- 1Business School, The University of Queensland, Brisbane, QLD, Australia
- 2ARC Centre of Excellence in Synthetic Biology, The University of Queensland, Brisbane, QLD, Australia
The need to meet the food demands of the world's growing population is the main challenge to global agricultural policy and economy. Issues in food security require innovative solutions. Modern biotechnology has a significant potential to contribute to food security, wealth, and sustainable development. Genetic engineering offers tools to improve nutrition, increase yield, and enhance crop resilience. New techniques of genome editing provide ample means to overcome limitations inherent in conventional plant breeding, but their industrial applicability depends on regulatory environment, decision making, and public perception. An alignment of goals between science and policy can help realise the potential of modern biotechnology to contribute to food security, wealth, and sustainable development.
Introduction
The development and use of plant breeding technologies can contribute to food security (Tester and Langridge, 2010; Zaidi et al., 2019). Agricultural biotechnology is part of the science of plant breeding that arguably provides solutions to world hunger (Mackey, 2003; Trivedi et al., 2017). Agricultural biotechnology is indispensable to plant breeding strategies aimed at improving abiotic stress tolerance (Varshney et al., 2011; Amin et al., 2019; Hossain et al., 2021).
However, the efforts of scientists advocating crop genetic improvement technologies have been met with public resistance to genetically modified (GM) foods and crops. Even regarded by scientists as safe, new genetic technologies come under public pressure questioning innovations on ethical and socio-economic grounds (Schurman and Kelso, 2003; Anyshchenko, 2019; Beumer, 2019). The years of debates over genetically modified organisms (GMOs) have been especially circulating around the ethics of cloning and combining genes from unrelated species (Rollin, 2012). It was suggested that GM debate overlooks the relevance of crop genetic improvement techniques to food security and environmental protection because we lack a broader vision of global issues (Popp et al., 2013). There are also other obstacles to the use of agricultural biotechnology in addressing the problem of climate change and food security, e.g., labelling requirements, coexistence management and intellectual property rights, including the property rights of indigenous peoples who may have cultivated landraces and selected wild relatives for centuries (Powledge, 2010).
The proximate cause of opposition to food produced from biotech crops is rooted in the complexity of socio-technical integration. Linear incorporation of biotechnological inventions into society can fail due to complex considerations involved in the matter of socio-technological integration. This possibility of failure begs the question: How to ensure that biotechnology contributes to sustainable agriculture in a safe and responsible way for the benefit of society? A hypothesis to test the research question is that new tools and methods of agricultural biotechnology as a solution to food security can ensure socially responsible scientific practise through building capacities which catalyse the endorsement of a new technology by stakeholders and its acceptance by end users.
Crop improvement is a cornerstone of global food security (Ravanbakhsh et al., 2021). Climate change sends serious challenge to global food security, exacerbating biotic and abiotic stresses that restrict plant productivity (Ul Haq et al., 2019). The threat that climate change poses to agriculture can be mitigated by using genome editing for the benefit of societies challenged by malnutrition. The use of CRISPR/Cas9 shows great promise as a powerful tool to improve plant nutrition, enhance plant disease resistance, and produce drought tolerant plants (Arora and Narula, 2017). The potential for biotechnology to contribute to sustainable agriculture is analysed trough the framework of issues and solutions sorted around socio-economic, environmental and technological aspects of the hypothesis (Table 1).
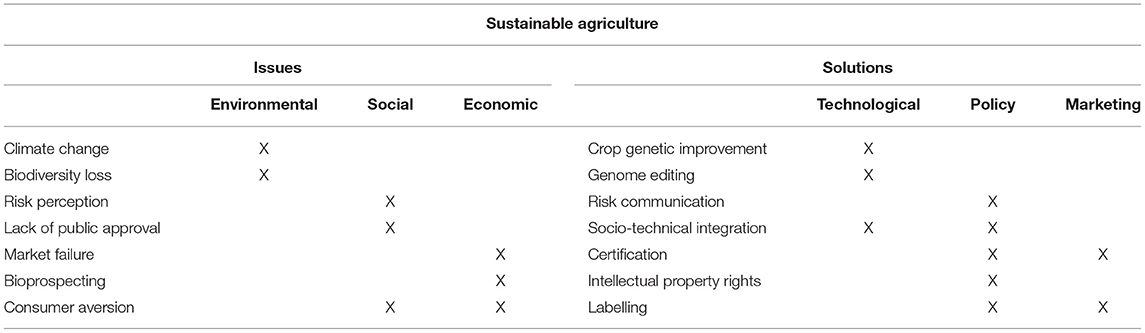
Table 1. Framework for analysing the ways in which biotechnology is hypothesised to contribute to sustainable agriculture.
The above table demonstrates that biotechnology is placed in a broad agricultural context. Among other solutions to issues in food security, genome editing has been the most recent and one of the most contested achievements of life sciences put to the purpose of feeding the world. Genome editing has proven to be an effective solution to the limitations posed by climate change. Researchers across the world have demonstrated that genome editing helps in increasing abiotic stress tolerance, enabling salinity, and drought tolerance, and enhancing disease resistance (Karavolias et al., 2021). Despite its success in research, genome editing has not always transitioned into marketed applications. Most of technological advances in genome editing have occurred recently, and the prospects of their commercialisation has yet to be estimated. On the one hand, genome editing is a powerful mechanism of agricultural improvement capable of providing solutions to issues in food security exacerbated by climate change. On the other hand, real success of genome editing depends on factors beyond technological solutions. Social justice, economic equity, regulatory clarity are among numerous non-technological issues that will determine the future of innovation in agricultural biotechnology.
The framework for analysing the ways in which biotechnology could contribute to sustainable agriculture is not exhaustive in its parameters because new technological developments are susceptible to various estimations of their costs and benefits. The history of agriculture has shown that risks are inherent in every solution, ie reduced biodiversity in result of monoculture farming, or soil contamination due to heavy use of pesticides and herbicides. As complex as it is, gene editing is but a technology embedded into the institutional infrastructure of science within society. The dynamic policy and socio-economic entanglements need to be considered when assessing the prospects of achieving food security in a changing world.
Food Security Under Climate Change
The development of industry and technology has caused anthropogenic climate change with increasingly devastating effects that damage crops and infrastructure. Climate change, the dominant driver of biodiversity loss (Bahadur et al., 2015), has a dramatic impact on plants. For example, the grain yield of tropical wheat is predicted to decline to a notably large amount if the increase in local temperature exceeds 3°C above pre-industrial levels, regardless of adaptive measures such as planting different crops (Parry, 2007). Some scientific assessments envisage that under higher emission scenarios a global average 2°C warming threshold is likely to be crossed between 2040 and 2060 (Joshi et al., 2011).
About 2 billion people in the world experience food insecurity (FAO, 2021). Some 900 million people, primarily in developing countries, have no sufficient access to food. By 2050 the world's population is estimated to have grown to more than nine billion (Godfray et al., 2010), which will increase global food demand by 60 per cent (Alexandratos and Bruinsma, 2012). Increase in food production will also increase the use of water and arable land areas. Agriculture is particularly vulnerable to climate change (Pörtner et al., In Press). There is not much prospect of meeting the nutritional needs of the world in the mid-21st century by means of current agricultural practises (Davies and Bowman, 2016). To meet the demand, food production must be improved.
