- 1International Magnesium Institute, College of Resources and Environment, Fujian Agriculture and Forestry University, Fuzhou, China
- 2Key Laboratory of Plant-Soil Interactions, Ministry of Education, College of Resources and Environmental Sciences, National Academy of Agriculture Green Development, China Agricultural University, Beijing, China
- 3Fujian Provincial Key Laboratory of Soil Environmental Health and Regulation, College of Resources and Environment, Fujian Agriculture and Forestry University, Fuzhou, China
- 4College of Economics, Fujian Agriculture and Forestry University, Fuzhou, China
- 5College of Plant and Science, Jilin University, Changchun, China
Orchards in acid soils are at risk of magnesium (Mg) deficiency which negatively affects the plant growth, yield, and quality. However, the impacts of Mg supplementation on fruit yield, quality, and environmental and economic benefits have only been rarely addressed. We conducted 15 pomelo (Citrus grandis L.) orchard trials in South China to assess more efficient integrated nutrient management (INM) practices, including local farmer fertilization practices (FP; average application rate of nitrogen, phosphorus, and potassium were 1,075 kg N ha−1, 826 kg P2O5 ha−1, and 948 kg K2O ha−1, respectively), optimum fertilization practice (OPT; average application rate of nitrogen, phosphorus, and potassium were 550 kg N ha−1, 295 kg P2O5 ha−1, and 498 kg K2O ha−1, respectively) and optimum fertilization supplemented with Mg (OPT+Mg; average application rate of Mg was 196 kg MgO ha−1). The results showed that the yield, total soluble solid-to-titratable acidity ratio, and economic benefits under OPT practice were not significantly different from those of FP, while those of OPT+Mg were significantly higher than those of FP, by 8.76, 8.79, and 15.00%, respectively, while titratable acidity contents were significantly lower by 7.35%. In addition, compared with those from FP, the energy inputs and greenhouse gas (GHG) emissions from OPT were 31.00 and 26.48% lower, and those from OPT+Mg were 26.71 and 23.40% lower, respectively. Compared with those of OPT, the marginal efficiency of energy, GHG emissions, and capital of Mg under OPT+Mg were reduced by 62.30, 44.19, and 21.07%, respectively. Overall, adopting OPT+Mg for pomelo production could further enhance yield, fruit quality, and economic benefits while reducing the environmental burdens.
Key Points
- Agronomic-environmental-economic indicators of pomelo production are evaluated.
- Integrated nutrient management (INM) practices can increase fruit yield and quality.
- INM practices reduced energy inputs by 23–27% and GHG emissions by 27–31%.
- INM practices increased the economic efficiency of energy inputs and GHG emissions.
- Magnesium helps to obtain more and better products with lower environmental costs.
Introduction
Excessive nitrogen (N), phosphorus (P), and potassium (K) fertilizer application in agricultural production has caused severe negative environmental impacts, such as soil acidification (Guo et al., 2010), eutrophication (Conley et al., 2009; Huang et al., 2017), decreased air and water quality (Liu et al., 2013), and global warming (Paerl and Scott, 2010; Steffen et al., 2015). Integrated nutrient management (INM), therefore, is urgently required for the sustainability of higher crop production while improving the soil health and environmental safety (Verma et al., 2010; Yu et al., 2017), which has also been proven to be achievable and highly successful (Chen et al., 2014; Cui et al., 2018). Currently, INM practices are applied to major cereal crops (Nath et al., 2011; Jiao et al., 2018) and greenhouse vegetables (Yang et al., 2016; Wang et al., 2021). However, little is known about the effects of INM in orchard systems. Therefore, it is imperative to address the issues and challenges related to nutrient management in fruit production systems to overcome the problems of poor yield and quality.
Pomelo (Citrus grandis L.) is the third major type of citrus in production after mandarin (Citrus reticulata L.) and oranges (Citrus sinensis L.) (Li et al., 2015). Pinghe County is a key area of pomelo cultivation in China (Wei et al., 2020). However, excessive fertilization has caused various problems in pomelo orchard, such as sharp declines in fruit and soil quality and with a high product carbon footprint in recent years (Zhang et al., 2003; Li et al., 2015; Guo et al., 2019; Chen et al., 2020). Large amounts of N, P, and K fertilizer are applied in this region, but soil magnesium (Mg) deficiency is typically ignored. In contrast, Mg deficiency frequently occurs in orchards (Wallace, 1940; Diao et al., 2013) and can affect yield (Li et al., 2015; Dechen et al., 2016). However, the impacts of Mg on yield and quality improvement in agricultural products have been overlooked (Yan and Hou, 2018; Guo et al., 2020). Recently, a meta-analysis of data from 99 field studies revealed that Mg application could increase crop yield by ~8.5% (Wang et al., 2020). Therefore, INM for citrus production must address the management of macro-elements and secondary macronutrients such as Mg during crop production system (Hien et al., 2017).
In China, energy consumption and greenhouse gas (GHG) emissions caused by agriculture account for approximately 6 and 17% of the national total energy consumption and GHG emissions, respectively (Dong et al., 2008; Lin and Fei, 2015). More agricultural production will lead to greater energy consumption and carbon emissions (Koondhar et al., 2020). Generally, fertilizer is considered an important factor influencing energy consumption and GHG emissions in different agricultural crop production systems (Moradi et al., 2018; Baran et al., 2020; Khanali et al., 2021). Whereas, reducing fertilizer application is known to reduce energy inputs and GHG emissions (Chen et al., 2020), but it remains unclear whether adding Mg fertilizer with reduced fertilizer inputs would further amplify this efficiency or not?
Overall, in addition to on-farm evaluations of fruit products and economic benefits, understanding and quantifying the environmental costs of fruit production under different nutrient management systems may provide additional information to help identify greener and more efficient INM strategies (Wang et al., 2018). Therefore, this study aims to demonstrate a suitable fertilization strategy for the sustainable production of pomelo in terms of yield, fruit quality, energy inputs, GHG emissions, and economic benefits and to provide a reference for highly intensive and potentially Mg-deficient citrus production worldwide.
Materials and Methods
Study Area
The study area is located in Pinghe County (24°02′-24°35′ N, 116°54′-117°31′ E, and 10–1,545 m above sea level), Zhangzhou city, Fujian Province, Southeast China (Figure 1). It is characterized by a subtropical oceanic monsoon climate with an annual average temperature of 17.5–21.3°C. The annual precipitation is approximately 1,600–2,000 mm. The soil types in this study area are ferralsols, classified as red soils in the Chinese soil classification (Smith, 2014). Low-elevation mountains and hills are the main landforms in Pinghe County; these landforms are distributed mostly in the valleys and intermountain regions of the Huashanxi Basin, accounting for 91.5% of the total area (www.pinghe.gov.cn).
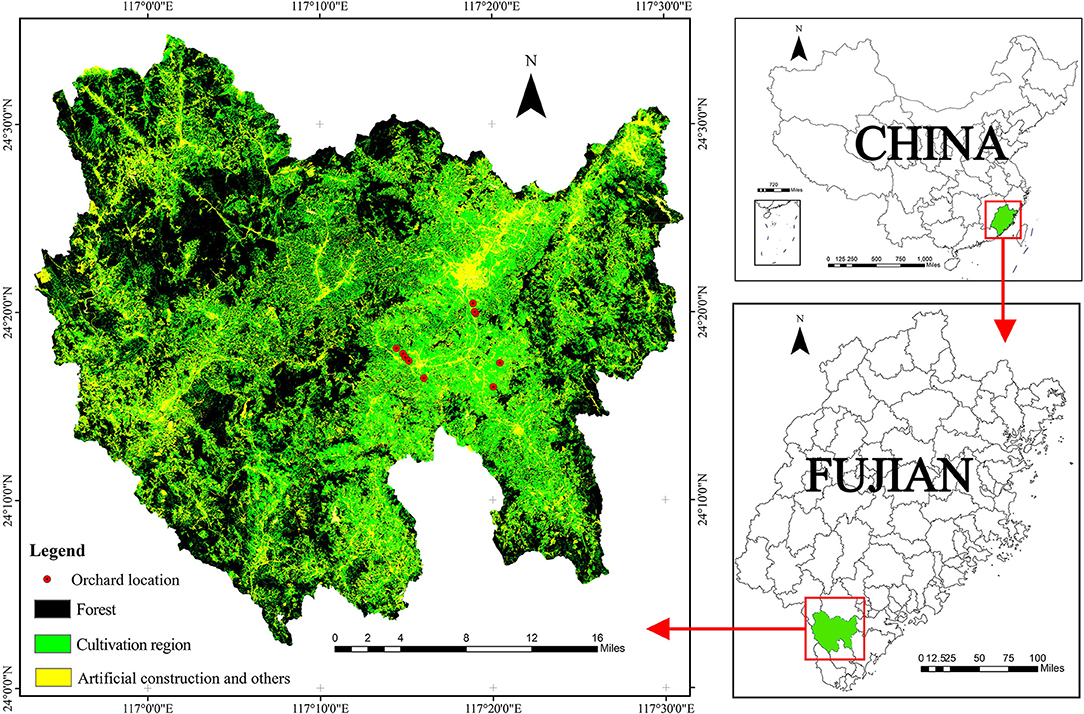
Figure 1. Geographical distribution of field experimental sites within Pinghe County, China, in 2017–2020.
