- Chemistry Education Study Program, Faculty of Teacher Training and Education, Universitas Sebelas Maret, Surakarta, Indonesia
Preparation of silver-alginate nanocomposite films as an antibacterial material has been carried out through the casting method of colloidal nanocomposite silver-alginate. Colloidal was made by chemical reduction of AgNO3 precursor salts using microwave irradiation with alginate as a stabilizer and reducing agent and NaOH as an accelerator. The appearance of a brownish yellow color, due to the addition of variation of AgNO3, and the localized surface plasmon resonance (LSPR) phenomenon were identified by UV-Vis spectrophotometer, indicating that silver nanoparticles have been formed. The properties of obtained silver nanoparticles was then examined. The shape and size distribution of silver particles were determined based on the image on transmission electron microscopy (TEM), chemical properties (FTIR), mechanical, crystallinity (XRD), and surface morphology (SEM). Testing of antibacterial activity was performed on silver-alginate nanocomposite films using the diffusion method for gram-positive (S. aureus and MRSA) and gram-negative (E. coli and ESBL) bacteria. The results showed that based on the UV-Vis spectrophotometer characterization results, the LSPR phenomenon appeared at the absorption peak of 401.01–409.00 nm, denoting silver nanoparticles with a spherical shape of 3–22 nm have been formed. Further, the presence of silver nanoparticles affected the mechanical properties of the film, where the tensile strength of the film tended to decrease with the increase in the silver nanoparticles concentration while the crystallinity increased. Next, based on the SEM results the nanocomposite films of silver-alginate had a rough and porous structure. The nanocomposite film had antibacterial activity against E. coli, S. aureus, ESBL, and MRSA. The antibacterial activity film was affected by the concentration of silver nanoparticles.
Introduction
Packaging is an important aspect to protect and preserve the material in it (Kontominas, 2020). Packaging of food and drugs that interact with the human body will affect the comfort of life. Protection of food contained in packaging includes the prevention of (i) physical and mechanical damage, (ii) biological changes that affect the safety of a product, and (iii) changes in physical and chemical properties due to light, oxygen, and moisture (Robertson, 2016). Polymers packaging commonly utilized today is synthetic polymers such as polyurethane, polyethylene, polylactide, polyglycolide, and polyacrylonitrile. However, these synthetic polymers have drawback of having weak adaptation to the conditions of living things or weak biocompatibility (Hashemi et al., 2021; Zhang M. X. et al., 2021). Hence, the development of biopolymers for medical purposes is urgently needed to replace synthetic polymeric packaging that is unsuitable for living things. In particular, the biopolymers have the properties of being non-toxic to living things, non-allergenic, easy to sterilize, durable, and strong. Besides, they also have good elasticity and high biocompatibility or compatibility with living things (Fadhilah bt Ibrahim, Azam, and Khairul, 2019). Alginate is an alternative material applied for biofilm production as packaging. The nature of alginate is that it is non-toxic, able to adapt to living things (biocompatible), can be decomposed by living things, and can be chemically modified (Ambrogi et al., 2020). Alginate-based biofilm can be used as an antibacterial and antifungal packaging material (Zhang M. et al., 2021). However, the antibacterial properties of alginate were not very high, as shown in a study where the inhibition zone of the alginate biofilm did not appear compared to the biofilm added with gold nanoparticles (Foliatini et al., 2014). Therefore, it is necessary to improve the antibacterial properties of alginate biofilms that can be applied to food packaging.
