- 1Indonesian Cereals Research Institute, Ministry of Agriculture Republic of Indonesia, Maros, Indonesia
- 2Department of Plantation Crop Cultivation, Pangkep State Polytechnic of Agriculture, Mandalle, Indonesia
- 3Agrotechnology Study Program, Faculty of Agriculture, Universitas Puangrimaggalatung, Sengkang City, Indonesia
- 4Department of Agronomy, Faculty of Agriculture, Khon Kaen University, Khon Kaen, Thailand
- 5Department of Agronomy and Horticulture, Faculty of Agriculture, IPB University, Bogor, Indonesia
Unpredictable rainfall in the tropics often increases the risk of waterlogging or even flooding in agricultural lands, hindering the efforts to fulfill maize demands. Breeding maize for waterlogging tolerance is necessary yet challenging since performing varietal testing on a set of hybrids might be biased toward the presence of genotype and environment interaction (GEI). This study aimed to elucidate the GEI effects on yield and related agronomic traits of tropical maize hybrids under normal irrigation and waterlogging conditions and to assess the adaptability of these hybrids in such conditions using several stability models. Ten hybrids including two commercial checks were evaluated across 14 environments under normal and waterlogging conditions in Indonesia from 2018 to 2020. Waterlogging imposed at the V6 stage for ten consecutive days significantly hampered the plant height and ear height, slightly delayed flowering dates, and reduced yield and yield components. The genotype, location, and genotype by location effects were significant on yield, but the genotype by waterlogging effect was not. Stress tolerance index is highly significantly correlated (p < 0.01) with yield in both normal (r = 0.90) and waterlogging (r = 0.96) conditions. The GGE biplot analysis on yield revealed five sectors, two mega-environments, and five vertex genotypes. This study indicated the possibility of breeding maize hybrids tolerant to waterlogging (G05), as well as high-yielding hybrids under both conditions (G07).
Introduction
Maize has high economic value and is an important crop in many Asian countries, including in Indonesia. Maize is increasingly used as a staple food (Grote et al., 2021), feed for poultry, fish (Erenstein, 2010), and dairy cows (Khan et al., 2015), and raw materials for the food industries including oil, flour, and snacks (Zhang et al., 2021). Rice-maize (R-M) cropping system, a maize cultivation system in rice fields, is generally carried out in the tropical regions of South-East (Agus et al., 2019) and South Asia (Timsina et al., 2010). This system is usually conducted at the end of the rainy season when sufficient rainfall for initial crop growth is still available. However, climate change causing erratic rainfall for extended periods (Arritt and Rummukainen, 2011; Liu et al., 2021) increases the risk of waterlogging on maize plants. This abiotic stress covers about 12% of the global land area, and in Southeast Asia, about 15% of the total maize growing area in poorly drained rice fields experiences flooding or waterlogging at the end of the rainy season (Rathore et al., 1998). Waterlogging limits the availability of oxygen from the soil to the plants resulting in a condition of soil hypoxia (Barrett-Lennard, 2003; Liu et al., 2020c). In maize, this anaerobic condition leads to the unbalanced transport of both nutrients and water to the leaf tissue (Sachs et al., 1996), reduced leaf water potential, stomatal closure, leaf curling (Sairam et al., 2008), and yield reduction (Ren et al., 2014). Maize yield reduction due to waterlogging can vary from 7 to 80% depending upon cultivar, crop growth stage (Ren et al., 2016a, 2017), and the duration of imposed stress (Tian et al., 2019).
Several strategies are proposed to alleviate the damage of waterlogging including drainage construction, proper planting schedule, and the adoption of waterlogging-tolerance cultivars (Liu et al., 2020b; Yan et al., 2022). The latter option is promising and can be achieved by hybrid breeding maize for waterlogging tolerance (Zaidi et al., 2004; Bailey-Serres et al., 2012) because of the presence of genetic stock (Tripathi et al., 2003) and considerable genotypic variation (Mano et al., 2002) for this trait. Waterlogging tolerance is a complex trait attributed to several factors including genotype and environment (Li et al., 2011; Prasanna and Ramarao, 2014). Grain yield is the most important trait in maize; however, this character is polygenic and sensitive to the exposed environmental changes (Acquaah, 2007). Under waterlogging stress, the low to moderate heritability estimates have been noticed (Edmeades et al., 1999) and the genotype by environment interaction (GEI) becomes greater for maize grain yield (Singamsetti et al., 2021). The purpose of the adaptation test is to determine the genetic potential and adaptability of candidate varieties in the targeted environments. This test involves the evaluation of a number of various candidates in several environments using the same experimental design, usually a randomized complete block or alpha-lattice design. Through the adaptation test, information on the GEI and the yield stability of these candidates across environments will be obtained. A better understanding of GEI is helpful to evaluate genotype stability and adaptability across environments, recommend certain genotypes for specific growing environments, and assess the ability of test environments to discriminate the tested genotypes (Yan and Tinker, 2006; Dias et al., 2018). Several models of stability analysis are provided to elucidate the complex nature of GEI phenomena under stressed environments (Mafouasson et al., 2018; Liu et al., 2022) and to utilize the obtained information for varietal selection (Kang, 1998; Liu et al., 2020a). These models include joint regression analysis (Finlay and Wilkinson, 1963; Eberhart and Russell, 1966), the additive main effects and multiplicative interaction (AMMI) (Gauch, 1992), and the genotype main effects and genotype × environment interaction (GGE) biplot analysis (Yan et al., 2000).
The Indonesian Cereals Research Institute (ICERI) and IPB University, Bogor, Indonesia have collaborated in breeding improved tropical maize cultivars tolerant to waterlogging stress. Our breeding strategy begins with establishing base populations by recombining potential genotypes through controlled cross-pollinations, followed by the development and evaluation of inbred lines, hybrid formation, and yield trials. From a previous study, we noticed several candidates of superior maize hybrids under both normal and waterlogging stress environments, namely G8xMGold, G9xMGold, G28xMGold, IPB L15xMR14, and IPB L39xMR14 (Syah, 2019). Since maize hybrids with stable performance across diverse environments (Khalil et al., 2011), high yield potential, and low yield reduction under optimum and stress conditions, respectively (Kenga, 2001) appeal to farmer's preference, multi-location trials representing the diverse agroecology of a targeted region is imperative prior to varietal releases. In the case of significant GEI, maize hybrids with high adaptability across normal and waterlogging conditions and tolerant genotypes under waterlogging stress would be interesting to investigate. Therefore, this study aimed to elucidate the GEI effects on yield and related agronomic traits of tropical maize hybrids under normal and waterlogging conditions and to assess the adaptability of these hybrids in such conditions using several stability models. Considering the lack of studies on GEI analysis in maize under waterlogging conditions, the information obtained in this study would help breeders in assigning the breeding strategy for waterlogging resilience. Besides, the selected hybrids could be adopted by farmers in the tropics to increase their production and income.
Materials and Methods
Plant Materials
The genetic materials used in the multi-environment test were eight maize single crosses, namely G01 (G8xMGold), G02 (G9xMGold), G03 (G21xMGold), G04 (G41xMGold), G05 (G28xMGold), G06 (IPB L12xMR14), G07 (IPB L15xMR14), G08 (IPB L39xMR14), and two commercial hybrid varieties as checks, namely Bima 9 (G09) and BISI 18 (G10). The MGold is a maize genotype tolerant to waterlogging introduced from the International Maize and Wheat Improvement Center (CIMMYT). The MR14, an inbred line developed by the ICERI, is a good combiner for grain yield and has been used in the formation of hybrid cultivars in Indonesia. The G8, G9, G21, G41, and G28 lines were developed by ICERI from the Yellow White population resulting from the recombination of downy mildew-resistant yellow seed populations (Nei9008 x MR14) with a CIMMYT line from Kenya, as part of the Affordable, Accessible Asian (AAA) Drought Tolerant Maize Project. The IPB L15 and IPB L39 were developed by IPB University from double cross hybrids populations.
