- 1Department of Plant Pathology, University of Wisconsin-Madison, Madison, WI, United States
- 2Department of Horticulture, University of Wisconsin-Madison, Madison, WI, United States
Cover crop-based reduced tillage (CCBRT) systems can provide multiple benefits in cucurbit cropping systems, including potential to reduce spread of soil-borne pathogens, minimize erosion, and decrease weed pressure. Despite benefits and farmer interest, adoption has been limited, in part due to inconsistent weed suppression and potential for reduced yields. Prior studies have suggested that N competition, allelopathy, and lower temperature may be factors in reducing vegetable yield in CCBRT systems. A strip tillage approach has been suggested as one strategy that could mitigate those issues, but cucurbit yields using these systems have shown mixed results in prior studies, some of which did not include other important considerations for growers such as the impact on weed and pest pressure. In 2018 and 2019, CCBRT strip till practices for organic acorn winter squash (Cucurbita pepo L.) production were assessed in Wisconsin on certified organic land. Combinations of different between-row (aisle) and in-row mulches were compared to attempt to identify reduced tillage combinations that effectively manage weeds while resulting in yields comparable to full tillage production, testing our hypothesis that no differences between production systems would be observed due to strip tillage and plastic mulch warming soil and minimizing competition while promoting cash crop growth. Aisle treatments included roller-crimped cereal rye (Secale cereale L.) mulch, straw mulch and cultivated bare ground, and in-row treatments included plastic mulch, ground straw mulch, and cultivated ground. Weed and pest counts, weed management time, and yields were compared between treatments. Plots managed with rye and straw in the aisles had significantly less weed pressure as compared to cultivated aisle treatments, although rye required more weed management time than ground straw mulch. In addition, rye resulted in lower marketable yield due to higher proportion unmarketable fruit in 2018, likely related to a 25 cm rain event 2 weeks prior to harvest. A significant row mulch × aisle mulch interaction was observed for marketable fruit m−1, showing that yield was not significantly affected by the type of in-row mulch in plots with crimped rye mulch in the aisle. Pressure from squash bugs (Anasa tristis) was also higher in treatments with organic or synthetic mulches (straw in aisles or rows, rye in aisles, and plastic in rows). Our results support previous evidence that crimped rye can be an effective mulching strategy to reduce weed pressure, with more efficient management than traditional straw mulch. However, crimped rye systems may have negative implications for yield and pest pressure regardless of the use of a strip-tillage approach, indicating that more research is needed to refine the production system.
Introduction
Weed management is consistently cited as a significant obstacle for organic farmers (Moynihan, 2010; Jenkins and Ory, 2016). To manage weeds in cucurbit crops, most organic growers rely heavily on either mechanical cultivation or black plastic mulches, both of which bring considerable economic and biological costs. For example, a recent survey of 105 organic farmers in Michigan revealed that the mean number of tillage passes in winter squash production was 6.5 per season, with some growers tilling as many as 15 times (Lowry and Brainard, 2019). Cover crops have been recognized as a valuable tool in the “many little hammers” approach to creating long-term organic production strategies that lower the weed seedbank while providing additional ecological benefits (Liebman et al., 1997; Baraibar et al., 2018; Wauters et al., 2021). Cover crops can support weed management through direct competition, the creation a physical barrier through crop residues, the release of allelochemicals, and the alteration of soil nutrient dynamics (Teasdale and Mohler, 2000; Sarrantonio and Gallandt, 2003; Hiltbrunner et al., 2007; Bezuidenhout et al., 2012; Teasdale et al., 2012; Brust et al., 2014). Beyond their weed suppressive benefits, cover crops also improve soil health and water quality by reducing erosion and increasing organic matter (Reicosky and Forcella, 1998; Sarrantonio and Gallandt, 2003; Ryder and Fares, 2008; Luo et al., 2010; De Baets et al., 2011; Kaspar et al., 2011).
Although cover crops are used extensively in organic production (USDA-NASS, 2019), adoption as a full-season weed control strategy has been limited, and cover crops are usually terminated and incorporated prior to planting the cash crop (Magdoff and Van Es, 2000), precluding potentially unique benefits afforded by full season cover crops (Deguchi et al., 2012). Shorter growing seasons in temperate climates (Snapp et al., 2005), and diverse, complex, and high value rotations on vegetable farms complicate integration of cover crops (Sarrantonio, 1992) into tillage-intensive production systems of northern cucurbit growers. Cover crop-based reduced tillage (CCBRT) encompasses a suite of practices which strategically integrate cover crops into a cash crop rotation with the goal of suppressing weeds while reducing soil disturbance (Vincent-Caboud et al., 2019). These practices frequently integrate the use of a roller-crimper to create an in-situ mulch of killed cover crop residue into which the cash crop can be planted, providing a thick layer of biomass allowing for season-long weed suppression without the need for tillage and cultivation (Smith et al., 2011; Delate et al., 2012; Mirsky et al., 2012; Silva, 2014; Silva and Delate, 2017). Such full season applications extend the environmental benefit of cover crops typically limited by cash crop seasonality, while also limiting the number of tillage passes required and thus reducing production costs.
While much of the research regarding CCBRT has been conducted with grain crops, an increasing number of studies have evaluated this system for organic vegetable production. The performance of CCBRT in organic vegetable systems has varied widely depending on the vegetable crop, cover crop, and environment (Forcella et al., 2015; Chehade et al., 2019; Lounsbury et al., 2020). In certain circumstances, the practice has resulted in equivalent or greater vegetable yields than those obtained from more typical organic systems using mechanical weed management (e.g., Creamer et al., 1996; Campiglia et al., 2010; Vollmer et al., 2010; Lounsbury and Weil, 2015; Jokela and Nair, 2016; Sportelli et al., 2022), while in other studies, the system resulted in reduced yields (e.g., Leavitt et al., 2011; Delate et al., 2012; Bietila et al., 2016; Jokela and Nair, 2016).
Reduced yields under CCBRT management can often be attributed to several factors, including insufficient weed suppression and competition of the cover crop with the cash crop, such as for nitrogen (Vincent-Caboud et al., 2019). Slow nitrogen mineralization rates associated with lower soil temperatures can limit available nitrogen at key phases of crop growth within CCBRT systems (Leavitt et al., 2011). Some of the most used cover crops found in CCBRT management, such as cereal rye (Secale cereale L.), are characterized by high carbon to nitrogen (N) ratios at maturity which can lead to N immobilization, especially if cover crop residue remains on the soil surface rather than incorporated into the soil (Clark et al., 1994; Van Den Bossche et al., 2009; Salon, 2012; Chehade et al., 2019). The effects of these phenomena can be observed in the results of several CCBRT vegetable studies. For example, in Iowa, organic bell pepper yields under CCBRT management were comparable in one season, but lower during the second year, with the differences being attributed to differences in temperature and nutrient availability in soil under no-till management (Jokela and Nair, 2016). This phenomenon may have also been a factor in the performance of CCBRT systems in the Northeastern US, where organic cabbage yields were reduced 21% and temperatures under rye mulch were 2–3°C lower than bare soil, although other factors such as stunting due to rye allelopathy may have also impacted final yields of the crop (Mochizuki et al., 2008). Leavitt et al. (2011) also suggested that lower temperatures in CCBRT treatments led to lower yields for organic tomato, pepper, and zucchini in Minnesota.
Strip tillage has been presented as an alternative management approach to mitigate the potential yield losses related to the adoption of CCBRT practices, including in organic vegetable systems (Delate et al., 2003, 2012; Mochizuki et al., 2007; Leavitt et al., 2011; Luna et al., 2012; Bietila et al., 2016; Ginakes and Grossman, 2021). With strip tillage management, primary tillage and associated cover crop incorporation is restricted to the in-row planting zone, with the aisles between the rows remaining undisturbed. Strip tillage systems have the potential to combine the weed management benefits of intensive cover cropping practices with soil-building and reduced soil disturbance, while reducing risk of yield loss compared to full NT systems (Thomas et al., 2001; Brainard et al., 2013). Strip tillage systems can promote plant growth and yields through quicker warming of soil temperatures comparable to conventional tillage systems but not as great as with the use of plastic mulch (Licht and Al-Kaisi, 2005; Tillman et al., 2015).
