- Department of Agricultural, Food and Nutritional Science, University of Alberta, Edmonton, AB, Canada
Humic-based products (HPs) are carbon-rich organic amendments in the forms of extracted humic substances from manure, compost, and raw and extracted forms of lignites, coals and peats. HPs are widely used in agriculture and have beneficial effects on plants. While the agronomic benefits of HPs have been widely reported, information on their impact on the soil microbial community composition and functions is lacking, despite claims made by companies of humic substances as biostimulants. In this review, we explored published research on microbial responses with HPs application in an agronomic context. Although research data are sparse, current results suggest indirect impacts of HPs on microbial community composition and activities. HPs application changes the physico-chemical properties of the soil and influence root exudation, which in turn impact the microbial structure and function of the soil and rhizosphere. Application of HPs to the soil as biostimulants seemed to favor plant/soil beneficial bacterial community composition. HPs impacts on microbial activities that influence soil biogeochemical functioning remain unclear; existing data are also inconsistent and contradictory. The structural properties of HPs caused inconsistencies in their reported impacts on soil properties and plants. The sources of HPs and forms (whether extracted or raw), soil type, geographic location, crop species, and management strategies, among others, affect microbial communities affecting HPs efficacy as biostimulants. A more holistic approach to research encompassing multiple influential factors and leveraging the next-generation sequencing technology is needed to unravel the impacts of HPs on the soil microbiome. Addressing these knowledge gaps facilitates sustainable and efficient use of HPs as organic agricultural amendments reducing the use of chemical fertilizers.
Introduction
The increasing demand for food production has intensified crop production, leading to the depletion of agricultural lands and soil and water pollution due to the intensive use of fertilizer and pesticide (Glibert et al., 2006; Galloway et al., 2008; Silva et al., 2019). Other main challenges that current agricultural practices face with are high inorganic fertilizer prices and low nutrient use efficiency by crops (e.g., nitrogen) due to the loss of nutrients by leaching, denitrification and volatilization (Malhi et al., 2001; Cassman et al., 2002). These environmental and economic concerns necessitate environmentally friendly agricultural management strategies that improve soil health and resilience. One strategy for sustainable farming practice is using less agrochemical input and more soil organic amendments, such as humic-based products (HPs).
Humic-based products are carbon-rich organic amendments in the forms of extracted humic substances from manure, compost, and raw and extracted forms of lignites, coals and peats. For decades, HPs have been widely reported to improve soil health and crop productivity (Guo et al., 2019; Nardi et al., 2021; Ampong et al., 2022). HPs can be synthetic, recycled wastes, or natural and have wide industrial applications (El-shazly et al., 2015; Dong et al., 2017; Bezuglova et al., 2019; Guo et al., 2019; Zhou et al., 2019). Natural HPs are geological deposits formed out of the natural decomposition of animal and plant material from millions of years ago that make up to 80% of soil organic matter (Olk et al., 2018). Humic acids (HAs) have been widely used in the world, with a market value exceeding USD 532.7 million in 2021 and a projected growth rate of 11.8% from 2022 to 2028 (Pulidindi and Prakash, 2021).
Numerous research studies have demonstrated positive crop responses to HPs from various sources in fields, greenhouse, and controlled environmental studies (Rose et al., 2014; Nardi et al., 2021; Yang et al., 2021; Bezuglova and Klimenko, 2022). Ampong et al. (2022) recently provided a comprehensive review on the significant positive impacts of humic acid (HA) on crop growth, yield, and soil physical and chemical properties. Plants are more stress-tolerant, productive, healthier, and yield better quality in soil with high humic acid content (Nardi et al., 2021; Ampong et al., 2022). Although the effect of HPs on the soil's physical and chemical properties and crop responses are well-studied, their effects on microbial structure and functions are poorly understood.
Soil is the most diverse and complex habitat containing more than 1,000 kg of microbial biomass carbon per hectare (Suttle, 2007; Paez-Espino et al., 2016). Soil microorganisms play vital roles in soil ecosystem functions and services, such as nutrient cycling, biogeochemical cycles, soil fertility and resilience, thereby affecting plant growth and health (Berg, 2009; Madsen, 2011; Chaparro et al., 2012). Soil microbial community structure and functions are well-known as soil health indicators. Parameters like basal respiration, nitrogen (N) mineralization, N2 fixation and microbial enzyme activities are used to determine microbial functional activities in the soil. Biochar-based humic products induced changes in microbial biomass, community composition and diversity, and biogeochemical processes (Xu et al., 2016). While HPs are known to stimulate soil microbial communities that drive vital soil processes needed for plant growth and health, we have yet to unravel these key microbiome players. The interactive effects of HPs with crop and soil types, various agricultural practices, and the soil microbiome structure and function are poorly understood.
Recent advances in marker genes, genomic and metagenomic research have significantly increased our ability to differentiate the microbial activities under different agricultural management practices. These advances enhance our knowledge of microbial metabolic capabilities and their influence on soil fertility, plant growth and health. The reliability of humic-based products as biostimulants/biofertilizers will improve as we learn and understand more about their impact on the microbiome community and functions across ranges of biotic and abiotic factors.
This review aims to provide key insights into the impact of HPs on soil microbial structure and activities and gain further insights into opportunities for future research with a specific emphasis on agriculture. We have summarized what has been learned from recent work on soil microbial communities and functions under humic-based amendments. This review is focused on HPs as an amendment to soil microbial community structure and functions. HPs have been used as biocontrol, but that is outside the scope of this review. While we acknowledge the controversies surrounding humic substances (Lehmann and Kleber, 2015; Kleber and Lehmann, 2019), the research work presented in the following sections are based on all forms of humic products, including the raw and extracted forms of lignite-based HPs and those that were extracted from humic-rich materials (i.e., vermicompost, sewage sludge, etc.) (Figure 1). Challenges and opportunities associated with managing soil microbial communities to maximize agricultural productivity and sustainability using naturally sourced humic-based products are discussed. This review also highlights key research directions that could shape the future of humic-based products.
Humic-based products in agriculture: A historical perspective
In agricultural systems, humic-based products are organic amendments in the forms of extracted humic substances from manure, compost, and extracted or raw naturally-sourced humic substances from earth's soils and sediments derived from decayed biomatter through the humification process. HPs are thought to stimulate soil microbiota that are beneficial to plants. Plant-beneficial microbiota have been shown to improve soil nutrient availability and thus plant growth and productivity.
The earliest account where humans used soil organic amendment was in Brazil's Amazon basin more than 2,000 years ago, where the ancient soil practice was using Terra Preta, also known as black soil (Neves et al., 2004). Terra Preta is the conceptual basis for the use of biochar to improve the fertility of soils. The native Indians in the Amazon basin would generate charcoal, mix it with organic matter, and apply it to their agricultural lands. This Amazonian black earth is characterized by its rich black color. It has been known that the black soil is due to high plant carbons that accumulated in the soil for thousands of years (Neves et al., 2004). Since the discovery of black soil, researchers around the world have explored the potential of charcoal and partially combusted organic waste or biochar to mimic the soil organic matter of the Amazonian black soil (Novotny et al., 2009).
There have been constant explorations of soil amendments for carbon addition to the soil. The agronomic potential of humic-based products has been investigated. Humic acid, as a part of humic substances in general, affects the soil physicochemical characteristics, nutrient availability, and plant growth and physiological characteristics (Khaled and Fawy, 2011). Most humic acids that are currently being used today come from lignite and sub-bituminous coal. These low-grade coals form mineable humic substances when oxidized. They are known as humalite in Alberta and leonardite in other parts of the world (Leonard, 2012). Leonardite deposits have been produced in North Dakota (since the 1920's) and Mexico.
Over 200 years ago, components of humic substances (HS)– humic acid, fulvic acid (FA), and humin were extracted as three distinct fractions. However, the alkali extraction process of HA is still hounded by controversy as the HA that is synthesized is not really an acid (Kleber and Lehmann, 2019). The mechanisms of the humic substances' formation, as well as their structure, are still a subject of discussion and controversy. While the agronomic benefits of humic substances have been well-studied, the debatable nature of HS slogs the flow of scientific data as regards humic products or humic substances' impact on the microbial communities in the plant-soil interface.
Impact of HPs on soil microbial communities
HPs serve as the sources of carbon and energy for the soil microbes. HPs alter the soil physicochemical characteristics and physiological responses of plants which in turn affect the soil microbial community composition (Figure 2) (Puglisi and Trevisan, 2013; Puglisi et al., 2013). Table 1 presents selected studies on the impact of HPs on soil microbial communities.
