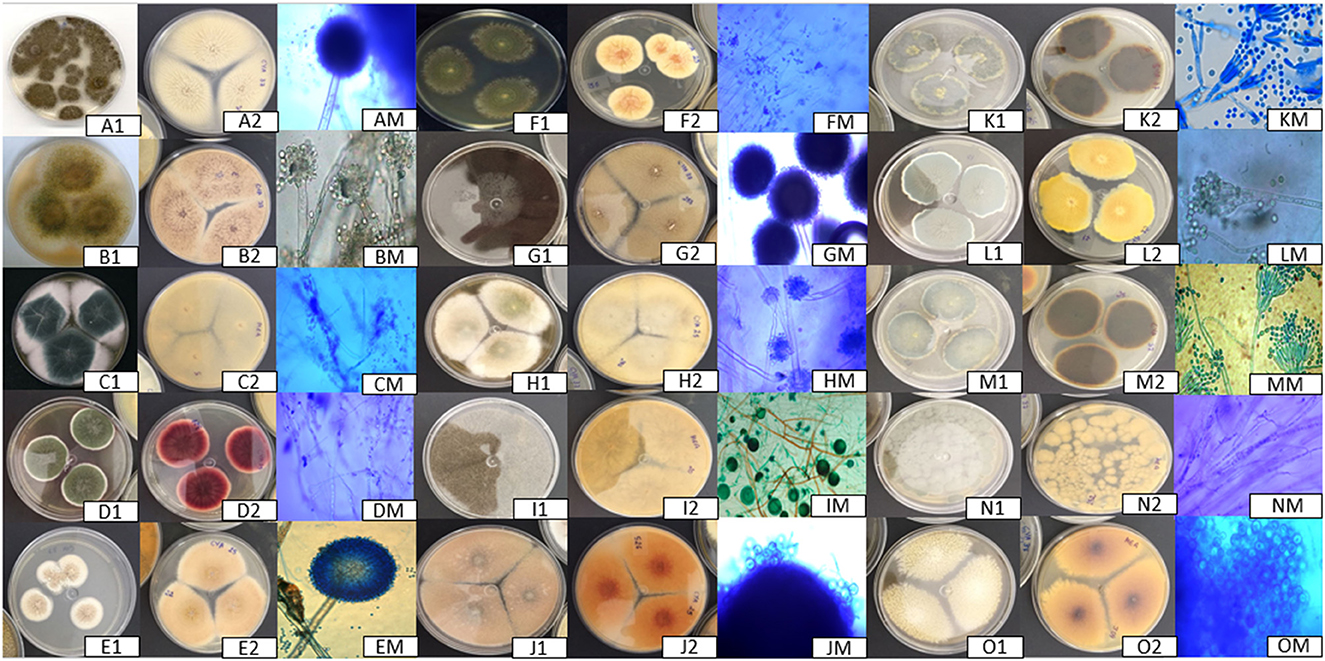
Figure 2. Photographs of isolated filamentous fungi. 1, Front view of the colony; 2, Back view of the colony; M, Microscopy image. A, Aspergillus tubigiensis; B, Aspergillus oryzae; C, Aspergillus fumigatus; D, Aspergillus sp.; E, Aspergillus ochraceus; F, Aspergillus caepitosus; G, Aspergillus niger; H, Aspergillus parasiticus; I, Rhizopus; J, Emericella sp.; K, Penicillium citrinum; L, Penicillium solitum; M, Penicillium implicatum; N, Penicillium sp.; O, Cladosporium.
At the beginning of the composting process, a substantial number of isolates and species diversity were observed in both yeast and filamentous fungi in the CI treatment. However, throughout the process, this number was higher and constant in the EM treatment, showing higher microbial activity and efficiency in the transformation of organic matter in the EM and IC treatments compared to the control treatment (Figure 3).
Inoculation of compost with starter cultures has several benefits related to microbial diversity, a reduction in composting time, and the highest and fastest rate of particle degradation (Manu et al., 2017; Jia et al., 2021; Huzairi et al., 2022). Yeasts perform essential roles in the composting process, P. kudriavzevii, for example, acts on the degradation of organic acids, which consequently causes an increase in pH and promotes bacterial growth that contributes significantly to the waste degradation (Ngoc et al., 2015). In general, filamentous fungi are considered fundamental in the degradation of recalcitrant fractions of agricultural residues to be composted (Sundberg et al., 2011; Huzairi et al., 2022).
Although some studies have shown that microbial inoculation can improve composting, mainly by reducing the processing time, increasing the enzymatic activity, and several other factors can affect the process (Gou et al., 2017; Singh et al., 2021), some microorganisms naturally present in the initial residues can be competitive or resistant to different environmental changes (López-González et al., 2015b; Huzairi et al., 2022). Nakasaki and Hirai (2017) observed that, despite stimulating the naturally occurring microbiota, the use of P. kudriavzevii as an organic waste compost inoculant did not positively influence the quality of the final compost.
3.3. Identification of yeast and filamentous fungi species
The criteria used to select for persistent microorganisms in each treatment was the detection of the same specie at 2 or more sampling times during the composting process because it shows that he appeared more than once and in different phases. Table 5 shows the yeast and filamentous fungi species identified from each treatment.
