- 1School of Environmental Sciences, University of Guelph, Guelph, ON, Canada
- 2Trent School of the Environment, Trent University, Peterborough, ON, Canada
- 3School of Science and Technology, Cape Breton University, Sydney, NS, Canada
Considering the critical importance of insect pollination to food security and documented declines in wild bee populations, it is imperative to develop effective conservation and management strategies that promote the health of wild bee communities associated with agroecosystems. Identifying wild bee visitors of crops, including crop-flower visitors and species that nest within cropping areas, may prove critical to this endeavor as optimal conservation strategies may differ among bee species and/or guilds, regions, and cropping systems. Although lists of bee species that are associated with North American crops are scattered throughout the literature, there is a need for a comprehensive compilation of those species by crop, region, and nesting guild. Here, we searched the literature to compile a list of wild bee species associated with 33 major crops in North America and assessed the overlap in bee species communities among crops and regions. Of the 739 crop-associated bee species retrieved, 405 species (54.8%) were ground nesters, and 438 species (59.3%) were identified as crop-flower visitors of at least one crop. Because of their nesting and foraging behavior, we argue that these species are more likely to be exposed to agricultural pesticide residues than bee species that do not nest in the ground or feed directly on crop flowers. We further compiled lists of wild bees that have been found to be associated with all of the four most surveyed perennial fruit crops (apple, blueberry, cranberry, strawberry) and all of the three most surveyed cucurbit crops (cucumber, Cucurbita, watermelon) in eastern North America. These lists of bee species can be used to focus attention on species needing better protection strategies within agroecosystems, especially for these two important North American crop groups and can also inform the development of multi-species pesticide risk assessment schemes.
Introduction
The importance of bees for crop pollination is well documented (Ricketts et al., 2004; Klein et al., 2007; Hoehn et al., 2008; Garibaldi et al., 2013; Potts et al., 2016), as is the high dependence of food production on wild pollinators (Aizen et al., 2009; Godfray and Garnett, 2014). Global agriculture has expanded considerably over the last decades, with an important increase in the cultivation of highly pollinator-dependent crops (Aizen et al., 2008, 2019). As a result of the increased demand for pollination services, crop pollination deficits are predicted to increase and, in fact, currently exist for many North American crops (Koh et al., 2016; Reilly et al., 2020).
While managed bee species like the western honey bee (Apis mellifera) and some species of mason bees (Osmia spp.), leafcutter bees (Megachile spp.), and bumblebees (Bombus spp.) play a major role in pollinating crops, diverse wild bee assemblages also contribute substantially to crop pollination globally and have been shown to increase yield quantity, quality and stability of pollinator-dependent crops (Hoehn et al., 2008; Winfree et al., 2008; Garibaldi et al., 2013). Indeed, it is now well established that managed bees cannot replace the contributions of diverse wild pollinators to crop pollination (Garibaldi et al., 2013). Moreover, dependence on a few managed bee species for all crop pollination services is highly risky as these species may suffer from devastating parasites and pathogens (e.g., Varroa destructor in honey bees), severe shortages in managed stocks, or may not be well suited to pollination of all crops (e.g., alfalfa; Haedo et al., 2022). As such protecting wild bees that are associated with agriculture and may provide pollination services to crops offers an ecologically sensible insurance against managed bee losses and is closely linked to the sustainability of human food systems (Potts et al., 2016).
There is evidence that conventional agricultural practices (e.g., intensive use of pesticides and high-disturbance agronomic practices such as tillage) are important drivers of documented declines in bee diversity and abundance at different spatial scales (Shuler et al., 2005; Brittain et al., 2010; Tuell and Isaacs, 2010; Park et al., 2015; Woodcock et al., 2016). Indeed, the current conventional crop production paradigm employs chemical pesticides that may be harmful to bees foraging or residing on agricultural lands (Kremen et al., 2002, 2012; Alkassab and Kirchner, 2017; Sponsler et al., 2019). Compared to assessments at the individual or colony level, there has been limited research on pesticide impacts to wild bees at the population or community level. Nonetheless, existing studies have reported negative effects of agricultural pesticide use on wild bee abundance and species richness in grape (Brittain et al., 2010), blueberry (Tuell and Isaacs, 2010), apple (Park et al., 2015), and oilseed rape (Woodcock et al., 2016). Agricultural insecticide and fungicide use can also reduce wild bee floral visitation in pollinator-dependent crops (Rundlöf et al., 2015; Bloom et al., 2021) and has been linked to long-term population changes in wild bees in England (Woodcock et al., 2016). Therefore, it is becoming increasingly critical to sustaining crop pollination services and global food production that the dual (and often conflicting) needs of protecting crops efficiently against pests and diseases and protecting bees from pesticide exposure are prioritized concurrently.
However, before we can develop and implement strategies to protect wild pollinators in North American agroecosystems, it is imperative to identify which wild bee species are living on agricultural lands or are visiting North American crops. Some species of bees associated with agriculture in North America may be of interest because of their widespread importance across crop categories and/or regions and these need to be identified as such. Other bees may need protection on a specific crop because of their importance as pollinators to that crop [e.g., Nomia melanderi on alfalfa, Cane (2008); or Eucera pruinosa on pumpkin/squash, Willis Chan and Raine (2021a)]. Moreover, agricultural intensification and practices may affect different wild bee nesting guilds disparately (Williams et al., 2010; Mallinger et al., 2015). For instance, ground-nesting bees may be more susceptible to soil disturbances (e.g., tillage or prolonged contact exposure to pesticide residues in soil) than those nesting above ground (Main et al., 2020; Willis Chan and Raine, 2021b). Additionally, as different crops have distinct pollinator communities (Garratt et al., 2014; Hutchinson et al., 2021), identifying known flower visitors to individual crops may help target management practices for improved pollination services.
Although lists of bee species that are associated with North American crops are scattered throughout the literature, there is a need for a comprehensive compilation of those species by crop, region, bee traits (e.g., sociality and nesting guilds), and crop flower visitor status. Furthermore, once agriculturally important bee species are identified both across regions or on specific crops, we must begin to reduce the chasms (gap is too small a word) in our understanding of the biology and life history of those agriculturally important wild bee species. Such information is especially needed to inform the development of pesticide risk assessment frameworks that protect all agriculturally important bees (Franklin and Raine, 2019).
Here, we established a comprehensive list of wild bee species associated with agroecosystems in North America north of Mexico (i.e., Canada and the United States), with a view to identifying groups of wild bee species of interest in the agricultural context. We then discuss our findings in terms of potential threats for wild bees from agricultural practices and, particularly, pesticide exposure. Specifically, we aimed to answer the following questions:
1. In which crops and regions of North America north of Mexico have field surveys of wild bees been undertaken?
2. What is the overlap in crop-associated wild bee species among regions, crop categories, and specific crops in North America?
3. Which nesting guilds of wild bees are most commonly found in association with North American crops?
4. Which wild bee species visit crop flowers, per crop type, in North America.
5. What are the knowledge gaps with respect to wild bee visitors of major crops in North America?
In the end, our study aims to promote the study of wild bee species identified as focal and to encourage the development of management actions and strategies to reduce the impact of agricultural practices on local populations.
Materials and methods
Selected crops
Our study aimed to compile a list of bee visitors to the most important agricultural crops (in terms of planted area) in North America north of Mexico (Canada and the United States). Using available data from Statistics Canada (2022a,b,c) and the USDA Farm Service Agency (USDA., 2021), we selected the top 10 bee attractive crops (USDA., 2017) with the greatest planted area in Canada and USA for each of these crop categories: field crops, fruits and nuts, and vegetables. Because of the large number of fruit and nut crops that are highly attractive to bees, an additional three crops (pear, plum, and caneberries) were included in this category (i.e., top 13 crops instead of 10). Greenhouse crops were excluded, as well as vegetable crops that are only attractive to bees when grown for seeds (e.g., carrots, cabbage). Since the focus of this study is the association between bees and agriculture and not crop pollination sensu stricto, we included crops that are known to attract bees independently of their requirements for insect pollination. Based on these criteria, the 33 crops that were included in this study are: alfalfa, beans, canola, corn, cotton, lentils, peas, sorghum, soybean, sunflower, almond, apple, blueberry, caneberries, cherry, cranberry, melon, orange, peach, pear, plum, strawberry, watermelon, avocado, Cucurbita (pumpkin, squash, zucchini), cucumber, eggplant, green and wax beans, green pea, peppers, potato, sweet potato, tomato.
