- 1Dairy Department, Food Industries and Nutrition Research Institute, National Research Centre, Cairo, Egypt
- 2Food and Dairy Science and Technology Department, Faculty of Agriculture, Damanhour University, Damanhour, Egypt
Carrot industry processing outputs 50% waste from raw materials; this waste contains polyphenols and carotenoids, which are a significant natural source of pro-vitamin A. Also, yogurt's high consumption globally allows for designing a new functional product. So the goal is to enhance the functionality of fortified stirred yogurt by incorporating carotenoid beads. The carotenoids were extracted from carrot waste using ultrasonication. Then nanoemulsion carotenoids incorporating with alginate to produce beads by extrusion technique. Measurement of carotenoid stability to nanoemulsion and beads. Manufactured five treatments of orange-flavored stirred yogurt and investigated its physicochemical properties, LAB survival, viscosity, and sensory acceptability. Findings – Carrot waste extract had about 44.75 ± 3.15 mg/g of β-carotene. The mean particle size of the nanoemulsion decreased with the increasing carotenoid addition (0.5%, 1%, and 1.5%) of carrot waste extract. The mean diameters of the alginate beads with nanoemulsions were 1.498 ± 0.245, 1.654 ± 0.310, and 1.792 ± 0.454 mm, respectively. The highest chemical stability of carotenoids showed with the alginate beads after Storage at 55°C to 14 days, compared with free or nanoemulsion carotenoids. Yogurt's physicochemical properties, viscosity, and LAB count improve when double-encapsulated carotenoids are added. Carotenoid double-encapsulation appeared to have a high ability to protect carotenoids from degradation and the ability to be applied in dairy and pharmaceutical products. Also, the resultant stirred yogurt with carotenoids-loaded beads gave carotenoids high stability and sensory acceptability.
Introduction
In recent decades, global trends in the manufacturing of functional foods have focused on developing nutrient-rich, health-improving products which include a suitable source of bioactive ingredients (Vulić et al., 2019; Banwo et al., 2021). Furthermore, the growth of the food business generates a substantial quantity of waste materials or particular by-products, the conversion of which into commercially viable goods is supported by the notion of “bioeconomy”. Recent research indicates that fruit and vegetable processing waste contains valuable molecules (antioxidants, proteins, natural colorants, dietary fibers, aroma compounds, etc.). Which can be extracted, purified, concentrated, and reused as functional ingredients in food, pharmaceutical, cosmetic, and healthcare products (Ravindran and Jaiswal, 2016; Brandão et al., 2021). Yogurt and fortified yogurt make up more than 70% of the functional food industry (Taksima et al., 2015; Sarkar, 2018). Standard yogurt is a fermented dairy product with distinguished texture and an acceptable tender taste, resulting from fermentation with Lactobacillus delbrueckii ssp. bulgaricus and Streptococcus thermophilus. Consumers favor yogurt because it improves the digestive tract and enhances immunity (Nontasan et al., 2012; Yildiz, 2016). Yogurt is an excellent nutritional vehicle for effectively delivering beneficial bacteria and bioactive ingredients into the human body (Gómez-Gallego et al., 2018). Yogurt stored for a long time without the proper protection may lose some vitamin A and C content. So, yogurt needs to be supplemented with other bioactive components to make up for or add to the lost vitamins. Adding carrot waste extract [Daucus carota (L.)], especially encapsulated, is one of the worthwhile substances to try in yogurt manufacturing (Šeregelj et al., 2017).
Carotenoids are a significant natural source of provitamin A and antioxidant components (Šeregelj et al., 2017), which are ideal associated with some health benefits, such as the inhibition of LDL oxidation, alleviation of oxidative stress, anti-inflammatory properties, and enhancement of immune response (Kiokias and Gordon, 2004; Šeregelj et al., 2020). Carotenoids are distinguished by their nonpolar structure and highly unsaturated molecules, so they have low water-solubility, poor chemical instability, and low bioaccessibility. Also, more liable to oxidation and isomerization during treatments or storage, resulting in the loss of its bioactive properties and organoleptic attributes (Sy et al., 2015; Xavier and Mercadante, 2019). In addition, carotenoids may undergo chemical changes in the gastrointestinal tract (GIT), affecting their potential health advantages (Donhowe and Kong, 2014). Consequently, carotenoid delivery systems need to develop to overcome these challenges (Donhowe and Kong, 2014). Colloidal delivery systems are one of the most practical ways to include hydrophobic bioactives (like carotenoids) into foods (Patel and Velikov, 2011; McClements, 2012).
They are encapsulated in tiny colloidal particles made of lipids, phospholipids, surfactants, or biopolymers (Patel and Velikov, 2011; McClements, 2014).
Solubilizing the lipophilic bioactive components in an oil phase and then homogenizing them with an emulsifier solution may produce typical bioactive-loaded colloidal systems (Marze, 2015).
One disadvantage of employing emulsions or nanoemulsions as delivery vehicles is that the chemical stability of encapsulated carotenoids is challenging to control. Because the lipid droplets are only coated with a thin layer of emulsifier molecules, any carotenoids at the droplet surfaces are vulnerable to chemical destruction caused by hydrophilic components in the aqueous phase (such as acids, transition metals, or enzymes) (Qian et al., 2012; Choi and McClements, 2020).
The carotenoid-loaded lipid droplets might be trapped inside hydrogel beads (“microgels”) to solve this problem. The hydrogel matrix that surrounds the carotenoids might then be engineered to create a protective environment for them. Many techniques and food-grade polymers, such as proteins and polysaccharides, produce hydrogel beads applicable in food systems (Zhang et al., 2016). Nutraceuticals and other active substances may be encapsulated, protected, and delivered using the injection-gelation technique, one of the most straightforward methods available (McClements, 2017).
The present study aimed to use carotenoids from carrot waste extract to prepare nanoemulsion (oil-in-water). Through carotenoids-loaded olive oil, droplets were used as delivery systems in sodium caseinate as emulsifier, then incorporated into alginate hydrogel microbeads. The chemical stability of carotenoids was compared between either encapsulated within nanoemulsions or filled hydrogel beads. Also, investigate the possibility of developing fortified Orange Flavored Stirred Yogurt by including carotenoid beads in the traditional formulation. Furthermore, functional orange-flavored stirred yogurt was evaluated during the yogurt shelf life.
Materials and methods
Materials
Sodium caseinate was obtained from Sigma/Aldrich (St. Louis, MO, USA); Olive and orange oils were obtained as pure (crude) oils from the oil extraction unit in National Research Center (NRC), Dokki, Cairo, Egypt. Alginic acid (sodium salt) was purchased from the Sigma Chemical Company (St. Louis, MO). Fresh buffalo's milk was obtained from the Dairy Industry Unit, Animal Production Research Institute, Ministry of Agriculture (Dokki, Cairo, Egypt), with an average composition of 17.69 ± 0.23 % total solids, 6.85 ± 0.05 % fat, 4.47 ± 0.05 % protein, 0.78 ± 0.03% ash,5.59 ± 0.10 % lactose, 0.15 ± 0.05% acidity. Commercial lyophilized DVS mixed bacterial starters: Yo Fast1 containing Lactobacillus (Lb.) delbrueckii ssp. Bulgaricus and Streptococcus thermophiles as a yogurt starter were supplied by the Chr-Hansen company (Horsholm, Denmark). Bacterial starter (freeze-dried) were activated separately in sterilized (121EC/10 min) skimmed cow's milk (0.1% fat and 10% SNF) using 0.02% (W/V) inoculums. The activated cultures were used for the inoculum of the buffalo's milk. All chemicals were of analytical grade.