Among the yeast species isolated from the compound inoculated with efficient microorganisms (EM) and commercial inoculum (CI), the genus Pichia corresponded to 66.6 and 40%, respectively. While the genera Candida and Blastobotrys were exclusive to treatments inoculated with EM, with 25.9 and 7.5% respectively. On the other hand, the genera Hanseniaspora and Wickerhanomyces corresponded to 33.3 and 26% of the compost with commercial inoculum (CI). Among the species of filamentous fungi, those of the genus Aspergillus were the most frequent, also occurring in the presence of the genera Penicillium, Rhizopus, and Cladosporium. In the control compost, the appearance of yeasts I. orientalis and P. kudriavzevii was constant between the composting phases, as well as filamentous fungi of the genus Aspergillus and Penicillium.
Each composting process has unique characteristics (Niu et al., 2019), and some yeast species, such as Blastobotrys adeninivorans, Blastobotrys raffinosifermentans, and Bipolaris sorokiniana, have not been previously reported in other composting processes. As an organic compost, several microorganisms, even phytopathogenic organisms, may be present in the early stages, such as B. sorokiniana (Song et al., 2018; Puspitaloka et al., 2022). However, this yeast was found only in the control treatment and during the thermophilic phase (Table 5), after which it was eliminated in the final composting stages.
The identified filamentous fungi mainly represented the Aspergillus, were present in both treatments and predominated at all sampling times (Table 5). This genus of filamentous fungi produces spores that are resistant to high temperatures (Wang S.-K. et al., 2022), and although it was widely detected throughout the composting process, it may not have participated in all stages since what could be present were the spores. In a previous study on composting organic waste, species of the genus Aspergillus were also detected (Zhao et al., 2017; Roca-Barcelo et al., 2020). Aspergillus and Penicillium species were detected during the composting of wheat straw for mushroom production (Zhang et al., 2014). Penicillium species were detected in composting from white wine distillers' grains (Yu et al., 2021). In composting organic waste, Di Piazza et al. (2020) reported having identified species of Penicillium spp., Rhizopus spp., and Aspergillus spp. Different fungi species have been isolated from different composting methods, including A. fumigatus, Chaetomonium thermophilum, Humicola grisea, Humicola insolens, Mucor pusilus, Talaromyces duponti, Scytalidium thermophilium (Yim et al., 2019). Species of the genus Rhizopus spp. were also reported by Huzairi et al. (2022). Additionally, filamentous fungi belonging to the genus Aspergillus have been detected during composting with bovine manure and construction wastes (Holman et al., 2016).
The genera Candida and the species A. fumigatus have also been detected during lignocellulosic material compost (Silva et al., 2009; Jurado M. et al., 2014). P. farinosa was the most representative yeast, as it was identified during the thermophilic phase of all the treatments (Table 5) and in the EM inoculum. When analyzing the lignocellulosic composting process, López-González et al. (2015a) identified the yeast species Candida railenenses, Candida sequanensis, Pichia fermentans and the filamentous fungi species A. fumigatus, P. solitum, and P. citrinum during the early composting phases.
In metaproteomic studies with two types of raw materials prepared by mixing commercial rabbit food with cooked rice at ratios of 7:3 and 5:5, the most abundant genera were Saccharomyces, Candida, and Schizosaccharomyces; species of the genera Kluyveromyces, Pichia, and Aspergillus were also detected (Nakasaki and Hirai, 2017).
Alternaria, Aspergillus, Candida, Cladospodium, Fusarium, Gibellulopsis, Ochrocladosporium, Penicillium, Plectosphaerella, and Scopulariopsis were also isolated previously by Jurado M. et al. (2014).
3.4. Characterization of the enzymatic activity of the identified organisms
Microbial enzymes are essential in the composting process (Huzairi et al., 2022), and according to Di Piazza et al. (2020), the mesophilic phase of the composting process is characterized by the most intense metabolic activity and the highest enzyme synthesis.
All microorganisms in the study were tested for enzyme activities and those with EI >2.0 were described and correlated with the taxonomy in Table 5.
The isolated yeasts showed a higher diversity of enzymatic activity compared to filamentous fungi among the inoculated treatments during the initial 40 days of the composting process. Among the yeasts, 7.84% have the ligninolytic capacity, 5.88% cellulolytic, 11.80% hemicellulolytic, 9.80% amylolytic, 5.88% proteolytic, 35.3% lipolytic, and 23.50% ammonification capacity.
At the end of composting, filamentous fungi predominated and yeasts were found to be absent in the culture medium. None of the isolated filamentous fungi exhibited the lipolytic, hemicellulolytic, amylolytic, or lipolytic activities tested. The filamentous fungi showed 33.0% cellulolytic, 31.90% pectinolytic, 28.60% ammonification, and 6.50% proteolytic capacity.