The 2030 Agenda for Sustainable Development comprises 17 Sustainable Development Goals (SDGs) aimed at ending poverty, hunger and inequality, taking action on the environment and climate change, and improving access to health and education. The need to feed the world is recognised by the UN General Assembly as a global issue: a firm intention to end hunger, achieve food security and improved nutrition and promote sustainable agriculture is the second goal of sustainable development. Global environmental challenges demand sustainable solutions to the issue of food security (Wichelns, 2015). Around 60 per cent of the world's ecosystems that help produce food, feed and fibre have been used unsustainably (Montanarella, 2012). The European Commission estimates that by 2050, if we continue using resources at the current rate, a better quality of life will not be achieved because we will need the equivalent of more than two planets to sustain us (European Commission, 2011).
Food crops cover about 10–11% of the world land area, animal feed crops use a further 22% of the world land area (Tzotzos et al., 2009, p. 5), and agricultural land area is approximately five billion hectares, or 38 percent of the global land area (FAO, 2021). High yields and enhanced nutritional value of crops are the cornerstones of food security (Foley et al., 2011). A technologically optimistic vision of the future suggests producing more food from less arable land area (Basiago, 1994), which will require further crop improvements. This will be unfeasible without industrial agriculture that meets the needs of growing urban populations.
Solutions to Issues in Food Security
Crop Genetic Improvement as a Technological Solution
Innovative solutions are needed to tackle the problems associated with climate change. New technologies allow solutions to environmental issues which had seemed impossible before. Science has developed several technological solutions to mitigate the impact of climate change in agriculture, such as carbon sequestration, manure and nutrient management, natural ecosystems restoration. Unlike agricultural biotechnology, these measures are not directly linked to plant breeding and food production. Developments in genome editing have promised to trigger a new revolution in biotechnology. Genome editing provides ample means to overcome limitations inherent in conventional plant breeding (Alexandratos and Bruinsma, 2012; Abdallah et al., 2015; Mahfouz et al., 2016; Nogué et al., 2016; Rani et al., 2016). CRISPR/Cas9 has revolutionised agriculture by a significant advancement in the precision of previously available genetic manipulations like gene knockouts and target genes' activation and repression (Arora and Narula, 2017).
Agricultural biotechnology has been extensively applied in field crop production to produce novel organisms with desired traits. With an accumulated biotech area of 2.7 billion hectares, it has been the fastest adopted crop technology in the history of agriculture (ISAAA, 2019). So-called cash crops—maize, cotton, soybean, and canola—have been grown on industrial scale, most of them are genetically modified. GM crops help meet the challenge of feeding the world. GM crops have been commercialised since 1996. GM crops have been cultivated to increase yield and gain a better profit. According to the International Service for the Acquisition of Agri-biotech Applications (ISAAA), farmers derive benefits from utilising agricultural biotechnology because biotech crops help increase productivity, conserve biodiversity, preclude deforestation, mitigate challenges associated with climate change, and improve economic, health and social benefits (ISAAA, 2019). At the same time, some authors point out that there are no empirical studies of impacts of genetic reproductive modification on biodiversity (Strauss et al., 2017).
Crop genetic improvement technologies can help achieve food security (Narayanan et al., 2019), but biotech crops are not only about benefits. Risks are indispensable to agricultural biotechnology. Consumer health, environmental safety, and biodiversity preservation occupy a central position in risk regulation and biosecurity strategy (Meyerson and Reaser, 2002). Scientific advancements prompt the governments all over the world to follow up with relevant rules and regulations. Laws, definitions, and regulatory approaches to crops derived from biotechnology vary considerably between different countries. Even though risk regulation in agricultural biotechnology is mainly concerned about the risks associated with new and emerging technologies and novel foods, agriculture has never been free from risks. For example, cultivation and hybridisation might pose substantial risks, reducing the fitness or even leading to the extinction of a natural population (Grant et al., 2017). Risks associated with GM include the possibility of allergic reactions, toxicity, and nutritional value (Metcalfe, 2003), but these issues are not specifically distinct from that of conventional or organic food.
The question whether agricultural biotechnology stands a good chance to contribute to biodiversity and sustainable development is a controversial matter. The potential of biotechnology to address the loss of biodiversity is recognised in Article 16(1) of the Convention on Biological Diversity which states that the access to and the transfer of biotechnology are essential elements for the attainment of the objectives of this Convention. Biodiversity is linked to sustainable development. Target 2.5 of the Sustainable Development Goals aims at maintaining genetic diversity of seeds, cultivated plants, farmed and domesticated animals and ensuring access to and fair and equitable sharing of benefits arising from the utilisation of genetic resources and associated traditional knowledge.
The usefulness of biotechnology in promoting sustainable agriculture is equivocal due to ecological, social, and economic controversies surrounding GMOs. International environmental agreements express concerns about risks, e.g., the Convention on Biological Diversity (CBD) envisions the possibility of GMOs to have adverse effects on biodiversity. Article 8(g) of the CBD calls upon to establish or maintain means to regulate, manage or control the risks associated with the use and release of living modified organisms resulting from biotechnology which are likely to have adverse environmental impacts that could affect the conservation and sustainable use of biological diversity, taking also into account the risks to human health. Under Article 14(1)(a) of the CBD, each country has an obligation to introduce appropriate procedures requiring environmental impact assessment of its proposed projects that are likely to have significant adverse effects on biological diversity with a view to avoiding or minimising such effects and, where appropriate, allow for public participation in such procedures. The assumption of potential risk is driven by the hypothesis that GMOs may pose hazards above the risks of conventional crop improvement methods due to the presence of genes from taxonomically distant species (Tzotzos et al., 2009, p. 34).
Broad impacts of biotech crops include “those on the sustainability of ecosystems (for example, reduced or increased use of water or pesticides), economies, markets, research and innovation, and social and cultural systems.” (Kuzma et al., 2009, p. 546). As a social problem, the question of risks associated with GMOs goes far beyond technical issues, bringing about concerns about public acceptance (Lu and Gursoy, 2016) and other factors relevant to risk management and regulation. Such factors are referred to in Article 26(1) of (Cartagena Protocol on Biosafety to the Convention on Biological Diversity, 29 January 2000, 2226 UNTS 208) as socio-economic considerations. While the essence of those factors remains largely unarticulated, the Cartagena Protocol clarifies that socio-economic considerations arise from the impact of living modified organisms on the conservation and sustainable use of biological diversity, especially with regard to the value of biological diversity to indigenous and local communities. Besides international agreements, other legitimate factors relevant to the matter under consideration are referred to in EU regulation of GMOs, e.g., in Articles 6 and 7 of Regulation (EC) No 178/2002 of the European Parliament and of the Council of 28 January 2002 laying down the general principles and requirements of food law, establishing the European Food Safety Authority and laying down procedures in matters of food safety and in Articles 7 and 19 of Regulation (EC) No 1829/2003 of the European Parliament and of the Council of 22 September 2003 on genetically modified food and feed. The emphasis that environmental policy and food regulations put on these factors is great enough to realise that socio-economic considerations can make a substantial difference to which crop genetic improvement technologies are developed and which are not. After more than two decades since genetically modified products have been marketed, the concerns about risk and safety of GMOs get more complicated when new breeding techniques (NBTs) cropped up, and even more complicated when synthetic biology and CRISPR showcased the breathtaking progress of biotechnology.
