- 1Unit of Microbiology, Department of Biology, Federal University of Lavras, Lavras, Brazil
- 2Center for Agricultural and Agro-Food Biotechnology of Alentejo (CEBAL), Beja, Portugal
- 3Unit of Microbiology, Department of Soil Science, Federal University of Lavras, Lavras, Brazil
- 4Unit of Tissue Culture, Department of Agriculture, Federal University of Lavras, Lavras, Brazil
- 5Institute of Natural Resources, Federal University of Itajubá, Itajubá, Brazil
- 6Department of Agriculture, São Paulo State University, Jaboticabal, Brazil
Organic wastes are considered the most significant components of urban solid waste, negatively affecting the environment. It is essential to use renewable resources to minimize environmental risks. Composting is one of the most sustainable methods for managing organic waste and involves transforming organic matter into a stable and nutrient-enriched biofertilizer, through the succession of microbial populations into a stabilized product. This work aimed to evaluate the efficiency of the new type of composter and the microbial and physiochemical dynamics during composting aiming to accelerate the degradation of organic waste and produce high-quality compost. Two inoculants were evaluated: (1) efficient microorganisms (EM); (2) commercial inoculum (CI), which were compared to a control treatment, without inoculation. Composting was performed by mixing organic waste from gardening with residues from the University's Restaurant (C/N ratio 30:1). The composting process was carried out in a 1 m3 composter with controlled temperature and aeration. The thermophilic phase for all treatments was reached on the second day. Mature compost was obtained after an average of 120 days, and composting in all treatments showed an increase in the availability of P and micronutrients. The new composter helped to accelerate the decomposition of residues, through the maintenance of adequate oxygen content and temperature control inside the cells, providing high metabolic activity of microorganisms, contributing to an increase in physicochemical characteristics, also reducing the composting time in both treatments. During composting, the bacteria and actinobacteria populations were higher than yeasts and filamentous fungi. The inoculated treatments presented advantages showing more significant mineralization of P-available and micronutrients such as Mn and Zn in terms of the quality of the final product in comparison to the control treatment. Finally, the new composter and the addition of inoculants contributed significantly to the efficiency of the process of composting organic waste.
Introduction
Food or organic wastes are considered the most significant components of urban solid waste and account for ~55% of total waste in developing countries, which face more significant challenges with food waste management than developed countries (Cerda et al., 2018; Oviedo-Ocaña et al., 2019; Awasthi et al., 2020). Globally, one-third of all food produced is wasted along the production chain (Awasthi et al., 2020). Organic waste treatment is a serious and urgent matter, and since such waste can negatively affect environmental health, sustainable technologies must be implemented to reduce the potential danger of unmanaged organic waste (Awasthi et al., 2020; Ince et al., 2020).
Organic waste treatment methods commonly used in developing countries include use as feed (rare), compost (1% to 6%), anaerobic digestion (<0.6%), and incineration and landfills (>90%) (Thi et al., 2015; Puspitaloka et al., 2022). Compost produced for agricultural use from food waste has received considerable attention, due to its low cost and the possibility of on-site processing (Awasthi et al., 2021), being recently considered a promising solution for organic waste treatment (Zhang et al., 2021). Composting involves transforming organic matter into a stable and nutrient-enriched biofertilizer, through the succession of microbial populations into a stabilized product and is considered an efficient and inexpensive technology for recycling organic waste (Zhang and Sun, 2018; Obeng et al., 2021). Beyond that, composting can help reduce CO2 emissions and energy consumption in agriculture (Deus et al., 2017; Bruni et al., 2020).
Several types of waste from different production chains have been used as base material for composting, including mature compost (resulting from composting in its stabilized form) (Wang et al., 2022). In addition, substrates such as residual sludge (Ince et al., 2020), green waste combined with food waste (Oviedo-Ocaña et al., 2019), and flower waste (Sharma et al., 2018), biodigester sludge combined with mature compost and sawdust (Huang et al., 2017). The combination of more than one raw material optimizes the processing of each component and the composting process (Awasthi et al., 2020).
Piles and windrows are the most commonly used stacking systems for composting, as they are easy to operate and require less equipment (Liu et al., 2020). However, they tend to be slower, requiring a relatively long time (Tong et al., 2019). In addition, composting by traditional methods involves problems with inadequate ventilation, uneven mixing, and other challenges (Liu et al., 2020). In this context, these disadvantages can be reduced by using composters that help control the process and provide optimal conditions for quality compost (Külcü and Yaldiz, 2014; Soto-Paz et al., 2019; Liu et al., 2020). The use of composters with forced ventilation and regular rotation are used in order to accelerate the composting process (Soto-Paz et al., 2019). Several aeration techniques for composting outdoor piles, including forced or passive aeration, have been extensively investigated in the literature and several types of composters have been developed with the intention of contributing positively to the composting process (Soto-Paz et al., 2019; Liu et al., 2020).
During the composting process, specific parameters are important to obtain quality compost, such as the C/N ratio, oxygen availability, pH, temperature, aeration, moisture content, type of composter, particle size, composting time, and humic acid presence, usually produced at the end of composting (Abdolali et al., 2014; Li et al., 2017; Wang et al., 2021b; Zhang et al., 2021). In addition, pile turning during the degradation stage is key to maintaining microbial diversity and, to some extent, the population profile present at the beginning of the process (Antunes et al., 2016). The physicochemical characterization of residues of composting determines the nature and level of biodegradation of residues organics, and such data can help a different maturity of composting, which provides a better agricultural application (Oviedo-Ocaña et al., 2019).
Throughout the composting process, there is a change in temperature due to the biological activity of microorganisms classified as mesophilic (15–45°C), thermophilic (45–80°C), and hyperthermophilic (>80°C) phases (Pan and Sen, 2013; Liu et al., 2022a). In the thermophilic and hyperthermophilic phase, normally actinobacteria and gram-positive bacteria are observed, which could suppress several pathogens, leading to reduced use of chemical controls, such as pesticides (Milinković et al., 2019). After this phase occurs the stabilization of compost temperature and the start of the bio-oxidative phase, where the microbial activity provides the biomineralization of organic compounds (Zhang and Sun, 2019).
Generally, the composting occurs with natural microbiota, including bacteria, actinobacteria, yeasts, and filamentous fungi, which are generally involved in the degradation of lignocellulosic components during aerobic composting, but make the composting process too long (Bohacz, 2017; Zhang et al., 2021; Wang et al., 2022). However, the utilization of biological methods such as the inoculation of selected microorganisms can provide high efficiency for waste management. These microorganisms can provide substantial improvement in the rate of waste degradation, reducing the composting time and improving the quality of the compost product (Nakasaki et al., 2013; Zhang and Sun, 2014; Kinet et al., 2015; Onwosi et al., 2017; Liu et al., 2018).
Several studies have identified inoculums of microorganisms that can promote the maturation of compost by increasing the microbial activity during the composting process (Sarkar et al., 2010; Yang et al., 2019) and have shown benefits of using inoculants in the physical structure of the compost (Wang et al., 2016). In general, the composting process depends on the combinations of composting mass and its relevant ratios, i.e., the carbon: nitrogen (C:N) ratio, the presence or absence of inoculants and an efficient composter (Puspitaloka et al., 2022). It is necessary a composter with optimal conditions and suitable ratios for waste and inoculants to control the process and provide a high-quality mature compost (Tong et al., 2019). The metabolic analysis of the composting process can help better understand the process parameters (Ince et al., 2020).
Due to controversial data in the literature about microbial inoculation and the need to increase the efficiency of biomineralization in composting, the objective of this work was to evaluate the efficiency of a new type of composter on the composting time. In addition, it was also to evaluate the impact of the treatments on the physical-chemical and microbiological characteristics during the processing of food waste, comparing the effectiveness of two inoculants: (1) efficient microorganisms (EM); (2) commercial inoculum (CI), aimed at accelerating the degradation of organic waste and producing a high quality compost.
Materials and methods
Inoculum preparation
The experiment was carried out using two different inoculants, one non-commercial (EM—efficient microorganisms) and one commercial [CI—biological product composed of a mix of lactic acid bacteria—LAB, with species not defined by the manufacturer, used for accelerating composting of organic solid waste from agricultural and household waste (Korin, 2022). According to the manufacturer, it promotes an exponential increase in microbial activity, improving the fermentation and composting process by converting organic matter into nutrients such as acids and nitrogen compounds quickly and safely].