In the last few decades, nanoscience and technology have developed rapidly, especially concerning antibacterial materials. Nanomaterials are generally better than traditional antibiotics because they have the ability to destroy cells and exhibit strong membrane permeability. Additionally, nanoparticles have less resistance than traditional antibiotics (Huh and Kwon, 2011; Hajipour et al., 2012). In general, nanotechnology can be defined as a technology for designing, manufacturing, and applying structures/materials with nanometer dimensions. This technology is not only about how to manufacture nanometer-sized materials or particles, but it also has a broader understanding, including how to manufacture and find out the use of new properties of the nanomaterials made. In particular, silver nanoparticles are one of the noble metals that have been extensively studied for their antibacterial properties. The nanoparticles have high resistance to pathogens, including bacteria that are resistant to antibiotics (Gelperina et al., 2005; Kumar-Krishnan et al., 2015). The presence of mechanical stress on the cell wall will induce the production of free radicals and reactive oxygen, then damage the components of the cell following the release of silver ions. However, the released silver ions and silver nanoparticles are potentially toxic to the host, so the applications of silver-based nanomaterials are limited (Gao et al., 2021). In fact, the combination of polymers with other antibacterial agents such as antimicrobial peptides and silver ions exhibits excellent antibacterial properties. Still, their toxicity and host compatibility has not been studied in-depth and systematically (Hamouda et al., 2001; Lee and Mooney, 2012; Kumar et al., 2018). In this context, alginate biopolymer is used in the synthesis of silver nanoparticles. Besides being employed as a stabilizer that inhibits the aggregation of metal nanoparticles, alginate can also act as a reducing agent, which can reduce gold nanoparticles from 3+ charged to uncharged. Silver nanoparticles can be synthesized by the chemical reduction method (Huang and Yang, 2004; Darroudi et al., 2010). This method is effective and common because it is easy, cost-efficient, and scalable. In this method, reducing agents and stabilizers have a crucial role because these two factors affect the shape and size of the resulting nanoparticles. The synthesis of silver nanoparticles by the chemical reduction method can be accelerated using microwave irradiation. This is evidenced by a study that successfully synthesized silver nanoparticles in 4 min using microwave irradiation (Susilowati et al., 2021) compared to using the same reducer and stabilizer without microwave irradiation, which took 3 h to make silver nanoparticles at a standard temperature (25°C). The microwave was fast and selected carefully with homogeneous heating in reaction systems at the molecular level for rapid and size-controlled preparation with silver nanoparticles on an unprecedented large scale (up to 100 mill moles per liter; Darroudi et al., 2010; Su et al., 2019.
In this research, we are continuing our previous work on silver-alginate nanocomposite that was synthesis using microwave with NaOH as an accelerator. The role of NaOH as an accelerator was providing hydroxyl functional group to make reduction process of AgNO3 into Ag0 become faster [19]. This study focus on silver-alginate nanocomposite films toward the effect of AgNO3 precursor concentration on the number of silver nanoparticles produced based on the LSPR (Localized Surface Plasmon Resonance) phenomenon identified via UV-Vis spectrophotometer. In addition, the properties of obtained silver-alginate nanocomposite films on the physical, chemical, mechanical, and antibacterial properties was also investigated.
Experimental section
Materials
The main ingredients were alginate from Sigma Aldrich, AgNO3, CH3COOH, and NaOH from Merck, and distilled water. As for gram-positive bacteria (S. aureus and Methicillin Resistant Staphylococcus Aureus, MRSA) and gram-negative bacteria (E. coli and Extended Spectrum βLactamase, ESBL) were purchased from Oxoid.
Synthesis of colloidal silver-alginate nanocomposite
1% (w/v) of sodium alginate solution was made in distilled water using a medium-speed stirrer until homogeneous. Next, 12.5 ml of the solution was poured into beakers and stirred at medium speed. Afterward, 0.12 g/ml AgNO3 solution was added to each beaker dropwise with volume variations of 0.25, 0.5, 0.75, 1.0, 1.25, and 1.50 ml while stirred with gentle speed for about 5 min. After that, 0.75 ml NaOH 2 M was added to each beaker dropwise while stirred at a medium speed for about 5 min. The beakers were then placed into a microwave at 100 Watt for 6 min. Then, 1% alginate solution (47.5 ml) was added to the six beakers (mixture of AgNO3 and NaOH) and stirred until homogeneous. Finally, the results of colloidal silver alginate nanocomposite with various concentration were sequentially coded as CALG1 (0.5%), CALG2 (1.0%), CALG3 (1.5%), CALG4 (2%), CALG5 (2.5%), and CALG6 (3%). In this case, the % AgNO3 concentration is expressed in m/v of AgNO3/Alginate.
Characterization of colloidal silver-alginate nanocomposite
The formation of silver nanoparticles due to the LSPR phenomenon was characterized via a UV-Vis spectrophotometer of Shimadzu UV3150 with a 300–600 nm wavelength range. The colloidal silver-alginate nanocomposite was diluted 10 times before being observed. Finally, the distribution and shape of nanoparticles in colloidal silver-alginate nanocomposite were observed by TEM.
Preparation of silver-alginate nanocomposite films
Silver-alginate nanocomposite films were prepared by casting from colloid and dried at room temperature. The dried silver-alginate nanocomposite films were then labeled as FALG1 (0.5%), FALG2 (1.0%), FALG3 (1.5%), FALG4 (2%), FALG5 (2.5%), and FALG6 (3%). The variation of % represent the ratio of AgNO3 to alginate (w AgNO3/w alginate).