A general concern about agricultural biotechnology is that the release of GMOs might upset the delicate balance of the biosphere (Hayward, 1998). Again, the possibility of such a harm is not inherent in biotech crops only. Any change in a living organism may have an effect in the future. Genome editing is not unique in respect of its impact on nature. Conventional agricultural practises have largely had a devastating effect on the environment (Nelner and Hood, 2011), although some agricultural practises can reduce the environmental impacts of agriculture (Englund et al., 2020; Chojnacka et al., 2021). The loss of biodiversity has been happening long enough to be sure that genetic engineering cannot be solely responsible for it. The pernicious influence of humans on nature has its origin in the domestication of wild plants in the last 10,000 years, resulting in the narrowing of the genetic base of the plants cultivated for food (Mba et al., 2012). Plant breeding as a process of preserving and propagating desirable traits can contribute to biodiversity loss (Tanksley and McCouch, 1997), although wise use of crop genetic diversity in plant breeding can improve biodiversity (Louwaars, 2018). Biodiversity in agricultural areas is diminished due to habitat fragmentation and alterations to natural vegetation (Rosenblatt et al., 1999; Crooks, 2002). The destruction of tropical forests for timber production and the conversion of woodlands into agricultural landscapes has had potentially dire consequences for tropical biodiversity (Gibson et al., 2011). It follows that agricultural biotechnology is not a sole factor in the loss of biodiversity because agriculture en masse has contributed to the worldwide loss of biodiversity (McLaughlin and Mineau, 1995). Innovation provides technological solutions to issues in biodiversity and food security, although technology is not a panacea to all the problems hindering the development of sustainable agriculture.
Solutions Beyond Technology
The question whether it is possible to achieve food security without technological solutions is a matter of debates (Dibden et al., 2013). For one thing, a better nutrition can be accomplished by a just distribution of resources (Herrera-Estrella and Alvarez-Morales, 2001). The idea of fair distribution is appealing but its feasibility is uncertain because the supply is bound to fluctuate with changes in the availability of resources and the cost of production. Another aspect of distributive justice is the equality of distribution. Food distributed equally among everyone is theoretically possible but in practise it is subject to temporal and spatial limits.
There are efficient ways to optimise the patterns of resource use aimed at waste reduction and healthy diet. For example, eating more vegetables and less meat can improve agricultural sustainability. Although the feasibility of environmental policies relying on the change of food habits and better management practises remains indeterminate due to the growth of human population that increases consumption and affects the need for food, studies demonstrate that incorporating consumers' patterns with producers' perspectives can improve food systems and contribute to the technical mitigation of greenhouse gas emission in the food industry (Garnett, 2013; Revell, 2015).
There are other non-technological solutions based on the marketing strategies which help balance the disruptive effect of new technologies with socio-economic considerations. Sustainability standards and certifications provide “market recognition to goods produced in accordance with social and environmental good practises, typically including practises to protect biodiversity.” (Milder et al., 2015, p. 309). Labelling rules, like Fair Trade, Rainforest Alliance, UTZ Certified, Common Code for the Coffee Community (4C), are examples of sustainability standards (Manning et al., 2012). Organic agriculture is also an example of sustainable crop production (Mansvelt, 1999; Bellon and Penvern, 2014; Nandwani, 2016; Etingoff, 2017) that pursues the general objective of establishing a sustainable management system for agriculture [Council Regulation (EC) No 834/2007 of 28 June 2007 on organic production and labelling of organic products and repealing Regulation (EEC) No 2092/91, Council Regulation (EC) No 834/2007 of 28 June 2007 on organic production and labelling of organic products and repealing Regulation (EEC) No 2092/91]. Labelling, especially eco- and organic labelling, can be considered as a specific policy instrument of sustainable development. In contrast, GMO-labelling does not seem to be perceived as a kind of sustainable production, and it might be justified by the protection of consumer rights. GMO labelling is not framed as a sustainability standard, it is rather a standard of protection of consumer rights which follows from the precautionary principle, though there may be other considerations as well. There is a “demilitarised zone” between organic and GM, called “conventional plant breeding” (Tanaka, 2013), that ensures most of the food needs of the world population and seems to be neutral with respect to the question of sustainability in the sense that it is always possible to convert conventional crops into either organic or GM.
Scientific studies show that organic food has no significant nutritional advantage in comparison to conventional food (Olson, 2017). Organic breeding is based on plants cultivated in conventional breeding, with certain specific characteristics pertinent to the organic way of breeding (Shorrocks, 2017). Compared to conventional farming, organic farming reportedly has lower productivity (Andersen et al., 2015; Kniss et al., 2016; Harbo et al., 2022), although the degree and effects of the yield gap vary between sites (Shah et al., 2017) and depend on the type of crops. Organic produce is able to compete with conventional farming with the support of consumers who believe that organic food is more sustainable than conventional food (Bezawada and Pauwels, 2013), especially given the possibility to narrow the yield gap by improving the fertilisation and diversification of cropping systems (Ponisio et al., 2015; Knapp and van der Heijden, 2018).
The extent to which product labelling could inform the consumer about sustainable production is uncertain because labelling is liable to different interpretations (Turner et al., 2011; Demkin, 2017). Policies to eliminate ambiguous food labelling by designing well-defined and clear labels, enhancing visibility of sustainable products or increasing sustainable choices through food labels can enhance certainty and consumer confidence (Wilson et al., 2017). If applied judiciously, labelling can potentially increase the effect of policies on sustainable agriculture. Additional measures were suggested to compliment labelling in promoting consumer choice in favour of sustainable production, such as enhancing visibility and accessibility of sustainable products, and encouraging sustainable preferences through social norms (Bucher et al., 2016; Wilson et al., 2016; Broers et al., 2017; Bauer and Reisch, 2018; Al-Khudairy et al., 2019; Ferrari et al., 2019; Cialdini and Jacobson, 2021).
While labelling can shift food policy pathways, the effects of eco-labelling vs. GMO-labelling can be completely opposite because the potential of agricultural biotechnology to contribute to food security is mostly not acknowledged by the consumer due to the perception that the use of innovative technologies in food production is per se unsustainable (Cavaliere and Ventura, 2018). Agricultural biotechnology can have environmental and health benefits, but it has been proven experimentally that even sustainably produced GM products failed to change negative consumer attitude due to the power of pre-existing positions (Scholderer and Frewer, 2003). The lack of consumer acceptance of GMOs has policy and regulatory implications that impede the development of crop genetic improvement technologies (Lucht and Hohn, 2015). Public attitude toward GMOs prevents framing agricultural biotechnology as a sustainable crop production and promoting it as a solution to food insecurity, and therefore it is important that new technology is implemented with due consideration to socio-economic strategies available to mitigate the effects of negative public perception.