Literature search
We searched the literature to compile a list of all bee visitors to these 33 North American crops. Using Web of Science Core Collection, a literature search was performed on November 11, 2021 to locate existing datasets of bee species observed foraging on or collected in the surroundings of crops from Canadian and American monitoring studies (see Appendix S1 for the complete list of search terms). No date restrictions were applied to the search strategy. This search resulted in 2,834 publications, of which duplicates, articles written in languages other than English, and articles that were not focused on bee monitoring for relevant crops within North America (Canada and USA) were excluded. The remaining publications were screened for the reporting of lists of wild bee species associated with one of the crops listed above. All studies that reported the identity of wild bee species observed or sampled within or adjacent (e.g., crop margins, hedgerows, flower plantings) to agricultural fields were included, while those providing identifications at the genus or morphogroup levels only were excluded. This resulted in 90 publications, to which 14 additional relevant articles sent by colleagues or from our personal collections of papers were added. Our final set of data included 104 articles (Supplementary Table S1).
Data extraction and compilation
We extracted the following information from the 104 studies selected: full bibliographical reference, country and province or state of study site, type of crop, scientific name of bee species recorded, monitoring method (i.e., visual observations, pan or vane traps, nest traps/emergence tents, or netting), and location of observation/sampling relative to the crop (i.e., crop flowers, crop field/orchard, field margin, hedgerow or flower strips, edge of wooded area, on farms). The “on farms” category refers to instances where the location of sampling relative to the crop was not explicitly specified. Flower visitation datasets were used to identify crop flower-visiting bees by crop type. Data compilation was restricted to bees that were identified at the species level, excluding Apis mellifera. Bombus impatiens was included, although it is unknown whether individuals were coming from wild populations or commercial colonies.
The taxonomic status of each bee species compiled was verified using the Integrated Taxonomic Information System (ITIS; www.itis.gov) and Discover Life (Ascher and Pickering, 2020). Invalid scientific names were changed for the valid ones (Supplementary Table S2), while species entries that could only be found from Discover Life but not ITIS were kept as reported in sourced literature. Species that could not be found from either reference databases were excluded (n = 6). Moreover, based on recent changes to the taxonomic classification of the genera Peponapis and Xenoglossa, all species in those genera were renamed as belonging to the genus Eucera (Dorchin et al., 2018), even if the taxonomic status of this species has not yet been updated in reference databases. Information on subspecies was not recorded (i.e., entries have been replaced by species name, without the subspecies information). We did not verify the identification of any of the species reported in studies using collection specimens.
Every bee species listed in our database was characterized by sociality (Bombus spp., social, solitary, variable) and functional nesting guilds (Bombus spp., cavity nester, cavity renter, cleptoparasite, ground nester) based on Grixti and Packer (2006), Sheffield et al. (2013), and Ascher and Pickering (2020). The “variable” social category refers to facultatively eusocial species, i.e., species that are able to express the solitary life strategy and the eusocial one (Mikát et al., 2022). Cavity nesters are defined as species that build their nests themselves in cavities (e.g., pithy stems, wood, tree hollows), whereas cavity renters use pre-existing cavities to nest (e.g., pithy stems, stone walls, snail shells). Finally, we divided the North American agricultural landscape into four regions (Western, Central, Northeastern, Southeastern) based on the availability of survey data and locations of study sites, differences in agricultural practices (Malaj et al., 2020), and similarities in the bee fauna (Supplementary Table S3). The overlap in bee species among crops and regions was assessed using a Venn diagram visualization tool (Oliveros, 2015).
Results
In what crops and regions have field surveys of wild bees taken place?
We compiled data for a total of 739 bee species associated with 23 different crops (Appendix S2; Supplementary Table S4). Our final database also includes survey data from three studies related to crops of interest, but in which the identity of the crops surveyed was not clearly specified: one study from multiple field crops such as corn, soybean, and wheat (Mogren et al., 2016), one study from small diversified vegetable farms (Delphia et al., 2019), and one study from diversified fruit farms (Frankie et al., 2018). Of the 33 crops that were included in our search strategy, no data was found for peas (including green pea), sorghum, lentils, beans (including green and wax beans), orange, peach, sweet potato, and avocado. Overall, blueberry and apple were the most surveyed crops, followed by watermelon, strawberry, sunflower, and cranberry (Figure 1; Supplementary Table S4).
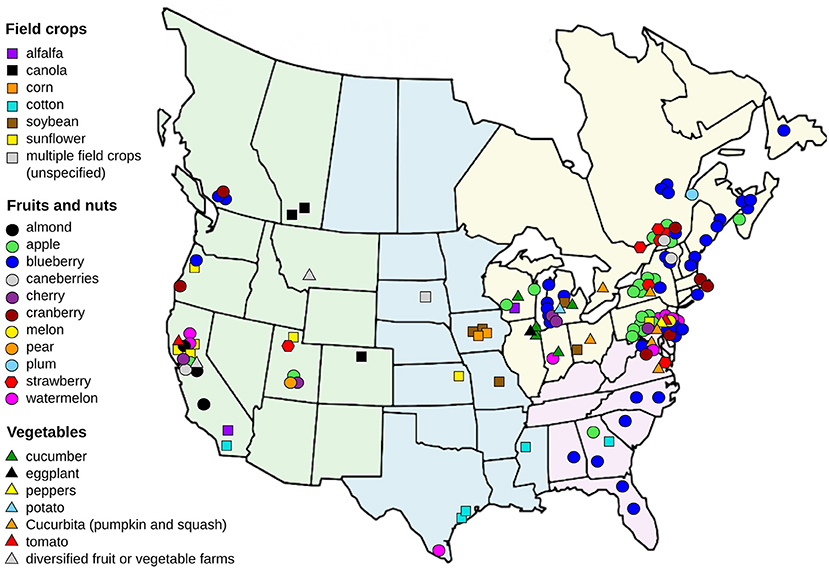
Figure 1. Map of study sites from the 104 studies from which lists of bees have been extracted by crop type. Sites are distributed within four regions: green = western, blue = central, yellow = northeastern, pink = southeastern. Crops were classified into three categories: field crops; fruits and nuts; and vegetables.
Most of the 104 selected studies (81%) were undertaken in the last decade (2010–2021), with <20% conducted before 2010 (Supplementary Figure S1). The geographical location of bee censuses recorded per crop type is presented in Figure 1. Eighty-one percent (n = 84) of the 104 studies containing survey data of bees associated with agriculture were conducted in the United States, while 17% (n = 18) were conducted in Canada, and 2% (n = 2) were conducted in both countries. Most of these studies (70%) were conducted in the eastern part of North America (northeastern and southeastern). Across both countries, 59.6% (n = 62) of studies were carried out in the northeastern region, with 21.2% (n = 22) from the western region, 8.6% (n = 9) from the central region, 8.6% (n = 9) from the southeastern region, and 1.9% (n = 2) from both the northeastern and western regions (Supplementary Table S1). In the southeastern and northeastern regions, studies have focused primarily on fruit and nut crops (Figure 1), which are major crops in these regions. On the other hand, all but one of the crops surveyed in the central region were field crops, and the crops surveyed in the west were more evenly distributed among crop categories. These differences among the types of crops surveyed largely reflect the major crops grown in each region but also highlight knowledge gaps for minor crops grown in each region.
From the 104 studies sourced, we compiled bee species data from 125 datasets reporting bee species by specific crop and location (i.e., some studies provided data from different crops and/or regions). The total number of datasets sourced per crop type and regions are presented in Figure 2 (see also Supplementary Table S3). Most (67%) of these datasets reported bee species surveyed from fruit and nut crops, while 20% were from field crops, and 13% were from vegetable crops. Overall, 40% of all datasets included in this study and 53% of the datasets from the northeastern and southeastern regions were conducted in blueberry or apple systems. Along with strawberry and cranberry, these crops account for 66% of all the datasets compiled for the eastern part of North America.
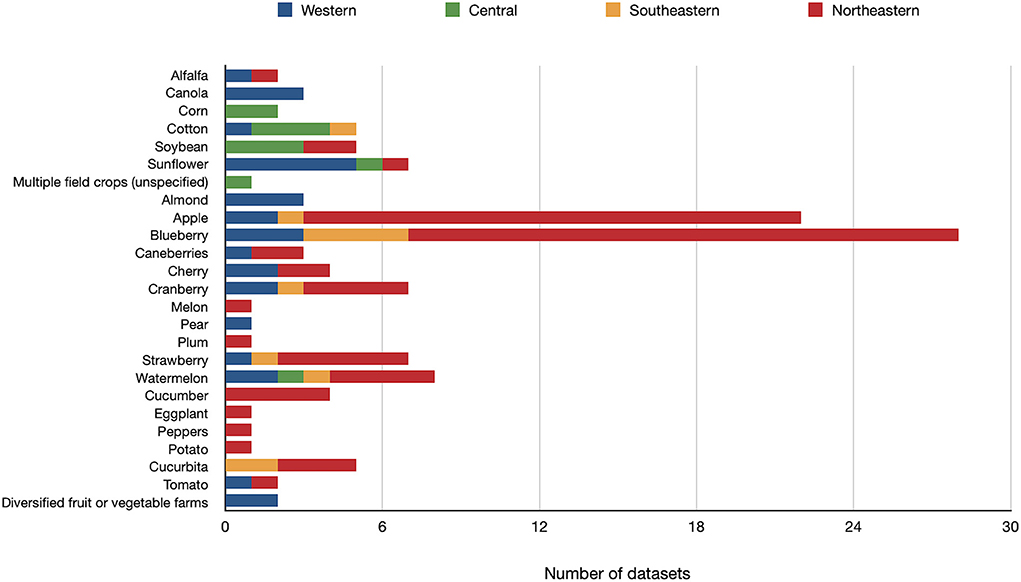
Figure 2. Number of datasets (n = 125) from which lists of bee species have been extracted, representing 25 different crop types. Datasets have been sourced from 104 publications from North America north of Mexico.