Chemical and physical characterization of films
The chemical properties of the films were analyzed using FTIR Shimadzu FT-IR 8201 PC. The transmission spectra of the nanocomposite films were recorded from 4,000 to 300 cm−1 wavenumber. Meanwhile, XRD analysis was employed to determine the crystallinity of the silver-alginate nanocomposite films. Prior, samples were prepared by making powder from silver-alginate nanoparticle films. It was then analyzed using XRD at 2θ of 5–60°. Next, the SEM instrument from Zeiss DSM 960 was performed to examine the surface morphology of the nanocomposite films with a 10 μm resolution, 10 kV voltage, and 2,500-time magnification.
Mechanical properties characterization of films
Mechanical properties of the film were examined by cutting the film sample with a size of 5 × 50 mm2 and then installing it on a test tool called Universal Tensile Machine type of Z 0.5. One end of the sample was clamped and mounted on a strain tool, and the other end was mounted on a test machine load measuring tool. The applied strain was 10 mm/min through motor movement. Then, the value of modulus young (elasticity) was obtained by comparing the tensile strength with elongation. The examination of the mechanical properties was carried out in a triplo.
Antibacterial activity test
Antibacterial activity of the film was assessed against standard bacteria of Escherichia coli (E. coli), Staphylococcus aureus (S. aureus), and multidrug-resistant bacteria of Extended-spectrum beta-lactamases (ESBL), and methicillin-resistant Staphylococcus aureus (MRSA). The assessment was conducted by observing the sample inhibition zone on the bacterial growth. The antibacterial assessment was performed by cutting the resulting film into a circle before preparing the antibacterial test medium. The antibacterial medium is Nutrient Broth (NB) liquid dissolved in water with a concentration of 0.8% (w/v). A colony of bacteria was taken using a needle loop, placed into 10 ml NB liquid medium in an Erlenmeyer, and left in an incubator for 24 h. The NB liquid medium that already contained the bacteria was then poured into a petri dish. Afterward, the silver-alginate non-composite films cut with a diameter of 6 mm were attached to the NB medium. They were then incubated for 24 h at 37°C. The results were observed by looking at the inhibition zone of the film against bacterial growth as measured by a digital caliper.
Results and discussion
Synthesis of silver-alginate nanocomposite films
Silver-alginate nanocomposite films were prepared by the casting method on colloidal silver nanoparticles with an alginate matrix. Colloidal silver nanoparticles were made using a chemical reduction method with AgNO3 as a precursor, NaOH as an accelerator, alginate as a stabilizer agent and reducing substance, and using 100 W microwave irradiation for 6 min. Figure 1 presents the colloidal silver-alginate nanocomposite formed with a brownish yellow color indicating the presence of silver nanoparticles.
Energy from microwave irradiation is employed to increase the reaction rate. In this reaction, Ag+ ions from the AgNO3 precursor will be reduced by alginate in the presence of a NaOH accelerator. Alginate also acts as a stabilizer to limit the aggregation of silver particles on the nano-size scale. The produced Ag nanoparticles were identified based on the LSPR phenomenon by UV-Vis spectrophotometric method. The LSPR phenomenon is caused by the collective excitation of electrons near the surface of metal nanoparticles, namely in the conduction band. The oscillating electrons are confined to certain vibrational modes depending on the size and shape of the particle. This process causes optical absorption in the UV-vis region so that the presence of silver nanoparticles in the colloidal system can be observed using the UV-Vis spectrophotometer (Susilowati et al., 2021). Figure 2 demonstrates that the formation of Ag nanoparticles was influenced by the concentration of AgNO3 as the precursor. The spectra reveal that the higher AgNO3 concentration precursor causes more nanoparticles to be produced, characterized by an increased absorbency due to the LSPR phenomenon. This is indicated that the number of silver nanoparticle formed is higher with the increased of concentration of AgNO3.
The size distribution and shape of the particles are identified based on the TEM images, as displayed in Figure 3. Ag particle size is influenced by the concentration of AgNO3 as the precursor. According to the TEM images of the CALg3 and CALg6 samples, the higher AgNO3 concentration causes the particle size to be larger. The appearance of a single absorption of LSPR in the UV-Vis test with a wavelength around 400 nm indicates spherical particles formed. This is in line with the images of TEM analysis in Figure 3, which demonstrates spherical particles. The size of silver nanopaerticle is range from 3 to 22 nm with average size of silver nanoparticles for CALG3 and CALG6 are 4.534 ± 1.737 and 11.397 ± 3.358 nm, respectively.