Further, strip tillage management allows for the incorporation of high-carbon crop residues with the planting zone, which supports microbial populations and promotes N mineralization (Brainard et al., 2013). In one case in a conventional pumpkin production system, the use of strip tillage in combination with a crimped rye/hairy vetch mixture increased the number of marketable fruits by reducing pathogen incidence (Everts, 2002).
CCBRT systems in vegetable production have shown clear promise for conserving soil health and playing a role in long term weed management. Yet results for pest pressure and, most critically, yield have been variable across different reduced tillage systems and vegetable crops (e.g., Delate et al., 2003; Snyder, 2015; Jabbour et al., 2016; Jokela and Nair, 2016; Skidmore et al., 2019), including for cucurbits specifically. For instance, Forcella et al. compared conventionally cultivated and crimped rye systems in Western Minnesota and found that cucumber (Cucumis sativus L.) yields were comparable, pumpkin (Cucurbita pepo L.) yields were 25% lower in rye, and watermelon (Citrullus lanatus) yields were 75% lower in rye (Forcella et al., 2015). However, few if any studies have assessed the feasibility of crimped rye as a management strategy for organic winter squash production while assessing critical management considerations such as yields, insect pest and weed pressure, and labor, all of which need to be understood before growers can be confident adapting CCBRT practices.
This study expands on previous research on organic CCBRT management for cucurbit systems by integrating strip tillage strategies, evaluating both in-row management of the tilled strips and between row (aisle) management strategies. Specific objectives included: (1) comparison of pest (weed, disease, and insect pressure) throughout the cucurbit production season, through the visual assessment of disease incidence and physical counts of insect and weed pressure; (2) comparison of yields through the fruit counts and weights of both marketable and unmarketable fruits; and (3) comparison of labor required for weed management throughout the cucurbit production season through tracking of hours needed for plot maintenance. Whole plot row mulch treatments representing possible strip tillage options included plastic mulch, straw mulch and bare cultivated ground, while split plot aisle mulch treatments included full tillage cultivated ground, straw mulch and crimped rye. Data collected included vegetable yield, plant survival rate, weed counts and management time, and cucumber beetle and squash bug counts.
Materials and methods
Site and treatment descriptions
Field trials were conducted at the University of Wisconsin's West Madison Agricultural Research Station (Verona, WI, USA) from September 2017 to September 2019. Two adjacent areas of certified organic land (43.0734, −89.5474 and 43.0744, −89.5465) were used for the experiment, both of which had been previously planted with a 3-year old alfalfa stand and managed in accordance with the United States Department of Agriculture National Organic Program (USDA-NOP) regulations (National Organic Program, 2000). Soil types were Batavia and Troxel silt loams, with organic matter content of 3.3% in 2018 and 2.9% in 2019, and pH 6.6 in 2018 and 7.2 in 2019. The experiment was established as a split-plot randomized complete block design with three replications, with row mulch as the whole-plot factor and aisle mulch as the strip-plot factor (Supplementary Figure 1). Each subplot had 10 plants. Whole plot, row mulch factors included a cultivated control, black plastic mulch, and ground straw mulch applied at a rate of 33,625 kg ha−1. Strip plot, aisle mulch treatments included cereal rye crimped at anthesis with a roller-crimper (I&J Manufacturing, Gap, PA), ground winter wheat straw mulch applied at a rate of 33,625 kg ha−1, and a cultivated control.
Field activities
Field activities are summarized in Table 1. Cereal rye was seeded in the entire study area with a Landoll grain drill (Landoll Corporation, Marysville, KS) at a rate of 250.96 kg ha−1 on September 25, 2017 and September 27, 2018, 2–3 weeks following the termination of a 3-year alfalfa stand with a Brillion Super Soil Builder Disk Chisel (Brillion Iron Works, Brillion, WI). The following spring, cultivated and straw mulched treatments were terminated when the cereal rye reached 0.25 m in height. Planting rows were strip tilled on 2.74 m centers within roller-crimped treatment plots using 900DRT Husqavarna walk-behind rototiller (Husqvarna Group, Stockholm, Sweden) to a 1.22 m width. In all treatment plots containing ground straw or cultivation, the cereal rye cover crop was mowed using a rotary mower followed by tillage using a Case IH JX65 tractor with 65 horsepower (Case IH, Racine, WI) with a PTO driven Land Pride RTA3576 tiller with a 1.83 m working width (Land Pride, Salinas, KS). One tillage event was adequate to terminate the rye in 2018, but a second tilling was required in 2019. Rye biomass was measured at anthesis immediately prior to crimping by clipping above ground growth in two 0.25 m2 sections, immediately adjacent to each rye plot but outside of the study area, so as not to affect weed pressure within plot. Biomass samples were then placed in a heated air dryer (54°C) at WMARS for 14 days and weighed. Remaining cereal rye within the rye aisle treatments was terminated by roller-crimping at anthesis, with the 4.57 m roller-crimper (I&J Manufacturing, Gap, PA).
Fertilizer was applied by hand within planting strips according to University of Wisconsin-Extension recommendations (Laboski and Peters, 2019) based on soil test results, including 134.5 kg ha−1 of N, followed by an additional shallow pass with the rototiller to incorporate fertilizer. Drip irrigation, plastic and straw mulches were applied by hand following final rye termination. Three-week old “Honey Bear F1” acorn squash (Cucurbita pepo) transplants grown in 50 cell trays were hand transplanted at 0.61 m in-row and 2.74 m between-row spacing 1 week after crimping, in both years. Drip irrigation placed under mulch was applied as needed throughout the season.
In both rows and aisles, weeds were categorized as broadleaf or grass weeds and counted within two randomly placed 0.25 m2 quadrats within 24 h prior to timed manual weeding (n = 18 per treatment at each date). Straw and plastic mulch treatments were weeded by hand, and cultivated treatments were managed with stirrup hoes supplemented by additional hand weeding close to plants. Total weeding time (for a single person) required for weed management after the planting of the cash crops was recorded separately for each row and aisle treatment at each weeding event (n = 9 per treatment at each date). Cucumber beetle, squash bug egg clusters, and adult squash bugs per plant were counted as close to a weekly basis as possible (n = 90 per treatment at each date). Squash was harvested at maturity, assessed visually by the condition of fruit peduncles and plant senescence in combination with projected days to maturity. In each plot, the final plant count was recorded, and all mature squash of marketable size were harvested and sorted as marketable or non-marketable as determined by visible evidence of rot, insect damage, surface blemishes, or being misshapen. Immature fruit (as assessed by very small size and green peduncles) were not counted.
Data analysis
Data were analyzed in R (R.app GUI 1.73 (7892 Catalina build), S. Urbanek & H.-J. Bibiko, © R Foundation for Statistical Computing, 2020). ANOVAs were done using the lme() function in the “nlme” package (Pinheiro and Bates, 2022) using the following model:
where Yijkl is the observation for the ith year, jth block, kth row mulch (whole plot) treatment, and lth aisle mulch (subplot) treatment, Ai is the fixed effect of the ith year (i = 2018, 2019), Bj(i) is the random effect of the jth block nested within the ith year (j = 1, 2, 3), WPk is the fixed effect of the kth whole plot row mulch treatment (k = cultivated, straw, plastic), δk(ji) is the random effect of the whole plot error term nested within the jth block within the ith year, SPl is the fixed effect of the lth subplot aisle mulch treatment (l = cultivated, straw, rye), (AWP)ik is the effect of the interaction between the ith year and kth aisle mulch, (ASP)il is the effect of the interaction between the ith year and lth row mulch, (AWPSP)ikl is the effect of the interaction between the ith year and kth aisle mulch and lth row mulch, and ϵijkl is the residual error associated with the observation for the ith year, jth block, kth row mulch (whole plot) treatment, and lth aisle mulch (subplot) treatment.
