- Agricultural Science and Engineering School, Liaocheng University, Liaocheng, China
Steam explosion, a novel effective technology for cereal modification, integrates high-temperature autohydrolysis and structural disruption, which can significantly influence the morphological and physicochemical characterization of the feedstocks. The deep knowledge of the structural changes that are brought about by the treatment severity is connected with the technological demands to improve the processing efficiency and to increase the industrial application of the feedstocks by steam explosion. In this study, the changes in morphological and physicochemical properties of soybean meal induced by steam explosion were investigated. The correlation of steam explosion severity with soybean meal's final quality was also analyzed. The results showed that steam explosion effectively increased the fractal dimension from 1.6553 to 1.8871, the glycinin content from 151.38 to 334.94 mg/g, and the 2,2-diphenylpicrylhydrazyl (DPPH) radical scavenging activity from 28.69 to 63.78%. The gray value, color (L* and a* values), and the total phenol and polysaccharide contents of soybean meal were reduced with greater steam explosion severity. Steam explosion severity had a remarkable positive correlation with the fractal dimension and DPPH radical scavenging activity. However, steam explosion severity had no significant correlation with the textural and adsorption properties of the soybean meal. This study focused on the morphological and physicochemical property changes of the soybean meal during a steam explosion process, which could guide the application of steam explosion in food systems.
Introduction
Steam explosion is an efficient, economic, and eco-friendly technology for the pretreatment of feedstock with high-pressure and high-temperature saturated steam for a set period of time, after which the pressure is released instantaneously (Sui et al., 2018). It modifies the feedstock with the combined effects of thermal reactions and physical-tearing actions during steam displacement, steam penetration, steam cooking, and instantaneous decompression stages (Sui and Chen, 2014b; Wang et al., 2018). Steam explosion was brought about by the hydrolysis reactions under mild acidic conditions, which arose due to a decrease in pH and the release of organic acids from steam-penetrated feedstock (Sui and Chen, 2015; Ruiz et al., 2020). Furthermore, the instantaneous decompression action during the explosion stage caused a physical structure change in the feedstock (Ruiz et al., 2020). In the past few decades, steam explosion is mainly used as a pretreatment method for overcoming the intrinsic characteristics (recalcitrance, heterogeneity, multicomposition, diversity, etc.) of lignocellulosic materials, such as corn stover (Sui and Chen, 2014a; Chen and Liu, 2015). Compared to acid and alkali, steam explosion does not require any chemicals, thereby it serves as an environment-friendly method and offers economically improved product yields and quality (Ruiz et al., 2021). Nowadays, steam explosion is getting an increasing amount of attention from the food processing industry (Gong et al., 2021; Kong et al., 2022b). It could effectively increase the soluble dietary fiber and 2,2-diphenylpicrylhydrazyl (DPPH) radical scavenging activity in wheat bran, which will promote the development of wheat bran products (Aktas-Akyildiz et al., 2017; Sui et al., 2018; Kong et al., 2020, 2021). Steam explosion enhances the extraction rate of protein from soybean meal and helps to improve the functional properties of the proteins (Zhang et al., 2013, 2017; Kong et al., 2022a). It also enhances the release of phenolic compounds in soybean seed coats and increases the soluble dietary fiber content of okara (Li et al., 2019; Chen et al., 2020). The application of steam explosion in food industries may bring with it a host of benefits, including modifying protein and fiber structures and fortifying the nutritional and functional properties of feedstock (Sui et al., 2018). Steam explosion integrates high-temperature autohydrolysis and structural disruption, which can significantly influence the morphological and physicochemical characterization of feedstocks. The severity factor is an important index that describes the relationship between the processing conditions and physicochemical changes, resulting in the modification of feedstock due to steam explosion (Chornet and Overend, 2017; Ruiz et al., 2021). The severity parameter could be considered as a strategy for scaling up in a batch-mode operation from the steam explosion reactor (Ruiz et al., 2020). The rise in steam explosion severity of a certain range could accelerate hydrothermal reactions, promote the release of soluble dietary fiber, and improve the solubility of carbohydrates (Aktas-Akyildiz et al., 2017; Sui et al., 2018). Therefore, it is greatly significant to understand the technology behind the steam explosion process that is based on the changing law of morphological and physicochemical properties induced by steam explosion severity.
Soybean meal, one of the most important protein sources, is obtained after the oil has been extracted from soybean (Mukherjee et al., 2016). The utilization of soybean meal as a raw material to make healthy food has received extensive attention owing to its nutritive value (Yang et al., 2020). In this study, soybean meal was used as the raw material, and the effect of steam explosion on the morphology, texture, color, chemical composition, and functional property of soybean meal was investigated. This study may serve as a reference for the development of multivariant soybean meal foods and the use of the steam explosion process, which could be controlled and adjusted for different requirements according to the correlation of steam explosion severity and soybean meal quality.
