- 1Department of Horticulture, Division of Applied Life Science (BK21 Four Program), Graduate School of Gyeongsang National University, Jinju, South Korea
- 2Institute of Agriculture and Life Science, Gyeongsang National University, Jinju, South Korea
- 3Research Institute of Life Science, Gyeongsang National University, Jinju, South Korea
Ammonium () toxicity hinders the cabbage yield because most subspecies or varieties exhibit extreme sensitivity to . Current knowledge indicates that silicon (Si) can alleviate or reverse the ammonium toxicity severity. However, few investigations have been conducted on -stressed cabbage to elucidate the mechanism underlying the Si alleviation. The study described herein analyzes induced physio-chemical changes to explore how Si helps mitigate toxicity. We applied one of three : ratios (0:100, 50:50, and 100:0) at a constant N (13 meq·L−1) to the cabbage plants, corresponding with two Si treatment levels (0 and 1.0 meq·L−1). Chlorosis and foliage necrosis along with stunted roots occurred following 100% application were ameliorated in the presence of Si. The toxicity ratio was reduced accordingly. Similarly, inhibition on the uptake of K and Ca, restricted photosynthesis (chlorophyll, stomatal conductance, and Fv/Fm), and accumulation of reactive oxygen species (ROS, , and H2O2), as well as lipid peroxidation (MDA, malondialdehyde) in -stressed cabbages were mitigated with added Si. The lower observed oxidative stresses in solely -treated plants were conferred by the boosted antioxidant enzymes (SOD, superoxide dismutase; CAT, catalase). Concomitantly, Si-treated plants showed higher activities of key assimilation enzymes (GS, glutamine synthetase; GOGAT, glutamate synthase; NADH-GDH, glutamate dehydrogenase) and content in leaves. However, excessive assimilations cause the acidic stress, which has been demonstrated to be the primary cause of toxicity. Therefore, further investigation regarding the Si effects on H+ regulation and distribution should be warranted.
Introduction
Nitrogen (N) is indispensable to all edible vegetables and plays a fundamental role in their growth and structural development; when limited, it hinders the biomass production (Finney et al., 2016; Leghari et al., 2016). This macronutrient is taken up mainly in the form of ammonium () and nitrate (). The acquisitions of these two available inorganic N forms by plants have been well shown in distinct manners concerning the biochemical and energetic processes (Guo et al., 2007; Luo et al., 2013). Theoretically, the applications of seem to be superior to that of because of the two, is in a readily assimilable form and accordingly provides less energy losses for plants (Cui and Song, 2007; Luo et al., 2013). Likewise, is not subject to leaching, conferring a potential means of enhancement on the assimilation efficiency and reduction of environmental hazards.
Paradoxically, exclusive source, or unintentionally supplied intensive nutrition, leading to the plant tissue acidification by assimilation and ultimately cause ammonium toxicity (Hachiya et al., 2021). This phenomenon has been reported in many greenhouse grown -sensitive species, such as tomato (Barreto et al., 2022), cabbage (Song et al., 2021), and salvia (Song et al., 2022a). It usually disrupts several aspects of metabolism manifested by the morphological and physiological dysfunctions. Indeed, the leaf chlorosis and root stunt are major phenotypic markers for toxicity symptoms (Bittsánszky et al., 2015; Song et al., 2021, 2022a). Apart from these visual detrimental signs, certain integrated effects also can be referred to during this development, mainly including the following: the ion balance is disordered as a consequence of the inhibited essential cation uptake (K+, Ca2+, Mg2+) (Britto and Kronzucker, 2002; Bittsánszky et al., 2015); the photosynthesis is interfered because the plants need to withdraw the carbon skeleton to detoxify (Claussen and Lenz, 1995; Bittsánszky et al., 2015; Song et al., 2022a); oxidative stresses and the consequent mediated ROS (reactive oxygen species) scavenging enzyme activities (Britto and Kronzucker, 2002; Esteban et al., 2016) are boosted; activities of key assimilation enzymes are altered for a better maintenance of the low cytosolic assimilation level (Esteban et al., 2016).
Cabbage is an important vegetable due to the distinguishing crisp texture and richness in the nutrient composition with various antioxidants and phytochemicals (Moreb et al., 2020; Li et al., 2021). Also, cabbage is in particular importance in Korea because it is the primary ingredient of “kimchi”- a traditional salted and fermented side dish (Asano et al., 2018). However, most of the cabbage subspecies or varieties hydroponically studied are sensitive to , such as Chinese cabbage var. “Chinensis” (Obreza and Vavrina, 1993) and “Jinwa no. 2” (Brassica pekinensis) (Hu et al., 2015). Therefore, the cultivations of cabbage were largely retarded by inappropriate use of fertilizers. Consequently, agronomic practices concerning the fertilization strategy in accordance with other beneficial elements are recommended. Meanwhile, studies on cabbage have shown that silicon benefits their growth and development; moreover, silicon addition reduces or mitigates various biotic and abiotic stresses as well as enhances the resistances of cabbage to harsh sufferings (Wu et al., 2016, 2017, 2018; Ahmad et al., 2021a).
Though silicon (Si) has not been considered as an essential plant nutrient for higher plants, the evidences of its benefits on physiological and biochemical characteristics are progressively increasing (Farooq and Dietz, 2015; Khan et al., 2017; Ahmad et al., 2021a; Shah et al., 2021). Si has been identified to be associated in the rigidity of cell walls, thereby constituting a better leaf architecture with bigger leaf area and greater light interception, leading to the improvement of the net photosynthetic assimilation (Ma and Yamaji, 2006; Verma et al., 2020). It's noteworthy that amorphous Si deposited on the leaf epidermis controls the stomatal conductance and results in the reduction of the transpiration rate, which may also favor the photosynthesis (Khan et al., 2017, 2018; Shah et al., 2021). On the one hand, the substantial amount of Si accumulated in roots promotes the hydraulic movement and is subject to locally stimulate other ion uptakes (Yeo et al., 1999; Zaheer et al., 2018); on the other hand, Si is suggested to interact with certain cations and the supplementation of Si can alleviate the K (potassium), Ca (calcium) deficiency symptoms, accordingly improving the nutritional efficiency (Chen et al., 2016a; Schaller et al., 2017; Da Silva et al., 2021). In addition, Si is believed to reduce lipid peroxidation and maintain the redox homeostasis via the modulation of ROS scavenging enzyme activities under various aspects of stresses (Gong et al., 2008; Farooq et al., 2019; Ahmad et al., 2021b). Equally important, previous reports disclosed that, the inhibitory effects of abiotic stresses on the major N assimilation enzymes, like GS (glutamine synthetase), GOGAT (glutamate synthase), and GDH (glutamate dehydrogenase), could be ameliorated by Si (Feng et al., 2010; Kochanová et al., 2014). While the mitigator role of Si on stresses has been intensively reported in many plant species (Barreto et al., 2016, 2017; Campos et al., 2016; Viciedo et al., 2019), relevant work on cabbage was very scarce.
Specifically, no consensus was built toward the comprehensive effects of Si on the biochemical traits of cabbage, in particular the photosynthesis, major antioxidant enzymes, and crucial N assimilation enzymes.
The study undertaken herein, therefore, aims to determine the significance of Si on the seedling growth, photosynthetic ability, ion uptake, antioxidant defense system and key assimilation enzymes in Cabbage (Brassica campestris L. ssp. pekinensis) subjected to stress.
Materials and Methods
Plant Materials and Experimental Conditions
Korean cabbage “Ssamchu” (Brassica campestris L. ssp. pekinensis) was selected as the plant material and the seeds were purchased from Asia Seed Company, Ltd (Seoul, Korea). The seeds were sown into a plug tray with 200 cells, containing BVB medium (Bas Van Buuren Substrate, EN-12580, De Lier, The Netherlands) and germinated under an intermittent mist propagation tunnel for 7 days after sowing (DAS). The seedlings were subsequently cultured with a standard MNS (multipurpose nutrient solution) (Song et al., 2021, 2022b) in a growth chamber under an alternating diurnal regime of 10-h light (300 μmol·m−2·s−1 PPFD-Photosynthetic Photon Flux Density, generated from artificial Led light) and 14-h darkness of air-conditioned temperatures at 23 and 18°C, respectively, for another week (14 DAS).
Treatment Solutions
Subsequently, seedlings with similar sizes and turned two to three true leaves stage were screened out and transplanted into new plug trays with 200 cells, and cultivated by tap running water for 3 days (17 DAS) to leach out all the nutrients. Afterwards, the seedlings were equally allocated to be irrigated by one of the three : solutions, corresponding with or without Si supplied by K2SiO3 (Table 1). The excessively introduced potassium from K2SiO3 in 0:100, 50:50, and 100:0 treatments were deducted from KNO3, K2SO4, and K2SO4, respectively, and the resultant losses of nitrate and sulfate were compensated with nitric and sulfuric acid. In specific, three ratios of : solutions with an increasing concentration but following a constant nitrogen (N) supply at 13.0 meq·L−1, were designed, namely 0:100, 50:50, and 100:0, according to the modified MNS (multipurpose nutrient solution); the optimized Si concentration at 1.0 meq·L−1 was chosen in this study following our pioneer publications (Park et al., 2018; Hu et al., 2020). The treatment solutions were applied every 2 days for 22 days (39 DAS). Each treatment test conducted was a randomized complete design in a 2 × 3 factorial scheme, by undertaking three independent biological replications, consisting of 20 plants. In total, 60 plants for each treatment were equally laid out in three 200-cell plug trays, where the blank columns between different treatments were intentionally kept.
Measurement of Plant Growth Parameters and Statistical Determination of Toxicity Ratios
After 3 weeks of cultivation (39 DAS), plants from different treatments were collected for the growth data. The whole plant weight was determined using an electronic balance. The root, shoot, and leaf samples were separated from the harvested seedlings. The leaf area was recorded using a leaf area scanning apparatus (LI-3000, Lincoln, NE, USA); the shoot length, leaf length and width, were measured with a metal ruler; the root morphology traits (root surface area and total root length) were collected and concomitantly imaged with the WinRHIZO Pro program 2007a version (Regent Instruments, Sainte-Foy, Canada) equipped with a desktop scanner (Expression 1000XL, Epson, CA, USA).
The toxicity ratio was determined by a total of 120 plants cultured with the 100:0 : treatment solution. The plants were equally divided into six groups and the toxicity ratio in each group was calculated as follows:
where “20” refers to the number of plants per group.