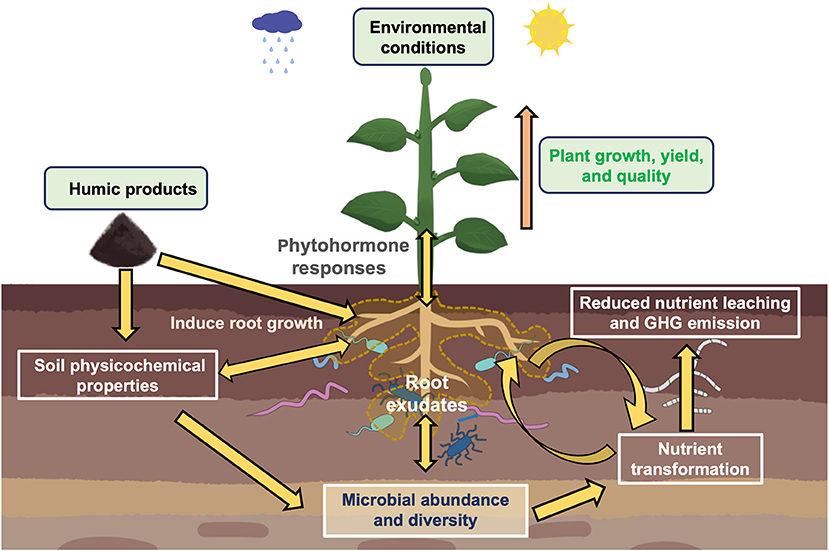
Figure 2. An illustration showing complex interactions determining impacts of humic-based products (HPs) application in agricultural soil. Multiple factors shape the microbial community assembly and functioning, such as sources of humic-based products and various biotic and abiotic factors (crop species, soil environmental parameters, microclimatic conditions, etc.). HPs application impacts the soil (physicochemical properties) and plant (root growth, root exudates, phytohormonal responses, shoot growth) and subsequent effects on microbial community composition and functions, such as soil nutrient transformations, a crucial soil ecosystem process for plant growth and health.
Previous studies show that the application of HPs into the soil strongly affects the bacterial community composition and abundance and to a lesser extent the bacterial group actinomycetes, soil fungi, and microalgae (Dong et al., 2009; Puglisi et al., 2009). A combined application of diverse microbial consortium and humic substances significantly changed the bacterial community structure, but not the fungal communities in the blueberry rhizosphere (Schoebitz et al., 2016). Application of vermicompost enriched with commercial alkaline-extracted HAs to the soil increased the diversity of rhizosphere bacteria and fungi as revealed by denaturing gradient gel electrophoresis (DGGE) profiles and enhanced nodulation of Pisum sativum by nodule-forming nitrogen-fixing bacteria was also found with HPs applications (Maji et al., 2017).
Studies using 16S rRNA gene-based phylogenetic microarrays revealed the impact of commercial HPs on the resident bacterial community in differing soil profiles (van Trump et al., 2011; Puglisi et al., 2013). The most probable number (MPN) enumeration of agricultural soils amended with HPs revealed large populations of nitrate-reducing bacterial communities. These nitrate-reducing bacterial communities were phylogenetically diverse and included members of Alphaproteobacteria, Betaproteobacteria, and Gammaproteobacteria (van Trump et al., 2011).
A leonardite-derived HA changed the microbiome structure of soil grown with potato plants under greenhouse conditions (Akimbekov et al., 2020). HA-treated plants showed higher microbial diversity and richness compared to the control and observed predominance in Proteobacteria. This study also demonstrated the beneficial impacts of HAs on potato plant growth (Akimbekov et al., 2020). Hita et al. (2020) isolated the cultivable bacteria from sedimentary humic acid-treated cucumber plants and recovered isolates from the phyla– Proteobacteria, Firmicutes, Actinobacteria, and Bacteroidetes. The isolates were also assayed for plant growth-promoting traits and found that most of the isolates were able to fix N2 and produce plant hormones–indole-3-acetic acid and several cytokinins (Hita et al., 2020).
The effects of humic acid extracted from vermicompost on rice plants have been investigated under growth chamber conditions, where the microbial groups (e.g., Chitinophaga and Pseudomonas) that were enriched in the presence of HA were known to be related to plant defense against pathogens (da Silva et al., 2021). It was noted that in stressful environments, such as pathogen attacks, HA may modulate the plants' physiological mechanisms, triggering the plants to recruit microbes with biocontrol potential (da Silva et al., 2021). Inoculation of plant growth-promoting bacteria and HA regulates genes related to plant protection, oxidative stress, and chitin metabolism even under non-stressful conditions in tomato plants (Galambos et al., 2020). These adaptive metabolic changes may alleviate plant stress in biotic and abiotic stress conditions (Galambos et al., 2020). It was found that secondary and plant defense metabolisms were stimulated in tomato plants when plant growth-promoting bacteria and HPs were applied together (Olivares et al., 2015).
In a long-term field experiment with peanuts amended with HA and inorganic fertilizers, changes in soil enzyme activity alter the soil microbial community structure: the number of beneficial bacteria increased while harmful bacteria decreased (Li et al., 2019a). Furthermore, taxonomic groups that were reported to be beneficial to plants, including Firmicutes (bacteria), Basidiomycota (fungi) and Mortierellomycota (fungi), increased following HA application (Li et al., 2019a).
The microbial community data involving HPs applications that are currently available are based on culture-dependent and molecular fingerprint methods. While these are informative, these data are unable to decipher microbial taxonomic compositions and the identification of potential key species. In addition, the culturable microbial community is largely underestimated as only a very small percentage can be cultivated. The next generation sequencing has been around in the last decade and has been pivotal in unraveling microbial community composition and function. These high throughput technologies will enable us to systematically identify microbial interactions and functions toward identifying the major players in nutrient cycling processes. While indirect effects of HPs on microbial communities have been demonstrated through their effects on rhizodeposition (Puglisi et al., 2013), much research still needs to be completed to investigate responses under differing biotic and abiotic stress conditions. Addressing these knowledge gaps facilitates a clear understanding in developing an optimal use of HPs toward reduction of chemical fertilizer use.
Impact of humic-based products on microbe-mediated biochemical activities in the soil
As discussed above, HPs alter microbial communities in the soil. Plants treated with HPs trigger phytohormone production in plants, causing changes in root and shoot growth, and rhizodeposition that will then influence the microbial community composition in the plant-soil system (Figure 2) (Puglisi et al., 2009, 2013; Canellas and Olivares, 2014; Olivares et al., 2017). This change in microbial communities influences microbial activities, particularly the soil enzyme activities that catalyze various key biogeochemical processes (Zhang et al., 2018; Dai et al., 2020). Table 1 provides a list of research work showing the impacts of HPs to soil microbial activities and functions.
Microbial nitrogen cycling
Mineralization and nitrification are the most crucial processes in the soil N cycle as these steps influence plant N uptake, nitrate leaching, and reactive N gas emissions (Philippot et al., 2007; Norton and Stark, 2011). Microbial-mediated mineralization of organic N into ammonium () and its subsequent nitrification processes– nitrite () or nitrate (), is of major importance of N availability (Jackson et al., 2008). Understanding the effects of HPs on the microbial N cycle in the soil is key toward modern sustainable agriculture. Humic-based input affects the soil physical and chemical structure of the soil, which in turn affects the microbial N cycling in the soil. Furthermore, microbial N cycling in the soil is also controlled by environmental factors. Specifically, oxygen availability is one of the most important parameters; high oxygen soil concentrations promote nitrification whereas anoxic conditions stimulate microbial denitrification (Braker and Conrad, 2011; Lam and Kuypers, 2011). Air-filled and water-filled pores affect the moisture content in the soil, which will then directly affect the oxygen supply. High water-filled soil pores result in the depletion of dissolved oxygen concentrations due to increased microbial respiration facilitating anaerobic processes (Drenovsky et al., 2004). Besides oxygen availability and soil moisture, pH, soil organic carbon contents, and N availability control the microbial N cycling processes (Nicol et al., 2008; Hsu and Buckley, 2009; Saarnio et al., 2013). As the impacts of HPs on various soil physical and chemical structures, such as soil pores, soil moisture, pH, soil organic carbon, etc. of the soil remain inconclusive (Ampong et al., 2022), and so their effects on microbial N cycling remain unknown.
Soil amended with HPs will affect the availability of many nutrients, trace elements, electron acceptors, and other compounds such as carbon substrates that determine microbial growth and activity (Figure 2). The addition of HPs (humic acids) as seed coating was reported to improve root growth (Gorim and Asch, 2012), resulting in increased root exudates, stimulating microbial activity in the rhizosphere of cereal plants. In addition, enhancement in plant growth also increases plant nutrient uptake, thus decreasing the bioavailable N in the soil affecting microbial activity (Saarnio et al., 2013).
While research is scarce on the impact of HS on microbially-mediated N cycling processes in agricultural systems, several research works have been completed in landfill leachate/wastewater treatments that will be incorporated in the subsections below. Humic acids, the main component in the endogenous substances in the landfill leachate system, usually account for ~4 to 44% of the total organic carbon (Liu et al., 2022). Humic acids play a dual role as both an organic pollutant and natural electron shuttle in the landfill leachate systems' concurrent nitrification, annamox, and denitrification technology (Liu et al., 2022). The system facilitates efficient HA biodegradation and efficient removal of N resulting from faster electron transfer efficiency that enhances enzymatic activity (Liu et al., 2022).
Nitrification
Many of the beneficial N-fixing bacteria live in root nodules of leguminous plants, while others are free-living in the soil (a.k.a diazotrophs). In the landfill leachate nitrification process, HA could enhance nitrifying bacteria's cellular permeability and act as an electron shuttle to reduce membrane transporter genes (Luo et al., 2019). Increasing HA concentration subsequently enriches Nitrospira abundance, which can oxidize nitrite to nitrate under aerobic conditions (Luo et al., 2019). In anaerobic conditions, the mechanism of redox action of HS is complex, as it participates in redox reactions as both an electron acceptor and electron donor for microbial respiration (Li Y. et al., 2019).