The film was produced by the colloidal casting method, and the drying process was conducted at room temperature. The films formed had a brown color which was getting darker as the concentration of silver was added, as presented in Figure 4.
Physical and chemical properties of films
The physical and chemical properties investigation included surface morphology using SEM, crystallinity using XRD, and functional groups using FTIR spectrophotometer. The chemical and physical properties were investigated by comparing two film samples, namely alginate films of FALG0 and FALG6. The FALG6 film was considered to have the greatest effect on its physical and chemical properties due to the presence of silver nanoparticles in the film. The results of SEM examination in Figure 5 show that the alginate film has a tight surface structure, no cracks, and a smooth surface. However, after silver nanoparticles were added, the film surface became uneven, wavy, and porous because the addition of Ag nanoparticles caused polymer chains of alginate to develop when interacting or bonding with Ag nanoparticles.
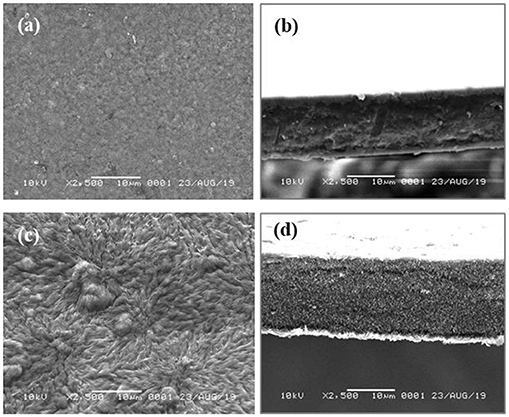
Figure 5. SEM images: (a) surface of alginate film, (b) cross-section of alginate film, (c) surface of FALG6 film, and (d) cross section of FALG6 film.
The silver-alginate nanocomposite films formed was then measured using an FTIR spectrophotometer to determine the presence of several bonds in organic compounds. The spectrum of the test results for the sodium alginate functional group is shown in Figure 6. It reveals absorptions in the 3,470 cm−1 regions
for hydroxyl, 1,680 cm−1 regions and 1,400 cm−1 are assigned for vibration of symmetric and asymmetric carboxylate group, -O-CO-, respectively. Meanwhile, stretching vibration of C-O bond present in carbohydrate rings of alginate was clearly seen in the range of 1,250–1,350 cm−1. Around 1,030 cm−1 area for C-O stretching vibration bonds. In addition, the bonding between silver nanoparticles with alginate was indicated by the new peak around 500–600 cm−1 (Zhou et al., 2020). The presence of alginate was denoted by the vibrations of hydroxyl, carbonyl, carboxyl, and bonds between carbons of -COOH and C-O-C.
To determine the crystallinity of the film, an analysis using XRD was performed. Figure 7 displays the XRD patterns of the alginate film (FALG0) and the silver-alginate nanocomposite films (FALG6), respectively. At the FALG6, there was a sharp peak at 2θ = 20°, corresponding to the crystalline nature of the silver alginate nanoparticles. The XRD pattern of silver-alginate nanoparticles demonstrated that the peak intensity increased significantly due to the addition of silver nanoparticles in the alginate, which increased the crystallinity of the alginate after modification. The diffraction of FALG6 showed that there were some new peaks that appeared and assigned as silver nanoparticle, namely peak at 2θ = 17°, 27°, and 38° (Mladenova et al., 2018). Moreover, the peak intensity of diffraction FALG6 is higher than that of FALG0 which suggesting that the silver nanoparticles incorporated in silver-alginate nanocomposite films.
Mechanical properties of films
The results of the mechanical properties examination of silver alginate nanocomposite films were analyzed using three parameters: tensile strength, elongation, and modulus young. The effect of silver nanoparticles concentration on tensile strength is displayed in Figure 8A. It is seen that FALG0 and FALG1 films have the highest tensile strength values compared to other samples. This means that the presence of silver nanoparticles tends to decline the tensile strength of the film due to a decrease in the bond strength between the alginate biopolymer chains. Meanwhile, the FALG6 sample exhibited the lowest tensile strength value among all samples. It is considered due to the uneven distribution of silver nanoparticles because of agglomeration in the alginate biopolymer. This also makes the tensile strength of the film not homogeneous in every area. Moist samples also affect the tensile strength properties. If the sample is not completely dry or moist, its tensile strength gets smaller.