Field Experimental Design
Fifteen pomelo orchards trials in total were conducted during 2017–2020 in Pinghe County (Figure 1) to explore the effects of reduced chemical fertilizers input and their integrated use with Mg fertilizer on the yield, quality, energy balance, GHG emissions, and economic benefits of pomelo production during the entire production and packing process. Urea, superphosphate, potassium sulfate, and magnesium sulfate were used as the sources of N, P2O5, K2O, and MgO in fertilizer, respectively, and were applied to pomelo orchards in each growing season (Figure 2). The tested nutrient management practices included local farmer practices (FP; in 10 orchards with 95 trees) as the control and INM strategies, namely, an optimum NPK treatment (OPT; in 13 orchards with 105 trees) and the optimum NPK treatment with magnesium (OPT+Mg; in 13 orchards with 105 trees), as the experimental treatments. The fertilizer application rates for the OPT strategies were determined by agronomist recommendations based on the target yield and the soil fertility level, which varied from field to field (Obreza and Morgan, 2008; Li et al., 2019). The mean fertilizer application rates for N, P2O5, K2O, and MgO have been shown in Figure 2. Except for the differences in fertilizer use, all management methods applied to the orchards were the same. The detailed information about the fertilizer usage and other material (energy) inputs are shown in Supplementary Table 1. Titratable acid (TA) was determined by the titration method (Jiang et al., 2004), and the total soluble solid (TSS) concentration was measured by a hand-held Brix meter (Japan, PAL-1). The soil physical and chemical properties of the experimental sites have been shown in Supplementary Table 2.
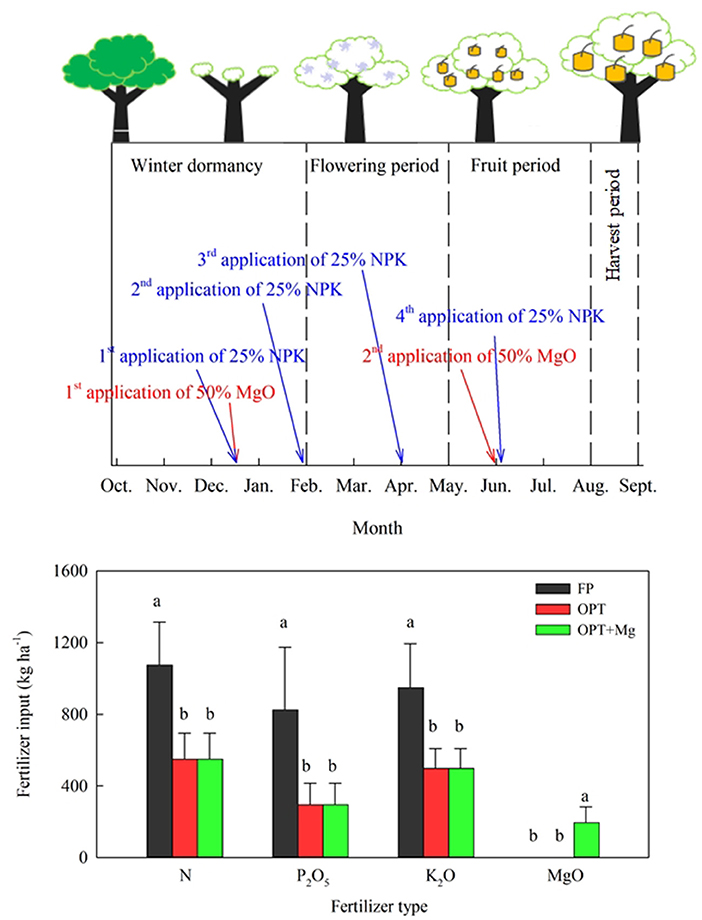
Figure 2. Chemical fertilizer application time and amount in the different treatments of pomelo production. The black error bars in the figure indicate the overall standard deviations (SDs). Different letters above histogram bars indicate statistically significant differences at P < 0.05.
System Boundaries and Functional Units
The system boundaries included the pomelo production upstream stage, the planting stage, and the postharvest stage (Figure 3). The upstream stage included all inputs used in pomelo production, such as chemical fertilizers, farmyard manure, pesticides, paper bags (for fruit bagging), diesel fuel, and human labor (considered only as an energy flow). The postharvest stage included electricity, packing bags, and human labor (considered only an energy flow). The energy output in the planting stage was highly dependent on the pomelo harvest, and the GHG emissions (in carbon dioxide equivalents; CO2 eq) from the planting stage included direct nitrous oxide (N2O), indirect N2O (ammonia (NH3) emissions, and nitrate () runoff and leaching), and methane (CH4) (IPCC, 2019; Chen et al., 2020). The functional units for pomelo production were one ton (t) of fresh product and one hectare (ha) of orchard area.
Energy and GHG Emission Quantification
The energy inputs, energy outputs, and net energy outputs of pomelo production were estimated using the following equations (Equations 1–3). The energy input per unit area (ha) was calculated as the sum of the partial energy equivalent of each input used in GJ ha−1 (GJ = 109 J).
Where I represent the kind of input, Ii is the amount of the ith input, EEi is the energy equivalent of the ith input, and EEpomelo represents the energy equivalent of the pomelo output.
The GHG emissions (t CO2 eq ha−1) were calculated using the following equations (Equations 4–9). The upstream GHG emissions per unit area (ha) were calculated as the sum of the partial CO2 emissions of each input used in pomelo production. The GHG emissions in the planting stage were estimated as described in Chen et al. (2020). The postharvest GHG emissions per unit area (ha) were calculated as the sum of the partial CO2 emissions from each input used in the pomelo postharvest stage.
Where j represents the type of upstream input; Ij is the amount of the jth input; EFj is the emission coefficient of the jth input; CN and MN are the quantities of N in chemical fertilizer and farmyard manure applied during the annual production season, respectively; MC represents the total carbon input from manure application; 1.20% (Xie et al., 2014), 10.80% (Ge et al., 2011), and 10.00% (Qian et al., 2012; Ventura et al., 2013) are the scaling factors for N2O, NH3, and emissions or runoff and leaching from chemical N fertilizer application; 0.60% and 29.30% represent the scaling factors for N2O and NH3 emissions from farmyard manure application (Zhang et al., 2017); 0.01 and 0.0075 are the conversion coefficients of NH3 volatilization and runoff and leaching, respectively, in N2O equivalents (Eggleston et al., 2006); 44/28 is the molecular conversion factor of N2 to N2O; 265 is the global warming potential of N2O for a 100-year period (Change, 2014); 0.20% is the CH4 emission coefficient of farmyard manure applied in the field (Wan et al., 2014); 16/12 is the molecular conversion factor of C to CH4; 28 is the global warming potential of CH4 for a 100-year period (Change, 2014); k represents the type of postharvest input; Ik is the amount of the kth input; and EFk is the emission coefficient of the kth input. All energy equivalents and CO2 emission coefficients used are listed in Table 1.
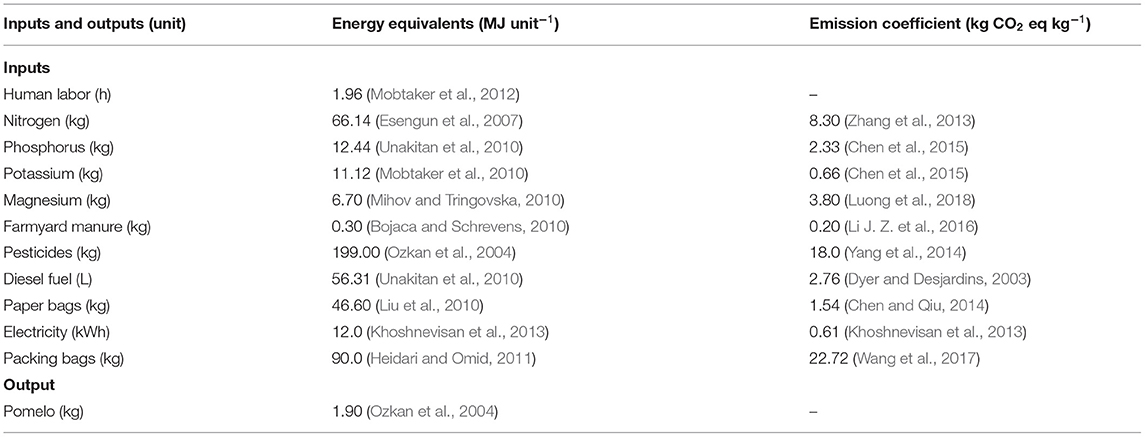
Table 1. Energy equivalents and greenhouse gas (GHG) emission coefficients in agricultural production.
The environmental cost efficiency was calculated with Equation (10). To measure the benefits of Mg fertilization, three indicators were set up and modeled on the concept of marginal benefit in economics (Slemrod and Yitzhaki, 2001): marginal energy efficiency, marginal GHG emission efficiency, and marginal capital efficiency (Equation 11). These three indicators represent the energy input cost, GHG emission cost, and capital investment cost of the increased yield due to Mg fertilization.
where U refers to energy input (GJ), GHG emissions (t CO2 eq), and capital investment (million CNY, only for marginal efficiency calculation).
Data Analysis
Data processing and visualization were performed using Microsoft Office Excel 2019, ArcGIS 10.2, and Easy Paint Tool SAI 1.2.0. Note that nearest-neighbor interpolation in the curve fitting tool of MATLAB R2019b was used to make the contour map of energy input, GHG emissions, and economic benefits. All statistical analyses were conducted using SPSS 21.0. One-way analysis of variance (ANOVA) and least significant difference (LSD) tests were used to detect the differences among different nutrient managements. The levels of significance were defined at P < 0.05 (*), P < 0.01 (**), and P < 0.001 (***).