Estimation of the Photosynthetic Capacity
The photosynthetic capacity of plants cultured with different treatments were characterized herein by the examinations of certain vital parameters. Specifically, the total chlorophyll content in leaf samples was estimated by a protocol as described by Sims and Gamon, 2002; after a 40-min active photoperiod, the stomatal conductance and Fv/Fm ratio (variable fluorescence dividing maximum fluorescence) were investigated toward the mid-lamina part of leaves using a Decagon Portable Leaf Porometer (SC-1 VERSION, Pullman, USA) and a FluorPen (100 model, Photon Systems Instruments, Drásov, Czech Republic), respectively, according to the batch manufacturer's manual.
Quantifications of Si, K, Ca, and Mg, and Destructive Sampling
Plants with various statuses and appearances from different treatments were individually demounted from the plug trays and the medium was washed off.
For the quantification of ions, the whole fresh plants were firstly placed in an air-forced oven at 60°C until the constant dry weight; afterward, 0.1 g of powdered, dried samples were ashed inside a furnace (Lilienthal, Bremen, Germany) for 2 h at 525°C. The ashed samples were dissolved with 5 ml 25% HCl (hydrochloric acid), and adjusted to 30 ml with 10 ml 25°C and 15 ml 60°C distilled water. The mixture was subjected to filtering to obtain clear and impurity-free solutions. Finally, the ion concentrations were measured three times via ICP (inductively coupled plasma) assay (Optima 4300DV/5300DV, Perkin Elmer, Germany).
Leaf specimens from each treatment were individually cut and immediately immersed in liquid N2, and stored in −80°C until further experiments.
Determinations of Antioxidant Enzyme Activities and Contents of (Superoxide), H2O2 (Hydrogen Peroxide) and MDA (Malondialdehyde)
The frozen samples were taken out and ground into a fine powder in a pre-chilled mortar over an ice bath. 100 mg of the samples were well-mixed with 1.5 ml 50 mM phosphate buffered saline (PBS) consisting of 0.05% triton-X, 1mM EDTA, and 1 mM polyvinylpyrrolidone at pH = 7.0. The supernatant was obtained after a centrifugation (13,000 rpm, 4°C) for 20 min and used for the total protein estimation and subsequent antioxidant enzymes activity determinations.
Bradford reagent (Sigma-Aldrich, MO, USA) was used for the quantifications of the total protein content (Bradford, 1976). Nitro blue tetrazolium (NBT) inhibition method was employed for the SOD (Superoxide dismutase) activity measurement (Giannopolitis and Ries, 1977); CAT (Catalase) activity was measured on the basis of decomposition of H2O2 (Cakmak and Marschner, 1992); APX (Ascorbate peroxidase) activity was estimated according to the principle on the H2O2 scavenge (Nakano and Asada, 1981); GPX (Guaiacol peroxidase) activity was determined following the reaction on guaiacol oxidation (Amako et al., 1994). The detailed procedure can be found in a previously established report (Soundararajan et al., 2014). All the antioxidant enzymes activities were spectrophotometrically determined by a spectrophotometer (Libra S22, Biochrom, Cambridge, UK).
The content was measured based on hydroxylamine oxidization following a protocol presented by Wu and von Tiedemann, 2002. H2O2 concentration was estimated via a rapid and sensitive procedure as described by Uchida et al., 2002. The lipid peroxidation level was assessed in terms of the MDA content (Amor et al., 2005). The detailed approach can be found in Li's publication (Li et al., 2022).
Analyses of Major Enzyme Activities in Assimilation
The major enzymes of assimilations in leaves analyzed in the present study include GS, GOGAT, and NADH-GDH, and were spectrophotometrically assayed with a spectrophotometer (Libra S22, Biochrom, Cambridge, UK) according to the protocol as proposed by Song et al., 2022a with minor modifications. Leaf frozen powdered samples were prepared by adopting the identical procedure as presented above.
Approximately 0.4 g finely ground samples were homogenized with a 3 ml protein extraction buffer (50 mM of Tris-HCl, 400 mM sucrose, 2 mM DTT-dithiothreitol, 2 mM MgSO4 pH 8.0). The homogenate was centrifuged at 13,000 rpm and 4°C for 20 min, after which the GS, GOGAT, NADH-GDH activities were assayed in the supernatant fraction.
The GS activity was measured in a reaction medium (0.1 M Tris-HCl, 80 mM MgSO4 and hydroxylamine hydrochloride, 20 mM sodium glutamate and cysteine, daily prepared 40 mM ATP (adenosine 5-triphosphate disodium salt) combined with an accurate 0.7 ml crude extracts) after an incubation in a water bath at 37°C for 30 min. 1 ml of 0.37 M FeCl3 in 0.6 M HCl was added immediately to terminate the reaction, after which the mixture was subjected to vigorously vortex for 6 min and centrifugation for 10 min (5,000 rpm, RT). The biosynthesis of γ-glutamyl hydroxamate (γ-GHM) was monitored at a 540 nm absorbance.
The GOGAT activity assay was done in a 3 ml reaction solution consisting of 1.75 ml of 25 mM Tris-HCl at pH 7.5, 0.1 ml of 10 mM KCl, 0.4 ml of 20 mM L-glutamine, 0.05 ml of 0.1 M α-oxoglutarate, and 0.2 ml of 3 mM NADH (nicotinamide adenine dinucleotide). The reaction was finally triggered by the addition of 0.5 ml crude extracts. NADH-oxidation resulting in the decrease in the absorbance at 340 nm within 3 min by 30-s intervals were defined as the GOGAT activity.
Aminating direction NADH-dependent GDH activity was determined in a 3.5 ml assess mixture containing 0.1 ml of 30 mM CaCl2 and 0.3 ml ddH2O buffered with 2.6 ml Tris-HCl (15.4 mM at pH = 8.0) containing 23.1 mM α-Ketoglutarate and 231 mM of NH4Cl. The reaction was initiated by adding 0.1 ml of 6 mM NADH and 0.4 ml crude extracts. The NADH-GDH activity was characterized by NADH consumption as spectrophotometrically monitored at 340 nm after a 30°C water bath for 3 min.
Assessment of the Content in Leaves
The content in leaves was estimated by using a colorimetric method based on the Berthelot reaction (Bräutigam et al., 2007). In brief, 100 mg frozen fine powder was extracted in a buffer consisting of 2 ml of 100 mM HCl and 1 ml pre-cooled chloroform. After mild shaking for 15 min at 4°C with a rotator (AG, FINEPCR, Seoul, Korea), the mixture was subjected to centrifugation for 10 min at 10,000 g and 4°C. The supernatant was acquired, well-homogenized with 50 mg activated charcoal, and centrifuged (12,000 g, 4°C) for 8 min. The absorbance of the aqueous phase at 620 nm was read with a spectrophotometer (Libra S22, Biochrom, Cambridge, UK). The content was quantified from an ammonium sulfate standard curve.
Statistical Analysis and Graphing
All the presented data are means ± standard errors generated from no <3 independent replicates, and statistically analyzed according to Duncan's multiple range test at p = 0.05 with SAS 8.0 program (SAS 8.2 Inst., Cary, NC, USA). Bar graphs and violin graph were drawn using the GraphPad Prism 8.0 software. A heat map was plotted by the image GP online tool (Chen et al., 2022), and matrix values were transformed as Log2, with Pearson distance matrix and complete cluster method.
Results
Effects of the : Ratio and Si Supply on Shoot Growth Attributes
For Si-deficiency plants, the shoot growth attributes were significantly influenced by the different forms of N supply, as is apparent in Figure 1A “Si (–) part”. Cabbage plants grown with a mixture of and showed better growth compared with solely -treated plants and, similar growth with solely -treated plants. Indeed, this phenomenon was further evidenced by investigations on the leaf area and whole plant weight. In comparison with plants supplied with 100% , the leaf area of plants cultured in 0:100 and 50:50 : were remarkably increased, by 197.3 and 191.5%, respectively (Figure 1B); the whole plant weight of plants in 0:100 and 50:50 : regimes dramatically enhanced by 1.17- and 1.28-fold, respectively, relative to that in the 100:0 : regime (Figure 1C).
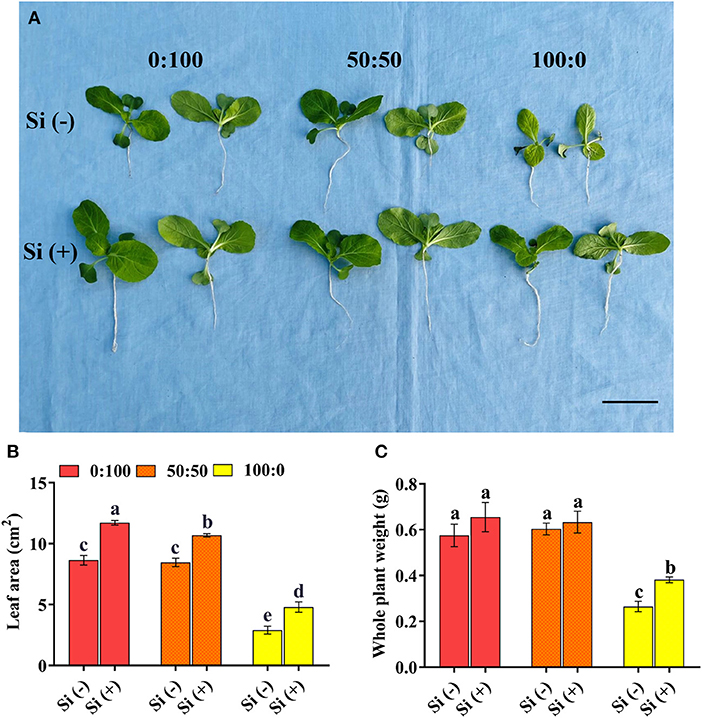
Figure 1. (A) Cabbage growth status regarding (B) leaf area and (C) whole plant weight as affected by the three : ratios and Si applications. Plant exposure to the same treatment is presented by a plant pair with similar growth. Data are the means plus standard errors (n = 6 independent replicates); different lowercases denote the significant difference, which was determined by Duncan's multiple range test at p = 0.05. Scale bar in (A) refers to 2 cm.