Experimental Sites, Experimental Design, and Crop Management
This study was conducted from 2018 to 2020 in eight locations differing in agro-ecologies including lowland zones (N1-N6) with altitudes ranging from 17 to 333 m above sea level (masl), a mid-altitude zone of 615 masl (N8), and a highland zone of 867 m asl (N7) (Table 1). Different types of soil can be found in the experimental location. These include alluvial, regosol, andosol, latosol, and red-yellow podzolic soil. The texture of the soil ranges from clay to clay loam. In the water logging experiment conducted in Maros, it was discovered that the clay loam texture is less porous, making it more susceptible to flooding during periods of intense rain. The pH of the soil at the experimental sites ranged from slightly acidic to close to neutral.
In each experimental site, a randomized completed block design (RCBD) with three replications was laid out. The harvested plot size was two rows of 5.0 m in length (7 m2), and plant spacing was 70 cm between and 20 cm within rows. Full tillage was performed prior to planting. Two seeds per hole were planted, and the seedlings were then thinned to one plant per hole at 10 days after planting (DAP). Fertilizers were applied two times, with the first application at 7–10 DAP for 350 kg/ha of NPK Phonska fertilizer (15:15:15) and 150 kg/ha of urea (46:0:0) and the second application at 30–35 DAP for 200 kg/ha of urea (46:0:0). Weeding was manually carried out and mulching was done by elevating the mounds and loosening the soil to create better soil aeration. Harvesting was performed at physiological maturity indicated by the appearance of a black layer of the grain.
Managed Waterlogging Stress
The waterlogging stress treatment referred to the procedure developed by Liu et al. (2010) and Zaidi et al. (2016). The treatment was imposed when the plants were roughly knee-high (V6 stage) at 4 weeks after planting (WAP). Waterlogging experiments were conducted in a field that had been purpose-built and leveled for this purpose. The treatment plots were initially completely submerged in water, which was maintained for ten consecutive days at a depth of about 10 cm by providing water at a rate greater than infiltration and evaporation, and then the process was repeated. Irrigation and excessive water drainage were regulated using a drainage pipe with two adjustable gates to keep the water about 10 cm above ground level and prevent overflow from flooded plots. The field was completely drained following the stress treatment.
Phenotypic Data Collection
Observations were performed on several agronomic traits, yield, and yield components according to the CIMMYT (2004). Five sample plants were randomly taken from each plot. The observed traits were: (1) days to 50% anthesis on a plot-basis; (2) days to 50% silking on a plot-basis; (3) anthesis-silking interval, calculated as the difference between days to silking and days to anthesis; (4) plant height, measured as the distance from ground level to the node bearing the flag leaf; (5) ear height as the distance from ground level to the node bearing the uppermost ear; (6) leaf length; (7) number of rows per ear; (8) number of kernels per row; (9) ear length; (10) ear diameter; (11) shelling percentage; and (12) grain yield (t/ha) at 15% moisture content, calculated using the following equation:
where MC is the actual grain moisture content at harvest, PS is harvested plot size (m2), EW is ear yield per plot (kg), and SP is shelling percentage.
Statistical Analysis
Combined analysis over experiments was performed to elucidate the effects of waterlogging treatment, location, genotype, and their interactions, with the following linear model:
where Yijkl is the observed response of waterlogging treatment-i, location-j, replication-k, and genotype-l; μ is the grand mean; Wi is the effect of waterlogging treatment; Lj(i) is the effect of location nested in waterlogging treatment; Bk(ji) is the effect of block nested in location and waterlogging treatment; GLlj(i) is the interaction effect of genotype and location; GWli is the interaction effect of genotype and waterlogging treatment; and εijkl is the experimental error. The least significance difference (LSD) test at a 5% probability level was used for comparing the mean of each tested hybrid and each check variety (Gomez and Gomez, 1984).
Several parameters of stress tolerance including stress intensity (SI), tolerance (TOL), stress susceptibility index (SSI), and stress tolerance index (STI) were estimated according to the Fernandez (1992) formulas below:
where Ys is the mean yield of each genotype under waterlogging stress conditions, Yp is the mean yield of each genotype under normal condition, is the grand mean of yield under waterlogging condition, and is the grand mean of yield under normal conditions.
Stability analyses were performed using several methods including yield and stability index (Kang, 1993), coefficient of variations (Francis and Kannenberg, 1978), regression of genotype mean yield on the environmental index (Finlay and Wilkinson, 1963; Eberhart and Russell, 1966), and ecovalence (Wricke, 1962). Besides, the GGE biplot analysis (Yan et al., 2000) was performed to identify the genotypes adapted to the specific environmental clusters. The above analyses used SAS 9 (SAS Institute, 2002) and PBSTAT-GE 3.0.3 (www.pbstat.com) software.
Results
Combined Analysis of Variance
A combined analysis of variance across 14 environments (11 normal irrigation and 3 waterlogging) for agronomic traits, yield components, and yield are presented in Table 2. The environment effect was partitioned into waterlogging effect (1 df) and the location within waterlogging effect (12 df). The effect of waterlogging treatment was significant on all observed traits except on anthesis-silking interval (ASI). The effect of location within the waterlogging treatment was significant on all observed traits. This indicated that the variability of test locations, including the altitude, the types of soil, and climate, affected the performance of the genotypes. The genotype effect was significant on all observed traits except ear height (EH), indicating that the hybrids evaluated in this study were phenotypically diverse in plant architecture, flowering dates, yield components, and grain yield. The interaction between genotype and location (G × L) effect was significant on all observed traits except for ASI and EH, and the interaction between genotype and waterlogging (G × W) effect was significant on all observed traits except on ASI, plant height (PH), EH, number of kernels per row (KR), ear length (EL), and grain yield (Y). These implied the different responses of varieties to the different environmental conditions.
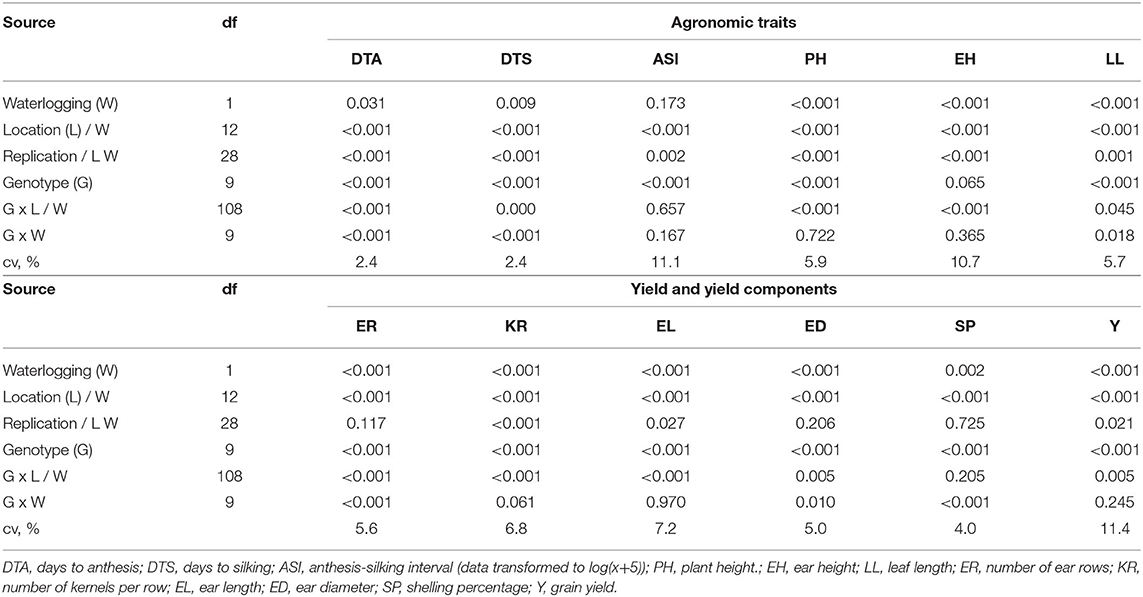
Table 2. Combined analysis of variance (p-values) across environments for agronomic and yield traits in maize.