Results
Yield and Quality
The different fertilization treatments significantly affected the pomelo yield and fruit quality (Table 2). The yield under OPT was not significantly different from that under FP, but OPT+Mg resulted in 8.76% higher yield than FP. The number of hanging fruits was the decisive factor in this significant difference. Compared with FP, OPT, and OPT+Mg did not affect the edible rate and TSS concentration, while OPT+Mg treatment significantly reduced the TA concentration by 7.35% and increased the TSS/TA by 8.79%.
Energy Balance
The energy input was highest under FP (176.29 GJ ha−1), while inputs of 129.60 and 135.04 GJ ha−1 were required for OPT and OPT+Mg, respectively (Figure 4A). Chemical fertilizer and paper bags were the major sources of energy input in all fertilization treatments (Figure 4B). The average chemical fertilizer and paper bag energy inputs of FP, OPT, and OPT+Mg accounted for 51.92 and 19.44%, 35.28 and 26.38%, and 34.89 and 27.66% of their total energy inputs, respectively.
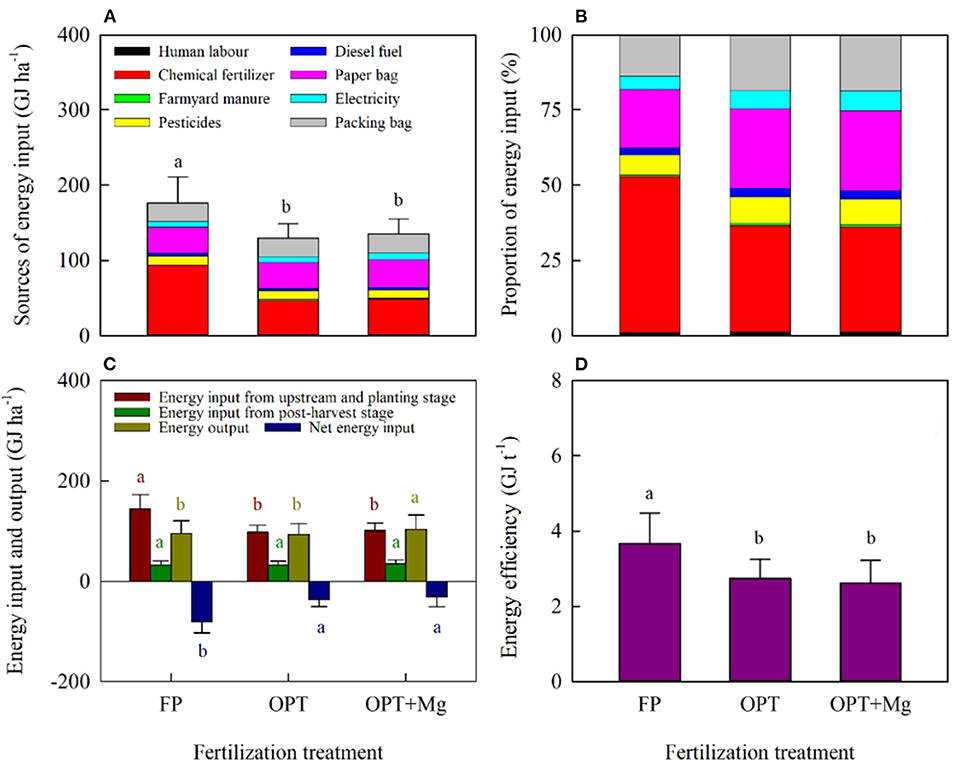
Figure 4. Different sources (A) and proportions (B) of energy input, energy input and output (C), and energy efficiency (D) under different fertilization treatments in pomelo production. The black error bars in the figure indicate the standard deviation (SDs). Different letters above histogram bars with the same color indicate statistically significant differences at P < 0.05.
Compared with the FP treatment, the OPT and OPT+Mg treatments significantly reduced the energy inputs of the upstream and planting stages, while no significant difference was recorded between these treatments (Figure 4C). The highest energy output for the postharvest stage was observed in OPT+Mg, but no significant difference was found between FP and OPT. In addition, the net energy outputs of OPT (−36.40 GJ ha−1) and OPT+Mg (−31.46 GJ ha−1) were also significantly different from that of FP (−81.07 GJ ha−1). The average energy efficiency values (Figure 4D) were 2.74 GJ t−1 under OPT and 2.62 GJ t−1 under OPT+Mg, which were significantly lower than that under FP (3.67 GJ t−1).
GHG Emissions
The GHG emissions from both OPT (18.99 t CO2 eq ha−1) and OPT+Mg (20.17 t CO2 eq ha−1) treatments were significantly lower than that from FP (27.52 t CO2 eq ha−1), and there was no significant difference between OPT and OPT+Mg (Figure 5A). The application of chemical fertilizer had the highest impact on the total GHG emissions, accounting for 63.90, 45.69, and 46.59% of emissions under FP, OPT, and OPT+Mg, respectively (Figures 5A,B). Packing bags were the second major source of GHG emissions, accounting for 22.43, 32.86, and 32.64%, and other emission sources accounted for 13.67, 21.45, and 20.77% of emissions from FP, OPT, and OPT+Mg, respectively.
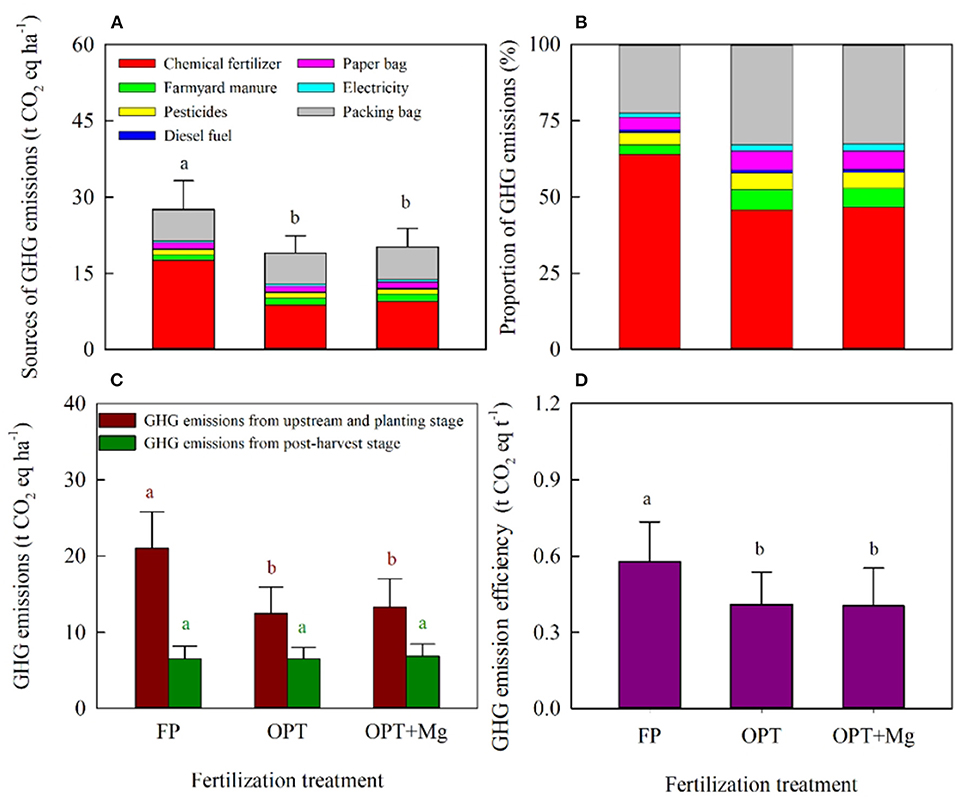
Figure 5. Different sources (A) and proportions (B) of greenhouse gas (GHG) emissions, GHG emissions from different stages (C), and GHG emissions efficiency (D) under different fertilization treatments in pomelo production. The black error bars in the figure indicate the standard deviations (SDs). Different letters above histogram bars with the same pattern indicate statistically significant differences at P < 0.05.
The GHG emissions from the upstream and planting stages under both OPT (12.48 t CO2 eq ha−1) and OPT+Mg (13.29 t CO2 eq ha−1) were significantly lower than those under FP (21.01 t CO2 eq ha−1), while there were no significant differences between OPT and OPT+Mg (Figure 5C). The GHG emissions from the postharvest stage were not significantly different among the three treatments. In this study, the GHG emission efficiency from FP was 0.58 t CO2 eq t−1, which was significantly higher than those from OPT and OPT+Mg (by 40.80 and 43.17%, respectively) (Figure 5D).
Economic Benefits
The economic costs of FP were significantly higher than those of OPT and OPT+Mg (by 13.49 and 4.97%, respectively), and the cost of OPT was significantly lower than that of OPT+Mg (Figure 6A). Human labor had the highest impact and represented 49.52, 55.02, and 53.57% of the total costs of FP, OPT, and OPT+Mg, respectively (Figures 6A,B). Compared with FP, the proportion of chemical fertilizer costs in OPT and OPT+Mg decreased from 21.56% to 11.39–14.52%. In addition, pesticides (accounting for 12.58–13.73% of costs) and paper bags (accounting for 11.01–12.56% of costs) were also important sources of economic costs.