What is the overlap in crop-associated wild bee species among regions, crop categories, and specific crops in North America?
We identified a total of 739 species (from 60 genera) of wild bees associated with crops in North America, representing 21.1% of the 3,500 species of bees native to Canada and the USA (The Xerces Society., 2021). Forty-seven (47%; 349/739) and 42 (42%; 314/739) percent of all bee species compiled have been found to be associated with apple and blueberry, respectively (Figure 3). Interestingly, while only two surveys have been conducted in diversified fruit or vegetable farms, these surveys recorded 34% (250/739) of all species listed from the sourced literature (Figure 3).
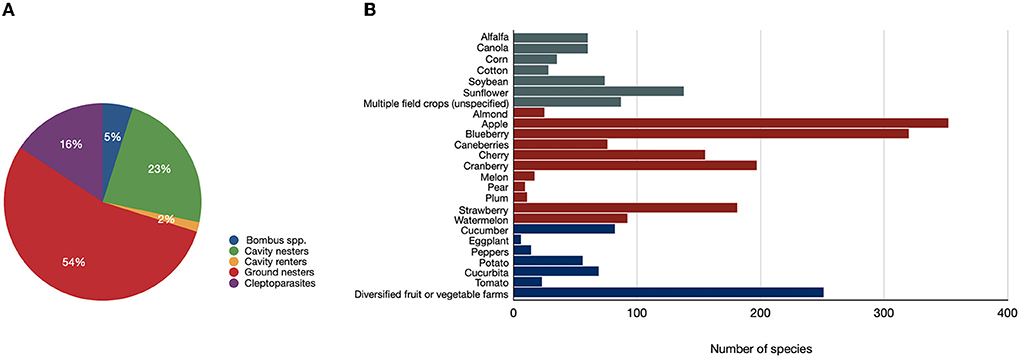
Figure 3. (A) Percentage of wild bee species representing each nesting guild (Bombus spp; cavity nesters; cavity renters; ground nesters; cleptoparasites) for all species (N = 739) compiled from available datasets; (B) Number of wild bee species associated with each crop type (dark green = field crops; dark red = fruit and nuts; dark blue = vegetables).
We compared the identity of crop-associated wild bee species among regions and crops to assess compositional dissimilarity (i.e., shared vs. unique species) among regions, crop categories, and specific crops across North America. There were 23 bee species found in common among all four regions (Figure 4), of which 14 (61%) were ground nesters (Table 1). All adjacent regions share some crop-associated bee species, but all regions also have many unique ones. It should be noted that differences among regions may be an artifact of unequal sampling. Among the 23 bee species shared by all regions, many were common to multiple crops, the most frequently reported in datasets being the bumblebee Bombus impatiens (64%; 80/125 of all datasets), followed by the ground-nesting bee Halictus ligatus (52%; 65/125 of all datasets). Bombus impatiens and H. ligatus were found to be associated with a total of 19 and 21 crops, respectively.
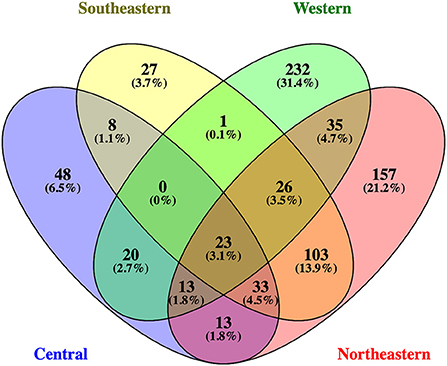
Figure 4. Venn diagram showing the overlap of bee species among North American regions. Numbers in overlapping areas among ellipses represent species found in common between shared regions.
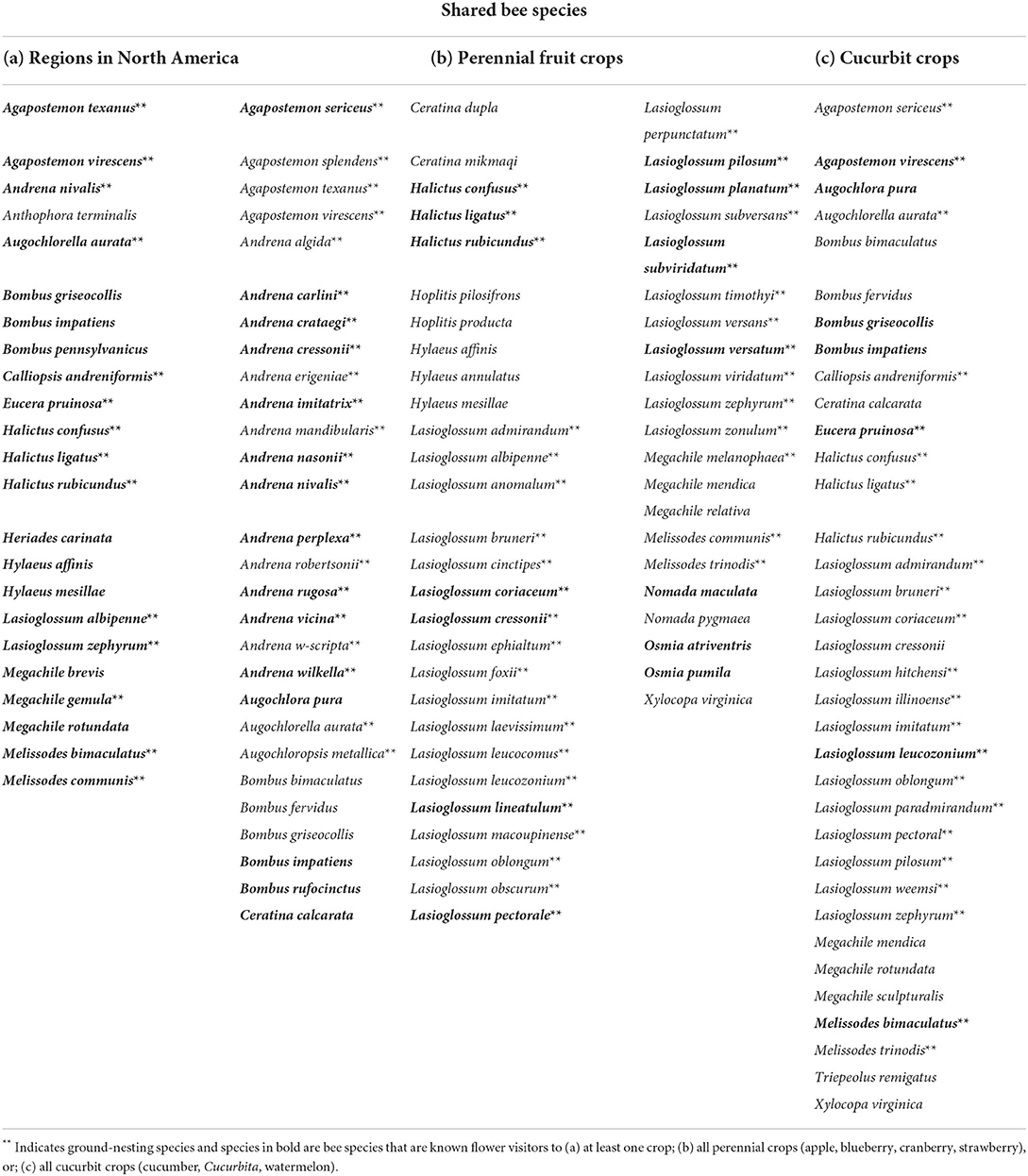
Table 1. List of bee species found in common among (a) all four North American regions (western, central, northeastern, southeastern) (Figure 4); (b) all four perennial fruit crops (apple, blueberry, cranberry, strawberry) in northeastern and southeastern North America (Figure 5A); and (c) all three cucurbit crops (cucumber, Cucurbita, watermelon) in northeastern and southeastern North America (Figure 6A).