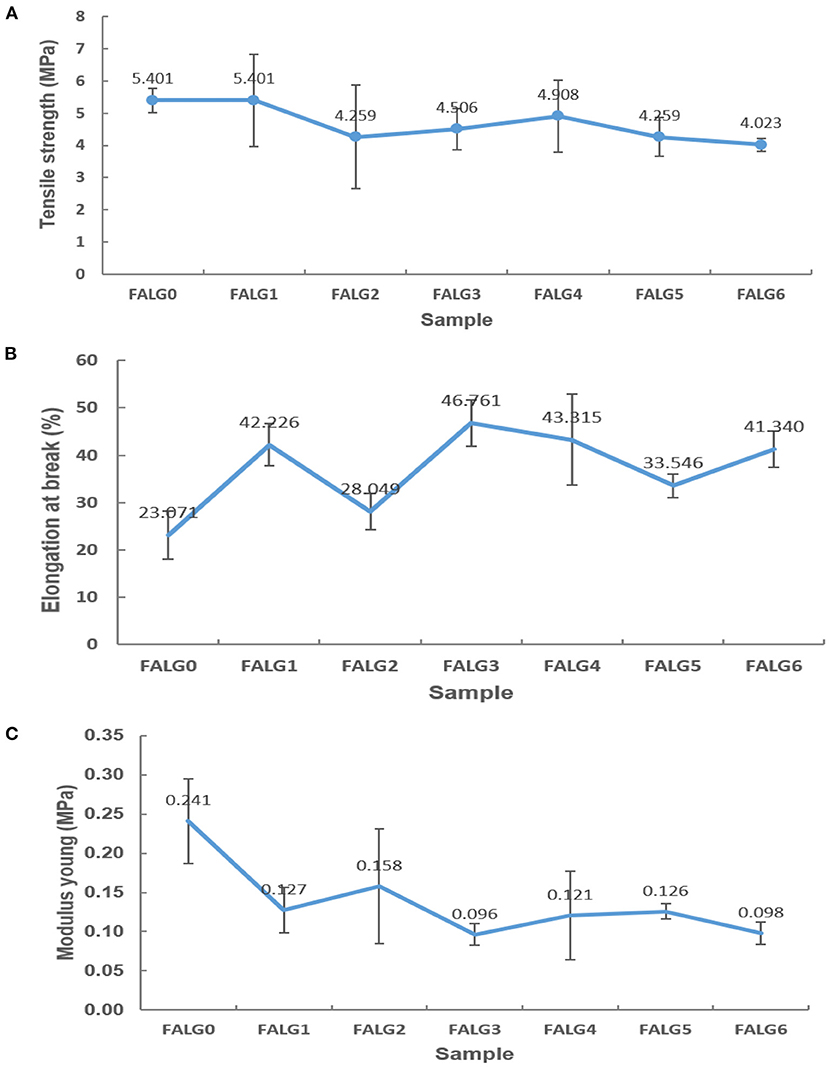
Figure 8. Test chart: (A) tensile strength, (B) elongation at break, and (C) modulus young of silver-alginate nanocomposite films.
The addition of silver nanoparticles concentration can reduce the percent elongation of the formed film due to the interaction between silver nanoparticles and biopolymer alginate chains. This interaction declines the mobility of the alginate biopolymer chain, which enhances the strengthening properties of the silver-alginate nanocomposite films. The peak percent elongation occurred in the FALG3 sample as the concentration of AgNO3 increased, but it decreased in FALG4 to FALG6 samples (Figure 8B). This is probably due to the uneven distribution of nanoparticles in the alginate biopolymer. Therefore, although there is a tendency to increase along with the increase in the concentration of silver nanoparticles, the elongation value fluctuates. The state of the sample also affects the measurement of elongation. If the sample is humid, it affects the measurement of the percent elongation.
Figure 8C shows that the elasticity of the silver-alginate nanocomposite films reduced with the increase in the concentration of silver nanoparticles (Figure 8C). Modulus young or elasticity is the tendency of a material to return to its original shape, whose value is related to the elongation and tensile strength of the material. The greater the number of silver nanoparticles, the more likely the film cannot return to its original shape.