Materials and methods
Sample preparation
Soybean meal was purchased from Jinshiliu Horticulture Co., Ltd. (Jiangsu, China). Steam explosion was carried out in a self-designed batch vessel that mainly consisted of a reactor chamber and a steam generator (WY19, Big Soldier Food Machinery, Henan, China) (Kong et al., 2022a). Soybean meal was rehydrated by distilled water with a 1:5 ratio of water to materials (w/w) on the dry weight of soybean meal. The soybean meal was loaded into a reactor chamber and treated at 0.3~0.7 MPa for 2~8 min, respectively. The holding temperature ranged from 133 to 165°C and the time for heating up to set pressure was kept below 10 s. The reaction system was then terminated when the steam-laden soybean meal suddenly exploded by opening the feed valve into the reception chamber.
The factor of steam explosion severity was calculated according to the method determined by Zhao et al. (2015), the experimental conditions of steam explosion and the corresponding severity factor are listed in Table 1. After the processing of steam explosion, the samples were dried in an oven at 60°C for 12 h. Then, 100 g of dried soybean meal was ground for 2 min in a ZT-150 high-speed grinder (Yongkang Zhanfan Industry and Trade Co., Ltd., Zhejiang, China).
Morphological evolution of soybean meal
Digital imaging was used to analyze the morphological property (gray value (GV) and fractal dimension) of soybean meal. The soybean meal before and after steam explosion treatment was photographed using a JSN-AL100a Honor mobile phone with the same spot (Figure 1). The images were acquired by Windows 10 system built-in Paint 3D at a resolution of 600 × 600 pixels. The average gray value (GV) of soybean meal was calculated by using MATLAB R2016a (MathWorks Inc., USA) (Zhao et al., 2015). Fractal dimensions of soybean meal were estimated by the box-counting method using the ImageJ-win64 (National Institutes of Health, USA) (Nai et al., 2021).
Textural property analysis
The textural property was measured by a CT3 Texture Analyzer (Brookfield Engineering Labs, Inc., USA). Dried soybean meal samples were compressed by the T-pattern analysis (TPA) at a speed of 0.5 mms−1 to a load of 10,000 g with a TA11 probe. All textural properties were analyzed by Texture Pro CT V1.6.
Color measurements
The color of the native and steam-exploded soybean meal flour was measured with a chromameter (Minolta CR-10, Japan). L* means the lightness of the flour, a* indicates green-red color, and b* indicates yellow-blue color (Yang et al., 2020). Chroma (An et al., 2021), Hue angle, and browning index (BI) (Maskan, 2001) were calculated using L*, a*, and b* values.
Chemical composition analysis
The total phenol content of soybean meal was determined by using the method described by Wu et al. (2013). One gram of soybean meal was mixed with 40 ml of 80% methanol solution and incubated at 50°C for 2 h in a thermostatic oscillation incubator and then centrifuged at 3,500 rpm (revolutions per minute) for 10 min. The supernatant was concentrated to a final volume of 10 mL and used to measure the total phenol content. Fifty microliters of the supernatant was added to the mixture (0.45 mL of distilled water and 2.5 mL of 10% Folin–Ciocalteu's reagent), kept at room temperature for 2 min. Then, 2 mL of 7.5% sodium carbonate solution was added, and the mixture was placed in the dark at room temperature for 40 min. The absorbance of the mixture was measured at 760 nm. The polysaccharide content of the soybean meal was determined by using the phenol–sulfuric acid method with modification (Nielsen, 2017). First, 100 mg of soybean meal was mixed with 1.0 mL of ethanol and 9.0 mL of 1 mol/L sodium hydroxide, incubated in boiling water for 10 min, and then filled to 100 mL. Then, 1 ml of the solution was added to the mixture (2 mL of distilled water, 1 mL of 5% phenol, and 5 mL of sulfuric acid) and kept at room temperature for 30 min. The absorbance of the mixture was measured at 490 nm.
Glycinin content in soybean meal was determined in duplicate using the Plant β-conglycinin ELISA Kit and Plant Histamine (HIS) ELISA Kit (ChunDuBio, Hubei, China). This method was used to determine the level of β-conglycinin and histamine in samples by the double antibody sandwich enzyme-linked immunosorbent assay (ELISA) method. The color change is measured by a Rayto RT-6100 microplate reader at a wavelength of 450 nm. Three replicate tests were carried out and the average values were reported.
Functional properties' analysis
Oil-holding capacity
The oil-holding capacity (OHC) of the soybean meal powder was determined according to the method of Sui et al. (2018) with some modifications. Commercial soybean oil was added to the dry soybean meal powder with the weight-to-volume ratio of 1:10, stirred, and left at 37°C for 30 min. After centrifugation at 4,500 rpm for 5 min, the underlayer deposition was weighed. The oil-holding capacity was expressed as a gram of oil held per gram of sample.
Cholesterol adsorbing capacity
The cholesterol adsorbing capacity (CAC) of the soybean meal powder was determined by using the method of Sangnark and Noomhorm (2003) with modifications. First, 1 g of soybean meal was mixed with 20 g of diluted egg yolk solution, adjusting the pH value of the system to 7.0, shaken at 37°C for 2 h, and then centrifuged at 4,000 rpm for 20 min. Then, 1 ml of the supernatant was diluted for 5 times with 90% acetic acid. The absorbance of the mixture was measured by using 0.2 mL of phthalaldehyde as the chromogenic agent at 550 nm.