Maize Performance Against Different Water Conditions
Maize genotypes grown under waterlogging conditions had higher averaged means than those under normal conditions on DTA and DTS (Table 3), indicating that excess water stress could slightly delay the flowering dates of maize. On the contrary, the considerable reductions of genotypic means on PH, EH, LL, and Y were noticed under waterlogging conditions, while small reductions of the means were found on ER, KR, EL, ED, and SP. These results illustrated that waterlogging stress could significantly restrict the vegetative growth and ear development of maize genotypes. The genotype means in the normal (N) conditions tended to be higher than in the waterlogging (W) conditions, except in N11 (Figure 1).
GEI and Stability Analysis Across Environments
The environment and genotype main effects, as well as the genotype by environment interaction (GEI) effect, were highly significant on yield. The environment contributed 76.7% to the total sum of square, greater than the contribution of GEI (7.0%) and genotype (4.2%). In spite of the relatively little contribution, the presence of the GEI may indicate two things: (1) the genotype ranks were inconsistent across test locations, and (2) yield stability analyses were further required to determine the stability and adaptability of test hybrids on yield over test environments. Moreover, the significance of GEI may lead to overestimations of the genetic variance and heritability if these estimates were obtained from one environment trial only.
The average yield (Yi) and yield and stability index (YSi) of four hybrids G02 (9.55 t ha−1; 8), G05 (9.72 t ha−1; 7), G07 (10.13 t ha−1; 5), and G08 (9.60 t ha−1; 9) were greater than the average Yi and YSi (9.26 t ha−1; 3.2), indicating that these hybrids were relatively high-yielding and stable across 14 test environments (Table 4). These hybrids also had low coefficients of variations (CV) ranging from 23.65 to 25.55%, slightly smaller than the average CV (26.14%).
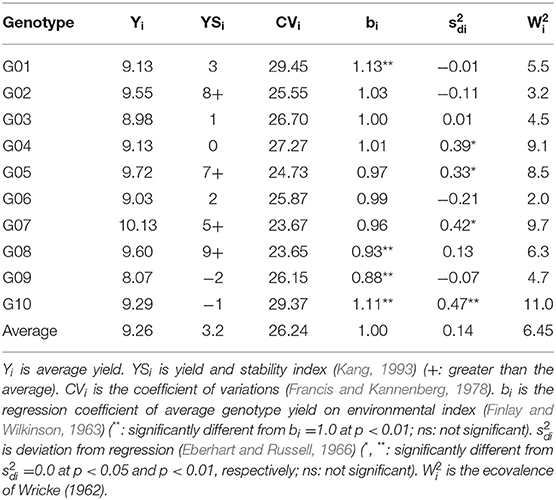
Table 4. Stability parameters on yield (t ha−1) for each genotype across normal and waterlogging conditions.
In regards to the GGE biplot analysis, the first two principal components (PC1 and PC2) could explain 66.4% of the G+GE variability. The environments were divided into five sectors and two mega-environments (Figure 2). The first mega-environment was represented by eight environments, namely N03, N05, N08, N09, N10, W12, W13, and W14. The second mega-environment was represented by five environments, namely N01, N02, N04, N06, and N07. One sector consisted of only a single environment N11 and therefore was not considered as a mega-environment. Besides, the biplot revealed five vertex genotypes, namely G07, G04, G09, G10, and G05, following the number of sectors established. Among those vertex genotypes, G07 and G05 belonged to the first and the second mega-environments, respectively, indicating that genotypes G07 and G05 were the most adaptive and high-yielding under each respective mega-environment. This result also indicated that the GEI effect was less dominant than the genotype main effect, even though both effects were highly significant on yield.
Waterlogging Stress Tolerance Indices
Stress tolerance indices (STIs) of test hybrids G01 and G03 were 0.59 and 0.60, respectively (Table 5), indicating that these hybrids were more susceptible to waterlogging and had lower yield potential than the other test hybrids. Test hybrids G02, G04, G05, G06, G07, and G08 had STI estimates of 0.70, 0.70, 0.74, 0.64, 0.90, and 0.79, respectively, implying that these hybrids were more tolerant to waterlogging and had higher yield potential than G01 and G03. Meanwhile, the two commercial checks G09 and G10 were more susceptible to waterlogging and had lower yield potential than the test hybrids since their STI estimates were 0.47 and 0.54, respectively.
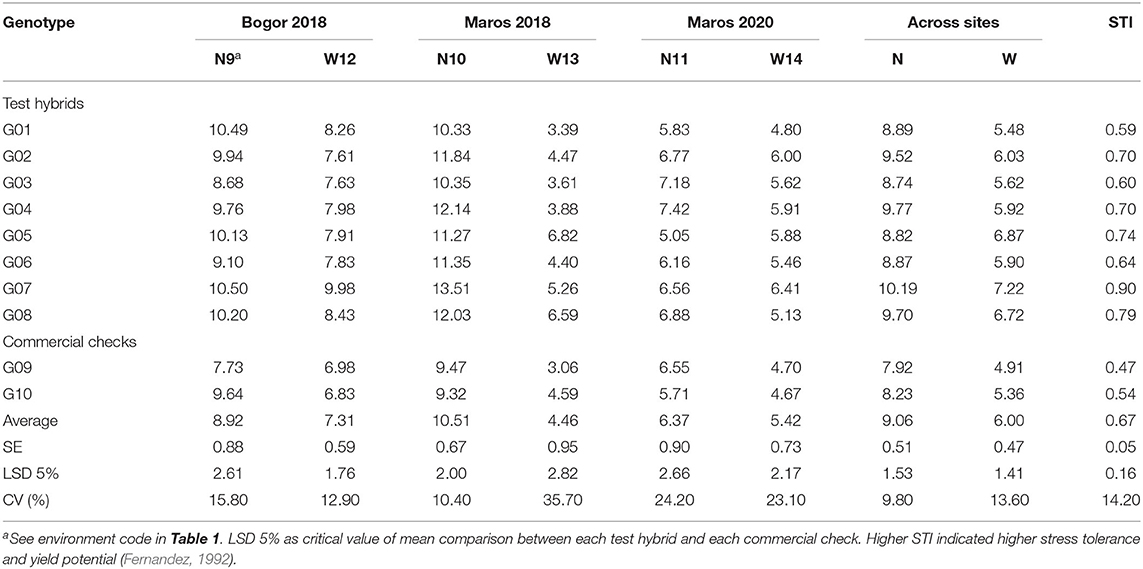
Table 5. Hybrid performance and stress tolerance index (STI) on yield (t ha−1) for each genotype evaluated under normal (N) and waterlogging (W) conditions in Bogor and Maros, 2018 and 2020.