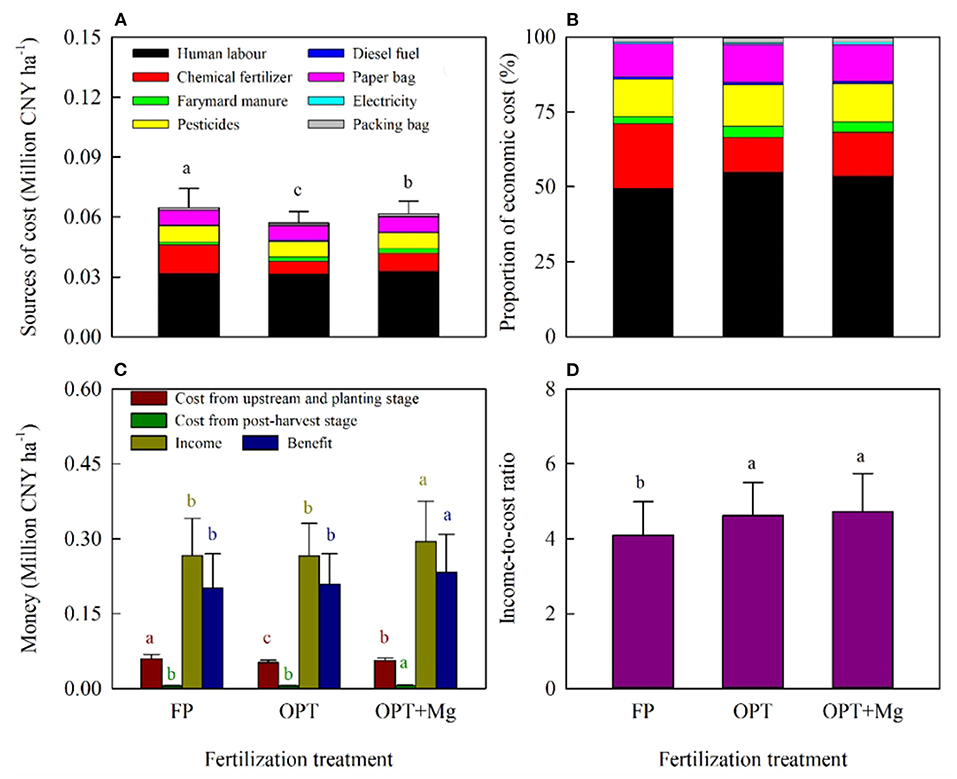
Figure 6. Different sources (A) and proportions of economic costs (B), costs and income (C), and income-to-cost ratio (D) under different fertilization treatments in pomelo production. The black error bars in the figure indicate the overall standard deviations (SDs). Different letters above histogram bars indicate statistically significant differences at P < 0.05.
The costs from the upstream stage to the planting stage of FP were significantly different from those of OPT and OPT+Mg, while the highest costs in the postharvest stage were observed under the OPT+Mg treatment. The income of OPT+Mg was significantly higher than that of FP and OPT, by 10.46 and 10.80%, respectively (Figure 6C). The economic benefit of OPT+Mg (0.23 million CNY ha−1) was significantly higher than that of FP (0.20 million CNY ha−1) and OPT (0.21 million CNY ha−1). The income-to-cost ratio was 4.62 under OPT and 4.72 under OPT+Mg, both significantly higher than that under FP (4.09) (Figure 6D).
Coupled Analysis of Yield, Energy Input, and GHG Emissions
Compared with FP, OPT and OPT+Mg resulted in the same yield with lower energy inputs and GHG emissions (Figures 7A,B). Although there was no significant linear relationship between economic benefit and GHG emissions or energy input (Figures 7C,D), the contour map of yield-energy input-GHG emissions clearly shows that OPT+Mg is better than OPT and FP and that OPT+Mg provided the highest economic benefits with the lowest energy inputs and GHG emissions (Figure 8).
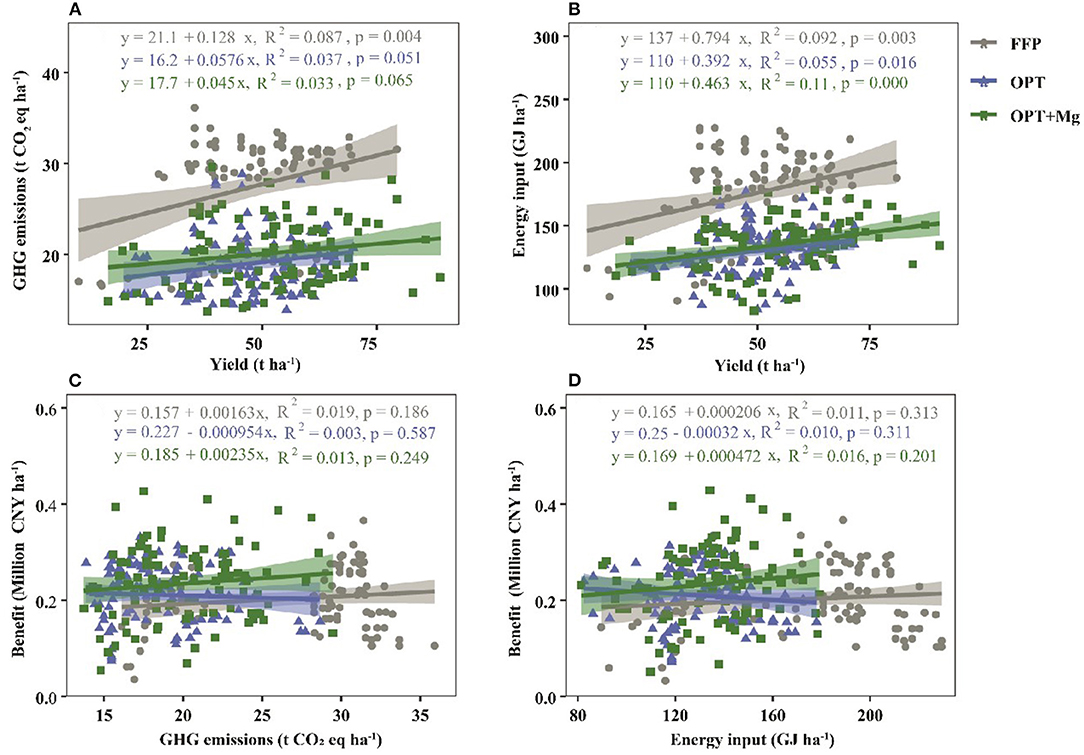
Figure 7. Linear relationship between yield and GHG emissions (A), yield and energy input (B), GHG emissions and benefit (C), energy input and benefit (D). The different colors of the scattered dots, lines, shadows, and formulas represent the different fertilizer treatments. The shaded areas represent the 95% confidence intervals.
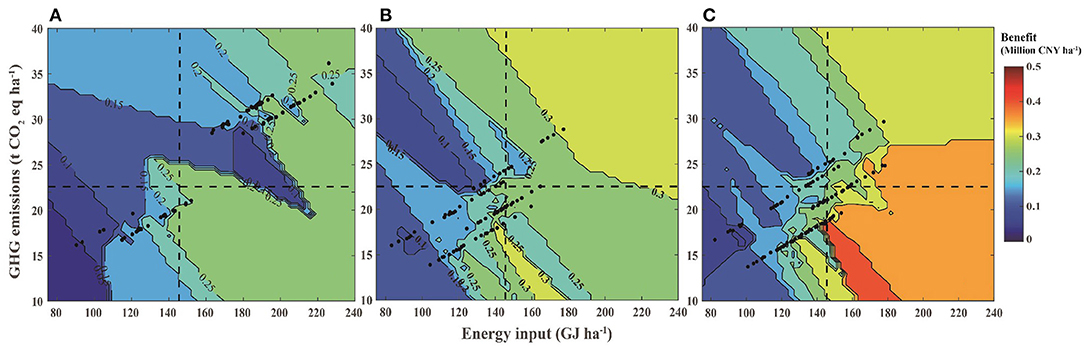
Figure 8. Contour maps of energy inputs, GHG emissions, and economic benefits in different fertilizer treatments. (A–C) represent FP, OPT, and OPT+Mg, respectively. The dotted lines represent the average values of energy input and GHG emissions. The black dots represent the three-dimensional coordinates of the original data points, and the different numbers on the contour lines indicate the economic benefit values.
Discussion
Integrated nutrient management (INM) is a feasible way to receive future economic and environmental benefits from agricultural production (Chen et al., 2014; Zhang et al., 2016; Cui et al., 2018). Our results suggest that Mg management during INM practices is integral to promoting green, high-quality development in agricultural systems.
Strengthening Mg and Optimizing NPK to Further Increase Yield
To achieve a high yield, smallholders in China often depend on using large amounts of external inputs (especially high levels of N, P, and K fertilizers) (Zhang Q. et al., 2020). Empirical evidence has shown that when fruit yield nears the theoretically highest level, the yield responses to additional N, P, and K fertilizer application become almost nil (Zhang et al., 2012; Ahmed et al., 2017), while the secondary macronutrient element, such as Mg has become the key factors responsible for further increases in fruit yield, especially in areas where soil Mg is deficient (Wang et al., 2020).