DPPH radical scavenging activity
The DPPH radical scavenging activity was determined according to the method of Wu et al. (2013). Fifty microliters of the supernatant was added with 2 mL of 6.25 × 10−5 mol/L DPPH methanol solution and left in the dark for 30 min to react. The absorbance of the mixture was measured at 517 nm.
Statistical analysis
Three replicate tests of color measurements, gray values, and glycinin content determination were carried out and the average values were reported. Colorful profiles were processed with a one-way analysis of variance (ANOVA) using IBM SPSS Statistics 20 (IBM, NY, USA) with Duncan's multiple range test (MRT) (p < 0.05). Experimental data were processed by Min–Max Normalization and Pearson's Correlation using IBM SPSS Statistics 20 (IBM, NY, USA).
Results and discussion
Morphological characteristics of soybean meal
Soybean meal is a complicated material with morphological characteristics, so digital imaging could serve as an appropriate method to provide an economic, convenient, and nondestructive way to acquire, extract, and evaluate the characteristics of soybean meal. To provide an intuitive quantitative description of the effect of the steam explosion severity factor on soybean meal, the color changing (gray value) quality of soybean meal under different steam explosion conditions was investigated. The greater the steam explosion severity factor (except LgR157.3 and LgR158.0), the more distinct ws the color-changing quality of soybean meal, which resulted in the smaller gray value of soybean meal (Figure 2; Zhao et al., 2015).
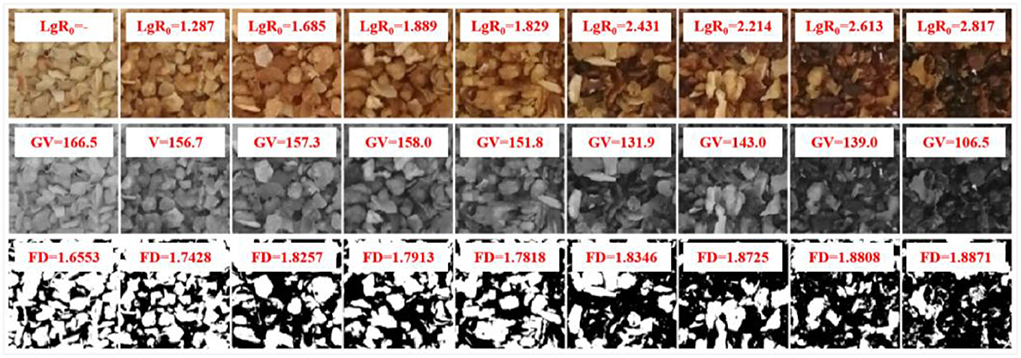
Figure 2. Morphological evolution of soybean meal. LgR indicates the steam explosion severity; GV, gray value; FD, fractal dimension.
Since the feedstock was complex and heterogeneous, a quantitative determination of the irregular, fragmented morphological property of soybean meal was impossible. The fractal dimension might be a new solution to the common problem of nonlinear complex changes in soybean meal during food processing. The calculated fractal geometry was used to quantify the morphological changes of mycelial growth through a series of steps, including image acquisition, feature extraction, and fractal dimension (Duan et al., 2012). The pore characteristics and fractal dimensions assessed the potential of the protein's performance in stabilizing gas cells produced during baking (Rahimi et al., 2020). After the treatment of steam explosion, the fractal dimension of soybean meal increased to varying degrees (Figure 3). The higher the fractal dimension, the more irregular the geometry of the soybean meal (Rahimi and Ngadi, 2016). The high irregularity of soybean meal geometry may be related to the decomposition of components or the formation of a porous structure by steam explosion (Kong et al., 2020). The gray value analysis and fractal dimension characterization of the steam explosion severity factor and the irregular shape-changing of steam-exploded soybean meal were carried out, which might provide a new method for the quantitative morphological evolution of feedstock modified by steam explosion.
Textural properties of soybean meal
Textural properties of soybean meal pretreated by the steam explosion were normalized and are presented in Figure 3. These properties of soybean meal were significantly changed by steam explosion, including hardness, springiness, cohesiveness, gumminess, and chewiness. Steam explosion increased the hardness of soybean meal, except for that of 1.685 and 1.889 steam explosion severity factors. The springiness of soybean meal was significantly decreased to varying degrees by steam explosion. Steam explosion reduced the cohesiveness, gumminess, and chewiness of soybean meal, except that of 2.431 steam explosion severity factor, compared with soybean meal without steam explosion treatment. It indicated that less energy was taken to destroy the structure of the steam-exploded soybean meal during the grinding process. Soybean meal without any steam explosion treatment showed better resistance to outside attack, and the grinding process should take more force and energy to break up the structure. Soybean meal has a tough structure of the cellulose fibers and pectin substances to form a complex matrix, which exhibits resistance to attacks (Zhang et al., 2013). The compact structure of lignocellulose was destroyed through chemical effects and mechanical forces by steam explosion (Wang et al., 2018), which might be conducive to the subsequent processing of soybean meal.