Several studies have demonstrated the positive effects of soil organic amendments, specifically biochar– another carbon-rich soil amendment product, on microbial N fixation. A combination of isotopic analysis and molecular techniques revealed increases in nitrogenase activities, proportion of soil N input originated from microbial N fixation, and abundance of N-fixing microorganisms in soil amended with biochar (Quilliam et al., 2013; Harter et al., 2014; Mia et al., 2014). Increased nifH gene copy numbers were also observed in controlled soil microcosm experiments (Ducey et al., 2013; Harter et al., 2014). Increase in the relative abundance of many known N-fixing families: Bradyrhizobiaceae, Frankiaceae, and Rhizobiaceae were observed in biochar-amended pot experiments and rice paddy study (Anderson et al., 2011; Chen et al., 2015). In a greenhouse experiment using a broad range of biochars from different feedstocks, Güereña et al. (2015) observed increased plant biomass, nodule number and biomass, and the proportion of N derived from symbiotic N fixation in common beans (Phaseolus vulgaris L.).
Fulvic acid induced the growth of Sinorhizobium meliloti, and this combination showed an increase in active nodules and yield in Medicago sativa (Capstaff et al., 2020). These FA-treated plants showed up-regulation in not only early nodulating genes, but also in various processes, such as defense, oxidoreduction, and carbon and nitrogen metabolism (Capstaff et al., 2020).
Denitrification
Denitrification is a key microbial process resulting in N loss to the atmosphere, where nitrate or nitrite is reduced to gaseous NO (nitric oxide), N2O (nitrous oxide), and N2 (dinitrogen), by denitrifying microbes (Conrad, 1996). NO and N2O are important greenhouse gases, with the latter holding the warming capacity that is 296 times higher than CO2 (Zumft, 1997). Thus, understanding the microbiome underpinning the biogeochemical processes is crucial due to the economic loss of N and greenhouse gas emissions resulting from incomplete denitrification.
Many of the denitrification processes were driven by heterotrophic denitrifying microorganisms. Fulvic acid can serve as electron shuttles between bacteria and electron acceptors (Li et al., 2016). These electrons are consumed by four key denitrifying enzymes (NAR, NIR, NOR, and NOS) that are mainly encoded by the genes narG, nirK/S, norB, and nosZ (Li et al., 2016). The authors observed that the addition of FA into denitrification bacterial culture accelerated the metabolism of carbon sources to generate ATP and NADH through microbial glycolysis metabolism. Increased dosage of FA (from 0.5 to 1 mM) improved anammox (conversion of ammonium and nitrite to N2 gas) bacterial activity, thereby increasing the production of extracellular polymeric substances, implying its potential role in quorum sensing performance, and enhanced N removal efficiency (Liu et al., 2020).
HPs have significant impacts in the N removal process in industrial sewage/wastewater treatment facilities. The data from this system would shift microbial composition and functional genes in relation to the soil N cycle. Concentration of FA affects microbial community, N conversion efficiency, and abundance of functional genes responsible for ammonia-N oxidation. The presence of low FA concentration (0–50 mg/L) enhances NADH generation that favors denitrification and nitrite reduction (Zhang et al., 2023).
The effect of HA on the simultaneous nitrification, anammox and denitrification (SNAD) treatment method in the landfill leachate system was investigated (Liu et al., 2022). The electron transfer efficiency of the SNAD system was enhanced due to the redox properties of HA. This efficient transfer enhanced the electron transport system activity resulting in an increase in adenosine triphosphate (ATP) that plays an essential role in microbial N metabolism. The enhanced metabolic activity by the SNAD method increased the enzymatic activities of ammonia-oxidizing bacteria, nitrite-oxidizing bacteria, and heterotrophic denitrifying bacteria, thereby facilitating efficient N removal (Liu et al., 2022).
Phosphorus solubilization
Phosphorous (P) is an essential element that can be solubilized by root exudates or microbes and is an essential macro nutrient for crop growth (Holford, 1997). Although soils generally contain a large amount of total P, only a small proportion is immediately available for plant uptake. Plants obtain P as orthophosphate anions (predominantly as and ) from the soil solution. In most soils, the concentration of orthophosphate is low (Richardson et al., 2009).
HPs affect the phosphorus cycle by altering the P uptake of plants by (1) providing a source of organic P, (2) enriching the microbial activity of P-solubilizing microbes, (3) increasing organic acid levels in soil, thereby stimulating P solubilization, and (4) increasing P availability in soil by forming a complex linkage with humic substances and metal ions (Jindo et al., 2020). Microbes involve in P cycling activities of the soil-rhizosphere interface are classified into two groups based on their P solubilizing strategies. The first group is microbes that produce nuclease enzymes, phospholipases, and phytases that hydrolyze P-organic compounds. Phytases are key enzymes in P cycling as 50% of the soil organic P is in phytate form. The second group is P-solubilizing microbes that convert sources of inorganic P into soluble orthophosphate ions.
The relationship between HPs and bioavailable P mediated by microbes is yet complex, wherein HPs are itself sources of organic P (Jindo et al., 2020). Interactions between HPs and rhizosphere microbiomes reportedly involve the central component in the auxin hormonal pathway. HPs affect the formation of lateral roots and root hair length and density, thereby stimulating the release of root exudates (O'donnell, 1973; Gorim and Asch, 2012). Some of these root exudates are precursors of IAA and other AUX-like compounds, including the amino acid L-Tryptophan (Jindo et al., 2020). Increased IAA in the rhizosphere results in acidification and consequent release of inorganic P into the soil (Chaiharn and Lumyong, 2011).
Application of the humic acid-rich vermicompost to plants resulted in increasing available P due to the promotion of phosphatase secretion and activity, microbial diversity, and abundance compared to fertilizer-only treatment (Maji et al., 2017). The authors observed increase in diversity and abundance of P-solubilizing microorganisms following humic acid-rich vermicompost treatments (Maji et al., 2017). Bezuglova et al. (2019) observed positive effect of HPs on P mobilization and crop productivity, possibly due to the activation of the rhizosphere microbiota through root exudates. Research on the interaction of HPs and soil/rhizosphere microbiomes in relation to P cycling is sparse; more research needs to be conducted.
Soil microbial biomass and enzyme activities
Microbial activities drive soil organic matter transformation and nutrient cycling processes. Understanding the impact of HPs on the soil microbial-mediated biogeochemical processes has important implications on soil health management and sustaining agricultural productivity. Microbial extracellular enzymes regulate the carbon degradation and nutrient release. Thus, enzymatic activities are sensitive indicators of soil health and can be used to determine soil responses to agricultural management practices and environmental changes (Xiao et al., 2018; Li et al., 2019b; Nannipieri et al., 2020). The key soil enzymes involved in soil C, N and P cycles are glucosidases, cellulases, hydrolases, ureases, invertases, laccases, peroxidases, proteases, nitrate reductases, and phosphatases (Nannipieri et al., 2012).
The complexity of humic substances deters the complete understanding of their impact on soil microbial activity. Previous studies show that the application of HPs had substantial impacts on soil enzyme activity (Mato et al., 1971, 1972; Malcolm and Vaughan, 1979; Pflug, 1980; Pflug and Ziechmann, 1981; Allison, 2006; Dou et al., 2018). A research study with nuclear magnetic resonance (NMR) spectroscopy showed that soil enzymes are encapsulated in humic substances, thereby causing a reduced or inhibited catalytic activity (Tomaszewski et al., 2011). Humic substances can alter bacterial enzymes causing the inactivation of extracellular enzymatic activity (van Trump et al., 2006; Mazzei et al., 2013). Humic acid fractions have been shown to inhibit phosphatase activities in several agricultural crops, and thus may have implications in relation to the humic acids' role in P nutrient cycling processes (Malcolm and Vaughan, 1979). The role of soil extracellular enzymes may be reduced because of contact with HS (Allison, 2006). Nuclear magnetic resonance revealed that β-glucosidase enzyme activity was substantially reduced by increasing fulvic acid concentration (Mazzei and Piccolo, 2013), possibly due to fulvic molecules covering the β-glucosidase active sites or the modification of the enzyme conformational structure during the humic-enzyme complex formation.
The inhibitory effects of HS on enzyme activity may be offset by increased stability and/or resistance to enzyme degradation (Marzadori et al., 2000a,b; Dong et al., 2008). The effects of humic substances on enzymes are dependent on pH, ionic strength, and mass ratio of HS/enzyme (Li et al., 2013). In particular, the enzyme activity in the HS-enzyme complexes was suppressed when ureases, the enzyme that catalyzes the hydrolysis of urea to form ammonia and carbamates, were oppositely charged to the humic substances (Li et al., 2013). Conversely, no enzyme complexes were formed when both ureases and HS were both negatively charged (Li et al., 2013). Structural characteristics of HAs affect urease activity. The high molecular weight HA fraction significantly improved urease activity and stability especially in alkaline soil (Dong et al., 2008).
Up to now, very limited research has been conducted on the impact of HPs on the microbial enzyme in the soil, and the results are contradictory. A combined application of NPK and humic acid in sugarcane rhizosphere significantly increased catalase, dehydrogenase, phosphatase activities, and microbial biomass (Sellamuthu and Govindaswamy, 2003). This enhanced soil enzymes activities and increased microbial biomass in the presence of HPs may be due to a greater carbon-rich substrates availability to microbes, thereby promoting heterotrophic growth (Blagodatskaya et al., 2014). A combined application of inorganic fertilizer and HA changed the soil microbial community structure that consequently changed the soil enzyme activities– sucrase, urease, and phosphatase (Li et al., 2019a). Changes in the C/N ratio of the soil due to the addition of HA favor conditions for microbial growth (Griffiths et al., 2012). A study by Dong et al. (2017) in landfill leachate system showed that HA shape bacterial community, increase enzyme activities and upregulate microbial genes related to denitrification. HA positively affected the denitrification process in landfill leachate that promoted the growth of denitrifying bacteria with the predominance of Thauera (Dong et al., 2017), thereby facilitating removal of N from leachate.