According to Li et al. (2019), the recommended rates of N, P2O5, and K2O fertilizer application to produce one ton of citrus fruit are 10.4, 5.2, and 8.2 kg on average, respectively. However, these values in Pinghe County were 21.45 (N), 16.48 (P2O5), and 18.92 (K2O) kg on average in conventional practices (Figure 2). It indicates that in Pinghe County, farmer knowledge about the nutrient management needed to meet pomelo nutrient requirements is very limited (Li Q. et al., 2016; Chen et al., 2020). The current study showed that the traditional fertilizer application rate could be reduced by nearly 53% (49, 47, and 64% for N, P2O5, and K2O, respectively) on average without significantly reducing the pomelo yield (Table 2). Therefore, this fertilizer reduction measure proved effective for pomelo production in Pinghe County. This strategy has also been proven effective for apple orchards in China (Wang et al., 2016).
As an essential nutrient for plant growth, Mg is involved in physiological and biochemical processes such as chlorophyll synthesis, photosynthesis, and enzyme activation (Cakmak and Yazici, 2010; Chen et al., 2018; Tian et al., 2021). Mg is also involved in carbohydrate transport from source-to-sink organs; hence, the excess carbohydrate accumulation in leaves rather than transported to fruit and grain results from Mg deficiency (Farhat et al., 2016; Tian et al., 2021). Therefore, an adequate amount of Mg is particularly required during the period of reproductive growth (Römheld and Kirkby, 2009). Various studies have shown that higher Mg concentration enhances the plant resistance to various environmental stresses such as light, heat, and drought (Cakmak, 2013; Gransee and Führs, 2013; Mengutay et al., 2013). Moreover, Mg deficiency also interferes with N metabolism, which has important implications for crop production and the environment (Grzebisz, 2013; Zhang et al., 2021). Therefore, Mg deficiency would affect plant development and the formation of yield and quality. However, despite this recognition, the role of Mg in crop nutrition management has not received much attention compared to other major nutrients (Cakmak and Yazici, 2010; Guo et al., 2016).
Mg uptake by plants can be low due to competition from other cations, including potassium (K+), ammonium (), and aluminum (Al3+), especially in acidic soils that are low in Mg (Gransee and Führs, 2013). The soil survey results showed that the soil pH of all 44 samples from pomelo orchards in Pinghe County ranged from 3.5 to 4.9, which is lower than the optimum range of 5.0–6.5 for pomelo growth (Guo et al., 2019). Previous research showed that the improvement of crop production by Mg in acidic soils was three times greater than that in calcareous soils (Wang et al., 2020). Despite the importance of Mg, few farmers have considered its role in pomelo production. Therefore, the positive response of pomelo yield to fertilizer reduction and Mg fertilizer addition is not surprising (Table 2; Figure 7). Although the yield under OPT was not significantly different from that under FP, OPT+Mg resulted in an 8.77% higher yield than FP (Table 2). Many other orchard experiments also revealed that Mg enhances the yield and quality of pear (Fawzi et al., 2010), vine grapes (Zlámalová et al., 2015), wax gourd (Zhang B. et al., 2020), and pepper (Lu et al., 2021). In short, to facilitate the sustainable and green development of agriculture in Mg-deficient regions, it is necessary to strengthen cooperation among governments, enterprises, and institutions to guide farmers (Zhang et al., 2016) to use the Mg fertilizer scientifically while reducing the application of N, P and K fertilizers.
Environmental Cost Efficiency
The energy inputs and GHG emissions of OPT and OPT+Mg were significantly lower than those of FP (Figures 4A, 5A) and were influenced mostly by chemical fertilizer (Figures 4B, 5B). Therefore, reducing fertilizer application is important for improving energy and GHG efficiency in pomelo production. These findings are consistent with existing reports that energy inputs and GHG emissions can be reduced substantially under advanced INM practices (Chen et al., 2014; Wu and Ma, 2015; Sarkar et al., 2018; Fathi et al., 2020). Although OPT+Mg slightly increased the energy inputs and GHG emissions in pomelo production by 4.20 and 6.21% (Figures 4A, 5A), respectively, the pomelo yield was significantly higher (by 11.14%) than that under OPT (Table 2). Indeed, the potential of Mg to increase the economic benefits of agricultural production cannot be neglected. Compared with FP, the economic benefits of OPT and OPT+Mg were 3.40 and 15.33% higher, respectively, because of cost savings and income increments (Figure 6A). Mg application can also improve economic efficiency in other systems, such as maize and tea gardens (Jat et al., 2018; Huang et al., 2020).
Further analysis revealed that adding Mg fertilizer can provide higher economic benefits with lower environmental costs (Figure 8). In addition, compared with that in OPT, the marginal efficiency of energy, GHG emissions and capital of Mg in OPT+Mg were reduced by 62.30, 44.19 and 21.07%, respectively (Table 2; Figures 3–5). INM practices can increase the economic efficiency of energy inputs and GHG emissions (Figure 9). The average energy consumption values needed to obtain one unit of economic benefit were 665.26 and 637.09 GJ per million CNY under OPT and OPT+Mg, respectively, which were significantly lower than that under FP (982.05 GJ per million CNY). The GHG emissions needed to obtain one unit of economic benefit under FP, OPT, and OPT+Mg were 155.01, 99.40, and 98.27 t CO2 eq per million CNY, respectively. Unfortunately, very little research has been conducted on the influences of Mg fertilizer application on energy and GHG emission efficiency in agroecosystems. Therefore, the present study provides strong evidence for promoting Mg application in agricultural production.
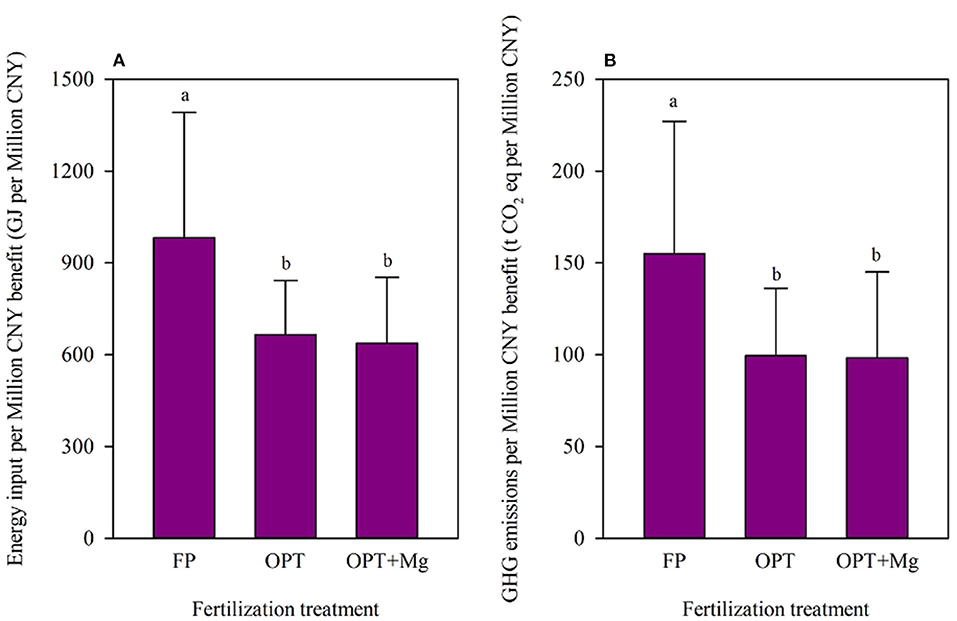
Figure 9. Energy input (A) and GHG emissions (B) per million CNY of economic benefit under different fertilization treatments. The black error bars in the figure indicate the standard deviations (SDs). Different letters on the histogram bars indicate statistically significant differences at P < 0.05.
Outlook and Limitations
Although we quantitatively evaluated the effects of different nutrient management strategies on yield, quality, economic benefits, energy consumption, and GHG emissions in pomelo production, the present study had several limitations. First, carbon sequestration in orchard systems (Chen et al., 2020) was ignored at the research boundary because this was not the focus of the present study. This will introduce uncertainty because the capability for carbon sequestration in orchards depends on fruit tree age (Wu et al., 2012), vegetation, climate, and management practices (Liu et al., 2011). Second, due to data and method limitations, we used a set of commonly used empirical N2O and NH3 emission parameters and runoff and leaching rates. However, reactive N loss and N surplus have an exponential relationship rather than a linear relationship (Cui et al., 2013), and N2O, NH3, and losses from fertilizer application are greatly impacted by the application rate (Ge et al., 2011; Wang et al., 2019), especially in extremely overfertilized systems. Therefore, the GHG emissions in this study may be underestimated. Third, fertilization based on the “4 Rs” (right rate, right source, right time, and right place) is the critical nutrient management approach for sustaining crop productivity (Mikkelsen, 2011; Chen et al., 2020). The findings of this study showed that reducing NPK fertilizer application while adding Mg fertilizer resulted in higher yields and better fruit quality with lower environmental costs. However, the potential for fertilizer reduction and a scientifically determined appropriate Mg application rate has yet to be precisely determined.
Conclusion
Over fertilization with nitrogen (N), phosphorus (P), and potassium (K) in pursuit of higher economic benefits is very common in the fruit production system of China. In the present study, different nutrient management strategies for pomelo production were evaluated based on yield, fruit quality, energy balance, greenhouse gas (GHG) emissions, and economic benefits. The results suggested that reducing conventional N, P, and K fertilization by 53% is feasible. This reduction would increase the efficiency of energy input and GHG emissions in pomelo production systems. Moreover, adding magnesium (Mg) while reducing N, P, and K fertilizer application could further mitigate the environmental costs and enhance the yield, fruit quality, and economic benefits (Figure 10). Therefore, China's traditional intensive, high-input nutrient management practices in fruit systems urgently need to be changed. A key focus of the future green development of fruit production will be how to obtain the highest return with the lowest input, and Mg could prove to be a key component.
