- Food Security and Safety Focus Area, Faculty of Natural and Agricultural Science, North-West University, Mafikeng, South Africa
Orphan legumes are now experiencing growing demand due to the constraints on available major food crops. However, due to focus on major food crops, little research has been conducted on orphan legumes compared to major food crops, especially in microbiome application to improve growth and yield. Recent developments have demonstrated the enormous potential of beneficial microbes in growth promotion and resistance to stress and diseases. Hence, the focus of this perspective is to examine the potential of plant growth promoting rhizobacteria (PGPR) to improve Bambara groundnut yield and quality. Further insights into the potential use of PGPR as a biological control agent in the crop are discussed. Finally, three PGPR genera commonly associated with plant growth and disease resistance (Bacillus, Pseudomonas, and Streptomyces) were highlighted as case studies for the growth promotion and disease control in BGN production.
Introduction
Food security is the ability of a population to access and afford enough food to live a healthy life (Olanrewaju et al., 2022). Climate change poses a significant threat to achieving food security, especially in developing countries. Rising temperatures and extreme weather events have caused droughts, floods, and increased soil salinity, which have reduced crop yields and caused food prices to spike. The impacts of climate change on our food system are already being felt in communities across developing countries (Lenaerts et al., 2019). Food security in sub-Saharan Africa is already threatened by climate change. As temperatures increase and rainfall patterns change, sub-Saharan Africa is expected to experience droughts and floods that will reduce crop yields, damage infrastructure, and drive-up food prices. These climate-induced shocks will have a devastating impact on people, many of whom live in poverty and cannot afford more food. The impact of climate change on food production in sub-Saharan Africa has led to programs such as the food for progress initiative, purchase for progress initiative, and other programs which are working to help smallholder farmers in sub-Saharan Africa improve their access to markets and invest in climate-resilient technologies, like drip irrigation systems (Crocker, 1986; Devereux, 2016).
Orphan legumes are a group of important but neglected crops that are an important part of a food-secure diet. Orphan legumes are also an important part of sub-Saharan African diets, as they are a nutritious source of protein, calcium, and other essential nutrients (Cullis and Kunert, 2017). In addition to climate change impacts, food security is threatened by a lack of research and investment in orphan legumes. The protein- and nutrient-rich seeds of orphan legume crops can be used to make flour and animal feed (Tadele, 2009; Adebowale et al., 2011; Adebola et al., 2017; Ruckle et al., 2017; Adeleke et al., 2018; Afolabi et al., 2018; Lambein et al., 2019; Oluwole et al., 2021). They include Bambara groundnut, African yam bean, winged bean, marama bean, grass pea, chick pea, and cowpea, among others.
In search of ways to mitigate climate change impact on food crops, use of beneficial microorganisms has become as important as any other form of plant improvement program. Many microorganisms have been used to make products, such as, nodumax and biofix, which have improved plant production (Akley et al., 2022). While there is a healthy debate about which plants are most important in the food system, the fact that plant-microbe interactions play a key role in the food system is undeniable. The variety of plants and microbial species that interact with one another in the food system is enormous. This means that an enormous amount of research is being done to identify the specific microbes that are involved in specific plant processes. The primary food source for these microbes in the rhizosphere is plant biomass. Plant microorganisms are a major component of the ecosystem and the food chain for all organisms on the planet. Incorporating plant-microbe interaction research into orphan legume crop production will be a great advancement in the production of these crops. We will focus on Bambara groundnut in this review as it is fast receiving improved research activities, but only one research on its microbiome has been reported so far (Ajilogba et al., 2022b).
Bambara groundnut (BGN) production is drawing attention, especially in sub-Saharan Africa, because it can be used as food, fiber, and medicine (Jideani and Jideani, 2021; Ajilogba et al., 2022a). The demand for BGN is increasing because of its high nutritional value and increasingly recognized medicinal value, which makes it a preferable alternative to the major crops.
Because of the absence of a well-annotated reference genome, studies have attempted to determine which elements of cultivation and genetics contribute to BGN traits by comparing them with the genomes of closely related crops (Ho et al., 2017). Hence, molecular markers have been developed in various studies (Molosiwa et al., 2015; Fatimah and Ardiarini, 2018). BGN yield is influenced by photoperiodism and plant density (Kendabie et al., 2020). However, little research has been conducted regarding the response of yield and other BGN traits to the application of plant-growth promoting rhizobacteria (PGPR), although studies have extensively demonstrated the importance of PGPR in the production of many crop species (Olanrewaju, 2016; Olanrewaju and Babalola, 2019a; Lee et al., 2020). For example, the priming of plant seeds with PGPR and the application of PGPR to plant roots reportedly stimulate plant growth through the production of nutrients and pathogen controlling metabolites. In addition, PGPR can improve tolerance to abiotic (e.g., drought and salinity) and biotic stresses (e.g., plant pathogens) (Babalola et al., 2019; Ojuederie et al., 2019; Olanrewaju et al., 2021a).
The exploitation of PGPR from orphan legume microbiome will play an important role in BGN production, and there is a clear need to better understand the relationship between the microbiome, BGN yield, and BGN disease resistance. This perspective summarizes the knowledge about the factors that contribute to BGN yield. In addition, we examine the potential role of PGPR, with a focus on Bacillus, Pseudomonas, and Streptomyces; three widely prevalent genera in achieving high yields, improved nutrient profiles, and disease resistance in BGN.
Strategies to increase BGN yield and quality
Production conditions that influence BGN yield and nutrient composition include plant genotype, environmental conditions (such as temperature, water availability, and fertilizer application), photoperiod, and plant development stage (Kendabie et al., 2020; Khan et al., 2020; Obidiebube et al., 2020; Olanrewaju et al., 2021b,c; Ajilogba et al., 2022b). At the physiological level, plant growth regulators can also affect the nutrient composition.