The non-commercial inoculum (EM) was prepared according to Xiaohou et al. (2008), with some modifications. For this, 700 g of unsalted rice cooked in distilled water were used (Figure 1). After cooking, the rice was placed on a plastic tray covered with canvas, which was placed in the native forest at the Federal University of Lavras. Litter was placed on the tray to collect the local microbiota, which took place for 15 days.
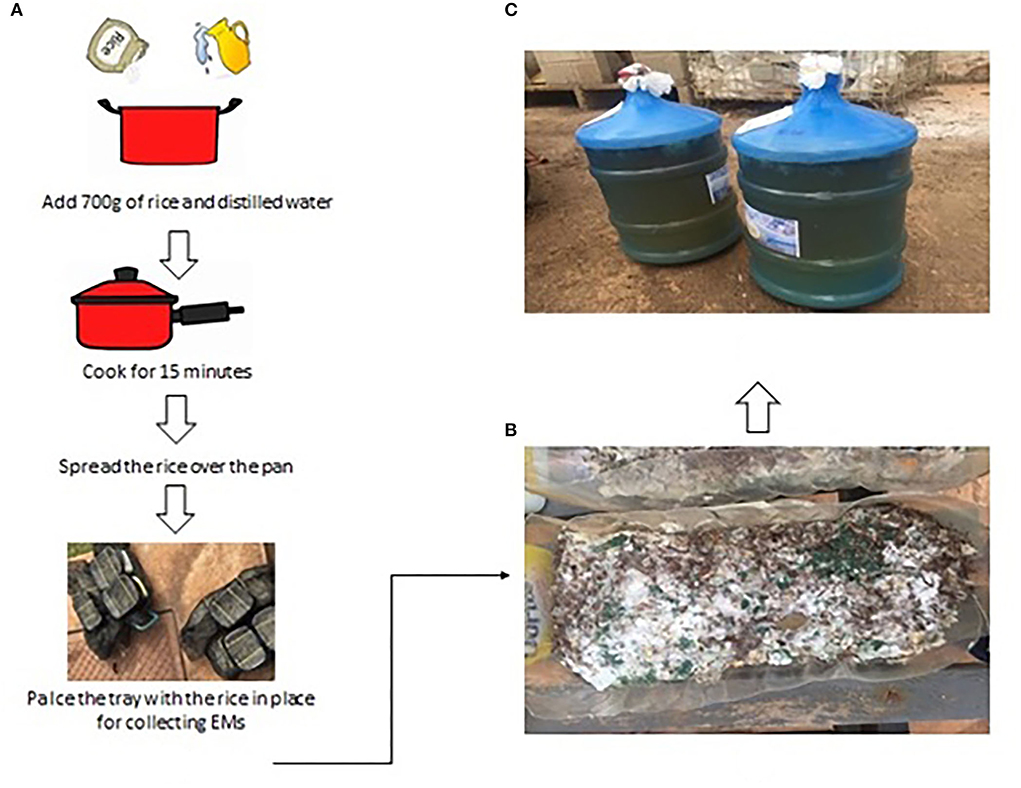
Figure 1. Process of obtaining and activating EM. (A) Procedures for capturing EM. (B) Aspect of the rice containing the EM before the activation. (C) Activation of EM in sugarcane juice (Nakagawa and Takahashi, 2015) (with adaptations).
Afterward, rice with microbial growth was deposited into a 20 L container, homogenized with 1 L of sterile sugar cane juice, and then made to a total volume of 20 L with distilled water. For 20 days, the container was stored in a cool, ventilated, and closed space.
The commercial inoculum was prepared according to the manufacturer's instructions in which the recommended amount of distilled water and sugar was added to the concentrated product, and after fermentation for 20 days at room temperature, the product was ready for use. Compost without the Addition of inoculants was used as the control treatment.
Preparation of composting
Composting was performed by mixing organic waste from the Lavras University's Restaurant (UR) and gardening waste, used to adjust the C:N ratio. The initial physicochemical characterization of both residues was carried out so that the initial C:N ratio was calculated (Table 1) and, consequently, the ideal proportion (30:1) of each residue to be composted to start the process in an ideal and satisfactory way. The control of this ratio is necessary since its excess or lack can compromise the composting process (Pisa et al., 2020).
The present work used a new structure for composting, built with concrete blocks with an air injection system that replaces the need to turn the compost pile. The NT (no-turning) composters had 1 m3 (1 m × 1 m × 1 m) of total volume. The temperature and aeration were monitored to ensure an oxygen concentration of at least 10% (Vargas-García et al., 2010). For this, temperature sensors were placed in the center of each composter (PT10 digital thermometer model MPT2, Lexitron-Guemisa, Madrid, Spain). Forced air with an approximate flow of 6.3 m3/min was injected from the bottom of the pile through a 5 cm diameter PVC pipe at the base of the compost cell. The pipe ran through the cell horizontally at 10 cm from the rear edge. The injection of air was made through 3 holes of 1 cm in diameter, distributed equidistantly along the length of the pipe (25, 50, and 75 cm), that was connected to a Ventibras® air injection pump (5cv), with axial fan. The aeration was performed every 3 days for 30 mins (Supplementary Figure S1).
The food waste was physical-chemically characterized by a C/N ratio of (30:1) and a moisture content of about 50–60%, ideal for the start of the process (Ince et al., 2020). The height of the compost piles was measured to evaluate compaction and the particle decomposition.
The organic waste from the UR were added directly to the garden waste at a ratio of 1:2.5, based on the initial characterization of waste (Table 1), mixed with the help of a tractor, resulting in a homogeneous mixture that was transferred to each composting cell. Immediately after, the starter cultures were inoculated applying the reactivated inoculum directly on the compost pile. In the initial time, 5 L of inoculum (both EM and CI) plus 15 L of water were used in each compost, except the compost with the control treatment.
Moisture content was controlled by the hand test consisting of “moistening and rubbing a little of the compost between the palms” if the compost is ready, it will not get dirty, loosen quickly, and kept between 50 and 60%, and irrigations were performed when necessary according to this standard throughout the composting process to maintain the ideal moisture content. Irrigations were performed every 3 days, with an average of 10 liters of water per compost each time.
Samples were collected in triplicate throughout the composting process (being three samples to 1–10 cm, 3 samples to 45 cm, and 3 samples to 90 cm, respectively) of the composter. Surface, interior, and bottom composting samples were homogeneously mixed for analysis and after 0, 60, and 120 days to analyze the physical, chemical, and microbiological dynamics. For microbial counting, samples were taken after 0, 5, 10, 20, 40, 60, and 120 days, period elapsed until entry into the stabilization phase. Another set of samples were taken during each change in the composting phase. These changes were detected through constant monitoring of the temperature.
Physicochemical analyses
The physicochemical analyzes were performed at 0, 60 and 120 days of composting. The moisture content was evaluated by drying at 105°C until reaching constant weight; three consecutive readings were performed to calculate the humidity. The shrinkage of the compost piles was measured using a tape measure to follow the material lodging and the particle decomposition. The fertility of the composting was determined according to the Manual of Soil Analysis (Teixeira et al., 2017). The following parameters were evaluated: C; N; C:N; H+Al (potential acidity); pH in H2O; P-rem (remaining phosphorus); P available; K; SO4; Na; Ca; Mg; S; B; Cu; Fe; Mn; Zn; organic matter (OM); organic carbon (OC); V (base saturation); m (aluminum saturation); SB (sum of bases); total CTC (cations exchange capacity) and effective CTC (Teixeira et al., 2017). The micronutrients were quantified using the Mehlich method.
Microbiological analyses
With the need for more straightforward and easily accessible analyzes, which may give us indicative of the microbial “quality” of the compound and guide us to try to understand the dynamics of microorganisms, microbial counting of microorganisms was carried out from inoculum and samples at intervals, 0, 5, 10, 20, 40, 60 and 120 days in triplicate. 25 g of each sample was added to 225 ml of sterile peptone water in a shaker at 120 revolutions per minute (rpm) for 30 min at room temperature (López-González et al., 2013). The samples were mixed in a stomacher at normal speed for 60s, and 10-fold dilutions were performed.