Pest data, weed management time, weed counts, and survival data were analyzed following the same procedure. However, pest counts and weed management time were transformed to cumulative counts, with only the final cumulative count analyzed. Weed counts and weed management time were analyzed with either the whole plot or subplot terms as appropriate, not both, and thus did not include the whole plot error term or associated interactions, so in-row weeding data was only associated with row mulch effects, and aisle weeding data was only associated with aisle mulch treatments. Pest and weed counts also included an additional subsampling error term γm(k(ji)) which was the random effect of the mth subsample (m = 1…10 where 10 is the number of plants per plot checked for pests, or where m = 1, 2 subsamples for weed counts).
Normality and equality of variances were checked visually with standardized residuals vs. fitted value plots and normal QQ plots, respectively (R Core Team, 2021). Right skewed count data for individual models (i.e., an entire given variable for a single model) were transformed with log(x + 1) when necessary to improve assumptions of normality and equality of variances. Pest count data could not be fully transformed to meet assumptions, but due to relative robustness of the F-test to deviations from normality and equal variances F-tests were performed anyway. Left skewed plant survival data was transformed with an arcsin(sqrt(x)) transformation. When ANOVA F-tests were significant, Tukey's Multiple Comparison Procedure was used to compare treatment means and develop significance groupings using the emmeans() function in the “emmeans” package, which is also how estimated marginal means for tables were obtained (Lenth, 2022). When two-way interactions between main effects were found, pairwise comparisons for the simple main effect were made for each level of the other factor, again using the emmeans() function with a Tukey adjustment. All figures are shown with non-transformed data though significance groupings are based on transformed data when applicable.
Results and discussion
Weather
Winter and spring precipitation leading into the 2018 season was slightly greater than average, with close to average temperatures and the accumulation of more growing degree day units (GDDU) than normal (Table 2). In contrast, winter conditions prior to the 2019 production season were colder and wetter than average, with a cooler and drier than average spring. Both 2018 and 2019 saw greater summer rainfall than average, with a single rain event in late August of 2018 releasing over 25 cm of rain within 24 h at the study site. Weed data was ended after that extreme rainfall event (MRCC, 2021).
Yield and plant survival
While the rye treatment yielded equivalent total fruit m−1 to the cultivated treatment, rye produced lower yields with respect to marketable fruit m−1 and a higher proportion and count of unmarketable fruit than cultivated aisles, regardless of row mulch (Table 3, Figure 1). The amount of marketable fruit plant−1 produced by cultivated aisles was similar to straw-mulched aisles; however, yield in terms of total fruit plant−1 was lower. Across aisle mulch treatments, plastic rows produced fewer total fruit m−1 than rows mulched with straw or cultivated rows, likely due to the low survival rate observed in plastic rows (Figure 2). With a lower number of unmarketable fruit, plastic rows produced yields of marketable fruit comparable to that of the rows mulched with straw despite the reduced number of total fruit, although the trend was toward lower marketable yields. Treatments utilizing straw produced greater total fruit yield in rows on a m−1 basis, and greater yields in both rows and aisles on a plant−1 basis but did not result in better marketable fruit yields due to producing more unmarketable fruit than cultivated treatments.
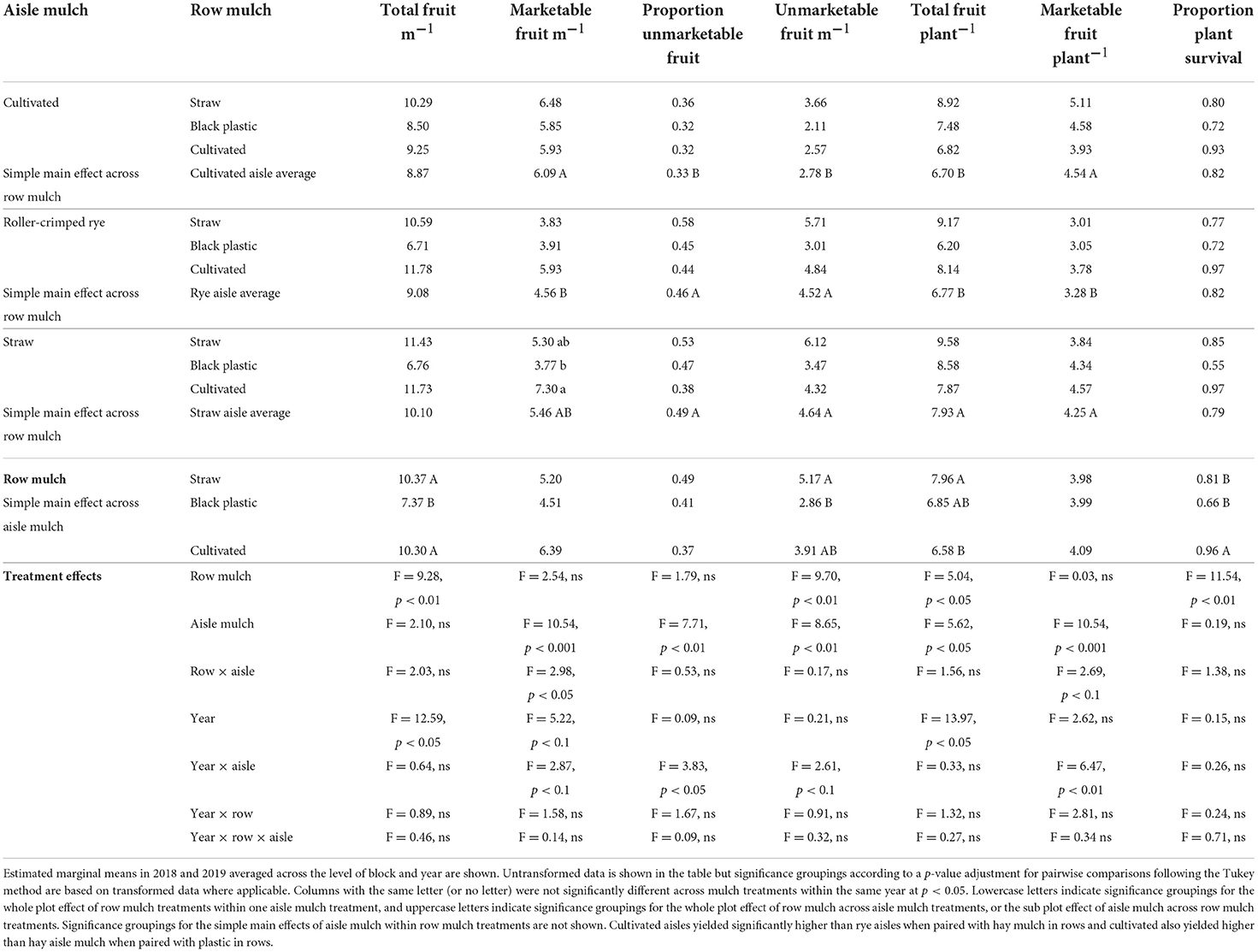
Table 3. Yield, quality, and survival data for organic squash managed with CCBRT by mulch treatment.
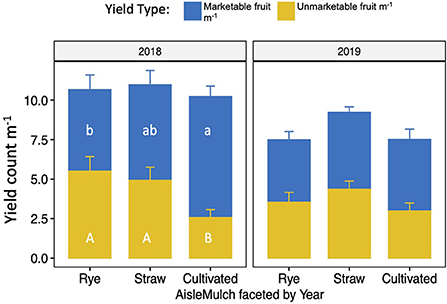
Figure 1. Yield m−1 of marketable and unmarketable of acorn squash grown using different aisle mulches, 2018 and 2019. Lowercase letters indicate significance groupings for marketable fruit m−1 and uppercase letters indicate significance groupings for unmarketable fruit m−1. Groups with the same letter (or no letter) did not differ across aisle mulch treatments within the same year at p < 0.05.
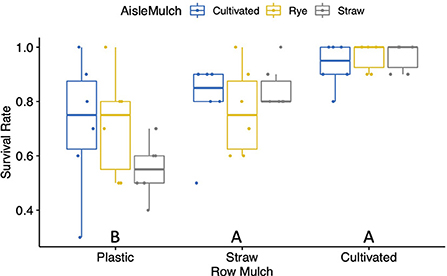
Figure 2. Proportion of squash plants surviving until fruit maturity by row and aisle mulches. Uppercase letters indicate significance groupings for row mulches. Groups with the same letter (or no letter) were not significantly different across years and aisle treatments at p < 0.05.