Reframing Sustainability Agenda Toward Greater Social Inclusion
Genome editing methods and techniques like CRISPR-Cas9 have been praised by scientists as revolutionary tools for efficient crop improvement (Leng et al., 2017; Zhang et al., 2018) that provide a solution to the problem of crop failure. However, genome editing as a technological solution requires appropriate policy strategies aimed at the integration of new technology with societal and consumer expectations. Social licence is a crucial element in the transformation of technological solutions into policy strategies that could ensure responsible innovation in the field of agricultural biotechnology. The spectrum of policy and societal responses to technological innovation are wide. The opposites of this spectrum can be illustrated by the regulatory approaches toward genome editing in the US vs. the EU. US regulatory agencies have generally recognised genome editing techniques as safe and declined to regulate crops, foods and feeds developed with developed with CRISPR/Cas9 and other genome editing techniques, while the EU regulates them the same way as conventional genetic engineering (Dederer and Hamburger, 2019). From the regulatory point of view, such a difference can be justified by risks associated with new technologies. While the argument of risks remains legitimate in policy and societal contexts, there is arguably no evidence that disproves scientific consensus on the safety of biotechnology. Stemming from the debates on potential hazards of GMOs, the policy and social acceptance of genome editing is far from being universal. New technology often falls short of entering manufacturing base due to ineffective integration with societal expectations.
The misbalance between scientific risk assessment and policy risk management suggests a specific pattern of technological development characterised by three distinct stages: research, policy design and social integration. Firstly, a new technology goes through the phase of research. During this stage, risks have been assessed by the experts directly involved in the development of technology. Research does not necessarily coincide with comprehensive risk assessment, and the criteria of risks assessment do not typically include the perception of risk.
Another stage of technological development represents policy design that develops regulatory framework for technology. Here, evidence about the safety of a technology can be contested because the data generated by research is rarely complete and accurate, which makes scientific evidence questioned. Socio-economic and ethical considerations create the need to balance policy priorities with scientific risk assessment. Affected by conflicting values, policy design shifts scientific consensus toward socio-political compromise. Research on new technologies that has secured social licence might not have any significant misbalance with policy design, but the lack of public approval can cause market failure. Research that develops products perceived as unsafe can fail in getting to the market if they do not have a proven added value. A failure in ensuring responsible innovation could arguably occur due to the linear development of socio-technological integration (Figure 1).
In response to pressing food security problems, scientists provide technological solutions to complex issues that have a significant component of social, economic, and ethical considerations. The public may not have the expertise of scientists, and risk management estimates hazards by criteria that include the perception of risk. Decision making is rarely based on scientific risk assessment only, which suggests that the linear model of socio-technical integration is unsuitable for solving complex issues because it does not engage with taking pre-emptive measures to manage risks associated with new technologies and mitigate undesirable societal implications of innovation (Genus and Stirling, 2018). It becomes apparent that genome editing as a solution to food security would be scaled-up more successfully if it was mandated under decision making that integrates social perspective with technical feasibility. New tools and methods of agricultural biotechnology as a solution to food security can ensure socially responsible scientific practise through building capacities which catalyse the endorsement of a new technology by stakeholders and its acceptance by end users. However, a suitable model to implement the solution needs to be explored further. The linear model might be efficient in providing fast solutions, but food security is too complex an issue to be reduced to simple answers. While the components of socio-technical integration, including research and development, policy strategy, and social integration are valid, it seems that a better solution lies in their mutual interrelation through a non-linear pattern (Figure 2).
Following the non-linear model of socio-technical integration, innovation is consistent with socio-economic considerations and ethical values, and new technology is implemented in a socially responsible manner (Fisher et al., 2015). Another distinct feature of this model is that societal expectations are integrated into new technology during, not after, research, which allows a greater degree of flexibility in aligning industrial products with public values (Fisher et al., 2006; Flipse et al., 2013).
Even a theoretically coherent policy model might be inconsistent in its application due to differences in regulatory frameworks between jurisdictions. The focus on social engagement implemented through a policy based on a universal design might nevertheless result in different regulatory frameworks. Sustainability is a concept that, among other global development paradigms, is probably closest to the representation of theoretical universality, but different policy strategies and regulatory frameworks may result in decision making inconsistent with theory. Experience with public opposition against crop genetic improvement suggests that decision making on genome editing and synthetic biology is bound to implement regulatory strategies with stringent controls. The socio-technical integration of innovations in the field of genome editing accommodates scientific progress with ethical viewpoints and cultural practises. The adoption of new technologies is not likely to be universal across the world. Research on genome editing tailors innovation to a range of scaled-up products that obtained social licence and policy mandate. Decision making on the products of such research will be more meaningful when linked to a specific jurisdiction because the status of regulations varies between different countries. Depending on a jurisdiction, a socio-political compromise could be found with regard to the products of innovation, e.g., Bt cotton has not been opposed by the public in Australia as much as Bt maize in Europe, which implies that biotech crops grown for clothing might enjoy greater global social approval than their counterparts grown for food and feed purposes. Policy on innovation in those two areas and jurisdictions adjusts, respectively, and such a flexible adjustment becomes a basis for further policy development. Although genome editing is associated with scientific novelty and technological advancement, policy and law will treat it within existing regulatory frameworks for biotechnology unless there are new regulatory frameworks specifically developed for genome editing. Regulators will face two questions:
1) Are existing regulatory frameworks adequate for genome editing?
2) Do the products of genome editing fall within the scope of existing regulatory frameworks?
To answer these questions, we can posit three basic facts in relation to the regulation on genome editing:
a) GMO laws and regulations will apply to genome editing;
b) Terms and definitions across different areas and jurisdictions are not coherent;
c) Applicable international agreements are not universally ratified.
From these facts it follows that the regulatory frameworks on genome editing will be developed from the same policy principles applied to biotechnology since the beginning of genetic engineering. An important policy question related to modern biotechnology has been whether regulation should be process-based or product-based; and the same question is relevant to genome editing. The product-based approach is governed by the principle of substantial equivalence. This approach considers whether a product of technology is as safe as its conventional counterparts. On the contrary, the process-based approach tends to implement more stringent rules on deliberate release and product authorisation. The process-based approach is de facto adopted in the EU, Australia and New Zealand, while countries like Canada and the US are represented by the product-based approach. US regulatory system relies on a qualitatively different risk management strategy. FDA and USDA have significantly more decision-making power over applications for authorisation, contrary to European Food Safety Authority whose activity does not exceed scientific advice. The US prioritises cost-benefit analysis over the precautionary principle. These approaches to regulation have different advantages and disadvantages. The process-based approach pays special attention to the precautionary principle as a significant political instrument to influence the distribution of costs and benefits of using new technologies; the product-based approach is result-oriented and pays attention to the principle of substantial equivalence. Because the US is the leader in biotechnology, it is legitimate to assume that in the future the global regulatory outcome will reflect the American model. On the other hand, a policy that aims at optimally balanced regulatory framework might prefer European approach to emerging technologies. Besides, it is quite realistic that the interaction of the two approaches will lead to an accommodation between them within certain jurisdictions, like it happens in Australia.
The extent to which genome editing will contribute to food security depends on how national governments place technological innovation in the regulatory frameworks. The striking contrast between the American product-based approach and the European process-based approach to the regulation of genome editing sends a challenge to countries in Africa and Asia struggling with malnutrition and low crop productivity. In Africa, the prevalence of undernourishment is the highest in the world, while 54 percent of the world's hungry people are in Asia (FAO, 2021).
Rural areas in the developing world are especially vulnerable to food insecurity and climate risk. Only a few countries in Africa and Southeast Asia have initiated public discussions and policy proposals initiating the development of national legal frameworks on genome editing. Integrating genome editing into their agricultural systems might be a difficult choice to make because the use biotechnology in agriculture implies the possibility to lose exports to the EU where genome editing is strictly regulated. However, difficult the choice, the current policy trend in the regulation of genome editing demonstrates prevailing movement toward exempting genome editing from the scope of GMO laws (Figure 3).