Antibacterial activity of films
The inhibition of silver nanoparticles against gram-positive bacteria S. aureus and MRSA was stronger than gram-negative bacteria E. coli and ESBL (Figure 9 and Table 1). The resistance of gram-negative bacteria E. coli and ESBL can be associated with the cell wall structure. Gram-negative bacteria have a thin layer of lipopolysaccharide structure on the outside of the membrane, so it has a more effective barrier ability than gram-negative bacteria. As a result, the penetration of silver nanoparticles on the cell membrane can be limited. Meanwhile, silver nanoparticles more easily access gram-positive bacteria due to the peptidoglycan layer. Silver-alginate nanocomposite films revealed good inhibitory activity against gram-positive and gram-negative bacteria by forming a clear zone on the agar medium containing these bacteria (Kong et al., 2010; Bakhsheshi-Rad et al., 2020).
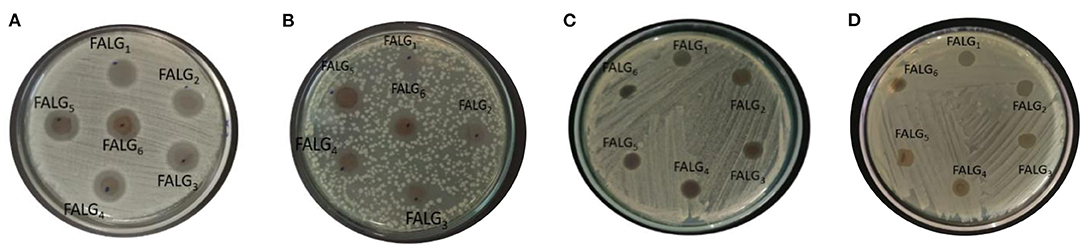
Figure 9. Antibacterial inhibitory activity of silver alginate nanocomposite films against bacteria (A) S. aureus; (B) E. coli; (C) MRSA; (D) ESBL.

Table 1. Antibacterial activity of silver alginate nanocomposite films against S. aureus, E. coli, MRSA, and ESBL bacteria.
The antibacterial activity of silver is related to the ability of silver nanoparticles to damage bacterial cell walls and inhibit metabolism so that cell development is disrupted. The size of these nanoparticles results in a large surface area, and the contact between silver particles and microorganism cells becomes more massive so that the antibacterial activity becomes greater (Rahimi et al., 2019; Zhang H. et al., 2021). The mechanism of antibacterial activity begins with contact with the cell membrane and then penetrates the cell wall. Cells will die because the diffusion of silver nanoparticles will attack cell respiration. Another mechanism is on a molecular scale through the interaction of the thiol sulfhydryl groups on the protein from the bacteria with silver ions. The H+ cation in the thiol sulfhydryl group is replaced by an ion (Ag+) and forms a stable S-Ag group on the surface. The formation of the S-Ag group causes the protein to become inactive, and the membrane becomes less permeable, which has an impact on cell death (Liang et al., 2020).
Conclusion
The silver-alginate nanocomposite films have been successfully synthesized via the chemical reduction method followed by the casting method from the colloidal system. The appearance of the absorption band peaks at 401.01–409.00 nm implies that silver alginate nanocomposites have produced silver nanoparticles. The silver nanoparticles have a spherical shape even though the concentration of AgNO3 was increased. The size of silver nanoparticles are in the range of 2–22 nm. The number of silver nanoparticles affects the mechanical and physical properties of the silver alginate nanocomposite films. The increase in the concentration of silver nanoparticles tends to reduce tensile strength, increase elongation, and decrease film elasticity but the crystallinity increases. This means that the nanocomposite films is easy to torn and less elastic but easier to elongate. The nanocomposite films of silver-alginate have a rough and porous structure based on SEM result. In addition, the number of silver nanoparticles affects the anti-bacterial activity, where the inhibitory power against gram-positive bacteria is higher than that of gram-negative. Because silver alginate nanocomposite films can inhibit standard bacteria (E. coli and S. aureus) and multidrug-resistant bacteria (ESBL and MRSA), it has potential for application in the medical field and antibacterial packaging.
Data availability statement
The original contributions presented in the study are included in the article/supplementary material, further inquiries can be directed to the corresponding authors.
Author contributions
ES: conceptualization, writing—review and editing, and supervision. LM: writing—review and editing. RH: data collection. All authors contributed to the article and approved the submitted version.
Funding
This work was funded under grant of Penelitian Unggulan (PU) with contract No. 260/UN27.22/HK.07.00/2021 by the Universitas Sebelas Maret.
Conflict of interest
The authors declare that the research was conducted in the absence of any commercial or financial relationships that could be construed as a potential conflict of interest.
Publisher's note
All claims expressed in this article are solely those of the authors and do not necessarily represent those of their affiliated organizations, or those of the publisher, the editors and the reviewers. Any product that may be evaluated in this article, or claim that may be made by its manufacturer, is not guaranteed or endorsed by the publisher.