The average yield in waterlogging (Ys) had a significantly positive correlation with that in normal conditions (Yp) (r = 0.76; p < 0.05) (Table 6). The stress susceptibility index (SSI) had a highly significant positive correlation with tolerance (TOL) (r = 0.87; p < 0.01), indicating that the higher the SSI, the more tolerant a genotype. Both Ys and Yp had positive and highly significant correlations with the stress tolerance index (STI) (r = 0.96 and r = 0.90, respectively; p < 0.01), but did not correlate with TOL (r = −0.12; p > 0.05). These results indicated that the STI could be included in the selection criteria to identify high-yielding genotypes in both normal and stress conditions.
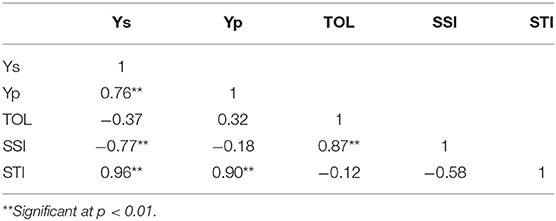
Table 6. Correlations among average yield in waterlogging (Ys) and normal (Yp) conditions, tolerance (TOL), stress susceptibility index (SSI), and stress tolerance index (STI).
A three-dimensional scatterplot was presented to visualize the average yield of each genotype under normal and stress conditions, as well as their tolerances to waterlogging (Figure 3). Test hybrid G05 was the most tolerant genotype with low yield reduction (1.95 t ha−1) from normal to waterlogging stress conditions. Besides, test hybrid G07 was the highest yielding genotype in both conditions.
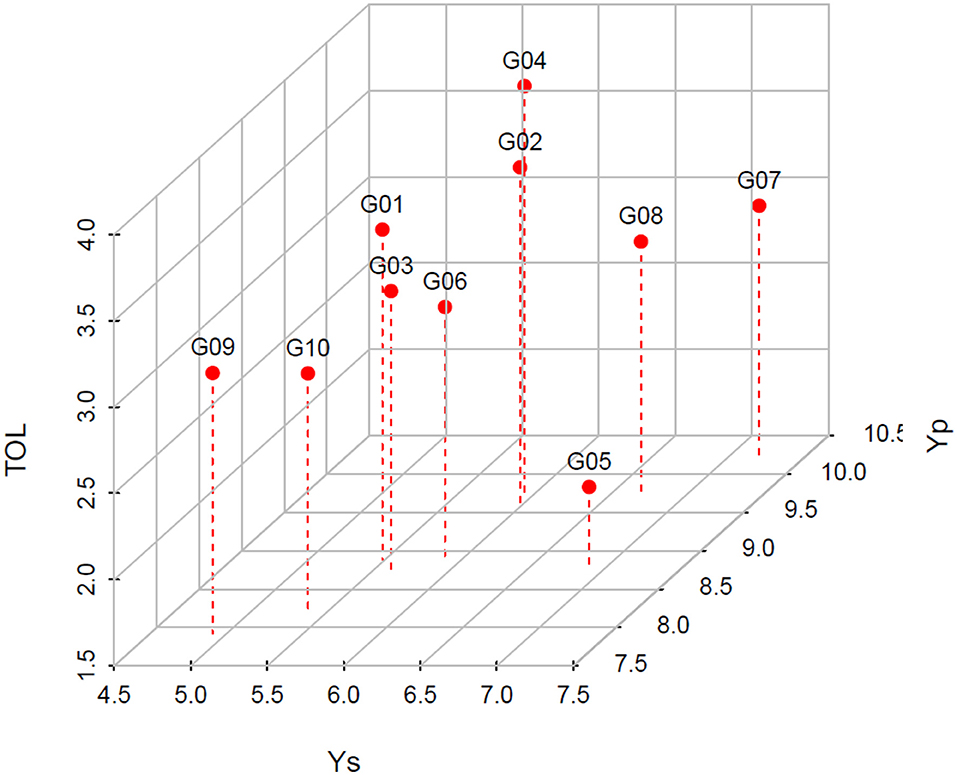
Figure 3. The average yields of each genotype under waterlogging (Ys) and normal (Yp) conditions and the tolerance (TOL) values.
Discussion
Effects of Environment, Genotype, and GEI
Genotype by environment interaction (GEI) in plant breeding is a phenomenon of the failure of the genotypes to be stable in different environmental conditions (Dudley and Moll, 1969). The significance of GEI on most observed traits in this study reaffirmed the necessity of complementary stability analysis such as joint regression analysis and GGE biplots to elucidate these interactions, determine the yield potential and stability of the test hybrids across environments, and identify adaptive hybrids in particular environmental conditions (Mafouasson et al., 2018).
The more significant effect of the genotype than the GEI on yield could be explained by the cultivar types (hybrid) and the source of parents for hybrid formation (well-adapted tropical x exotic). Most studies in maize on GEI interaction and yield stability focused on hybrid's evaluation, including our present study. Hybrid cultivars had been reported to be less sensitive to the GEI effect than inbred cultivars (Falcon et al., 2019). In addition to cultivar types, it seemed that the use of exotic germplasm such as MGold from CIMMYT and tropical germplasm MR14 from ICERI might enhance the significance of the genotype effect. The exploitations of exotic germplasm for crop breeding purposes had been reported in previous reports. Diverse waxy corn genotypes derived from subtropical China and tropical Vietnam as well as sweet corn genotypes with the half pedigree of the USA were introduced for enhancing the genotypic variance and genetic gains of sweet-waxy corn hybrids on agronomic traits, yield, eating quality, and kernel carbohydrate profiles (Dermail et al., 2020, 2022; Fuengtee et al., 2020).
Our study noticed that the GEI contributed more than the genotype to the total sum of the square, yet the genotype showed a more significant effect than the GEI on maize yield. This finding corroborated previous investigations in quality protein maize hybrids under different management conditions including optimum, drought, and low N (Mebratu et al., 2019) and in maize inbreds under different irrigation conditions (Falcon et al., 2019). Using the mean square to explain the total phenotypic variance, they mentioned the greater proportion of the GEI than the genotype. Other studies relying on the proportion of sum of squares to explain the total variation found the predominance effect of the test environment and the ratio of genotype effect over genotype + GEI was poor in tropical maize yield (Badu-Apraku et al., 2012; Mafouasson et al., 2018).
Waterlogging Stress Is Responsible for the Worse Performance of Tropical Maize
Waterlogging is one of the major abiotic stresses limiting crop growth. Hypoxia and anoxia are two key features when the waterlogging occurs for short and long periods, respectively (Dennis et al., 2000), and these effects are much more critical in maize, a well-known susceptible crop to waterlogging conditions compared to other crop species (Mano et al., 2006). The presence of waterlogging is generally led by several factors such as torrent precipitations, inappropriate irrigation practices, lacking or poor drainage system, and poor soil properties (Tian et al., 2020), and this stress would be more severe in maize growing areas under tropical rainforest such in Indonesia, which are regularly exposed by high precipitations as low as 60 mm per month in the driest month (Beck et al., 2018). Understanding the plant responses to waterlogging is necessary prior to improving the tolerance level via either conventional or molecular breeding (Lone et al., 2016).
In this study, we highlighted that the imposed waterlogging significantly inhibited maize plant height and ear height, slightly delayed maize flowering dates, and reduced maize yield components contributing to significant yield reduction. This finding supported previous studies reporting the adverse impacts of waterlogging in maize by reducing crop growth rate, plant height, ear height, leaf area index, chlorophyll content, photosynthetic rate, stomatal conductance, transpiration rate, root length, root length density, the number of root tips, ear length, ear diameter, grain row number per ear, 100-grain weight, grain number per ear, biomass accumulation, harvest index, and yield in summer maize (Ren et al., 2014, 2016b,c; Tian et al., 2019; Huang et al., 2022).