Plant-growth promoting rhizobacteria for BGN production
Plant growth-promoting rhizobacteria are present in plant roots and have been associated with plant growth by providing nutrients for plants, production of growth hormones, induction of the plant immune system, and production of metabolites which support plants against pathogens (Olanrewaju, 2016; Olanrewaju et al., 2017; Backer et al., 2018; Etesami and Maheshwari, 2018; Babalola et al., 2019; Singh et al., 2019; Hakim et al., 2021). PGPR is important for sustainable crop production and achieving food security (Ajilogba et al., 2022a). PGPR for crop yield and quality increase as well as disease/pathogen control has been extensively studied in many crops such as soybean (Silva et al., 2019; Riviezzi et al., 2021), rice (Raja et al., 2017; Xu et al., 2019), maize (Ke et al., 2019; Olanrewaju and Babalola, 2019a; Liu et al., 2020), wheat (Kumar et al., 2018; Ma et al., 2021), chickpea (Laranjeira et al., 2021; Khalifa et al., 2022), and cowpea (Kanthaiah and Velu, 2019; Kumar et al., 2021).
Yield and quality enhancements associated with plant-growth promoting rhizobacteria
Compared to the major food crops, there is relatively little data to support the use of PGPR on BGN production. Most research data on this crop has emerged from evaluation studies for growth traits, nutrient and antinutrient components, antioxidant compositions, and recently, medicinal uses. In the study by Ikenganyia et al. (2017), the effects of bioinoculant methods (soil inoculation and seed inoculation methods) on BGN growth and yield were compared. Both methods increased the yield of BGN, with the soil inoculation method having the highest yield result. Another study by Gomoung et al. (2017) reported the effect of cross inoculation of groundnut and BGN rhizobium on the growth and yield of both crops. Their study further proved that rhizobacteria on a plant species can improve the growth and yield of another plant species. A previous study showed that PGPR improved nutrient acquisition in BGN (Oyewole et al., 2018). Many studies have shown the effect of PGPR on plant growth and nutrient composition (Bakhshandeh et al., 2020; Castaldi et al., 2021; Guo et al., 2021; Kushwaha et al., 2021; Laranjeira et al., 2021); we can therefore postulate that PGPR will improve the nutrient composition and yield of BGN. It is important to determine the impact of PGPR on BGN growth and nutrient composition at various growth stages and different environments.
Our laboratory has already illustrated that bacteria isolated from a plant species or habitat can improve growth promotion and activate stress responses in other plant species (Ndeddy Aka and Babalola, 2016; Olanrewaju and Babalola, 2019a). This means that the tested PGPR can improve BGN growth either in single inoculation or in consortia (Olanrewaju and Babalola, 2019a). As a result, we believe that future research will confirm that PGPR-based inoculants can impact BGN nutrient composition, increase the growth and yield of BGN, promote BGN stress tolerance, and control pathogens.
Biological control and disease resistance associated with plant-growth promoting rhizobacteria
Streptomycetes, Bacillus, Pseudomonas, Rhizobium, and Azospirillum are widely used as biocontrol agents (Olanrewaju et al., 2017; Olanrewaju and Babalola, 2019b). Many studies have reported their use in controlling various plant pathogens. For example, by producing the metabolite, wuyiencin, Streptomyces albulus CK-15 controls powdery mildew of cucumber (Yang et al., 2021), while Bacillus (Cui et al., 2019, 2020; Castaldi et al., 2021), Rhizobium (Wong et al., 2021; Kawaguchi and Noutoshi, 2022), and Pseudomonas (Omoboye et al., 2019; Khalifa et al., 2022) species have been reported in the biocontrol of some plant species.
PGPR acts via direct and indirect mechanisms to control plant pathogens. Antibiosis and the production of secondary metabolites that act as toxins to pathogens are examples of direct mechanisms, whereas indirect mechanisms include nutrient competition and the induction of induced systemic resistance (ISR) (Beneduzi et al., 2012; Olanrewaju et al., 2017, 2019; Backer et al., 2018). ISR is strongly connected to the jasmonic acid and ethylene-sensitive pathways (Van Der Ent et al., 2009; Olanrewaju et al., 2019). An effective PGPR must be able to properly colonize its host (Olanrewaju and Babalola, 2019b); hence, PGPR-induced ISR is largely dependent on the rhizobacterium-colonizing ability (Beneduzi et al., 2012). Upon successful activation, ISR can improve the plant's defense capabilities by activating the expression of defense-related genes. Through the production of hormones such as indole acetic acid and gibberellin, PGPR increases the plant's ability to defend against pathogens. ACC-producing PGPR reportedly improves the plant's capacity to produce ethylene and increases its immunity by the induction of ISR (Glick, 2005). However, triggering ethylene and jasmonic acid-dependent plant responses to pathogens does not always correlate with an increase in the phytohormones, as reported in the study by Beneduzi et al. (2012). Hence, PGPR research on BGN should focus on the ability of PGPR to control pathogen infection in BGN through the activation of ISR.
Pathogen control in BGN cultivation: insight into the potential of PGPR applications
The ability of BGN to produce its seeds embedded in the soil has improved its resistance to pest attack but not pathogens. It hosts pathogens varying from bacteria, fungal, virus, and nematode origins, and they have a significant economic impact through yield loss (Figure 1) (Olanrewaju et al., 2022). Brink et al. (2006) reported fungal diseases such as cercospora leaf spot (Cercospora spp.), powdery mildew (Erysiphe polygoni), and Fusarium wilt (Fusarium oxysporum). Fourie et al. (2017) also reported the activities of a root-knot nematode (Meloidogyne javanica) on the plant. Pengnoo et al. (2006) also reported the occurrence of leaf blight disease in BGN. In their study, they isolated Bacillus sp. strains that were used to control the disease.