Concomitantly, the addition of Si individually to three treatment solutions drastically promoted the shoot growth, irrespective of the : ratios (Figure 1A). Compared with the Si-deficiency plants, the Si supply increased the leaf area of plants in 0:100 : by 35.7% and that of plants in 50:50 : by 26.3%, respectively (Figure 1B); the whole plant weight increased by 13.9 and 5.0%, respectively, when the same comparison was performed. Outstandingly, the added Si to 100:0 : solution rapidly enhanced the plant leaf area and whole plant weight by 65.3 and 44.2%, respectively (Figures 1B,C).
Additionally, certain differences were shaped concerning the dry weight, shoot length, leaf length and width, regardless of the N form and Si treatments (Table 2). Overall, an increasing level of from 50 to 100% resulted in substantial reductions of these four parameters, while additional application of Si can upgrade their development. F-test results displayed a strong interaction between the N form and Si application (0.001 < P < 0.01) on dry weight and leaf length (Table 2).
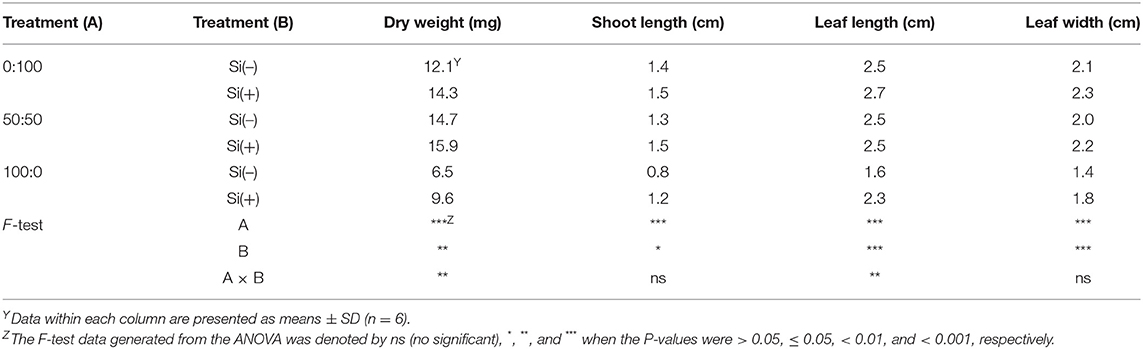
Table 2. Dry weight, shoot length, leaf length and width as affected by three : ratios and Si application.
Effects of the : Ratio and Si Supply on the Root Morphological Traits
The plant root appearances were directly in relation to the nutrient availability, and we therefore assessed the key root morphological traits including the root surface area and total root length in response to the N form with or without Si.
In a whole of Figure 2, root morphology along with the two parameters analyzed herein greatly varied among the N treatments and Si treatments. Independent of the Si supply, a severely declined tendency on the root system was conferred with an increasing level of from 50 to 100%, while merely slight changes were recorded between the 0:100 and 50:50 : (Figure 2A). However, as compared with Si (–), larger and deeper root systems were observed in terms of improved branching and density in the presence of Si regardless of the : ratios (Figure 2A).
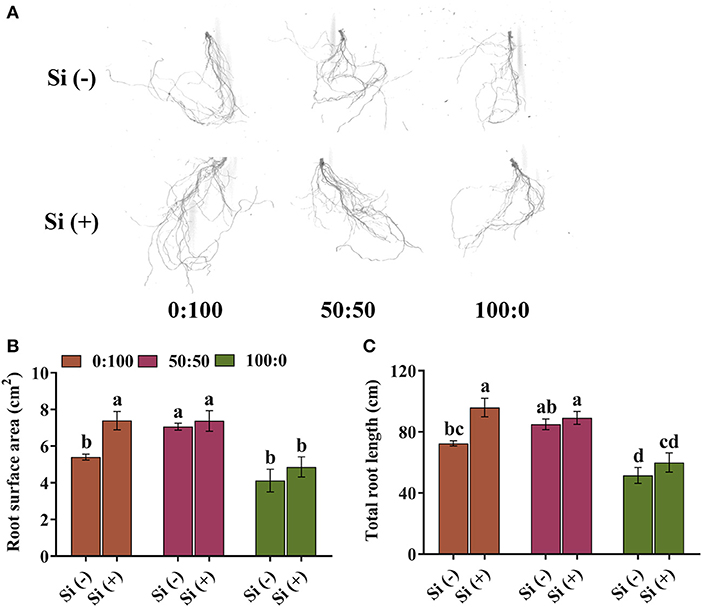
Figure 2. (A) Scanned images of the root morphology and monitored parameters of (B) root surface area and (C) total root length in response to three : ratios with and without Si supplementations. Data in (B) and (C) are the mean ± SD of n = 6 biological samples; different letters over the columns represent significant differences at p < 0.05 following Duncan's multiple range test.
In line with the root scanned images, distinguished responses toward root surface area and total root length were observed to an increasing level of , and at least, the detrimental effects advanced by excessive were partly prevented by Si (Figures 2B,C). The root surface area and total root length of plants cultured with 50:50 : supplemented with Si gained minor increases (4.4 and 5.0%, respectively) than that with the same N treatments without Si (Figures 2B,C). Similarly but more significantly, compared with the Si-deficiency plants, solely -fed plants with Si possessed 17.9 and 16.2% improvements, respectively, on the root surface area and total root length (Figures 2B,C). Interestingly, the addition of Si to solely -fed plants sharply increased the root surface area and total root length by 36.8 and 32.4%, respectively (Figures 2B,C).
Toxicity Appearance as Affected by Si Supplementation
Growth and development of plant shoots and roots were remarkably restricted in response to 100% supply. Notably, in this investigation, the cabbage plants cultured in 100:0 : advanced toxicity symptoms, as physiologically characterized by visual leaf chlorosis together with burned tips, and even necrosis, accompanied by stunted roots (Figure 3A). Thus, under a controlled environment, Korean cabbage “Ssamchu” displayed extremely sensitive to high level.
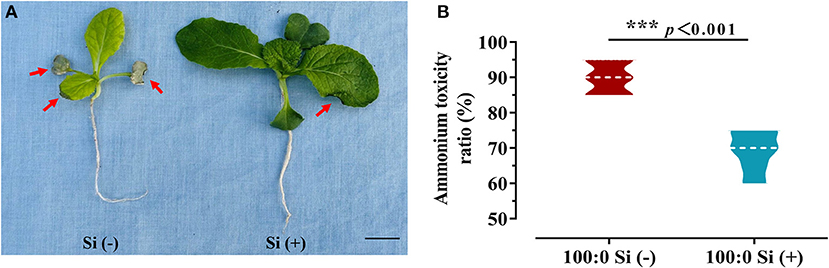
Figure 3. (A) Growth analysis and (B) ammonium toxicity appearance ratio (%) of cabbage plants in response to 100% without Si (–) and with Si (+). Data in (B) are the means of 120 plants underpinning 6 independent replicates ± SD from different treatment groups; ***P < 0.001 was calculated by Student's two-sided t-test. Plants with identical treatments showed similar growth and the representative one was selected for photographing. Red arrows point to the typical toxicity symptoms. Scale bar in (A) represents 2 cm.
More importantly, as expected, the application of Si to some extent inhibited the appearance of toxicity symptoms. Regarding the plant status and behavior under different Si treatments, augmentation of Si to the plants resulted in a healthier foliage with only one spot toxicity and robust root system, whereas chlorosis, dead fronds, and smaller roots were observed in Si-deficiency plants (Figure 3A). Statistically, the toxicity ratio of plants grown with 100:0 : was significantly decreased from 90 to 70% when Si was supplemented (Figure 3B). Succinctly, the toxicity effects on plant growth and toxicity ratio can be partly ameliorated by the Si application.
Uptake of Silicon (Si), Potassium (K), Calcium (Ca), and Magnesium (Mg)
The external drenching application of Si to the plants greatly altered not only the accumulations of Si in plant tissues, but also the contents of other uptake-related ions, such as K, Ca, and Mg.
In the absence of Si, minor changes of Si uptake were recorded among the three : treatments (Figure 4A), while the K level was remarkably decreased when the external concentration was raised from 0 to 50% (Figure 4B). Similarly, the uptake of Ca and Mg were significantly restricted in response to an increasing external level of from 50 to 100% (Figure 4B).
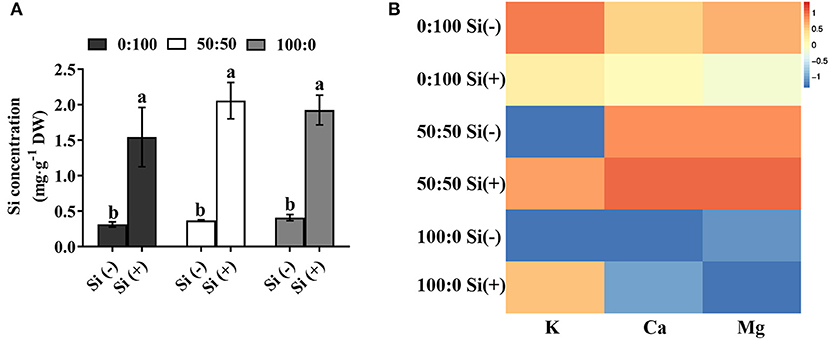
Figure 4. Contents of (A) Si and (B) other ions (K, Ca, Mg) as affected by the three different : ratios with or without Si supplementations. Data in (A) are means of three independent replicates ± SD; statistical significance was denoted by different lowercases according to Duncan's multiple range test at p = 0.05. Concentrations of K, Ca, and Mg in plants were compared and illustrated via a heatmap; original data was averaged, Log2 normalized and clustered.
On the contrary, it's highly noteworthy that, with the exception of the Mg content in 100:0 :, the supply of Si to plants solely cultured with and mixtures of and rapidly reinforced the K and Ca uptake (Figure 4B). Also, solely -treated plants cultivated with an increasing external Si supply showed a parallel increase in the Ca uptake (Figure 4B). Additionally, plants grown with a combination of and supplemented with Si displayed the highest Ca and Mg levels, compared with those in the other treatments (Figure 4B).
Total Chlorophyll, Stomatal Conductance and Fv/Fm Values (Quantum Efficiency)
For Si-deficiency plants, the chlorophyll content and stomatal conductance improved by 5.8 and 0.3%, respectively, in response to an increasing external level from 0 to 50%; by contrast, when the external level was raised from 50 to 100%, the total chlorophyll content, stomatal conductance and Fv/Fm notably diminished by 32.4, 75.4, and 13.1%, respectively (Figure 5 “Si (–) part”).