Methods
Preparation of carrot pomace powder
Purchase high-quality Carrot (Daucus carota L.) from a local market in Giza, Egypt. Carrots were cleaned and chopped with a knife by hand. Carrot pomace was obtained using the MJ-M176P home juice extractor (Panasonic Manufacturing Berhad, Malaysia). The freeze-drying was done in a lab-scale freeze-dryer (Labconco Equipment Co., Kansas City, MO, USA). The temperature (40°C) and pressure (10 5 Pa) were automatically adjusted. The raw material was held in the freezer for 24 h before freeze-drying at −80°C. Distribute the raw material at a weight of 4.0 kg m2 on the dryer plates. The dried pomace was coarsely pulverized using a blade grinder after freeze-drying and then kept in a desiccator until further usage.
Ultrasound-assisted extraction
UAE of the carotenoids from carrot pomace was carried out in an ultrasonic processor of 750W (Sonic Vibra cell the USA) at a frequency and amplitude level of 20 kHz 70%, respectively, by Extraction using ethanol 55%, at 35°C/ 20 min. The dried ground pomace sample was placed in a 300 mL jacketed vessel at 5 g/250 mL. The temperature was automatically controlled, and when the temperature reached 35°C, the sonication was started. After Extraction, the filtration of the samples was performed with filter papers (Whatman No. 41 paper), and the extracts were centrifuged at 4000 rpm for 10 min. Then, evaporation was conducted at 30°C to remove the solvent, and the waste extract was stored at 4°C in micro-tubes until further use to determine total carotenoids (the method for determining the analytes is detailed below). These are perfect conditions, as described by Umair (Umair et al., 2021).
HPLC
Preparation of carotenoids-loaded nanoemulsions
Nanoemulsion was prepared by dispersing 1% (w/w) sodium caseinate in phosphate buffer solution (pH 7.0, 10 mM). The β-carotene-loaded lipid was prepared by dispersing different ratios of carotenoids extract (3.00, 4.5, and 6.00 grams equal to 1200, 1800, and 2400 mg β-carotene) respectively in olive oil by heating (55°C, 10 min) and then ultrasonic processing (2 min) with the vibrational frequency of 40 kHz. This procedure was repeated several times to ensure the complete dissolution of carotenoids in the oil phase and then added orange oil was at 1%. Coarse emulsions were prepared by mixing an aqueous phase (90%, w/w) with an oil phase (10%, w/w) using a high-speed blender (T25 digital ULTRA-TURRAX, IKA, Germany) at 12000 rpm for 3 min. Emulsions were then further homogenized using an ultra-sonication 160 W power, 40 kHz frequency, and 60% pulse (Sonic Vibra cell USA) for 15 min with 20 sac time intervals. Increases in temperature during ultra-sonication were minimized by placing the sample container in a bigger beaker containing ice. Before use, the resulting β-carotene-loaded emulsions were stored in a refrigerator at 4°C (Liu et al., 2019).
Fatty acid composition
To determine the fatty acid composition of the tested oils, fatty acid methyl esters were prepared according to AOAC (AOAC, 2016). Determination of fatty acids composition was carried out according to Hamed et al. (2019) using a Hewlett Packard HP 6890 gas chromatograph, operated under the following conditions: Detector, flame ionization (FID); column, capillary, 30.0 m X530 μm, 1.0 μm thickness, polyethylene glycol phase(INNO Wax); N2 with flow rate, 15 ml/ min with average velocity 89 cm/s (8.2 psi); H2 flow rate, 30 ml/min; air flow rate, 300 ml/min; split ratio, 8:1, split flow, 120 ml/min; gas saver, 20 ml/min. Detector temperature,280°C; column temperature, 240°C; injection temperature, 280°C. Programmed temperature starting from 100°C to a maximum of 240°C was used to elute the fatty acid methyl esters. The peaks were identified as compared with chromatograms of standard fatty acids methyl esters (Sigma, USA).
Particle size and ζ-potential measurement for nanoemulsion
The mean particle size and ζ-potential of each nanoemulsion were determined as described previously by Hamed et al. (2019) using a Zetasizer (Nano-ZS, Malvern Instruments, UK). Before measurement, samples were diluted with phosphate buffer solution (10 mM, pH 7).
Encapsulation of β-carotene in alginate beads
Aqueous solutions containing alginic acid (1% w/w) dissolve in distilled water, stirring continuously at 60°C for 1 h, then reducing the temperature to 35°C. Alginate solutions and carotenoids-loaded emulsions were then mixed (1:1, mass ratio) for 2 h with continuous stirring to form a mixture that contained 10% oil (w/w) and either 0.5% alginate (w/w). The carotenoids-loaded hydrogel beads were prepared using a commercial encapsulation unit (Encapsulator B-390, BUCHI, Switzerland) with a 150 μm vibrating nozzle to inject the β-carotene/alginate solution into a 10% calcium chloride solution. The encapsulation device was operated under fixed conditions: 800 Hz; electrode 800 V; and pressure 450 mbar. The hydrogel beads were held in the Ca+2 solution for 1h at ambient temperature to promote crosslinking. The beads were then collected by filtration and washed with distilled water and phosphate buffer to remove any excess ions from their surfaces. The formed beads were then stored in a refrigerator to remove any residual external water, and then their total weight was determined (McClements, 2017).
Measurement of carotenoids stability
The physico-chemical stability of encapsulating carrot waste extract (carotenoids) in the nanoemulsions and the filled hydrogel particles were examined. Four mL of nanoemulsions (10% oil) or 4 mL filled hydrogel beads (5% oil) were transferred into glass tubes and stored in the dark at 55°C for 14 days (pH 7). The carotenoids were isolated from the samples using a solvent extraction method: each sample was extracted by chloroform and alcohol (volume fraction 1:1) solution at least twice. For the filled hydrogel beads, saturated ethylenediaminetetraacetic acid (EDTA) solution was used to dissolve the beads and release the encapsulated carotenoids. The transparent lower organic phase containing the carotenoids was collected. The carotenoids loaded the microbead measured according to Umair et al. (2021) using an Agilent 1100 series HPLC diode array detection (DAD) system with Agilent Zorbax Eclipse XDB-C18 Column (4.6 × 150 mm, with 5 μm particle size, USA).
Prepared orange-flavored stirred yogurt
For prepared orange-flavored stirred yogurt, using carotenoids beads that have the best results of high encapsulation efficiency, prepared by 1 g carotenoids extract in nanoemulsion and 0.5 % sodium alginate (containing 450 μg of β-carotene/g beads). Buffalo milk was heated to 85–90°C for 5 min, cooling to 42 °C. It was inoculated with 3% of starter cultures, then incubated at 42°C for 3 h until the formation curd. The curd was refrigerated at 5°C overnight before being fortified with the Flavoreded of orange, then divided into five portions. The first portion orange-flavored stirred yogurt without addition control (C), the second portion fortified with 5 g micro-beads carotenoids (T1), the third portion fortified with 10 g micro-beads of carotenoids (T2), the fourth portion fortified with 2.2 mg free carotenoids (T3), the final portion fortified with 4.4 mg free carotenoids (T4), after that, dispensed all portions into a plastic container (125 ml). The plastic containers were stored in a refrigerator at 5 ± 1°C, and their chemical, rheological, and sensory properties were evaluated during storage (Darwish et al., 2021).
Physicochemical properties of stirred yogurt
The pH of stirred yogurt samples was measured using a pH meter and ATC probe combination (model IQ 240, IQ Scientific Instruments Inc., San Diego, Calif., USA). Also, measured acidity according to Feldsine et al. (2002). The apparent Viscosity of the stirred yogurt was measured according to Shehata and Soliman (2021) using a Bohlin coaxial cylinder viscometer (Bohlin Instrument Inc., Sweden) attached to a workstation loaded with the software of the V88 viscometery programme. The viscometer probe, system C30, was placed in the yogurt sample cup, and viscosity measurements were carried out at 20°C ± 2°C in the up mode at shear rates ranging from 37 to 1238 1/s.
Antioxidant activity of stirred yogurt
Antioxidant capacity was evaluated by radical scavenging capacity assay and cation radical scavenging capacity test.