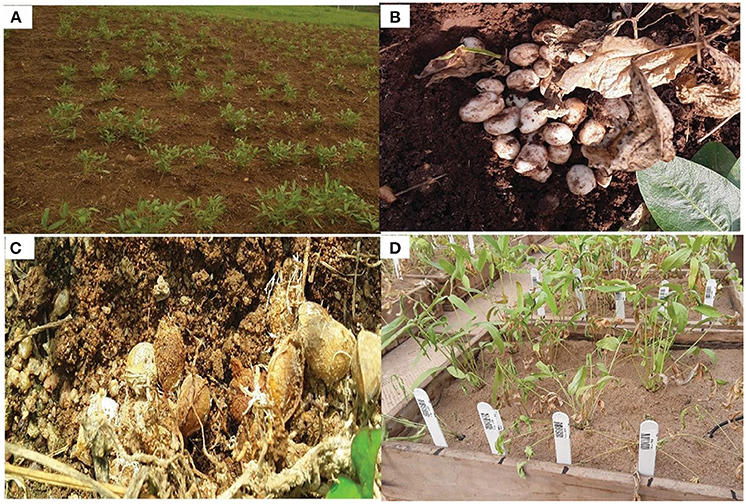
Figure 1. (A) Bambara groundnut (BGN) in the field; (B) healthy matured BGN pods during harvest; (C) infected BGN pods; (D) drought stressed BGN plants. [Source: Olanrewaju et al., 2022].
It is important to effectively address these threats, to prevent yield losses in BGN production. Biocontrol of pathogens has more advantages for plants, humans, animals, and the environment compared to chemical controls. Bacillus subtilis effectively controls leaf blight disease in BGN (Pengnoo et al., 2006), Meloidogyne incognita on Capsicum annuum cv. Qiemen (Cao et al., 2019) (Table 1).
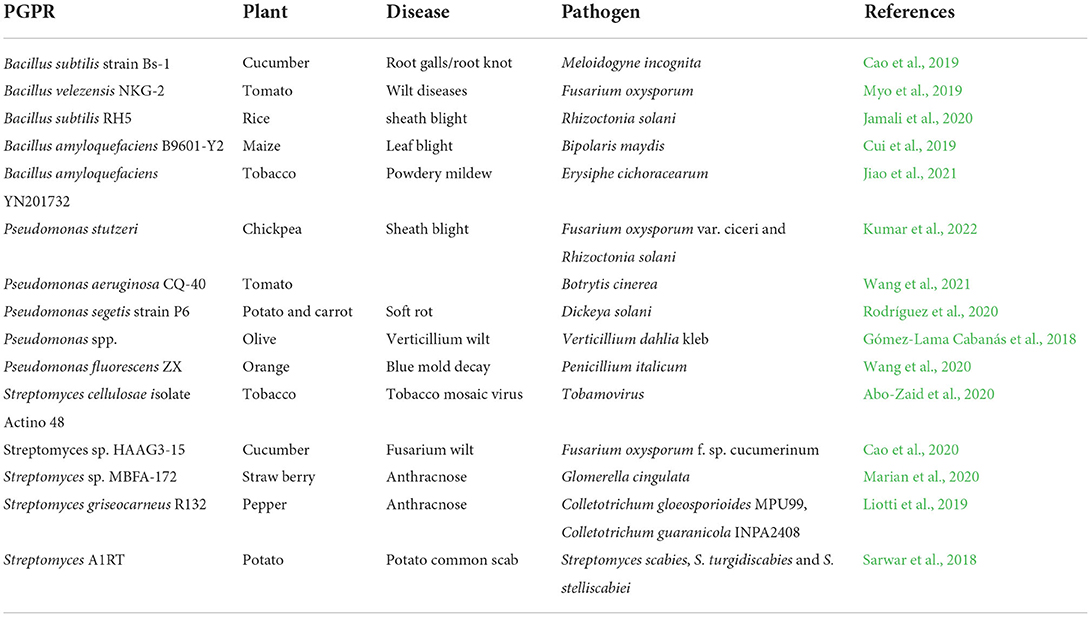
Table 1. Biocontrol activities of Bacillus spp., Pseudomonas spp., and Streptomyces spp. on various plants.
These results in Table 1 suggest that inoculating BGN with PGPR may assist in controlling disease infestations, representing a substantial advantage over currently available chemical control methods. In addition, consumption of chemical residues through the seeds because of being washed into the soil to the seeds can be eliminated with the application of an effective biocontrol agent. This can be applied as a root trench in place of spraying to minimize damage caused by sprays on young leaves.
Examples of widely prevalent phytomicrobiome members: Pseudomonas, Bacillus, and Streptomyces for growth promotion and disease control in BGN
Pseudomonas spp., Bacillus spp., and Streptomyces spp. promote plant growth by synthesizing phytohormones, fixing nitrogen, and solubilizing phosphate for plant use (Santoyo et al., 2012; Olanrewaju and Babalola, 2019b). Pseudomonas and Streptomyces are good plant colonizers and inhibitors of plant pathogens (Olanrewaju et al., 2017). They help the hosts to control pathogens by producing antipathogenic compounds such as siderophores, cyanides, DAPG, antibiotics, lipopeptides, and polysaccharides (Beneduzi et al., 2012; Santoyo et al., 2012). In addition, Bacillus make use of their spores as biocontrol agents (Ji et al., 2013).
Studies on various plant species have shown that Bacillus spp., Pseudomonas spp., and Streptomyces spp. can promote plant growth and suppress/control plant diseases (Cui et al., 2019, 2020; Olanrewaju and Babalola, 2019a; Xu et al., 2019; Liu et al., 2020; Yang et al., 2021). The success of plant growth promotion and disease control by PGPR is host-dependent. Studies on Bacillus spp. as biocontrol agents have mainly focused on activation of ISR to improve plant resilience, direct plant growth promotion, and aspects of microbial ecology, while studies on Streptomyces spp. have mainly focused on its biocontrol ability through the production of secondary metabolites and antibiotics (Olanrewaju and Babalola, 2019b). Inhibition of Fusarium and Armillaria pine rot diseases was inhibited by Streptomyces kasugaensis in the study reported by De Vasconcellos and Cardoso (2009). Another study by Wonglom et al. (2019) reported the biological control of leaf spot diseases of Brassica rapa subsp. pekinensis by Streptomyces angustmyceticus NR8-2.
