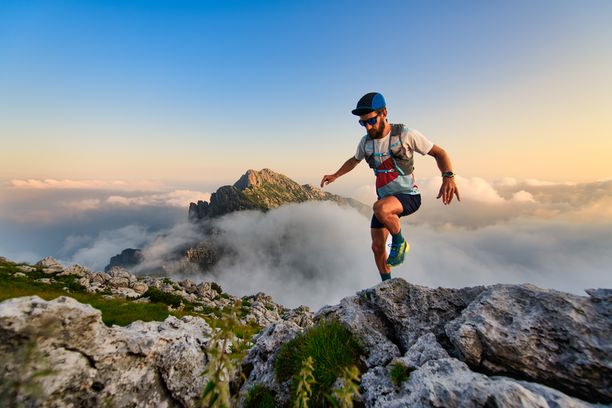
95% of researchers rate our articles as excellent or good
Learn more about the work of our research integrity team to safeguard the quality of each article we publish.
Find out more
ORIGINAL RESEARCH article
Front. Sustain. Food Syst., 10 November 2022
Sec. Sustainable Food Processing
Volume 6 - 2022 | https://doi.org/10.3389/fsufs.2022.891662
This article is part of the Research TopicEmerging Non-Thermal Technology Applications for Sustainable Food ProcessingView all 6 articles
The effect of ultrasound treatments (40 kHz; 40, 50, or 60°C; 5 or 10 min) and thermal treatment (90°C; 30 s) on the stability parameters of orange-carrot juice were evaluated. Microscopic structure, particle size distribution and turbidity were analyzed on the first day. Sedimentation and cloudiness were evaluated over 22 days of storage at 7 and 25°C. Changes in microstructure and disruption of the cell wall were evidenced after treatment at 60°C/10 min. The particle size distribution was heterogeneous with an increase of small particles after ultrasonication. Ultrasonicated and thermal treated samples did not show any differences in turbidity. Cloudiness increased after sonication and decreased over the storage in all samples. Sedimentation process at 7°C was homogeneous among samples while it was delayed in samples treated with ultrasounds at 60°C for 10 min. Ultrasound processing improved the quality of juices and can be proposed as a potential novel processing technique for blended vegetable-fruit juices.
The change in food consumption caused a greater demand for nutritious and natural foods, including fruit juices. They are sources of vitamins, carotenoids, flavonoids, and fibers, that contribute to the maintenance of health (Rajauria and Tiwari, 2018). Several factors influence the purchase of fruit and vegetable juices. Visual characteristics such as appearance and color as well as taste, texture, color, and even shape and temperature can influence consumers' choices (Priyadarshini and Priyadarshini, 2018). Nowadays, innovative, and more attractive drinks are available in the market and the combination of fruits and vegetables, which are rich in antioxidants, contributes to improving the color of the drink (Priyadarshini and Priyadarshini, 2018). Furthermore, juices blending allows increasing the sensory quality, reduce the pH value, and consequently improve the stability of the product (Nadeem et al., 2018).
Besides the nutritional quality, the product must be safe and have an extended shelf life. Thermal treatments are commonly applied for the microbiological control of juice during processing and storage. However, heat can detrimentally affect the nutritional and sensory quality of juices, significantly reducing their vitamins and phenolic compounds content, and causing color changes (Ordóñez-Santos et al., 2017). Consequently, new food processing technologies, like high pressure, pulsed electric field, ultraviolet irradiation, and ultrasound, have been studied as an alternative to thermal treatments. Ultrasound (US) technology has been successfully investigated as an alternative to thermal pasteurization. Reduced microbial load, minimal nutritional losses, and improved sensorial properties in US treated foods were detected (Ertugay and Başlar, 2014; Rojas et al., 2016; Campoli et al., 2018).
Ultrasonication, which consists of treating the foods with high-intensity ultrasound waves (>1 Wcm−2, 20–100 kHz), and has been shown as a promising technology to increase the shelf life of juices due to the inactivation of enzymes and microorganisms (Kentish, 2017; São José et al., 2018; Alvarenga et al., 2020). The principal mechanism of action is cavitation, consisting of the formation, growth, and collapse of bubbles (Martínez-Flores et al., 2015; Rojas et al., 2016; Pokhrel et al., 2017; Campoli et al., 2018; Menelli et al., 2021). The modifications associated to the pressure exerted during bubble collapse also promotes microscopic changes such as particle surface erosion and size reduction and increase of suspended particles in the medium (Aadil et al., 2013; Ertugay and Başlar, 2014; Rojas et al., 2016; Campoli et al., 2018).
From an industrial point of view, microscopic cellular changes induced by ultrasound can reduce the need for the addition of stabilizers to the juice (Rojas et al., 2016). These modifications enhance the stability, improve the color, increase the turbidity and apparent viscosity, delay pulp sedimentation giving rise to a better sensory quality of juices. Ultrasound could or could not affect juice consistency depending on the treatment time. However, increasing the treatment times could detrimentally affect the juice composition. In fact, with increasing cell disruption and intracellular content leakage, bioactive plant compounds, such as carotenoids, are released and become more prone to oxidation reactions by free radicals formed during cavitation process (Rojas et al., 2016; Campoli et al., 2018). Thus, there is still need of clarifying the effects of ultrasound technology on fruit juices stabilization and individuate the operating conditions suitable to obtain favorable physical and microscopic properties in the juices (Rojas et al., 2016; Wang et al., 2020). In fact, as previously described by the authors, ultrasound can induce desirable or undesirable structural changes in fruit juices (Campoli et al., 2018).