Biochar and lignite-based amendments showed contradictory effects on microbial activities and greenhouse gas emissions in laboratory-incubated microcosms using agricultural soil (Li et al., 2021). This study demonstrated that biochar-amended treatment increased microbial community growth and ability to utilize diverse carbon sources, which contrasted with the lignite-based amended treatment. Biochar-amended treatment also showed increased carbon dioxide emission, which was inhibited in the lignite-based setup. In contrast, nitrous oxide emission decreased in the biochar-amended soil but increased in the lignite-amended treatment (Li et al., 2021).
Increased application of lignite-based HA displayed an increasing trend in soil enzyme activities (Ma et al., 2022). Repeated annual application of HA (1,500 kg/ha) at a dryland experimental farm in North Central China planted with oats found increased urease, invertase and catalase activities. The authors explained that these increased enzyme activities might be the result of increased soil organic carbon and improved soil physicochemical properties that provided a condition conducive to the growth and activities of soil microorganisms (Ma et al., 2022).
Tolerance to abiotic stress
The utilization of biostimulants, such as HPs was reported to potentially mitigate the adverse effects of environmental stresses on plants (Canellas and Olivares, 2014). Previous reports indicated that HPs induce changes in the root morphology to facilitate colonization of plant beneficial microbes (Canellas et al., 2013; Canellas and Olivares, 2017). Canellas and Olivares (2017) demonstrated that the exogenous application of humic acid in maize facilitated the release of border cells from root tips resulting in increased colonization density of the diazotrophic bacteria, Herbaspirillum seropedicae in the roots. Borders cells exuded from the roots are the first living partition at the plant-soil interface in the root cap (Driouich et al., 2012). Borders cells are sources of biologically active chemicals that can modulate the division of root tip cells, expansion and gravitropism in plants (Zhu et al., 1997). Borders cells were also reported to neutralize toxic chemicals near the roots and possibly inhibit or promote the growth of other rhizosphere organisms (Bais et al., 2006).
The soil is a complex habitat with intricate interacting biotic and abiotic components. The application of HPs on the soil affects its physicochemical properties, and on the plants its growth and rhizodeposition, that in turn impact the microbial community composition and function. The plant-soil feedbacks affecting microbial communities are crucial in soil nutrient cycling and plant uptake processes. Up to now, data are scarce, and results are inconclusive on how HPs impact the soil. Specifically, it remains unknown how HPs and their different forms and sources affect the soil pH (Ampong et al., 2022), which is a key predictor of microbial structure and composition at field and continental scales (Lauber et al., 2009; Chu et al., 2010; Banerjee and van der Heijden, 2022). For instance, the complex nature of HPs caused inconsistencies regarding whether HPs form an enzyme complex that stabilizes urease activity, or whether HPs negatively affect urease activity. A stable urease activity in an enzyme complex facilitates the gradual release of plant-available form nitrogen that will then promote plant uptake and lessen nutrient leaching. It also remains unclear whether HP application will have stimulating effects on plant beneficial bacteria. Thus, more research work needs to be done on how the application of HPs and their various forms from different sources in different crop species covering various soil types and environmental conditions impact the soil microbial community composition, structure, and function, which consequently impact plant growth and health (Figure 2).
Interactive effects of humic-based products with other biologicals and amendments on microbial community and function
Microbial inoculants
Inoculants with organic amendments alter soil microbiota and promote plant growth (Olivares et al., 2017). The combined use of humic acids with Pseudomonas spp. and Bacillus amyloliquefaciens in maize demonstrated superior effects on P absorption compared with the inoculation of each bacterial strain alone. The greatest P uptake was observed when B. amyloliquefaciens was combined with HA and AMF and Pseudomonas spp. with HA were applied to soil. This work also noted significant changes in bacterial and fungal diversity with HA application (Cozzolino et al., 2021). Selected studies showing combined effects of HPs with other inputs on microbial community structure and functions are presented in Table 2.
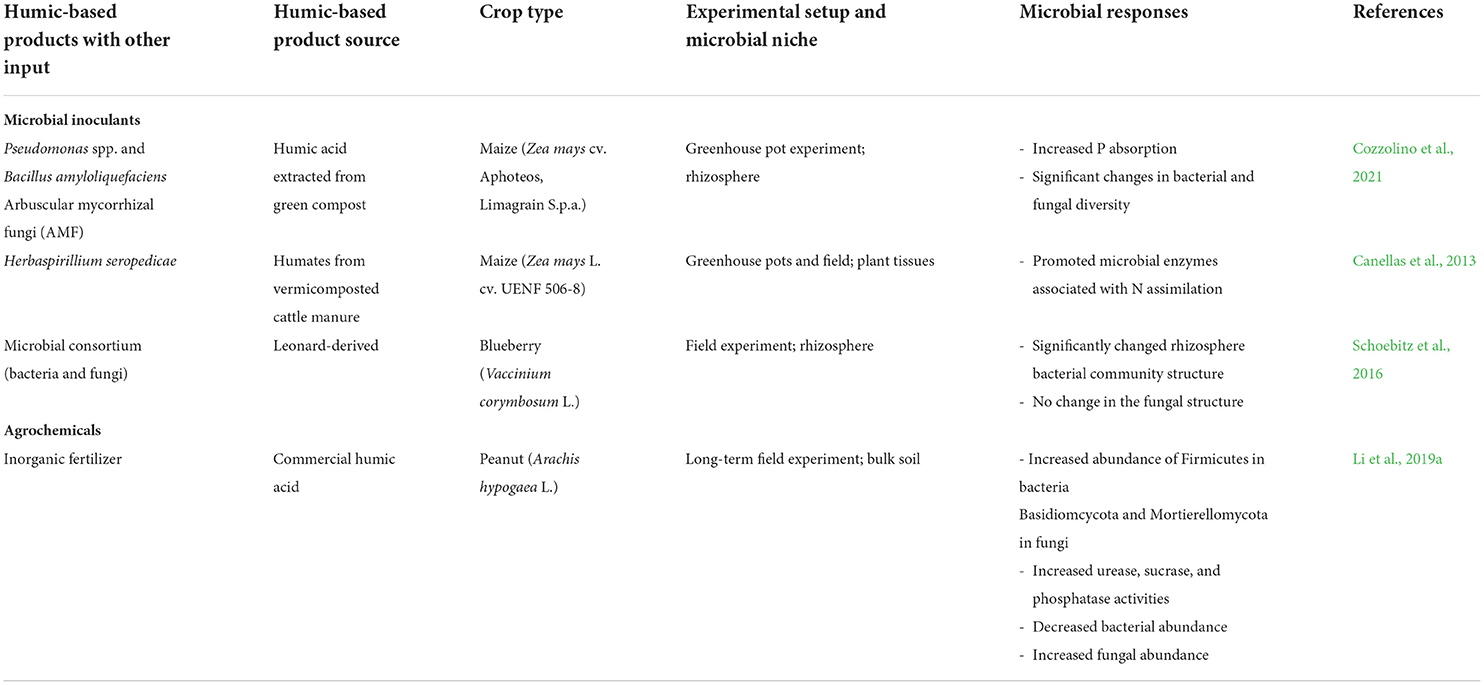
Table 2. The effect of humic-based products with other inputs on microbial community and activities.
Microbial enzymes associated with N assimilation were promoted when HA and Herbaspirillium seropedicae were applied to maize plants (Canellas et al., 2013). Similarly, HA application combined with Enterobacter sp. 32 A inoculant induced genes related to N assimilation in tomatoes (Galambos et al., 2020). Moreover, the combined application of beneficial bacteria and humate form HPs increased productivity in tomatoes and stimulated secondary metabolism and plant defense (Olivares et al., 2017). Galambos et al. (2020) also observed gene expression related to plant hormones, such as jasmonic acid, auxins, gibberellins, and cytokinins with the combined application of HA and plant growth-promoting bacteria.
Application of sodium humate to soybean inoculated with Bradyrhizobium in the presence of molybdate improved soybean yield, nodule number, and biological nitrogen fixation (Til'ba and Sinegovskaya, 2013). The greater efficiency of nodulation in the presence of HPs may be linked to the ability of these substances to regulate quorum sensing in rhizobia. Quorum sensing plays an essential role in the growth and development of legume-rhizobia symbiosis (Bogino et al., 2015). In the presence of water-soluble HPs, a greater increase in N fixation was observed in soybean inoculated with Bradyrhizobium liaoningense in greenhouse experiments (Guo Gao et al., 2015). These results revealed the direct effect of HPs on bacteria and their potential to improve microbial symbiosis with the host plant.
It was found that HPs (at a rate of ~ 800 mg/L) and saprophytic microorganisms as biofertilizers enhanced the growth of mycelium and mycorrhizal fungus formation by Glomus claroideum BEG23 in a hydroponic system, compared with control treatments with either only biofertilizer or no HS (Gryndler et al., 2005). Conversely, mycorrhizal colonization and hyphal length in laurel roots (Laurus nobilis L.) were inhibited by the presence of HS at a concentration of >800 mg/kg (Vallini et al., 1993).