Overall, previous studies have shown that these three PGPR genera have great promise in promoting plant growth and biocontrol of plant pathogens (Raja et al., 2017; Babalola et al., 2019; Ke et al., 2019; Olanrewaju and Babalola, 2019a,b; Omoboye et al., 2019; Xu et al., 2019; Cui et al., 2020; Guo et al., 2021; Kushwaha et al., 2021; Olanrewaju et al., 2021a; Yang et al., 2021; Khalifa et al., 2022). However, their applications on BGN have not been exploited. Based on a recent study on the microbiome of BGN at various growth stages, these genera are well represented in the rhizosphere of BGN (Ajilogba et al., 2022b). It would be interesting to determine if any strains of these genera can be formulated and tailored specifically for the improvement of BGN yield and disease control.
Conclusions and prospects
BGN is a critical crop for achieving food and nutrition security, particularly in Sub-Saharan Africa and other developing world regions where it is cultivated. Hence, BGN is fast becoming an important crop. Hence, BGN is fast becoming an important crop. PGPR has been associated with benefits such as nutrient mobilization, phytohormone production, stress mitigation, and biocontrol abilities. As a result, studying PGPR inoculants on BGN can increase yield in an environmentally sustainable manner. The microbiome has enormous potential for improving BGN production by increasing yield, mitigating drought stress, and controlling disease.
Data availability statement
The original contributions presented in the study are included in the article/supplementary material, further inquiries can be directed to the corresponding author/s.
Author contributions
OSO researched the data, wrote, and edited the manuscript. OOB supervised the writing of the manuscript. Both authors contributed to the article and approved the submitted version.
Acknowledgments
North-West University is gratefully acknowledged for a school bursary to OSO.
Conflict of interest
The authors declare that the research was conducted in the absence of any commercial or financial relationships that could be construed as a potential conflict of interest.
Publisher's note
All claims expressed in this article are solely those of the authors and do not necessarily represent those of their affiliated organizations, or those of the publisher, the editors and the reviewers. Any product that may be evaluated in this article, or claim that may be made by its manufacturer, is not guaranteed or endorsed by the publisher.
References
Abo-Zaid, G. A., Matar, S. M., and Abdelkhalek, A. (2020). Induction of plant resistance against tobacco mosaic virus using the biocontrol agent Streptomyces cellulosae isolate Actino 48. Agronomy 10, 1620. doi: 10.3390/agronomy10111620
Adebola, M. I., Adelanwa, M. A., Mohammed, M. S., and Esson, A. E. (2017). Variation mineral compositions of some Bambara groundnut (Vigna subterranea (L.) Verdc.) accessions. Recent Res. Sci. Technol. 9, 06–09. doi: 10.25081/rrst.2017.9.3355
Adebowale, Y. A., Schwarzenbolz, U., and Henle, T. (2011). Protein isolates from Bambara groundnut (Voandzeia Subterranean L.): chemical characterization and functional properties. Int. J. Food Prop. 14, 758–775. doi: 10.1080/10942910903420743
Adeleke, O. R., Adiamo, O. Q., and Fawale, O. S. (2018). Nutritional, physicochemical, and functional properties of protein concentrate and isolate of newly-developed Bambara groundnut (Vigna subterrenea L.) cultivars. Food Sci. Nutr. 6, 229–242. doi: 10.1002/fsn3.552
Afolabi, T. A., Opara, A. O., Kareem, S. O., and Oladoyinbo, F. O. (2018). In vitro digestibility of hydrothermally modified Bambara groundnut (Vigna subterranea L.) starch and flour. Food Sci. Nutr. 6, 36–46. doi: 10.1002/fsn3.510
Ajilogba, C. F., Olanrewaju, O. S., and Babalola, O. O. (2022a). Improving Bambara groundnut production: insight into the role of omics and beneficial bacteria. Front. Plant Sci. 13, 836133. doi: 10.3389/fpls.2022.836133
Ajilogba, C. F., Olanrewaju, O. S., and Babalola, O. O. (2022b). Plant growth stage drives the temporal and spatial dynamics of the bacterial microbiome in the rhizosphere of Vigna subterranea. Front. Microbiol. 13, 825377. doi: 10.3389/fmicb.2022.825377
Akley, E. K., Rice, C. W., Adotey, N., Ampim, P. A.Y., Vara Prasad, P. V., Danquah, E. O., et al. (2022). Residual Bradyrhizobium inoculation effects on soybean performance and selected soil health parameters. Agron. J. 114, 1627–1641. doi: 10.1002/agj2.21037
Babalola, O. O., Ayangbenro, A. S., and Olanrewaju, O. S. (2019). Draft genome sequences of three rhizospheric plant growth-promoting bacteria. Microbiol. Resour. Announc. 8, e00455-00419. doi: 10.1128/MRA.00455-19
Backer, R., Rokem, J. S., Ilangumaran, G., Lamont, J., Praslickova, D., Ricci, E., et al. (2018). Plant growth-promoting rhizobacteria: context, mechanisms of action, and roadmap to commercialization of biostimulants for sustainable agriculture. Front. Plant Sci. 9, 1473. doi: 10.3389/fpls.2018.01473
Bakhshandeh, E., Pirdashti, H., Shahsavarpour Lendeh, K., Gilani, Z., Yaghoubi Khanghahi, M., and Crecchio, C. (2020). Effects of plant growth promoting microorganisms inoculums on mineral nutrition, growth and productivity of rice (Oryza sativa L.). J. Plant Nutr. 43, 1643–1660. doi: 10.1080/01904167.2020.1739297
Beneduzi, A., Ambrosini, A., and Passaglia, L. M. (2012). Plant growth-promoting rhizobacteria (PGPR): their potential as antagonists and biocontrol agents. Genet. Mol. Biol. 35, 1044–1051. doi: 10.1590/S1415-47572012000600020
Brink, M., Ramolemana, G. M., and Sibuga, K. P. (2006). “Vigna subterranea (L.) Verdc,” in Plant Resources of Tropical Africa/Ressources végétales de l'Afrique tropicale, eds M. Brink, and G. Belay (Wageningen: PROTA), 27.