The question remains as to what extent those regulatory frameworks aim at food security facilitated by genome editing. A serious examination of the role of genome editing in delivering societal missions in addition to industrial objectives is required because the goals of sustainable development shift the focus of new technology from innovation outcome and economic performance to social and environmental impact. The persistence of environmental and social challenges demonstrates the urgency of transforming innovation policy and adjusting policy design on genome editing toward its contribution to sustainable development. Although a better public acceptance of new technologies can be achieved through science communication (Pfotenhauer et al., 2019), policy on innovation aims at a broader application than addressing the deficit of knowledge about technological advances. In addition to framing policy issues under the imperative that mandates more innovation as a solution to existing social and environmental challenges, policy makers face systemic problems that demand further transformation in their approach to innovation.
Lack of improvement in the problems of climate and society gives grounds to argue in favour of a transformative approach to innovation policy that extends the commercial outcomes of science and technology to ensure that technological innovation is socially inclusive and environmentally beneficial. In a nutshell, transformative innovation policy is focused on the environmental and social aspects of innovation in addition to educating, communicating and reducing the knowledge gap. It is a policy built on the premise that the political underpinnings of innovation need systemic transformation based on equity in the distribution of costs and benefits of new technologies. Transformative innovation policy aims at harnessing technological progress to expand social missions and open democratic processes alongside industrial and commercial processes. Transformative change forms one of the three pillars of innovation policy together with research and development and the systems of innovation (Schot and Steinmueller, 2018).
Transformative innovation policy in a genome editing context provides a toolkit to estimate the extent to which new technologies have the potential to solve societal problems and mitigate tensions in public opinion on gene technology. It is important to ensure the socio-technical integration of innovation in a way that secures positive public perception because genome editing as a solution to food security will inevitably be contested due to risks inherent in innovation. Applied to genome editing and its potential for crop genetic improvement, food security as a goal of sustainable development provides a common denominator for innovation policy aiming at an outcome that in addition to producing more food will also address pressing social challenges such as food-related diseases and equity in access to nutrition. Expanding the circle of stakeholders engaged in the process of policy deliberation will improve chances for a greater social inclusion and commitment to open dialogue in policy making. The industrial applicability of genome editing depends on regulatory environment, decision making and public perception. An alignment of goals between science and policy toward addressing societal challenges by means of genome editing can help realise the potential of modern biotechnology to contribute to food security, wealth, and sustainable development.
Conclusion
The quantitative and qualitative demands for food rise with time. Climate change and environmental degradation pose a serious threat to sustainable agriculture. Environments have become increasingly hostile to crops due to dramatic changes in the weather patterns of rainfalls, storms, floods, and droughts which are commonly attributed to the effects of climate change. Since the human population is projected to grow to more than 9 billion people by 2050, it is unlikely that the current agriculture is capable to meet the food needs of future generations without developing crop genetic improvement technologies. On this background, sustainable agriculture depends on the development of high-yielding crops and the improvement of agricultural practises.
Science, technology, and innovation underpin sustainable development. Further advancement of the science of plant breeding is necessary for achieving goals of sustainability. Modern biotechnology has a significant potential to contribute to food security, wealth, and sustainable development. Although it is hard to defy the argument of potential risks associated with crop genetic improvement techniques, it is equally difficult to find a more attractive argument in favour of innovative technologies than their apparent utility to meet the needs of growing population. Crop genetic improvement technologies may not be a perfect solution for meeting nutritional needs of growing world population in the environment growing increasingly hostile under the pressure of climate change, but the techniques of genome editing show great promise. Even though genome editing alone does not seem to be able to make up for the negative impact of harsh weather conditions on crops, it is an effective tool to mitigate that impact. However, it is impossible to address the issue of biodiversity and sustainability by means of science only. The complexity of global issues in food security requires the interface between science and policy to ensure the synergetic effect of socio-technical integration consistent with socio-economic considerations and implemented in a socially responsible manner. Innovation policy that aims at transforming its approach to genome editing toward a greater social inclusion, stakeholder engagement and sustainable development could ensure that genome editing contributes to sustainable agriculture by improving food security in a safe and responsible way for the benefit of society.
Data Availability Statement
The original contributions presented in the study are included in the article/supplementary material, further inquiries can be directed to the corresponding author/s.
Author Contributions
The author confirms being the sole contributor of this work and has approved it for publication.
Funding
This research was conducted by the Australian Research Council Centre of Excellence in Synthetic Biology (project number CE200100029) and funded by the Australian Government.
Conflict of Interest
The author declares that the research was conducted in the absence of any commercial or financial relationships that could be construed as a potential conflict of interest.
Publisher's Note
All claims expressed in this article are solely those of the authors and do not necessarily represent those of their affiliated organizations, or those of the publisher, the editors and the reviewers. Any product that may be evaluated in this article, or claim that may be made by its manufacturer, is not guaranteed or endorsed by the publisher.
References
Abdallah, N., Prakash, C., and Mchughen, A. (2015). Genome editing for crop improvement: challenges and opportunities. Gm Crops Food-Biotechnol. Agric. Food Chain 6, 183–205. doi: 10.1080/21645698.2015.1129937
Alexandratos, N., and Bruinsma, J. (2012). World Agriculture Towards 2030/2050: The 2012 Revision. ESA Working Paper No. 12-03. Rome: FAO.
Al-Khudairy, L., Uthman, O. A., Walmsley, R., Johnson, S., and Oyebode, O. (2019). Choice architecture interventions to improve diet and/or dietary behaviour by healthcare staff in high-income countries: a systematic review. BMJ Open 9, e023687. doi: 10.1136/bmjopen-2018-023687
Amin, A. B., Rathnayake, K. N., Yim, W. C., Garcia, T. M., Wone, B., Cushman, J. C., et al. (2019). Crassulacean acid metabolism abiotic stress-responsive transcription factors: a potential genetic engineering approach for improving crop tolerance to abiotic stress. Front. Plant Sci. 10, 129. doi: 10.3389/fpls.2019.00129
Andersen, M. M., Landes, X., Xiang, W., Anyshchenko, A., Falhof, J., Østerberg, J. T., et al. (2015). Feasibility of new breeding techniques for organic farming. Trends Plant Sci. 20, 426–434. doi: 10.1016/j.tplants.2015.04.011
Anyshchenko, A. (2019). The precautionary principle in EU regulation of GMOs: Socio-economic considerations and ethical implications of biotechnology. J. Agric. Environ. Ethics 32, 855–872. doi: 10.1007/s10806-019-09802-2
Arora, L., and Narula, A. (2017). Gene editing and crop improvement using CRISPR-cas9 system. Front. Plant Sci. 8, 1932. doi: 10.3389/fpls.2017.01932
Bahadur, B., Rajam, M. V., Sahijram, L., and Krishnamurthy, K. V. (2015). Plant Biology and Biotechnology, Volume I, Plant Diversity, Organization, Function and Improvement. New Delhi: Springer. doi: 10.1007/978-81-322-2286-6
Basiago, A. (1994). The limits of technological optimism. Environmentalist 14, 17–22. doi: 10.1007/BF01902656
Bauer, J. M., and Reisch, L. A. (2018). Behavioural insights and (un)healthy dietary choices: a review of current evidence. J. Consum. Policy 42, 3–45. doi: 10.1007/s10603-018-9387-y
Bellon, S., and Penvern, S. (2014). Organic Farming, Prototype for Sustainable Agricultures. Dordrecht: Springer. doi: 10.1007/978-94-007-7927-3
Beumer, K. (2019). How to include socio-economic considerations in decision-making on agricultural biotechnology?: Two models from Kenya and South Africa. Agric. Human Values 36, 669–684. doi: 10.1007/s10460-019-09934-1
Bezawada, R., and Pauwels, K. (2013). What is special about marketing organic products? How organic assortment, price, and promotions drive retailer performance. J. Market. 77, 31–51. doi: 10.1509/jm.10.0229
Broers, V. J. V., De Breucker, C., Van Den Broucke, S., and Luminet, O. (2017). A systematic review and meta-analysis of the effectiveness of nudging to increase fruit and vegetable choice. Eur. J. Public Health 27, 912–920. doi: 10.1093/eurpub/ckx085
Bucher, T., Collins, C., Rollo, M. E., McCaffrey, T. A., De Vlieger, N., Van der Bend, D., et al. (2016). Nudging consumers towards healthier choices: a systematic review of positional influences on food choice. Br. J. Nutr. 115, 2252–2263. doi: 10.1017/S0007114516001653
Cartagena Protocol on Biosafety to the Convention on Biological Diversity 29 January 2000, 2226 UNTS 208 (entered into force 11 September 2003).