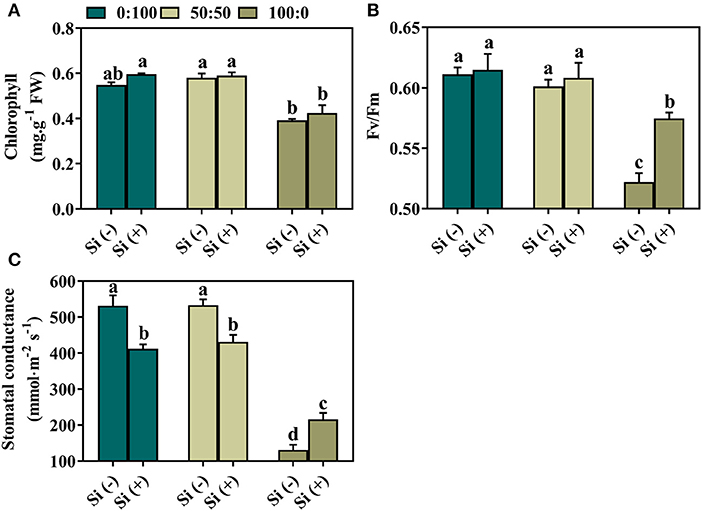
Figure 5. The responses of photosynthesis-related parameters regarding the (A) chlorophyll content, (B) Fv/Fm (quantum efficiency), and (C) stomatal conductance to three : ratios in the absence and presence of Si. Data are the average of n = 6 independent replicates ± standard deviation (SD); different lowercases indicate significant differences at p < 0.05 level according to Duncan's multiple range test.
After the nourishments of Si, the plants cultivated with sole nutrient and nutrient drastically enhanced the chlorophyll content by 8.8 and 8.4%, respectively, as compared to the plants cultured without Si (Figure 5A). Likewise, but more significantly, we detected an increase of Fv/Fm at 10.0% for solely -treated plants after Si supplementation (Figure 5B). The application of Si sharply decreased the stomatal conductance of plants cultivated with 0:100 and 50:50 : treatments, whereas solely -treated plants displayed an enhanced trend in response to Si supply (Figure 5C).
Analysis of the Antioxidative Enzyme Activities and Oxidative Stresses
When plants were exposed to external stresses, oxidative damage emerged and yielded plenty of antioxidative enzymes (SOD, CAT, APX, and GPX) to lower the stress degree. We, therefore, monitored and compared the activities of antioxidant enzymes in response to different treatments.
The activity of SOD under 50:50 and 100:0 : Si (–) declined 3.1 and 20.8%, respectively, relative to that cultivated with 0% nutrition (Figure 6A). As compared to the 0:100, 50:50, and 100:0 : Si (–), Si addition remarkably boosted the SOD activity by 53.4%, 20.8%, and 1.27-fold, respectively (Figure 6A). Similar, but less pronounced trends were acquired as shown by CAT activity, the plants supplied with 100% nutrition showed a significant enhancement after Si was added (Figure 6B). Unexpectedly, with the exception of GPX activity in 50:50 : regime, individual addition of Si to three : treatments failed to reinforce the antioxidative defense system regarding APX and GPX (Figures 6C,D).
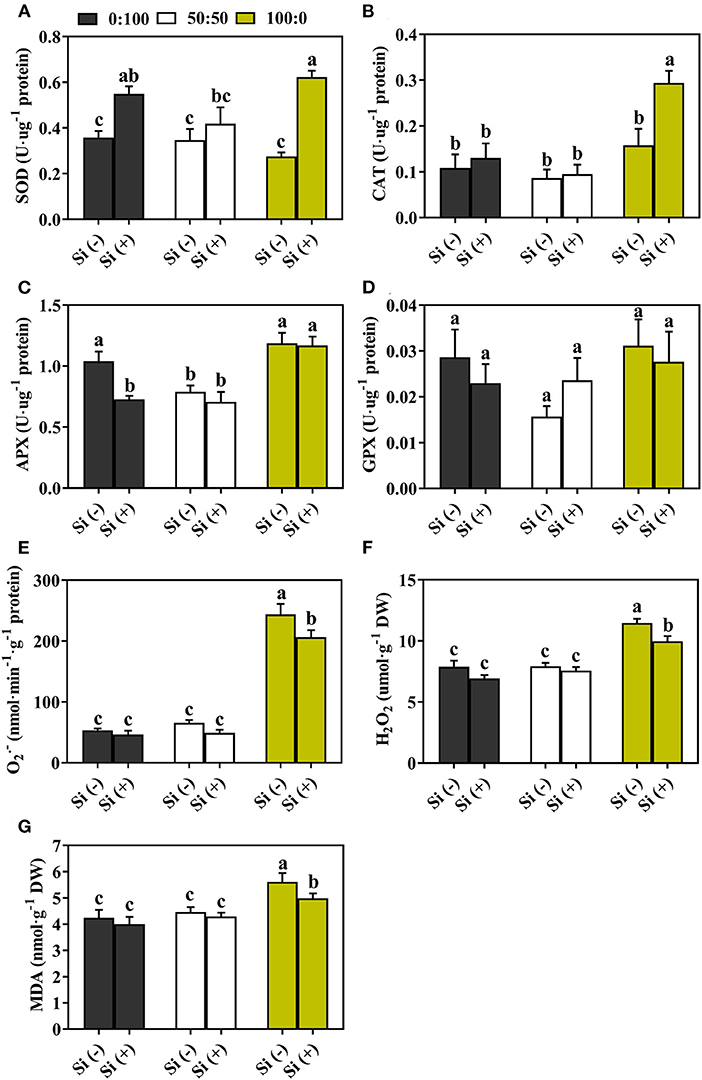
Figure 6. Antioxidative enzyme activities of (A) SOD, (B) CAT, (C) APX, (D) GPX and contents of (E) (superoxide), (F) H2O2 (hydrogen peroxide), and (G) MDA (malondialdehyde) in response to three : ratios with or without Si supplementations. Data are the average of at least n = 4 independent replicates ± standard deviation (SD); different letters over bars indicate the significant difference, which was determined by Duncan's multiple range test at p = 0.05.
Thereafter, the degree of oxidative stresses in cabbage was determined in terms of the accumulation of and H2O2. When plants were subjected to a high level of supply, excessive amounts of ROS and higher lipid peroxidation (LPO) were pronounced. For instance, we observed that plants supplied with exclusive nutrition had markedly improved contents of , H2O2, and MDA, regardless of the Si addition (Figures 6E–G). While the augmentations of Si notably mitigated disproportionate accumulation of ROS: the addition of Si to solely -treated plants significantly decreased the concentration of and H2O2 by 15.4 and 13.0%, respectively (Figures 6E,F). The presence of Si rapidly diminished the MDA content in 100:0 : plants by 11.2% while other combinations showed no significant reductions (Figure 6G).
Key Enzyme Activities Involved in the Assimilation Pathway
To investigate whether Si application modified the major assimilation enzyme activities, we monitored the activities of GS, GOGAT, and NADH-dependent GDH in response to an increasing level of external supply supplemented with and without Si. In the absence of Si, as compared to the plants cultivated exclusively with , 50 and 100% significantly increased the GS activities by 62.2% and 1.85-fold, respectively (Figure 7A); similarly, the activity of NADH-GDH was increased by 36.7% and 1.51-fold when the same comparison was employed (Figure 7C). Oppositely, with an increasing level of external supply, GOGAT activities of plants grown in 50:50 and 100:0 : Si (–) decreased by 35.2 and 51.9%, respectively, compared with that in 0:100 : Si (–) (Figure 7B). The activities of the three major enzymes were to varying degrees increased after addition of Si to each treatment solution. As depicted in Figure 7A, the supplementation of Si to 0:100, 50:50, and 100:0 : solutions remarkably enhanced the GS activities by 12.1, 60.8, and 35.1%, respectively. Furthermore, increases by 2.6, 19.4, and 15.1% on GOGAT activities were conferred by Si addition to 0, 50, and 100% supply, respectively (Figure 7B). And the application of Si upon solely -treated plants not only exhibited the highest reinforcement (by 35.6%), but also gained the most active NADH-GDH (Figure 7C).
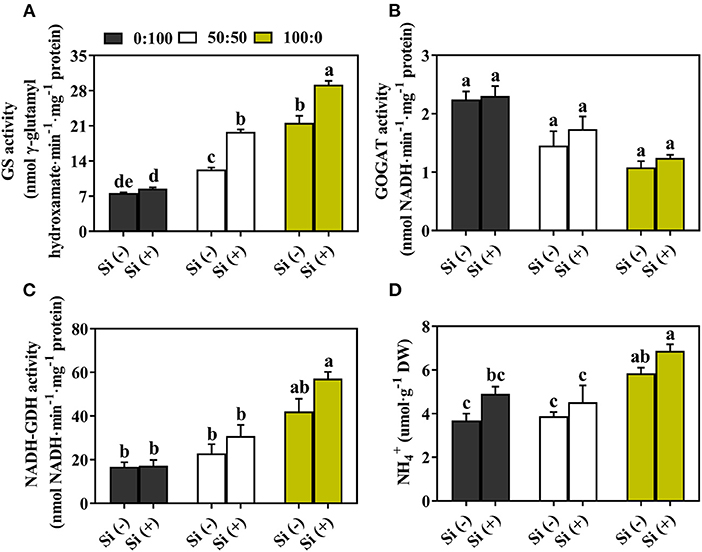
Figure 7. Effects of the : ratio and Si application on the activities of major N assimilation enzymes concerning (A) GS, (B) GOGAT, (C) NADH-dependent GDH and (D) contents of in leaves. Data displayed are means of n = 6 independent replicates ± SD; significant differences were determined by Duncan's multiple range test at p = 0.05 and shown by different letters over bars.
The content in plant leaves increased after the supplementation of Si (Figure 7D). The highest content of was recorded when the plants were grown with 100:0 : supply, regardless of the Si level; Si addition to the 100:0 : solution significantly improved the by 15.0% (Figure 7D).
Discussion
Si-induced mitigation against excessive toxicity symptoms has been reported in many plant species, such as cucumber (Campos et al., 2016), tomato (Barreto et al., 2016), and broccoli (Barreto et al., 2017). However, very limited data are available for cabbage. Thus, this study was carried out to evaluate the effects of Si on the plant growth traits in response to an increasing level of supply, to unveil the associated mechanisms that are responsible for the alleviatory role of Si on toxicity in cabbage.