DPPH activity
The radical scavenging capacity estimation of each sample was performed using 2,2-diphenyl-1- picrylhydrazyl radical (DPPH•, Sigma Aldrich) according to the method of Zaky et al. (2020). 500 μL was taken from each sample, mixed with DPPH• reagent (0.1 mM, 1,000 μL) for 30 min. Each mixture was read at 517 nm in a double-beam Ultraviolet-Visible (UV-Vis) spectrophotometer (Thermo Scientific, AQ8000, USA).
ABTS assay
The cation radical scavenging capacity of each sample was estimated using the cation radical 2,2'-azinobis (3-ethyl benzothiazoline 6-sulfonic acid) (ABTS+•) according to the method of Zaky et al. (2019). From each sample, 30 μL was taken, and mixed with the reagent ABTS+• (Aλ-754: 0.7, 1470 μL) for 5 min. Each mixture was read at 754 nm in the spectrophotometer.
Sensory evaluation
Stirred yogurt samples were judged for flavore (45 points), body and texture (40 points), and color and appearance (15 points) by 15 panelists from the staff members of the Dairy Department, National Research Center, Dokki, Cairo, Egypt. The scorecard was designed as described by Shehata and Soliman (2021).
Statistical analysis
All measurements were made on at least triplicate prepared samples. The results are reported as averages and standard deviations. The difference among treatments was calculated based on an analysis of variance (ANOVA) and a post-hoc Duncan test with a confidence level of 95%. These analyses were carried out using statistical software (SPSS, version 22.0.; IBM Corporation, Armonk, NY, USA).
Results
Fatty acid composition of olive oil and solubility of carotenoids
Lipids' capacity to solubilize lipophilic bioactive components vary with fatty acid content. The fatty acid content of the olive oil was first determined. According to Table 1, the olive oil mostly consisted of long-chain fatty acids, such as C18:1 (oleic acid, 67.22%) and C18:2 linoleic acid, 8.07%. In contrast, Carotenoids are often lipophilic owing to the existence of long unsaturated aliphatic chains. In humans and other species, the physiological absorption of these fat-soluble vitamins directly depends on the availability of fats and bile salts (Bertuccioli and Monteleone, 2014).
High-performance liquid chromatography of carrot waste extract
β -carotene and lutein estimation using high-performance liquid chromatography (HPLC) The carotenoids are the main lipophilic antioxidants in carrot waste extract. The conventional -carotene and lutein chromatograms showed retention times of 14.85 and 14.43 min, respectively (Figure 1). Carrot waste extract had about 44.75 ± 3.15 mg/g of β-carotene and 0.07 ± 0.01 mg/g of lutein, respectively. On the other hand, fresh carrot has roughly 71 ppm of -carotene, according to (Mustafa et al., 2012).
Characterization of nanoemulsion carrot waste extract (carotenoids)
Emulsifiers' emulsifying ability is determined by the diameter of the floating oil droplets, whether they precipitate or not, and the oil-water separation.
Table 2 shows the properties of the carotenoids-olive oil nanoemulsion. The sodium caseinate-prepared carotenoids-olive oil nanoemulsion exhibited a small particle size and a homogeneous distribution, suggesting that the caseinate had good emulsifying properties. The mean particle size of the nanoemulsion had decreased with the increasing carotenoid addition (0.5, 1, and 1.5%) of 227.5, 139.3, and 72.31 nm, respectively. The PDI indexes were 0.442, 0.469, and 0.532, respectively. The nanoemulsion with 1.5% carrot waste extract (carotenoids) had the smallest diameter and most electronegativity. It could result from the high sodium caseinate electronegativity and carotenoid hydrophobic linkages. For the manufacture of O/W nano-emulsions, (Jo and Kwon, 2014) found that emulsifiers with small molecular weights and high hydrophilic-lipophilic balancing values were beneficial. Furthermore, (Charoen et al., 2011; Ozturk and McClements, 2016) discovered that sodium caseinate low molecular weight (10–50 kDa), high electronegativity, and gelatinization capacity, as well as a thick interfacial layer, increased mutual repulsion between oil droplets and reduced flocculation and aggregation, resulting in emulsifying stability. According to Pilosof (2017), sodium caseinate electronegativity and emulsion interface thickness significantly influence emulsion stability. According to (Charoen et al., 2011), increasing the surface charge of the emulsion improved repulsive forces between the droplets, which prevented flocculation and accumulation and was beneficial to oil-water interface stability.

Table 2. Effect of carrot carotenoids on particle size, pdI, and zeta potential of olive oil nanoemulsion.
The most common method for determining the surface charge is the zeta potential. In colloidal systems, zeta potential is the electrical potential that determines colloidal stability in dispersion, macromolecule stability, and material surface stability (Honary and Zahir, 2013). Nanoparticles composition and dispersion media affect this characteristic (Wu et al., 2011). The electrophoretic mobility that correlates to the border of the surrounding liquid layer connected to the moving particles in the medium is used to calculate the zeta potential (Wu et al., 2011). Higher and lower than 30 mV values encourage excellent stability and inhibit particle aggregation (Honary and Zahir, 2013). ζ-potential of the nanoemulsion had decreased with the increasing carotenoid addition (0.5, 1, and 1.5%) of −30.10 ± 3.04, −34.0 ± 4.45, and −35.84 ± 5.31 mv, respectively.
Transmission electron microscope
Figure 2 shows the TEM micrograph of carotenoid nanoemulsion. The surface morphology of nanoemulsion containing carotenoid ratios confirmed the formation of spherical particles and homogeneous dispersion. The TEM image showed that the carotenoid nanoemulsions were slightly homogeneous shaped and particle sizes for different ratios of Carotenoid ranged between 48.23 and 104.24 nm. The particle diameter decreased by increasing carotenoid content; these results agree with the data trend of DLS. But the particle size is less than DLS due to the vacuum treatment when preparing the sample for imaging by TEM.
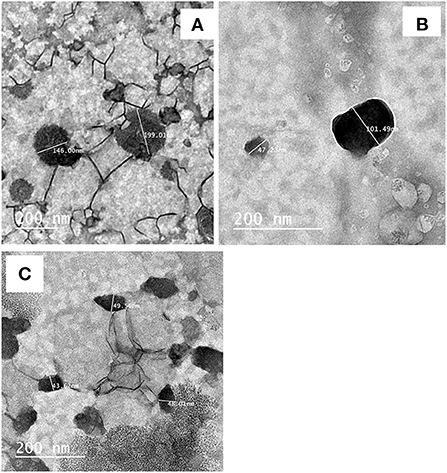
Figure 2. Transmission electron microscopy of carotenoid nanoemulsion. (A) Nanoemulsion containing 0.5% carrot extract; (B): nanoemulsion containing 1% carrot extract; (C): nanoemulsion containing 1.5% carrot extract.
Formation of filled hydrogel beads by carotenoids and its chemical stability
An extrusion apparatus with a vibrating nozzle generated filled hydrogel beads by injecting a combination of nanoemulsions and 0.5% alginate solution into a Ca2 solution. Three different carotenoid concentrations (0.5, 1, and 1.5) of nanoemulsions with 0.5% alginate was used to make hydrogel beads with different characteristics, sizes, and appearances. The mean diameters of the Carotenoid (0.5, 1, and 1.5) beads are 1.498 ± 0.245, 1.654 ± 0.310, and 1.792 ± 0.454 mm, respectively. These findings suggest that the physical characteristics and dimensions of the beads do not change among treatments but rather are dependent on alginate concentration. The small size of the beads might be due to the alginate solution's decreased Viscosity, which made bead production easier. The fact that alginate beads went higher shows that the oil droplets' (low-density) decline in bead density was larger than the alginate molecules' (high density) rise in density (Matalanis and McClements, 2013).
Gao and Kispert (2003) found carotenoids have a high degree of unsaturation. So, it is known to oxidize under certain conditions. Consequently, we examined the chemical stability of carotenoids for encapsulated loaded lipid droplets in filled hydrogel beads and nanoemulsions. The decrease in carotenoid concentration during storage for 14 days at 55°C compared to the initial carotenoid value was measured for the different delivery systems (Figure 3).