Cao, H., Jiao, Y., Yin, N., Li, Y., Ling, J., Mao, Z., et al. (2019). Analysis of the activity and biological control efficacy of the Bacillus subtilis strain Bs-1 against Meloidogyne incognita. Crop Protect. 122, 125–135. doi: 10.1016/j.cropro.2019.04.021
Cao, P., Li, C., Wang, H., Yu, Z., Xu, X., Wang, X., et al. (2020). Community structures and antifungal activity of root-associated endophytic actinobacteria in healthy and diseased cucumber plants and Streptomyces sp. HAAG3-15 as a promising biocontrol agent. Microorganisms 8, 236. doi: 10.3390/microorganisms8020236
Castaldi, S., Petrillo, C., Donadio, G., Piaz, F. D., Cimmino, A., Masi, M., et al. (2021). Plant growth promotion function of Bacillus sp. strains isolated from salt-pan rhizosphere and their biocontrol potential against Macrophomina phaseolina. Int. J. Mol. Sci. 22, 3324. doi: 10.3390/ijms22073324
Crocker, C. A. (1986). FY 1987 Assistance Request for Sub-Saharan Africa. Washington, DC: US Department of State, Bureau of Public Affairs, Office of Public.
Cui, L., Yang, C., Wei, L., Li, T., and Chen, X. (2020). Isolation and identification of an endophytic bacteria Bacillus velezensis 8-4 exhibiting biocontrol activity against potato scab. Biol. Control 141, 104156. doi: 10.1016/j.biocontrol.2019.104156
Cui, W., He, P., Munir, S., He, P., Li, X., Li, Y., et al. (2019). Efficacy of plant growth promoting bacteria Bacillus amyloliquefaciens B9601-Y2 for biocontrol of southern corn leaf blight. Biol. Control 139, 104080. doi: 10.1016/j.biocontrol.2019.104080
Cullis, C., and Kunert, K. J. (2017). Unlocking the potential of orphan legumes. J. Exp. Bot. 68, 1895–1903. doi: 10.1093/jxb/erw437
De Vasconcellos, R. L. F., and Cardoso, E. J. B. N. (2009). Rhizospheric streptomycetes as potential biocontrol agents of Fusarium and Armillaria pine rot and as PGPR for Pinus taeda. BioControl 54, 807–816. doi: 10.1007/s10526-009-9226-9
Devereux, S. (2016). Social protection for enhanced food security in sub-Saharan Africa. Food Policy 60, 52–62. doi: 10.1016/j.foodpol.2015.03.009
Etesami, H., and Maheshwari, D. K. (2018). Use of plant growth promoting rhizobacteria (PGPRs) with multiple plant growth promoting traits in stress agriculture: action mechanisms and future prospects. Ecotoxicol. Environ. Saf. 156, 225–246. doi: 10.1016/j.ecoenv.2018.03.013
Fatimah, S., and Ardiarini, N. (2018). Genetic diversity of Madurese Bambara groundnut (Vigna subterranea L. Verdc.) lines based on morphological and RAPD markers. SABRAO J. Breed. Genet. 50, 101–114.
Fourie, H., Mc Donald, A. H., Steenkamp, S., and De Waele, D. (2017). “Nematode pests of leguminous and oilseed crops,” in Nematology in South Africa: A View From the 21st Century (Springer), 201–230. doi: 10.1007/978-3-319-44210-5_9
Glick, B. R. (2005). Modulation of plant ethylene levels by the bacterial enzyme ACC deaminase. FEMS Microbiol. Lett. 251, 1–7. doi: 10.1016/j.femsle.2005.07.030
Gómez-Lama Cabanás, C., Legarda, G., Ruano-Rosa, D., Pizarro-Tobías, P., Valverde-Corredor, A., Niqui, J. L., et al. (2018). Indigenous Pseudomonas spp. Strains from the Olive (Olea europaea L.) rhizosphere as effective biocontrol agents against Verticillium dahliae: from the host roots to the bacterial genomes. Front. Microbiol. 9, 00277. doi: 10.3389/fmicb.2018.00277
Gomoung, D., Mbailao, M., Toukam, S. T., and Ngakou, A. (2017). Influence of cross-inoculation on groundnut and Bambara groundnut-rhizobium symbiosis: contribution to plant growth and yield in the field at Sarh (Chad) and Ngaoundere (Cameroon). Am. J. Plant Sci. 8, 1953–1966. doi: 10.4236/ajps.2017.88131
Guo, Q., Sun, Y., Shi, M., Han, X., Jing, Y., Li, Y., et al. (2021). Pseudomonas koreensis promotes tomato growth and shows potential to induce stress tolerance via auxin and polyphenol-related pathways. Plant Soil 462, 141–158. doi: 10.1007/s11104-021-04837-9
Hakim, S., Naqqash, T., Nawaz, M. S., Laraib, I., Siddique, M. J., Zia, R., et al. (2021). Rhizosphere engineering with plant growth-promoting microorganisms for agriculture and ecological sustainability. Front. Sustain. Food Syst. 5, 1–23. doi: 10.3389/fsufs.2021.617157
Ho, W. K., Chai, H. H., Kendabie, P., Ahmad, N. S., Jani, J., Massawe, F., et al. (2017). Integrating genetic maps in Bambara groundnut [Vigna subterranea (L) Verdc.] and their syntenic relationships among closely related legumes. BMC Genom. 18, 1–9. doi: 10.1186/s12864-016-3393-8
Ikenganyia, E., Anikwe, M., and Ngwu, O. (2017). Influence of rhizobacteria inoculant application methods and phosphate fertilizer rates on dry matter accumulation, yield of Bambara groundnut [Vigna subterranea (L.) Verdc] and soil total nitrogen content in a degraded Ultisol in Southeast Nigeria. Agrotechnology 6, 1–7. doi: 10.9734/IJPSS/2017/32606
Jamali, H., Sharma, A., Roohi, and Srivastava, A. K. (2020). Biocontrol potential of Bacillus subtilis RH5 against sheath blight of rice caused by Rhizoctonia solani. J. Basic Microbiol. 60, 268–280. doi: 10.1002/jobm.201900347