A significant row mulch × aisle mulch interaction was observed for marketable fruit m−1 (Figure 3). Where rows were cultivated, yield was similar regardless of the combination with straw, rye or cultivation in the aisle. Similarly, whenever aisles were cultivated, equivalent yields were observed regardless of row mulch treatment. The use of straw mulch within the row resulted in higher yields when coupled with cultivated aisles as compared to rye aisles (Figure 3A). Within rows with the plastic mulch treatment, higher yields were observed for plots with cultivated aisles as compared to straw or rye in the aisle (Figure 3A). No significant differences were observed for row mulch treatments utilizing cultivated or rye aisles. However, within straw-mulched treatments, the marketable fruit yield utilizing cultivated rows was double that of treatments utilizing plastic rows (Figure 3B).
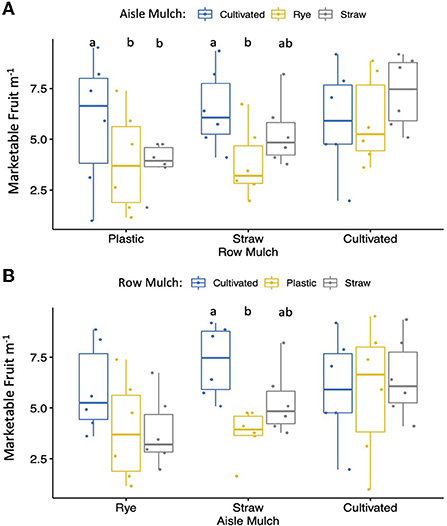
Figure 3. Marketable fruit yield m−1 of acorn squash grown using different row mulch (A) and aisle mulch (B) treatments. (A) Lowercase letters indicate significance groupings for aisle mulch within a given row mulch group, p < 0.05. (B) Lower letters indicate significance groupings for row mulch treatments within a given aisle mulch group, p < 0.05.
Differences in marketability were driven by a significant year × aisle mulch interaction, with clearly higher proportions of unmarketable fruits for both straw mulched aisles and crimped rye in 2018, the year the field flooded prior to harvest, and no significant differences in 2019 (Figure 1; Supplementary Tables 1, 2). Similarly, a year × aisle mulch interaction was observed for marketable fruit plant−1 (Supplementary Figure 2). In both years, rot was the most common cause of fruit being deemed unmarketable, followed by rodent damage.
While the primary yield declines in this study appeared to be caused by the 2018 rain event and subsequent fruit rot, the crimped rye treatments also produced fewer total fruit plant−1 than treatments with straw mulch in the aisle, suggesting there may be other mechanisms impacting yield. One such mechanism may be N immobilization with rye cover crops (e.g., Delate et al., 2008; Van Den Bossche et al., 2009; Chehade et al., 2019) or reduced N mineralization thanks to lower temperatures (as suggested by Leavitt et al., 2011).
Although previous research suggests that supplementary fertilization could improve vegetable yields in reduced tillage systems, studies largely focus on either fertigation or sidedressing (e.g., Delate et al., 2008; Schellenberg et al., 2009; Jokela and Nair, 2016). Future studies assessing the benefits of supplementary fertilizers should compare approaches, timing, and rates within a single study. Choosing cover crop species or mixes that include the benefit of nitrogen fixation from legumes and optimizing management to maximize nitrogen cycling may also be an option for reducing the potential for yield declines (Ginakes and Grossman, 2021).
In general, a stronger effect from row mulch than aisle mulch on total yield m−1 was observed in our study. Cultivated rows had higher yields than mulched rows, again pointing to the sensitivity of these systems to environmental conditions. These system × environment interactions indicate the need for further study of disease and pest dynamics within CCBRT systems as driven by different environmental conditions. Despite the potential for reduced yields, all treatments generally produced well relative to the advertised marketable yield plant−1 for the variety used (All American Selections, 2009).
CCBRT systems provide the notable benefit of resilience in the face of extreme rainfall events through protecting the soil and reducing erosion. However, while soil is protected under wet conditions, our study indicated that trade-offs may exist with respect to the system exacerbating disease pressure. While some research has investigated disease dynamics in CCBRT systems for cucurbits (e.g., Maglione et al., 2022), it is crucial that such research also simultaneously integrates the assessment other agronomic impacts such as yield quantity and weed management in order to form a more holistic picture of system performance.
Insect pest pressure
Striped cucumber beetle
Striped cucumber beetle counts were very low overall, especially in 2018, and the only clear effect was from year (Table 4; Supplementary Table 3).
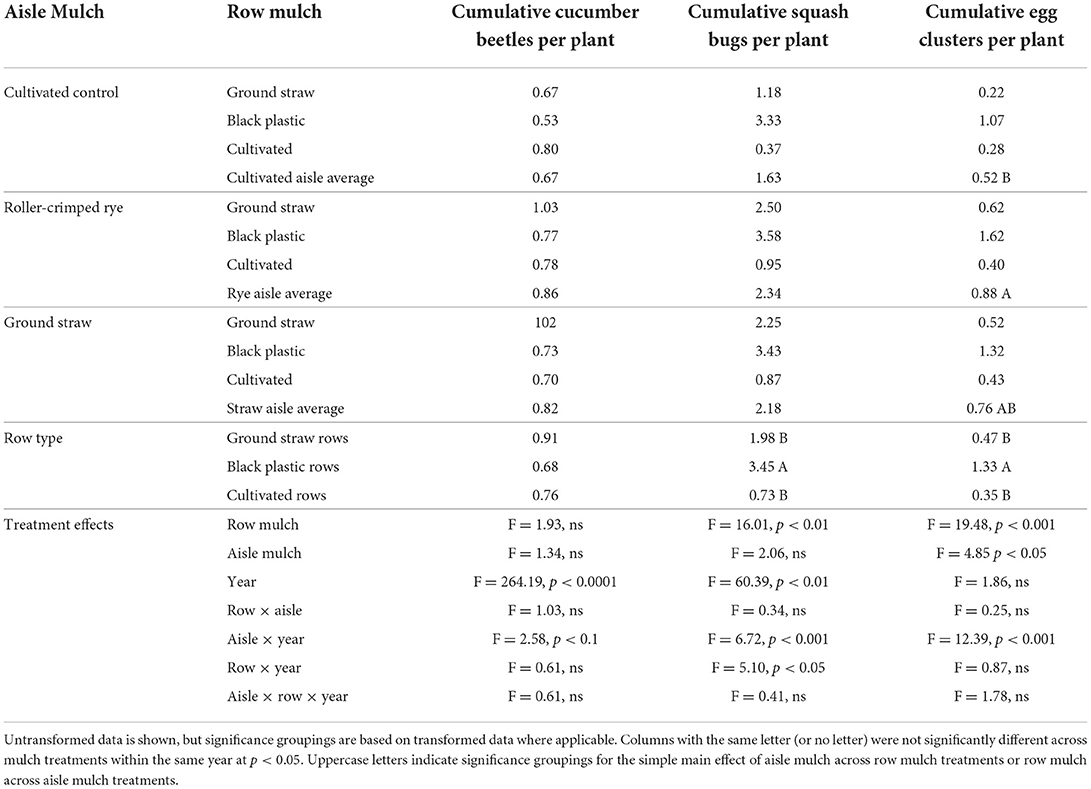
Table 4. Average (2018 and 2019) cumulative cucumber beetle, squash bugs and egg cluster counts by aisle mulch treatment.
Squash bug adults
Significant aisle mulch × year and row mulch × year interactions were observed in explaining squash bug pressure due to lower counts in 2019, with overall effects driven primarily by 2018 (Figure 4). The simple main effect of year was also significant due to the low counts in 2019. Both aisle and row mulches were significant in 2018. Rows with straw and plastic mulch had higher numbers than cultivated rows across aisle mulch levels, while cultivated aisles also resulted in lower numbers than the mulched treatments of ground straw or rye aisles across row mulch levels. Overall, cultivated treatments resulted in lower populations compared to other mulches, and across all aisle mulch treatments rows with plastic mulch consistently resulted in the highest counts.