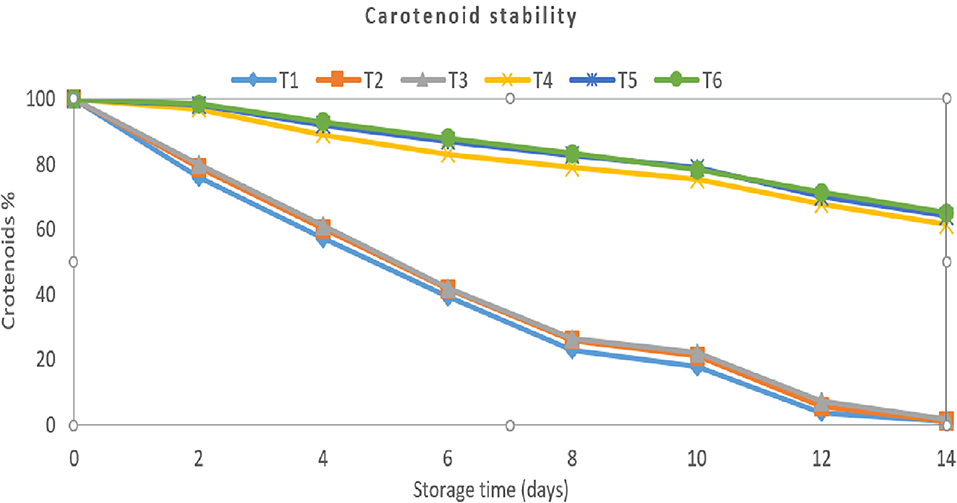
Figure 3. Carotenoid degradation rates in two delivery methods (nanoemulsions and hydrogel beads) during storage (14 days) at 55°C. T1: nanoemulsion containing 0.5 % carotenoid; T2: nanoemulsion containing 1 % carotenoid, T3: nanoemulsion containing 1.5 % carotenoid, T4: hydrogel beads containing 0.5% carotenoid nanoemulsion, T5: hydrogel beads containing 1% carotenoid nanoemulsion, T6: hydrogel beads containing 1.5% carotenoid nanoemulsion.
After 14 days of storage at 55°C, the relative carotenoid content decreased from 100% immediately after production to approximately 0.98, 1.13, 1.54, and 61.35, 64.15, and 65.08% for the 0.5, 1, and 1.5 % carotenoid loaded-nanoemulsion and 0.5, 1, and 1.5 % carotenoid loaded-hydrogel beads, respectively. These findings suggested that the Carotenoid in the nanoemulsion was very susceptible to degradation and that encapsulating the lipid droplets in hydrogel beads might increase its stability greatly. The physical barrier created by encapsulating the lipid droplets may have increased carotenoids stability by limiting the flow of pro-oxidants or free radicals into the hydrogel bead core, where they may interact with the carotenoids. Encapsulation might have increased carotenoid stability because the alginate in the hydrogel beads could bind and inactivate pro-oxidants; for example, anionic alginate molecules could have bound cationic transition metals (such as Fe2+ or Fe3+). When the amount of Carotenoid added to the alginate beads increased, the Carotenoid seemed to be more stable. Zhang et al. (2016) found that the b-carotene, encapsulated in free lipid droplets (nanoemulsions), degraded rapidly when kept at high temperatures. While chemical stability was substantially increased by including the lipid droplets containing b-carotene in alginate beads.
Microstructure of carotenoids beads
Figure 4A showed smooth beads, and the particle size increased with the increasing in carotenoid content in hydrogel beads. Figure 4B the alginate microgel-caseinate nanoemulsion mixture in the continuous phase may lead to microgel smoothness due to the decrease in osmotic pressure. Ching et al. (2015) found that sodium caseinate protein and gelled alginate were able to form a protein-hydrocolloid gel complex by electrostatic interactions. The SEM showed the presence of nanoemulsion droplets throughout the alginate matrix.
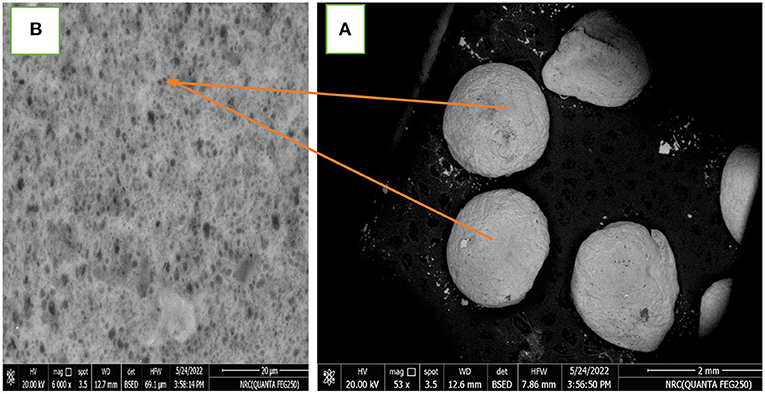
Figure 4. Scanning electron microscopy (SEM) of microstructures of carotenoids 0.5 % alginate beads (A) alginate microparticles, (B) the surface of alginate microparticle.
Physicochemical properties of orange flavoreded stirred yogurt with carrot waste extract (carotenoids)
Table 3 shows the orange Flavoreded-stirred yogurt's chemical composition fortified with free carotenoids or double encapsulated carotenoids. Total solids, protein content, fat, and ash values of control was 17.69 ± 0.17 %, 6.85 ± 0.03 %, 4.47 ± 0.05 %, and 0.78 ± 0.005 respectively. Also, insignificant changes with T3 and T4 in all total solids, protein content, fat, and ash values. At the same time, T1 and T2 significantly increased total solids, protein content, fat, and ash values. Darwish et al. (2021) showed similar results when producing functional yogurt fortified with Fe and folic acid nanoparticles.
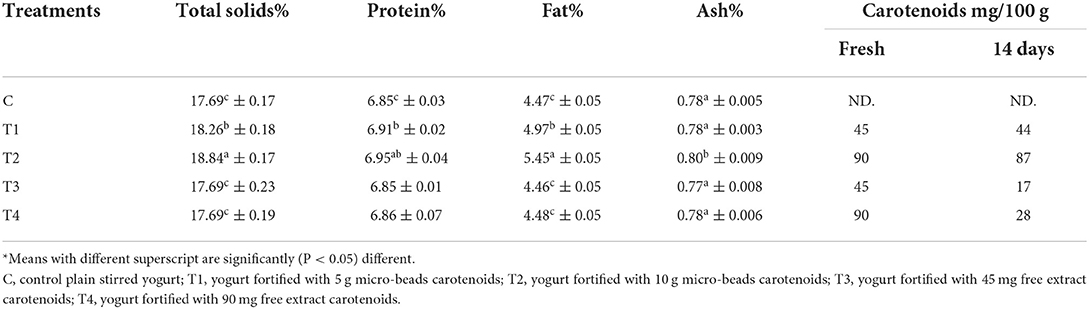
Table 3. Chemical composition of orange Flavored-stirred yogurt fortified with free Carotenoid or double encapsulated.
Nondairy optional components may be included in a maximum proportion of 50% (m/m) of the final product, according to the Codex Alimentarius guidelines for milk and milk products established by the Food and Agriculture Organization of the United Nations (FAO) and the World Health Organization (WHO). The beads with concentrations of 5 and 10/100 g were utilized to produce orange Flavoreded-stirred yogurt. Also, adding natural carotenoids to yogurt may boost its health advantages. The quantitative evaluation revealed an increase in carotenoid concentration when the amount of free carotenoids or beads in the formulation increased, as shown in Table 3. According to epidemiological research, plasma levels of carotene should approach 0.4 mol/L to reap preventative health benefits. 2–4 mg/d may accomplish this (Biesalski et al., 1997). A bottle of fortified yogurts 125 ml with a carotenoid content of 6.25 g per serving are likely to meet 2.25% of the daily requirement, while those with 12.5 grams per serving are likely to meet 4.50%.