Cavaliere, A., and Ventura, V. (2018). Mismatch between food sustainability and consumer acceptance toward innovation technologies among Millennial students: the case of Shelf Life Extension. J. Clean. Prod. 175, 641–650. doi: 10.1016/j.jclepro.2017.12.087
Chojnacka, K., Mikula, K., Izydorczyk, G., Skrzypczak, D., Witek-Krowiak, A., Gersz, A., et al. (2021). Innovative high digestibility protein feed materials reducing environmental impact through improved nitrogen-use efficiency in sustainable agriculture. J. Environ. Manage. 291, 112693–112693. doi: 10.1016/j.jenvman.2021.112693
Cialdini, R. B., and Jacobson, R. P. (2021). Influences of social norms on climate change-related behaviors. Curr. Opin. Behav. Sci. 42, 1–8. doi: 10.1016/j.cobeha.2021.01.005
Convention on Biological Diversity (CBD) 5 June 1992, 1760 UNTS 79, 31 ILM 818 (entered into force 29 December 1993).
Council Regulation (EC) No 834/2007 of 28 June 2007 on organic production and labelling of organic products and repealing Regulation (EEC) No 2092/91 (OJ L 189, 20.7.2007).
Crooks, K. R. (2002). Relative sensitivities of mammalian carnivores to habitat fragmentation. Conserv. Biol. 16, 488–502. doi: 10.1046/j.1523-1739.2002.00386.x
Davies, F., and Bowman, J. (2016). Horticulture, food security, and the challenge of feeding the world. Acta Hortic. 1128, 1–5. doi: 10.17660/ActaHortic.2016.1128.1
Dederer, H., and Hamburger, D. (2019). Regulation of Genome Editing in Plant Biotechnology: A Comparative Analysis of Regulatory Frameworks. Cham: Springer International Publishing AG. doi: 10.1007/978-3-030-17119-3
Demkin, T. (2017). Pediatric food allergies: pitfalls in current food labeling regulations. Pediatr. Nurs. 43, 237–240.
Dibden, J., Gibbs, D., and Cocklin, C. (2013). Framing GM crops as a food security solution. J. Rural Stud. 29, 59–70. doi: 10.1016/j.jrurstud.2011.11.001
Englund, O., Börjesson, P., Berndes, G., Scarlat, N., Dallemand, J.-F., Grizzetti, B., et al. (2020). Beneficial land use change: strategic expansion of new biomass plantations can reduce environmental impacts from EU agriculture. Glob. Environ. Change 60, 101990. doi: 10.1016/j.gloenvcha.2019.101990
Etingoff, K. (2017). Sustainable Development of Organic Agriculture, Historical Perspectives. Oakville, ON, Waretown, NJ: Apple Academic Press. doi: 10.1201/9781315365800
European Commission (2011). Roadmap to a Resource Efficient Europe.. COM (2011) 571. Brussels: European Commission.
FAO IFAD, UNICEF, WFP and WHO. (2021). The State of Food Security and Nutrition in the World 2021. Transforming Food Systems for Food Security, Improved Nutrition and Affordable Healthy Diets for All. Rome: FAO, doi: 10.4060/cb4474en
Ferrari, L., Cavaliere, A., De Marchi, E., and Banterle, A. (2019). Can nudging improve the environmental impact of food supply chain? A systematic review. Trends Food Sci. Technol. 91, 184–192. doi: 10.1016/j.tifs.2019.07.004
Fisher, E., Mahajan, R. L., and Mitcham, C. (2006). Midstream modulation of technology: governance from within. Bull. Sci. Technol. Soc. 26, 485–496. doi: 10.1177/0270467606295402
Fisher, E., O'Rourke, M., Evans, R., Kennedy, E. B., Gorman, M. E., and Seager, T. P. (2015). Mapping the integrative field: taking stock of socio-technical collaborations. J. Responsible Innov. 2, 39–61. doi: 10.1080/23299460.2014.1001671
Flipse, S., Sanden, M., and Osseweijer, M. (2013). Midstream modulation in biotechnology industry: redefining what is ‘Part of the Job' of researchers in industry. Sci. Eng. Ethics 19, 1141–1164. doi: 10.1007/s11948-012-9411-6
Foley, J. A., Ramankutty, N., Brauman, K. A., Cassidy, E. S., Gerber, J. S., Johnston, M., et al. (2011). Solutions for a cultivated planet. Nature 478, 337–342. doi: 10.1038/nature10452
Garnett, T. (2013). Food sustainability: problems, perspectives and solutions. Proc. Nutr. Soc. 72, 29–39. doi: 10.1017/S0029665112002947
Genus, A., and Stirling, A. (2018). Collingridge and the dilemma of control: towards responsible and accountable innovation. Res. Policy 47, 61–69. doi: 10.1016/j.respol.2017.09.012
Gibson, L., Lee, T., Koh, L., Brook, B., Gardner, T., Barlow, J., et al. (2011). Primary forests are irreplaceable for sustaining tropical biodiversity. Nature 478, 378–381. doi: 10.1038/nature10425
Godfray, H., Beddington, J., Crute, I., Haddad, L., Lawrence, D., Muir, J., et al. (2010). Food security: the challenge of feeding 9 billion people. Science 327, 812–818. doi: 10.1126/science.1185383
Grant, W., Jasper, S., Bekkevold, J., and Adkison, D. (2017). Responsible genetic approach to stock restoration, sea ranching and stock enhancement of marine fishes and invertebrates. Rev. Fish Biol. Fish 27, 615–649. doi: 10.1007/s11160-017-9489-7
Harbo, L. S., De Notaris, C., Zhao, J., and Olesen, J. E. (2022). Productivity, light interception and radiation use efficiency of organic and conventional arable cropping systems. Europ. J. Agron. 132, 126407. doi: 10.1016/j.eja.2021.126407
Hayward, S. (1998). Towards a political economy of biotechnology development: a sectoral analysis of Europe. New Politic. Econ. 3, 79–101. doi: 10.1080/13563469808406334
Herrera-Estrella, L., and Alvarez-Morales, A. (2001). Genetically modified crops: hope for developing countries? EMBO Rep. 2, 256–258. doi: 10.1093/embo-reports/kve075
Hossain, M. A., Alam, M., Seneweera, S., Rakshit, S., Henry, R., Acuna, A., et al. (2021). Molecular Breeding in Wheat, Maize and Sorghum. Oxford: CAB International.