Furthermore, when waterlogging occurs, the roots are the first part of the plant to suffer damage, which can result in a reduction in the dry weight of the roots compared to normal conditions. Liu et al. (2010) reported that due to the significant performance differences between waterlogged and normal conditions, the dry weight of maize roots can be used as a selection criterion during genotype screening. The results of the study in the early vegetative phase indicated that hybrids G02 and G06 that were adaptive to waterlogging stress had a higher root shell weight than other hybrids that were sensitive to waterlogging (Syah et al., 2019). The G02 and G06 hybrids had a greater adventive root length than other hybrids that were susceptible to flooding. Certain maize genotypes can adapt to flooding conditions by developing aerenchyma tissue in their roots and stimulating adventitious root growth, which enables the roots to absorb oxygen from the air (Postma and Lynch, 2011).
The yield reduction of maize as affected by waterlogging is depending upon many factors including cultivar, crop growth stage, the duration of imposed stress, and the depth of water level. In our study, the imposed waterlogging was performed at the V6 stage for consecutive 10 days with 10 cm of water depth above ground level. Meanwhile, at the V3 stage, plant tissues and cell membranes are still soft so cell organelles, such as mitochondria and chloroplasts, could be damaged in waterlogging conditions (Ren et al., 2016b). Previous studies mentioned that the most detrimental effect of waterlogging on maize grain yield was at the V3 stage, followed by the V6 and VT stages (Ren et al., 2016a,b; Tian et al., 2019; Huang et al., 2022) at six consecutive days of imposed waterlogging (Ren et al., 2016a).
GEI and Stability Analysis Across Environments
Pre-commercial hybrid cultivars require extensive field trials in multiple representative locations for several years to determine their performance and adaptability (Rezende et al., 2020). Since the presence of GEI might generate the crossover performance of test hybrids and complicate hybrid evaluation under diverse environments (Kang, 1993), breeding programs generally include at least one stability analysis method to effectively parse the GEI effect and to be able to sort out genotypes that are stable, specifically adapted, or adapted to a wide range of environments (Farshadfar et al., 2012; Di Matteo et al., 2016).
This current study performed five stability parameters including the yield-stability index (Kang, 1993), the mean-coefficient of variations (CV) method (Francis and Kannenberg, 1978), the linear regression coefficient (Finlay and Wilkinson, 1963), the deviation from regression (Eberhart and Russell, 1966), and the ecovalence of Wricke (1962). In our study, four test hybrids (G02, G05, G07, and G08) showed high and positive both yield and stability index, surpassing the average means (Table 4). Kang (1993) suggested that the selected genotypes should possess higher values of stability index than the average value. Those hybrids also had lower CVs than the average estimates. Francis and Kannenberg (1978) mentioned that the genotypes with high yield and low CV across environments are favorable and stable. Furthermore, among those selected hybrids, genotype G02 was the most favorable for the other three parameters (Table 4). This hybrid showed a regression coefficient (bi) that was not significantly different from 1.0, a deviation from regression () that was not significantly different from zero, and a small ecovalence, indicating that it performed well in regards to the average stability and broad adaptation (Wricke, 1962; Finlay and Wilkinson, 1963; Eberhart and Russell, 1966).
The GGE biplot displayed a polygon view of the “which-won-where” patterns by dividing all environments into different sectors, and each sector would have a particular vertex genotype that represents the most adaptive and highest yielding genotype in the respective sector (Yan et al., 2000; Yan and Tinker, 2005, 2006). In our study, 14 sets of environments were divided into five sectors; thus, five vertex genotypes (G04, G09, G10, G05, and G07) could be noticed (Figure 2). However, two out of the five sectors where G09 and G10 were the vertex genotypes had no environment, indicating that these genotypes performed poorly in some or even all of the test environments. Meanwhile, the rest of the genotypes (G01, G02, G03, G06, and G08) in our study were relatively more stable or less responsive than the vertex genotypes because those genotypes were located within the polygon. Two of the five sectors where G07 and G05 were the vertex genotypes were designated as mega-environments because they comprised most subsets of the test environments and shared the best set of genotypes (Yan and Rajcan, 2002). Gauch and Zobel (1997) suggested that it is necessary to promote different genotypes for different mega-environments.
The above explanations elucidated that five stability parameters altogether used in this study could notice four favorable hybrids, and performing the GGE biplot as a complementary stability analysis can refine the preliminary results to be more convincing, for instance, four favorable hybrids (G02, G05, G07, and G08) derived from five stability parameters to two selected hybrids derived from the GGE biplot (G05 and G07). These results indicated that five stability parameters complemented by the GGE biplot can be applied for simultaneous selection in genotype evaluation.
Waterlogging Stress Tolerance Indices
Breeding maize hybrids for tolerance and adaptability to waterlogging is of interest, especially for now and the near future, as the world is facing the challenges of climate change. While maize hybrids adapted to waterlogging could benefit farmers with their high yield in waterlogging conditions, tolerant genotypes could serve as germplasm for breeding programs to develop high yielding hybrids in both normal and waterlogging conditions with small yield reduction. However, the development of hybrid maize with good yield retention under various levels of waterlogging is more challenging than with other crops because maize plants have no naturally occurring aerenchyma; the air spaces in the roots are for retaining the oxygen, like in the rice plants (Colmer, 2003). Instead, maize plants are still able to establish the aerenchyma made from the death of cells in the root apex after the plants produce the ethylene as the first signal of experiencing stress (Jackson, 1989; Lone et al., 2016). Previous studies proposed several morpho-physiological and anatomical traits related to plant mechanisms to withstand the waterlogging stress including the presence of early brace root, the capacity to form aerenchyma, enhanced root porosity, and suberization, reduced CO2 exchange and leaf chlorophyll contents, ethanolic fermentation, and increased available soluble sugar and carbohydrate stocks (Zaidi and Singh, 2001; Subbaiah and Sachs, 2003; Mano et al., 2005; Zaidi et al., 2007). In our present study, genotype G05 possessed the best yield retention in waterlogging and G07 was the most adaptive in both conditions (Figure 3). Genotypes G05 and G07 have been released in 2021 as new maize hybrid varieties in Indonesia named “JHG 01” and “JHG 02”, respectively. Future studies are encouraged to emphasize how those two varieties could withstand the waterlogging stress by elucidating the morpho-physio-anatomical adaptations.
Our study showed that the stress tolerance index (STI) had significant and positive correlations with both yields in waterlogging (Ys) and in normal (Yp) conditions. Conventional breeding is a numbers game (Araus and Cairns, 2004): the more genotypes are evaluated, the higher possibilities to obtain favorable ones. Therefore, it is suggested that STI can be included in the selection criteria for practical reasons since this parameter is rooted in a yield-equation basis which does not require any extent investment in labor, time, and other resources. Previous studies adopted this concept to evaluate the maize genotypes tolerant to drought (Tollenaar and Lee, 2002) and salinity (Masuda et al., 2021).
Conclusion
Genotype, location, waterlogging, and GEI effects were significant on several agronomic traits of tropical maize hybrids evaluated across 14 environments under normal and waterlogging conditions. G05 is the most tolerant genotype to waterlogging, while G07 was the highest yielding genotype under normal and waterlogging conditions. Stress tolerance index is correlated with yield in both conditions, and therefore may be considered as a selection criterion along with stability indices for breeding widely adapted maize hybrids.
Data Availability Statement
The datasets presented in this article are not readily available because they may be used in future meta-analysis studies. Requests to access the datasets should be directed to azraimulia@gmail.com.
Author Contributions
All authors listed have made a substantial, direct, and intellectual contribution to the work and approved it for publication.