According to (Böhm et al. 2021), naturally produced carotenoids and those already present in foods, such as indirect food fortification through previously supplemented fruit/vegetable extract or supplements with synthetic carotenoids. These “food items” are based on sufficient dietary carotenoids calculated to achieve a proposed 1000 nm (1 M) total plasma carotenoid concentration and to avoid excessive intakes of single carotenoids >30 mg/d or more.
pH and acidity
Figure 5 shows the pH and titratable acidity (TA) of yogurt samples fortified with free or double-encapsulate carotenoids. The results reveal no significant changes between yogurt treatments concurrently for the product storage. In contrast, the pH values decreased significantly (p 0.05) after cold storage for 14 days and ranged from 4.53 to 4.47. This might be because starting cultures' metabolic activity increases throughout storage (Bonczar et al., 2002). Cakmakci et al. (2014) found that fortified foods with nanoparticles do not influence pH. The opposite trend with titrable acidity of all yogurt treatments. The TA in control is 0.68% after cold storage for 14 days increased significantly to 0.92%.
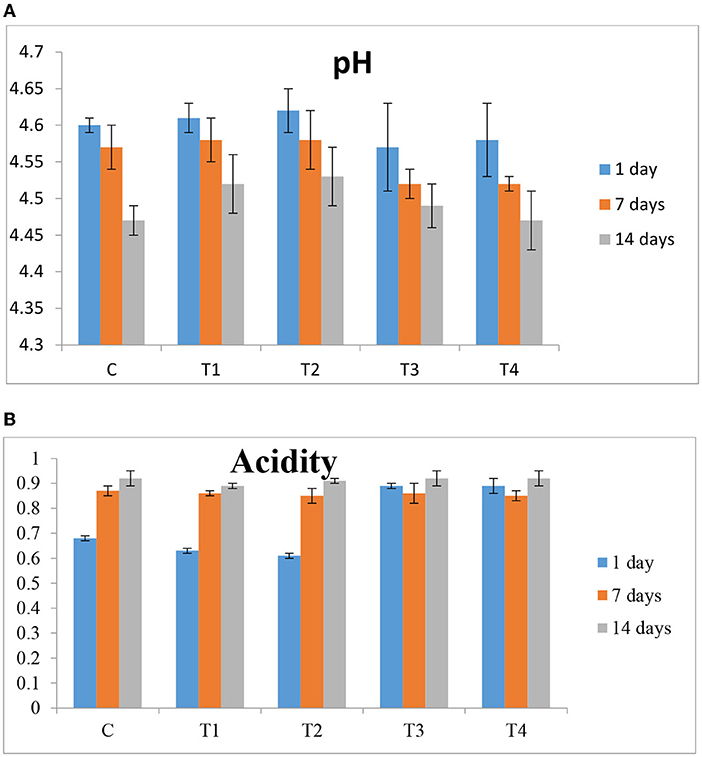
Figure 5. (A): pH values and (B): Titratable acidity % of orange Flavored stirred yogurt with Carotenoid changes during the storage period. See footnote Table 3.
Cakmakci et al. (2014) found that titratable acidity increased after storage in control yogurt and yogurt supplemented with carrot juice. The TA of control yogurt and supplemented yogurt with 15% carrot juice was not statistically significant (P > 0.05). The pH and acidity measurements indicate the enhanced starter and probiotic bacteria activity (Cakmakci et al., 2014).
Viability of lactic acid bacteria in orange flavored stirred yogurt with carotenoid during storage
The yogurt fermentation process can provide an optimal concentration of viable LAB bacteria, but it's imperative to keep them alive throughout the storage period (Santillán-Urquiza et al., 2017). Furthermore, dietary components such as bioactive components, processing-related variables, and microbiological contaminations may affect the survival of LAB culture in yogurt, according to Meybodi et al. (2020). As a result, the survivability of the LAB culture was assessed throughout a 14-day storage period at 4°C, taking into account the number of bacteria, and yogurt pH levels.
Table 4 presents LAB counts for orange flavored stirred yogurt with Carotenoid during storage. Initial inocula were 106 CFU/ml. At the end of the fermentation (at pH = 4.60), counts varied from 1.25 to 1.91*1010 CFU/ml to fresh yogurt production. Including prebiotic alginate and carotenoids may contribute to probiotic bacteria's improved vitality during storage. It's also possible that the product's pH didn't decrease much during storage. Furthermore, our research found that all fermented milk variations examined had a high concentration of live probiotic bacteria (at least 1010 CFU/ml) during refrigerated storage, with a rise in their number, ensuring that the therapeutic minimum was met.
According to the microbial examination, Carrot extract, caseinate, and alginate (as the carrier) were safe in the encapsulation method. The encapsulation process was done under aseptic conditions, following good laboratory practices, so it did not detect microbial contamination in carotenoid beads. Moreover, the control orange flavored stirred yogurt and supplemented with Carotenoid showed a suitable microbiological profile for consumption since examined microorganisms were not observed after the fermentation and packaging.
According to (Meybodi et al., 2020), a milliliter of yogurt should include 107 live lactic acid bacteria cells. Reduced levels of viable organisms arise due to fermentation's decreased carbon source (Tamime and Robinson, 1999).
Antioxidant activity
Antioxidant components can help promote health by scavenging free radicals such as reactive oxygen species and extending the shelf life of foods by delaying the lipid oxidation process (Shima et al., 2009). Previous studies found that yogurt, in general, may have antioxidant action due to its bioactive peptide content (Sah et al., 2015; Abdel-Hamid et al., 2020). The antioxidant activity was evaluated by DPPH and ABTS radical scavenging activity for different yogurt samples after 1, 7, and 14 days of storage.
Table 5 shows antioxidant activity in adding encapsulated carotenoids with different ratios of 5 and 10 g beads or free carotenoids (5 and 10 mg) to orange flavored stirred yogurt samples based on the DPPH and ABTS methods. The control yogurt showed the lowest antioxidant activity value of 23.52 ± 2.19 % by the DPPH method, followed by the fortified yogurt with beads of carotenoids at concentrations of 5 g and 10 g were 93.74 ± 2.01 and 96.97 ± 4.65 μM Trolox equivalent respectively. But, the highest value was for free carotenoids at a concentration of 100 mg of 496.97 ± 4.65 μM Trolox equivalent, as compared to that observed for the control and fortified with encapsulated carotenoids which might be due to a higher free content of total phenolic content in the water extract of the yogurt. Also, Table 5 cleared the same trend for antioxidant activity in the ABTS results. In both methods, we find that the antioxidant activity decreases significantly in all stirred yogurt treatments with the increase of the cold storage period. Changes in the antioxidant activity of the encapsulated carotenoids yogurt demonstrated using DPPH and ABTS during storage primarily reflect the contribution of the added carrot waste extract polyphenols in the water-soluble extract of the yogurt and indicate the stability of these polyphenols, which remained unchanged during 14 days of refrigeration.
The yogurt fortified with carotenoids-loaded beads appears less than that fortified with free carotenoids in radical scavenging activity. But in fact, the carotenoids-loaded beads are more stable, not degraded but not burst in yogurt, so the carotenoids are still inner the beads. Therefore, do not give a real reading during the measurement of antioxidants.
Several studies have shown a link between total phenolic content and the ability of fruit polyphenolic extracts to scavenge free radicals (Skrede et al., 2004; Öztürk, 2018).
The creation of protein–polyphenol complexes is generally highest directly below the protein's PI, where protein-protein electrostatic repulsion is least (Caillet et al., 2006). These interactions might have influenced antioxidant active sites' capacity to donate hydrogen to quench radicals using DPPH or ABTS radical scavenging methods.