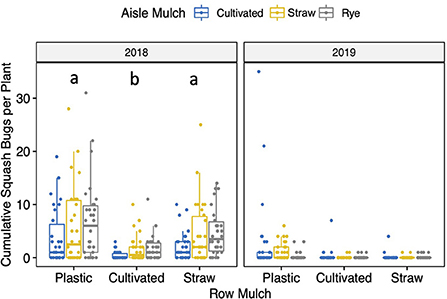
Figure 4. Cumulative numbers of squash bugs per plant by row and aisle mulch, 2018 and 2019. Lowercase letters indicate significance groupings for row mulch across aisle mulch treatments within the same year. Groups with the same letter were not significantly different at p < 0.05.
Squash bug egg clusters
A significant aisle mulch × year interaction explained cumulative squash bug egg cluster counts per plant (Figure 5). The simple main effects of row mulch and aisle mulch were also significant across years. Similar to results for squash bug adults, cultivated treatments had lower egg cluster counts. For row mulches, ground straw performed similarly to cultivation, with lower counts than plastic. In aisles, rye resulted in higher egg cluster counts as compared to cultivation.
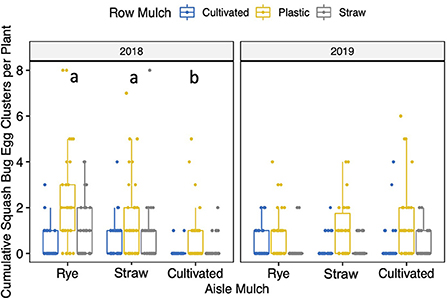
Figure 5. Cumulative squash bug egg cluster counts per plant by aisle and row mulch treatments, 2018 and 2019. Lowercase letters indicate significance groupings for aisle mulch across row mulch treatments within the same year. Groups with the same letter or no letter were not significantly different at p < 0.05.
Results regarding both squash bug adults and their egg clusters are consistent with observations reported by Doughty et al. (2016) who suggested that squash bugs will often be found in the planting holes of plastic mulches, a behavior that could make it difficult for a grower to effectively apply pesticide when needed. While the effect of row mulches was clear, the results of our 2-year study showed inconsistent effects of aisle mulching (either as crimped rye or ground straw) on squash bugs, with 2018 demonstrating greater squash bug pressure with aisle mulching, and 2019 showing no clear effect, when counts were lower across treatments. Habitat provided by mulches may benefit cash crops by promoting within-field natural enemy activity and biological control (Tonhasca and Byrne, 1994; Langellotto and Denno, 2004; Bryant et al., 2013; Hinds and Cerruti, 2013), but our results indicated that the habitat could also benefit pests. Cranshaw et al. (2001) also showed increased damage to pumpkin by squash bugs when using straw or plastic mulches.
The strongest effect was seen from plastic mulch in rows, so applying hay mulch within the tilled planting strip may be a better option than black plastic for growers adopting CCBRT practices for cucurbit production with the potential for high pest pressure. In general, pest abundance on the squash was relatively low in our experimental field during the study period, which may have contributed to the variable response between years.
Weed populations and management time
Aisle weed counts and management time
Cultivated aisles resulted in the highest total, broadleaf, and grass weed counts and required the greatest weed management time inputs (Table 5). Rye aisles resulted in fewer weeds and required less weed management time as compared to cultivated treatments but had significantly more weeds and took longer to manage than straw mulch (Figure 6). There was a significant aisle mulch × year interaction for all weed related data points due primarily to changes in significance level in pairwise comparisons between aisle mulches because of generally higher weed counts in 2019 than 2018, except for higher broadleaf weed counts in 2018 (Supplementary Table 4). There were no significant crossover interactions, except for rye and straw aisle mulches being similar in 2019 for grass weeds. Broadleaf and grass weeds differed in 2018 and 2019 within cultivated aisle treatments, but not total weeds, as more broadleaf weeds were present in 2018 and more grass weeds in 2019. Overall, year was significant for both broadleaf and grass weed counts because of the higher counts in 2018 and 2019, respectively.
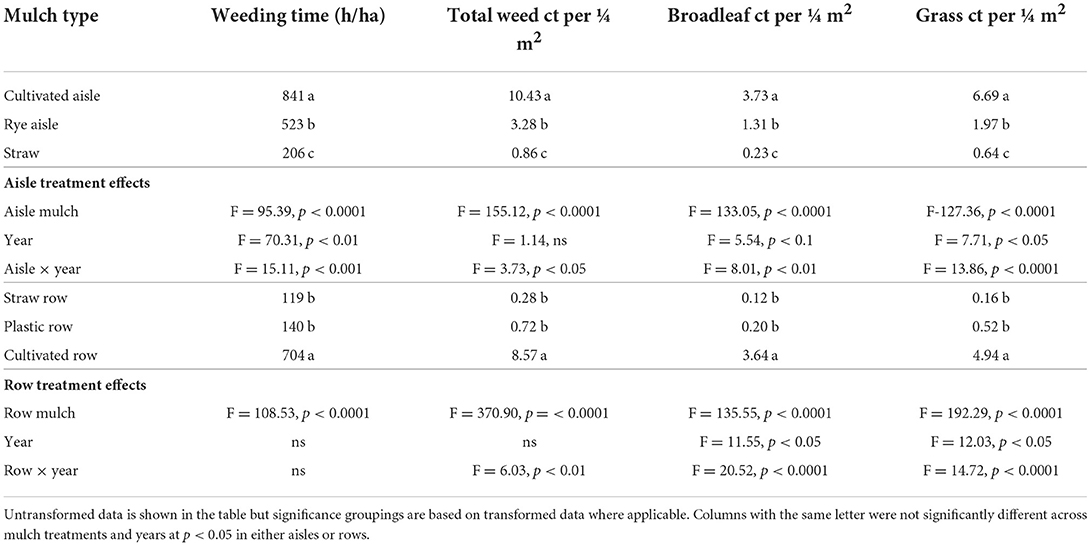
Table 5. Weed counts and management time in 2018 and 2019 relative to row and aisle mulch treatments.
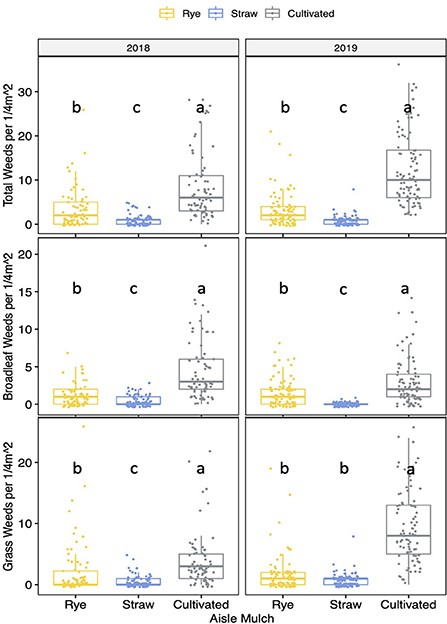
Figure 6. Total (Top), broadleaf (Middle) and grass (Bottom) weed counts per 0.25 m2, 2018 and 2019. Lowercase letters indicate significance groupings for aisle mulch within a given year. Groups with the same letter were not significantly different at p < 0.05.
The effectiveness of the cereal rye treatment with respect to weed suppression was likely influenced by heavy mulch residue created by the rye cover crop. One key factor affecting successful weed suppression of CCBRT systems is the cover crop biomass at termination; cover crop biomass on the soil surface should reach 8–9 Mg ha−1 to obtain satisfactory weed suppression without additional weed control methods, which can include time-consuming and labor-intensive hand-weeding to rescue the vegetable crop from excessive yield loss (Smith et al., 2011; Mirsky et al., 2012; Bietila et al., 2016). In the 2 years of the study, the biomass of cover crop produced reached or nearly reached the threshold needed for adequate weed suppression (mean biomass of 11,756 kg ha−1 in 2018 and 7,866 kg ha−1 in 2019). Lower biomass in 2019 may have contributed to that year's higher weed counts.