Many species showed some enzymatic activity, but only a few were potential enzyme producers, such as yeast species of the Pichia and Issatchenkia genera, with an enzymatic index of lignolytic, cellulolytic and proteolytic activities >4.0, and filamentous fungi of the genus Aspergillus, with an enzymatic index for cellulolytic activity reaching 5.0. Among isolates of the same species, differences in enzyme production were found, showing different biotechnological potentials and differences in the same species. The isolates with high potential for enzyme production (i.e., EI > 2.0) are presented in Table 6. Issatchenkia orientalis was the yeast species with the highest enzymatic potential for ligninolytic activity (EI = 4.2). Regarding the cellulolytic and amylolytic activities, C. tropicalis stood out, with EIs of 4.7 and 2.8, respectively. P. farinosa presented EIs of 2.0, 4.5, and 3.3 for hemicellulolytic, proteolytic, and lipolytic activities, respectively. Aspergillus spp. presented high enzymatic potentials for cellulolytic activity with EIs = 5.0. Thus, the results obtained allow us to identify the I. orientalis, C. tropicalis, P. farinosa, and Aspergillus spp. isolates as promising enzyme producers (Table 7).
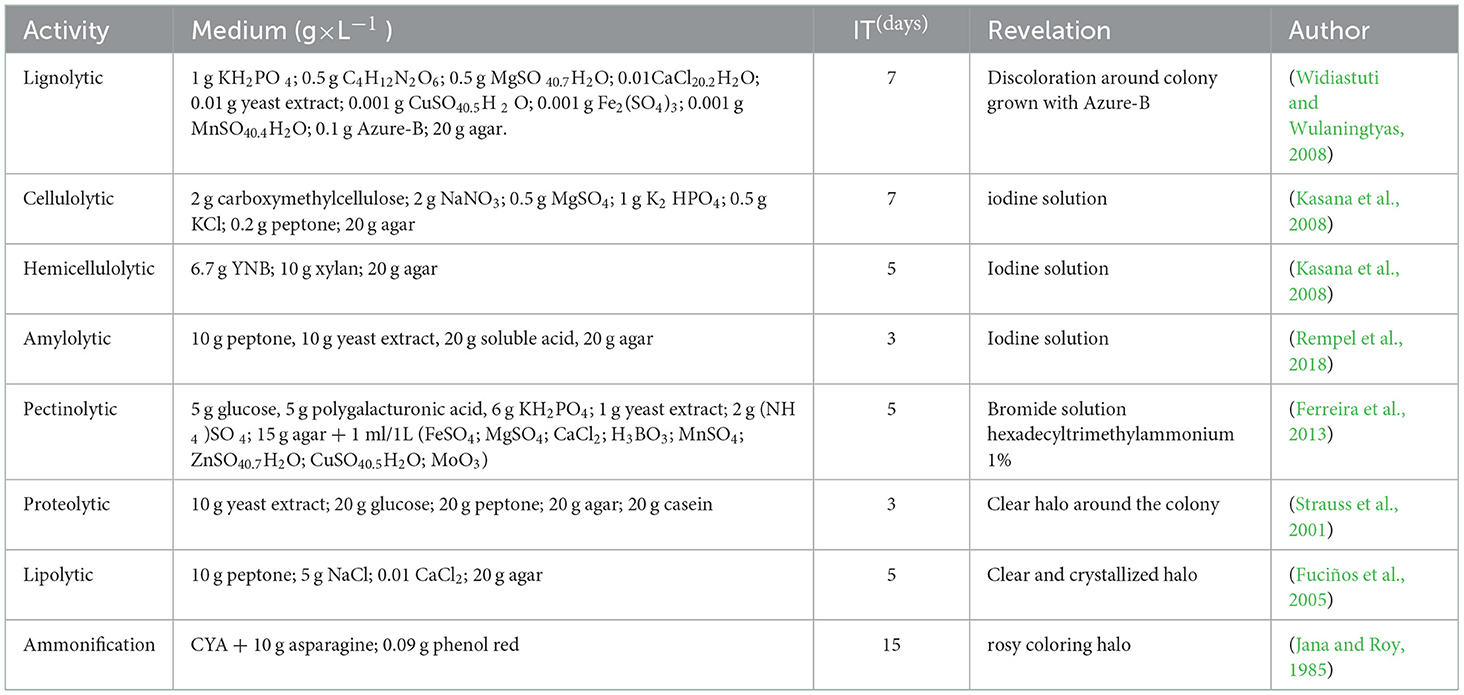
Table 6. Enzymatic activities and their respective culture media, incubation times and development mode.
In composting, enzymes are important because the biotransformation of organic materials occurs under the action of the enzymatic activity of the microorganisms that can improve and accelerate the degradation processes, directly reflecting on the final product (Zhang and Sun, 2016; Chen et al., 2021). Applying compost with a microorganism with good enzymatic activity can help improve soil fertility (Huzairi et al., 2022). In this sense, the presence of enzyme-producing microorganisms in composting is essential for the degradation of solid waste and, subsequently, when applied in the form of compost, for maintaining soil dynamics and in cycles of nitrogen, carbon, or phosphorus (Deus et al., 2017; Zhang et al., 2021). I. orientalis strains can hydrolyze phytates in the soil, which improves the absorption of phosphorus by plants and, consequently, reduces the concentration of fertilizers added to the soil (Mezeli et al., 2017). Species of the Aspergillus genus can degrade residual herbicides that are extremely dangerous to the environment (Sondhia et al., 2016).