Seven different types of culture media were used to study the microbial communities. Nutrient Agar (NA, Merck) was used as a general medium for the viable mesophilic bacteria population (Devika et al., 2021), GYC (50 g glucose, 10 g yeast extract, 5 g CaCO3, and 20 g agar) for acetic acid bacteria (Zahoor et al., 2006). MRS (De Man Rogosa Sharpe, Merck) agar containing 0.1% cysteine—HCl was used for Lactic acid bacteria growth under anaerobic conditions (Lee and Lee, 2008). MRS plates were incubated in acrylic anaerobic jars. After spreading, the plates were incubated at 28 for 48 h. The counting and isolation of actinobacteria were performed using an Aaronson medium (2 g KNO3, 0.8 g casein, 2 g NaCl, 2 g K2HPO4, 50 mg MgSO4·7H2O, 20 mg CaCO3, 40 mg FeSO4·7H2O, 15 g agar), incubated at 45°C for 72–120 h (Silva et al., 2009). The counting and isolation of yeasts were performed using YEPG (10 g yeast extract, 10 g bacteriological peptone, 20 g glucose, and 20 g agar with pH 3.5) and incubated at 28°C for 48 h (Kurtzman et al., 2011). The filamentous fungi population was counted using PDA (200 g raw potatoes, 20 g dextrose, 20 g agar, and 1 L distilled water) and incubated at 25°C for 7 days (Colla et al., 2008).
The morphological characteristics of the colonies (cell size, cell shape, edge, color, and brightness) were recorded, and the square root of the number of colonies counted for each morphotype was purified by streaking on new agar plates (Sengun et al., 2009).
The phenotypic characterization of the bacterial colonies was performed using Gram staining, catalase and oxidase activities, and motility tests (Bergey, 1994)The pure cultures were stored in an ultra-freezer at −80°C in the same broth culture media used for plating, containing 20% glycerol (w/w). Yeast colonies were characterized for morphology and biochemical assessments as described by Kurtzman et al. (2011). Filamentous fungi were observed by morphotype analysis of the colony, especially color and appearance, using the proposals of Pitt and Hocking (1997).
Experimental design
The composting process was carried out in three treatments: (1) control (without inoculation), (2) non-commercial inoculum (EM) and (3) commercial inoculum (CI), with six replications each, totaling 18 composters. The composters were distributed in a completely randomized design (CRD) in plots subdivided in time. The data obtained during composting, including: temperature, humidity, pile height, physical, chemical and microbiological parameters, were analyzed by analysis of variance with the statistical software Sisvar and analysis of principal components with the STATISTICA 7.0 software.
Results and discussion
Evolution of temperature
Temperature is one of the main parameters used to monitor the composting process and microorganisms' activity and determine the decomposition rate of organic matter (Wang et al., 2021a). The thermal profile of the compost (Figure 2) allowed distinguishing two phases: bio-oxidative (40 days) and maturation phase (80 days), independent of the treatment, totaling 120 days of composting until maturation, a shorter period than described in the literature. Some authors describe an average of 180 days of composting (Song et al., 2009; Jurado et al., 2014; Mahapatra et al., 2022), sometimes reaching more than a year (Torrijos et al., 2021; Liu et al., 2022b). Regardless of the time, the composting process is advantageous because it happens by recycling materials that could pollute or cause public health problems. In addition, the NT compost system allows the process to be carried out without the constant turning required by traditional methods.
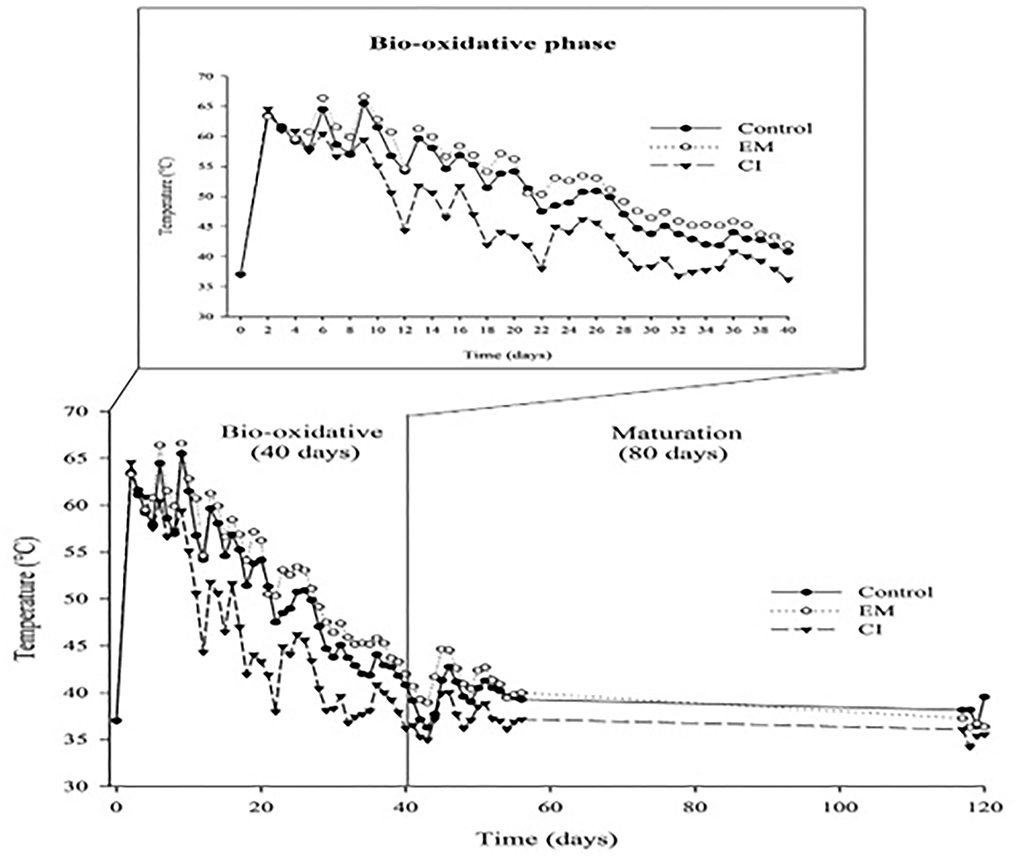
Figure 2. Thermal profile (°C) of the three treatments Control; Efficient microorganisms (EM) and commercial inoculum (CI) during the composting process.
During the bio-oxidative phase, a maximum temperature of 65°C was reached for all treatments. Higher temperatures (thermophiles) (>50°C) may be related to the gradual degradation of readily biodegradable material in the bio-oxidative phase, releasing thermal energy from the high microbial metabolism, and therefore increasing the temperature of the compost stack (Cáceres et al., 2015; Oviedo-Ocaña et al., 2019). The temperature profile showed a rapid increase, reaching 60°C on the second day, which indicated a satisfactory nutrient balance and microbial activity (Fan et al., 2018b). For at least 3 days, the thermophilic phase is important to eliminate potential pathogens and weeds and sanitize the compost (Pandey et al., 2016). In addition, high temperatures for several consecutive days indicate a good efficiency of the composting process (Oviedo-Ocaña et al., 2019).
In the present study, the three treatments followed this trend, but EM and control maintained an average of 5°C higher than CI. The end of the bio-oxidative phase exhibited fluctuations in temperature and was followed by a cooling phase, at which point the temperature was ~40°C (Figure 2), which is characteristic of a stable mesophilic phase (Huang et al., 2017; Puspitaloka et al., 2022).
The temperature profiles for the composting process with EM, CI, and control treatment showed a similar trend (Figure 2). The temperature increased dramatically within the first few days of the composting process and decreased gradually after reaching the peak around 15–18 days due to the degradation of more easily degradable materials (Wang et al., 2018). Increased temperature is caused by heat generation from microbial metabolism by the respiration and decomposition of the substrate. Therefore, microbial inoculum might significantly affect this profile if the input material does not have a sufficient microbial population for the degradation process (Fan et al., 2018a). The control and EM treatments presented ~5–7°C higher than the CI throughout the composting process, resulting in the stabilization of this compost several days in advance.
Physical and chemical dynamics
The physicochemical changes during the composting process are shown in Table 2 and Figure 3.