Viscosity of stirred yogurt
Figure 6 shows the apparent Viscosity of orange-flavored stirred yogurt enhanced with nano-encapsulated carrot waste extract. Stirred yogurt has a complicated, shear-thinning, time-dependent behavior. The Viscosity of orange-flavored stirred yogurt is thus critical for processing, handling, design, product development, and quality control (Meira et al., 2015).
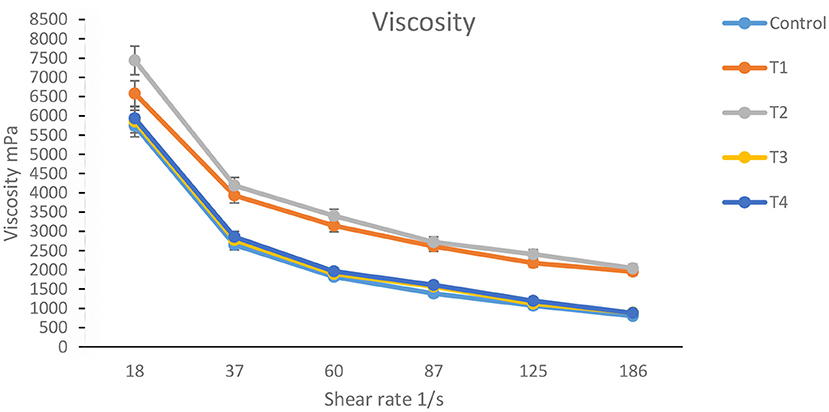
Figure 6. Viscosity of orange-flavored stirred yogurt enhanced with carotenoids. See footnote Table 3.
The Viscosity of orange flavored stirred yogurt (control) was the lowest of the treatments, followed by orange flavored stirred yogurt fortified with free carrot waste extract. The Viscosity increased with the increasing addition of carrot waste extract. While the highest Viscosity appeared with orange flavored stirred yogurt supplemented with nano encapsulated carrot waste extract. Ibrahim et al. (2021) discovered that yogurt enriched with nanoparticles had the greatest apparent viscosity values compared to the control stirred yogurt. Stirred yogurt is a thixotropic fluid, and viscosity investigations showed thixotropic properties of yogurt samples that indicated a reduction in Viscosity with time, consistent with findings reported in the literature corroborated by Lee and Lucey (2010).
Sensory acceptability of yogurt
Yogurt and other fermented milk products' quality and acceptability are heavily influenced by their flavor and texture (Hassan et al., 2003). The flavore, body and texture of yogurt are influenced by a wide range of factors, including milk base composition, starter culture, incubator temperature and other processing variables (Tamine, 1999). The rheological characteristics of yogurt enriched with 4.5 % date fiber were negatively influenced by texture (Hashim et al., 2009).
The addition of double-encapsulated carotenoids to yogurt improves its functionality. However, free carotenoid yogurt showed decreased degree of Sensory judgment of the product (Figure 7). In general, yogurt fortified with encapsulated carotenoids was 90.25% ± 1.50 and 90, 35 ± 1.73 for T1 and T2, respectively, and has even greater sensory acceptability than yogurt fortified with free carotenoids T3 and T4 were 88.25 and 86.75 respectively, or without fortification (control) was 89.75%, particularly after the acceptable storage period. Taksima et al. (2015) noticed alterations in the flavor and texture of yogurt enhanced with astaxanthin-loaded alginate-chitosan beads, although the yogurt's appearance, color, and scent were the same.
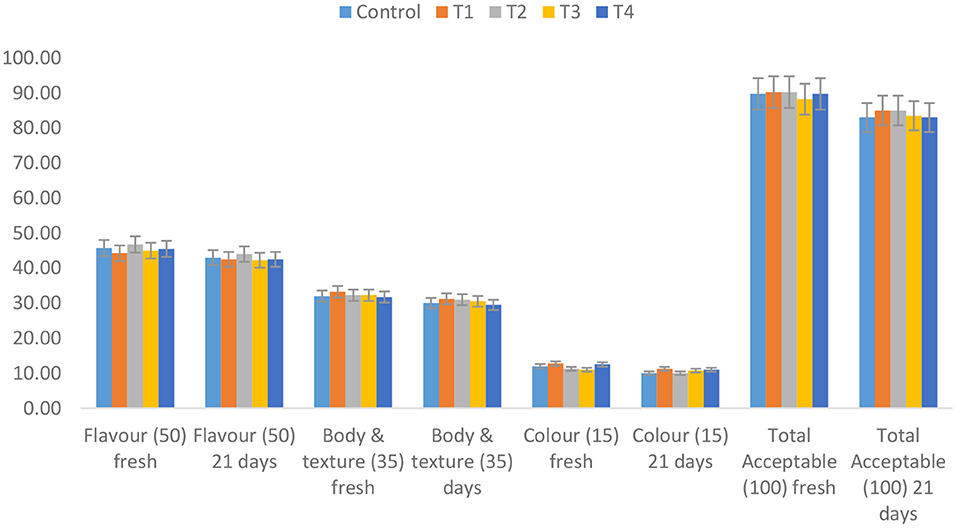
Figure 7. Sensory acceptability of orange-flavored stirred yogurt enhanced with carotenoids during storage period at 5 ± 2°C for 14 days. See footnote Table 3.
Conclusion
This study used the extrusion technique to make filled hydrogel beads from a combination of carotenoids-loaded lipid droplets and alginate molecules in a calcium solution. According to investigations on chemical stability, the hydrogel beads seemed to shield the carotenoids against chemical degradation. Stirred yogurts fortified with carotenoid (carrot waste extract) in beads showed a significant increase in physicochemical properties, Viscosity, LAB count, and sensory acceptability. Carotenoids in yogurt have bioactive potential and offer consumers an optional functional meal for their daily diet. Sensory acceptability testing, which is used to determine whether or not the final product is acceptable to customers, might be given particular consideration in the optimal selection of fortified yogurt with carotenoid beads.
Data availability statement
The original contributions presented in the study are included in the article/supplementary material, further inquiries can be directed to the corresponding author.
Author contributions
TS: visualization, methodology, and writing—review and editing. SN: methodology and validation. All authors contributed to the article and approved the submitted version.
Conflict of interest
The authors declare that the research was conducted in the absence of any commercial or financial relationships that could be construed as a potential conflict of interest.
Publisher's note
All claims expressed in this article are solely those of the authors and do not necessarily represent those of their affiliated organizations, or those of the publisher, the editors and the reviewers. Any product that may be evaluated in this article, or claim that may be made by its manufacturer, is not guaranteed or endorsed by the publisher.
References
Abdel-Hamid, M., Romeih, E., Huang, Z., Enomoto, T., Huang, L., Bioactive, L., et al. (2020). properties of probiotic set-yogurt supplemented with Siraitia grosvenorii fruit extract. Food Chem. 303, 125400. doi: 10.1016/j.foodchem.2019.125400
AOAC (2016). Official Methods of Analysis of the Association of Official Analytical Chemists. Gaithersburg, MD, AOAC International.