Color measurements of the soybean meal powder
The results of L*, a*, and b* values of samples are shown in Table 2. L* values denote the lightness to darkness (0 = black and 100 = white), while a* (redness to greenness) and b* (yellowness to blueness) represent the color-opponent dimensions, respectively (Ma et al., 2011). The steam explosion-treated soybean meal samples had lower L* and a* values compared to soybean meal without steam explosion treatment (L = 65.5 a* = 19.87, and b* = 25.80). Nonenzymatic browning takes place in the steam explosion process (Guo et al., 2015; Zhao et al., 2021). The Chroma values indicated the purity or saturation and showed no significant variation compared to native powder (An et al., 2021). Except for the LgR 1.685, steam explosion significantly decreased the Chroma values. When the steam explosion severity factor was >1.889, steam explosion increased the Hue values from 1.09 to 1.22. It suggested an increment from a greener color to an orange-red color of soybean meal. These results indicated that the partial steam explosion affected the color quality of soybean meal flour and produced more browning compound(s). With the increase of steam explosion severity factor, the color of steam-exploded soybean meal became darker. For cookies or extruded snacks, where a golden or brownish color was desired, this color-changing quality of steam-exploded soybean meal might turn out to be advantageous (Turan et al., 2015). A similar result was obtained, wherein steam-exploded wheat bran exhibited a significantly darker color than raw wheat bran (Guo et al., 2015; Zhao et al., 2021). Since the color of soybean meal is a determinant factor in deciding the consumer's acceptance of the product, the process could be controlled and adjusted to suit different requirements according to the fitting trend lines of color change (Guo et al., 2015).
Chemical compositions of the soybean meal powder
The nutrient composition of soybean meal can be changed after chemical and mechanical action with steam explosion (Figure 4). The phenol content gradually decreased when the steam explosion severity factor increased, which was different from that mentioned in previous studies (Kong et al., 2021; Wang et al., 2021). Compared with soybean meal without steam explosion treatment, the glycinin content of steam-exploded soybean meal was significantly increased. Glycinin is the most abundant protein in soybean, due to its good gelatinous properties in the aqueous phase, as well as good emulsifying and foaming activities; it is widely used as a filler in the food industry (Rickert et al., 2004; Golubovic et al., 2005). However, a fast, efficient, and inexpensive method for obtaining pure glycinin from soybean meal has not yet been developed. The soybean meal fibers and pectin substances form a complex matrix that clumps with proteins in the soybean meal, thus reducing the availability of soy protein (Carpita and Gibeaut, 1993; Antoine et al., 2003; Zhang et al., 2013). The extraction efficiency of protein can be enhanced by steam explosion, which is beneficial to the development and utilization of soybean meal proteins (Zhang et al., 2013). A downward trend in polysaccharides of soybean meal could be observed. Steam explosion promoted the conversion of insoluble dietary fiber to soluble dietary fiber, which facilitated the dissolution of dietary fiber (Sui et al., 2018).
Functional characteristics of soybean meal powder
The oil-holding capacity, cholesterol absorbing capacity (CAC), and DPPH radical scavenging activity of soybean meal with/without steam explosion treatment were compared and are shown in Figure 5. There is no significant change in the oil-holding capacity of soybean meal powder treated by steam explosion, but it is different from that given in the previous study (Sui et al., 2018; Kong et al., 2020). Steam explosion process could efficiently improve the cholesterol absorbing capacity (CAC) of soybean meal powder from 1.287 to 1.829 severity factor while reducing the CAC from 1.889 to 2.817 severity factor. Steam explosion increased the DPPH radical scavenging activity of soybean meal, except that of 1.889 steam explosion severity factor. The steam-exploded soybean meal powder of an extract with 2.817 severity factor had the strongest DPPH radical scavenging activity (63.78%), higher than that of narrow windrow burning (NWB) powder (28.69%). The steam-exploded soybean meal powder (steam explosion severity factor 2.214–2.817) might be a free radical inhibitor and limit the occurrence of free radical damage.
Correlation of various parameters of soybean meal
Elucidating the correlations of the morphological, nutritional, and physicochemical properties of soybean meal flour, as shown in Figure 6, it might be conducive to develop an indirect processing strategy to control the flour characteristics according to the changes in steam explosion conditions. Phenolics exhibited significant (p < 0.05) correlations with gray values, L* values, a* values, Chroma values, and Hue values of soybean meal flour. A negative correlation was found between the fractal dimension and gray values (r = −0.73, p < 0.05), phenols (r = −0.95, p < 0.05), polysaccharide content (r = −0.95, p < 0.05), and a* (r = −0.81, p < 0.05), L*, and Chroma values (r = −0.86, p < 0.05). Cohesiveness was positively related to gumminess (r = 1.00) and chewiness (r = 0.77). Glycinin content was positively related to chewiness but negatively connected to Chroma values. Polysaccharide content showed a strong negative relationship with the fractal dimension, browning index (BI), and Hue values, while the polysaccharide content was positively correlated with the gray values, a* values, L* values, springiness, and Chroma values.