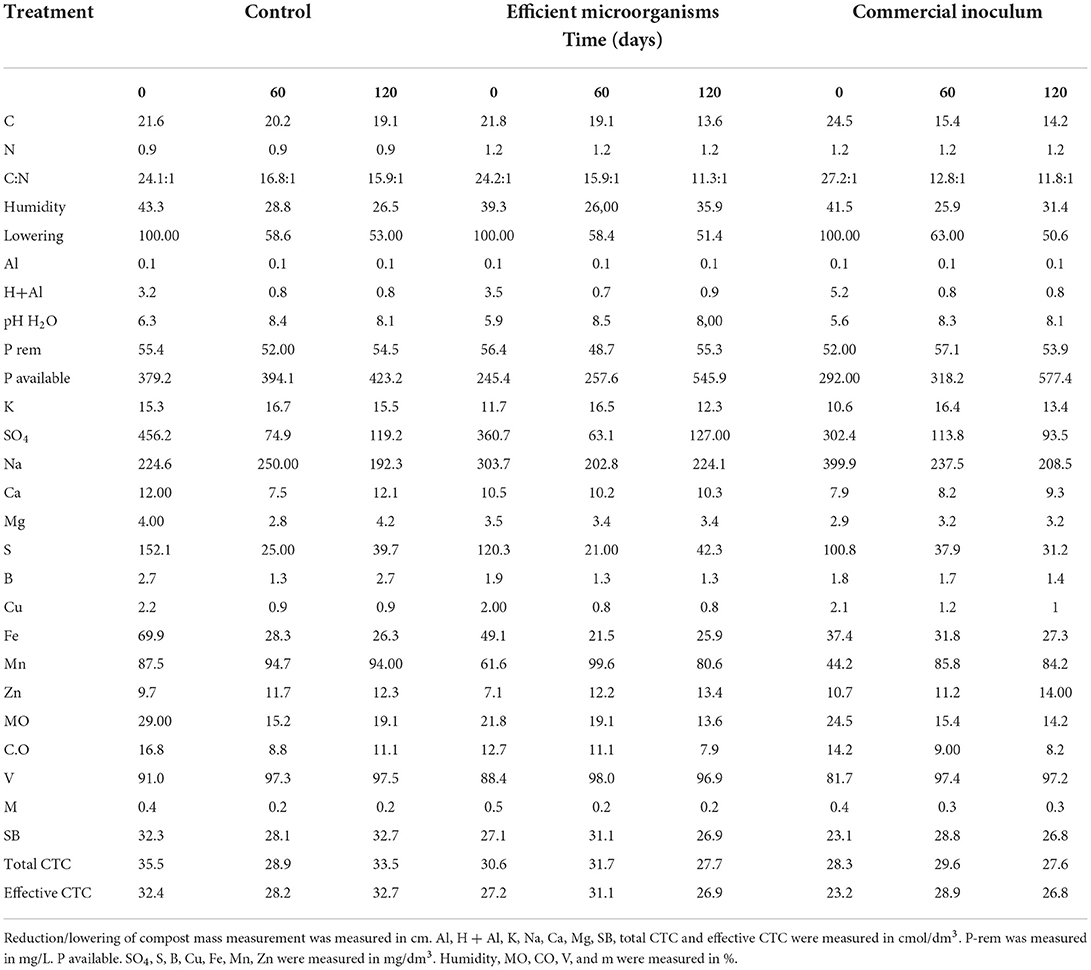
Table 2. Physical and chemical parameters during composting for control, efficient microorganisms, and commercial inoculum treatments.
Aeration is one of the key parameters in controlling the activities of the waste being composted, as it influences the temperature, moisture, and O2 supply to the microorganisms (Wu et al., 2019). In the composting process, the predominant microorganisms are aerobic. Therefore, they need oxygen to survive and maintain their metabolism (Zeng et al., 2016). The oxygen present in composting also removes excess moisture from the composting mass, avoiding the need for higher temperatures (Zhang et al., 2021).
There was a lowering tendency for moisture to decrease over time and between treatments. The variable moisture presented a fall over time but not a constant. The process of assimilation of nutrients by microorganisms occurs through their cell walls so that the metabolic activities of organic matter degradation can occur. Therefore, the humidity of the medium must be adequate (Alege et al., 2022). Even with periodic irrigation, the humidity did not always remain in the ideal range, between 50 and 60%, with values around 40% or bellow being more frequently observed. According to Ho et al. (2022), waste mixtures with low moisture content (below 50%) can inhibit microbial activity, as water is essential for metabolism in any other biological process. However, the results showed that the periodic irrigation scheme and the observed humidity range did not restrict the biological activities.
The reduction in the height of the compost pile can be indicative of process evolution due to the decomposition of the parent material into smaller particles, which implies a reduction in reducing the porosity of the pile.
One goal of the present study, which used garden waste and restaurant waste, was to assess the composting substrates, including adjusting the C/N ratio and any increases in the availability of nutrients. Oviedo-Ocaña et al. (2019) observed that carbon and nitrogen used by microorganisms for energy and growth resulted in changes in the C/N ratio during the composting process. During the composting process, organic matter degradation resulted in carbon reduction, which decreased at the end of composting (Table 2).
The C:N ratio for EM and CI compost samples decreased significantly with time and stabilized on 60 days in EM treatment (12.8) and 120 days in CI treatment (15.9). However, both samples showed C:N below 12 (11.8 and 11.3, respectively), which indicated direct mineralization of organic nitrogen (Margaritis et al., 2018). The control showed C:N 15.9 at the end of the time, meaning that composting without inoculum could not be finished at 120 days, as the proper C/N ratio at the end of the process is between 10 and 15 (Wang et al., 2022). C: N ratio, followed a trend, decreasing as the degradation process proceeded (especially in EM and CI), and C was computed mainly as carbon dioxide (Hubbe et al., 2010; Wang et al., 2022).
The variation of total acidity and pH value results from organic acid production by microbial metabolism (Wei et al., 2018). The pH can vary in the composting process, which can determine the predominance of a certain group of microorganisms in the process (Puspitaloka et al., 2022). The increase in pH value and the decrease in the exchangeable acidity (H+Al) (Table 2) are characteristics of mature compost and result in increased degradation of organic acids and amino acids, which provides additional nutrients (Makan and Mountadar, 2012; López-Cano et al., 2016). The pH value, in all treatments, in the first weeks was acidic (5.6–6.3) due to the release of organic acids from food waste (Table 2) (Oviedo-Ocaña et al., 2019). It gradually turned from acidic to a range of neutral and weakly alkaline and turned basic (8.0–8.1) due to the releases of ammonia, complete mineralization of the intermediate metabolites, and the conversion of organic acid into CO2 by microbial activity (Iqbal et al., 2015; Oviedo-Ocaña et al., 2019) (Table 2), indicating organic matter's stability (Ince et al., 2020).
The increase in P availability during composting may be related to organic matter degradation, increase in pH, decrease in Fe content in all treatments (Table 2), and the phosphatase activity, which is produced by phosphate-solubilizing microorganisms (Hu et al., 2018).
In general, the variables sodium, total acidity, base saturation index, copper, manganese, and ion exchange capacity were the variables that most contributed to explaining the variability of the data. The organic matter contents presented initial values of 29.0, 21.8, and 24.5% and final values of 19.1, 13.6, and 14.2%, showing decrease of 9.9, 8.2, and 10.3% in control, MS, and IC treatments, respectively, throughout the process. Concerning the macronutrients, available phosphorus levels increased during the composting process, reaching 423.3, 545.9, and 577.4 mg/dm3 in control, EM, and CI treatments, respectively. The initial values of K in control, EM, and CI treatments were 15.3, 11.7, and 10.6 cmol/dm3, while the final values were slightly higher, with 15.5, 12.3, and 13.4 cmol/dm3. The Ca values remained constant, with an initial mean of 12.1 cmol/dm3 and a final average of 10.5 cmol/dm3. The Mg values increased with a change from 3.4 to 3.6 cmol/dm3. The opposite occurred with the values of S and Na, which decreased in both treatments throughout the process.
The higher the decomposition rate, the higher the mineralization rate of some nutrients at the end of composting, and the higher the quality of the biofertilizer (Zhou et al., 2018). Only Mn and Zn showed a significant increase in micronutrients during composting.
The nutrients are not volatile. Therefore, the increase in P and K content was mainly due to the loss of compost mass due to the biodegradation of organic matter. However, the content of these nutrients increases the metabolic activity of the microorganisms during composting and thus increases the organic waste decomposition process (Varma et al., 2017; Ince et al., 2020). These results showed that these elements' increase in both treatments indicated a satisfactory decomposition rate despite the decrease in the contents of some nutrients, which were not significant.