Banwo, K., Olojede, A. O., Adesulu-Dahunsi, A. T., Verma, D. K., Thakur, M., Tripathy, S., et al. (2021). Functional importance of bioactive compounds of foods with Potential Health Benefits: a review on recent trends. Food Biosci. 43, 101320. doi: 10.1016/j.fbio.2021.101320
Bertuccioli, M., and Monteleone, E. (2014). “The sensory quality of extra-virgin olive oil,” in The Extra-Virgin Olive Oil Handbook,ed. Peri, C. pp. 35–58. doi: 10.1002/9781118460412.ch4
Biesalski, H. K., Böhles, H., Esterbauer, H., Fürst, P., Gey, F., Hundsdörfer, G., et al. (1997). Antioxidant vitamins in prevention. Clin. Nutr. 16, 151–155. doi: 10.1016/S0261-5614(97)80245-2
Böhm, V., Lietz, G., Olmedilla-Alonso, B., Phelan, D., Reboul, E., Bánati, D., et al. (2021). From carotenoid intake to carotenoid blood and tissue concentrations–implications for dietary intake recommendations. Nutr. Rev. 79, 544–573. doi: 10.1093/nutrit/nuaa008
Bonczar, G., Wszołek, M., and Siuta, A. (2002). The effects of certain factors on the properties of yogurt made from ewe's milk. Food Chem. 79, 85–91. doi: 10.1016/S0308-8146(02)00182-6
Brandão, A. S., Goncalves, A., and Santos, J. M. (2021). Circular bioeconomy strategies: From scientific research to commercially viable products. J. Clean. Prod. 295, 126407. doi: 10.1016/j.jclepro.2021.126407
Caillet, S., Salmiéri, S., and Lacroix, M. (2006). Evaluation of free radical-scavenging properties of commercial grape phenol extracts by a fast colorimetric method. Food Chem. 95, 1–8. doi: 10.1016/j.foodchem.2004.12.011
Cakmakci, S., Tahmas-Kahyaoglu, D., Erkaya, T., Cebi, K., and Hayaloglu, AA. (2014). β-Carotene contents and quality properties of set type yogurt supplemented with carrot juice and sugar. J. Food Process. Preserv. 38, 1155–1163. doi: 10.1111/jfpp.12075
Charoen, R., Jangchud, A., Jangchud, K., Harnsilawat, T., Naivikul, O., McClements, D. J., et al. (2011). Influence of biopolymer emulsifier type on formation and stability of rice bran oil-in-water emulsions: whey protein, gum arabic, and modified starch. J. Food Sci. 76, E165–E172. doi: 10.1111/j.1750-3841.2010.01959.x
Ching, S. H., Bhandari, B., Webb, R., and Bansal, N. (2015). Visualizing the interaction between sodium caseinate and calcium alginate microgel particles. Food Hydrocoll. 43, 165–171. doi: 10.1016/j.foodhyd.2014.05.013
Choi, S. J., and McClements, D. J. (2020). Nanoemulsions as delivery systems for lipophilic nutraceuticals: Strategies for improving their formulation, stability, functionality and bioavailability. Food Sci. Biotechnol. 29, 149–168. doi: 10.1007/s10068-019-00731-4
Darwish, A. M., Soliman, T. N., Elhendy, H. A., and El-Kholy, W. M. (2021). Nano-encapsulated iron and folic acid-fortified functional yogurt enhance anemia in albino rats. Front. Nutr. 8, 654624. doi: 10.3389/fnut.2021.654624
Donhowe, E. G., and Kong, F. (2014). Beta-carotene: Digestion, microencapsulation, and in vitro bioavailability. Food Bioprocess Technol. 7, 338–354. doi: 10.1007/s11947-013-1244-z
Feldsine, P., Abeyta, C., and Andrews, W. H. A. O. A. C. (2002). International methods committee guidelines for validation of qualitative and quantitative food microbiological official methods of analysis. J. AOAC Int. 85, 1187–1200. doi: 10.1093/jaoac/85.5.1187
Gao, Y., and Kispert, L. D. (2003). Reaction of carotenoids and ferric chloride: equilibria, isomerization, and products. J. Phy. Chem. B. 107, 5333–5338. doi: 10.1021/jp034063q
Gómez-Gallego, C., Gueimonde, M., and Salminen, S. (2018). The role of yogurt in food-based dietary guidelines. Nutr. Rev. 76, 29–39. doi: 10.1093/nutrit/nuy059
Hamed, S. F., Soliman, T. N., Hassan, L. K., and Abo-Elwafa, G. (2019). Preparation of functional yogurt fortified with fish oil-in-water nanoemulsion. Egypt. J. Chem. 62, 301–314. doi: 10.21608/ejchem.2019.18621.2149
Hashim, I. B., Khalil, A. H., and Afifi, H. S. (2009). Quality characteristics and consumer acceptance of yogurt fortified with date fiber. J. Dairy Sci. 92, 5403–5407. doi: 10.3168/jds.2009-2234
Hassan, A. N., Ipsen, R., Janzen, T., and Qvist, K. B. (2003). Microstructure and rheology of yogurt made with cultures differing only in their ability to produce exopolysaccharides. J. Dairy Sci. 86, 1632.1638. doi: 10.3168/jds.S0022-0302(03)73748-5
Honary, S., and Zahir, F. (2013). Effect of zeta potential on the properties of nano-drug delivery systems-a review (Part 2). Trop. J. Pharm. Res. 12, 265–273. doi: 10.4314/tjpr.v12i2.19
Ibrahim, R. A., Shahin, A. A. F., Al-Okba, M., and Soliman, TN. (2021). Functional flavored Mandarin Fermented Goat Milk with Algae Oil Nanoemulsion. Int. J. Dairy Sci. 16, 116–126. doi: 10.3923/ijds.2021.116.126
Jo, Y. J., and Kwon, Y. J. (2014). Characterization of β-carotene nanoemulsions prepared by microfluidization technique. Food Sci. Biotechnol. 23, 107–113. doi: 10.1007/s10068-014-0014-7
Kiokias, S., and Gordon, M. H. (2004). Antioxidant properties of carotenoids in vitro and in vivo. Food Rev. Int. 20, 99–121. doi: 10.1081/FRI-120037155
Lee, W. J., and Lucey, J. A. (2010). Formation and physical properties of yogurt. Asian-australas. J. Anim. Sci. 23, 1127–1136. doi: 10.5713/ajas.2010.r.05
Liu, Q., Chen, J., Qin, Y., Jiang, B., and Zhang, T. (2019). Encapsulation of pterostilbene in nanoemulsions: influence of lipid composition on physical stability, in vitro digestion, bioaccessibility, and Caco-2 cell monolayer permeability. Food Funct. 10, 6604–6614. doi: 10.1039/C9FO01260E
Marze, S. (2015). Bioaccessibility of lipophilic micro-constituents from a lipid emulsion. Food Funct. 6, 3218–3227. doi: 10.1039/C5FO00441A
Matalanis, A., and McClements, D. J. (2013). Hydrogel microspheres for encapsulation of lipophilic components: Optimization of fabrication and performance. Food Hydrocoll. 31, 15–25. doi: 10.1016/j.foodhyd.2012.09.012
McClements, D. J. (2012). Advances in fabrication of emulsions with enhanced functionality using structural design principles. Curr. Opin. Colloid Interface Sci. 17, 235–245. doi: 10.1016/j.cocis.2012.06.002
McClements, D. J. (2014). Nanoparticle-and Microparticle-Based Delivery Systems: Encapsulation, Protection and Release of Active Compounds. Boca Raton, FL: CRC press.