ISAAA (2019). Global Status of Commercialized Biotech/GM Crops in 2019: Biotech Crops Drive Socio-Economic Development and Sustainable Environment in the New Frontier. ISAAA Brief No. 55. Ithaca, NY: ISAAA.
Joshi, M., Hawkins, E., Sutton, R., Lowe, J., and Frame, D. (2011). Projections of when temperature change will exceed 2 degree C above pre-industrial levels. Nat. Clim. Chang. 1, 407–412. doi: 10.1038/nclimate1261
Karavolias, N., Horner, W., Abugu, M., and Evanega, S. (2021). Application of gene editing for climate change in agriculture. Front. Sustain. Food Syst. 5, 685801. doi: 10.3389/fsufs.2021.685801
Knapp, S., and van der Heijden, M. G. A. (2018). A global meta-analysis of yield stability in organic and conservation agriculture. Nat. Commun. 9, 3632–3639. doi: 10.1038/s41467-018-05956-1
Kniss, A. R., Savage, S. D., and Jabbour, R. (2016). Commercial crop yields reveal strengths and weaknesses for organic agriculture in the United States. PLoS ONE 11, e0161673. doi: 10.1371/journal.pone.0161673
Kuzma, J., Najmaie, P., and Larson, J. (2009). Evaluating oversight systems for emerging technologies: a case study of genetically engineered organisms. J. Law Med. Ethics 37, 546–586. doi: 10.1111/j.1748-720X.2009.00431.x
Leng, P., Lubberstedt, T., and Xu, M. (2017). Genomics-assisted breeding - a revolutionary strategy for crop improvement. J. Integr. Agric. 16, 2674–2685. doi: 10.1016/S2095-3119(17)61813-6
Louwaars, N. P. (2018). Plant breeding and diversity : a troubled relationship? Euphytica 214, 1–9. doi: 10.1007/s10681-018-2192-5
Lu, L., and Gursoy, D. (2016). Would consumers pay more for nongenetically modified menu items? an examination of factors influencing diners' behavioral intentions. J. Hospital. Market. Manage. 26, 215–237. doi: 10.1080/19368623.2016.1178618
Lucht, J. M., and Hohn, T. (2015). Public acceptance of plant biotechnology and GM crops. Viruses 7, 4254–4281. doi: 10.3390/v7082819
Mackey, M. (2003). The developing world benefits from plant biotechnology. J. Nutr. Educ. Behav. 35, 210–214. doi: 10.1016/S1499-4046(06)60336-9
Mahfouz, M., Cardi, T., and Neal Stewart, C. (2016). Next-generation precision genome engineering and plant biotechnology. Plant Cell Rep. 35, 1397–1399. doi: 10.1007/s00299-016-2009-8
Manning, S., Boons, F., Hagen, O., and Reinecke, J. (2012). National contexts matter: the co-evolution of sustainability standards in global value chains. Ecol. Econ. 83, 197–209. doi: 10.1016/j.ecolecon.2011.08.029
Mansvelt, J. (1999). Checklist for Sustainable Landscape Management, Final Report of the EU Concerted Action AIR3-CT93-1210, The Landscape and Nature Production Capacity of Organic/Sustainable Types of Agriculture (1st ed.). Amsterdam, New York, NY: Elsevier.
Mba, C., Guimaraes, E., and Ghosh, K. (2012). Re-orienting crop improvement for the changing climatic conditions of the 21st century. Agric. Food Secur. 1, 7. doi: 10.1186/2048-7010-1-7
McLaughlin, A., and Mineau, P. (1995). The impact of agricultural practices on biodiversity. Agric. Ecosyst. Environ. 55, 201–212. doi: 10.1016/0167-8809(95)00609-V
Metcalfe, D. (2003). Introduction: What are the issues in addressing the allergenic potential of genetically modified foods? Environ. Health Perspect. 111, 1110–1113. doi: 10.1289/ehp.5810
Meyerson, L., and Reaser, J. (2002). Biosecurity: moving toward a comprehensive approach. Bioscience 52, 593–600. doi: 10.1641/0006-3568(2002)052[0593:BMTACA]2.0.CO;2
Milder, J. C., Arbuthnot, M., Blackman, A., Brooks, S. E., Giovannucci, D., Gross, L., et al. (2015). An agenda for assessing and improving conservation impacts of sustainability standards in tropical agriculture. Conserv. Biol. 29, 309–320. doi: 10.1111/cobi.12411
Montanarella, L. (2012). “Global soils: preserving the capacity for food production,” in Soils and Food Security, eds R. Hester, and R. M. Harrison (Cambridge: Royal Society of Chemistry). doi: 10.1039/9781849735438-00031
Nandwani, D. (2016). Organic Farming for Sustainable Agriculture. Cham: Springer. doi: 10.1007/978-3-319-26803-3
Narayanan, N., Beyene, G., Chauhan, R. D., Gaitan-Solis, E., Gehan, J., Butts, P., et al. (2019). Biofortification of field-grown cassava by engineering expression of an iron transporter and ferritin. Nat. Biotechnol. 37, 144–151. doi: 10.1038/s41587-018-0002-1
Nelner, T. B., and Hood, G. A. (2011). Effect of agriculture and presence of American beaver Castor canadensis on winter biodiversity of mammals. Wildlife Biol. 17, 326–336. doi: 10.2981/09-097
Nogué, F., Mara, K., Collonnier, C., and Casacuberta, J. (2016). Genome engineering and plant breeding: impact on trait discovery and development. Plant Cell Rep. 35, 1475–1486. doi: 10.1007/s00299-016-1993-z
Olson, E. L. (2017). The rationalization and persistence of organic food beliefs in the face of contrary evidence. J. Clean. Prod. 140, 1007–1013. doi: 10.1016/j.jclepro.2016.06.005
Parry, M. (2007). Climate Change 2007, Impacts, Adaptation and Vulnerability, Working Group I contribution to the Fourth Assessment Report of the IPCC. Cambridge: Cambridge University Press.
Pfotenhauer, S. M., Juhl, J., and Aarden, E. (2019). Challenging the “deficit model” of innovation: framing policy issues under the innovation imperative. Res. Policy 48, 895–904. doi: 10.1016/j.respol.2018.10.015
Ponisio, L. C., M'gonigle, L. K., Mace, K. C., Palomino, J., Valpine, P. D., and Kremen, C. (2015). Diversification practices reduce organic to conventional yield gap. Proc. Royal Soc. B Biol. Sci. 282, 20141396. doi: 10.1098/rspb.2014.1396
Popp, J., Pet,o, K., Magda, R., and Lakner, Z. (2013). Economic impact of GM hysteria on EU feed market. Am. J. Plant Sci. 04, 1547–1553. doi: 10.4236/ajps.2013.48186
Pörtner R. Adams H. Adler C. Aldunce P. Ali E. Ara B. (In Press). Climate Change 2022: Impacts, Adaptation Vulnerability. Cambridge University Press.