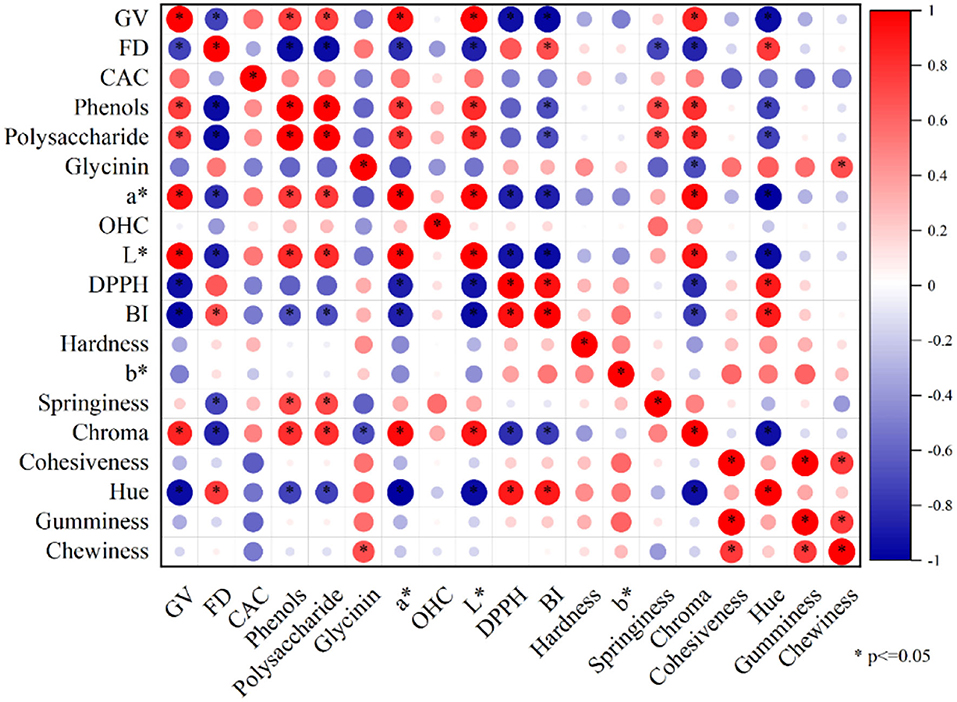
Figure 6. Pearson's correlation of various parameters of soybean meal flour. OHC, oil-holding capacity; CAC, cholesterol absorbing capacity; DPPH, 2,2-diphenylpicrylhydrazyl (DPPH) radical scavenging activity; GV, gray values; FD, fractal dimension; BI, browning index.
Correlation between steam explosion severity factor and soybean meal quality
The linear correlation coefficients between the steam explosion severity factor and the morphological, textural, and physicochemical properties of soybean meal are presented in Figure 7. Fractal dimension and Hue values of steam-exploded soybean meal exhibited a significantly positive correlation (p < 0.01) with the steam explosion severity factor. DPPH radical scavenging activity and browning index (BI) of soybean meal had a strong positive correlation (p < 0.05) with the steam explosion severity factor. The gray value, color parameters (L*, a*, and Chroma values), polysaccharide and phenol contents exhibited significant negative correlations (p < 0.01) with the steam explosion severity factor. Steam explosion severity factor had no significant correlation (p > 0.05) with glycinin content and textural and adsorption properties of soybean meal.
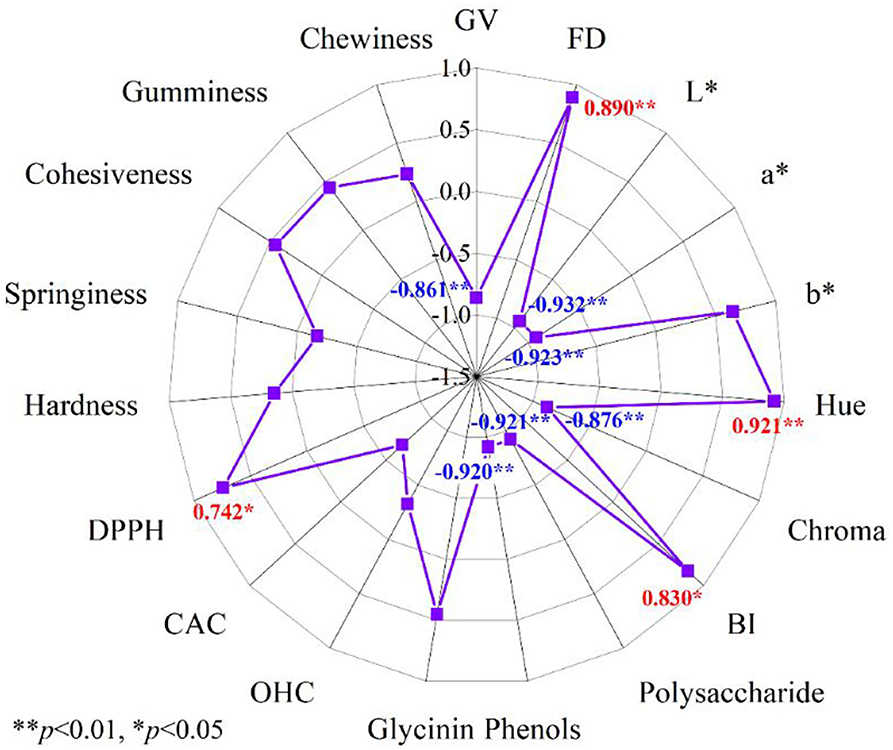
Figure 7. Correlation of steam explosion intensity and morphological and physicochemical properties of soybean meal. OHC, oil-holding capacity; CAC, cholesterol absorbing capacity; DPPH, 2,2-diphenylpicrylhydrazyl (DPPH) radical scavenging activity; GV, gray values; FD, fractal dimension; BI, browning index.