McClements, D. J. (2017). Recent progress in hydrogel delivery systems for improving nutraceutical bioavailability. Food Hydrocoll. 68, 238–245. doi: 10.1016/j.foodhyd.2016.05.037
Meira, Q. G., Magnani, M., Medeiros Jollss in FC, do Egito, RD., Madruga, MS., Gullón, B., et al. (2015). Effects of added Lactobacillus acidophilus and Bifidobacterium lactis probiotics on the quality characteristics of goat ricotta and their survival under simulated gastrointestinal conditions. Food Res. Int. 76, 828–838. doi: 10.1016/j.foodres.2015.08.002
Meybodi, N. M., Mortazavian, A. M., Arab, M., and Nematollahi, A. (2020). Probiotic viability in yogurt: a review of influential factors. Int. Dairy J. 109, 104793. doi: 10.1016/j.idairyj.2020.104793
Mustafa, A., Trevino, L. M., and Turner, C. (2012). Pressurized hot ethanol extraction of carotenoids from carrot by-products. Molecules. 17, 1809–1818. doi: 10.3390/molecules17021809
Nontasan, S., Moongngarm, A., and Deeseenthum, S. (2012). Application of functional colorant prepared from black rice bran in yogurt. Apcbee Procedia. 2, 62–67. doi: 10.1016/j.apcbee.2012.06.012
Ozturk, B., and McClements, D. J. (2016). Progress in natural emulsifiers for utilization in food emulsions. Curr. Opinion Food Sci. 7, 1–6. doi: 10.1016/j.cofs.2015.07.008
Öztürk, H. I., Aydin S., Sözeri D., Demirci T., Sert D., Akin N. (2018). Fortification of set-type yogurts with Elaeagnus angustifolia L. flours: effects on physicochemical, textural, and microstructural characteristics. LWT. 90, 620–626. doi: 10.1016/j.lwt.2018.01.012
Patel, A. R., and Velikov, K. P. (2011). Colloidal delivery systems in foods: A general comparison with oral drug delivery. LWT Food Sci. Technol. 44, 1958–1964. doi: 10.1016/j.lwt.2011.04.005
Pilosof, A. M. (2017). Potential impact of interfacial composition of proteins and polysaccharides stabilized emulsions on the modulation of lipolysis. The role of bile salts. Food Hydrocolloids. 68, 178–185. doi: 10.1016/j.foodhyd.2016.08.030
Qian, C., Decker, E. A., Xiao, H., and McClements, D. J. (2012). Inhibition of β-carotene degradation in oil-in-water nanoemulsions: influence of oil-soluble and water-soluble antioxidants. Food Chem. 135, 1036–1043. doi: 10.1016/j.foodchem.2012.05.085
Ravindran, R., and Jaiswal, A. K. (2016). Exploitation of food industry waste for high-value products. Trends Biotechnol. 34, 58–69. doi: 10.1016/j.tibtech.2015.10.008
Sah, B. N., Vasiljevic, T., McKechnie, S., and Donkor, O. N. (2015). Effect of refrigerated storage on probiotic viability and the production and stability of antimutagenic and antioxidant peptides in yogurt supplemented with pineapple peel. J. Dairy Sci. 98, 5905–5916. doi: 10.3168/jds.2015-9450
Santillán-Urquiza, E., Ruiz-Espinosa, H., Angulo-Molina, A., Ruiz, J. F., and Méndez-Rojas, M. A. (2017). Applications of nanomaterials in functional fortified dairy products: benefits and implications for human health. In: Nutrient Delivery. Cambridge, MA: Academic Press. p. 293–328.
Sarkar, S. (2018). Potentiality of probiotic yoghurt as a functional food–a review. Nutr. Food Sci. 49, 182–202. doi: 10.1108/NFS-05-2018-0139
Šeregelj, V., Vulić, J., Cetković, G., Canadanovć-Brunet, J., Šaponjac, V. T., Stajčić, S., et al. (2020). Natural bioactive compounds in carrot waste for food applications and health benefits. Stud. Nat. Prod. Chem. 67, 307–344. doi: 10.1016/B978-0-12-819483-6.00009-6
Šeregelj, V. N., Cetković, G. S., Canadanović-Brunet, J. M., Tumbas-Šaponjac, V. T., Vulić, J. J, Stajčić, S. S., et al. (2017). Extraction and encapuslation of bioactive compounds from carrots. Acta Periodica Technologica. 2017, 261–273. doi: 10.2298/APT1748261S
Shehata, S. H., and Soliman, T. N. (2021). Preparation and characterization of functional yogurt using incorporated encapsulated curcumin by caseinate. Int. J. Dairy Sci. 2021, 11–17. doi: 10.3923/ijds.2021.11.17
Shima, M., Matsuo, T., Yamashita, M., and Adachi, S. (2009). Protection of Lactobacillus acidophilus from bile salts in a model intestinal juice by incorporation into the inner-water phase of a W/O/W emulsion. Food Hydrocoll. 23, 281–285. doi: 10.1016/j.foodhyd.2008.01.008
Skrede, G., Larsen, V. B., Aaby, K., Jørgensen, A. S., and Birkeland, S. E. (2004). Antioxidative properties of commercial fruit preparations and stability of bilberry and black currant extracts in milk products. J. Food Sci. 69, S351–S356. doi: 10.1111/j.1365-2621.2004.tb09948.x
Sy, C., Dangles, O., Borel, P., and Caris-Veyrat, C. (2015). Stability of bacterial carotenoids in the presence of iron in a model of the gastric compartment–Comparison with dietary reference carotenoids. Arch. Biochem. Biophys. 572, 89–100. doi: 10.1016/j.abb.2014.12.030
Taksima, T., Limpawattana, M., and Klaypradit, W. (2015). Astaxanthin encapsulated in beads using ultrasonic atomizer and application in yogurt as evaluated by consumer sensory profile. LWT Food Sci. Technol. 62, 431–437. doi: 10.1016/j.lwt.2015.01.011
Tamime, A. Y., and Robinson, R. K. (1999). Yogurt. Cambridge: Science and Technology Woodhead Publishing Limited.
Umair, M., Jabbar, S., Nasiru, M. M., Lu, Z., Zhang, J., Abid, M., et al. (2021). Ultrasound-assisted extraction of carotenoids from carrot pomace and their optimization through response surface methodology. Molecules. 26, 6763. doi: 10.3390/molecules26226763
Vulić, J., Šeregelj, V., Kalušević, A., Lević, S., Nedović, V., Tumbas Šaponjac, V., et al. (2019). Bioavailability and bioactivity of encapsulated phenolics and carotenoids isolated from red pepper waste. Molecules. 24, 2837. doi: 10.3390/molecules24152837
Wu, L., Zhang, J., and Watanabe, W. (2011). Physical and chemical stability of drug nanoparticles. Adv. Drug Deliv. Rev. 63, 456–69. doi: 10.1016/j.addr.2011.02.001
Xavier, A. A., and Mercadante, A. Z. (2019). The bioaccessibility of carotenoids impacts the design of functional foods. Curr. Opin. Food Sci. 26, 1–8. doi: 10.1016/j.cofs.2019.02.015
Yildiz, F. (2016). Overview of yogurt and other fermented dairy products. Development and Manufacture of Yogurt and Other Functional Dairy Products. Boca Raton, FL: CRC Press.
Zaky, A. A., Chen, Z., Liu, Y., Li, S., and Jia, Y. (2019). Preparation and assessment of bioactive extracts having antioxidant activity from rice bran protein hydrolysates. J. Food Measure. Charact. 13, 2542–2548. doi: 10.1007/s11694-019-00174-9
Zaky, A. A., Liu, Y., Han, P., Ma, A., and Jia, Y. (2020). Effect of flavorzyme digestion on the antioxidant capacities of ultra-filtrated rice bran protein hydrolyzates. J Food Proces Preserv. 44, e14551. doi: 10.1111/jfpp.14551
Keywords: caseinate, nanoemulsion, alginate beads, functional yogurt, carrot waste, carotenoids
Citation: Soliman TN and Nasser SA (2022) Characterization of carotenoids double-encapsulated and incorporate in functional stirred yogurt. Front. Sustain. Food Syst. 6:979252. doi: 10.3389/fsufs.2022.979252
Received: 27 June 2022; Accepted: 09 August 2022;
Published: 29 August 2022.
Edited by:
Leonardo Sepúlveda, Autonomous University of Coahuila, MexicoReviewed by:
Orlando Daniel De La Rosa Flores, Universidad Autónoma de Coahuila, MexicoJuanita Mendez, Universidad Autónoma de Coahuila, Mexico
Copyright © 2022 Soliman and Nasser. This is an open-access article distributed under the terms of the Creative Commons Attribution License (CC BY). The use, distribution or reproduction in other forums is permitted, provided the original author(s) and the copyright owner(s) are credited and that the original publication in this journal is cited, in accordance with accepted academic practice. No use, distribution or reproduction is permitted which does not comply with these terms.
*Correspondence: Tarek Nour Soliman, dGFyaWtub3VyLm5yY0BnbWFpbC5jb20=