As most sourced datasets were from the northeastern and southeastern regions, we also compared wild bee species recorded among some of the most surveyed crops in eastern North America to assess potential overlap among spring-blooming perennial fruit crops (apple, blueberry, cranberry, strawberry) and among cucurbit crops (Cucurbita, cucumber, watermelon). Melon was excluded from the analysis for cucurbits as only one dataset reported bee species for this crop in all North America. For perennial crops, many species were found to be exclusively associated with either apple, blueberry, cranberry, or strawberry, while species overlap was found for all binary combinations of crops (Figure 5A). The largest number of shared bee species was identified for apple and blueberry (n = 226), followed by apple and cranberry (n = 142). A total of 77 species were found to be associated with all four perennial fruit crops, of which 55 (72%) were ground nesters (Figure 5; Table 1). Similar trends were observed for cucurbits, with cucumber having the largest number of unique species (Figure 6A). A total of 35 species (26.9%) were shared among Cucurbita, cucumber, and watermelon, of which 23 (66%) were ground nesters (Figure 6; Table 1).
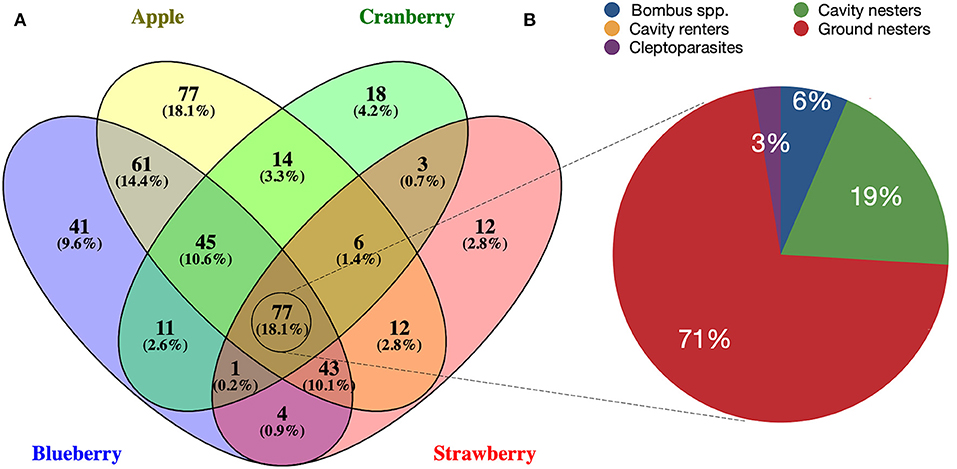
Figure 5. Schematic diagram depicting (A) wild bee species found in common among perennial fruit crops (apple, blueberry, cranberry, strawberry) in northeastern and southeastern North America; (B) percentage of the 77 species common to all four crops representing each functional guild: Bombus spp., cavity nesters, cavity renters, cleptoparasites, ground nesters.
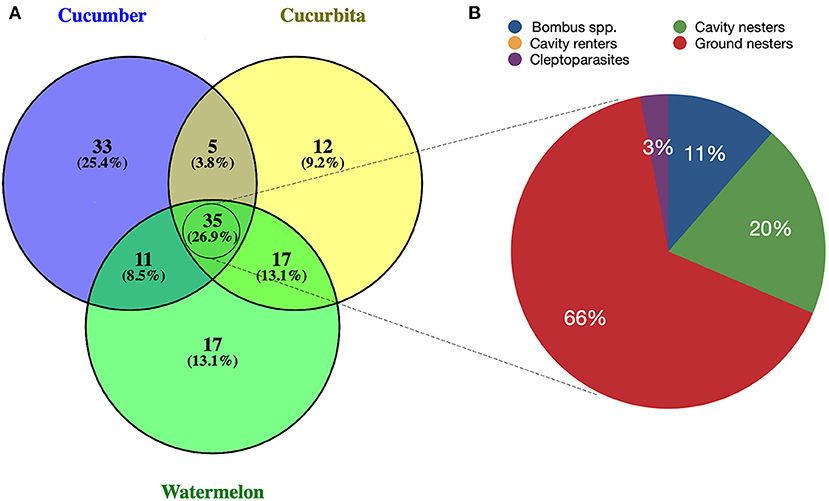
Figure 6. Schematic diagram depicting (A) wild bee species found in common among cucurbit crops (cucumber, Cucurbita, watermelon) in northeastern and southeastern North America; (B) percentage of the 35 species found in common among all three crops representing each functional guild: Bombus spp., cavity nesters, cavity renters, cleptoparasites, ground nesters.
Which wild bee guilds are most commonly found in association with North American crops?
When compared by guilds, ground-nesting bee species (54.8%) were the most common, followed by cavity nesters (22.7%), cleptoparasites (16.5%), Bombus spp. (4.5%), and cavity renters (1.5%) (Figure 3). Although the proportion of wild bee species reported for each guild varied somewhat among crops (Supplementary Figures S2–S4), ground-nesters accounted for the majority of species recorded, averaging 65% of all species for well-studied crops like apple and blueberry. Variability in nesting guild composition among crops may be related to unstandardized sampling methods and variability in the numbers of datasets sourced per crop (e.g., 28 datasets available for blueberry vs. one for pear). Moreover, some studies only reported species of bees that are easier to identify, such as bumblebees, which may be overrepresented for certain crops. Indeed, many of the datasets we included used visual observations or hand netting of bees observed on flowers to identify bee species. The numbers of bee species compiled for each crop are provided in Figure 3.
Which wild bee species visit crop flowers, per crop type, in North America?
Data on known flower visitors were available for 20 crops across the four regions (Supplementary Table S5). In total, 438 species from 51 genera have been observed foraging on flowers of at least one crop, representing 59% of the total number of species compiled. This suggests that about 40% of wild bee species may coexist in the same space as crops without visiting their flowers, although this percentage may be inflated by the relative difficulty of sampling and identifying bees to species through visual observations or hand netting of individuals observed on flowers compared to passive sampling. Most crop flower visitors were Lasioglossum, Andrena, and Bombus species, with some variation among crops (Supplementary Figure S5). In the northeastern region, flower visitors of apple and blueberry were the most studied, while data for the other crops and regions were more scattered (Supplementary Table S5).
Interestingly, records of flower visitors for watermelon were available across all four regions, allowing for regional comparisons (Supplementary Figure S6). While 60 species of watermelon flower visitors were shared between the northeastern and southeastern regions, only a few species from the central and western regions were shared with any other regions. However, it is important to mention that very few species were identified for these two regions. One bee species, Halictus ligatus, was found to visit watermelon flowers across all four regions. Indeed, across North America, H. ligatus has been observed visiting the flowers of 14 crops, including field crops (alfalfa, cotton, soybean, sunflower), fruits (apple, blueberry, caneberries, cranberry, melon, strawberry, watermelon), and vegetables (Cucurbita, peppers, tomato).
Across North America, the largest number of known flower-visiting bees have been identified for blueberry (n = 131), strawberry (n = 82), apple (n = 83), cherry (n = 68), watermelon (n = 62), and cranberry (n = 61). Of all crop flower-visiting bees identified, 256 (58%) were ground nesters. The likelihood of exposure to pesticide residues from both contaminated nectar and pollen and soil is amplified for these species. The complete list of flower-visiting bee species per crop and region is available in Appendix S2.
Discussion
Wild bee visitors of crops and potential threats from agricultural practices
Our study is the first to inventory the entire wild bee species community associated with major crops across Canada and the USA and to assess the overlap in bee species across North American regions and crops. Our analysis of crop-associated wild bee species may enable North American conservation practitioners and risk assessors to better focus their attention on the relatively narrow number of wild bee species (i.e., <25% of wild bee species in North America) associated with agricultural systems when developing strategies and management actions to reduce the impact of agricultural practices on local populations. Below, we discuss how some groups of wild bees may be impacted by agricultural practices particularly, pesticide exposure.
For wild bees that visit crop flowers, living within agroecosystems may bring rewards in the form of extensive access to nectar- and pollen-producing plants (Westphal et al., 2009; Holzschuh et al., 2013). However, such association may also present threats, such as acute or chronic exposure to pesticide residues through the crops themselves or wildflowers growing on crop edges (Botías et al., 2015; David et al., 2016; Main et al., 2020). For crop flower visitors, the overlap of foraging activity with crop pesticide application may determine the extent of exposure (Sponsler et al., 2019). Bees foraging on the flowers of crops treated with systemic pesticides may also be exposed to residues in pollen and nectar many weeks after treatment (Krupke et al., 2012; David et al., 2016; Azpiazu et al., 2019; Willis Chan et al., 2019).
In addition to crop flower-visiting species, exposure to agricultural pesticides may also be higher for ground-nesting bees than for other nesting guilds because females may build their nests within treated cropping areas and may be exposed to pesticide residues in soil as they excavate their nests (Willis Chan et al., 2019; Willis Chan and Raine, 2021b; Rondeau and Raine, 2022). Furthermore, both male and female ground-nesting bees spend most of their life cycle within underground nest cells (Harmon-Threatt, 2020; Antoine and Forrest, 2021) and may be exposed to soil residues at the larval or pupal stages of development (Sgolastra et al., 2019; Willis Chan et al., 2019). Underground bee nests may also be destroyed by agricultural machinery during tillage (Shuler et al., 2005). As such, both crop flower-visiting species and ground-nesting bees deserve special attention when developing strategies to limit the impact of agricultural practices for bees associated with agriculture.