Composting has stood out among the most viable alternatives for the use of waste because it is a sustainable technology that adds value to organic waste (Zhang et al., 2021). Besides, the final product allows for the recycling of organic waste—however, the type of waste in composting changes the microbiota present in each process (Bohacz, 2016; Torrijos et al., 2021; Huzairi et al., 2022).
In general, isolates that present some biotechnologically relevant enzymatic activity has a range of metabolic activities and physiological capabilities (i.e., such as the production of enzymes and improvements in the composting process, including shorter processing time) that make them potentially suitable for a wide variety of applications (Jurado M. M. et al., 2014; Sajid et al., 2022).
4. Conclusions
Several species of yeasts and filamentous fungi act in the process of organic waste degradation, and the use of inoculants during the composting process directly affects microbiological diversity. The microbial communities were similar throughout the process in the two inoculated treatments, which showed more significant microbial activity, diversity, and efficiency in the transformation of organic matter, and consequently, advantages in terms of the final product quality compared to the control treatment.
The microorganisms with the highest enzymatic indices were Issatchenkia orientalis, Candida tropicalis, Pichia farinosa, and Aspergillus spp. The yeast species Pichia kudriavzevii, Pichia farinosa, Issatchenkia orientalis, and the filamentous fungi of the genus Aspergillus spp., proved to be persistent and with enzymatic activities of high biotechnological value. Although each restaurant has varied organic residues, the good values obtained in this study show that these results can be extended to other contexts. For example, the persistent species with high enzymatic indexes identified in this study could be cultivated in the laboratory to formulate a possible cocktail to be used as an inoculant and accelerator of the composting process, with initial populations of 9.0 log UFC g-1 for process efficiency and viability.
In general, yeast and filamentous fungi isolates exhibit metabolic capabilities that can have biotechnological applications, and this study reaffirms the potential of composting as a source of biotechnologically relevant microorganisms.
Data availability statement
The datasets presented in this study can be found in online repositories. The names of the repository/repositories and accession number(s) can be found in the article/Supplementary material.
Author contributions
SS: conceptualization, data curation, investigation, methodology, project administration, validation, visualization, roles, and writing-original draft. LA: conceptualization, investigation, and methodology. MPe: formal analysis and methodology. MPr: investigation. VB and GM: data curation, software, and writing-review and editing. VB, RS, and MPa: conceptualization, formal analysis, funding acquisition, methodology, project administration, resources, supervision, validation, visualization, roles, writing-original draft, and writing-review and editing. JD, MPa, ER, and RC: conceptualization, funding acquisition, methodology, project administration, resources, and supervision. All authors contributed to the article and approved the submitted version.
Funding
The authors thank Capes for financial support with grant number 320040100114P, CNPq, and FAPEMIG for financial support; to the Federal University of Lavras (UFLA) for the structure provided to the accomplishment of the experiments.
Acknowledgments
We would like to thank the reviewers for their helpful and constructive comments on the earlier version of our manuscript.
Conflict of interest
The authors declare that the research was conducted in the absence of any commercial or financial relationships that could be construed as a potential conflict of interest.
Publisher's note
All claims expressed in this article are solely those of the authors and do not necessarily represent those of their affiliated organizations, or those of the publisher, the editors and the reviewers. Any product that may be evaluated in this article, or claim that may be made by its manufacturer, is not guaranteed or endorsed by the publisher.
Supplementary material
The Supplementary Material for this article can be found online at: https://www.frontiersin.org/articles/10.3389/fsufs.2023.1013361/full#supplementary-material
Supplementary Figure 1. (A–C) Graphical representations of masonry composers. (D) Installation of the experiment. Source (A–C): Passos (2014).