Several studies have shown that the application of Si could remarkably promote the plant growth and development, oscillating from crops to vegetables (Savant et al., 1996; Khan et al., 2015; Nyawade et al., 2020; Da Silva et al., 2021). In our trials, the cabbage shoot and root growth were also enhanced to varying extents by the supplementation of Si. With the exception of the whole plant weight, the addition of Si to solely -treated plants significantly enhanced the leaf area, total root length, and root surface area (Figures 1B,C, 2B,C), which well agreed with the report where Si upgraded the effects of on cauliflower (Barreto et al., 2017). Likewise, plants cultured with a mixture of and showed slight improvements on the whole plant weight and root-related parameters (total root length and root surface area), whereas the leaf area was dramatically increased (Figure 1B). These findings established the beneficial roles of Si involved in the plant growth performance, contributing to the crop productivity and sustainable production (Ma and Yamaji, 2006; Coskun et al., 2016; Ahmad et al., 2021a).
Furthermore, toxicity can induce many detrimental changes regarding the biochemical, physiological, and nutritional aspects (Britto and Kronzucker, 2002; Bittsánszky et al., 2015). Indeed, many visual symptoms, i.e., leaf chlorosis and necrosis, together with a stunted root, were usually observed (Britto and Kronzucker, 2002; Bittsánszky et al., 2015; Esteban et al., 2016). In this study, cabbage cultivated with 100% developed typical toxicity symptoms, which was in line with our previous publications (Song et al., 2021, 2022a). Interestingly, this study was carried out in a controlled environment where solely -treated plants had a similar growth with the plants grown in 50:50 : regime, whereas they showed a relative poor status under greenhouse condition (Song et al., 2021). More importantly, Si has been suggested to be able to improve the abiotic damage prevention and stress resistance against toxicity in many plants (Campos et al., 2020). In this context, after the solely -treated plants were nourished with Si, the growth was ensured and the symptoms conferred by toxicity were visibly alleviated (Figure 3A). Certain damaged traits, like leaf area and whole plant weight (Figures 1B,C), as well as root surface area and total root length (Figures 2B,C), were also ameliorated.
Consequently, as expected, the presence of Si in the 100% nutrient solution significantly reduced the appearance ratio of toxicity symptoms (Figure 3B). It demonstrated that the toxicity in cabbage could be lessened by the application of Si.
In order to determine the uptake of Si and quadratic effects upon the modulations of other ions, we quantified the ion concentrations in the cabbages subjected with three : ratios supplied with and without Si. Apparently, independent of the : ratios, the presence of Si in the nutrient solutions markedly improved the accumulation of Si in the plants, presenting a parallel uptake rate of Si with previous studies (Mecfel et al., 2007; Barreto et al., 2017; Schaller et al., 2019; Viciedo et al., 2019). It is worthy to note that plants suffering from toxicity resulted in a great amount of cation extrusion, especially K, Ca, and Mg. The K was believed to act in an antagonistic way against (Liang, 1999; Balkos et al., 2010; Song et al., 2022b); besides, a K-related nutrient balance in plant cells could be significantly managed by Si (Chen et al., 2016b). Conversely, excess supply restricted the depletion of anions due to the competitive behaviors (Britto and Kronzucker, 2002; Bittsánszky et al., 2015; Campos et al., 2020).
Indeed, in the absence of Si, we noticed that K, Ca, and Mg contents were significantly diminished when the external level improved from 50 to 100% (Figure 4B). On the other hand, in the presence of Si, the plants cultivated with a mixture of and showed greater levels of cations, regardless of the types (Figure 4B), which could be the reason for a promoted growth status compared with 50:50 : Si (–) (Figure 1A). In the same way, with the exception of Mg, addition of Si to the 100% solution dramatically rescued the losses of K and Ca, leading to the higher contents of these cations in plants (Figure 4B). As well agreed by many previous reports, there was a interaction between Si application and K absorption (Chen et al., 2016a,b). The influence of Si on the uptake of K could be explained by the fact that the former possessed the ability to activate the membrane-located H-ATPase, an enzyme contributing to the K influx (Liang, 1999; Zhang et al., 2021). Thereby harsh : treatments instigated the involvement of Si for the maintenance of the ion homeostasis. Succinctly, Si can ameliorate the inhibitions of K and Ca uptake by toxicity.
Plants with higher contents of chlorophyll were more prone to show greater light absorption rate and photosynthetic ability (Buttery and Buzzell, 1977; Zhang et al., 2011; Song et al., 2022a). In a whole, taking the Si supply aside, when the supply of N was solely adopted in the form of , cabbage plants exhibited the lowest chlorophyll contents, while higher chlorophyll levels were conferred by the treatments of 50:50 and 0:100 :; the addition of Si to individual treatments suppressed the degradation of chlorophyll (Figure 5A) (Barreto et al., 2017; Campos et al., 2020). Fv/Fm of plant leaves is a crucial reflection of the maximum photosystem II photochemistry (PSII), which has been widely adopted to judge the degree of injury under stresses (Saeck et al., 2016). Notably, plants solely cultured with nutrient had the lowest Fv/Fm, whereas it was significantly improved after the nourishment of Si (Figure 5B), which agrees with many previous findings in support of the protective role of Si on photosynthetic mechanisms (Feng et al., 2010; Mahdieh et al., 2015; Ahmad et al., 2021b; Li et al., 2022). Besides, high supply inhibited the stomatal conductance and respiration in cabbage (Shang and Shen, 2018; Song et al., 2021). Inconsistent with the plants cultured with 50:50 and 0:100 :, the augmentation of Si considerably improved the plants' stomatal conductance when the N source was solely supplied by (Figure 5C). Possibly, the precipitation of Si on the leaf epidermis modulated the internal CO2 concentration and water dissipation, favoring the photosynthetic efficiency (Barreto et al., 2017; Campos et al., 2020). Surprisingly, but still in accordance with previous findings, added Si led to the increase of stomatal conductance of solely -treated plants, probably because of the severe injuries caused by acidic stress, but rendering a higher CO2 uptake rate and photosynthetic ability for sufficient supply of carbohydrates in assisting the ammonium detoxification strategy (Vatehová et al., 2012; Barreto et al., 2017; Viciedo et al., 2019; Song et al., 2022a). Therefore, the addition of Si to the 100:0 : solution effectively attenuated the reductions of chlorophyll content and Fv/Fm, and accordingly guaranteed the photosynthetic ability.
Plants are able to well-maintain a dynamic equilibrium between the production and scavenging of reactive oxygen species (ROS), mainly including the (superoxide) and H2O2 (hydrogen peroxide) (Zhu et al., 2000; Gong et al., 2008). Concurrently, this steady-state level of ROS in plants was tightly regulated by efficient antioxidant defense systems, such as stimulated antioxidative enzymes (Mittler et al., 2004). SOD is a prime candidate not only for the conversion of into H2O2, but also the subsequent rapid decomposition of H2O2 by CAT, APX, and GPX (Mittler et al., 2004; Gong et al., 2008). Nevertheless, excessively elicited ROS in unfavorable conditions, like toxicity, damages the defense system, resulting in the imbalances and disturbances of redox signaling, as characterized by the over-dose lipid peroxidation and decreased photosynthetic pigment levels. In our experiment of Si-deficiency plants, exclusive supply promoted the upregulations of CAT, APX, GPX (Figures 6B–D), illustrating a strategy against toxicity. Similar results were previously acquired in spinach (Domínguez-Valdivia et al., 2008) and wheat (Liu et al., 2017); consistently, as mentioned above, plants grown in 100% possessed the lowest chlorophyll (Figure 5A), suggesting more serious damages caused by toxicity (Bittsánszky et al., 2015).
Afterwards, the accumulations of and H2O2 significantly increased when the external supply reached 100% (Figures 6E,F), indicating that substantial supply disturbed the dynamic balance between production and elimination of ROS in cabbage. The disproportionate accumulation of and H2O2 impaired the cell membrane, leading to the improvement in the MDA level (Figure 6G). These biochemical responses of cabbage to high supply were in agreement with certain pioneer reports (Barreto et al., 2016; Yang et al., 2021).
The effects of Si supplementation on the regulation of the defense system against oxidative stresses has been well-established (Soundararajan et al., 2014; Wu et al., 2018; Ahmad et al., 2021a; Shah et al., 2021). It was frequently observed that Si addition partly stimulated higher activities of antioxidant enzymes, rendering a protective role from oxidative damages on the cell membrane (Gong et al., 2008; Farooq et al., 2019; Campos et al., 2020). Indeed, in the present work, 100% supply in particular, the supplemented Si promoted the activity of SOD and CAT, thereby scavenging the excessive and H2O2 and reducing the MDA level (Figures 6A,B,E–G). Surprisingly, APX and GPX failed to, or showed minor upregulation, in response to Si supplementation (Figures 6C,D), probably due to the fact that, unlike CAT is more referred to in the detoxification of H2O2 and , while APX and GPX act more as prime signaling molecules in plants (Apel and Hirt, 2004; Cuypers et al., 2011). It's worthy to note that the antioxidant enzymes were partly promoted, probably because of the fact that the antioxidative defense system varies among species (Dumanović et al., 2021). For instance, Soundararajan revealed that activities of APX and GPX increased, while CAT decreased after the application of Si in salt-stressed salvia (Soundararajan et al., 2014). The present study on Si-supplemented cabbages showed minor reductions of APX activity which is corroborative with a previous finding by Shah et al., 2021. Moreover, the triggered antioxidant machinery by the constitutive involvement of Si modulates the ROS equilibrium in plant cells, as revealed by the decline of , H2O2, and MDA contents (Figures 6E–G). Outstandingly, as compared with 50:50 and 0:100 :, plants treated with sole nutrition exhibited more rapid decreases in the ROS accumulation and lipid peroxidation after Si nourishment. It can be therefore suggested that the toxicity-induced excess oxidative stresses and lipid peroxidation can be mitigated by the application of Si.
is incorporated, catalyzed, and assimilated in higher plants principally through the glutamine synthetase (GS)/glutamate synthetase (GOGAT) and alternatively via the glutamate dehydrogenase (GDH) routes (Bittsánszky et al., 2015; Song et al., 2022a). Numerous studies previously disclosed that plants enhanced the tolerance by rapid and sustained upregulations of assimilation enzyme activities (Horchani et al., 2010; Esteban et al., 2016; Xian et al., 2020; Song et al., 2022a). Which well agreed with that cabbage plants examined in this study progressively enhanced the activities of GS and NADH-dependent GDH in response to an increasing external supply (Figures 7A,C). By contrast, GOGAT activity displayed opposite regulatory patterns from that of GS and NADH-GDH (Figure 7B). GS converted the glutamate into glutamine, and the latter acted as a major substrate involved in the GOGAT reaction, which contributed to the synthesis of glutamate and is more pronounced when the was limited (Guillamón et al., 2001).