The principal component analysis (PCA) showed the physical and chemical parameters obtained during composting, for better elucidation of these (Ge et al., 2020; Wani, 2021). In this analysis, the principal components (PC) 1 and 2 explained 66.19% of the data variance (Figure 3). Four groups (Figure 3) were observed. The group I was formed by treatment CI at all times (CI-T0; CI-T60; CI-T120); the group II was formed by control treatment in 0 and 60 days (C-T0 and C-T60), and the treatments EM-T120 and C-T120 formed the Group III and the treatment EM-T60 and EM-T120 formed the group IV.
There was a high relation between PC1 and the physicochemical variables. That high relation shows the influence of the treatments with EM and CI. Only variables Cu, pH, and degradation of organic material showed low relation with the PC1. However, with PC2, the influence of CI treatment showed great influence on Ca, Mg, lowering, Na, P-rem, B, S. C, pH, and MO available, already EM showed influence for Cu and H + Al values (Figure 3).
These results showed the evident influence the inoculations in the dynamics of the physicochemical parameters when compared with the control treatment during the composting, with better results obtained with CI than with the inoculation of EM (Figure 3).
Microbial communities
Compost maturity is reached when microbiological decomposition is complete and accompanied by the components' mineralization, making them available to the plants and improving the soil's physical, chemical, and biological properties (Gou et al., 2017).
The yeast population in the EM inoculum was 5.5-log CFU g−1, lower than the CI inoculum yeast count of 8.0-log CFU g−1. The two inoculums to be added to the compost piles did not show any fungal growth (Figure 4). However, the aerobic mesophilic and thermophilic bacterial population remained stable during the composting process, with ~8.0 log CFU g−1 of compost, even in the thermophilic phase (T5 and T10) in both treatments (Figures 4A–C), which can be justified due to heat resistance and spore formation (Wang et al., 2022).
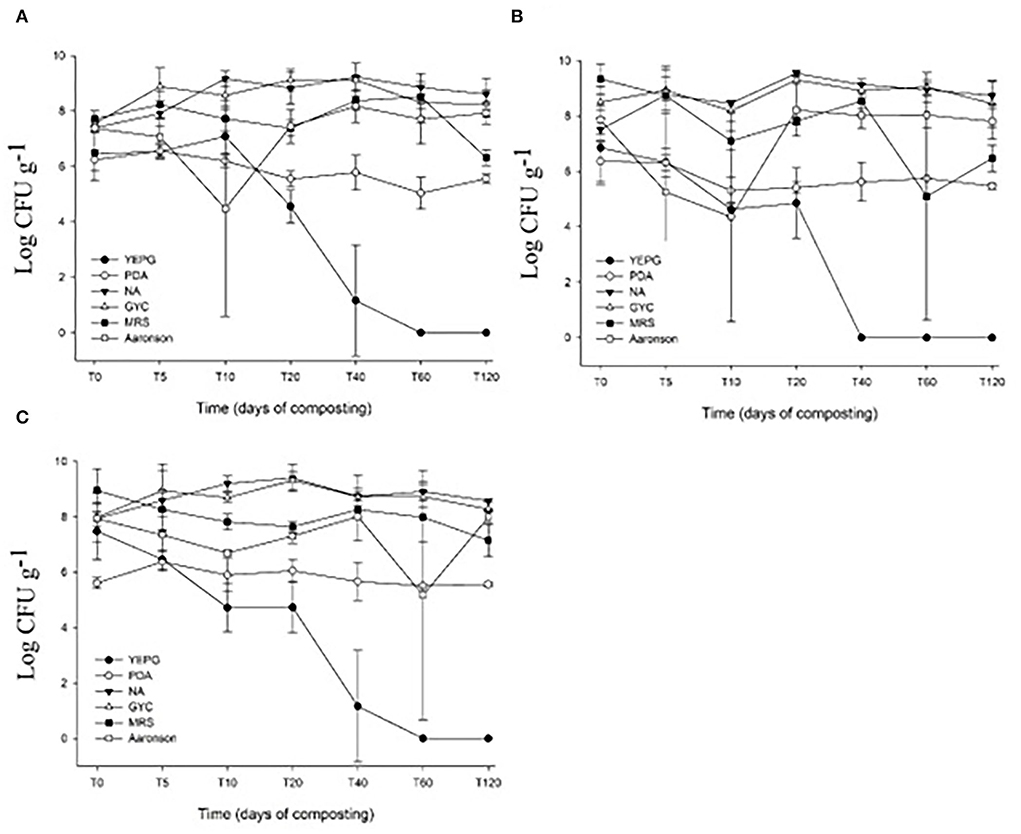
Figure 4. Variation in the total count of microorganisms (log UFC g−1 of compost) during composting. (A) Treatments with efficient microorganisms EM. (B) Control treatment. (C) Treatment with commercial inoculum. YEPG, yeast; PDA, filamentous fungi; NA, total mesophilic bacteria; MRS, lactic bacteria; GYC, bacteria of acetic acid and Aaronson–Actinobacteria. *CFU, colony form units.
The population of actinobacteria remained constant only in the CI treatment (Figure 4C), whereas in control (Figure 4B) and EM (Figure 4A) treatments, there was a decrease in the population in the thermophilic phase (T5 and T10). The yeast population varied throughout composting time, showing similar behavior in all treatments (Figures 4A–C). The yeast counting was lower than bacteria, and actinobacteria remained stable in the first 20 days of composting but showed no growth after 40 days. However, the population of filamentous fungi from the compost pile remained stable over time, in all treatments (Figures 4A–C), with ~5.0 log CFU g−1 of the compound. Even at the beginning of the bio-oxidative phase presenting high temperatures (>50°C), thermotolerant mesophilic microorganisms can withstand (Liu et al., 2018; Wang et al., 2022).
The population of prokaryotes (bacteria and actinobacteria) was higher than that of eukaryotes (fungi and yeasts) in the treatments EM and CI, as also observed by López-González et al. (2013). Even when there is no change in the total plate count of microorganisms, there may be a reduction in diversity indices, favoring certain microbial groups (Huang et al., 2019).
Microbial communities were similar throughout the process in both treatments, showing that the EM and CI inoculation did not influence the dynamics of microorganisms during the composting process. In composts without microbial starter, several changes naturally occur in the physical and chemical structure because the epiphytic microorganisms present are capable of biological activity and increasing the temperature (55–70°C), which provides the dominance of thermophilic microorganisms with high degradability of organic matter (Xu et al., 2022). Thus, the endogenous populations of microorganisms adapt to environmental variations provided by the composting process and play an essential role in the degradation of organic matter, mineralization of nutrients, control of pathogens, and stabilization of the compost (Khalil et al., 2011; Huang et al., 2019).
Many microbial inoculums help in the composting process by controlling the temperature and physical-chemical aspects. For example, the inoculation of the microorganisms contributes to temperature stability, chemical composition, and enzyme production during the composting process (Awasthi et al., 2020), also important in the degradation of organic matter e.g., in kitchen waste composting. Yang et al. (2019), as well as Wang et al. (2022) in studies with food waste composting, reported that the use of inoculants accelerates the composting process. However, studies on the suitability of different inoculants are still inconclusive, likely due to the complexity of the composting process and the nature of organic wastes (Cerda et al., 2018). Studies by Wang et al. (2017) have demonstrated that inoculation using mature compost may be more indicative than the inoculation of starter cultures in the composting process, as there was no significant effect on the composting time and quality of the final compost. Although the dynamics of microorganisms did not show great differences, an additional study with the identification of these microorganisms should be carried out to show the species present in each treatment and the control.
Conclusions
The NT composter, in addition to reducing the laboriousness of the process by eliminating the need to turn the compost pile, also contributed to the maintenance of adequate oxygen content and temperature control inside the cells, preserving the metabolic activity of microorganisms, improving physicochemical characteristics and keeping the composting time within the acceptable range. The analysis of organic matter transformation during the composting of organic wastes showed complex physical, chemical, and microbiological interactions. Although the dynamics of microorganisms did not show a significant difference throughout the composting process, between the treatments and the control, the EM and CI treatments presented advantages in terms of the physical-chemical aspects and the quality of the final product compared to the control treatment. During the inoculated composting processes, there was greater mineralization of P-available and micronutrients such as Mn and Zn, enabling the compost to become a biofertilizer. In general, the NT composter and the addition of inoculants contributed significantly to the efficiency of the process of composting organic waste.
Data availability statement
The original contributions presented in the study are included in the article/Supplementary material, further inquiries can be directed to the corresponding author.