References
Awasthi, S. K., Sarsaiya, S., Awasthi, M. K., Liu, T., Zhao, J., Kumar, S., et al. (2020). Changes in global trends in food waste composting: research challenges and opportunities. Bioresour. Technol. 299, 122555. doi: 10.1016/j.biortech.2019.122555
Bensch, K., Braun, U., Groenewald, J. Z., and Crous, P. W. (2012). The genus Cladosporium. Stud. Mycol. 72, 1–401. doi: 10.3114/sim0003
Bohacz, J. (2016). Lignocellulose-degrading enzymes, free-radical transformations during composting of lignocellulosic waste and biothermal phases in small-scale reactors. Sci. Total Environ. 580, 744–754. doi: 10.1016/j.scitotenv.2016.12.021
Chen, Z., Li, Y., Peng, Y., Ye, C., and Zhang, S. (2021). Effects of antibiotics on hydrolase activity and structure of microbial community during aerobic co-composting of food waste with sewage sludge. Bioresour. Technol. 321, 124506. doi: 10.1016/j.biortech.2020.124506
Corato, U. (2020). Agricultural waste recycling in horticultural intensive farming systems by on-farm composting and compost-based tea application improves soil quality and plant health: a review under the perspective of a circular economy. Sci. Total Environ. 738, 139840. doi: 10.1016/j.scitotenv.2020.139840
Deus, R. M., Battistelle, R. A. G., and Silva, G. H. R. (2017). Scenario evaluation for the management of household solid waste in small Brazilian municipalities. Clean Technol. Environ. Policy 19, 205–214. doi: 10.1007/s10098-016-1205-0
Di Piazza, S., Houbraken, J., Meijer, M., Cecchi, G., Kraak, B., Rosa, E., et al. (2020). Thermotolerant and thermophilic mycobiota in different steps of compost maturation. Microorganisms 8, 1–9. doi: 10.3390/microorganisms8060880
Du, X., Li, B., Chen, K., Zhao, C., Xu, L., Yang, Z., et al. (2021). Rice straw addition and biological inoculation promote the maturation of aerobic compost of rice straw biogas residue. Biomass Convers. Biorefin. 11, 1885–1896. doi: 10.1007/s13399-019-00587-y
Fan, Y. V., Lee, C. T., Rí, J., Kleme, S. D. J, Chua, L. S., et al. (2018). Evaluation of effective microorganisms on home scale organic waste composting. J. Environ. Manage. 216, 41–48. doi: 10.1016/j.jenvman.2017.04.019
Ferreira, C., Danielle, S., Vilela, M., Cordeiro, C. S., Ferreira, W., Disney, D., et al. (2013). Evaluation of a potential starter culture for enhance quality of coffee fermentation. World J. Microbiol. Biotechnol. 29, 235–247. doi: 10.1007/s11274-012-1175-2
Fuciños, P., Abadín, C. M., Sanromán, A., Longo, M. A., Pastrana, L., and Rúa, M. L. (2005). Identification of extracellular lipases/esterases produced by Thermus thermophilus HB27: partial purification and preliminary biochemical characterisation. J. Biotechnol. 117, 233–241. doi: 10.1016/j.jbiotec.2005.01.019
Gaspar, S. S., Assis, L. L. R., Carvalho, C. A., Buttrós, V. H., Ferreira, G. M., Schwan, F., et al. (2022). Dynamics of microbiota and physicochemical characterization of food waste in a new type of composter. Front. Sustain. Food Syst. 6, 960196. doi: 10.3389/fsufs.2022.960196
Gou, C., Wang, Y., Zhang, X., Lou, Y., and Gao, Y. (2017). Inoculation with a psychrotrophic-thermophilic complex microbial agent accelerates onset and promotes maturity of dairy manure-rice straw composting under cold climate conditions. Bioresour. Technol. 243, 339–346. doi: 10.1016/j.biortech.2017.06.097
Hankin, L., and Anagnostakis, S. L. (1975). The use of solid media for detection of enzyme production by fungi. Mycologia 67, 597–607. doi: 10.1080/00275514.1975.12019782
Holman, D. B., Hao, X., Topp, E., Yang, H. E., and Alexander, T. W. (2016). Effect of co-composting cattle manure with construction and demolition waste on the archaeal, bacterial, and fungal microbiota, and on antimicrobial resistance determinants. PLoS ONE 11, 1–21. doi: 10.1371/journal.pone.0157539
Huang, T., Liu, X., and Zong, Y. (2022). Identification of microbial flora and isolation of functional strains related to the degradation of domestic waste. bioRxiv [Preprint]. doi: 10.1101/2022.03.20.485016
Huzairi, M., Zainudin, M., Zulkarnain, A., Azmi, A. S., Muniandy, S., Sakai, K., et al. (2022). Enhancement of agro-industrial waste composting process via the microbial inoculation: a brief review. Agronomy 12, 198. doi: 10.3390/agronomy12010198
Jana, B. B., and Roy, S. K. (1985). Distribution patterns of protein mineralizing and ammonifying bacterial populations in fish-farming ponds under different management systems. Aquaculture 44, 57–65. doi: 10.1016/0044-8486(85)90042-0
Jia, X., Qin, X., Tian, X., Zhao, Y., Yang, T., and Huang, J. (2021). Inoculating with the microbial agents to start up the aerobic composting of mushroom residue and wood chips at low temperature. J. Environ. Chem. Eng.9, 105294. doi: 10.1016/j.jece.2021.105294
Jurado, M., López, M. J., Suárez-Estrella, F., Vargas-García, M. C., López-González, J. A., and Moreno, J. (2014). Exploiting composting biodiversity: Study of the persistent and biotechnologically relevant microorganisms from lignocellulose-based composting. Bioresour. Technol. 162, 283–293. doi: 10.1016/j.biortech.2014.03.145
Jurado, M. M., Suárez-Estrella, F., Vargas-García, M. C., López, M. J., López-González, J. A., and Moreno, J. (2014). Evolution of enzymatic activities and carbon fractions throughout composting of plant waste. J. Environ. Manage. 133, 355–364. doi: 10.1016/j.jenvman.2013.12.020
Kasana, R. C., Salwan, R., Dhar, H., Dutt, S., and Gulati, A. (2008). A rapid and easy method for the detection of microbial cellulases on agar plates using gram's iodine. Curr. Microbiol. 57, 503–507. doi: 10.1007/s00284-008-9276-8
Kim, A. L., Park, S., Hong, Y. K., Shin, J. H., and Joo, S. H. (2021). Isolation and characterization of beneficial bacteria from food process wastes. Microorganisms 9, 1156. doi: 10.3390/microorganisms9061156
Kuznetsova, T. A., Vecherskii, M. V., Khayrullin, D. R., Stepankov, A. A., Maximova, I. A., and Kachalkin, A. V. (2022), Dramatic effect of black soldier fly larvae on fungal community in a compost. J. Sci. Food Agric. 102, 2598–2603. doi: 10.1002/jsfa.11601.