References
Ambrogi, V., Pietrella, D., Donnadio, A., Latterini, L., Di Michele, A., Luffarelli, I., et al. (2020). Biocompatible alginate silica supported silver nanoparticles composite films for wound dressing with antibiofilm activity. Mater. Sci. Eng. C. 112:110863. doi: 10.1016/j.msec.2020.110863
Bakhsheshi-Rad, H. R., Hadisi, Z., Ismail, A., Akbari, M., Berto, F., and Chen, X. (2020). In vitro and in vivo evaluation of chitosan-alginate/gentamicin wound dressing nanofibrous with high antibacterial performance. Polym. Test. 82:106298. doi: 10.1016/j.polymertesting.2019.106298
Darroudi, M., Bin Ahmad, M., Abdullah, A. H., Ibrahim, N. A., and Shameli, K. (2010). Effect of accelerator in green synthesis of silver nanoparticles. Int. J. Mol. Sci. 11, 3898–3905. doi: 10.3390/ijms11103898
Fadhilah bt Ibrahim, S., Azam, N. A. N. M, and Khairul, K. M. A. (2019). Sodium alginate film: the effect of crosslinker on physical andmechanical properties. IOP Conf. Ser.: Mater. Sci. Eng. 509:012063.
Foliatini, F., Yulizar, Y., and Hafizah, M. A. E. (2014). Microwave-assisted synthesis of alginate-stabilized gold nanoparticles. Makara J. Sci. 18, 111–118. doi: 10.7454/mss.v18i4.4281
Gao, X., Li, H., Niu, X., Zhang, D., Wang, Y., Fan, H., et al. (2021). Carbon quantum dots modified Ag2S/CS nanocomposite as effective antibacterial agents. J. Inorg. Biochem. 220:111456. doi: 10.1016/j.jinorgbio.2021.111456
Gelperina, S., Kisich, K. M., Iseman, D., and Heifets, L. (2005). The potential advantages of nanoparticle drug delivery systems in chemotherapy of tuberculosis. Am. J. Respirat. Crit. Care Med. 172, 1487–1490. doi: 10.1164/rccm.200504-613PP
Hajipour, M. J., Fromm, K. M., Ashkarran, A. A., de Aberasutri, D. J., de Larramendi, I. R. T., Rojo, V., et al. (2012). Antibacterial properties of nanoparticles. Trends Biotechnol. 30, 499–511. doi: 10.1016/j.tibtech.2012.06.004
Hamouda, T., Myc, A., Donovan, B., Shih, A. Y., Reuter, J. D., and Baker, J. R. (2001). A novel surfactant nanoemulsion with a unique non-irritant topical antimicrobial activity against bacteria, enveloped viruses and fungi. Microbiol. Res. 156, 1–7. doi: 10.1078/0944-5013-00069
Hashemi, Z., Mohammadyan, M., Naderi, S., Fakhar, M., Biparva, P., Akhtari, J., et al. (2021). Green synthesis of silver nanoparticles using Ferula persica extract (Fp-NPs): characterization, antibacterial, antileishmanial, and in vitro anticancer activities. Mater. Tod. Commun. 27:102264. doi: 10.1016/j.mtcomm.2021.102264
Huang, H., and Yang, X. (2004). Synthesis of polysaccharide-stabilized gold and silver nanoparticles: a green method. Carbohydrate Res. 339, 2627–2631. doi: 10.1016/j.carres.2004.08.005
Huh, A. J., and Kwon, Y. J. (2011). “Nanoantibiotics”: a new paradigm for treating infectious diseases using nanomaterials in the antibiotics resistant era. J. Control. Rel. 156, 128–145. doi: 10.1016/j.jconrel.2011.07.002
Kong, M., Chen, X. G., Xing, K., and Park, H. J. (2010). Antimicrobial properties of chitosan and mode of action: a state of the art review. Int. J. Food Microbiol. 144, 51–63. doi: 10.1016/j.ijfoodmicro.2010.09.012
Kontominas, M. G. (2020). Use of alginates as food packaging materials. Foods. 9:101440. doi: 10.3390/foods9101440
Kumar, S. S. D., Rajendran, N. K., Houreld, N. N., and Abrahamse, H. (2018). Recent advances on silver nanoparticle and biopolymer-based biomaterials for wound healing applications. Int. J. Biol. Macromol. 115, 165–175. doi: 10.1016/j.ijbiomac.2018.04.003
Kumar-Krishnan, S., Evgen, P., Hernandez-Ituriaga, M., Morta-Morales, J., Vazquez-Lepe, D., Kovalenko Yuri, M., et al. (2015). Chitosan/silver nanocomposites: synergistic antibacterial action of silver nanoparticles and silver ions. Eur. Polym. J. 67, 242–251. doi: 10.1016/j.eurpolymj.2015.03.066
Lee, K. Y., and Mooney, D. J. (2012). Alginate: properties and biomedical applications. Progr. Polym. Sci. 37, 106–126. doi: 10.1016/j.progpolymsci.2011.06.003
Liang, L., Hou, T., Ouyang, Q., Xie, L., Zhong, S., Li, S., and Li, C. (2020). Antimicrobial sodium alginate dressing immobilized with polydopamine-silver composite nanospheres. Compos. B Eng. 188:107877. doi: 10.1016/j.compositesb.2020.107877
Mladenova, B. B., Miusheva, Y., Karsheva, M., Hinkov, I., Stankulov, T., and Boukoureshtlieva, G. (2018). Nanosized Ag particles as catalyst in gas-diffusion electrodes for ORR. Bulgarian Chem. Commun. 50, 114–118.