Agrochemical fertilizers
HPs can increase the soil nutrient holding capacity from agrochemical inputs by enhancing the nutrient cycling within different compartments of the soil organic matters as well as the exchange of dissolved nutrients in the soil pore water (Ampong et al., 2022). Consequently, the amount of dissolved nutrients in the pore water can be reduced but is replenished over time. Since organic amendments could act as slow-release fertilizers, this beneficial situation could be maintained over long periods of time, in contrast to the mineral fertilizers alone. The combined application of humic acid and inorganic fertilizers increased peanut yield and quality in a long-term experiment (Li et al., 2019a). HA alleviate problems adherent to continuous cropping systems. In the presence of HA, plant-available NPK, and soil organic matter increase, resulting in increased plant NPK uptake (Li et al., 2019a). Additionally, improvements in soil physico-chemical properties and plant growth leads to enhanced soil microbial diversity and soil enzymatic activities (Li et al., 2019a).
Conclusions, future directions, and perspectives
Environmental problems and increasing production costs of chemical fertilizer use have driven interest in finding sustainable methods to maintain agricultural soil health. Biostimulants, such as humic substances from a raw or extracted form of oxidized lignite and extracted humic products (also popularly known as humic acids) from other organic materials, are being explored for their potential to reduce chemical fertilizer use. Despite extensive research on the agronomic benefits of HPs, their impacts on microbial communities are not fully explored. Interactions of HPs and microbial communities remain unknown, even though HPs are widely sold as biostimulants that purportedly stimulate beneficial bacteria in the rhizosphere. Until substantial research works have been done on the impact of various HPs on the soil across varying ecological zones, it is difficult to draw conclusions about the mechanisms and interactions of HPs regulating the microbial community structure and functions in the plant-soil interface. Current research results are contradictory due to the complex nature of HPs.
HPs are sold in various forms: humic acid, fulvic acid, humate and the properties vary depending on the source or origin. While microbial data on the effects of HPs are scarce, current results on their impacts on biogeochemical enzyme activities have also been contradictory. In addition, most research studies were performed under controlled environmental conditions, thus there is a need for field research across soil types covering various crop species and rotations. The effectiveness of HPs depends on the nature of humic materials and where they have been sourced (Rose et al., 2014). Different HPs properties, experimental setups, and biotic and abiotic conditions will have differing effects on the soil microbial structure and composition (Figure 2). Humic-based products can promote root growth and root exudation, which can also have indirect effects on the soil microbiome. The direct effects of HPs on microbial communities have yet to be unraveled. To decipher the impact of HPs, a more holistic approach to research should be done considering interactions among different crop species/genotype under varying ecosystems and environments.
Knowledge of the long-term effects of HP application on soil physical, chemical, and biological (microbial) properties remains unclear. We hope that the current advancements in sequencing technologies will accelerate more research on investigating humic-based products on microbial community structure and functions. Addressing the fundamental knowledge gaps will facilitate the successful application of HPs in agricultural crop production.
Author contributions
RL wrote the manuscript and contributed to the concept and outline of the manuscript. MT and LG contributed to the concept and outline of the manuscript, provided comments/suggestions, and edited the manuscript. All authors contributed to the article and approved the submitted version.
Funding
This research was jointly supported by Canadian Agricultural Partnership, Results Driven Agriculture Research (RDAR), and Prairie Mines and Royalty ULC (PMRU)—Project # 2022D008RC/RES0056474.
Acknowledgments
We acknowledge Michele Tran for creating the graph in Figure 2.
Conflict of interest
The authors declare that the research was conducted in the absence of any commercial or financial relationships that could be construed as a potential conflict of interest.
Publisher's note
All claims expressed in this article are solely those of the authors and do not necessarily represent those of their affiliated organizations, or those of the publisher, the editors and the reviewers. Any product that may be evaluated in this article, or claim that may be made by its manufacturer, is not guaranteed or endorsed by the publisher.
References
Akimbekov, N., Qiao, X., Digel, I., Abdieva, G., Ualieva, P., and Zhubanova, A. (2020). The effect of leonardite-derived amendments on soil microbiome structure and potato yield. Agriculture 10, 147. doi: 10.3390/agriculture10050147
Allison, S. D. (2006). Soil minerals and humic acids alter enzyme stability: implications for ecosystem processes. Biogeochemistry 81, 361–373. doi: 10.1007/s10533-006-9046-2
Ampong, K., Thilakaranthna, M. S., and Gorim, L. Y. (2022). Understanding the role of humic acids on crop performance and soil health. Front. Agron. 4, 10. doi: 10.3389/fagro.2022.848621
Anderson, C. R., Condron, L. M., Clough, T. J., Fiers, M., Stewart, A., Hill, R. A., et al. (2011). Biochar induced soil microbial community change: implications for biogeochemical cycling of carbon, nitrogen and phosphorus. Pedobiologia 54, 309–320. doi: 10.1016/j.pedobi.2011.07.005
Bais, H. P., Weir, T. L., Perry, L. G., Gilroy, S., and Vivanco, J. M. (2006). The role of root exudates in rhizosphere interactions with plants and other organisms. Annu. Rev. Plant Biol. 57, 233–266. doi: 10.1146/annurev.arplant.57.032905.105159
Banerjee, S., and van der Heijden, M. G. A. (2022). Soil microbiomes and one health. Nat. Rev. Microbiol. 38, 1–15. doi: 10.1038/s41579-022-00779-w
Berg, G. (2009). Plant-microbe interactions promoting plant growth and health: perspectives for controlled use of microorganisms in agriculture. Appl. Microbiol. Biotechnol. 84, 11–18. doi: 10.1007/s00253-009-2092-7
Bezuglova, O., and Klimenko, A. (2022). Application of humic substances in agricultural industry. Agronomy 12, 584. doi: 10.3390/agronomy12030584
Bezuglova, O. S., Gorovtsov, A. V., Polienko, E. A., Zinchenko, V. E., Grinko, A. V., Lykhman, V. A., et al. (2019). Effect of humic preparation on winter wheat productivity and rhizosphere microbial community under herbicide-induced stress. J. Soils Sediments 19, 2665–2675. doi: 10.1007/s11368-018-02240-z
Blagodatskaya, E., Blagodatsky, S., Anderson, T.-H., and Kuzyakov, Y. (2014). Microbial growth and carbon use efficiency in the rhizosphere and root-free soil. PLoS ONE 9, e93282. doi: 10.1371/journal.pone.0093282
Bogino, P. C., Nievas, F. L., and Giordano, W. (2015). A review: quorum sensing in Bradyrhizobium. Appl. Soil Ecol. 94, 49–58. doi: 10.1016/j.apsoil.2015.04.016
Braker, G., and Conrad, R. (2011). “Diversity, structure, and size of N2O-producing microbial communities in soils—what matters for their functioning?,” in Advances in Applied Microbiology, eds. A. I. Laskin, S. Sariaslani, and G. M. Gadd (New York, NY: Academic Press), 33–70. doi: 10.1016/B978-0-12-387046-9.00002-5
Canellas, L. P., Balmori, D. M., Médici, L. O., Aguiar, N. O., Campostrini, E., Rosa, R. C. C., et al. (2013). A combination of humic substances and Herbaspirillum seropedicae inoculation enhances the growth of maize (Zea mays L.). Plant Soil 366, 119–132. doi: 10.1007/s11104-012-1382-5
Canellas, L. P., and Olivares, F. L. (2014). Physiological responses to humic substances as plant growth promoter. Chem. Biol. Technol. Agric. 1, 1–11. doi: 10.1186/2196-5641-1-3
Canellas, L. P., and Olivares, F. L. (2017). Production of border cells and colonization of maize root tips by Herbaspirillum seropedicae are modulated by humic acid. Plant Soil 417, 403–413. doi: 10.1007/s11104-017-3267-0
Capstaff, N. M., Morrison, F., Cheema, J., Brett, P., Hill, L., Muñoz-García, J. C., et al. (2020). Fulvic acid increases forage legume growth inducing preferential up-regulation of nodulation and signalling-related genes. J. Exp. Bot. 71, 5689–5704. doi: 10.1093/jxb/eraa283
Cassman, K. G., Dobermann, A., and Walters, D. T. (2002). Agroecosystems, nitrogen-use efficiency, and nitrogen management. AMBIO 31, 132–140. doi: 10.1579/0044-7447-31.2.132