Data Availability Statement
The original contributions presented in the study are included in the article/Supplementary Material, further inquiries can be directed to the corresponding author/s.
Author Contributions
XC: conceptualization, writing—original draft, writing-review and editing, formal analysis, and visualization. XY: conceptualization, investigation, writing—original draft, formal analysis, and visualization. MM: writing—review and editing. XW and CM: visualization. YC: formal analysis and visualization. YL, SZha, WZ, and WY: data collection. LW: conceptualization, resources, supervision, and funding acquisition. SZho: supervision. FZ: resources, supervision, and funding acquisition. All authors contributed to the article and approved the submitted version.
Funding
This research was supported by the Technology Innovation Fund Project of Fujian Agriculture and Forestry University (KFA19075A) and the National Natural Science Foundation of China (31501832).
Conflict of Interest
The authors declare that the research was conducted in the absence of any commercial or financial relationships that could be construed as a potential conflict of interest.
Publisher's Note
All claims expressed in this article are solely those of the authors and do not necessarily represent those of their affiliated organizations, or those of the publisher, the editors and the reviewers. Any product that may be evaluated in this article, or claim that may be made by its manufacturer, is not guaranteed or endorsed by the publisher.
Supplementary Material
The Supplementary Material for this article can be found online at: https://www.frontiersin.org/articles/10.3389/fsufs.2022.948810/full#supplementary-material
References
Ahmed, M., Rauf, M., Mukhtar, Z., and Saeed, N. A. (2017). Excessive use of nitrogenous fertilizers: an unawareness causing serious threats to environment and human health. Environ. Sci. Pollut. R. 24, 26983–26987. doi: 10.1007/s11356-017-0589-7
Baran, M. F., Eren, O., Gökdogan, O., and Oguz, H. I. (2020). Determination of energy efficiency and greenhouse gas (GHG) emissions in organic almond production in Turkey. Erwerbs Obstbau 62, 341–346. doi: 10.1007/s10341-020-00507-9
Bojaca, C., and Schrevens, E. (2010). Energy assessment of peri-urban horticulture and its uncertainty: case study for Bogota, Colombia. Energy 35, 2109–2118. doi: 10.1016/j.energy.2010.01.029
Cakmak, I. (2013). Magnesium in crop production, food quality and human health. Plant Soil 368, 1–4. doi: 10.1007/s11104-013-1781-2
Cakmak, I., and Yazici, A. M. (2010). Magnesium: a forgotten element in crop production. Better Crop. 94, 23–25.
Change, I. C. (2014). “Mitigation of climate change,” in Contribution of Working Group III to the Fifth Assessment Report of the Intergovernmental Panel on Climate Change 1454.
Chen, C., and Qiu, R. Z. (2014). Estimation of energy consumption and carbon emissions of China's pulp and paper industry. China Pulp. 33, 50e55. (in Chinese).
Chen, S., Lu, F., and Wang, X. K. (2015). Estimation of greenhouse gases emission factors of China's nitrogen, phosphate and potash fertilizers. Acta Eclo. Sin. 35, 6371e6383. (in Chinese). doi: 10.5846/stxb201402210304
Chen, X., Cui, Z., Fan, M., Vitousek, P., Zhao, M., Ma, W., et al. (2014). Producing more grain with lower environmental costs. Nature 514, 486–489. doi: 10.1038/nature13609
Chen, X., Xu, X., Lu, Z., Zhang, W., Yang, J., Hou, Y., et al. (2020). Carbon footprint of a typical pomelo production region in China based on farm survey data. J. Clean. Prod. 277, 124041. doi: 10.1016/j.jclepro.2020.124041
Chen, Z. C., Peng, W. T., Li, J., and Liao, H. (2018). Functional dissection and transport mechanism of magnesium in plants. Semin. Cell Dev. Biol. 74, 412–152. doi: 10.1016/j.semcdb.2017.08.005
Conley, D. J., Paerl, H. W., Howarth, R. W., Boesch, D. F., Seitzinger, S. P., Havens, K. E., et al. (2009). Controlling eutrophication: nitrogen and phosphorus. Science 323, 1014–1015. doi: 10.1126/science.1167755
Cui, Z., Zhang, H., Chen, X., Zhang, C., Ma, W., Huang, C., et al. (2018). Pursuing sustainable productivity with millions of smallholder farmers. Nature 555, 363–366. doi: 10.1038/nature25785
Cui, Z. L., Yue, S. C., Wang, G. L., Zhang, F. S., and Chen, X. P. (2013). In-Season root-zone N management for mitigating greenhouse gas emission and reactive N losses in intensive wheat production. Environ. Sci. Technol. 47, 60105–60122. doi: 10.1021/es4003026
Dechen, A., Carmello, Q., Monteiro, F., and Nogueirol, R. (2016). Role of magnesium in food production: an overview. Crop Pasture Sci. 66, 1213–1218. doi: 10.1071/CP15094
Diao, L. H., Peng, L. Z., Chun, C. P., Ling, L. L., Li, X., Xue, J., et al. (2013). Survey of soil available magnesium content at navel orange orchards in southern Jiangxi province. J. Fruit Sci. 30, 241–247. (in Chinese).
Dong, H., Li, Y. e, Tao, X., Peng, X., Li, N., et al. (2008). China greenhouse gas emissions from agricultural activities and its mitigation strategy. Trans. Chin. Soc. Agr. Eng. 24, 269–273. (in Chinese).
Dyer, J., and Desjardins, R. (2003). Simulated farm fieldwork, energy consumption and related greenhouse gas emissions in Canada. Biosyst. Eng. 85, 503–513. doi: 10.1016/S1537-5110(03)00072-2
Eggleston, H., Buendia, L., Miwa, K., Ngara, T., and Tanabe, K. (2006). 2006 IPCC Guidelines for National Greenhouse Gas Inventories. Japan. Available online at: https://www.ipcc-tfi.iges.or.jp/meeting/pdfiles/Washington_Report.pdf. (accessed April 16, 2021).
Esengun, K., Gündüz, O., and Erdal, G. (2007). Input–output energy analysis in dry apricot production of Turkey. Energ. Convers. Manage. 48, 592–598. doi: 10.1016/j.enconman.2006.06.006
Farhat, N., Elkhouni, A., Zorrig, W., Smaoui, A., Abdelly, C., and Rabhi, M. (2016). Effects of magnesium deficiency on photosynthesis and carbohydrate partitioning. Acta Physiol. Plant. 38, 145. doi: 10.1007/s11738-016-2165-z
Fathi, A., Barari Tari, D., Fallah Amoli, H., and Niknejad, Y. (2020). Study of energy consumption and greenhouse gas (GHG) emissions in corn production systems: influence of different tillage systems and use of fertilizer. Commun. Soil Sci. Plan. 51, 769–778. doi: 10.1080/00103624.2020.1729373
Fawzi, M., Shahin, F., Elham, A., and Kandil, E. (2010). Effect of organic and bio-fertilizers and magnesium sulphate on growth yield, chemical composition and fruit quality of “Le-Conte” Pear trees. Nat. Sci. 8, 273–280.
Ge, S. F., Jiang, Y. M., Wei, S. C., and Fang, X. J. (2011). Nitrogen balance under different nitrogen application rates in young apple orchards. Plant Nutr. Fert. Sci. 17, 949e955. (in Chinese).
Gransee, A., and Führs, H. (2013). Magnesium mobility in soils as a challenge for soil and plant analysis, magnesium fertilization and root uptake under adverse growth conditions. Plant Soil 368, 5–21. doi: 10.1007/s11104-012-1567-y
Grzebisz, W. (2013). Crop response to magnesium fertilization as affected by nitrogen supply. Plant Soil 368, 23–39. doi: 10.1007/s11104-012-1574-z
Guo, J. H., Liu, X. J., Zhang, Y., Shen, J. L., Han, W. X., Zhang, W. F., et al. (2010). Significant acidification in major Chinese croplands. Science 327, 1008–1010. doi: 10.1126/science.1182570
Guo, J. X., Yang, J. C., Zhang, L. J., Chen, H. H., Jia, Y. M., Wang, Z., et al. (2019). Lower soil chemical quality of pomelo orchards compared with that of paddy and vegetable fields in acidic red soil hilly regions of Southern China. J. Soil Sediments 19, 2752–2763. doi: 10.1007/s11368-019-02273-y
Guo, W. L., Nazim, H., Liang, Z. S., and Yang, D. F. (2016). Magnesium deficiency in plants: an urgent problem. Crop J. 4, 83–91. doi: 10.1016/j.cj.2015.11.003
Guo, Y., Chen, Y., Searchinger, T. D., Zhou, M., Pan, D., Yang, J., et al. (2020). Air quality, nitrogen use efficiency and food security in China are improved by cost-effective agricultural nitrogen management. Nat. Food 1, 648–658. doi: 10.1038/s43016-020-00162-z
Heidari, M., and Omid, M. (2011). Energy use patterns and econometric models of major greenhouse vegetable productions in Iran. Energy 36, 220–225. doi: 10.1016/j.energy.2010.10.048
Hien, H. N., Maneepong, S., and Suraninpong, P. (2017). Effects of potassium, calcium, and magnesium ratios in soil on their uptake and fruit quality of pummelo. J. Agr. Sci. 9, 110–121. doi: 10.5539/jas.v9n12p110
Huang, J., Xu, C. C., Ridoutt, B. G., Wang, X. C., and Ren, P. A. (2017). Nitrogen and phosphorus losses and eutrophication potential associated with fertilizer application to cropland in China. J. Clean. Prod. 159, 171–179. doi: 10.1016/j.jclepro.2017.05.008
Huang, Z., Yin, J., Huang, Y., Su, D., and Wu, L. (2020). Magnesium fertilizer efficiency and its relation to the nutrient absorption and utilization of tea plant in oolong tea producing area. J. South. Agr. 51, 2120-2129. (in Chinese).