Kwon, Y. J., Ma, A. Z., Li, Q., Wang, F., Zhuang, G. Q., and Liu, C. Z. (2011). Effect of lignocellulosic inhibitory compounds on growth and ethanol fermentation of newly-isolated thermotolerant Issatchenkia orientalis. Bioresour. Technol. 102, 8099–8104. doi: 10.1016/j.biortech.2011.06.035
Langarica-Fuentes, A., Zafar, U., Heyworth, A., Brown, T., Fox, G., and Robson, G. D. (2014). Fungal succession in an in-vessel composting system characterized using 454 pyrosequencing. FEMS Microbiol. Ecol. 88, 296–308. doi: 10.1111/1574-6941.12293
Liu, L., Wang, S., Guo, X., Zhao, T., and Zhang, B. (2018). Succession and diversity of microorganisms and their association with physicochemical properties during green waste thermophilic composting. Waste Manag. 73, 101–112. doi: 10.1016/j.wasman.2017.12.026
López-González, J. A., Del, M., Vargas-García, C., López, M. J., Suárez-Estrella, F., Del Mar Jurado, M., et al. (2015a). Biodiversity and succession of mycobiota associated to agricultural lignocellulosic waste-based composting. Bioresour. Technol. 187, 305–313. doi: 10.1016/j.biortech.2015.03.124
López-González, J. A., Lopez, M. J., Vargas-Garcia, M. C., Suarez-Estrella, F., Jurado, M., and Moreno, J. (2013). Tracking organic matter and microbiota dynamics during the stages of lignocellulosic waste composting. Bioresour. Technol. 146, 574–584. doi: 10.1016/j.biortech.2013.07.122
López-González, J. A., Suárez-Estrella, F., Vargas-García, M. C., López, M. J., Jurado, M. M., and Moreno, J. (2015b). Dynamics of bacterial microbiota during lignocellulosic waste composting: studies upon its structure, functionality and biodiversity. Bioresour. Technol. 175, 406–416. doi: 10.1016/j.biortech.2014.10.123
Manu, M. K., Kumar, R., and Garg, A. (2017). Performance assessment of improved composting system for food waste with varying aeration and use of microbial inoculum. Bioresour. Technol. 234, 167–177. doi: 10.1016/j.biortech.2017.03.023
Meng, X., Liu, B., Xi, C., Luo, X., Yuan, X., Wang, X., et al. (2018). Effect of pig manure on the chemical composition and microbial diversity during co-composting with spent mushroom substrate and rice husks. Bioresour. Technol. 251, 22–30. doi: 10.1016/j.biortech.2017.09.077
Mezeli, M. M., Menezes-blackburn, D., George, T. S., Giles, C. D., Neilson, R., and Haygarth, P. M. (2017). Geoderma Effect of citrate on Aspergillus niger phytase adsorption and catalytic activity in soil. Geoderma 305, 346–353. doi: 10.1016/j.geoderma.2017.06.015
Nakasaki, K., and Hirai, H. (2017). Temperature control strategy to enhance the activity of yeast inoculated into compost raw material for accelerated composting. Waste Manag. 65, 29–36. doi: 10.1016/j.wasman.2017.04.019
Nathaniel, O., Sam, A. R. M., Lim, N. H. A. S., Adebisi, O., and Abdulkareem, M. (2020). Biogenic approach for concrete durability and sustainability using effective microorganisms: a review. Constr. Build. Mater. 261, 119664. doi: 10.1016/j.conbuildmat.2020.119664
Ngoc, Q., Tran, M., Mimoto, H., and Nakasaki, K. (2015). Inoculation of lactic acid bacterium accelerates organic matter degradation during composting. Int. Biodeterior. Biodegradation 104, 377–383. doi: 10.1016/j.ibiod.2015.07.007
Niu, F.-X., Huang, Y.-B., Ji, L.-N., and Liu, J.-Z. (2019). Genomic and transcriptional changes in response to pinene tolerance and overproduction in evolved Escherichia coli. Synth. Syst. Biotechnol. 4, 113–119. doi: 10.1016/j.synbio.2019.05.001
Oliveira, D. L., Wilbey, R. A., Grandison, A. S., Duarte, L. C., and Roseiro, L. B. (2012). Separation of oligosaccharides from caprine milk whey, prior to prebiotic evaluation. Int. Dairy J. 24, 102–106. doi: 10.1016/j.idairyj.2011.12.012
Onwosi, C. O., Igbokwe, V. C., Odimba, J. N., Eke, I. E., Nwankwoala, M. O., Iroh, I. N., et al. (2017). Composting technology in waste stabilization: on the methods, challenges and future prospects. J. Environ. Manage. 190, 140–157. doi: 10.1016/j.jenvman.2016.12.051
Oviedo-Ocaña, E. R., Dominguez, I., Komilis, D., and Sánchez, A. (2019). Co-composting of green waste mixed with unprocessed and processed food waste: influence on the composting process and product quality. Waste Biomass Valorization 10, 63–74. doi: 10.1007/s12649-017-0047-2
Palaniveloo, K., Amran, M. A., Norhashim, N. A., Mohamad-Fauzi, N., Peng-Hui, F., Hui-Wen, L., et al. (2020). Food waste composting and microbial community structure profiling. Processes 8, 1–30. doi: 10.3390/pr8060723
Passos, L. C. (2014). Methods of Composting Aeration Using Co-Products from Generation of Oil (Bachelor's Thesis). UFLA, Lavras, Brazil. p. 47.