Chaiharn, M., and Lumyong, S. (2011). Screening and optimization of indole-3-acetic acid production and phosphate solubilization from rhizobacteria aimed at improving plant growth. Curr. Microbiol. 62, 173–181. doi: 10.1007/s00284-010-9674-6
Chaparro, J. M., Sheflin, A. M., Manter, D. K., and Vivanco, J. M. (2012). Manipulating the soil microbiome to increase soil health and plant fertility. Biol. Fertil. Soils 48, 489–499. doi: 10.1007/s00374-012-0691-4
Chen, J., Liu, X., Li, L., Zheng, J., Qu, J., Zheng, J., et al. (2015). Consistent increase in abundance and diversity but variable change in community composition of bacteria in topsoil of rice paddy under short term biochar treatment across three sites from South China. Appl. Soil Ecol. 91, 68–79. doi: 10.1016/j.apsoil.2015.02.012
Chu, H., Fierer, N., Lauber, C. L., Caporaso, J. G., Knight, R., and Grogan, P. (2010). Soil bacterial diversity in the Arctic is not fundamentally different from that found in other biomes. Environ. Microbiol. 12, 2998–3006. doi: 10.1111/j.1462-2920.2010.02277.x
Conrad, R. (1996). Soil microorganisms as controllers of atmospheric trace gases (H2, CO, CH4, OCS, N2O, and NO). Microbiol. Rev. 60, 609–640. doi: 10.1128/mr.60.4.609-640.1996
Cozzolino, V., Monda, H., Savy, D., di Meo, V., Vinci, G., Smalla, K., et al. (2021). Cooperation among phosphate-solubilizing bacteria, humic acids and arbuscular mycorrhizal fungi induces soil microbiome shifts and enhances plant nutrient uptake. Chem. Biol. Technol. Agric. 8, 1–18. doi: 10.1186/s40538-021-00230-x
da Silva, M. S. R. A., Tavares, O. C. H., Ribeiro, T. G., de Andrade da Silva, C. S. R., García-Mina, J. M., Baldani, V. L. D., et al. (2021). Humic acids enrich the plant microbiota with bacterial candidates for the suppression of pathogens. Appl. Soil Ecol. 168, 104146. doi: 10.1016/j.apsoil.2021.104146
Dai, Z., Liu, G., Chen, H., Chen, C., Wang, J., Ai, S., et al. (2020). Long-term nutrient inputs shift soil microbial functional profiles of phosphorus cycling in diverse agroecosystems. ISME J. 14, 757–770. doi: 10.1038/s41396-019-0567-9
Dong, L., Córdova-Kreylos, A. L., Yang, J., Yuan, H., and Scow, K. M. (2009). Humic acids buffer the effects of urea on soil ammonia oxidizers and potential nitrification. Soil Biol. Biochem. 41, 1612–1621. doi: 10.1016/j.soilbio.2009.04.023
Dong, L. H., Yang, J. S., Yuan, H. L., Wang, E. T., and Chen, W. X. (2008). Chemical characteristics and influences of two fractions of Chinese lignite humic acids on urease. Eur. J. Soil Biol. 44, 166–171. doi: 10.1016/j.ejsobi.2007.07.002
Dong, S., Li, M., and Chen, Y. (2017). Inherent humic substance promotes microbial denitrification of landfill leachate via shifting bacterial community, improving enzyme activity and up-regulating gene. Sci. Rep. 7, 12215. doi: 10.1038/s41598-017-12565-3
Dou, R.-N., Wang, J.-H., Chen, Y.-C., and Hu, Y.-Y. (2018). The transformation of triclosan by laccase: Effect of humic acid on the reaction kinetics, products and pathway. Environ. Pollut. 234, 88–95. doi: 10.1016/j.envpol.2017.10.119
Drenovsky, R. E., Vo, D., Graham, K. J., and Scow, K. M. (2004). Soil water content and organic carbon availability are major determinants of soil microbial community composition. Microb. Ecol. 48, 424–430. doi: 10.1007/s00248-003-1063-2
Driouich, A., Cannesan, M.-A., Dardelle, F., Durand, C., Plancot, B., Bernard, S., et al. (2012). “Unity is strength: the power of border cells and border-like cells in relation with plant defense,” in Secretions and Exudates in Biological Systems. Signaling and Communication in Plants, eds. J. Vivanco and F. Baluška (Berlin; Heidelberg: Springer-Verlag), 91–107. doi: 10.1007/978-3-642-23047-9_5
Ducey, T. F., Ippolito, J. A., Cantrell, K. B., Novak, J. M., and Lentz, R. D. (2013). Addition of activated switchgrass biochar to an aridic subsoil increases microbial nitrogen cycling gene abundances. Appl. Soil Ecol. 65, 65–72. doi: 10.1016/j.apsoil.2013.01.006
El-shazly, M. D., Henderson, B., and Beall, G. W. (2015). Reduced humic acid nanosheets and its uses as nanofiller. J. Phys. Chem. Solids 85, 86–90. doi: 10.1016/j.jpcs.2015.05.001
Galambos, N., Compant, S., Moretto, M., Sicher, C., Puopolo, G., Wäckers, F., et al. (2020). Humic acid enhances the growth of tomato promoted by endophytic bacterial strains through the activation of hormone-, growth-, and transcription-related processes. Front. Plant Sci. 11, 582267. doi: 10.3389/fpls.2020.582267
Galloway, J. N., Townsend, A. R., Erisman, J. W., Bekunda, M., Cai, Z., Freney, J. R., et al. (2008). Transformation of the nitrogen cycle: recent trends, questions, and potential solutions. Science 320, 889–892. doi: 10.1126/science.1136674
Glibert, P. M., Harrison, J., Heil, C., and Seitzinger, S. (2006). Escalating worldwide use of urea – a global change contributing to coastal eutrophication. Biogeochemistry 77, 441–463. doi: 10.1007/s10533-005-3070-5
Gorim, L., and Asch, F. (2012). Effects of composition and share of seed coatings on the mobilization efficiency of cereal seeds during germination. J. Agron. Crop Sci. 198, 81–91. doi: 10.1111/j.1439-037X.2011.00490.x
Griffiths, B. S., Spilles, A., and Bonkowski, M. (2012). C:N:P stoichiometry and nutrient limitation of the soil microbial biomass in a grazed grassland site under experimental P limitation or excess. Ecol. Process 1, 1–11. doi: 10.1186/2192-1709-1-6
Gryndler, M., Hršelová, H., Sudová, R., Gryndlerová, H., Rezáčová, V., and Merhautová, V. (2005). Hyphal growth and mycorrhiza formation by the arbuscular mycorrhizal fungus Glomus claroideum BEG 23 is stimulated by humic substances. Mycorrhiza 15, 483–488. doi: 10.1007/s00572-005-0352-7
Güereña, D. T., Lehmann, J., Thies, J. E., Enders, A., Karanja, N., and Neufeldt, H. (2015). Partitioning the contributions of biochar properties to enhanced biological nitrogen fixation in common bean (Phaseolus vulgaris). Biol. Fertil. Soils 51, 479–491. doi: 10.1007/s00374-014-0990-z
Guo Gao, T., Yuan Xu, Y., Jiang, F., Zhen Li, B., Shui Yang, J., Tao Wang, E., et al. (2015). Nodulation characterization and proteomic profiling of Bradyrhizobium liaoningense CCBAU05525 in response to water-soluble humic materials. Sci. Rep. 5, 10836. doi: 10.1038/srep10836
Guo, X., Liu, H., and Wu, S. (2019). Humic substances developed during organic waste composting: Formation mechanisms, structural properties, and agronomic functions. Sci. Total Environ. 662, 501–510. doi: 10.1016/j.scitotenv.2019.01.137
Harter, J., Krause, H.-M., Schuettler, S., Ruser, R., Fromme, M., Scholten, T., et al. (2014). Linking N2O emissions from biochar-amended soil to the structure and function of the N-cycling microbial community. ISME J. 8, 660–674. doi: 10.1038/ismej.2013.160
Hita, D., de Fuentes, M., Zamarreño, A. M., Ruiz, Y., Garcia-Mina, J. M., de Hita, D., et al. (2020). Culturable bacterial endophytes from sedimentary humic acid-treated plants. Front. Plant Sci. 11, 837. doi: 10.3389/fpls.2020.00837
Holford, I. C. R. (1997). Soil phosphorus: its measurement, and its uptake by plants. Soil Res. 35, 227–240. doi: 10.1071/S96047
Hsu, S.-F., and Buckley, D. H. (2009). Evidence for the functional significance of diazotroph community structure in soil. ISME J. 3, 124–136. doi: 10.1038/ismej.2008.82
Jackson, L. E., Burger, M., and Cavagnaro, T. R. (2008). Roots, nitrogen transformations, and ecosystem services. Annu. Rev. Plant Biol. 59, 341–363. doi: 10.1146/annurev.arplant.59.032607.092932
Jindo, K., Canellas, L., Albacete, A., Figueiredo dos Santos, L., Frinhani Rocha, R., Carvalho Baia, D., et al. (2020). Interaction between humic substances and plant hormones for phosphorous acquisition. Agronomy 10, 640. doi: 10.3390/agronomy10050640
Khaled, H., and Fawy, H. A. (2011). Effect of different levels of humic acids on the nutrient content, plant growth, and soil properties under conditions of salinity. Soil Water Res. 6, 21–29. doi: 10.17221/4/2010-SWR
Kleber, M., and Lehmann, J. (2019). Humic substances extracted by alkali are invalid proxies for the dynamics and functions of organic matter in terrestrial and aquatic ecosystems. J. Environ. Qual. 48, 207–216. doi: 10.2134/jeq2019.01.0036
Lam, P., and Kuypers, M. M. M. (2011). Microbial nitrogen cycling processes in oxygen minimum zones. Ann. Rev. Mar. Sci. 3, 317–345. doi: 10.1146/annurev-marine-120709-142814
Lauber, C. L., Hamady, M., Knight, R., and Fierer, N. (2009). Pyrosequencing-based assessment of soil pH as a predictor of soil bacterial community structure at the continental scale. Appl. Environ. Microbiol. 75, 5111–5120. doi: 10.1128/AEM.00335-09
Lehmann, J., and Kleber, M. (2015). The contentious nature of soil organic matter. Nature 528, 60–68. doi: 10.1038/nature16069
Leonard, C. (2012). The Use of Humic Substances In Agriculture: Origins, Science and Applications. Available online at: https://cdn2.hubspot.net/hub/148034/file-17893304-pdf/docs/humic_substances-white-paper.pdf (accessed June 22, 2022).