In an attempt to provide a starting point for discussions on the development and implementation of wider protection and risk assessment schemes for wild bees, we compiled lists of crop flower-visiting bee species that have been found to be associated with all of the four most surveyed perennial fruit crops and all of the three most surveyed cucurbit crops in eastern North America. These crop-flower visitors have been identified from observational studies. However, it is important to note that, although these crop flower-visiting species are potentially contributing to crop pollination, we did not assess their actual contribution to pollination services. Additionally, we identified which of these crop flower-visiting bees also nest underground. By identifying these groups of bee species, we have built the basis for targeted protection strategies for a defined number of wild bee species associated with two important North American crop groups.
Of all the wild bee species found to be associated with major crops in North America, 23 species were common among all four regions, including 14 ground-nesters. Most of these species are common visitors to a wide range of host plants and are widespread throughout Canada and the United States. One exception is Eucera pruinosa (formerly Peponapis pruinosa), a pollen specialist on the genus Cucurbita whose geographical range expansion throughout North America has been facilitated by the domestication of pumpkins and squash (López-Uribe et al., 2016). All 14 species of ground-nesting bees shared among regions have been found to be associated with blueberry, apple, and an average of 12 other crops and to visit the flowers of at least some of these crops (range = 1–14 crops; mean = 8 crops; Appendix S2). Given their wide distribution and close association with agriculture, management for these species across North America should be considered as a potential first step toward conservation on agricultural lands.
Seventy-seven wild bee species were found in common among perennial fruit crops (apple, blueberry, cranberry, and strawberry) in eastern North America, of which 56 are ground-nesters and 28 were identified as flower visitors of all four crops. These spring bees are likely to experience exceptionally high pesticide exposure because of the severe soil contamination encountered in perennial crops (Silva et al., 2019; Tang and Maggi, 2021) and the overlap of pesticide application on spring-blooming perennial fruit crops with bees' foraging period. Recent evidence shows that exposure of ground-nesting bees to pesticide residues in soil varies among cropping systems (Rondeau et al., 2022), with soils from perennial crops containing the highest pesticide concentrations and number of detectable pesticides compared to vegetables, field crops, cereals, root crops, and diversified farms (Silva et al., 2019; Tang and Maggi, 2021). For instance, mixtures of up to 29 different pesticides have been found in orchard soils collected at bumblebee hibernation sites in Ontario, Canada, flagging apple orchards as risky environments for bees that nest or hibernate underground (Rondeau et al., 2022). High contamination levels in perennial crop soils can be explained by the intensive use of pesticides and absence of crop rotation or tillage in these systems. Another major concern is the potential direct exposure of springtime forager bees to early-season pesticide sprays. Indeed, many pest insects and fungal diseases of perennial fruit crops coincide with bloom, and pesticides (mostly fungicides) are commonly sprayed when these crops are flowering (Gradish et al., 2012; Melathopoulos et al., 2014; Fulcher et al., 2015; Mallinger et al., 2015; McPhie and Burrack, 2016; Graham et al., 2021). This represents an additional hazard for bees foraging on these crops.
Thirty-five species of wild bees were found in common among cucurbit crops (cucumber, Cucurbita, watermelon) in eastern North America, of which 23 are ground-nesters and seven were identified as flower visitors of all three crops. For these bees, the likelihood of exposure to residues of systemic pesticides used as seed coatings or applied to soil via drenches is high (Willis Chan et al., 2019; Willis Chan and Raine, 2021a). In addition to accumulating in soil, residues of systemic pesticides can also translocate in pollen and nectar of Cucurbita flowers (Stoner and Eitzer, 2012). Many of these systemic compounds are highly toxic insecticides like neonicotinoids, which can have detrimental effects on the pollen harvesting, nesting behavior and reproduction of ground-nesting bees when applied to cucurbit crops via soil (Willis Chan and Raine, 2021b). Moreover, cucurbit crops have an extended flowering period that often coincides with the appearance of fungal diseases, which can lead to frequent foliar fungicide applications during bloom (Azpiazu et al., 2019). Because of concerns about fungicide resistance, rotation of fungicides with different modes of action is encouraged (Wyenandt et al., 2017), increasing the risk of exposure to pesticide mixtures for bees (Rondeau and Raine, 2022). Finally, of all flower visitors of cucurbit crops, bee species with smaller foraging breadth like E. pruinosa and Melissodes bimaculatus may be more vulnerable to pesticides applied on cucumber, Cucurbita, and watermelon. Although it is a pollen specialist, E. pruinosa has also been found foraging on alfalfa and sunflower crops (Hurd and Linsley, 1964). In both bee species, adults are active in late summer and fall, when their preferred plants, including cucurbits, are in bloom, resulting in a temporal overlap between foraging activity and fungicide use on these crops.
Lasioglossum species were the most prevalent species found in common for both perennial fruits crops and cucurbit crops, although few of them were identified as shared crop flower visitors. Instead, more than half of the crop flower visitors identified in common for perennial crops were Andrena species, which tend to emerge earlier in the spring and have shorter foraging periods than Lasioglossum (Michener, 2000). While these Andrena species may be more at risk of exposure to pesticides sprayed during early-season bloom (Brittain and Potts, 2011), Lasioglossum are more likely to be exposed to season-long pesticide applications (Mallinger et al., 2015). And while both groups may be exposed to residues in soils during nest construction and development, many Lasioglossum are smaller and have shorter foraging ranges than Andrena (Michener, 2000; Gathmann and Tscharntke, 2002; Greenleaf et al., 2007; Mallinger et al., 2015), meaning that their nests are more likely to be located near or within the cropping area they forage on. For social Halictus and Lasioglossum species, sensitivity to pesticides may also be increased if they are applied early in the season when queens are active and colonies are still at an early stage of formation (Williams et al., 2010; Brittain and Potts, 2011; Mallinger et al., 2015). Indeed, there is evidence that some groups of bees, namely small bodied Lasioglossum, may be more impacted by pesticide exposure in orchard systems than other bees for the reasons mentioned above (Mallinger et al., 2015). Although not ground nesters per se, it is worth noting that many species of bumblebees (Bombus spp.) were also found in common among crops in both crop groups. Bumblebees are important pollinators of both perennial fruit crops and cucurbit crops (Javorek et al., 2002; Artz and Nault, 2011; Nayak et al., 2020; Willis Chan and Raine, 2021a) and their close association with agriculture deserves attention. Indeed, in addition to their potential oral exposure to diverse pesticides throughout the growing season, bumblebees may also be exposed to soil residues in underground nest or during hibernation (Rondeau et al., 2022).
Knowledge gaps, constraints, and limitations
The geographical distribution of published datasets of bee species associated with agricultural crops in North America is skewed toward the northeastern region, with a lack of published data from Canada compared to the United States. Moreover, most of the existing literature has investigated bee communities in highly pollinator-dependent fruit crops, with a complete lack of data for many bee-attractive crops that do not require insect pollination (e.g., orange, green and wax beans, peas). This is problematic from a conservation perspective as virtually any crop that attracts pollinators can lead to pesticide exposure for bees, with important variability in pesticide use among crops and potential for overlap between bee phenology and timing of pesticide application. Surveying bees in these crops should be a focus of future research. Moreover, we know little about the biology of many of the bees identified in the present study, highlighting the need for descriptive natural history studies for these species. Information about the life cycle and habitat requirements of these wild bee species is particularly needed before elements of the landscape that might be critical to their persistence can be integrated more fully into the development of proper management and/or conservation strategies. Our study provides a starting point to address these knowledge gaps by identifying the cropping systems and geographic regions that require research investment and subsets of agriculturally important bee species that require protection.
In this study, we have focused on bee species found in common among multiple crops sharing similar management practices and pesticide use patterns. However, it is important to also acknowledge the large number of bee species that are strictly associated with individual crops and/or regions. For instance, focusing on species that are common to multiple crops may not be protective to many specialist and rare bee species. Protecting a larger diversity of species from exposure to agricultural pesticides may be especially important in a context of high species turnover (Winfree et al., 2018). The choice of targeting one group over the other also depends on the protection goals. While it may be sensible to start with shared species among crops when developing strategies to protect ground-nesting bees on farmlands overall, developing a regional or crop by crop approach may be more appropriate in other cases. By listing all the wild bee species associated with the most important agricultural crops in North America by nesting guild, crop flower visitor status, crop type, and region, Appendix S2 can be used as a tool to determine the species that need protection from potentially harmful agricultural practices according to different targeted goals.