Funding
We would like to express our gratitude to the Ministry of Research and Technology/National Research and Innovation Agency of the Republic of Indonesia (BRIN) for funding research support under this National Research Priority Grant Program in 2020 (Contract Number: 16/E1/PRN/2020) and to the Indonesian Agency for Agricultural Research and Development, Ministry of Agriculture, Indonesia, for funding research support in 2019 (Contract Number: SP DIPA-018.09.2.238080/20117-2019).
Conflict of Interest
The authors declare that the research was conducted in the absence of any commercial or financial relationships that could be construed as a potential conflict of interest.
Publisher's Note
All claims expressed in this article are solely those of the authors and do not necessarily represent those of their affiliated organizations, or those of the publisher, the editors and the reviewers. Any product that may be evaluated in this article, or claim that may be made by its manufacturer, is not guaranteed or endorsed by the publisher.
Acknowledgments
We wish to thank CIMMYT for sharing one of the inbred lines as the parent of the tester in this research.
References
Agus, F., Andrade, J. F., Rattalino Edreira, J. I., Deng, N., Purwantomo, D. K. G., and Agustiani, N. (2019). Yield gaps in intensive rice-maize cropping sequences in the humid tropics of Indonesia. Field Crops Res. 237, 12–22. doi: 10.1016/j.fcr.2019.04.006
Araus, J. L., and Cairns, J. E. (2004). Field high-throughput phenotyping: the new crop breeding frontier. Trends Plant Sci. 19, 52–61. doi: 10.1016/j.tplants.2013.09.008
Arritt, R. W., and Rummukainen, M. (2011). Challenges in regional-scale climate modeling. Bull. Am. Meteorol. Soc. 92, 365–368. doi: 10.1175/2010BAMS2971.1
Badu-Apraku, B., Oyekunle, M., Obeng-Antwi, K., Osuman, A. S., Ado, S. G., Coulibay, N., et al. (2012). Performance of extra-early maize cultivars based on GGE biplot and AMMI analysis. J. Agric. Sci. 150, 473–483. doi: 10.1017/S0021859611000761
Bailey-Serres, J., Lee, S. C., and Brinton, E. (2012). Waterproofing crops: effective flooding survival strategies. Plant Physiol. 160, 1698–1709. doi: 10.1104/pp.112.208173
Barrett-Lennard, E. G. (2003). The interaction between water logging and salinity in higher plants: Causes, consequences and implications. Plant Soil. 253, 35–54. doi: 10.1023/A:1024574622669
Beck, H. E., Zimmermann, N. E., McVicar, T. R., Vergopolan, N., Berg, A., and Wood, E. F. (2018). Present and future Köppen-Geiger climate classification maps at 1-km resolution. Sci. Data 5. doi: 10.1038/sdata.2018.214
CIMMYT (2004). The CIMMYT Maize Program. Maize Diseases: A Guide for Field Identification, 4th Edn. Mexico, D.F.: CIMMYT.
Colmer, T. D. (2003). Aerenchyma and an inducible barrier to radial oxygen loss facilitate root aeration in upland, paddy and deep-water rice (Oryza sativa L.). Ann. Bot. 91, 301–309. doi: 10.1093/aob/mcf114
Dennis, E. S., Dolferus, R., Ellis, M., Rahman, M., Wu, Y., Hoeren, F. U., et al. (2000). Molecular strategies for improving waterlogging tolerance in plants. J. Exp. Bot. 51, 89–97. doi: 10.1093/jexbot/51.342.89
Dermail, A., Fuengtee, A., Lertrat, K., Suwarno, W. B., Lübberstedt, T., and Suriharn, K. (2022). Simultaneous selection of sweet-waxy corn ideotypes appealing to hybrid seed producers, growers, and consumers in Thailand. Agronomy 12, 87. doi: 10.3390/agronomy12010087
Dermail, A., Suriharn, B., Chankaew, S., Sanitchon, J., and Lertrat, K. (2020). Hybrid prediction based on SSR-genetic distance, heterosis and combining ability on agronomic traits and yields in sweet and waxy corn. Sci. Hortic. 259, 108817. doi: 10.1016/j.scienta.2019.108817
Di Matteo, J. A., Ferreyra, J. M., Cerrudo, A. A., Echarte, L., and Andrade, F. H. (2016). Yield potential and yield stability of Argentine maize hybrids over 45 years of breeding. Field Crops Res. 197, 107–116. doi: 10.1016/j.fcr.2016.07.023
Dias, K. O. D. G., Gezan, S. A., Guimarães, C. T., Parentoni, S. N., Guimarães, P. E. D. O., Carneiro, N. P., et al. (2018). Estimating genotype × environment interaction for and genetic correlations among drought tolerance traits in maize via factor analytic multiplicative mixed models. Crop Sci. 58, 72–83. doi: 10.2135/cropsci2016.07.0566
Dudley, J. W., and Moll, R. H. (1969). Interpretation and use of estimates of heritability and genetic variances in plant breeding. Crop Sci. 9, 257–262 doi: 10.2135/cropsci1969.0011183X000900030001x
Eberhart, S. A., and Russell, W. A. (1966). Stability parameters for comparing varieties. Crop Sci. 6, 36–40. doi: 10.2135/cropsci1966.0011183X000600010011x
Edmeades, G. O., Bolanos, J., Chapman, S. C., Lafitte, H. R., and Banziger, M. (1999). Selection improves drought tolerance in tropical maize populations. I. Gains in biomass, grain yield, and harvest index. Crop Sci. 39, 1306–1315. doi: 10.2135/cropsci1999.3951306x
Erenstein, O. (2010). The evolving maize sector in Asia: challenges and opportunities. J. New Seeds 11, 1–15. doi: 10.1080/15228860903517770
Falcon, C. M., Kaeppler, S. M., Spalding, E. P., Miller, N. D., Haase, N., AlKhalifah, N., et al. (2019). Relative utility of agronomic, phenological, and morphological traits for assessing genotype-by-environment interaction in maize inbreds. Crop Sci. 60, 62–81. doi: 10.1002/csc2.20035
Farshadfar, E., Mohammadi, R., Aghaee, M., and Vaisi, Z. (2012). GGE biplot analysis of genotype x environment interaction in wheat-barley disomic addition lines. Aust. J. Crop Sci. 6, 1074–1079.
Fernandez, G. C. J. (1992). “Effective selection criteria for assessing plant stress tolerance” in Proceedings of the International Symposium on Adaptation of Vegetables and Other Food Crops in Temperature and Water Stress Chapter 25, ed. C. G. Kuo. Tainan: Shanhua: AVRDC Publication. p. 257–270.
Finlay, K. W., and Wilkinson, G. N. (1963). The analysis of adaptation in a plant breeding programme. Aust. J. Agric. Res. 14, 742–754. doi: 10.1071/AR9630742
Francis, T. R., and Kannenberg, L. Y. (1978). Yield stability studies in short-season maize. 1. A descriptive method for grouping genotypes. Can. J. Plant Sci. 58, 1029–1034. doi: 10.4141/cjps78-157
Fuengtee, A., Dermail, A., Simla, S., Lertrat, K., Sanitchon, J., Chankaew, S., et al. (2020). Combining ability for carbohydrate components associated with consumer preferences in tropical sweet and waxy corn derived from exotic germplasm. Turk. J. Field Crops 25, 147–155. doi: 10.17557/tjfc.831964
Gauch, H., and Zobel, R. W. (1997). Identifying mega-environments and targeting genotypes. Crop Sci. 37, 311–326. doi: 10.2135/cropsci1997.0011183X003700020002x
Gauch, H. G. (1992). Statistical Analysis of Regional Yield Trials: AMMI Analysis of Factorial Designs. Amsterdam: Elsevier.
Gomez, K. A., and Gomez, A. A. (1984). Statistical Procedure for Agricultural Research. Singapore: John Wiley and Sons.