Si-treated plants had higher activities of these assimilation enzymes, which confirmed the positive influences provided by Si because plants exhibited better growth after the replenishment of Si (Figures 1, 2). In particular, it's noteworthy that the added Si remarkably promoted the GS and NADH-GDH activities and attenuated the toxicity deterioration in plants cultivated with 100% supply (Figures 3A, 7A,C). As a consequence, the upgraded GS/GOGAT cycle and GDH route are able to improve the nitrogen use efficiency (NUE) and subsequent synthesis of N-containing metabolites (such as chlorophyll protein) used for plant growth and development (Fan et al., 2019). Furthermore, higher levels of were detected in Si-accumulated plants relative to the Si-deprived counterparts (Figure 7D), probably due to the findings that Si acted as the structural basis can stabilize the cell membranes, which increased the uptake rate of (Sheng et al., 2018). Our results were in accordance with Feng's report, where he proposed that the inhibitions of the GS/GDH cycle and the restricted uptake, could be reversed by Si supplementation (Feng et al., 2010).
However, it seems paradoxical that the improved activities of GS and GDH by Si could impose more assimilation, accordingly resulted in the plant tissue acidification, which has been considered as the primary cause of toxicity (Hachiya et al., 2021). In this respect, the mitigator role of Si against acidic stress inflicted toxicity should be inevitably provoked: Si was found to increase the membrane fluidity and activity of plasma membrane H+-ATPase (Liang, 1999; Liang et al., 2006). The latter acts as a transporter that extrude the H+ out of the cells, thereby generating an electrochemical proton gradient and enabling the intracellular pH regulation and nutrition uptake (Zhang et al., 2017). Therefore, the alleviation of toxicity degree studied in cabbage may be ascribed to the involvement of Si on the modulation of H+.
Conclusion
To sum up, the present study is a documentation to confirm that the toxicity degree in cabbage with Si supplementation was markedly alleviated. This amelioration effect delivered by Si on -stressed cabbages was involved with the fluctuation of various aspects, for instance, better growth, enhanced net influx of K and Ca, and improved photosynthetic ability. Outstandingly, the application of Si promoted the expression of antioxidant enzymes (SOD and CAT), leading to the rapid decline of ROS ( and H2O2) and lower lipid peroxidation (MDA); Si-treated cabbages also enhanced the activities of major assimilation enzymes (GS, GOGAT, NADH-GDH) and concentration in leaves. The current endeavor of Si application will be of immense practical value in integrated management subject for enhancing cabbage productivity.
Data Availability Statement
The original contributions presented in the study are included in the article/supplementary material, further inquiries can be directed to the corresponding author/s.
Author Contributions
JS and BRJ conceived and designed the study and edited and finalized the manuscript. JS and JY preformed the experiments. JS analyzed the data and drafted the manuscript. All authors read and approved the submitted version.
Funding
JS and JY were supported by the BK21 Four Program, Ministry of Education, Republic of Korea.
Conflict of Interest
The authors declare that the research was conducted in the absence of any commercial or financial relationships that could be construed as a potential conflict of interest.
Publisher's Note
All claims expressed in this article are solely those of the authors and do not necessarily represent those of their affiliated organizations, or those of the publisher, the editors and the reviewers. Any product that may be evaluated in this article, or claim that may be made by its manufacturer, is not guaranteed or endorsed by the publisher.
Acknowledgments
The authors acknowledge Professor Seung Jae Hwang and Mr. Hyeon Woo Jeong, Division of Applied Life Science, Graduate School of Gyeongsang National University, Korea, for providing access to the WinRHIZO Pro program.
References
Ahmad, A., Khan, W. U., Shah, A. A., Yasin, N. A., Naz, S., Ali, A., et al. (2021a). Synergistic effects of nitric oxide and silicon on promoting plant growth, oxidative stress tolerance and reduction of arsenic uptake in Brassica juncea. Chemosphere 262, 128384. doi: 10.1016/j.chemosphere.2020.128384
Ahmad, A., Yasin, N. A., Khan, W. U., Akram, W., Wang, R., Shah, A. A., et al. (2021b). Silicon assisted ameliorative effects of iron nanoparticles against cadmium stress: Attaining new equilibrium among physiochemical parameters, antioxidative machinery, and osmoregulators of Phaseolus lunatus. Plant Physiol. Bioch. 166, 874–886. doi: 10.1016/j.plaphy.2021.06.016
Amako, K., Chen, G.-X., and Asada, K. (1994). Separate assays specific for ascorbate peroxidase and guaiacol peroxidase and for the chloroplastic and cytosolic isozymes of ascorbate peroxidase in plants. Plant Cell Physiol. 35, 497–504.
Amor, N. B., Hamed, K. B., Debez, A., Grignon, C., and Abdelly, C. (2005). Physiological and antioxidant responses of the perennial halophyte Crithmum maritimum to salinity. Plant Sci. 168, 889–899. doi: 10.1016/j.plantsci.2004.11.002
Apel, K., and Hirt, H. (2004). Reactive oxygen species: metabolism, oxidative stress, and signal transduction. Annu. Rev. Plant Biol. 55, 373–399. doi: 10.1146/annurev.arplant.55.031903.141701
Asano, K., Yang, H., Lee, Y., and Yoon, J. (2018). Designing optimized food intake patterns for Korean adults using linear programming (I): analysis of data from the 2010~2014 Korea National Health and Nutrition Examination Survey. J. Nutr. Health 51, 73–86. doi: 10.4163/jnh.2018.51.1.73
Balkos, K. D., Britto, D. T., and Kronzucker, H. J. (2010). Optimization of ammonium acquisition and metabolism by potassium in rice (Oryza sativa L. cv. IR-72). Plant Cell Environ. 33(1), 23-34. doi: 10.1111/j.1365-3040.2009.02046.x
Barreto, R., Prado, R., Leal, A., Troleis, M., Junior, G. S., Monteiro, C., et al. (2016). Mitigation of ammonium toxicity by silicon in tomato depends on the ammonium concentration. Acta Agr. Scand. B—S. P. 66, 483–488. doi: 10.1080/09064710.2016.1178324
Barreto, R. F., de Mello Prado, R., Lúcio, J. C. B., López-Díaz, I., Carrera, E., and Carvalho, R. F. (2022). Ammonium toxicity alleviation by silicon is dependent on cytokinins in tomato cv. Micro-tom. J. Plant Growth Regul. 41, 417–428. doi: 10.1007/s00344-021-10314-5
Barreto, R. F., Júnior, A. A. S., Maggio, M. A., and de Mello Prado, R. (2017). Silicon alleviates ammonium toxicity in cauliflower and in broccoli. Sci. Hortic. 225, 743–750. doi: 10.1016/j.scienta.2017.08.014
Bittsánszky, A., Pilinszky, K., Gyulai, G., and Komives, T. (2015). Overcoming ammonium toxicity. Plant Sci. 231, 184–190. doi: 10.1016/j.plantsci.2014.12.005
Bradford, M. M.. (1976). A rapid and sensitive method for the quantitation of microgram quantities of protein utilizing the principle of protein-dye binding. Anal. Biochem. 72, 248–254. doi: 10.1016/0003-2697(76)90527-3
Bräutigam, A., Gagneul, D., and Weber, A. P. (2007). High-throughput colorimetric method for the parallel assay of glyoxylic acid and ammonium in a single extract. Anal. Biochem. 362, 151–153. doi: 10.1016/j.ab.2006.12.033
Britto, D. T., and Kronzucker, H. J. (2002). toxicity in higher plants: a critical review. J. Plant Physiol. 159, 567–584. doi: 10.1078/0176-1617-0774
Buttery, B., and Buzzell, R. (1977). The relationship between chlorophyll content and rate of photosynthesis in soybeans. Can. J. Plant Sci. 57, 1–5. doi: 10.4141/cjps77-001
Cakmak, I., and Marschner, H. (1992). Magnesium deficiency and high light intensity enhance activities of superoxide dismutase, ascorbate peroxidase, and glutathione reductase in bean leaves. Plant Physiol. 98, 1222–1227. doi: 10.1104/pp.98.4.1222
Campos, C. N. S., de Mello Prado, R., and Caione, G. (2016). Silicon and excess ammonium and nitrate in cucumber plants. Afr. J. Agr. Res. 11, 276–283. doi: 10.5897/AJAR2015.10221
Campos, C. N. S., de Silva Júnior, G. B., de Prado, R. M., de David, C. H. O., de Souza Junior, J. P., and Teodoro, P. E. (2020). Silicon mitigates ammonium toxicity in plants. Agron. J. 112, 635–647. doi: 10.1002/agj2.20069
Chen, D., Cao, B., Qi, L., Yin, L., Wang, S., and Deng, X. (2016a). Silicon-moderated K-deficiency-induced leaf chlorosis by decreasing putrescine accumulation in sorghum. Ann. Bot. 118, 305–315. doi: 10.1093/aob/mcw111
Chen, D., Cao, B., Wang, S., Liu, P., Deng, X., Yin, L., et al. (2016b). Silicon moderated the K deficiency by improving the plant-water status in sorghum. Sci. Rep. 6, 1–14. doi: 10.1038/srep22882
Chen, T., Liu, Y. X., and Huang, L. (2022). ImageGP: An easy-to-use data visualization web server for scientific researchers. iMeta, 1, e5. doi: 10.1002/imt2.5