Ji, S. H., Paul, N. C., Deng, J. X., Kim, Y. S., Yun, B.-S., and Yu, S. H. (2013). Biocontrol activity of Bacillus amyloliquefaciens CNU114001 against fungal plant diseases. Mycobiology 41, 234–242. doi: 10.5941/MYCO.2013.41.4.234
Jiao, R., Cai, Y., He, P., Munir, S., Li, X., Wu, Y., et al. (2021). Bacillus amyloliquefaciens YN201732 produces lipopeptides with promising biocontrol activity against fungal pathogen Erysiphe cichoracearum. Front. Cell. Infect. Microbiol. 11, 598999. doi: 10.3389/fcimb.2021.598999
Jideani, V. A., and Jideani, A. I. (2021). “Ethnonutritional and ethnomedicinal uses of Bambara groundnut,” in Bambara groundnut: Utilization and Future Prospects, ed C. F. Diedericks (Switzerland: Springer), 49–60. doi: 10.1007/978-3-030-76077-9_4
Kanthaiah, K., and Velu, R. K. (2019). Characterization of the bioactive metabolite from a plant growth promoting rhizobacteria Pseudomonas aeruginosa VRKK1 and exploitation of antibacterial behaviour against Xanthomonas campestris a causative agent of bacterial blight disease in cowpea. Arch. Phytopathol. Plant Protect. 55, 1–18. doi: 10.1080/03235408.2018.1557883
Kawaguchi, A., and Noutoshi, Y. (2022). Migration of biological control agent Rhizobium vitis strain ARK-1 in grapevine stems and inhibition of galls caused by tumorigenic strain of R. vitis. J. Gen. Plant Pathol. 88, 63–68. doi: 10.1007/s10327-021-01043-4
Ke, X., Feng, S., Wang, J., Lu, W., Zhang, W., Chen, M., et al. (2019). Effect of inoculation with nitrogen-fixing bacterium Pseudomonas stutzeri A1501 on maize plant growth and the microbiome indigenous to the rhizosphere. Syst. Appl. Microbiol. 42, 248–260. doi: 10.1016/j.syapm.2018.10.010
Kendabie, P., Jørgensen, S. T., Massawe, F., Fernandez, J., Azam-Ali, S., and Mayes, S. (2020). Photoperiod control of yield and sink capacity in Bambara groundnut (Vigna subterranea) genotypes. Food Energy Secur. 9, e240. doi: 10.1002/fes3.240
Khalifa, M. W., Rouag, N., and Bouhadida, M. (2022). Evaluation of the antagonistic effect of Pseudomonas rhizobacteria on Fusarium wilt of chickpea. Agriculture 12, 429. doi: 10.3390/agriculture12030429
Khan, M. M. H., Rafii, M. Y., Ramlee, S. I., Jusoh, M., and Mamun, A. (2020). Genetic variability, heritability, and clustering pattern exploration of Bambara groundnut (Vigna subterranea L. Verdc) accessions for the perfection of yield and yield-related traits. BioMed Res. Int. 2020, 2195797. doi: 10.1155/2020/2195797
Kumar, A., Chandra, D., and Sharma, A. K. (2021). Impact of seed applied rhizobacterial inoculants on growth of wheat (Triticum aestivum) and Cowpea [Vigna unguiculata] and their influence on rhizospheric microbial diversity. Agric. Res. 11, 1–14. doi: 10.1007/s40003-021-00546-y
Kumar, A., Kumar, S. P. J., Chintagunta, A. D., Agarwal, D. K., Pal, G., Singh, A. N., et al. (2022). Biocontrol potential of Pseudomonas stutzeri endophyte from Withania somnifera (Ashwagandha) seed extract against pathogenic Fusarium oxysporum and Rhizoctonia solani. Arch. Phytopathol. Plant Protect. 55, 1–18. doi: 10.1080/03235408.2021.1983384
Kumar, P., Thakur, S., Dhingra, G., Singh, A., Pal, M. K., Harshvardhan, K., et al. (2018). Inoculation of siderophore producing rhizobacteria and their consortium for growth enhancement of wheat plant. Biocatal. Agric. Biotechnol. 15, 264–269. doi: 10.1016/j.bcab.2018.06.019
Kushwaha, P., Srivastava, R., Pandiyan, K., Singh, A., Chakdar, H., Kashyap, P. L., et al. (2021). Enhancement in plant growth and zinc biofortification of chickpea (Cicer arietinum L.) by Bacillus altitudinis. J. Soil Sci. Plant Nutr. 21, 922–935. doi: 10.1007/s42729-021-00411-5
Lambein, F., Travella, S., Kuo, Y.-H., Van Montagu, M., and Heijde, M. (2019). Grass pea (Lathyrus sativus L.): orphan crop, nutraceutical or just plain food? Planta 250, 821–838. doi: 10.1007/s00425-018-03084-0
Laranjeira, S., Fernandes-Silva, A., Reis, S., Torcato, C., Raimundo, F., Ferreira, L., et al. (2021). Inoculation of plant growth promoting bacteria and Arbuscular mycorrhizal fungi improve chickpea performance under water deficit conditions. Appl. Soil Ecol. 164, 103927. doi: 10.1016/j.apsoil.2021.103927
Lee, S., Tr?nh, C. S., Lee, W. J., Jeong, C. Y., Truong, H. A., Chung, N., et al. (2020). Bacillus subtilis strain L1 promotes nitrate reductase activity in Arabidopsis and elicits enhanced growth performance in Arabidopsis, lettuce, and wheat. J. Plant Res. 133, 231–244. doi: 10.1007/s10265-019-01160-4
Lenaerts, B., Collard, B. C., and Demont, M. (2019). Improving global food security through accelerated plant breeding. Plant Sci. 287, 110207. doi: 10.1016/j.plantsci.2019.110207
Liotti, R. G., Da Silva Figueiredo, M. I., and Soares, M. A. (2019). Streptomyces griseocarneus R132 controls phytopathogens and promotes growth of pepper (Capsicum annuum). Biol. Control 138, 104065. doi: 10.1016/j.biocontrol.2019.104065
Liu, Y., Teng, K., Wang, T., Dong, E., Zhang, M., Tao, Y., et al. (2020). Antimicrobial Bacillus velezensis HC6: production of three kinds of lipopeptides and biocontrol potential in maize. J. Appl. Microbiol. 128, 242–254. doi: 10.1111/jam.14459