Author contributions
SG, LA, CC, VB, GF, RS, MP, FR, ER, RC, and JD designed the study and interpreted the results. SG, LA, CC, GF, and RC conducted the experiments and collected test data. VB, RS, MP, FR, ER, RC, and JD revised the final manuscript and managed and financed the experiment. All authors have read and approved the manuscript.
Funding
The authors thank Capes, CNPq, and FAPEMIG for financial support, the Federal University of Lavras (UFLA) for the structure provided to accomplish the experiments, and the technical support of the teachers.
Conflict of interest
The authors declare that the research was conducted in the absence of any commercial or financial relationships that could be construed as a potential conflict of interest.
Publisher's note
All claims expressed in this article are solely those of the authors and do not necessarily represent those of their affiliated organizations, or those of the publisher, the editors and the reviewers. Any product that may be evaluated in this article, or claim that may be made by its manufacturer, is not guaranteed or endorsed by the publisher.
Supplementary material
The Supplementary Material for this article can be found online at: https://www.frontiersin.org/articles/10.3389/fsufs.2022.960196/full#supplementary-material
References
Abdolali, A., Guo, W. S., Ngo, H. H., Chen, S. S., Nguyen, N. C., and Tung, K. L. (2014). Typical lignocellulosic wastes and by-products for biosorption process in water and wastewater treatment: a critical review. Bioresour. Technol. 160, 57–66. doi: 10.1016/j.biortech.2013.12.037
Alege, F. P., Tao, H., Miito, G. J., Devetter, L. W., and Ndegwa, P. M. (2022). Influence of moisture content on recovery and durability of dairy manure compost pellets. Bioresour. Technol. Rep. 18, 101076. doi: 10.1016/j.biteb.2022.101076
Antunes, L. E. C., Reisser Junior, C., and Schwengber, J. E. (2016). Morangueiro. Brasília, DF: Embrapa.
Awasthi, A. K., Cheela, V. R. S., D'adamo, I., Iacovidou, E., Islam, M. R., Johnson, M., et al. (2021). Zero waste approach towards a sustainable waste management. Resour. Environ. Sustain. 3, 100014. doi: 10.1016/j.resenv.2021.100014
Awasthi, S. K., Sarsaiya, S., Awasthi, M. K., Liu, T., Zhao, J., Kumar, S., et al. (2020). Changes in global trends in food waste composting: research challenges and opportunities. Bioresour. Technol. 299, 122555. doi: 10.1016/j.biortech.2019.122555
Bergey, D. H. (1994). Bergey's Manual of Determinative Bacteriology. Philadelphia, PA: Lippincott Williams and Wilkins.
Bohacz, J. (2017). Lignocellulose-degrading enzymes, free-radical transformations during composting of lignocellulosic waste and biothermal phases in small-scale reactors. Sci. Total Environ. 580, 744–754. doi: 10.1016/j.scitotenv.2016.12.021
Bruni, C., Akyol, Ç., Cipolletta, G., Eusebi, A. L., Caniani, D., Masi, S., et al. (2020). Decentralized community composting: past, present and future aspects of Italy. Sustainability 12, 3319. doi: 10.3390/su12083319
Cáceres, R., Coromina, N., Malińska, K., and Marfà, O. (2015). Evolution of process control parameters during extended co-composting of green waste and solid fraction of cattle slurry to obtain growing media. Bioresour. Technol. 179, 398–406. doi: 10.1016/j.biortech.2014.12.051
Cerda, A., Artola, A., Font, X., Barrena, R., Gea, T., and Sánchez, A. (2018). Composting of food wastes: status and challenges. Bioresour. Technol. 248, 57–67. doi: 10.1016/j.biortech.2017.06.133
Colla, L., Primaz, A., Lima, M., Bertolin, T., and Costa, J. A. (2008). Isolamento e seleção de fungos para biorremediação a partir de solo contaminado com herbicidas triazínicos. Ciencia E Agrotecnol. 32. doi: 10.1590/S1413-70542008000300016
Deus, R. M., Battistelle, R. G., and Silva, G.H.R. (2017). Scenario evaluation for the management of household solid waste in small Brazilian municipalities. Clean Technol. Environ. Policy 19, 205–214. doi: 10.1007/s10098-016-1205-0
Devika, C., Singhalage, D., and Seneviratne, G. (2021). Modification of nutrient agar medium to culture yet-unculturable bacteria living in unsanitary landfills. Ceylon J. Sci. 50, 505. doi: 10.4038/cjs.v50i4.7949
Fan, Y. V., Klemeš, J. J., Lee, C. T., and Ho, C. S. (2018a). Efficiency of microbial inoculation for a cleaner composting technology. Clean Technol. Environ. Policy 20, 517–527. doi: 10.1007/s10098-017-1439-5
Fan, Y. V., Lee, C. T., Klemeš, J. J., Chua, L. S., Sarmidi, M. R., and Leow, C. W. (2018b). Evaluation of effective microorganisms on home scale organic waste composting. J. Environ. Manage. 216, 41–48. doi: 10.1016/j.jenvman.2017.04.019
Ge, M., Zhou, H., Shen, Y., Meng, H., Li, R., Zhou, J., et al. (2020). Effect of aeration rates on enzymatic activity and bacterial community succession during cattle manure composting. Bioresour. Technol. 304, 122928. doi: 10.1016/j.biortech.2020.122928
Gou, C., Wang, Y., Zhang, X., Lou, Y., and Gao, Y. (2017). Inoculation with a psychrotrophic-thermophilic complex microbial agent accelerates onset and promotes maturity of dairy manure-rice straw composting under cold climate conditions. Bioresour. Technol. 243, 339–346. doi: 10.1016/j.biortech.2017.06.097
Ho, T. T. K., Tra, V. T., Le, T. H., Nguyen, N.-K.-Q., Tran, C.-S., Nguyen, P.-T., et al. (2022). Compost to improve sustainable soil cultivation and crop productivity. Case Stud. Chem. Environ. Eng. 6, 100211. doi: 10.1016/j.cscee.2022.100211
Hu, Y., Xia, Y., Sun, Q., Liu, K., Chen, X., Ge, T., et al. (2018). Effects of long-term fertilization on phoD-harboring bacterial community in Karst soils. Sci. Total Environ. 628–629, 53–63. doi: 10.1016/j.scitotenv.2018.01.314
Huang, Y., Li, D., Wang, L., Yong, C., Sun, E., Jin, H., et al. (2019). Decreased enzyme activities, ammonification rate and ammonifiers contribute to higher nitrogen retention in hyperthermophilic pretreatment composting. Bioresour. Technol. 272, 521–528. doi: 10.1016/j.biortech.2018.10.070
Huang, Y.-L., Sun, Z.-Y., Zhong, X.-Z., Wang, T.-T., Tan, L., Tang, Y.-Q., et al. (2017). Aerobic composting of digested residue eluted from dry methane fermentation to develop a zero-emission process. Waste Manage. 61, 206–212. doi: 10.1016/j.wasman.2017.01.007
Hubbe, M. A., Nazhad, M., and Sánchez, C. (2010). Composting as a way to convert cellulosic biomass and organic waste into high-value soil amendments: a review. BioResources 5, 2808–2854. doi: 10.15376/biores.5.4.2808-2854
Ince, O., Ozbayram, E. G., Akyol, Ç., Erdem, E. I., Gunel, G., and Ince, B. (2020). Bacterial succession in the thermophilic phase of composting of anaerobic digestates. Waste Biomass Valor. 11, 841–849. doi: 10.1007/s12649-018-0531-3
Iqbal, H., Garcia-Perez, M., and Flury, M. (2015). Effect of biochar on leaching of organic carbon, nitrogen, and phosphorus from compost in bioretention systems. Sci. Total Environ. 521–522, 37–45. doi: 10.1016/j.scitotenv.2015.03.060
Jurado, M., López, M. J., Suárez-Estrella, F., Vargas-García, M. C., López-González, J. A., and Moreno, J. (2014). Exploiting composting biodiversity: study of the persistent and biotechnologically relevant microorganisms from lignocellulose-based composting. Bioresour. Technol. 162, 283–293. doi: 10.1016/j.biortech.2014.03.145
Khalil, A. I., Hassouna, M. S., El-Ashqar, H. M. A., and Fawzi, M. (2011). Changes in physical, chemical and microbial parameters during the composting of municipal sewage sludge. World J. Microbiol. Biotechnol. 27, 2359–2369. doi: 10.1007/s11274-011-0704-8
Kinet, R., Destain, J., Hiligsmann, S., Thonart, P., Delhalle, L., Taminiau, B., et al. (2015). Thermophilic and cellulolytic consortium isolated from composting plants improves anaerobic digestion of cellulosic biomass: toward a microbial resource management approach. Bioresour. Technol. 189, 138–144. doi: 10.1016/j.biortech.2015.04.010
Korin (2022). Korin Agriculture and Environment. Available online at: https://korinagricultura.com.br/pt/embiotic-compostagem/ (accessed March 30, 2022).