Conclusions
In this study, the effect of soybean meal modification caused by steam explosion and the relationship of steam explosion severity with soybean meal final quality were investigated. Steam explosion effectively increased the fractal dimension, glycinin content, and the DPPH radical scavenging activity. The steam-exploded soybean meal showed lower gray value, L and a* color parameters, and total phenol and polysaccharide contents compared with those of soybean meal without steam explosion treatment. Good linear correlations between steam explosion severity factor and morphological property, color parameters, DPPH radical scavenging activity, and certain chemical compositions were obtained. These findings provided valuable information on the relationship between steam explosion severity and morphological and physicochemical characterization, which will further guide us in the application of steam explosion on feedstocks modification. In a future study, we will improve the efficiency and performance of self-designed steam explosion devices and investigate the processing adaptability of soybean meal in food products.
Data availability statement
The original contributions presented in the study are included in the article/supplementary material, further inquiries can be directed to the corresponding author.
Author contributions
FK and XG: conceptualization, resources, and supervision. FK, YL, and QZ: methodology validation and investigation. FK: software, formal analysis, data curation, writing—original draft preparation, writing—review and editing, project administration, and funding acquisition. QZ and FK: visualization. All authors have read and agreed to the published version of the manuscript.
Funding
This study was financially supported by the Natural Science Foundation of Shandong Province (Nos. ZR2022QC242 and ZR2020QC061) and the Doctoral Research Startup Foundation of Liaocheng University (No. 318052122).
Conflict of interest
The authors declare that the research was conducted in the absence of any commercial or financial relationships that could be construed as a potential conflict of interest.
Publisher's note
All claims expressed in this article are solely those of the authors and do not necessarily represent those of their affiliated organizations, or those of the publisher, the editors and the reviewers. Any product that may be evaluated in this article, or claim that may be made by its manufacturer, is not guaranteed or endorsed by the publisher.
References
Aktas-Akyildiz, E., Mattila, O., Sozer, N., Poutanen, K., Koksel, H., and Nordlund, E. (2017). Effect of steam explosion on enzymatic hydrolysis and baking quality of wheat bran. J. Cereal Sci. 78, 25–32. doi: 10.1016/j.jcs.2017.06.011
An, N. N., Sun, W. H., Li, B. Z., Wang, Y., Shang, N., Lv, W. Q., et al. (2021). Effect of different drying techniques on drying kinetics, nutritional components, antioxidant capacity, physical properties and microstructure of edamame. Food Chem. 373, 131412. doi: 10.1016/j.foodchem.2021.131412
Antoine, C., Peyron, S., Mabille, F., Lapierre, C., Bouchet, B., Abecassis, J., et al. (2003). Individual contribution of grain outer layers and their cell wall structure to the mechanical properties of wheat bran. J. Agric. Food Chem. 51, 2026–2033. doi: 10.1021/jf0261598
Carpita, N. C., and Gibeaut, D. M. (1993). Structural models of primary cell walls in flowering plants: consistency of molecular structure with the physical properties of the walls during growth. Plant J. 3, 1–30. doi: 10.1046/j.1365-313X.1993.00999.x
Chen, H. Z., and Liu, Z. H. (2015). Steam explosion and its combinatorial pretreatment refining technology of plant biomass to bio-based products. Biotechnol. J. 10, 866–885. doi: 10.1002/biot.201400705
Chen, Y., Shan, S., Cao, D., and Tang, D. (2020). Steam flash explosion pretreatment enhances soybean seed coat phenolic profiles and antioxidant activity. Food Chem. 319, 126552. doi: 10.1016/j.foodchem.2020.126552
Chornet, E., and Overend, R. P. (2017). “How the severity factor in biomass hydrolysis came about,” in Hydrothermal Processing in Biorefineries: Production of Bioethanol and High Added-Value Compounds of Second and Third Generation Biomass, eds H. A. Ruiz, M. Hedegaard Thomsen, and H. L. Trajano (Cham: Springer International Publishing).