Ma, Z., Yi, Z., Bayar, K., Fu, Y., and Liu, H. (2021). Community dynamics in rhizosphere microorganisms at different development stages of wheat growing in confined isolation environments. Appl. Microbiol. Biotechnol. 105, 3843–3857. doi: 10.1007/s00253-021-11283-1
Marian, M., Ohno, T., Suzuki, H., Kitamura, H., Kuroda, K., and Shimizu, M. (2020). A novel strain of endophytic Streptomyces for the biocontrol of strawberry anthracnose caused by Glomerella cingulata. Microbiol. Res. 234, 126428. doi: 10.1016/j.micres.2020.126428
Molosiwa, O. O., Aliyu, S., Stadler, F., Mayes, K., Massawe, F., Kilian, A., et al. (2015). SSR marker development, genetic diversity and population structure analysis of Bambara groundnut [Vigna subterranea (L.) Verdc.] landraces. Genet. Resour. Crop Evol. 62, 1225–1243. doi: 10.1007/s10722-015-0226-6
Myo, E. M., Liu, B., Ma, J., Shi, L., Jiang, M., Zhang, K., et al. (2019). Evaluation of Bacillus velezensis NKG-2 for bio-control activities against fungal diseases and potential plant growth promotion. Biol. Control 134, 23–31. doi: 10.1016/j.biocontrol.2019.03.017
Ndeddy Aka, R. J., and Babalola, O. O. (2016). Effect of bacterial inoculation of strains of Pseudomonas aeruginosa, Alcaligenes feacalis and Bacillus subtilis on germination, growth and heavy metal (cd, cr, and ni) uptake of Brassica juncea. Int. J. Phytoremediation 18, 200–209. doi: 10.1080/15226514.2015.1073671
Obidiebube, E., Eruotor, P., Akparobi, S., Okolie, H., and Obasi, C. (2020). Assessment of Bambara groundnut (Vigna Subterranea (L) Verdc) varieties for adaptation to rainforest agro-ecological zone of Anambra state of Nigeria. Crops 5, 1–6. doi: 10.20448/803.5.1.1.6
Ojuederie, O. B., Olanrewaju, O. S., and Babalola, O. O. (2019). Plant growth promoting rhizobacterial mitigation of drought stress in crop plants: implications for sustainable agriculture. Agronomy 9, 712. doi: 10.3390/agronomy9110712
Olanrewaju, O. S. (2016). Isolation of Bacterial Strains for Improved Maize Production (MSc). North-West University.
Olanrewaju, O. S., Ayangbenro, A. S., Glick, B. R., and Babalola, O. O. (2019). Plant health: feedback effect of root exudates-rhizobiome interactions. Appl. Microbiol. Biotechnol. 103, 1155–1166. doi: 10.1007/s00253-018-9556-6
Olanrewaju, O. S., Ayilara, M. S., Ayangbenro, A. S., and Babalola, O. O. (2021a). Genome mining of three plant growth-promoting Bacillus species from Maize rhizosphere. Appl. Biochem. Biotechnol. 193, 1–21. doi: 10.1007/s12010-021-03660-3
Olanrewaju, O. S., and Babalola, O. O. (2019a). Bacterial consortium for improved Maize (Zea mays L.) production. Microorganisms 7, 519. doi: 10.3390/microorganisms7110519
Olanrewaju, O. S., and Babalola, O. O. (2019b). Streptomyces: implications and interactions in plant growth promotion. Appl. Microbiol. Biotechnol. 103, 1179–1188. doi: 10.1007/s00253-018-09577-y
Olanrewaju, O. S., Glick, B. R., and Babalola, O. O. (2017). Mechanisms of action of plant growth promoting bacteria. World J. Microbiol. Biotechnol. 33, 197. doi: 10.1007/s11274-017-2364-9
Olanrewaju, O. S., Oyatomi, O., Babalola, O. O., and Abberton, M. (2021b). Genetic diversity and environmental influence on growth and yield parameters of Bambara groundnut. Front. Plant Sci. 12, 796352. doi: 10.3389/fpls.2021.796352
Olanrewaju, O. S., Oyatomi, O., Babalola, O. O., and Abberton, M. (2021c). GGE biplot analysis of genotype × environment interaction and yield stability in Bambara groundnut. Agronomy 11, 1839. doi: 10.3390/agronomy11091839
Olanrewaju, O. S., Oyatomi, O., Babalola, O. O., and Abberton, M. (2022). Breeding potentials of Bambara groundnut for food and nutrition security in the face of climate change. Front. Plant Sci. 12, 798993. doi: 10.3389/fpls.2021.798993
Oluwole, O. O., Aworunse, O. S., Aina, A. I., Oyesola, O. L., Popoola, J. O., Oyatomi, O. A., et al. (2021). A review of biotechnological approaches towards crop improvement in African yam bean (Sphenostylis stenocarpa Hochst. Ex A. Rich.). Heliyon 7, e08481. doi: 10.1016/j.heliyon.2021.e08481
Omoboye, O. O., Oni, F. E., Batool, H., Yimer, H. Z., De Mot, R., and Höfte, M. (2019). Pseudomonas cyclic lipopeptides suppress the rice blast fungus Magnaporthe oryzae by induced resistance and direct antagonism. Front. Plant Sci. 10, 901. doi: 10.3389/fpls.2019.00901
Oyewole, O., Oladele, S., Awodun, M., and Ajala, R. (2018). Evaluation of four microbial bio-effectors for growth promotion and nutrient acquisition in bambara nut Vigna subterranea (L.) Verdc. (Fabales: Fabaceae) without mineral fertilization. Braz. J. Biol. Sci. 5, 837–849. doi: 10.21472/bjbs.051119
Pengnoo, A., Wiwattanapattapee, R., Chumthong, A., and Kanjanamaneesathian, M. (2006). Bacterial Antagonist as Seed Treatment to Control Leaf Blight Disease of Bambara Groundnut (Vigna subterranea). World J. Microbiol. Biotechnol. 22, 9–14. doi: 10.1007/s11274-005-2820-9