Külcü, R., and Yaldiz, O. (2014). The composting of agricultural wastes and the new parameter for the assessment of the process. Ecol. Eng. 69, 220–225. doi: 10.1016/j.ecoleng.2014.03.097
Kurtzman, C. P., Fell, J. W., and Boekhout, T. (2011). The Yeasts: A Taxonomic Study. Amsterdam: Elsevier.
Lee, H. M., and Lee, Y. (2008). A differential medium for lactic acid-producing bacteria in a mixed culture. Lett. Appl. Microbiol. 46, 676–681. doi: 10.1111/j.1472-765X.2008.02371.x
Li, S., Li, D., Li, J., Li, G., and Zhang, B. (2017). Evaluation of humic substances during co-composting of sewage sludge and corn stalk under different aeration rates. Bioresour. Technol. 245, 1299–1302. doi: 10.1016/j.biortech.2017.08.177
Liu, L., Wang, S., Guo, X., Zhao, T., and Zhang, B. (2018). Succession and diversity of microorganisms and their association with physicochemical properties during green waste thermophilic composting. Waste Manag. 73, 101–112. doi: 10.1016/j.wasman.2017.12.026
Liu, Q., He, X., Luo, G., Wang, K., and Li, D. (2022a). Deciphering the dominant components and functions of bacterial communities for lignocellulose degradation at the composting thermophilic phase. Bioresour. Technol. 348, 126808. doi: 10.1016/j.biortech.2022.126808
Liu, Y., Ptacek, C. J., Beauchemin, S., Mackinnon, T., and Blowes, D. W. (2022b). Effect of composting and amendment with biochar and woodchips on the fate and leachability of pharmaceuticals in biosolids destined for land application. Sci. Total Environ. 810, 151193. doi: 10.1016/j.scitotenv.2021.151193
Liu, Z., Wang, X., Wang, F., Bai, Z., Chadwick, D., Misselbrook, T., et al. (2020). The progress of composting technologies from static heap to intelligent reactor: benefits and limitations. J. Clean. Prod. 270, 122328. doi: 10.1016/j.jclepro.2020.122328
López-Cano, I., Roig, A., Cayuela, M. L., Alburquerque, J. A., and Sánchez-Monedero, M. A. (2016). Biochar improves N cycling during composting of olive mill wastes and sheep manure. Waste Manag. 49, 553–559. doi: 10.1016/j.wasman.2015.12.031
López-González, J. A., López, M. J., Vargas-García, M. C., Suárez-Estrella, F., Jurado, M., and Moreno, J. (2013). Tracking organic matter and microbiota dynamics during the stages of lignocellulosic waste composting. Bioresour. Technol. 146, 574–584. doi: 10.1016/j.biortech.2013.07.122
Mahapatra, S., Ali, M. H., and Samal, K. (2022). Assessment of compost maturity-stability indices and recent development of composting bin. Energy Nexus 6, 100062. doi: 10.1016/j.nexus.2022.100062
Makan, A., and Mountadar, M. (2012). Effect of C/N ratio on the in-vessel composting under air pressure of organic fraction of municipal solid waste in Morocco. J. Mater. Cycles Waste Manag. 14, 241–249. doi: 10.1007/s10163-012-0062-0
Margaritis, M., Psarras, K., Panaretou, V., Thanos, A. G., Malamis, D., and Sotiropoulos, A. (2018). Improvement of home composting process of food waste using different minerals. Waste Manag. 73, 87–100. doi: 10.1016/j.wasman.2017.12.009
Milinković, M., Lalević, B., Jovičić-Petrović, J., Golubović-Curguz, V., Kljujev, I., and Raičević, V. (2019). Biopotential of compost and compost products derived from horticultural waste—effect on plant growth and plant pathogens' suppression. Process Saf. Environ. Protect. 121, 299–306. doi: 10.1016/j.psep.2018.09.024
Nakagawa, T., and Takahashi, R. (2015). Nitrosomonas stercoris sp. nov., a chemoautotrophic ammonia-oxidizing bacterium tolerant of high ammonium isolated from composted cattle manure. Microbes Environ. 30, 221–227. doi: 10.1264/jsme2.ME15072
Nakasaki, K., Araya, S., and Mimoto, H. (2013). Inoculation of Pichia kudriavzevii RB1 degrades the organic acids present in raw compost material and accelerates composting. Bioresour. Technol. 144, 521–528. doi: 10.1016/j.biortech.2013.07.005
Obeng, A. S., Byalebeka, J. B., Flarian, M. M., Ssekandi, J., and Noel, G. L. (2021). Effect of co-applied corncob biochar with farmyard manure and NPK fertilizer on tropical soil. Res. Environ. Sustain. 5, 100034. doi: 10.1016/j.resenv.2021.100034
Onwosi, C. O., Igbokwe, V. C., Odimba, J. N., Eke, I. E., Nwankwoala, M. O., Iroh, I. N., et al. (2017). Composting technology in waste stabilization: on the methods, challenges and future prospects. J. Environ. Manage. 190, 140–157. doi: 10.1016/j.jenvman.2016.12.051
Oviedo-Ocaña, E. R., Dominguez, I., Komilis, D., and Sánchez, A. (2019). Co-composting of green waste mixed with unprocessed and processed food waste: influence on the composting process and product quality. Waste Biomass Valor. 10, 63–74. doi: 10.1007/s12649-017-0047-2
Pan, I., and Sen, S. (2013). Microbial and physico-chemical analysis of composting process of wheat straw. Indian J. Biotechnol. 12, 120–128.
Pandey, P. K., Cao, W., Biswas, S., and Vaddella, V. (2016). A new closed loop heating system for composting of green and food wastes. J. Clean. Prod. 133, 1252–1259. doi: 10.1016/j.jclepro.2016.05.114
Pisa, C., Wuta, M., and Muchaonyerwa, P. (2020). Effects of incorporation of vermiculite on carbon and nitrogen retention and concentration of other nutrients during composting of cattle manure. Bioresour. Technol. Rep. 9, 100383. doi: 10.1016/j.biteb.2020.100383
Pitt, J. I., and Hocking, A. D. (1997). “Methods for isolation, enumeration and identification,” in Fungi and Food Spoilage (Boston, MA: Springer US), 21–57. doi: 10.1007/978-1-4615-6391-4_4
Puspitaloka, H., Mimoto, H., Tran, Q. N. M., Koyama, M., and Nakasaki, K. (2022). Effect of aeration methods on the organic matter degradation, microbial community and their catabolic function during composting. Waste Biomass Valor. 13, 1195–1205. doi: 10.1007/s12649-021-01560-5
Sarkar, S., Banerjee, R., Chanda, S., Das, P., Ganguly, S., and Pal, S. (2010). Effectiveness of inoculation with isolated Geobacillus strains in the thermophilic stage of vegetable waste composting. Bioresour. Technol. 101, 2892–2895. doi: 10.1016/j.biortech.2009.11.095
Sengun, I., Nielsen, D., Karapinar, M., and Jakobsen, M. (2009). Identification of lactic acid bacteria isolated from Tarhana, a traditional Turkish fermented food. Int. J. Food Microbiol. 135, 105–111. doi: 10.1016/j.ijfoodmicro.2009.07.033
Sharma, D., Yadav, K. D., and Kumar, S. (2018). Biotransformation of flower waste composting: Optimization of waste combinations using response surface methodology. Bioresour. Technol. 270, 198–207. doi: 10.1016/j.biortech.2018.09.036
Silva, C. F., Azevedo, R. S., Braga, C., Silva, R. D., Dias, E. S., and Schwan, R. F (2009). Microbial diversity in a bagasse-based compost prepared for the production of Agaricus brasiliensis. Braz. J. Microbiol. 40, 590–600 doi: 10.1590/S1517-83822009000300023
Song, J. H., Murphy, R. J., Narayan, R., and Davies, G. B. H. (2009). Biodegradable and compostable alternatives to conventional plastics. Philos. Trans. R. Soc. B Biol. Sci. 364, 2127–2139. doi: 10.1098/rstb.2008.0289
Soto-Paz, J., Oviedo-Ocaña, E. R., Manyoma-Velásquez, P. C., Torres-Lozada, P., and Gea, T. (2019). Evaluation of mixing ratio and frequency of turning in the co-composting of biowaste with sugarcane filter cake and star grass. Waste Manage. 96, 86–95. doi: 10.1016/j.wasman.2019.07.015
Teixeira, P. C., Donagemma, G. K., Fontana, A., and Teixeira, W. G. (2017). “Manual de Métodos de Análise de Solo”, ed. E. B. D. P. Agropecuária. 3rd Edition ed. (Brasília, DF: Embrapa Solos, Ministério da Agricultura, Pecuária e Abastecimento.).