Rahimi, M., Ahmadi, R., Samadi Kafil, H., and Shafiei-Irannejad, V. (2019). A novel bioactive quaternized chitosan and its silver-containing nanocomposites as a potent antimicrobial wound dressing: structural and biological properties. Mater. Sci. Eng. C. 101, 360–369. doi: 10.1016/j.msec.2019.03.092
Robertson, G. L. (2016). Food Packaging, 3rd Edn. Gordon L. Robertson Principles and Practice. Boca Raton, FL: CRC Press, Taylor & Francis Group.
Su, D., Li, P., Ning, M., Li, G., and Shan, Y. (2019). Microwave assisted green synthesis of pectin based silver nanoparticles and their antibacterial and antifungal activities. Mater. Lett. 244, 35–38. doi: 10.1016/j.matlet.2019.02.059
Susilowati, E., Masykuri, M., Ulfa, M., and Hardini, R. D. (2021). Synthesis of silver-alginate nanocomposites colloidal assisted by microwave irradiation as antibacterial material. J. Phys. Conf. Ser. 1912:16. doi: 10.1088/1742-6596/1912/1/012016
Zhang, H., Cheng, J., and Ao, Q. (2021). Preparation of Alginate-Based Biomaterials and Their Applications in Biomedicine. 19:264. doi: 10.3390/md19050264
Zhang, M., Qiao, X., Han, W., Jiang, T., Liu, F., and Zhao, X. (2021). Alginate-chitosan oligosaccharide-ZnO composite hydrogel for accelerating wound healing. Carbohydrate Polym. 266:118100. doi: 10.1016/j.carbpol.2021.118100
Zhang, M. X., Wang, G., Wang, D., Zheng, Y, Li, Y., Meng, W., et al. (2021). Ag@MOF-loaded chitosan nanoparticle and polyvinyl alcohol/sodium alginate/chitosan bilayer dressing for wound healing applications. Int. J. Biol. Macromol. 175, 481–494. doi: 10.1016/j.ijbiomac.2021.02.045
Keywords: nanocomposite, silver nanoparticle, alginate, properties, antibacterial activity
Citation: Susilowati E, Mahardiani L and Hardini RD (2022) The effect of silver nanoparticles toward properties and antibacterial activity of silver-alginate nanocomposite films. Front. Sustain. Food Syst. 6:913750. doi: 10.3389/fsufs.2022.913750
Received: 06 April 2022; Accepted: 27 June 2022;
Published: 01 August 2022.
Edited by:
I. Made Joni, Universitas Padjadjaran, IndonesiaCopyright © 2022 Susilowati, Mahardiani and Hardini. This is an open-access article distributed under the terms of the Creative Commons Attribution License (CC BY). The use, distribution or reproduction in other forums is permitted, provided the original author(s) and the copyright owner(s) are credited and that the original publication in this journal is cited, in accordance with accepted academic practice. No use, distribution or reproduction is permitted which does not comply with these terms.
*Correspondence: Endang Susilowati, ZW5kYW5nX3M3MEBzdGFmZi51bnMuYWMuaWQ=; Lina Mahardiani, bWFoYXJkaWFuaS5saW5hQHN0YWZmLnVucy5hYy5pZA==