We acknowledge the limitations of the approach we used to identify the wild bee species reported in this study. For instance, despite our efforts to identify all pertinent literature, some relevant studies may not have been included in our compiled list of crop-associated bee species. While we thoroughly searched all peer-reviewed studies available through Web of Science, we identified 14 additional relevant studies that have not been captured through this search. We recognize that not all journals meet the requirements for inclusion in any single database and that there might be delays between publication and the availability of published work in the database. However, overall, we are confident that most of the relevant literature has been identified and accurately summarized in this work. It is also important to mention the unstandardized nature of the sampling methods used in studies from which we extracted lists of bee species. Across studies, larger bees from the genera Bombus and Andrena were more often identified to species, while smaller inconspicuous species, especially those of the genus Lasioglossum, were often only identified at the genus level and hence, not included in our database. This might explain the overall low number of Lasioglossum species reported as crop flower visitors from the literature, as crop flower visitors were typically identified through visual observations or hand netting of bees from flowers and most Lasioglossum species included in our database were collected using pan traps.
Conclusions and conservation implications
In this study, we have identified and narrowed down the number of wild bee species associated with agriculture in North America to a fraction (<25%) of the total number of bee species in this geography. As our dependency on insect pollinators to produce food increases (Aizen et al., 2008, 2019), so does our reliance upon this fraction of wild bees. As such, it is important to reflect on the traits (e.g., sociality, nesting guild, or whether or not those bee species forage on crops) that may contribute to their vulnerability to management practices used on the crops they are associated with.
Moreover, pesticide use varies significantly by crop and region, leading to disparate exposure for bees (Sponsler et al., 2019). As such, assessing the overlap in bee species among crops with similar agricultural practices and pesticide use patterns, as we have done, can refine the development of pesticide risk management schemes that are based on the actual crop and bee species involved. We understand that it may be necessary to use a few model species to represent these bees in pesticide risk assessments and our study may help identifying species of interest for that purpose. For example, the hoary squash bee (E. pruinosa) has recently been suggested as a model species for pesticide risk assessments (Willis Chan and Raine, 2021b). The fact that this species was found across all North American regions and foraging on all cucurbit crops emphasizes the relevance of using E. pruinosa as a surrogate species for solitary ground-nesting bees, although more work may be needed to compensate for size differences between larger bees such as E. pruinosa and smaller bees such as those of the genus Lasioglossum.
Data availability statement
The complete dataset generated and analyzed through the current study is publicly available. This data can be found here: https://doi.org/10.6084/m9.figshare.21350316.
Author contributions
Conceptualization, methodology, data curation, and writing–review and editing: SR, DW, and AP. Formal analysis: AP, with assistance from SR and DW. Funding acquisition: AP. Visualization: SR and AP. Writing–original draft: SR and DW. All authors contributed to the article and approved the submitted version.
Funding
SR is supported by graduate scholarships from the Arrell Food Institute at the University of Guelph, the Fonds de recherche du Québec–Nature et technologies (FRQNT) and the Ontario Agricultural College. DW is supported by George Weston Ltd. as a post-doctoral fellow at the University of Guelph. AP is supported as the Weston Family Visiting Professor of Ecosystem Health and Food Security by the Weston Family Foundation.
Acknowledgments
Thanks are due to Sydney Fritz, who provided invaluable help at the early stages of data collection.
Conflict of interest
The authors declare that the research was conducted in the absence of any commercial or financial relationships that could be construed as a potential conflict of interest.
Publisher's note
All claims expressed in this article are solely those of the authors and do not necessarily represent those of their affiliated organizations, or those of the publisher, the editors and the reviewers. Any product that may be evaluated in this article, or claim that may be made by its manufacturer, is not guaranteed or endorsed by the publisher.
Supplementary material
The Supplementary Material for this article can be found online at: https://www.frontiersin.org/articles/10.3389/fsufs.2022.943237/full#supplementary-material
References
Aizen, M. A., Aguiar, S., Biesmeijer, J. C., Garibaldi, L. A., Inouye, D. W., Jung, C. L., et al. (2019). Global agricultural productivity is threatened by increasing pollinator dependence without a parallel increase in crop diversification. Glob. Chang. Biol. 25, 3516–3527. doi: 10.1111/gcb.14736
Aizen, M. A., Garibaldi, L. A., Cunningham, S. A., and Klein, A. M. (2008). Long-term global trends in crop yield and production reveal no current pollination shortage but increasing pollinator dependency. Curr. Biol. 18, 1572–1575. doi: 10.1016/j.cub.2008.08.066
Aizen, M. A., Garibaldi, L. A., Cunningham, S. A., and Klein, A. M. (2009). How much does agriculture depend on pollinators? Lessons from long-term trends in crop production. Ann. Bot. 103, 1579–1588. doi: 10.1093/aob/mcp076
Alkassab, A. T., and Kirchner, W. H. (2017). Sublethal exposure to neonicotinoids and related side effects on insect pollinators: honeybees, bumblebees, and solitary bees. J. Plant Dis. Prot. 124, 1–30. doi: 10.1007/s41348-016-0041-0
Antoine, C. M., and Forrest, J. R. K. (2021). Nesting habitat of ground-nesting bees: a review. Ecol. Entomol. 46, 143–159. doi: 10.1111/een.12986
Artz, D. R., and Nault, B. A. (2011). Performance of Apis mellifera, Bombus impatiens, and Peponapis pruinosa (hymenoptera: apidae) as pollinators of pumpkin. J. Econ. Entomol. 104, 1153–1161. doi: 10.1603/EC10431
Ascher, J., and Pickering, J. (2020). “Discover life bee species guide and world checklist (Hymenoptera: Apoidea: Anthophila)”. Available online at: http://www.discoverlife.org/mp/20q?guide=Apoidea_species
Azpiazu, C., Bosch, J., Vinuela, E., Medrzycki, P., Teper, D., and Sgolastra, F. (2019). Chronic oral exposure to field-realistic pesticide combinations via pollen and nectar: effects on feeding and thermal performance in a solitary bee. Sci. Rep. 9, 13770. doi: 10.1038/s41598-019-50255-4
Bloom, E. H., Wood, T. J., Hung, K. -L. J., Ternest, J. J., Ingwell, L. L., Goodell, K., et al. (2021). Synergism between local- and landscape-level pesticides reduces wild bee floral visitation in pollinator-dependent crops. J. Appl. Ecol. 58, 1187–1198. doi: 10.1111/1365-2664.13871
Botías, C., David, a., Horwood, J., Abdul-Sada, A., Nicholls, E., Hill, E., and Goulson, D. (2015). Neonicotinoid residues in wildflowers, a potential route of chronic exposure for bees. Environ. Sci. Technol. 49, 12731–12740. doi: 10.1021/acs.est.5b03459
Brittain, C., and Potts, S. G. (2011). The potential impacts of insecticides on the life-history traits of bees and the consequences for pollination. Basic Appl. Ecol. 12, 321–331. doi: 10.1016/j.baae.2010.12.004
Brittain, C. A., Vighi, M., Bommarco, R., Settele, J., and Potts, S. G. (2010). Impacts of a pesticide on pollinator species richness at different spatial scales. Basic Appl. Ecol. 11, 106–115. doi: 10.1016/j.baae.2009.11.007