Thi, N. B. D., Kumar, G., and Lin, C.-Y. (2015). An overview of food waste management in developing countries: Current status and future perspective. J. Environ. Manage. 157, 220–229. doi: 10.1016/j.jenvman.2015.04.022
Tong, B., Wang, X., Wang, S., Ma, L., and Ma, W. (2019). Transformation of nitrogen and carbon during composting of manure litter with different methods. Bioresour. Technol. 293, 122046. doi: 10.1016/j.biortech.2019.122046
Torrijos, V., Calvo Dopico, D., and Soto, M. (2021). Integration of food waste composting and vegetable gardens in a university campus. J. Clean. Prod. 315, 128175. doi: 10.1016/j.jclepro.2021.128175
Vargas-García, M. C., Suárez-Estrella, F., López, M. J., and Moreno, J. (2010). Microbial population dynamics and enzyme activities in composting processes with different starting materials. Waste Manage. 30, 771–778. doi: 10.1016/j.wasman.2009.12.019
Varma, V. S., Nashine, S., Sastri, C. V., and Kalamdhad, A. S. (2017). Influence of carbide sludge on microbial diversity and degradation of lignocellulose during in-vessel composting of agricultural waste. Ecol. Eng. 101, 155–161. doi: 10.1016/j.ecoleng.2017.01.022
Wang, J., Guleria, S., Koffas, M. G., and Yan, Y. (2016). Microbial production of value-added nutraceuticals. Curr. Opin. Biotechnol. 37, 97–104. doi: 10.1016/j.copbio.2015.11.003
Wang, K., Wu, Y., Li, W., Wu, C., and Chen, Z. (2018). Insight into effects of mature compost recycling on N2O emission and denitrification genes in sludge composting. Bioresour. Technol. 251, 320–326. doi: 10.1016/j.biortech.2017.12.077
Wang, X., Pan, S., Zhang, Z., Lin, X., Zhang, Y., and Chen, S. (2017). Effects of the feeding ratio of food waste on fed-batch aerobic composting and its microbial community. Bioresour. Technol. 224, 397–404. doi: 10.1016/j.biortech.2016.11.076
Wang, Y., Tang, Y., Li, M., and Yuan, Z. (2021a). Aeration rate improves the compost quality of food waste and promotes the decomposition of toxic materials in leachate by changing the bacterial community. Bioresour. Technol. 340, 125716. doi: 10.1016/j.biortech.2021.125716
Wang, Y., Tang, Y., and Yuan, Z. (2022). Improving food waste composting efficiency with mature compost addition. Bioresour. Technol. 349, 126830. doi: 10.1016/j.biortech.2022.126830
Wang, Y., Yuan, Z., and Tang, Y. (2021b). Enhancing food security and environmental sustainability: a critical review of food loss and waste management. Resour. Environ. Sustain. 4, 100023. doi: 10.1016/j.resenv.2021.100023
Wani, S. A. (2021). Assessment of changes in soil organic carbon fractions and enzyme activities under apple growing ecosystems in temperate North-Western Himalayas. Resour. Environ. Sustain. 6, 100036. doi: 10.1016/j.resenv.2021.100036
Wei, Y., Zhao, Y., Shi, M., Cao, Z., Lu, Q., Yang, T., et al. (2018). Effect of organic acids production and bacterial community on the possible mechanism of phosphorus solubilization during composting with enriched phosphate-solubilizing bacteria inoculation. Bioresour. Technol. 247, 190–199. doi: 10.1016/j.biortech.2017.09.092
Wu, J., Zhao, Y., Yu, H., Wei, D., Yang, T., Wei, Z., et al. (2019). Effects of aeration rates on the structural changes in humic substance during co-composting of digestates and chicken manure. Sci. Total Environ. 658, 510–520. doi: 10.1016/j.scitotenv.2018.12.198
Xiaohou, S., Min, T., Ping, J., and Weiling, C. (2008). Effect of EM Bokashi application on control of secondary soil salinization. Water Sci. Eng. 1, 99–106.
Xu, Y., Bi, Z., Zhang, Y., Wu, H., Zhou, L., and Zhang, H. (2022). Impact of wine grape pomace on humification performance and microbial dynamics during pig manure composting. Bioresour. Technol. 358, 127380. doi: 10.1016/j.biortech.2022.127380
Yang, F., Li, Y., Han, Y., Qian, W., Li, G., and Luo, W. (2019). Performance of mature compost to control gaseous emissions in kitchen waste composting. Sci. Total Environ. 657, 262–269. doi: 10.1016/j.scitotenv.2018.12.030
Zahoor, T., Siddique, F., and Farooq, U. (2006). Isolation and characterization of vinegar culture (Acetobacter aceti) from indigenous sources. Br. Food J. 108, 429–439. doi: 10.1108/00070700610668405
Zeng, J., Shen, X., Han, L., and Huang, G. (2016). Dynamics of oxygen supply and consumption during mainstream large-scale composting in China. Bioresour. Technol. 220, 104–109. doi: 10.1016/j.biortech.2016.08.070
Zhang, L., and Sun, X. (2014). Changes in physical, chemical, and microbiological properties during the two-stage co-composting of green waste with spent mushroom compost and biochar. Bioresour. Technol. 171, 274–284. doi: 10.1016/j.biortech.2014.08.079
Zhang, L., and Sun, X. (2018). Effects of bean dregs and crab shell powder additives on the composting of green waste. Bioresour. Technol. 260, 283–293. doi: 10.1016/j.biortech.2018.03.126
Zhang, L., and Sun, X. (2019). Changes in physical, chemical, and microbiological properties during the two-stage composting of green waste due to the addition of β-cyclodextrin. Compost Sci. Utiliz. 27, 46–60. doi: 10.1080/1065657X.2019.1585304
Zhang, S., Wang, J., Chen, X., Gui, J., Sun, Y., and Wu, D. (2021). Industrial-scale food waste composting: effects of aeration frequencies on oxygen consumption, enzymatic activities and bacterial community succession. Bioresour. Technol. 320, 124357. doi: 10.1016/j.biortech.2020.124357
Keywords: composting, inoculants, microorganisms, organic matter, sustainable methods
Citation: Gaspar SS, Assis LLR, Carvalho CA, Buttrós VH, Ferreira GMdR, Schwan RF, Pasqual M, Rodrigues FA, Rigobelo EC, Castro RP and Dória J (2022) Dynamics of microbiota and physicochemical characterization of food waste in a new type of composter. Front. Sustain. Food Syst. 6:960196. doi: 10.3389/fsufs.2022.960196
Received: 02 June 2022; Accepted: 08 September 2022;
Published: 19 October 2022.
Edited by:
Laurent Dufossé, Université de la Réunion, FranceReviewed by:
Sandeep Mishra, Sardar Vallabhbhai National Institute of Technology Surat, IndiaMuhammad Waqas, Kohat University of Science and Technology, Pakistan
Copyright © 2022 Gaspar, Assis, Carvalho, Buttrós, Ferreira, Schwan, Pasqual, Rodrigues, Rigobelo, Castro and Dória. This is an open-access article distributed under the terms of the Creative Commons Attribution License (CC BY). The use, distribution or reproduction in other forums is permitted, provided the original author(s) and the copyright owner(s) are credited and that the original publication in this journal is cited, in accordance with accepted academic practice. No use, distribution or reproduction is permitted which does not comply with these terms.
*Correspondence: Joyce Dória, am95Y2UuZG9yaWEmI3gwMDA0MDt1ZmxhLmJy