IPCC (2019). “N2O emissions from managed soils, and CO2 emssions from lime and urea application,” in 2019 Refinement to the 2006 IPCC Guidelines for National Greenhouse Gas Inventories (Chapter 11), Change, I.P.o.C. (Ed.). Cambridge, New York, NY: Cambridge University Press.
Jat, R., Jat, H. S., Nanwal, R., Yadav, A., Bana, A., Choudhary, K., et al. (2018). Conservation agriculture and precision nutrient management practices in maize-wheat system: Effects on crop and water productivity and economic profitability. Field Crop. Res. 222, 111–120. doi: 10.1016/j.fcr.2018.03.025
Jiang, Y. M., Pen, L. T., and Li, J. R. (2004). Use of citric acid for shelf life and quality maintenance of fresh-cut Chinese water chestnut. J. Food Eng. 63, 325–328. doi: 10.1016/j.jfoodeng.2003.08.004
Jiao, X., Mongol, N., and Zhang, F. (2018). The transformation of agriculture in China: looking back and looking forward. J. Integr. Agr. 17, 755–764. doi: 10.1016/S2095-3119(17)61774-X
Khanali, M., Akram, A., Behzadi, J., Mostashari-Rad, F., Saber, Z., Chau, K. W., et al. (2021). Multi-objective optimization of energy use and environmental emissions for walnut production using imperialist competitive algorithm. Appl. Energ. 284, 116342. doi: 10.1016/j.apenergy.2020.116342
Khoshnevisan, B., Rafiee, S., Omid, M., Yousefi, M., and Movahedi, M. (2013). Modeling of energy consumption and GHG (greenhouse gas) emissions in wheat production in Esfahan province of Iran using artificial neural networks. Energy 52, 333–338. doi: 10.1016/j.energy.2013.01.028
Koondhar, M. A., Li, H., Wang, H., Bold, S., and Kong, R. (2020). Looking back over the past two decades on the nexus between air pollution, energy consumption, and agricultural productivity in China: a qualitative analysis based on the ARDL bounds testing model. Environ. Sci. Pollut. R. 27, 13575–13589. doi: 10.1007/s11356-019-07501-z
Li, Y., Han, M. Q., Lin, F., Ten, Y., Lin, J., Zhu, D. H., et al. (2015). Soil chemical properties, ‘Guanximiyou' pummelo leaf mineral nutrient status and fruit quality in the southern region of Fujian province, China. J. Soil Sci. Plant Nutr. 15, 615–628. doi: 10.4067/S0718-95162015005000029
Li, Y. J., Yang, M., Zhang, Z. Z., Li, W. L., Guo, C. Y., Chen, X. P., et al. (2019). An ecological research on potential for zero-growth of chemical fertilizer use in citrus production in China. Ekoloji. 28, 1049–1059.
Li, J. Z., Wang, D. L., Wang, L. G., Wang, Y. C., and Li, H. (2016). Evaluation of nitrogen and water management on greenhouse gas mitigation in winter wheat-summer maize cropland system in North China. Plant Nutr. Fert. Sci. 22, 921–929. (in Chinese). doi: 10.11674/zwyf.15252
Li, Q., Wang, F., He, C., Lin, C., Zhong, S., and Huang, L. (2016). Investigation and analysis on fertilization status of Pinghe Guanxi pomelo. J. South. Agr. 47, 2059–2064. (in Chinese). doi: 10.3969/j.issn.2095-1191.2016.12.2059
Lin, B., and Fei, R. (2015). Regional differences of CO2 emissions performance in China's agricultural sector: a Malmquist index approach. Eur. J. Agron. 70, 33–40. doi: 10.1016/j.eja.2015.06.009
Liu, D. L., Chan, K. Y., Conyers, M. K., Li, G., and Poile, G. J. (2011). Simulation of soil organic carbon dynamics under different pasture managements using the RothC carbon model. Geoderma 165, 69–77. doi: 10.1016/j.geoderma.2011.07.005
Liu, X., Zhang, Y., Han, W., Tang, A., Shen, J., Cui, Z., et al. (2013). Enhanced nitrogen deposition over China. Nature 494, 459–462. doi: 10.1038/nature11917
Liu, Y., Langer, V., Høgh-Jensen, H., and Egelyng, H. (2010). Life cycle assessment of fossil energy use and greenhouse gas emissions in Chinese pear production. J. Clean. Prod. 18, 1423–1430. doi: 10.1016/j.jclepro.2010.05.025
Lu, M., Liu, D., Shi, Z., Gao, X., Liang, Y., Yao, Z., et al. (2021). Nutritional quality and health risk of pepper fruit as affected by magnesium fertilization. J. Sci. Food and Agr. 101, 582–592. doi: 10.1002/jsfa.10670
Luong, V. T., Amal, R., Scott, J. A., Ehrenberger, S., and Tran, T. (2018). A comparison of carbon footprints of magnesium oxide and magnesium hydroxide produced from conventional processes. J. Clean. Prod. 202, 1035–1044. doi: 10.1016/j.jclepro.2018.08.225
Mengutay, M., Ceylan, Y., Kutman, U. B., and Cakmak, I. (2013). Adequate magnesium nutrition mitigates adverse effects of heat stress on maize and wheat. Plant Soil 368, 57–72. doi: 10.1007/s11104-013-1761-6
Mihov, M., and Tringovska, I. (2010). Energy efficiency improvement of greenhouse tomato production by applying new biofertilizers. Bulg. J. Agric. Sci. 16, 454–458.
Mikkelsen, R. L. (2011). The “4R” nutrient stewardship framework for horticulture. HortTechnology 21, 658–662. doi: 10.21273/HORTTECH.21.6.658
Mobtaker, H. G., Akram, A., and Keyhani, A. (2012). Energy use and sensitivity analysis of energy inputs for alfalfa production in Iran. Energy Sustain. Dev. 16, 84–89. doi: 10.1016/j.esd.2011.10.009
Mobtaker, H. G., Keyhani, A., Mohammadi, A., Rafiee, S., and Akram, A. (2010). Sensitivity analysis of energy inputs for barley production in Hamedan Province of Iran. Agr. Ecosyst. Environ. 137, 367–372. doi: 10.1016/j.agee.2010.03.011
Moradi, M., Nematollahi, M. A., Khaneghah, A. M., Pishgar-Komleh, S. H., and Rajabi, M. R. (2018). Comparison of energy consumption of wheat production in conservation and conventional agriculture using DEA. Environ. Sci. Pollut. R. 25, 35200–35209. doi: 10.1007/s11356-018-3424-x
Nath, D., Ozah, B., Baruah, R., Barooah, R., and Borah, D. (2011). Effect of integrated nutrient management on soil enzymes, microbial biomass carbon and bacterial populations under rice (Oryza sativa)-wheat (Triticum aestivum) sequence. Indian J. Agr. Sci. 81, 1143–1148.
Obreza, T. T., and Morgan, K. T. (2008). Nutrition of Florida Citrus Trees, 2nd Edn. Soil and Water Science Department, Florida Cooperative Extension Service, Institute of Food and Agricultural Sciences, University of Florida, EDIS 2008. Available online at: https://journals.flvc.org/edis/article/view/117225 (accessed July 3, 2021).
Ozkan, B., Akcaoz, H., and Karadeniz, F. (2004). Energy requirement and economic analysis of citrus production in Turkey. Energ. Convers. Manage. 45, 1821–1830. doi: 10.1016/j.enconman.2003.10.002
Paerl, H. W., and Scott, J. T. (2010). Throwing fuel on the fire: synergistic effects of excessive nitrogen inputs and global warming on harmful algal blooms. Environ. Sci. Technol. 44, 7756–7758. doi: 10.1021/es102665e
Qian, X. Y., Shen, G. X., Gu, H. R., Pugliese, M., and Gullino, M. L. (2012). Effects of drip fertigation management on nutrient losses and pear production at Chongming Dongtan in Yangzi River Estuary, China. Adv. Mater. Res. 396–398, 1716–1724. doi: 10.4028/www.scientific.net/AMR.396-398.1716
Römheld, V., and Kirkby, E. A. (2009). Magnesium functions in crop nutrition and yield. Fertil. Fertil. 34, 163–182.
Sarkar, D., Baishya, L. K., Meitei, C. B., Naorem, G. C., Thokchom, R. C., Singh, J., et al. (2018). Can sustainability of maize-mustard cropping system be achieved through balanced nutrient management? Field Crop. Res. 225, 9–21. doi: 10.1016/j.fcr.2018.05.018
Slemrod, J., and Yitzhaki, S. (2001). Integrating expenditure and tax decisions: the marginal cost of funds and the marginal benefit of projects. Nat. Tax. J. 54, 189–201. doi: 10.17310/ntj.2001.2.01
Smith, D. (2014). Soil Survey Staff: Keys to Soil Taxonomy. Washington, DC: Natural Resources Conservation Service.