Pisa, C., Wuta, M., and Muchaonyerwa, P. (2020). Effects of incorporation of vermiculite on carbon and nitrogen retention and concentration of other nutrients during composting of cattle manure. Bioresour. Technol. Rep. 9, 100383. doi: 10.1016/j.biteb.2020.100383
Puspitaloka, H., Mimoto, H., Tran, Q. N. M., Koyama, M., and Nakasaki, K. (2022). Effect of aeration methods on the organic matter degradation, microbial community and their catabolic function during composting. Waste Biomass Valorization 13, 1195–1205. doi: 10.1007/s12649-021-01560-5
Rempel, A., Machado, T., Treichel, H., Colla, E., Margarites, A. C., and Colla, M. (2018). Saccharification of Spirulina platensis biomass using free and immobilized amylolytic enzymes. Bioresour. Technol. 263, 163–171. doi: 10.1016/j.biortech.2018.04.114
Roca-Barcelo, A., Douglas, P., Fecht, D., Sterrantino, A. F., Williams, B., Blangiardo, M., et al. (2020). Risk of respiratory hospital admission associated with modelled concentrations of Aspergillus fumigatus from composting facilities in England. Environ. Res. 183, 108949. doi: 10.1016/j.envres.2019.108949
Sajid, S., Kudakwashe Zveushe, O., Resco de Dios, V., Nabi, F., Lee, Y. K., Kaleri, A. R., et al. (2022). Pretreatment of rice straw by newly isolated fungal consortium enhanced lignocellulose degradation and humification during composting. Bioresour. Technol. 354, 127150. doi: 10.1016/j.biortech.2022.127150
Scatamburlo, T. M., Yamazi, A. K., Cavicchioli, V. Q., Pieri, F. A., and Nero, L. A. (2015). Spoilage potential of Pseudomonas species isolated from goat milk. J. Dairy Sci. 98, 759–764. doi: 10.3168/jds.2014-8747
Sharma, A., Saha, T. N., Arora, A., Shah, R., and Nain, L. (2017). Efficient microorganism compost benefits plant growth and improves soil health in calendula and marigold. Hortic. Plant J. 3, 67–72. doi: 10.1016/j.hpj.2017.07.003
Silva, C. F., Azevedo, R. S., Braga, C., Silva, R., and Dias, E. S. (2009). Microbial diversity in a bagasse-based compost prepared for the production of Agaricus brasiliensis. Braz. J. Microbiol. 590–600. doi: 10.1590/S1517-83822009000300023
Singh, R., Das, R., Sangwan, S., Rohatgi, B., Khanam, R., Peera, S. K. P. G., et al. (2021). Utilisation of agro-industrial waste for sustainable green production: a review. Environ. Sustain. 4:619–636. doi: 10.1007/s42398-021-00200-x
Sondhia, S., Rajput, S., Varma, R. K., and Kumar, A. (2016). Biodegradation of the herbicide penoxsulam (triazolopyrimidine sulphonamide) by fungal strains of Aspergillus in soil. Appl. Soil Ecol. 105, 196–206. doi: 10.1016/j.apsoil.2016.03.010
Song, C., Li, M., Qi, H., Zhang, Y., Liu, D., Xia, X., et al. (2018). Impact of anti-acidification microbial consortium on carbohydrate metabolism of key microbes during food waste composting. Bioresour. Technol. 259, 1–9. doi: 10.1016/j.biortech.2018.03.022
Strauss, M. L. A., Jolly, N. P., Lambrechts, M. G., and Van Rensburg, P. (2001). Screening for the production of extracellular hydrolytic enzymes by non- Saccharomyces wine yeasts. J. Appl. Microbiol. 91, 182–190. doi: 10.1046/j.1365-2672.2001.01379.x
Sundberg, C., Franke-Whittle, I. H., Kauppi, S., Yu, D., Romantschuk, M., Insam, H., et al. (2011). Characterisation of source-separated household waste intended for composting. Bioresour. Technol. 102, 2859–2867. doi: 10.1016/j.biortech.2010.10.075
Torrijos, V., Calvo Dopico, D., and Soto, M. (2021). Integration of food waste composting and vegetable gardens in a university campus. J. Clean. Prod. 315:128175. doi: 10.1016/j.jclepro.2021.128175
Visagie, C. M., Houbraken, J., Frisvad, J. C., Hong, S. B., Klaassen, C. H., Perrone, G., et al. (2014). Identification, and nomenclature of the genus Penicillium. Stud. Mycol. 78, 343–371. doi: 10.1016/j.simyco.2014.09.001