Duan, Y., Wang, L., and Chen, H. (2012). Digital image analysis and fractal-based kinetic modelling for fungal biomass determination in solid-state fermentation. Biochem. Eng. J. 67, 60–67. doi: 10.1016/j.bej.2012.04.020
Golubovic, M., Van Hateren, S. H., Ottens, M., Witkamp, G.-J., and Van Der Wielen, L. A. M. (2005). Novel method for the production of pure glycinin from soybeans. J. Agric. Food Chem. 53, 5265–5269. doi: 10.1021/jf0478206
Gong, J., Weng, Q., Sun, J., Wang, D., Qiu, S., Li, L., et al. (2021). Steam explosion pretreatment alters the composition of phenolic compounds and antioxidant capacities in Chrysanthemum morifolium Ramat cv. “Hangbaiju”. J. Food Proc. Preservat. 45, 15376. doi: 10.1111/jfpp.15376
Guo, J., Bian, Y.-Y., Zhu, K.-X., Guo, X.-N., Peng, W., and Zhou, H.-M. (2015). Effect of steam flash explosion pretreatment on phytate degradation of wheat bran. Food Bioproc. Technol. 8, 1552–1560. doi: 10.1007/s11947-015-1517-9
Kong, F., Wang, L., Chen, H., and Zhao, X. (2021). Improving storage property of wheat bran by steam explosion. Int. J. Food Sci. Technol. 56, 287–292. doi: 10.1111/ijfs.14630
Kong, F., Wang, L., Gao, H., and Chen, H. (2020). Process of steam explosion assisted superfine grinding on particle size, chemical composition and physico-chemical properties of wheat bran powder. Powder Technol. 371, 154–160. doi: 10.1016/j.powtec.2020.05.067
Kong, F., Zeng, Q., Li, Y., and Guo, X. (2022a). Effect of steam explosion on structural characteristics of β-conglycinin and morphology, chemical compositions of soybean meal. Front. Nutr. 9, 896664. doi: 10.3389/fnut.2022.896664
Kong, F., Zeng, Q., Li, Y., Zhao, Y., and Guo, X. (2022b). Improving bioaccessibility and physicochemical property of blue-grained wholemeal flour by steam explosion. Front. Nutr. 9, 877704. doi: 10.3389/fnut.2022.877704
Li, B., Yang, W., Nie, Y., Kang, F., Goff, H. D., and Cui, S. W. (2019). Effect of steam explosion on dietary fiber, polysaccharide, protein and physicochemical properties of okara. Food Hydrocoll. 94, 48–56. doi: 10.1016/j.foodhyd.2019.02.042
Ma, Z., Boye, J. I., Simpson, B. K., Prasher, S. O., Monpetit, D., and Malcolmson, L. (2011). Thermal processing effects on the functional properties and microstructure of lentil, chickpea, and pea flours. Food Res. Int. 44, 2534–2544. doi: 10.1016/j.foodres.2010.12.017
Maskan, M. (2001). Kinetics of colour change of kiwifruits during hot air and microwave drying. J. Food Eng. 48, 169–175. doi: 10.1016/S0260-8774(00)00154-0
Mukherjee, R., Chakraborty, R., and Dutta, A. (2016). Role of fermentation in improving nutritional quality of soybean meal—A Review. Asian-Australas J. Anim. Sci. 29, 1523–1529. doi: 10.5713/ajas.15.0627
Nai, G. A., Martelli, C. A. T., Medina, D. A. L., Oliveira, M. S. C. D., Caldeira, I. D., Henriques, B. C., et al. (2021). Fractal dimension analysis: a new tool for analyzing colony-forming units. MethodsX 8, 101228. doi: 10.1016/j.mex.2021.101228
Nielsen, S. S. (2017). “Total carbohydrate by phenol-sulfuric acid method,” in Food Analysis Laboratory Manual, ed S. S. Nielsen (Cham: Springer International Publishing).