Li, C., Xiong, Y., Zou, J., Dong, L., Ren, P., and Huang, G. (2021). Impact of biochar and lignite-based amendments on microbial communities and greenhouse gas emissions from agricultural soil. Vadose Zone J. 20, e20105. doi: 10.1002/vzj2.20105
Li, M., Su, Y., Chen, Y., Wan, R., Zheng, X., and Liu, K. (2016). The effects of fulvic acid on microbial denitrification: promotion of NADH generation, electron transfer, and consumption. Appl. Microbiol. Biotechnol. 100, 5607–5618. doi: 10.1007/s00253-016-7383-1
Li, S., Braun, J.-C., Buchner, D., and Haderlein, S. B. (2019). Denitrifier method for nitrite removal in electrochemical analysis of the electron accepting capacity of humic substances. Anal. Chem. 92, 616–621. doi: 10.1021/acs.analchem.9b03683
Li, Y., Fang, F., Wei, J., Wu, X., Cui, R., Li, G., et al. (2019a). Humic acid fertilizer improved soil properties and soil microbial diversity of continuous cropping peanut: a three-year experiment. Sci. Rep. 9, 12014. doi: 10.1038/s41598-019-48620-4
Li, Y., Nie, C., Liu, Y., Du, W., and He, P. (2019b). Soil microbial community composition closely associates with specific enzyme activities and soil carbon chemistry in a long-term nitrogen fertilized grassland. Sci. Total Environ. 654, 264–274. doi: 10.1016/j.scitotenv.2018.11.031
Li, Y., Tan, W., Koopal, L. K., Wang, M., Liu, F., and Norde, W. (2013). Influence of soil humic and fulvic acid on the activity and stability of lysozyme and urease. Environ. Sci. Technol. 47, 5050–5056. doi: 10.1021/es3053027
Liu, L., Ji, M., Wang, F., Tian, Z., Wang, T., Wang, S., et al. (2020). Insight into the short-term effect of fulvic acid on nitrogen removal performance and N-acylated-L-homoserine lactones (AHLs) release in the anammox system. Sci. Total Environ. 704, 135285. doi: 10.1016/j.scitotenv.2019.135285
Liu, Y., Han, Y., Zhang, J., Hou, Y., Song, Y., Lu, C., et al. (2022). Deciphering effects of humic acid in landfill leachate on the simultaneous nitrification, anammox and denitrification (SNAD) system from performance, electron transfer and microbial community. Sci. Total Environ. 809, 151178. doi: 10.1016/j.scitotenv.2021.151178
Luo, X., Shen, L., and Meng, F. (2019). Response of microbial community structures and functions of nitrosifying consortia to biorefractory humic substances. ACS Sustain. Chem. Eng. 7, 4744–4754. doi: 10.1021/acssuschemeng.8b04853
Ma, B., Ma, B.-L., McLaughlin, N. B., Li, M., and Liu, J. (2022). Improvement in dryland crop performance and soil properties with multiple annual applications of lignite-derived humic amendment. Soil Tillage Res. 218, 105306. doi: 10.1016/j.still.2021.105306
Madsen, E. L. (2011). Microorganisms and their roles in fundamental biogeochemical cycles. Curr. Opin. Biotechnol. 22, 456–464. doi: 10.1016/j.copbio.2011.01.008
Maji, D., Misra, P., Singh, S., and Kalra, A. (2017). Humic acid rich vermicompost promotes plant growth by improving microbial community structure of soil as well as root nodulation and mycorrhizal colonization in the roots of Pisum sativum. Appl. Soil Ecol. 110, 97–108. doi: 10.1016/j.apsoil.2016.10.008
Malcolm, R. E., and Vaughan, D. (1979). Humic substances and phosphatase activities in plant tissues. Soil Biol. Biochem. 11, 253–259. doi: 10.1016/0038-0717(79)90070-1
Malhi, S. S., Grant, C. A., Johnston, A. M., and Gill, K. S. (2001). Nitrogen fertilization management for no-till cereal production in the Canadian Great Plains: a review. Soil Tillage Res. 60, 101–122. doi: 10.1016/S0167-1987(01)00176-3
Marzadori, C., Francioso, O., Ciavatta, C., and Gessa, C. (2000a). Activity and stability of jack bean urease in the presence of peat humic acids obtained using different extractants. Biol. Fertil. Soils 32, 415–420. doi: 10.1007/s003740000272
Marzadori, C., Francioso, O., Ciavatta, C., and Gessa, C. (2000b). The influence of the content of heavy metals and molecular weight of humic acids fractions on the activity and stability of urease. Soil Biol. Biochem. 32, 1893–1898. doi: 10.1016/S0038-0717(00)00163-2
Mato, M. C., Fábregas, R., and Méndez, J. (1971). Inhibitory effect of soil humic acids on indoleacetic acid-oxidase. Soil Biol. Biochem. 3, 285–288. doi: 10.1016/0038-0717(71)90037-X
Mato, M. C., Olmedo, M. G., and Méndez, J. (1972). Inhibition of indoleacetic acid-oxidase by soil humic acids fractionated on sephadex. Soil Biol. Biochem. 4, 469–473. doi: 10.1016/0038-0717(72)90062-4
Mazzei, P., Oschkinat, H., and Piccolo, A. (2013). Reduced activity of alkaline phosphatase due to host–guest interactions with humic superstructures. Chemosphere 93, 1972–1979. doi: 10.1016/j.chemosphere.2013.07.015
Mazzei, P., and Piccolo, A. (2013). Reduced activity of β-glucosidase resulting from host-guest interactions with dissolved fulvic acids as revealed by NMR spectroscopy. Eur. J. Soil Sci. 64, 508–515. doi: 10.1111/ejss.12044
Mia, S., van Groenigen, J. W., van de Voorde, T. F. J., Oram, N. J., Bezemer, T. M., Mommer, L., et al. (2014). Biochar application rate affects biological nitrogen fixation in red clover conditional on potassium availability. Agric. Ecosyst. Environ. 191, 83–91. doi: 10.1016/j.agee.2014.03.011
Nannipieri, P., Ascher-Jenull, J., Ceccherini, M. T., Pietramellara, G., Renella, G., and Schloter, M. (2020). Beyond microbial diversity for predicting soil functions: a mini review. Pedosphere 30, 5–17. doi: 10.1016/S1002-0160(19)60824-6
Nannipieri, P., Giagnoni, L., Renella, G., Puglisi, E., Ceccanti, B., Masciandaro, G., et al. (2012). Soil enzymology: classical and molecular approaches. Biol. Fertil. Soils 48, 743–762. doi: 10.1007/s00374-012-0723-0
Nardi, S., Schiavon, M., and Francioso, O. (2021). Chemical structure and biological activity of humic substances define their role as plant growth promoters. Molecules 26, 2256. doi: 10.3390/molecules26082256
Neves, E. G., Petersen, J. B., Bartone, R. N., and Heckenberger, M. J. (2004). “The timing of formation in the central Amazon: Archaeological data from three sites,” in Amazonian Dark Earths: Explorations in Space and Time, (Berlin, Heidelberg: Springer Berlin Heidelberg), 125–134. doi: 10.1007/978-3-662-05683-7_9
Nicol, G. W., Leininger, S., Schleper, C., and Prosser, J. I. (2008). The influence of soil pH on the diversity, abundance and transcriptional activity of ammonia oxidizing archaea and bacteria. Environ. Microbiol. 10, 2966–2978. doi: 10.1111/j.1462-2920.2008.01701.x
Norton, J. M., and Stark, J. M. (2011). Regulation and measurement of nitrification in terrestrial systems. Meth. Enzymol. 486, 343–368. doi: 10.1016/B978-0-12-381294-0.00015-8
Novotny, E. H., Hayes, M. H. B., Madari, B. E., Bonagamba, T. J., Azevedo, E. R., de Souza, A. A., et al. (2009). Lessons from the Terra Preta de Índios of the Amazon region for the utilisation of charcoal for soil amendment. J. Braz. Chem. Soc. 20. 1003–1010. doi: 10.1590/S0103-50532009000600002
O'donnell, R. (1973). The auxin-like effects of humic preparations from leonardite. Soil Sci. 116, 106–112. doi: 10.1097/00010694-197308000-00007
Olivares, F. L., Aguiar, N. O., Rosa, R. C. C., and Canellas, L. P. (2015). Substrate biofortification in combination with foliar sprays of plant growth promoting bacteria and humic substances boosts production of organic tomatoes. Sci. Hortic. 183, 100–108. doi: 10.1016/j.scienta.2014.11.012
Olivares, F. L., Busato, J. G., de Paula, A. M., da Silva Lima, L., Aguiar, N. O., and Canellas, L. P. (2017). Plant growth promoting bacteria and humic substances: crop promotion and mechanisms of action. Chem. Biol. Technol. Agric. 4, 30. doi: 10.1186/s40538-017-0112-x
Olk, D. C., Dinnes, D. L., Scoresby, J. R., Callaway, C. R., and Darlington, J. W. (2018). Humic products in agriculture: potential benefits and research challenges—a review. J. Soils Sediments 18, 2881–2891. doi: 10.1007/s11368-018-1916-4