Cane, J. H. (2008). A native ground-nesting bee (Nomia melanderi) sustainably managed to pollinate alfalfa across an intensively agricultural landscape. Apidologie. 39, 315–323. doi: 10.1051/apido:2008013
David, A., Botias, C., Abdul-Sada, A., Nicholls, E., Rotheray, E. L., Hill, E. M., et al. (2016). Widespread contamination of wildflower and bee-collected pollen with complex mixtures of neonicotinoids and fungicides commonly applied to crops. Environ. Int. 88, 169–178. doi: 10.1016/j.envint.2015.12.011
Delphia, C. M., Griswold, T., Reese, E. G., O'Neill, K. M., and Burkle, L. A. (2019). Checklist of bees (Hymenoptera: Apoidea) from small diversified vegetable farms in south-western Montana. Biodivers. Data J. 7. doi: 10.3897/BDJ.7.e30062.figure1
Dorchin, A., López-Uribe, M. M., Praz, C. J., Griswold, T., and Danforth, B. N. (2018). Phylogeny, new generic-level classification, and historical biogeography of the Eucera complex (Hymenoptera: Apidae). Mol. Phylogenet. Evol. 119, 81–92. doi: 10.1016/j.ympev.2017.10.007
Frankie, G. W., Pawelek, J. C., Guerrero, S. S. L., Thorp, R. W., Rizzardi, M. A., Chase, M. H., et al. (2018). Survey of native and honey bees from agricultural brentwood and their constructed bee gardens in Northern California, 2010–2018. J. Kans. Entomol. Soc. 91, 310–349. doi: 10.2317/0022-8567-91.4.310
Franklin, E. L., and Raine, N. E. (2019). Moving beyond honeybee-centric pesticide risk assessments to protect all pollinators. Nat. Ecol. Evolut. 3, 1373–1375. doi: 10.1038/s41559-019-0987-y
Fulcher, A., Gauthier, N. W., Klingeman, W. E., Hale, F., and White, S. A. (2015). Blueberry culture and pest, disease, and abiotic disorder management during nursery production in the southeastern U.S.: a review. J. Environ. Hortic. 33, 33–47. doi: 10.24266/0738-2898-33.1.33
Garibaldi, L. A., Steffan-Dewenter, I., Winfree, R., Aizen, M. A., Bommarco, R., Cunningham, S. A., et al. (2013). Wild pollinators enhance fruit set of crops regardless of honey bee abundance. Science. 339, 1608. doi: 10.1126/science.1230200
Garratt, M. P. D., Coston, D. J., Truslove, C. L., Lappage, M. G., Polce, C., Dean, R., et al. (2014). The identity of crop pollinators helps target conservation for improved ecosystem services. Biol. Conserv. 169, 128–135. doi: 10.1016/j.biocon.2013.11.001
Gathmann, A., and Tscharntke, T. (2002). Foraging ranges of solitary bees. J. Anim. Ecol. 71, 757–764. doi: 10.1046/j.1365-2656.2002.00641.x
Godfray, H. C. J., and Garnett, T. (2014). Food security and sustainable intensification. Philos. Trans. R. Soc. Lond. B Biol. Sci. 369, 20120273. doi: 10.1098/rstb.2012.0273
Gradish, A. E., Scott-Dupree, C. D., Frewin, A. J., and Cutler, G. C. (2012). Lethal and sublethal effects of some insecticides recommended for wild blueberry on the pollinator Bombus impatiens. Can. Entomol. 144, 478–486. doi: 10.4039/tce.2012.40
Graham, K. K., Milbrath, M. O., Zhang, Y., Soehnlen, A., Baert, N., McArt, S., et al. (2021). Identities, concentrations, and sources of pesticide exposure in pollen collected by managed bees during blueberry pollination. Sci. Rep. 11, 16857. doi: 10.1038/s41598-021-96249-z
Greenleaf, S. S., Williams, N. M., Winfree, R., and Kremen, C. (2007). Bee foraging ranges and their relationship to body size. Oecologia. 153, 589–596. doi: 10.1007/s00442-007-0752-9
Grixti, J. C., and Packer, L. (2006). Changes in the bee fauna (Hymenoptera: Apoidea) of an old field site in southern Ontario, revisited after 34 years. Can. Entomol. 138, 147–164. doi: 10.4039/n05-034
Haedo, J. P., Martínez, L. C., Graffigna, S., Marrero, H. J., and Torretta, J. P. (2022). Managed and wild bees contribute to alfalfa (Medicago sativa) pollination. Agric. Ecosyst. Environ. 324, 107711. doi: 10.1016/j.agee.2021.107711
Harmon-Threatt, A. (2020). Influence of nesting characteristics on health of wild bee communities. Annu. Rev. Entomol. 65, 39–56. doi: 10.1146/annurev-ento-011019-024955
Hoehn, P., Tscharntke, T., Tylianakis, J. M., and Steffan-Dewenter, I. (2008). Functional group diversity of bee pollinators increases crop yield. Proc. R. Soc. B: Biol. Sci. 275, 2283–2291. doi: 10.1098/rspb.2008.0405
Holzschuh, A., Dormann, C. F., Tscharntke, T., and Steffan-Dewenter, I. (2013). Mass-flowering crops enhance wild bee abundance. Oecologia. 172, 477–484. doi: 10.1007/s00442-012-2515-5
Hurd, P., and Linsley, E. (1964). The squash and gourd bees—genera peponapis robertson and xenoglossa smith—inhabiting America north of Mexico (Hymenoptera: Apoidea). Hilgardia. 35, 375–477. doi: 10.3733/hilg.v35n15p375
Hutchinson, L. A., Oliver, T. H., Breeze, T. D., Bailes, E. J., Brunjes, L., Campbell, A. J., et al. (2021). Using ecological and field survey data to establish a national list of the wild bee pollinators of crops. Agric. Ecosyst. Environ. 315, 107447. doi: 10.1016/j.agee.2021.107447
Javorek, S., Mackenzie, K., and Vander Kloet, S. (2002). Comparative pollination effectiveness among bees (Hymenoptera: Apoidea) on lowbush blueberry (Ericaceae: Vaccinium angustifolium). Ann. Entomol. Soc. Am. 95, 345–351. doi: 10.1603/0013-8746(2002)095[0345:CPEABH]2.0.CO;2
Klein, A. M., Vaissiere, B. E., Cane, J. H., Steffan-Dewenter, I., Cunningham, S. A., Kremen, C., et al. (2007). Importance of pollinators in changing landscapes for world crops. Proc. R. Soc. Lond. B. Biol. Sci. 274, 303–313. doi: 10.1098/rspb.2006.3721
Koh, I., Lonsdorf, E. V., Williams, N. M., Brittain, C., Isaacs, R., Gibbs, J., et al. (2016). Modeling the status, trends, and impacts of wild bee abundance in the United States. Proc. Natl. Acad. Sci. U.S.A. 113, 140–145. doi: 10.1073/pnas.1517685113
Kremen, C., Iles, A., and Bacon, C. (2012). Diversified farming systems: an agroecological, systems-based alternative to modern industrial agriculture. Ecol. Soc. 17, 44. doi: 10.5751/ES-05103-170444
Kremen, C., Williams, N. M., and Thorp, R. W. (2002). Crop pollination from native bees at risk from agricultural intensification. Proc. Natl. Acad. Sci. U.S.A. 99, 16812–16816. doi: 10.1073/pnas.262413599
Krupke, C. H., Hunt, G. J., Eitzer, B. D., Andino, G., and Given, K. (2012). Multiple routes of pesticide exposure for honey bees living near agricultural fields. PLoS ONE. 7, e29268. doi: 10.1371/journal.pone.0029268
López-Uribe, M. M., Cane, J. H., Minckley, R. L., and Danforth, B. N. (2016). Crop domestication facilitated rapid geographical expansion of a specialist pollinator, the squash bee Peponapis pruinosa. Proc. R. Soc. Lond. B. Biol. Sci. 283, 20160443. doi: 10.1098/rspb.2016.0443
Main, A. R., Webb, E. B., Goyne, K. W., and Mengel, D. (2020a). Reduced species richness of native bees in field margins associated with neonicotinoid concentrations in non-target soils. Agric. Ecosyst. Environ. 287, 106693. doi: 10.1016/j.agee.2019.106693
Malaj, E., Freistadt, L., and Morrissey, C. A. (2020). Spatio-temporal patterns of crops and agrochemicals in canada over 35 years. Front. Environ. Sci. 8, 556452. doi: 10.3389/fenvs.2020.556452
Mallinger, R. E., Werts, P., and Gratton, C. (2015). Pesticide use within a pollinator-dependent crop has negative effects on the abundance and species richness of sweat bees, Lasioglossum spp., and on bumble bee colony growth. J. Insect Conserv. 19, 999–1010. doi: 10.1007/s10841-015-9816-z
McPhie, D., and Burrack, H. J. (2016). Effects of microbial, organically acceptable, and reduced risk insecticides on Anthonomus signatus (Curculionidae: Coleoptera) in strawberries (Fragaria x ananassa). Crop Protection. 89, 255–258. doi: 10.1016/j.cropro.2016.07.034
Melathopoulos, A. P., Tyedmers, P., and Cutler, G. C. (2014). Contextualising pollination benefits: effect of insecticide and fungicide use on fruit set and weight from bee pollination in lowbush blueberry. Ann. Appl. Biol. 165, 387–394. doi: 10.1111/aab.12143
Mikát, M., Franková, T., Benda, D., and Straka, J. (2022). Evidence of sociality in European small Carpenter bees (Ceratina). Apidologie. 53, 18. doi: 10.1007/s13592-022-00931-8
Mogren, C. L., Rand, T. A., Fausti, S. W., and Lundgren, J. G. (2016). The effects of crop intensification on the diversity of native pollinator communities. Environ. Entomol. 45, 865–872. doi: 10.1093/ee/nvw066