While the use of CCBRT techniques in this study did result in fewer weeds as compared to management with cultivation, a small number of weeds were still present in the field throughout the production season. In organic production, crop canopy cover is another important tool for continued weed suppression (Hoad et al., 2012). Variety trials conducted within CCBRT management systems could further optimize the system toward complete elimination of weed seed production; for example, the cultivar in this trial was a semi-bush type, and vining cucurbit cultivars providing greater ground cover which could further contribute to weed suppression, especially during years where cover crop biomass might be lower than the ideal range.
The weed suppression provided by the CCBRT approach translated into fewer weeding hours required for crop management as compared to cultivation. Despite the decreased yields observed in 2018 using the metric of marketable fruit, this approach could still be considered advantageous to farmers, as labor needs across the entire farm during the peak production times of mid-summer can be limiting, and the opportunity costs of not having the ability to use that labor elsewhere on the farm (e.g., harvesting crops or attending a market), as well as the actual costs of the labor, may justify the tolerance of the lower yields.
A notable benefit of CCBRT systems is the potential to reduce erosion during extreme rainfall events, such as the one in late August 2018 at this study site, but if the CCBRT systems are at risk of increasing disease pressure after such events then growers need more information to be able to adequately assess tradeoffs. Previous research suggests that supplementary fertilization could improve vegetable yields in reduced tillage systems, but studies largely focus on either fertigation or sidedressing, rather than comparing approaches and rates within a single study (e.g., Schellenberg et al., 2009; Jokela and Nair, 2016). In addition, the large Rodale-style chevron blade roller-crimpers, such as the one used in this study, rely on weight to effectively crimp, and thus require relatively large tractors with adequate horsepower to operate; testing the efficacy of smaller crimper types, such as those that mount on a walk-behind tractor or do not rely solely on weight as a crimping mechanism (Kornecki and Reyes, 2020) would further elucidate the adaptability of the system to small scale vegetable production.
Row weed counts and management time
Overall, weed counts and management time were higher in cultivated rows than in those mulched with either straw or plastic. Similar to aisle weed counts, a significant effect of year was observed with respect to broadleaf and grass weed counts due to higher counts in 2018 and 2019, respectively, and a significant row mulch × year interaction for cultivated rows was observed due to those higher counts. A crossover interaction for row weed counts was also observed; straw and plastic mulches were equivalent for total and grass weed counts in 2018, but plastic had higher counts than straw in 2019. This interaction was likely due to the overall increased prevalence of grass weeds in 2019, exacerbated by the difficulty of managing weeds at the shoulders of the beds with plastic mulch where exposed soil was present, whereas the in-row straw mulch extended to the rye or straw mulches in aisles.
Mulching with straw resulted in adequate weed suppression and increased the total fruit yield, while avoiding the problems of plastic mulch with respect to increased squash bug pest pressure. Thus, applying straw mulch within the tilled planting strip may be a better option than black plastic (which also resulted in higher pest pressure) for growers adopting CCBRT practices for cucurbit production. Anecdotally, the straw mulch was also easier to apply in combination with rye than it was to dig the plastic mulch in by hand since conventional mulch-layers could not deal with the heavy residue at the edge of the tilled strip.
Conclusions
The primary goal of this study was to evaluate the impact of strip tillage management with CCBRT practices for organic squash production. The data derived from this work demonstrated that the use of CCBRT practices with strip tillage techniques in organic cucurbit systems has the potential to produce overall yields comparable to that of standard organic cucurbit production practices using cultivation, with total fruit m−1 equivalent between approaches in both years and marketable fruit comparable in 2019. This supports the suggestions of previous research that strip tillage in CCBRT systems can be a viable alternative to full tillage systems (Forcella et al., 2015; Tillman et al., 2015; Jokela and Nair, 2016). However, reduced marketable fruits plant−1 and m−1 were observed in 2018 as a result of increased rates of unmarketable fruit in that year, likely influenced by the record-breaking rain event that released 25 cm of precipitation in < 24 h two weeks prior to harvest.
Overall, rolled-crimped management strategies for organic cucurbit management were demonstrated to be a valuable tool for organic vegetable farmers in the upper Midwestern US. However, our research did highlight questions related to the interaction between specific management choices and environmental conditions and the resulting agronomic impacts; providing answers to these questions will reduce risk for growers and drive further adoption of this practice. Thus, future research should focus on understanding the more nuanced management aspects of the system, including the identification of cultivars adapted to reduced tillage systems, supplementary fertilization methods that might result in more reliable yields, and longer term studies that explore disease and pest dynamics (such as the potential for cover crop species to provide alternate hosts for diseases, residue to increase fruit rot incidence by maintaining higher soil moisture, and predator populations and predation of common pests).
Data availability statement
The raw data supporting the conclusions of this article will be made available by the authors upon request, without undue reservation.
Author contributions
ES: conceptualization and funding application. ES and DB: research design. DB: data collection. DB, ES, and JD: data analysis and interpretation, writing manuscript, and editing manuscript. All authors contributed to the article and approved the submitted version.
Funding
This work was supported by the United States Department of Agriculture Organic Research and Extension Initiative Grant #2016-51300-25732.
Acknowledgments
We like to thank Dr. Zsofia Szendrei of Michigan State University for her leadership on the multi-state project of into which this study was integrated. We would also like to thank Janet Hedtcke and the other staff of the West Madison Agricultural Research Station, and the undergraduate field crew, especially Brett Dvorak and Connor McGinley.
Conflict of interest
The authors declare that the research was conducted in the absence of any commercial or financial relationships that could be construed as a potential conflict of interest.
Publisher's note
All claims expressed in this article are solely those of the authors and do not necessarily represent those of their affiliated organizations, or those of the publisher, the editors and the reviewers. Any product that may be evaluated in this article, or claim that may be made by its manufacturer, is not guaranteed or endorsed by the publisher.
Supplementary material
The Supplementary Material for this article can be found online at: https://www.frontiersin.org/articles/10.3389/fsufs.2022.991463/full#supplementary-material
References
All American Selections. (2009). Squash Honey Bear F1. Available online at: https://all-americaselections.org/product/squash-honey-bear/ (accessed July 04, 2022).
Baraibar, B., Hunter, M. C., Schipanski, M. E., Hamilton, A., and Mortensen, D. A. (2018). Weed suppression in cover crop monocultures and mixtures. Weed Sci. 66, 121–133. doi: 10.1017/wsc.2017.59
Bezuidenhout, S. R., Reinhardt, C. F., and Whitwell, M. I. (2012). Cover crops of oats, stooling rye and three annual ryegrass cultivars influence maize and Cyperus esculentus growth. Weed Res. 52, 153–160. doi: 10.1111/j.1365-3180.2011.00900.x
Bietila, E., Silva, E. M., Pfeiffer, A. C., and Colquhoun, J. B. (2016). Fall-sown cover crops as mulches for weed suppression in organic small-scale diversified vegetable production. Renew. Agricult. Food Syst. 32,349. doi: 10.1017/S1742170516000259
Brainard, D. C., Peachey, R. E., Haramoto, E. R., Luna, J. M., and Rangarajan, A. (2013). Weed ecology and nonchemical management under strip-tillage: implications for northern US vegetable cropping systems. Weed Technol. 27, 18–230. doi: 10.1614/WT-D-12-00068.1
Brust, J., Claupein, W., and Gerhards, R. (2014). Growth and weed suppression ability of common and new cover crops in Germany. Crop Prot. 63, 1–8. doi: 10.1016/j.cropro.2014.04.022
Bryant, A., Brainard, D. C., Haramoto, E. R., and Szendrei, Z. (2013). Cover crop mulch and weed management influence arthropod communities in strip-tilled cabbage. Environ. Entomol. 42, 293–306. doi: 10.1603/EN12192
Campiglia, E., Caporali, F., Radicetti, E., and Mancinelli, R. (2010). Hairy vetch (Vicia villosa Roth.) cover crop residue management for improving weed control and yield in no-tillage tomato (Lycopersicon esculentum Mill.) production. Eur. J. Agron. 33, 94–102. doi: 10.1016/j.eja.2010.04.001
Chehade, L. A., Antichi, D., Martelloni, L., Frasconi, C., Sbrana, M., Mazzoncini, M., et al. (2019). Evaluation of the agronomic performance of organic processing tomato as affected by different cover crop residues management. Agronomy 9, 504. doi: 10.3390/agronomy9090504
Clark, A. J., Decker, M., and Meisinger, J. J. (1994). Seeding rate and kill date effects on hairy vetch-cereal rye cover crop mixtures for corn production. Agron. J. 86, 1065–1070. doi: 10.2134/agronj1994.00021962008600060025x
Cranshaw, W., Bartolo, M., and Schweissing, F. (2001). Control of squash bug (Hemiptera: Coreidae) injury: Management manipulations at the base of pumpkin. Southwestern Entomol. 26, 147–150.