Powledge, F. (2010). Food, hunger, and insecurity: Of the world's current population of 6.8 billion, 5 billion are living at levels of poverty that deprive them of their basic needs, and more than 1 billion are going hungry. Bioscience 60, 260–265. doi: 10.1525/bio.2010.60.4.3
Rani, R., Yadav, P., Barbadikar, K., Baliyan, N., Malhotra, E., Singh, B., et al. (2016). CRISPR/Cas9: a promising way to exploit genetic variation in plants. Biotechnol. Lett. 38, 1991–2006. doi: 10.1007/s10529-016-2195-z
Ravanbakhsh, M., Kowalchuk, G., and Jousset, A. (2021). Targeted plant hologenome editing for plant trait enhancement. New Phytol. 229, 1067–1077. doi: 10.1111/nph.16867
Regulation (EC) No 178/2002 of the European Parliament and of the Council of 28 January 2002 laying down the general principles and requirements of food law establishing the European Food Safety Authority and laying down procedures in matters of food safety (OJ L 31, 1.2.2002, 1–24).
Regulation (EC) No 1829/2003 of the European Parliament and of the Council of 22 September 2003 on genetically modified food and feed (OJ L 268 18.10.2003, 1–23).
Revell, B. (2015). One Man's Meat. 2050? Ruminations on future meat demand in the context of global warming. J. Agric. Econ. 66, 573–614. doi: 10.1111/1477-9552.12121
Rollin, B. (2012). The perfect storm—genetic engineering, science, and ethics. Sci. Educ. 23, 509–517. doi: 10.1007/s11191-012-9511-3
Rosenblatt, D. L., Heske, E. J., Nelson, S. L., Barber, D. M., Miller, M. A., and MacAllister, B. (1999). Forest fragments in east-central Illinois: island or habitat patches for mammals? Am. Midl. Nat. 114, 115–123. doi: 10.1674/0003-0031(1999)141[0115:FFIECI]2.0.CO;2
Scholderer, J., and Frewer, L. (2003). The biotechnology communication paradox: experimental evidence and the need for a new strategy. J. Consum. Policy 26, 125–157. doi: 10.1023/A:1023695519981
Schot, J., and Steinmueller, E. (2018). Three frames for innovation policy: R&D, systems of innovation and transformative change. Res. Policy 47, 1554–1567. doi: 10.1016/j.respol.2018.08.011
Schurman, R., and Kelso, D. (2003). Engineering Trouble: Biotechnology and its Discontents. Berkeley, CA: University of California Press. doi: 10.1525/california/9780520237612.001.0001
Shah, A., Askegaard, M., Rasmussen, I. A., Jimenez, E. M. C., and Olesen, J. E. (2017). Productivity of organic and conventional arable cropping systems in long-term experiments in Denmark. Eur. J. Agron. 90, 12–22. doi: 10.1016/j.eja.2017.07.001
Shorrocks, V. (2017). Conventional and Organic Farming, A Comprehensive Review Through the Lens of Agricultural Science. Sheffield: 5m Publishing.
Strauss, S. H., Jones, K. N., Lu, H., Petit, J. D., Klocko, A. L., Betts, M. G., et al. (2017). Reproductive modification in forest plantations: impacts on biodiversity and society. New Phytol. 213, 1000–1021. doi: 10.1111/nph.14374
Tanaka, Y. (2013). Attitude gaps between conventional plant breeding crops and genetically modified crops, and psychological models determining the acceptance of the two crops. J. Risk Res. 16, 69–80. doi: 10.1080/13669877.2012.726236
Tanksley, S. D., and McCouch, S. R. (1997). Seed banking and molecular maps: unlocking genetic potential from the wild. Science 277, 1063. doi: 10.1126/science.277.5329.1063
Tester, M., and Langridge, P. (2010). Breeding technologies to increase crop production in a changing world. Science 327, 818–822. doi: 10.1126/science.1183700
Trivedi, P., Schenk, P. M., Wallenstein, M. D., and Singh, B. K. (2017). Tiny microbes, big yields: enhancing food crop production with biological solutions. Microb. Biotechnol. 10, 999–1003. doi: 10.1111/1751-7915.12804
Turner, P. J., Kemp, A. S., and Campbell, D. E. (2011). Advisory food labels: consumers with allergies need more than “traces” of information. BMJ 343, 1671–1832. doi: 10.1136/bmj.d6180
Tzotzos, G., Head, G., raham, P., and Hull, R. (2009). Genetically Modified Plants, Assessing Safety and Managing Risk. Amsterdam: Elsevier.
Ul Haq, S., Khan, A., Ali, M., Khattak, A. M., Gai, W.-X., Zhang, H.-X., et al. (2019). Heat shock proteins: Dynamic biomolecules to counter plant biotic and abiotic stresses. Int. J. Mol. Sci. 20, 5321. doi: 10.3390/ijms20215321
Varshney, R. K., Bansal, K. C., Aggarwal, P. K., Datta, S. K., and Craufurd, P. Q. (2011). Agricultural biotechnology for crop improvement in a variable climate: hope or hype? Trends Plant Sci. 16, 363–371. doi: 10.1016/j.tplants.2011.03.004
Wichelns, D. (2015). Achieving water and food security in 2050: outlook, policies, and investments. Agriculture 5, 188–220. doi: 10.3390/agriculture5020188
Wilson, A. L., Buckley, E., Buckley, J. D., and Bogomolova, S. (2016). Nudging healthier food and beverage choices through salience and priming. evidence from a systematic review. Food Qual. Preference 51, 47–64. doi: 10.1016/j.foodqual.2016.02.009
Wilson, R., Rickard, B., Saputo, R., and Ho, S.-T. (2017). Food waste: the role of date labels, package size, and product category. Food Qual. Prefer. 55, 35–44. doi: 10.1016/j.foodqual.2016.08.004
Zaidi, S. S., Vanderschuren, H., Qaim, M., Mahfouz, M. M., Kohli, A., Mansoor, S., et al. (2019). New plant breeding technologies for food security. Science 363, 1390–1391. doi: 10.1126/science.aav6316
Keywords: food security policy, agricultural biotechnology, genome editing, public perception, genetically modified crops, sustainability, innovation policy
Citation: Anyshchenko A (2022) Aligning Policy Design With Science to Achieve Food Security: The Contribution of Genome Editing to Sustainable Agriculture. Front. Sustain. Food Syst. 6:897643. doi: 10.3389/fsufs.2022.897643
Received: 16 March 2022; Accepted: 29 April 2022;
Published: 26 May 2022.
Edited by:
Rachana Verma, International Centre for Genetic Engineering and Biotechnology, IndiaReviewed by:
Ajay Kumar, Central University of Kerala, IndiaVishwas Ananat Bapat, Shivaji University, India
Copyright © 2022 Anyshchenko. This is an open-access article distributed under the terms of the Creative Commons Attribution License (CC BY). The use, distribution or reproduction in other forums is permitted, provided the original author(s) and the copyright owner(s) are credited and that the original publication in this journal is cited, in accordance with accepted academic practice. No use, distribution or reproduction is permitted which does not comply with these terms.
*Correspondence: Artem Anyshchenko, YS5hbnlzaGNoZW5rb0B1cS5lZHUuYXU=