Rahimi, J., Baur, J., and Singh, A. (2020). Digital imaging as a tool to study the structure of porous baked foods. J. Cereal Sci. 95, 103084. doi: 10.1016/j.jcs.2020.103084
Rahimi, J., and Ngadi, M. O. (2016). Structure and irregularities of surface of fried batters studied by fractal dimension and lacunarity analysis. Food Struct. 9, 13–21. doi: 10.1016/j.foostr.2016.07.002
Rickert, D. A., Johnson, L. A., and Murphy, P. A. (2004). Functional properties of improved glycinin and β-nglycinin fractions. J. Food Sci. 69, FCT303-FCT311. doi: 10.1111/j.1365-2621.2004.tb06332.x
Ruiz, H. A., Conrad, M., Sun, S. N., Sanchez, A., Rocha, G. J. M., Romani, A., et al. (2020). Engineering aspects of hydrothermal pretreatment: from batch to continuous operation, scale-up and pilot reactor under biorefinery concept. Bioresour. Technol. 299, 122685. doi: 10.1016/j.biortech.2019.122685
Ruiz, H. A., Galbe, M., Garrote, G., Ramirez-Gutierrez, D. M., Ximenes, E., Sun, S.-N., et al. (2021). Severity factor kinetic model as a strategic parameter of hydrothermal processing (steam explosion and liquid hot water) for biomass fractionation under biorefinery concept. Bioresour. Technol. 342, 125961. doi: 10.1016/j.biortech.2021.125961
Sangnark, A., and Noomhorm, A. (2003). Effect of particle sizes on functional properties of dietary fibre prepared from sugarcane bagasse. Food Chem. 80, 221–229. doi: 10.1016/S0308-8146(02)00257-1
Sui, W., and Chen, H. (2014a). Extraction enhancing mechanism of steam exploded Radix Astragali. Process Biochem. 49, 2181–2190. doi: 10.1016/j.procbio.2014.08.010
Sui, W., and Chen, H. (2014b). Multi-stage energy analysis of steam explosion process. Chem. Eng. Sci. 116, 254–262. doi: 10.1016/j.ces.2014.05.012
Sui, W., and Chen, H. (2015). Study on loading coefficient in steam explosion process of corn stalk. Bioresour. Technol. 179, 534–542. doi: 10.1016/j.biortech.2014.12.045
Sui, W., Xie, X., Liu, R., Wu, T., and Zhang, M. (2018). Effect of wheat bran modification by steam explosion on structural characteristics and rheological properties of wheat flour dough. Food Hydrocoll. 84, 571–580. doi: 10.1016/j.foodhyd.2018.06.027
Turan, D., Capanoglu, E., and Altay, F. (2015). Investigating the effect of roasting on functional properties of defatted hazelnut flour by response surface methodology (RSM). LWT Food Sci. Technol. 63, 758–765. doi: 10.1016/j.lwt.2015.03.061
Wang, L., Kong, F., and Chen, H. (2018). “Steam explosion pretreatment and saccharification of lignocellulosic biomass,” in Handbook of Biorefinery Research and Technology, ed J. M. Park (Dordrecht: Springer Netherlands).
Wang, W., Yang, B., Li, W., Zhou, Q., Liu, C., and Zheng, C. (2021). Effects of steam explosion pretreatment on the bioactive components and characteristics of rapeseed and rapeseed products. LWT 143, 111172. doi: 10.1016/j.lwt.2021.111172
Wu, L., Huang, Z., Qin, P., and Ren, G. (2013). Effects of processing on phytochemical profiles and biological activities for production of sorghum tea. Food Res. Int. 53, 678–685. doi: 10.1016/j.foodres.2012.07.062
Yang, H., Qu, Y., Li, J., Liu, X., Wu, R., and Wu, J. (2020). Improvement of the protein quality and degradation of allergens in soybean meal by combination fermentation and enzymatic hydrolysis. LWT 128, 109442. doi: 10.1016/j.lwt.2020.109442
Zhang, Y., Yang, R., Zhang, W., Hu, Z., and Zhao, W. (2017). Structural characterization and physicochemical properties of protein extracted from soybean meal assisted by steam flash-explosion with dilute acid soaking. Food Chem. 219, 48–53. doi: 10.1016/j.foodchem.2016.09.079
Zhang, Y., Yang, R., Zhao, W., Hua, X., and Zhang, W. (2013). Application of high density steam flash-explosion in protein extraction of soybean meal. J. Food Eng. 116, 430–435. doi: 10.1016/j.jfoodeng.2012.12.006
Zhao, G., Gao, Q., Hadiatullah, H., Zhang, J., Zhang, A., and Yao, Y. (2021). Effect of wheat bran steam explosion pretreatment on flavors of nonenzymatic browning products. LWT 135, 110026. doi: 10.1016/j.lwt.2020.110026
Keywords: steam explosion, digital imaging, soybean meal, physicochemical property, fractal dimension
Citation: Zeng Q, Kong F, Li Y and Guo X (2022) Correlation of steam explosion severity with morphological and physicochemical characterization of soybean meal. Front. Sustain. Food Syst. 6:991888. doi: 10.3389/fsufs.2022.991888
Received: 12 July 2022; Accepted: 06 October 2022;
Published: 08 November 2022.
Edited by:
Bo Wang, Australian Catholic University, AustraliaReviewed by:
Hyun-Jae Shin, Chosun University, South KoreaHéctor A. Ruiz, Universidad Autónoma de Coahuila, Mexico
Copyright © 2022 Zeng, Kong, Li and Guo. This is an open-access article distributed under the terms of the Creative Commons Attribution License (CC BY). The use, distribution or reproduction in other forums is permitted, provided the original author(s) and the copyright owner(s) are credited and that the original publication in this journal is cited, in accordance with accepted academic practice. No use, distribution or reproduction is permitted which does not comply with these terms.
*Correspondence: Feng Kong, d3lrZjIwMTJAMTI2LmNvbQ==
†These authors have contributed equally to this work and share first authorship