Creamer, N. G., Bennett, M. A., Stinner, B. R., and Cardina, J. (1996). A comparison of four processing tomato production systems differing in cover crop and chemical inputs. J. Am. Soc. Horticult. Sci. 121, 559–568. doi: 10.21273/JASHS.121.3.559
De Baets, S., Poesen, J., Meersmans, J., and Serlet, L. (2011). Cover crops and their erosion-reducing effects during concentrated flow erosion. Catena 85, 237–244. doi: 10.1016/j.catena.2011.01.009
Deguchi, S., Uozumi, S., Touno, E., and Kaneko, M. Tawaraya, K. (2012). Arbuscular mycorrhizal colonization increases phosphorus uptake and growth of corn in a white clover living mulch system. Soil Sci. Plant Nutr. 58, 169–172. doi: 10.1080/00380768.2012.662697
Delate, K., Cambardella, C., and McKern, A. (2008). Effects of organic fertilization and cover crops on an organic pepper system. Horttechnology 18, 15–226. doi: 10.21273/HORTTECH.18.2.215
Delate, K., Cwach, D., and Chase, C. (2012). Organic no-tillage system effects on soybean, corn and irrigated tomato production and economic performance in Iowa, USA. Renew. Agricult. Food Syst. 27, 49–59. doi: 10.1017/S1742170511000524
Delate, K., Friedrich, H., and Lawson, V. (2003). Organic pepper production systems using compost and cover crops. Biol. Agricult. Horticult. 21, 131–150. doi: 10.1080/01448765.2003.9755258
Doughty, H. B., Wilson, J. M., Schultz, P. B., and Kuhar, T. P. (2016). Squash bug (Hemiptera: Coreidae): biology and management in cucurbitaceous crops. J. Integrated Pest Manag. 7:1–6. doi: 10.1093/jipm/pmv024
Everts, K. L. (2002). Reduced fungicide applications and host resistance for managing three diseases in pumpkin grown on a no-till cover crop. Plant Dis. 86, 1134–1141. doi: 10.1094/PDIS.2002.86.10.1134
Forcella, F., Eklund, J., and Peterson, D. (2015). Rolled-crimped winter rye cover effects on hand-weeding times and fruit yield and quality of cucurbits. Int. J. Veg. Sci. 21, 386–396.
Ginakes, P., and Grossman, J. M. (2021). Extending cover crop benefits with zone till management in northern organic summer squash production. Agron. 11, 983.
Hiltbrunner, J., Jeanneret, P., Liedgens, M., Stamp, P., and Streit, B. (2007). Response of weed communities to legume living mulches in winter wheat. J. Agron. Crop Sci. 193, 93–102. doi: 10.1111/j.1439-037X.2007.00250.x
Hinds, J., and Cerruti, R. R. H. (2013). Population dynamics of arthropods in a sunn-hemp zucchini interplanting system. Crop Prot. 53, 6–12. doi: 10.1016/j.cropro.2013.06.003
Hoad, S. P., Bertholdsson, N-Ø., Neuhoff, D., and Köpke, U. (2012). “Approaches to breed for improved weed suppression in organically grown cereals,” in Organic Crop Breeding, 61–76.
Jabbour, R., Pisani-Gareau, T., Smith, R. G., Mullen, C., and Barbercheck, M. (2016). Cover crop and tillage intensities alter ground-dwelling arthropod communities during the transition to organic production. Renew. Agric. Food Syst. 31, 361–374. doi: 10.1017/S1742170515000290
Jenkins, D., and Ory, J. (2016). 2016 National Organic Research Agenda. Organic Farming Research Foundation, Santa Cruz CA.
Jokela, D., and Nair, A. (2016). Effects of reduced tillage and fertilizer application method on plant growth, yield, and soil health in organic bell pepper production. Soil Tillage Res. 163, 243–254. doi: 10.1016/j.still.2016.06.010
Kaspar, T. C., Singer, J. W., Hatfield, J. L., and Sauer, T. J. (2011). The Use of Cover Crops to Manage Soil, Vol. 409. Madison, WI: American Society of Agronomy and Soil Science Society of America.
Kornecki, T. S., and Reyes, M. R. (2020). Equipment development for small and urban conservation farming systems. Agriculture 10, 595. doi: 10.3390/agriculture10120595
Laboski, C. A. M., and Peters, J. B. (2019). Nutrient Application Guidelines for Field, Vegetable, and Fruit Crops in WI. A2809. University of Wisconsin Cooperative Extension.
Langellotto, G. A., and Denno, R. F. (2004). Responses of invertebrate natural enemies to complex-structured habitats: a meta-analytical synthesis. Oecologia 139, 1–10. doi: 10.1007/s00442-004-1497-3
Leavitt, M. J., Sheaffer, C. C., Wyse, D. L., and Allan, D. L. (2011). Rolled winter rye and hairy vetch cover crops lower weed density but reduce vegetable yields in no-tillage organic production. HortScience 46, 387–395. doi: 10.21273/HORTSCI.46.3.387
Lenth, R. V. (2022). emmeans: Estimated Marginal Means, aka Least-Squares Means. R package version 1.7.2. Available online at: https://CRAN.R-project.org/package=emmeans (accessed July 04, 2022).
Licht, M. A., and Al-Kaisi, M. (2005). Strip-tillage effect on seedbed soil temperature and other soil physical properties. Soil Tillage Res. 80, 233–249. doi: 10.1016/j.still.2004.03.017
Liebman, M., Gallandt, E. R., and Jackson, L. E. (1997). Many little hammers: ecological management of crop-weed interactions. Ecol. Agric. 1, 291–343. doi: 10.1016/B978-012378260-1/50010-5
Lounsbury, N. P., Warren, N. D., Wolfe, S. D., and Smith, R. G. (2020). Investigating tarps to facilitate organic no-till cabbage production with high-residue cover crops. Renew. Agric. Food Syst. 35, 227–233.
Lounsbury, N. P., and Weil, R. R. (2015). No-till seeded spinach after winterkilled cover crops in an organic production system. Renew. Agric. Food Syst. 30, 473–485. doi: 10.1017/S1742170514000301
Lowry, C. J., and Brainard, D. C. (2019). Organic farmer perceptions of reduced tillage: A Michigan farmer survey. Renew. Agric. Food Syst. 34, 103–115.
Luna, J. M., Mitchell, J. P., and Shrestha, A. (2012). Conservation tillage for organic agriculture: evolution toward hybrid systems in the western USA. Renew. Agric. Food Syst. 27, 21–30. doi: 10.1017/S1742170511000494
Luo, Z., Wang, E., and Sun, O. (2010). Can no-tillage stimulate carbon sequestration in agricultural soils? A meta-analysis of paired experiments. Agric. Ecosyst. Environ. 2010, 224–231. doi: 10.1016/j.agee.2010.08.006
Magdoff, F., and Van Es, H. (2000). Building Soils for Better Crops (No. 631.584/M188b). Beltsville: Sustainable Agriculture Network.