Raja, K., Karthikeyan, M., Johnson, I., Latha, P., and Saravanakumar, D. (2017). Antagonistic ACC deaminase producing Pseudomonas fluorescens with polymer seed coating for the management of rice fallow black gram diseases. Adv. Res. 10, 1–12. doi: 10.9734/AIR/2017/34227
Riviezzi, B., García-Laviña, C. X., Morel, M. A., and Castro-Sowinski, S. (2021). Facing the communication between soybean plants and microorganisms (Bradyrhizobium and Delftia) by quantitative shotgun proteomics. Symbiosis 83, 293–304. doi: 10.1007/s13199-021-00758-4
Rodríguez, M., Torres, M., Blanco, L., Béjar, V., Sampedro, I., and Llamas, I. (2020). Plant growth-promoting activity and quorum quenching-mediated biocontrol of bacterial phytopathogens by Pseudomonas segetis strain P6. Sci. Rep. 10, 4121. doi: 10.1038/s41598-020-61084-1
Ruckle, M. E., Meier, M. A., Frey, L., Eicke, S., Kölliker, R., Zeeman, S. C., et al. (2017). Diurnal leaf starch content: an orphan trait in forage legumes. Agronomy 7, 16. doi: 10.3390/agronomy7010016
Santoyo, G., Orozco-Mosqueda, M. D. C., and Govindappa, M. (2012). Mechanisms of biocontrol and plant growth-promoting activity in soil bacterial species of Bacillus and Pseudomonas: a review. Biocontrol Sci. Technol. 22, 855–872. doi: 10.1080/09583157.2012.694413
Sarwar, A., Latif, Z., Zhang, S., Zhu, J., Zechel, D. L., and Bechthold, A. (2018). Biological control of potato common scab with rare isatropolone C compound produced by plant growth promoting Streptomyces A1RT. Front. Microbiol. 9, 01126. doi: 10.3389/fmicb.2018.01126
Silva, E. R., Zoz, J., Oliveira, C. E. S., Zuffo, A. M., Steiner, F., Zoz, T., et al. (2019). Can co-inoculation of Bradyrhizobium and Azospirillum alleviate adverse effects of drought stress on soybean (Glycine max L. Merrill.)? Arch. Microbiol. 201, 325–335. doi: 10.1007/s00203-018-01617-5
Singh, M., Singh, D., Gupta, A., Pandey, K. D., Singh, P., and Kumar, A. (2019). “Plant growth promoting rhizobacteria: application in biofertilizers and biocontrol of phytopathogens,” in PGPR Amelioration in Sustainable Agriculture (Cambridge, MA: Elsevier), 41–66. doi: 10.1016/B978-0-12-815879-1.00003-3
Tadele, Z. (2009). Role of orphan crops in enhancing and diversifying food production in Africa. Afr. Technol. Dev. Forum J. 6, 9–15.
Van Der Ent, S., Van Wees, S. C., and Pieterse, C. M. (2009). Jasmonate signaling in plant interactions with resistance-inducing beneficial microbes. Phytochemistry 70, 1581–1588. doi: 10.1016/j.phytochem.2009.06.009
Wang, X., Zhou, X., Cai, Z., Guo, L., Chen, X., Chen, X., et al. (2021). A Biocontrol strain of Pseudomonas aeruginosa CQ-40 promote growth and control Botrytis cinerea in Tomato. Pathogens 10, 22. doi: 10.3390/pathogens10010022
Wang, Z., Mei, X., Du, M., Chen, K., Jiang, M., Wang, K., et al. (2020). Potential modes of action of Pseudomonas fluorescens ZX during biocontrol of blue mold decay on postharvest citrus. J. Sci. Food Agric. 100, 744–754. doi: 10.1002/jsfa.10079
Wong, A. T., Kawaguchi, A., and Nita, M. (2021). Efficacy of a biological control agent Rhizobium vitis ARK-1 against Virginia R. vitis isolates, and relative relationship among Japanese and Virginia R. vitis isolates. Crop Protect. 146, 105685. doi: 10.1016/j.cropro.2021.105685
Wonglom, P., Suwannarach, N., Lumyong, S., Ito, S.-I., Matsui, K., and Sunpapao, A. (2019). Streptomyces angustmyceticus NR8-2 as a potential microorganism for the biological control of leaf spots of Brassica rapa subsp. pekinensis caused by Colletotrichum sp. and Curvularia lunata. Biol. Control 138, 104046. doi: 10.1016/j.biocontrol.2019.104046
Xu, T., Cao, L., Zeng, J., Franco, C. M., Yang, Y., Hu, X., et al. (2019). The antifungal action mode of the rice endophyte Streptomyces hygroscopicus OsiSh-2 as a potential biocontrol agent against the rice blast pathogen. Pestic. Biochem. Physiol. 160, 58–69. doi: 10.1016/j.pestbp.2019.06.015
Keywords: Bacillus, Bambara groundnut, food security, plant growth promoting bacteria, Pseudomonas, Streptomyces, sub-Saharan Africa
Citation: Olanrewaju OS and Babalola OO (2022) Plant growth-promoting rhizobacteria for orphan legume production: Focus on yield and disease resistance in Bambara groundnut. Front. Sustain. Food Syst. 6:922156. doi: 10.3389/fsufs.2022.922156
Received: 17 April 2022; Accepted: 24 June 2022;
Published: 14 July 2022.
Edited by:
Everlon Cid Rigobelo, São Paulo State University, BrazilReviewed by:
Metin Turan, Yeditepe University, TurkeyCopyright © 2022 Olanrewaju and Babalola. This is an open-access article distributed under the terms of the Creative Commons Attribution License (CC BY). The use, distribution or reproduction in other forums is permitted, provided the original author(s) and the copyright owner(s) are credited and that the original publication in this journal is cited, in accordance with accepted academic practice. No use, distribution or reproduction is permitted which does not comply with these terms.
*Correspondence: Olubukola Oluranti Babalola, T2x1YnVrb2xhLmJhYmFsb2xhJiN4MDAwNDA7bnd1LmFjLnph