Wang, S.-K., Yang, K.-X., Zhu, Y.-R., Zhu, X.-Y., Nie, D.-F., Jiao, N., et al. (2022). One-step co-cultivation and flocculation of microalgae with filamentous fungi to valorize starch wastewater into high-value biomass. Bioresour. Technol. 361, 127625. doi: 10.1016/j.biortech.2022.127625
Wang, Y., Tang, Y., and Yuan, Z. (2022). Improving food waste composting efficiency with mature compost addition. Bioresour. Technol. 34, 126830. doi: 10.1016/j.biortech.2022.126830
Wang, Y., Yuan, Z., and Tang, Y. (2021). Enhancing food security and environmental sustainability: a critical review of food loss and waste management. Resour. Environ. Sustainabil. 4, 100023. doi: 10.1016/j.resenv.2021.100023
Widiastuti, H., and Wulaningtyas, A. (2008). Activity of ligninolytic enzymes during growth and fruiting body development of white rot fungi Omphalina sp. and Pleurotus ostreatus. HAYATI J. Biosci. 15, 140–144. doi: 10.4308/hjb.15.4.140
Yim, S. S., Johns, N. I., Park, J., Gomes, A. L., McBee, R. M., Richardson, M., et al. (2019). Multiplex transcriptional characterizations across diverse bacterial species using cell-free systems. Mol. Syst. Biol. 15, 1–15. doi: 10.15252/msb.20198875
Yu, H., Xie, B., Khan, R., Dong, J., and Shen, G. (2021). The changes in macronutrients and microbial community structure during the co-composting of white wine distillers' grains and potassium silicate. J. Clean. Prod. 319, 128681. doi: 10.1016/j.jclepro.2021.128681
Zhang, L., and Sun, X. (2016). Improving green waste composting by addition of sugarcane bagasse and exhausted grape marc. Bioresour. Technol. 218, 335–343. doi: 10.1016/j.biortech.2016.06.097
Zhang, L., and Sun, X. (2019). Changes in physical, chemical, and microbiological properties during the two-stage composting of green waste due to the addition of β-cyclodextrin. Compost. Sci. Utilizat. 27, 46–60. doi: 10.1080/1065657X.2019.1585304
Zhang, S., Wang, J., Chen, X., Gui, J., Sun, Y., and Wu, D. (2021). Industrial-scale food waste composting: effects of aeration frequencies on oxygen consumption, enzymatic activities, and bacterial community succession. Bioresour. Technol. 320, 124357. doi: 10.1016/j.biortech.2020.124357
Zhang, X., Zhong, Y., Yang, S., Zhang, W., Xu, M., Ma, A., et al. (2014). Diversity and dynamics of the microbial community on decomposing wheat straw during mushroom compost production. Bioresour. Technol. 170, 183–195. doi: 10.1016/j.biortech.2014.07.093
Zhao, K., Xu, R., Zhang, Y., Tang, H., Zhou, C., Cao, A., et al. (2017). Development of a novel compound microbial agent for degradation of kitchen waste. Braz. J. Microbiol. 48, 442–450. doi: 10.1016/j.bjm.2016.12.011
Keywords: DNA sequencing, enzymes, gardening waste, microbiota, molecular techniques, organic residues
Citation: da Silva Gaspar S, Assis LLRd, Prado MPRd, Pedroso Miguel MG, Magno dos Reis Ferreira G, Schwan RF, Pasqual M, Rigobelo EC, Castro RP, Buttrós VH and Dória J (2023) Diversity and enzymatic activity of the microbiota isolated from compost based on restaurant waste and yard trimmings. Front. Sustain. Food Syst. 7:1013361. doi: 10.3389/fsufs.2023.1013361
Received: 06 August 2022; Accepted: 09 January 2023;
Published: 30 January 2023.
Edited by:
Manosh Kumar Biswas, University of Leicester, United KingdomReviewed by:
Macarena M. Jurado, University of Almeria, SpainJustyna Bohacz, University of Life Sciences of Lublin, Poland
Copyright © 2023 da Silva Gaspar, Assis, Prado, Pedroso Miguel, Magno dos Reis Ferreira, Schwan, Pasqual, Rigobelo, Castro, Buttrós and Dória. This is an open-access article distributed under the terms of the Creative Commons Attribution License (CC BY). The use, distribution or reproduction in other forums is permitted, provided the original author(s) and the copyright owner(s) are credited and that the original publication in this journal is cited, in accordance with accepted academic practice. No use, distribution or reproduction is permitted which does not comply with these terms.
*Correspondence: Joyce Dória, am95Y2UuZG9yaWFAdWZsYS5icg==