Maglione, R., Ciotola, M., Cadieux, M., Toussaint, V., Laforest, M., and Kembel, S. W. (2022). Winter rye cover cropping changes squash (Cucurbita pepo) phyllosphere microbiota and reduces Pseudomonas syringae symptoms. Phytobiomes J. 6, 3–12. doi: 10.1094/PBIOMES-04-21-0029-R
Mirsky, S. B., Ryan, M. R., Curran, W. S., Teasdale, J. R., Maul, J., Spargo, J. T., et al. (2012). Conservation tillage issues: cover crop-based organic rotational no-till grain production in the mid-Atlantic region, USA. Renew. Agric. Food Syst. 27, 31–40. doi: 10.1017/S1742170511000457
Mochizuki, M. J., Rangarajan, A., Bellinder, R. R., Björkman, T., and van Es, H. M. (2007). Overcoming compaction limitations on cabbage growth and yield in the transition to reduced tillage. HortScience 42, 1690–1694. doi: 10.21273/HORTSCI.42.7.1690
Mochizuki, M. J., Rangarajan, A., Bellinder, R. R., van Es, H. M., and Björkman, T. (2008). Rye mulch management affects short-term indicators of soil quality in the transition to conservation tillage for cabbage. HortScience 43, 862–867. doi: 10.21273/HORTSCI.43.3.862
Moynihan, M. (2010). Status of Organic Agriculture in Minnesota. Available online at: https://www.leg.mn.gov/docs/2010/mandated/100851.pdf (accessed July 04, 2022).
MRCC (2021). (1978-2019) Daily precipitation and temperatures - UW-Madison Arboretum Station, Midwestern Regional Climate Center.
Pinheiro, J., and Bates, D. R. (2022). nlme: Linear and Nonlinear Mixed Effects Models. R package version 3.1-157. Available online at: https://CRAN.R-project.org/package=nlme (accessed July 4, 2022).
R Core Team. (2021). R.app GUI 1.4 “Juliet Rose” (df86b69e, 2021-05-24). R: A language and environment for statistical computing. R Foundation for Statistical Computing, Vienna, Austria. Available online at: https://www.R-project.org/ (accessed July 04, 2022)
Reicosky, D. C., and Forcella, F. (1998). Cover crop and soil quality interactions in agroecosystems. J. Soil Water Conserv. 53, 224–229.
Ryder, M. H., and Fares, A. (2008). Evaluating cover crops (Sudex, Sunn Hemp, Oats) for use as vegetative filters to control sediment and nutrient loading from agricultural runoff in a Hawaiian watershed. J. Am. Water Resour. Assoc. 44, 640–653. doi: 10.1111/j.1752-1688.2008.00189.x
Sarrantonio, M. (1992). Opportunities and challenges for the inclusion of soil-improving crops in vegetable production systems. HortScience, 27, 754–758.
Sarrantonio, M., and Gallandt, E. (2003). The role of cover crops in North American cropping systems. J. Crop Prod. 8, 53–74. doi: 10.1300/J144v08n01_04
Schellenberg, D. L., Morse, R. D., and Welbaum, G. E. (2009). Organic broccoli production on transition soils: comparing cover crops, tillage and sidedress N. Renew. Agric. Food Syst. 2, 85–91. doi: 10.1017/S1742170508002470
Silva, E. M. (2014). Screening five fall-sown cover crops for use in organic no-till crop production in the Upper Midwest. Agroecol. Sust. Food Syst. 38, 748–763. doi: 10.1080/21683565.2014.901275
Silva, E. M., and Delate, K. (2017). A decade of progress in organic cover crop-based reduced tillage practices in the upper midwestern USA. Agriculture 7, 44. doi: 10.3390/agriculture7050044
Skidmore, A., Wilson, N., Williams, M., and Bessin, R. (2019). The impact of tillage regime and row cover use on insect pests and yield in organic cucurbit production. Renew. Agric. Food Syst. 34, 338–348.
Smith, A. N., Reberg-Horton, S. C., Place, G. T., Meijer, A. D., Arellano, C., and Mueller, J. P. (2011). Rolled rye mulch for weed suppression in organic no-tillage soybeans. Weed Sci. 59, 224–231. doi: 10.1614/WS-D-10-00112.1
Snapp, S.S., Swinton, S.M., Labarta, R., Mutch, D., Black, J.R., Leep, R., et al. (2005). Evaluating cover crops for benefits, costs and performance within cropping system niches. Agron J. 97, 322–332.
Snyder, W. E. (2015). Managing Cucumber Beetles in Organic Farming Systems. Department of Entomology, Washington State University Pullman, WA, United States. Available online at: https://eorganic.org/node/5307/
Sportelli, M., Frasconi, C., Fontanelli, M., Pirchio, M., Gagliardi, L., Raffaelli, M., et al. (2022). Innovative living mulch management strategies for organic conservation field vegetables: evaluation of continuous mowing, flaming, and tillage performances. Agronomy 12, 622. doi: 10.3390/agronomy12030622
Teasdale, J. R., and Mohler, C. L. (2000). The quantitative relationship between weed emergence and the physical properties of mulches. Weed Sci. 48, 385–392. doi: 10.1614/0043-1745(2000)0480385:TQRBWE2.0.CO;2
Teasdale, J. R., Rice, C. P., Cai, G., and Mangum, R. W. (2012). Expression of allelopathy in the soil environment: soil concentration and activity of benzoxazinoid compounds released by rye cover crop residue. Plant Ecol. 213, 893–1905. doi: 10.1007/s11258-012-0057-x
Thomas, R., O'Sullivan, J., Hamill, A., and Swanton, C. J. (2001). Conservation tillage systems for processing tomato production. HortScience 36, 1264–1268. doi: 10.21273/HORTSCI.36.7.1264
Tillman, J., Nair, A., Gleason, M., and Batzer, J. (2015). Evaluating strip tillage and rowcover use in organic and conventional muskmelon production. Horttechnology 25, 487–495. doi: 10.21273/HORTTECH.25.4.487
Tonhasca, A. Jr., and Byrne, D. N. (1994). The effects of crop diversification on herbivorous insects: a meta-analysis approach. Ecol. Entomol. 19, 239–244. doi: 10.1111/j.1365-2311.1994.tb00415.x
USDA-NASS (2019). 2019 Organic Survey (2017 Census of Agriculture Special Report). United States Department of Agriculture – National Agricultural Statistics Service. Available online at: https://www.nass.usda.gov/Surveys/Guide_to_NASS_Surveys/Organic_Production/ (accessed on July 04, 2022).
Van Den Bossche, A., De Bolle, S., De Neve, S., and Hofman, G. (2009). Effect of tillage intensity on N mineralization of different crop residues in a temperate climate. Soil Tillage Res. 103, 316–324. doi: 10.1016/j.still.2008.10.019
Vincent-Caboud, L., Casagrande, M., David, C., Ryan, M. R., Silva, E. M., and Peigne, J. (2019). Using mulch from cover crops to facilitate organic no-till soybean and maize production. A review. Agron. Sust. Dev. 39, 1–15. doi: 10.1007/s13593-019-0590-2
Vollmer, E. R., Creamer, N., Reberg-Horton, C., and Hoyt, G. (2010). Evaluating cover crop mulches for no-till organic production of onions. HortScience Horts. 45, 61–70. Available online at: https://journals.ashs.org/hortsci/view/journals/hortsci/45/1/article-p61.xml
Keywords: organic agriculture, cover crop-based reduced tillage, acorn squash, cover crops, crimped rye, no till vegetables
Citation: Bruce D, Silva EM and Dawson JC (2022) Cover crop-based reduced tillage management impacts organic squash yield, pest pressure, and management time. Front. Sustain. Food Syst. 6:991463. doi: 10.3389/fsufs.2022.991463
Received: 11 July 2022; Accepted: 14 September 2022;
Published: 08 November 2022.
Edited by:
E. Britt Moore, University of North Carolina Wilmington, United StatesReviewed by:
Vesna Radovanović, Educons University, SerbiaShah Fahad, The University of Haripur, Pakistan
Copyright © 2022 Bruce, Silva and Dawson. This is an open-access article distributed under the terms of the Creative Commons Attribution License (CC BY). The use, distribution or reproduction in other forums is permitted, provided the original author(s) and the copyright owner(s) are credited and that the original publication in this journal is cited, in accordance with accepted academic practice. No use, distribution or reproduction is permitted which does not comply with these terms.
*Correspondence: Erin M. Silva, emsilva@wisc.edu