Claussen, W., and Lenz, F. (1995). Effect of ammonium and nitrate on net photosynthesis, flower formation, growth and yield of eggplants (Solanum melongena L.). Plant Soil 171, 267–274. doi: 10.1007/BF00010281
Coskun, D., Britto, D. T., Huynh, W. Q., and Kronzucker, H. J. (2016). The role of silicon in higher plants under salinity and drought stress. Front. Plant Sci. 7, 1072. doi: 10.3389/fpls.2016.01072
Cui, X., and Song, J. (2007). Soil / nitrogen characteristics in primary forests and the adaptability of some coniferous species. Front. For. China 2, 1–10. doi: 10.1007/s11461-007-0001-8
Cuypers, A., Karen, S., Jos, R., Kelly, O., Els, K., Tony, R., et al. (2011). The cellular redox state as a modulator in cadmium and copper responses in Arabidopsis thaliana seedlings. J. Plant Physiol. 168, 309–316. doi: 10.1016/j.jplph.2010.07.010
Da Silva, D. L., de Mello Prado, R., Tenesaca, L. F. L., da Silva, J. L. F., and Mattiuz, B.-H. (2021). Silicon attenuates calcium deficiency by increasing ascorbic acid content, growth and quality of cabbage leaves. Sci. Rep. 11, 1–9. doi: 10.1038/s41598-020-80934-6
Domínguez-Valdivia, M. D., Aparicio-Tejo, P. M., Lamsfus, C., Cruz, C., Martins-Loução, M. A., and Moran, J. F. (2008). Nitrogen nutrition and antioxidant metabolism in ammonium-tolerant and-sensitive plants. Physiol. Plantarum 132, 359–369. doi: 10.1111/j.1399-3054.2007.01022.x
Dumanović, J., Nepovimova, E., Natić, M., Kuča, K., and Jaćević, V. (2021). The significance of reactive oxygen species and antioxidant defense system in plants: a concise overview. Front. Plant Sci., 2106. doi: 10.3389/fpls.2020.552969
Esteban, R., Ariz, I., Cruz, C., and Moran, J. F. (2016). Mechanisms of ammonium toxicity and the quest for tolerance. Plant Sci. 248, 92–101. doi: 10.1016/j.plantsci.2016.04.008
Fan, K., Zhang, Q., Liu, M., Ma, L., Shi, Y., and Ruan, J. (2019). Metabolomic and transcriptional analyses reveal the mechanism of C, N allocation from source leaf to flower in tea plant (Camellia sinensis. L). J. Plant Physiol. 232, 200–208. doi: 10.1016/j.jplph.2018.11.007
Farooq, M. A., and Dietz, K.-J. (2015). Silicon as versatile player in plant and human biology: overlooked and poorly understood. Front. Plant Sci. 6, 994. doi: 10.3389/fpls.2015.00994
Farooq, M. A., Saqib, Z. A., Akhtar, J., Bakhat, H. F., Pasala, R.-K., and Dietz, K.-J. (2019). Protective role of silicon (Si) against combined stress of salinity and boron (B) toxicity by improving antioxidant enzymes activity in rice. Silicon 11, 2193–2197. doi: 10.1007/s12633-015-9346-z
Feng, J., Shi, Q., Wang, X., Wei, M., Yang, F., and Xu, H. (2010). Silicon supplementation ameliorated the inhibition of photosynthesis and nitrate metabolism by cadmium (Cd) toxicity in Cucumis sativus L. Sci. Hortic. 123, 521–530. doi: 10.1016/j.scienta.2009.10.013
Finney, D. M., White, C. M., and Kaye, J. P. (2016). Biomass production and carbon/nitrogen ratio influence ecosystem services from cover crop mixtures. Agron. J. 108, 39–52. doi: 10.2134/agronj15.0182
Giannopolitis, C. N., and Ries, S. K. (1977). Superoxide dismutases: I. Occurrence in higher plants. Plant Physiol. 59, 309–314. doi: 10.1104/pp.59.2.309
Gong, H., Chen, K., Zhao, Z., Chen, G., and Zhou, W. (2008). Effects of silicon on defense of wheat against oxidative stress under drought at different developmental stages. Biol. Plantarum 52, 592–596. doi: 10.1007/s10535-008-0118-0
Guillamón, J. M., van Riel, N. A., Giuseppin, M. L., and Verrips, C. T. (2001). The glutamate synthase (GOGAT) of Saccharomyces cerevisiae plays an important role in central nitrogen metabolism. FEMS Yeast Res. 1, 169–175. doi: 10.1016/S1567-1356(01)00034-4
Guo, S., Zhou, Y., Shen, Q., and Zhang, F. (2007). Effect of ammonium and nitrate nutrition on some physiological processes in higher plants-growth, photosynthesis, photorespiration, and water relations. Plant Biol. 9, 21–29. doi: 10.1055/s-2006-924541
Hachiya, T., Inaba, J., Wakazaki, M., Sato, M., Toyooka, K., Miyagi, A., et al. (2021). Excessive ammonium assimilation by plastidic glutamine synthetase causes ammonium toxicity in Arabidopsis thaliana. Nat. Commun. 12, 1–10. doi: 10.1038/s41467-021-25238-7
Horchani, F., Hajri, R., and Aschi-Smiti, S. (2010). Effect of ammonium or nitrate nutrition on photosynthesis, growth, and nitrogen assimilation in tomato plants. J. Plant Nutr. Soil Sci. 173, 610–617. doi: 10.1002/jpln.201000055
Hu, J., Li, Y., and Jeong, B. R. (2020). Silicon alleviates temperature stresses in poinsettia by regulating stomata, photosynthesis, and oxidative damages. Agronomy 10, 1419. doi: 10.3390/agronomy10091419
Hu, L., Yu, J., Liao, W., Zhang, G., Xie, J., Lv, J., et al. (2015). Moderate ammonium: nitrate alleviates low light intensity stress in mini Chinese cabbage seedling by regulating root architecture and photosynthesis. Sci. Hortic. 186, 143–153. doi: 10.1016/j.scienta.2015.02.020
Khan, W. U. D., Aziz, T., Hussain, I., Ramzani, P. M. A., and Reichenauer, T. G. (2017). Silicon: a beneficial nutrient for maize crop to enhance photochemical efficiency of photosystem II under salt stress. Arch. Agron. Soil Sci. 63, 599–611. doi: 10.1080/03650340.2016.1233322
Khan, W. U. D., Aziz, T., Maqsood, M. A., Farooq, M., Abdullah, Y., Ramzani, P. M. A., et al. (2018). Silicon nutrition mitigates salinity stress in maize by modulating ion accumulation, photosynthesis, and antioxidants. Photosynthetica 56, 1047–1057. doi: 10.1007/s11099-018-0812-x
Khan, W. U. D., Aziz, T., Waraich, E. A., and Khalid, M. (2015). Silicon application improves germination and vegetative growth in maize grown under salt stress. Pak. J. Agr. Sci. 52, 937–944.
Kochanová, Z., Jašková, K., Sedláková, B., and Luxová, M. (2014). Silicon improves salinity tolerance and affects ammonia assimilation in maize roots. Biologia 69, 1164–1171. doi: 10.2478/s11756-014-0411-7
Leghari, S. J., Wahocho, N. A., Laghari, G. M., HafeezLaghari, A., MustafaBhabhan, G., HussainTalpur, K., et al. (2016). Role of nitrogen for plant growth and development: A review. Adv. Environ. Biol. 10, 209–219.
Li, G., Shah, A. A., Khan, W. U., Yasin, N. A., Ahmad, A., Abbas, M., et al. (2021). Hydrogen sulfide mitigates cadmium induced toxicity in Brassica rapa by modulating physiochemical attributes, osmolyte metabolism and antioxidative machinery. Chemosphere 263, 127999. doi: 10.1016/j.chemosphere.2020.127999
Li, N., Wang, K., Lv, Y., Zhang, Z., Cao, B., Chen, Z., et al. (2022). Silicon enhanced the resistance of Chinese cabbage (Brassica rapa L. ssp. pekinensis) to ofloxacin on the growth, photosynthetic characteristics and antioxidant system. Plant Physiol. Bioch. 175, 44–57. doi: 10.1016/j.plaphy.2022.02.010
Liang, Y.. (1999). Effects of silicon on enzyme activity and sodium, potassium and calcium concentration in barley under salt stress. Plant Soil 209, 217–224. doi: 10.1023/A:1004526604913
Liang, Y., Zhang, W., Chen, Q., Liu, Y., and Ding, R. (2006). Effect of exogenous silicon (Si) on H+-ATPase activity, phospholipids and fluidity of plasma membrane in leaves of salt-stressed barley (Hordeum vulgare L.). Environ. Exp. Bot. 57, 212–219. doi: 10.1016/j.envexpbot.2005.05.012
Liu, Y., Sun, J., Tian, Z., Hakeem, A., Wang, F., Jiang, D., et al. (2017). Physiological responses of wheat (Triticum aestivum L.) germination to elevated ammonium concentrations: reserve mobilization, sugar utilization, and antioxidant metabolism. Plant Growth Regul. 81, 209–220. doi: 10.1007/s10725-016-0198-3
Luo, J., Li, H., Liu, T., Polle, A., Peng, C., and Luo, Z.-B. (2013). Nitrogen metabolism of two contrasting poplar species during acclimation to limiting nitrogen availability. J. Exp. Bot. 64, 4207–4224. doi: 10.1093/jxb/ert234
Ma, J. F., and Yamaji, N. (2006). Silicon uptake and accumulation in higher plants. Trends Plant Sci. 11, 392–397. doi: 10.1016/j.tplants.2006.06.007
Mahdieh, M., Habibollahi, N., Amirjani, M., Abnosi, M., and Ghorbanpour, M. (2015). Exogenous silicon nutrition ameliorates salt-induced stress by improving growth and efficiency of PSII in Oryza sativa L. cultivars. J. Soil Sci. Plant Nut. 15, 1050–1060. doi: 10.4067/S0718-95162015005000073
Mecfel, J., Hinke, S., Goedel, W. A., Marx, G., Fehlhaber, R., Bäucker, E., et al. (2007). Effect of silicon fertilizers on silicon accumulation in wheat. J. Plant Nutr. Soil Sci. 170, 769–772. doi: 10.1002/jpln.200625038
Mittler, R., Vanderauwera, S., Gollery, M., and Van Breusegem, F. (2004). Reactive oxygen gene network of plants. Trends Plant Sci. 9, 490–498. doi: 10.1016/j.tplants.2004.08.009
Moreb, N., Murphy, A., Jaiswal, S., and Jaiswal, A. K. (2020). “Cabbage,” in Nutritional Composition and Antioxidant Properties of Fruits and Vegetables, ed A. K. Jaiswal (Cambridge, MA: Academic Press), 33–54. doi: 10.1016/B978-0-12-812780-3.00003-9
Nakano, Y., and Asada, K. (1981). Hydrogen peroxide is scavenged by ascorbate-specific peroxidase in spinach chloroplasts. Plant Cell Physiol. 22, 867–880.