Grote, U., Fasse, A., Nguyen, T. T., and Erenstein, O. (2021). Food security and the dynamics of wheat and maize value chains in Africa and Asia. Front. Sustain. Food Syst. 4, 317. doi: 10.3389/fsufs.2020.617009
Huang, C., Gao, Y., Qin, A., Liu, Z., Zhao, B., Ning, D., et al. (2022). Effects of waterlogging at different stages and durations on maize growth and grain yields. Agric. Water Manag. 261, 107334. doi: 10.1016/j.agwat.2021.107334
Jackson, M. B. (1989). “Regulation of aerenchyma formation in roots and shoots by oxygen and ethylene” in Cell Separation in Plants. NATO ASI Series (Series H: Cell Biology). eds. D. J. Osborne and M. B. Jackson. Berlin: Springer. vol 35. doi: 10.1007/978-3-642-74161-6_25
Kang, M. S. (1993). Simultaneous selection for yield and stability in crop performance trials: consequences for growers. Agron. J. 85, 754–757. doi: 10.2134/agronj1993.00021962008500030042x
Kang, M. S. (1998). Using genotype-by-environment interaction for crop cultivar development. Adv. Agron. 62:199–252. doi: 10.1016/S0065-2113(08)60569-6
Kenga, R. (2001). Combining ability estimates and heterosis in selected tropical sorghum (Sorghum bicolor (L.) Moench). [dissertation thesis]. [Zaria]: Ahmadu Bello University Zaria.
Khalil, I. A., Rahman, H. U., Rehman, N. U., Arif, M., Khalil, I. H., Iqbal, M., et al. (2011). Evaluation of maize hybrids for grain yield stability in north-west of Pakistan. Sarhad J. Agric. 27, 213–218.
Khan, N. A., Yu, P., Ali, M., Cone, J. W., and Hendriks, W. H. (2015). Nutritive value of maize silage in relation to dairy cow performance and milk quality. J. Sci. Food Agric. 95, 238–252. doi: 10.1002/jsfa.6703
Li, C., Jiang, D., Wollenweber, B., Li, Y., Dai, T., and Cao, W. (2011). Waterlogging pretreatment during vegetative growth improves tolerance to waterlogging after anthesis in wheat. Plant Sci. 180, 672–678. doi: 10.1016/j.plantsci.2011.01.009
Liu, K., Harrison, M. T., Archontoulis, S. V., Huth, N., Yang, R., Liu, D. L., et al. (2021). Climate change shifts forward flowering and reduces crop waterlogging stress. Environ. Res. Lett. 16, 094017. doi: 10.1088/1748-9326/ac1b5a
Liu, K., Harrison, M. T., Hunt, J., Angessa, T. T., Meinke, H., Li, C., et al. (2020a). Identifying optimal sowing and flowering periods for barley in Australia: a modelling approach. Agric. For. Meteorol. 282,107871. doi: 10.1016/j.agrformet.2019.107871
Liu, K., Harrison, M. T., Ibrahim, A., Manik, S. M. N., Johnson, P., Tian, X., et al. (2020b). Genetic factors increasing barley grain yields under soil waterlogging. Food Energy Secur. 9, e238. doi: 10.1002/fes3.238
Liu, K., Harrison, M. T., Shabala, S., Meinke, H., Ahmed, I., Zhang, Y., et al. (2020c). The state of the art in modeling waterlogging impacts on plants: what do we know and what do we need to know. Earth's Futur. 8, e2020EF001801. doi: 10.1029/2020EF001801
Liu, K., Harrison, M. T., Wang, B., Yang, R., Yan, H., Zou, J., et al. (2022). Designing high-yielding wheat crops under late sowing: a case study in southern China. Agron. Sustain. Dev. 42, 29. doi: 10.1007/s13593-022-00764-w
Liu, Y. Z., Tang, B., Zheng, Y. L., Ma, K. J., Xu, S. Z., and Qiu, F. Z. (2010). Screening methods for waterlogging tolerance at maize (Zea mays L.) seedling stage. Agri. Sci. China 9, 362–369. doi: 10.1016/S1671-2927(09)60105-X
Lone, A. A., Khan, M. H., Dar, Z. A., and Wani, S. H. (2016). Breeding strategies for improving growth and yield under waterlogging conditions in maize: a review. Maydica 61, 11.
Mafouasson, H. N. A., Gracen, V., Yeboah, M. A., Ntsomboh-Ntsefong, G., Tandzi, N. L., and Mutengwa, C. (2018). Genotype-by-environment interaction and yield stability of maize single cross hybrids developed from tropical inbred lines. Agronomy 8, 62, 1–17. doi: 10.3390/agronomy8050062
Mano, Y., Muraki, M., Fujimori, M., Takamizo, T., and Kindiger, B. (2005). AFLP-SSR maps of maize x teosinte and maize x maize: comparison of map length and segregation distortion. Plant Breed. 124, 432–439. doi: 10.1111/j.1439-0523.2005.01128.x
Mano, Y., Muraki, M., Komatsu, T., Fujimori, M., Akiyama, F., and Takamizo, T. (2002). Varietal difference in pre-germination flooding tolerance and waterlogging tolerance at the seedling stage in maize inbred accessions. Jpn. J. Crop Sci. 71, 361–367. doi: 10.1626/jcs.71.361
Mano, Y., Muraki, M., and Takamizo, T. (2006). Identification of QTL controlling flooding tolerance in reducing soil conditions in maize (Zea mays L.) seedlings. Plant Prod. Sci. 9, 176–181. doi: 10.1626/pps.9.176
Masuda, M. S., Azad, M. A. K., Hasanuzzaman, M., and Arifuzzaman, M. (2021). Evaluation of salt tolerance in maize (Zea mays L.) at seedling stage through morphological characters and salt tolerance index. Plant Physiol. Rep. 26, 419–427. doi: 10.1007/s40502-021-00611-2
Mebratu, A., Wegary, D., Mohammed, W., Teklewold, A., and Tarekegne, A. (2019). Genotype environment interaction of quality protein maize hybrids under contrasting management conditions in eastern and southern Africa. Crop Sci. 59, 1576–1589. doi: 10.2135/cropsci2018.12.0722
Postma, J. A., and Lynch, J. P. (2011). Root cortical aerenchyma enhances the growth of maize on soils with suboptimal availability of nitrogen, phosphorus, and potassium. Plant Physiol. 156, 1190–201. doilink[10.1104/pp.111.175489]10.1104/pp.111.175489
Prasanna, Y. L., and Ramarao, G. (2014). Effect of waterlogging on physiological and biochemical parameters and seed yield in greengram genotypes. Int. J. Food Agric. Vet. Sci. 4,176–183.
Rathore, T. R., Warsi, M. Z. K., Lothrop, J. E., and Singh, N. N. (1998). “Production of maize under excess soil moisture (waterlogging) conditions” in Proceedings of the Second Asian Regional Maize Workshop PACARD (Los Banos: CIMMYT), 23–27.