Steffen, W., Richardson, K., Rockström, J., Cornell, S. E., Fetzer, I., Bennett, E. M., et al. (2015). Planetary boundaries: guiding human development on a changing planet. Science 347, 6223. doi: 10.1126/science.1259855
Tian, X. Y., He, D. D., Bai, S., Zeng, W. Z., Wang, Z., Wang, M., et al. (2021). Physiological and molecular advances in magnesium nutrition of plants. Plant Soil 468. 1–17. doi: 10.1007/s11104-021-05139-w
Unakitan, G., Hurma, H., and Yilmaz, F. (2010). An analysis of energy use efficiency of canola production in Turkey. Energy 35, 3623–3627. doi: 10.1016/j.energy.2010.05.005
Ventura, M., Sorrenti, G., Panzacchi, P., George, E., and Tongon, G. (2013). Biochar reduces short-term nitrate leaching from A horizon in an apple orchard. J. Environ. Qual. 42, 76e82. doi: 10.2134/jeq2012.0250
Verma, G., Mathur, A., Bhandari, S., and Kanthaliya, P. (2010). Long-term effect of integrated nutrient management on properties of a Typic Haplustept under maize-wheat cropping system. J. Indian Soc. Soil Sci. 58, 299–302.
Wallace, T. (1940). Magnesium-deficiency of fruit trees. J. Pomo. Hortic. Sci. 17, 150–166. doi: 10.1080/03683621.1940.11513536
Wan, H. F., Zhao, C. Y., Zhong, J., Ge, Z., Wei, Y. S., Zheng, J. X., et al. (2014). Emission of CH4, N2O and NH3 from vegetable field applied with animal manure composts. Environ. Sci. 35, 892e900. (in Chinese). doi: 10.13227/j.hjkx.2014.03.012
Wang, N., Wolf, J., and Zhang, F. (2016). Towards sustainable intensification of apple production in China–yield gaps and nutrient use efficiency in apple farming systems. J. Integr. Agr. 15, 716–725. doi: 10.1016/S2095-3119(15)61099-1
Wang, S., Wei, S., Liang, H., Zheng, W., Li, X., Hu, C., et al. (2019). Nitrogen stock and leaching rates in a thick vadose zone below areas of long-term nitrogen fertilizer application in the North China Plain: a future groundwater quality threat. J. Hydrol. 576, 28–40. doi: 10.1016/j.jhydrol.2019.06.012
Wang, X., Dou, Z., Shi, X., Zou, C., Liu, D., Wang, Z., et al. (2021). Innovative management programme reduces environmental impacts in Chinese vegetable production. Nat. Food 2, 47–53. doi: 10.1038/s43016-020-00199-0
Wang, X., Zou, C., Zhang, Y., Shi, X., Liu, J., Fan, S., et al. (2018). Environmental impacts of pepper (Capsicum annuum L) production affected by nutrient management: a case study in southwest China. J. Clean. Prod. 171, 934–943. doi: 10.1016/j.jclepro.2017.09.258
Wang, Z., Chen, J., Mao, S., Han, Y., Chen, F., Zhang, L., et al. (2017). Comparison of greenhouse gas emissions of chemical fertilizer types in China's crop production. J. Clean. Prod. 141, 1267–1274. doi: 10.1016/j.jclepro.2016.09.120
Wang, Z., Hassan, M. U., Nadeem, F., Wu, L., Zhang, F., and Li, X. (2020). Magnesium fertilization improves crop yield in most production systems: a meta-analysis. Front. Plant Sci. 10 (1727). doi: 10.3389/fpls.2019.01727
Wei, H., He, C., Zhang, S., Xiong, H., Ni, H., and Li, Q. (2020). Effects of four storage conditions on the sugar content, acidity and flavor of ‘Guanxi'honey pomelo. J. Food Process. Pres. 45, e15088. doi: 10.1111/jfpp.15088
Wu, T., Wang, Y., Yu, C., Chiarawipa, R., Zhang, X., Han, Z., et al. (2012). Carbon sequestration by fruit trees-Chinese apple orchards as an example. PLoS ONE 7, e38883. doi: 10.1371/journal.pone.0038883
Wu, W., and Ma, B. (2015). Integrated nutrient management (INM) for sustaining crop productivity and reducing environmental impact: a review. Sci. Total Environ. 512, 415–427. doi: 10.1016/j.scitotenv.2014.12.101
Xie, B. H., Yu, J. B., Zheng, X. H., Qu, F. Z., Xu, Y., and Lin, H. (2014). N2O emissions from an apple orchard in the coastal area of Bohai Bay, China. Sci. World J. 2014, 164732. doi: 10.1155/2014/164732
Yan, B., and Hou, Y. (2018). Effect of soil magnesium on plants: a review. IOP Conf. Series Earth Environ. Sci. 170, 022168. doi: 10.1088/1755-1315/170/2/022168
Yang, L., Huang, B., Mao, M., Yao, L., Niedermann, S., Hu, W., et al. (2016). Sustainability assessment of greenhouse vegetable farming practices from environmental, economic, and socio-institutional perspectives in China. Environ. Sci. Pollut. R. 23, 17287–17297. doi: 10.1007/s11356-016-6937-1
Yang, X., Gao, W., Zhang, M., Chen, Y., and Sui, P. (2014). Reducing agricultural carbon footprint through diversified crop rotation systems in the North China Plain. J. Clean. Prod. 76, 131–139. doi: 10.1016/j.jclepro.2014.03.063
Yu, C., Xiao, Y., and Ni, S. (2017). Changing patterns of urban-rural nutrient flows in China: driving forces and options. Sci. Bull. 62, 83–91. doi: 10.1016/j.scib.2016.12.006
Zhang, B., Cakmak, I., Feng, J., Yu, C., Chen, X., Xie, D., Wu, L., Song, Z., Cao, J., and He, Y. (2020). Magnesium deficiency reduced the yield and seed germination in wax gourd by affecting the carbohydrate translocation. Front. Plant Sci. 11, 797. doi: 10.3389/fpls.2020.00797
Zhang, D., Shen, J. B., Zhang, F. S., Li, Y. E., and Zhang, W. F. (2017). Carbon footprint of grain production in China. Sci. Rep. 7, 4126. doi: 10.1038/s41598-017-04182-x
Zhang, F., Cui, Z., Chen, X., Ju, X., Shen, J., Chen, Q., et al. (2012). Integrated nutrient management for food security and environmental quality in China. Adv. Agron. 116, 1–40. doi: 10.1016/B978-0-12-394277-7.00001-4
Zhang, M. L., Geng, Y. H., Cao, G. J., Zou, X., Qi, X. Y., and Stephano, M. F. (2021). Effect of magnesium fertilizer combined with straw return on nitrogen use efficiency. Agronomy J. 113, 345–357. doi: 10.1002/agj2.20483
Zhang, Q., Chu, Y., Xue, Y., Ying, H., Chen, X., Zhao, Y., Ma, W., Ma, L., Zhang, J., Yin, Y., and Cui, Z. (2020). Outlook of China's agriculture transforming from smallholder operation to sustainable production. Glob. Food Secur. 26, 100444. doi: 10.1016/j.gfs.2020.100444
Zhang, W. F., Cao, G. X., Li, X. L., Zhang, H. Y., Wang, C., Liu, Q. Q., et al. (2016). Closing yield gaps in China by empowering smallholder farmers. Nature 537, 671–674. doi: 10.1038/nature19368
Zhang, W. F., Dou, Z. X., He, P., Ju, X. T., Powlson, D., Chadwick, D., et al. (2013). New technologies reduce greenhouse gas emissions from nitrogenous fertilizer in China. Proc. Natl. Acad. Sci. U. S. A 110, 8375e8380. doi: 10.1073/pnas.1210447110
Zhang, M. Q., Lin, Q., Yang, J., Peng, J. G., and Yan, M. J. (2003). Balanced fertilization and nutrients condition of Guanxi pomelo garden in Pinghe County. Fujian J. Agri. Sci. 18, 163–167. (in Chinese). doi: 10.19303/j.issn.1008-0384.2003.03.009
Keywords: magnesium fertilizer, yield, energy balance, greenhouse gas emission, economic benefit
Citation: Chen X, Yan X, Muneer MA, Weng X, Cai Y, Ma C, Liu Y, Zhang S, Zhang W, Yang W, Wu L, Zhou S and Zhang F (2022) Pomelo Green Production on Acidic Soil: Reduce Traditional Fertilizers, but Do Not Ignore Magnesium. Front. Sustain. Food Syst. 6:948810. doi: 10.3389/fsufs.2022.948810
Received: 20 May 2022; Accepted: 16 June 2022;
Published: 07 July 2022.
Edited by:
Shaghef Ejaz, Bahauddin Zakariya University, PakistanReviewed by:
Muhammad Qayyum, Bahauddin Zakariya University, PakistanZhao Xiao Hu, Huazhong Agricultural University, China
Copyright © 2022 Chen, Yan, Muneer, Weng, Cai, Ma, Liu, Zhang, Zhang, Yang, Wu, Zhou and Zhang. This is an open-access article distributed under the terms of the Creative Commons Attribution License (CC BY). The use, distribution or reproduction in other forums is permitted, provided the original author(s) and the copyright owner(s) are credited and that the original publication in this journal is cited, in accordance with accepted academic practice. No use, distribution or reproduction is permitted which does not comply with these terms.
*Correspondence: Liangquan Wu, bGlhbmdxdWFuMDEmI3gwMDA0MDsxNjMuY29t
†These authors have contributed equally to this work and share first authorship