Paez-Espino, D., Eloe-Fadrosh, E. A., Pavlopoulos, G. A., Thomas, A. D., Huntemann, M., Mikhailova, N., et al. (2016). Uncovering Earth's virome. Nature 536, 425–430. doi: 10.1038/nature19094
Pflug, W. (1980). Effect of humic acids on the activity of two peroxidases. Zeitschrift Pflanzenernährung Bodenkunde 143, 432–440. doi: 10.1002/jpln.19801430409
Pflug, W., and Ziechmann, W. (1981). Inhibition of malate dehydrogenase by humic acids. Soil Biol. Biochem. 13, 293–299. doi: 10.1016/0038-0717(81)90065-1
Philippot, L., Hallin, S., and Schloter, M. (2007). Ecology of denitrifying prokaryotes in agricultural soil. Adv. Agron. 96, 249–305. doi: 10.1016/S0065-2113(07)96003-4
Pozdnyakov, L. A., Stepanov, A. L., Gasanov, M. E., Semenov, M. V., Yakimenko, O. S., Suada, I. K., et al. (2020). Effect of lignohumate on soil biological activity on the Bali island, Indonesia. Eurasian Soil Sci. 53, 653–660. doi: 10.1134/S1064229320050117
Puglisi, E., Fragoulis, G., Ricciuti, P., Cappa, F., Spaccini, R., Piccolo, A., et al. (2009). Effects of a humic acid and its size-fractions on the bacterial community of soil rhizosphere under maize (Zea mays L.). Chemosphere 77, 829–837. doi: 10.1016/j.chemosphere.2009.07.077
Puglisi, E., Pascazio, S., Suciu, N., Cattani, I., Fait, G., Spaccini, R., et al. (2013). Rhizosphere microbial diversity as influenced by humic substance amendments and chemical composition of rhizodeposits. J. Geochem. Explor. 129, 82–94. doi: 10.1016/j.gexplo.2012.10.006
Puglisi, E., and Trevisan, M. (2013). “Combining rhizobox, reporter gene systems, and molecular analyses to assess the effects of humic substances on plant–microbes interactions in soil rhizosphere,” in Molecular Microbial Ecology of the Rhizosphere, ed F. J. de Bruijn (Hoboken, NJ: John Wiley & Sons. Inc.), 933–942. doi: 10.1002/9781118297674.ch88
Pulidindi, K., and Prakash, A. (2021). Humic Acid Market Size, by Application (Agriculture, Ecological Bioremediation, Horticulture, Dietary Supplements), Industry Analysis Report, Regional Outlook, COVID-19 Impact Analysis, Growth Potential, Price Trends, Competitive Market Share & Forecast, 2022 – 2028. Available online at: https://www.gminsights.com/industry-analysis/humic-acid-market (accessed June 13, 2022).
Quilliam, R. S., DeLuca, T. H., and Jones, D. L. (2013). Biochar application reduces nodulation but increases nitrogenase activity in clover. Plant Soil 366, 83–92. doi: 10.1007/s11104-012-1411-4
Richardson, A. E., Barea, J. M., McNeill, A. M., and Prigent-Combaret, C. (2009). Acquisition of phosphorus and nitrogen in the rhizosphere and plant growth promotion by microorganisms. Plant Soil 321, 305–339. doi: 10.1007/s11104-009-9895-2
Rose, M. T., Patti, A. F., Little, K. R., Brown, A. L., Jackson, W. R., and Cavagnaro, T. R. (2014). A meta-analysis and review of plant-growth response to humic substances: practical implications for agriculture. Adv. Agron. 124, 37–89. doi: 10.1016/B978-0-12-800138-7.00002-4
Saarnio, S., Heimonen, K., and Kettunen, R. (2013). Biochar addition indirectly affects N2O emissions via soil moisture and plant N uptake. Soil Biol. Biochem. 58, 99–106. doi: 10.1016/j.soilbio.2012.10.035
Schoebitz, M., López, M. D., Serrí, H., Martínez, O., and Zagal, E. (2016). Combined application of microbial consortium and humic substances to improve the growth performance of blueberry seedlings. J. Soil Sci. Plant Nutr. 16, 1010–1023. doi: 10.4067/S0718-95162016005000074
Sellamuthu, K. M., and Govindaswamy, M. (2003). Effect of fertiliser and humic acid on rhizosphere microorganisms and soil enzymes at an early stage of sugarcane growth. Sugar Tech. 5, 273–277. doi: 10.1007/BF02942484
Silva, V., Mol, H. G. J., Zomer, P., Tienstra, M., Ritsema, C. J., and Geissen, V. (2019). Pesticide residues in European agricultural soils – A hidden reality unfolded. Sci. Total Environ. 653, 1532–1545. doi: 10.1016/j.scitotenv.2018.10.441
Suttle, C. A. (2007). Marine viruses — major players in the global ecosystem. Nat. Rev. Microbiol. 5, 801–812. doi: 10.1038/nrmicro1750
Til'ba, V. A., and Sinegovskaya, V. T. (2013). Role of symbiotic nitrogen fixation in increasing photosynthetic productivity of soybean. Russ. Agric. Sci. 38, 361–363. doi: 10.3103/S1068367412050199
Tomaszewski, J. E., Schwarzenbach, R. P., and Sander, M. (2011). Protein encapsulation by humic substances. Environ. Sci. Technol. 45, 6003–6010. doi: 10.1021/es200663h
Vallini, G., Pera, A., Avio, L., Valdrighi, M., and Giovannetti, M. (1993). Influence of humic acids on laurel growth, associated rhizospheric microorganisms, and mycorrhizal fungi. Biol. Fertil. Soils 16, 1–4. doi: 10.1007/BF00336506
van Trump, J. I., Sun, Y., and Coates, J. D. (2006). Microbial interactions with humic substances. Adv. Appl. Microbiol. 60, 55–96. doi: 10.1016/S0065-2164(06)60003-8
van Trump, J. I., Wrighton, K. C., Thrash, J. C., Weber, K. A., Andersen, G. L., and Coates, J. D. (2011). Humic acid-oxidizing, nitrate-reducing bacteria in agricultural soils. MBio 2, 44–55. doi: 10.1128/mBio.00044-11
Xiao, W., Chen, X., Jing, X., and Zhu, B. (2018). A meta-analysis of soil extracellular enzyme activities in response to global change. Soil Biol. Biochem. 123, 21–32. doi: 10.1016/j.soilbio.2018.05.001
Xu, N., Tan, G., Wang, H., and Gai, X. (2016). Effect of biochar additions to soil on nitrogen leaching, microbial biomass and bacterial community structure. Eur. J. Soil Biol. 74, 1–8. doi: 10.1016/j.ejsobi.2016.02.004
Yang, F., Tang, C., and Antonietti, M. (2021). Natural and artificial humic substances to manage minerals, ions, water, and soil microorganisms. Chem. Soc. Rev. 50, 6221–6239. doi: 10.1039/D0CS01363C
Zhang, L., Jing, Y., Xiang, Y., Zhang, R., and Lu, H. (2018). Responses of soil microbial community structure changes and activities to biochar addition: a meta-analysis. Sci. Total Environ. 643, 926–935. doi: 10.1016/j.scitotenv.2018.06.231
Zhang, L., Lan, S., Dou, Q., Hao, S., Wang, Y., Wang, X., et al. (2023). Metagenomic insights into responses of microbial population and key functional genes to fulvic acid during partial nitritation. J. Environ. Sci. 124, 952–962. doi: 10.1016/j.jes.2022.03.003
Zhou, L., Yuan, L., Zhao, B., Li, Y., and Lin, Z. (2019). Structural characteristics of humic acids derived from Chinese weathered coal under different oxidizing conditions. PLoS ONE 14, e0217469. doi: 10.1371/journal.pone.0217469
Zhu, Y., Pierson III, L. S., and Hawes, M. C. (1997). Induction of microbial genes for pathogenesis and symbiosis by chemicals from root border cells. Plant Physiol. 115, 1691–1698. doi: 10.1104/pp.115.4.1691
Keywords: humic-based products, humic acids, soil amendments, biostimulants, microbial communities, microbial activities
Citation: Lumactud RA, Gorim LY and Thilakarathna MS (2022) Impacts of humic-based products on the microbial community structure and functions toward sustainable agriculture. Front. Sustain. Food Syst. 6:977121. doi: 10.3389/fsufs.2022.977121
Received: 24 June 2022; Accepted: 07 November 2022;
Published: 22 November 2022.
Edited by:
Marouane Baslam, Niigata University, JapanReviewed by:
S. Rakesh, National Academy of Agricultural Research Management (ICAR), IndiaElshazly Duraia, Suez Canal University, Egypt
Copyright © 2022 Lumactud, Gorim and Thilakarathna. This is an open-access article distributed under the terms of the Creative Commons Attribution License (CC BY). The use, distribution or reproduction in other forums is permitted, provided the original author(s) and the copyright owner(s) are credited and that the original publication in this journal is cited, in accordance with accepted academic practice. No use, distribution or reproduction is permitted which does not comply with these terms.
*Correspondence: Malinda S. Thilakarathna, malinda.thilakarathna@ualberta.ca