Ren, B., Dong, S., Zhao, B., Liu, P., and Zhang, J. (2017). Responses of nitrogen metabolism, uptake and translocation of maize to waterlogging at different growth stages. Front. Plant Sci. 8, 1216. doi: 10.3389/fpls.2017.01216
Ren, B., Zhang, J., Dong, S., Liu, P., and Zhao, B. (2016b). Effects of waterlogging on leaf mesophyll cell ultrastructure and photosynthetic characteristics of summer maize. PLoS ONE 11, e0161424. doi: 10.1371/journal.pone.0161424
Ren, B., Zhang, J., Dong, S., Liu, P., and Zhao, B. (2016c). Effects of duration of waterlogging at different growth stages on grain growth of summer maize (Zea mays L.) under field conditions. J. Agron. Crop Sci. 202, 564–575. doi: 10.1111/jac.12183
Ren, B. Z., Zhang, J. W., Dong, S. T., Liu, P., and Zhao, B. (2016a). Root and shoot responses of summer maize to waterlogging at different stages. Agron. J. 108, 1060–1069. doi: 10.2134/agronj2015.0547
Ren, B. Z., Zhang, J. W., Li, X., Fan, X., Dong, S. T., Liu, P., et al. (2014). Effects of waterlogging on the yield and growth of summer maize under field conditions. Can. J. Crop Sci. 94, 23–31. doi: 10.4141/cjps2013-175
Rezende, W. S., Beyene, Y., Mugo, S., Ndou, E., Gowda, M., serumaga, S, et al. (2020). Performance and yield stability of maize hybrids in stress-prone environments in eastern Africa. Crop J. 8, 107–118. doi: 10.1016/j.cj.2019.08.001
Sachs, M. M., Subbaiah, C. C., and Saab, I. N. (1996). Anaerobic gene expression and flooding tolerance in maize. J. Exp. Bot. 47, 1–15. doi: 10.1093/jxb/47.1.1
Sairam, R., Kumutha, D., Ezhilmathi, K., Deshmukh, P., and Srivastava, G. (2008). Physiology and biochemistry of waterlogging tolerance in plants. Biol. Plant 52, 401–412. doi: 10.1007/s10535-008-0084-6
Singamsetti, A., Shahi, J. P., Zaidi, P. H., Seetharam, K., Vinayan, M. T., Kumar, M., et al. (2021). Genotype × environment interaction and selection of maize (Zea mays L.) hybrids across moisture regimes. Field Crops Res. 270, 108224. doi: 10.1016/j.fcr.2021.108224
Subbaiah, C. C., and Sachs, M. M. (2003). Molecular and cellular adaptations of maize to flooding stress. Ann. Bot. 91, 119–127. doi: 10.1093/aob/mcf210
Syah, U. T. (2019). Adaptability of Corn Genotypes to Waterlogging Stress. MS Thesis, Bogor, West Java: IPB University. (In Indonesian).
Syah, U. T., Suwarno, W. B., and Azrai, M. (2019). Vegetative-phase selection trait for adaptation to waterlogging stress in maize. J. Agron. Indonesia 47, 134–140. doi: 10.24831/jai.v47i2.24356
Tian, L., Bi, W., Ren, X., Li, W., Sun, L., and Li, J. (2020). Flooding has more adverse effects on the stem structure and yield of spring maize (Zea mays L.) than waterlogging in Northeast China. Eur. J. Agron. 117, 126054. doi: 10.1016/j.eja.2020.126054
Tian, L., Li, J., Bi, W., Zuo, S., Li, L., Li, W., et al. (2019). Effects of waterlogging stress at different growth stages on the photosynthetic characteristics and grain yield of spring maize (Zea mays L.) under field conditions. Agric. Water Manag. 218, 250–258. doi: 10.1016/j.agwat.2019.03.054
Timsina, J., Jat, M. L., and Majumdar, K. (2010). Rice-maize systems of South Asia: Current status, future prospects and research priorities for nutrient management. Plant Soil 335, 65–82. doi: 10.1007/s11104-010-0418-y
Tollenaar, M., and Lee, E. A. (2002). Yield potential, yield stability and stress tolerance in maize. Field Crops Res. 75, 161–169. doi: 10.1016/S0378-4290(02)00024-2
Tripathi, S., Warsi, M. Z. K., and Verma, S. S. (2003). Water logging tolerance in inbred lines of maize (Zea mays L.). Cereal Res. Commun. 31, 221–226. doi: 10.1007/BF03543271
Wricke, G. (1962). On a method of understanding the biological diversity in field research. Z. Planzenzuchtg 47, 92–146.
Yan, H., Harrison, M. T., Liu, K., Wang, B., Feng, P., Fahad, S., et al. (2022). Crop traits enabling yield gains under more frequent extreme climatic events. Sci. Total Environ. 808, 152170. doi: 10.1016/j.scitotenv.2021.152170
Yan, W., Hunt, L. A., Sheng, Q., and Szlavnics, Z. (2000). Cultivar evaluation and mega-environment investigation based on GGE biplot. Crop Sci. 40, 597–605. doi: 10.2135/cropsci2000.403597x
Yan, W., and Rajcan, I. (2002). Biplot evaluation of test sites and trait relations of soybean in Ontario. Crop Sci. 42, 11–20. doi: 10.2135/cropsci2002.1100
Yan, W., and Tinker, N. A. (2006). Biplot analysis of multi-environment trial data: principles and applications. Can. J. Plant Sci. 86, 623–645. doi: 10.4141/P05-169
Yan, W. K., and Tinker, N. A. (2005). An integrated biplot analysis system for displaying, interpreting, and exploring genotype × environment interaction. Crop Sci. 45, 1004–1016. doi: 10.2135/cropsci2004.0076
Zaidi, P. H., Maniselvan, P., Sultana, R., Yadav, M., Singh, R. P., Singh, S. B., et al. (2007). Importance of secondary traits in improvement of maize (Zea mays L.) for enhancing tolerance to excessive soil moisture stress. Cer. Res. Commun. 35, 1427–1435 doi: 10.1556/CRC.35.2007.3.7
Zaidi, P. H., Rafique, S., Rai, P. K., Singh, N. N., and Srinivasan, G. (2004). Tolerance to excess moisture in maize (Zea mays L.): susceptibile crop stages and identification of tolerant genotypes. Field Crops Res. 90, 189–202. doi: 10.1016/j.fcr.2004.03.002
Zaidi, P. H., and Singh, N. N. (2001). Effect of waterlogging on growth, biochemical compositions and reproduction in maize. J. Plant Biol. 28, 61–70.
Zaidi, P. H., Zaman-Allah, M., Trachsel, S., Seetharam, K., Cairns, J. E., and Vinayan, M. T. (2016). Phenotyping for Abiotic Stress Tolerance in Maize Heat Stress: A Field Manual. Mexico City: CIMMYT.
Keywords: hybrid breeding, maize, stress tolerance index (STI), waterlogging stress, yield stability
Citation: Azrai M, Efendi R, Muliadi A, Aqil M, Suwarti, Zainuddin B, Syam A, Junaedi, Syah UT, Dermail A, Marwiyah S and Suwarno WB (2022) Genotype by Environment Interaction on Tropical Maize Hybrids Under Normal Irrigation and Waterlogging Conditions. Front. Sustain. Food Syst. 6:913211. doi: 10.3389/fsufs.2022.913211
Received: 05 April 2022; Accepted: 19 May 2022;
Published: 24 June 2022.
Edited by:
Matteo Balderacchi, Independent Researcher, Piacenza, ItalyReviewed by:
Baizhao Ren, Shandong Agricultural University, ChinaKe Liu, University of Tasmania, Australia
Feng Yu, Hubei University, China
Hermann Restrepo-Diaz, National University of Colombia, Colombia
Copyright © 2022 Azrai, Efendi, Muliadi, Aqil, Suwarti, Zainuddin, Syam, Junaedi, Syah, Dermail, Marwiyah and Suwarno. This is an open-access article distributed under the terms of the Creative Commons Attribution License (CC BY). The use, distribution or reproduction in other forums is permitted, provided the original author(s) and the copyright owner(s) are credited and that the original publication in this journal is cited, in accordance with accepted academic practice. No use, distribution or reproduction is permitted which does not comply with these terms.
*Correspondence: Willy Bayuardi Suwarno, willy@apps.ipb.ac.id