Nyawade, S., Gitari, H. I., Karanja, N. N., Gachene, C. K., Schulte-Geldermann, E., Sharma, K., et al. (2020). Enhancing climate resilience of rain-fed potato through legume intercropping and silicon application. Front. Sustain. Food Syst. 4, 202. doi: 10.3389/fsufs.2020.566345
Obreza, T., and Vavrina, C. (1993). Production of Chinese cabbage in relation to nitrogen source, rate, and leaf nutrient concentration. Commun. Soil Sci. Plan. 24, 1465–1479. doi: 10.1080/00103629309368892
Park, Y. G., Muneer, S., Kim, S., Hwang, S. J., and Jeong, B. R. (2018). Foliar or subirrigational silicon supply modulates salt stress in strawberry during vegetative propagation. Hortic. Environ. Biote. 59, 11–18. doi: 10.1007/s13580-018-0002-6
Saeck, E. A., Brien, K. R. O., and Burford, M. A. (2016). Nitrogen response of natural phytoplankton communities: a new indicator based on photosynthetic efficiency Fv/Fm. Mar. Ecol. Prog. Ser. 552, 81–92. doi: 10.3354/meps11729
Savant, N., Snyder, G., and Datnoff, L. (1996). Silicon management and sustainable rice production. Adv. Agron. 58, 151–199. doi: 10.1016/S0065-2113(08)60255-2
Schaller, J., Heimes, R., Ma, J. F., Meunier, J.-D., Shao, J. F., Fujii-Kashino, M., et al. (2019). Silicon accumulation in rice plant aboveground biomass affects leaf carbon quality. Plant Soil 444, 399–407. doi: 10.1007/s11104-019-04267-8
Schaller, J., Hodson, M. J., and Struyf, E. (2017). Is relative Si/Ca availability crucial to the performance of grassland ecosystems? Ecosphere 8, e01726. doi: 10.1002/ecs2.1726
Shah, A. A., Yasin, N. A., Akram, K., Ahmad, A., Khan, W. U., Akram, W., et al. (2021). Ameliorative role of Bacillus subtilis FBL-10 and silicon against lead induced stress in Solanum melongena. Plant Physiol. Bioch. 158, 486–496. doi: 10.1016/j.plaphy.2020.11.037
Shang, H., and Shen, G. (2018). Effect of ammonium/nitrate ratio on pak choi (Brassica chinensis L.) photosynthetic capacity and biomass accumulation under low light intensity and water deficit. Photosynthetica 56, 1039–1046. doi: 10.1007/s11099-018-0815-7
Sheng, H., Ma, J., Pu, J., and Wang, L. (2018). Cell wall-bound silicon optimizes ammonium uptake and metabolism in rice cells. Ann. Bot. 122, 303–313. doi: 10.1093/aob/mcy068
Sims, D. A., and Gamon, J. A. (2002). Relationships between leaf pigment content and spectral reflectance across a wide range of species, leaf structures and developmental stages. Remote Sens. Environ. 81, 337–354. doi: 10.1016/S0034-4257(02)00010-X
Song, J., Yang, J., and Jeong, B. R. (2021). Growth, quality, and nitrogen assimilation in response to high ammonium or nitrate supply in cabbage (Brassica campestris L.) and lettuce (Lactuca sativa L.). Agronomy 11, 2556. doi: 10.3390/agronomy11122556
Song, J., Yang, J., and Jeong, B. R. (2022a). Root GS and NADH-GDH play important roles in enhancing the ammonium tolerance in three bedding plants. Int. J. Mol. Sci. 23, 1061. doi: 10.3390/ijms23031061
Song, J., Yang, J., and Jeong, B. R. (2022b). Decreased solution pH and increased K+ uptake are related to ammonium tolerance in hydroponically cultured plants. Horticulturae 8, 228. doi: 10.3390/horticulturae8030228
Soundararajan, P., Sivanesan, I., Jana, S., and Jeong, B. R. (2014). Influence of silicon supplementation on the growth and tolerance to high temperature in Salvia splendens. Hortic. Environ. Biote. 55, 271–279. doi: 10.1007/s13580-014-0023-8
Uchida, A., Jagendorf, A. T., Hibino, T., Takabe, T., and Takabe, T. (2002). Effects of hydrogen peroxide and nitric oxide on both salt and heat stress tolerance in rice. Plant Sci. 163, 515–523. doi: 10.1016/S0168-9452(02)00159-0
Vatehová, Z., Kollárov,á, K., Zelko, I., Richterová-Kučerov,á, D., Bujdo,š, M., and Liškov,á, D. (2012). Interaction of silicon and cadmium in Brassica juncea and Brassica napus. Biologia 67, 498–504. doi: 10.2478/s11756-012-0034-9
Verma, K. K., Liu, X.-H., Wu, K.-C., Singh, R. K., Song, Q.-Q., Malviya, M. K., et al. (2020). The impact of silicon on photosynthetic and biochemical responses of sugarcane under different soil moisture levels. Silicon 12, 1355–1367. doi: 10.1007/s12633-019-00228-z
Viciedo, D. O., de Mello Prado, R., Lizcano Toledo, R., dos Santos, L. C. N., Calero Hurtado, A., Nedd, L. L. T., et al. (2019). Silicon supplementation alleviates ammonium toxicity in sugar beet (Beta vulgaris L.). J. Soil Sci. Plant Nut. 19, 413–419. doi: 10.1007/s42729-019-00043-w
Wu, Y. X., and von Tiedemann, A. (2002). Impact of fungicides on active oxygen species and antioxidant enzymes in spring barley (Hordeum vulgare L.) exposed to ozone. Environ. Pollut. 116, 37–47. doi: 10.1016/S0269-7491(01)00174-9
Wu, Z., Liu, S., Zhao, J., Wang, F., Du, Y., Zou, S., et al. (2017). Comparative responses to silicon and selenium in relation to antioxidant enzyme system and the glutathione-ascorbate cycle in flowering Chinese cabbage (Brassica campestris L. ssp. chinensis var. utilis) under cadmium stress. Environ. Exp. Bot. 133, 1–11. doi: 10.1016/j.envexpbot.2016.09.005
Wu, Z., Wang, F., Liu, S., Du, Y., Li, F., Du, R., et al. (2016). Comparative responses to silicon and selenium in relation to cadmium uptake, compartmentation in roots, and xylem transport in flowering Chinese cabbage (Brassica campestris L. ssp. chinensis var. utilis) under cadmium stress. Environ. Exp. Bot. 131, 173–180. doi: 10.1016/j.envexpbot.2016.07.012
Wu, Z., Xu, S., Shi, H., Zhao, P., Liu, X., Li, F., et al. (2018). Comparison of foliar silicon and selenium on cadmium absorption, compartmentation, translocation and the antioxidant system in Chinese flowering cabbage. Ecotox. Environ. Safe. 166, 157–164. doi: 10.1016/j.ecoenv.2018.09.085
Xian, L., Zhang, Y., Cao, Y., Wan, T., Gong, Y., Dai, C., et al. (2020). Glutamate dehydrogenase plays an important role in ammonium detoxification by submerged macrophytes. Sci. Total Environ. 722, 137859. doi: 10.1016/j.scitotenv.2020.137859
Yang, L., LI, Y.-X., LI, Y.-X., TIAN, Z.-W., HU, J.-L., Adkins, S., et al. (2021). Changes of oxidative metabolism in the roots of wheat (Triticum aestivum L.) seedlings in response to elevated ammonium concentrations. J. Integr. Agr. 20, 1216–1228. doi: 10.1016/S2095-3119(20)63216-6
Yeo, A., Flowers, S., Rao, G., Welfare, K., Senanayake, N., and Flowers, T. (1999). Silicon reduces sodium uptake in rice (Oryza sativa L.) in saline conditions and this is accounted for by a reduction in the transpirational bypass flow. Plant Cell Environ. 22, 559–565. doi: 10.1046/j.1365-3040.1999.00418.x
Zaheer, M. M., Yasin, N. A., Ahmad, S. R., Khan, W. U., Ahmad, A., Ali, A., et al. (2018). Amelioration of cadmium stress in gladiolus (Gladiolus grandiflora L.) by application of potassium and silicon. J. Plant Nutr. 41, 461–476. doi: 10.1080/01904167.2017.1385808
Zhang, J., Wei, J., Li, D., Kong, X., Rengel, Z., Chen, L., et al. (2017). The role of the plasma membrane H+-ATPase in plant responses to aluminum toxicity. Front. Plant Sci. 8, 1757. doi: 10.3389/fpls.2017.01757
Zhang, L., Song, H., Li, B., Wang, M., Di, D., Lin, X., et al. (2021). Induction of S-nitrosoglutathione reductase protects root growth from ammonium toxicity by regulating potassium homeostasis in Arabidopsis and rice. J. Exp. Bot. 72, 4548–4564. doi: 10.1093/jxb/erab140
Zhang, Y., Xie, Z., Wang, Y., Su, P., An, L., and Gao, H. (2011). Effect of water stress on leaf photosynthesis, chlorophyll content, and growth of oriental lily. Russ. J. Plant Physiol. 58, 844–850. doi: 10.1134/S1021443711050268
Keywords: ion uptake, photosynthetic capacity, antioxidant enzyme activities, GS (glutamine synthetase), GOGAT (glutamate synthase), GDH (glutamate dehydrogenase)
Citation: Song J, Yang J and Jeong BR (2022) Silicon Mitigates Ammonium Toxicity in Cabbage (Brassica campestris L. ssp. pekinensis) ‘Ssamchu’. Front. Sustain. Food Syst. 6:922666. doi: 10.3389/fsufs.2022.922666
Received: 18 April 2022; Accepted: 27 May 2022;
Published: 21 June 2022.
Edited by:
Waqas ud din Khan, Government College University, Lahore, PakistanReviewed by:
Aqeel Ahmad, Chinese Academy of Sciences (CAS), ChinaHabib-ur-Rehman Athar, Bahauddin Zakariya University, Pakistan
Copyright © 2022 Song, Yang and Jeong. This is an open-access article distributed under the terms of the Creative Commons Attribution License (CC BY). The use, distribution or reproduction in other forums is permitted, provided the original author(s) and the copyright owner(s) are credited and that the original publication in this journal is cited, in accordance with accepted academic practice. No use, distribution or reproduction is permitted which does not comply with these terms.
*Correspondence: Byoung Ryong Jeong, brjeong@gnu.ac.kr