Nayak, R. K., Rana, K., Bairwa, V. K., Singh, P., and Bharthi, V. D. (2020). A review on role of bumblebee pollination in fruits and vegetables. Phytopathology. 9, 1328–1334. doi: 10.22271/phyto.2020.v9.i3v.11494
Oliveros, J. C. (2015). “Venny. An interactive tool for comparing lists with Venn's diagrams”. Available online at: https://bioinfogp.cnb.csic.es/tools/venny/index.html
Park, M. G., Blitzer, E. J., Gibbs, J., Losey, J. E., and Danforth, B. N. (2015). Negative effects of pesticides on wild bee communities can be buffered by landscape context. Proc. R. Soc. B: Biol. Sci. 282, 9. doi: 10.1098/rspb.2015.0299
Potts, S. G., Imperatriz-Fonseca, V., Ngo, H. T., Aizen, M. A., Biesmeijer, J. C., Breeze, T. D., et al. (2016). Safeguarding pollinators and their values to human well-being. Nature. 540, 220–229. doi: 10.1038/nature20588
Reilly, J. R., Artz, D. R., Biddinger, D., Bobiwash, K., Boyle, N. K., Brittain, C., et al. (2020). Crop production in the USA is frequently limited by a lack of pollinators. Proc. R. Soc. B: Biol. Sci. 287, 20200922. doi: 10.1098/rspb.2020.0922
Ricketts, T. H., Daily, G. C., Ehrlich, P. R., and Michener, C. D. (2004). Economic value of tropical forest to coffee production. Proc. Natl. Acad. Sci. U.S.A. 101, 12579–12582. doi: 10.1073/pnas.0405147101
Rondeau, S., Baert, N., McArt, S., and Raine, N. E. (2022). Quantifying exposure of bumblebee (Bombus spp.) queens to pesticide residues when hibernating in agricultural soils. Environ. Pollut. 309, 119722. doi: 10.1016/j.envpol.2022.119722
Rondeau, S., and Raine, N. E. (2022). Fungicides and bees: a review of exposure and risk. Environ. Int. 165, 107311. doi: 10.1016/j.envint.2022.107311
Rundlöf, M., Andersson, G., Bommarco, R., Fries, I., Hederstrom, V., Herbertsson, L., et al. (2015). Seed coating with a neonicotinoid insecticide negatively affects wild bees. Nature 521, 77–80. doi: 10.1038/nature14420
Sgolastra, F., Hinarejos, S., Pitts-Singer, T. L., Boyle, N. K., Joseph, T., Luckmann, J., et al. (2019). Pesticide exposure assessment paradigm for solitary bees. Environ. Entomol. 48, 22–35. doi: 10.1093/ee/nvy105
Sheffield, C. S., Pindar, A., Packer, L., and Kevan, P. G. (2013). The potential of cleptoparasitic bees as indicator taxa for assessing bee communities. Apidologie. 44, 501–510. doi: 10.1007/s13592-013-0200-2
Shuler, R. E., Roulston, T. H., and Farris, G. E. (2005). Farming practices influence wild pollinator populations on squash and pumpkin. J. Econ. Entomol. 98, 790–795. doi: 10.1603/0022-0493-98.3.790
Silva, V., Mol, H. G. J., Zomer, P., Tienstra, M., Ritsema, C. J., and Geissen, V. (2019). Pesticide residues in European agricultural soils-a hidden reality unfolded. Sci. Total Environ. 653, 1532–1545. doi: 10.1016/j.scitotenv.2018.10.441
Sponsler, D. B., Grozinger, C. M., Hitaj, C., Rundlöf, M., Botías, C., Code, A., et al. (2019). Pesticides and pollinators: a socioecological synthesis. Sci. Total Environ. doi: 10.1016/j.scitotenv.2019.01.016
Statistics Canada. (2022a). Table 32-10-0364-01. Area, Production and Farm Gate Value of Marketed Fruits. doi: 10.25318/3210036401-eng
Statistics Canada. (2022b). Table 32-10-0359-01. Estimated Areas, Yield, Production, Average Farm Price and Total Farm Value of Principal Field Crops, in Metric and Imperial Units. doi: 10.25318/3210035901-eng
Statistics Canada. (2022c). Table 32-10-0365-01. Area, Production and Farm Gate Value of Marketed Vegetables. doi: 10.25318/3210036501-eng
Stoner, K. A., and Eitzer, B. D. (2012). Movement of soil-applied imidacloprid and thiamethoxam into nectar and pollen of squash (Cucurbita pepo). PLoS ONE. 7, 5. doi: 10.1371/journal.pone.0039114
Tang, F. H. M., and Maggi, F. (2021). Pesticide mixtures in soil: a global outlook. Environ. Res. Lett. 16, 044051. doi: 10.1088/1748-9326/abe5d6
The Xerces Society. (2021). Xerces Society for Invertebrate Conservation. Available online at: https://www.xerces.org/ (accessed February, 2021).
Tuell, J. K., and Isaacs, R. (2010). Community and species-specific responses of wild bees to insect pest control programs applied to a pollinator-dependent crop. J. Econ. Entomol. 103, 668–675. doi: 10.1603/EC09314
USDA. (2017). Attractiveness of Agricultural Crops to Pollinating Bees for the Collection of Nectar and/or Pollen, 2015. Available online at: https://www.usda.gov/sites/default/files/documents/Attractiveness-of-Agriculture-Crops-to-Pollinating-Bees-Report-FINAL-Web-Version-Jan-3-2018.pdf (accessed November 2021).
USDA. (2021). Crop Acreage Data. Available online at: https://www.fsa.usda.gov/news-room/efoia/electronic-reading-room/frequently-requested-information/crop-acreage-data/index (accessed November, 2021).
Westphal, C., Steffan-Dewenter, I., and Tscharntke, T. (2009). Mass flowering oilseed rape improves early colony growth but not sexual reproduction of bumblebees. J. Appl. Ecol. 46, 187–193. doi: 10.1111/j.1365-2664.2008.01580.x
Williams, N. M., Crone, E. E., Roulston, T. H., Minckley, R. L., Packer, L., and Potts, S. G. (2010). Ecological and life-history traits predict bee species responses to environmental disturbances. Biol. Conserv. 143, 2280–2291. doi: 10.1016/j.biocon.2010.03.024
Willis Chan, D. S., Prosser, R. S., Rodríguez-Gil, J. L., and Raine, N. E. (2019). Assessment of risk to hoary squash bees (Peponapis pruinosa) and other ground-nesting bees from systemic insecticides in agricultural soil. Sci. Rep. 9, 11870. doi: 10.1038/s41598-019-47805-1
Willis Chan, D. S., and Raine, N. E. (2021a). Hoary squash bees (Eucera pruinosa: Hymenoptera: Apidae) provide abundant and reliable pollination services to Cucurbita crops in Ontario (Canada). Environ. Entomol. 50, 968–981. doi: 10.1093/ee/nvab045
Willis Chan, D. S., and Raine, N. E. (2021b). Population decline in a ground-nesting solitary squash bee (Eucera pruinosa) following exposure to a neonicotinoid insecticide treated crop (Cucurbita pepo). Sci. Rep. 11, 4241. doi: 10.1038/s41598-021-83341-7
Winfree, R., Reilly, J. R., Bartomeus, I., Cariveau, D. P., Williams, N. M., and Gibbs, J. (2018). Species turnover promotes the importance of bee diversity for crop pollination at regional scales. Science. 359, 791–793. doi: 10.1126/science.aao2117
Winfree, R., Williams, N. M., Gaines, H., Ascher, J. S., and Kremen, C. (2008). Wild bee pollinators provide the majority of crop visitation across land-use gradients in New Jersey and Pennsylvania, USA. J. Appl. Ecol. 45, 793–802. doi: 10.1111/j.1365-2664.2007.01418.x
Woodcock, B. A., Isaac, N. J. B., Bullock, J. M., Roy, D. B., Garthwaite, D. G., Crowe, A., et al. (2016). Impacts of neonicotinoid use on long-term population changes in wild bees in England. Nature Commun. 7, 12459. doi: 10.1038/ncomms12459
Keywords: agriculture, agroecosystems, cucurbits, ground-nesting bees, nesting guilds, perennial crops, pesticide exposure, wild pollinators
Citation: Rondeau S, Willis Chan DS and Pindar A (2022) Identifying wild bee visitors of major crops in North America with notes on potential threats from agricultural practices. Front. Sustain. Food Syst. 6:943237. doi: 10.3389/fsufs.2022.943237
Received: 13 May 2022; Accepted: 12 October 2022;
Published: 04 November 2022.
Edited by:
Veronica Zamora-Gutierrez, Centro Interdisciplinario de Investigación para el Desarrollo Integral Regional Unidad Durango (IPN), MexicoReviewed by:
Johnattan Hernández Cumplido, National Autonomous University of Mexico, MexicoCarlos Andrés Cultid Medina, CONACYT, Mexico
Copyright © 2022 Rondeau, Willis Chan and Pindar. This is an open-access article distributed under the terms of the Creative Commons Attribution License (CC BY). The use, distribution or reproduction in other forums is permitted, provided the original author(s) and the copyright owner(s) are credited and that the original publication in this journal is cited, in accordance with accepted academic practice. No use, distribution or reproduction is permitted which does not comply with these terms.
*Correspondence: D. Susan Willis Chan, ZGNoYW4wNUB1b2d1ZWxwaC5jYQ==