When the literature is examined, there are a few studies that evaluated rheological properties, and stability parameters of blended juices treated with thermosonication. Thus, this study aimed to evaluate the impact of different temperatures and times of processing with ultrasound on the physical, and rheological stability of orange-carrot juice, and compare it to that of the thermally treated one.
The experiment was conducted in a completely randomized design and was conducted in three replicates. Oranges (Citrus sinensis L. Osbeck) cultivar “Pêra” and carrots (Daucus carota) were selected and obtained from a local market of Vitória (Espírito Santo State, Brazil). Foods with the whole appearance were selected, discarding those that were damaged, malformed or rotted. Subsequently, surface dirt was washed away with tap water, and the vegetables were sanitized with sodium dichloroisocyanurate solution at 100 mg/L for 10 min (Hidrosteril®, Itapevi, São Paulo, Brazil). The oranges were halved, and the juice was extracted with a domestic electric citrus juicer (Mondial® Professional E-10 Bivolt-250 W; Conceição do Jacuípe, Bahia, Brazil). The carrots were peeled and sliced with a previously sanitized stainless-steel knife. The orange-carrot juice was prepared in a blender without the addition of water or other ingredients. According to the results of previous tests (data not shown), the juice was prepared using 700 ml of orange juice and 300 g of carrot, followed by sieving to remove larger particles. After preparation of the juice, samples of 400 ml were bottled in previously sterilized glass bottles and stored in refrigerated condition at 7°C until further use.
Treatment temperature and time applied were selected based on previous experiments (data not shown) and scientific literature. The untreated juice was considered as the control sample. The samples were subjected to the thermal treatment in a bath (90°C for 30 s) according to Lee and Coates (2003). Treatments with ultrasonic bath (Branson®, Model CPX3800H, 110 W−40 kHz, Danbury, United States) were performed at 40, 50, and 60°C for 5 and 10 min. The sonicated samples were placed in the center of the equipment, at a distance of 15.0 and 7.5 cm from the sides. After the treatments, all samples were stored away from the light and maintained at 7 ± 1°C until the moment of analysis.
To understand and discriminate the effects on blended juice of ultrasound processing at different conditions, the following measurements were performed shortly after treatment: optical microscopy, particle size distribution, and turbidity. To analyze the stability of the juice during storage at 7 ± 1°C, cloudiness and sedimentation measurements were performed over 22 days.
The particle size distribution of the samples was measured by light scattering (Microtrac Zetatrac Nanotechnology Particle Size and Charge Measurement Analyzer, Microtrac Ltda., USA). Samples (50 ml) were diluted in 100 ml of distilled water, and the mean diameter and mean particle area were measured. All analysis was conducted according to Kubo et al. (2013).
The microscopic characteristics of the juice were evaluated according to Rojas et al. (2016). Juice samples (~100 μl) were dispersed on a glass slide and observed with a forty-fold increase in an optical microscope (Carl Zeiss® Microscopy, model 37081, Göttingen, Germany) equipped with a digital camera (Carl Zeiss® 135 AxioCam Erc 5s, Germany). The images were captured at least five times for each sample and analyzed qualitatively.
The turbidity of the juices was evaluated just after the preparation and treatment of the samples, according to Bhat and Goh (2017). The absorbance of ~4 ml of each sample was read on a UV-Visible SP-2100UV spectrophotometer (Visor Spectrum®, Piracicaba, São Paulo, Brazil), at 610 nm. The turbidity of samples was calculated according to equation 1, using that of distilled water as reference:
where: Abs is the absorbance of the sample.
Juice cloudiness was assessed according to Kubo et al. (2013). This analysis aimed to verify the behavior of juice components, as well as the turbidity of the samples during storage. For that, 10 ml of samples were centrifuged (Kasvi® K14-0815ª, São José dos Pinhais, Paraná, Brazil) for 10 min at 20°C and 3,500 rpm. The absorbance of ~4 ml of the supernatant was measured at 660 nm using a spectrophotometer (Novainstruments® Serie 2000−325 A 1,000 nm, Piracicaba, São Paulo, Brazil) and directly related to cloudiness.
The sedimentation of the juice pulp was evaluated according to Leite et al. (2015), with adaptations. After the treatments, samples were transferred in graduated cylinders and stored at 7 and 25 ± 1°C for 22 days. The objective was to evaluate the stability of juice during storage and how the storage temperature influences visually and qualitatively the sedimentation. The sedimentation index (SI) was calculated according to Equation 2 (Silva et al., 2010). The analysis was performed on the first 6 days and later on days 8, 18, and 22 of storage.
Turbidity data were subjected to analysis of variance (ANOVA), and means were analyzed by Tukey's test at 0.05 of significance. Cloudiness data were subjected to analysis of variance (ANOVA) to evaluate the influence of treatment and storage time. Subsequently, the qualitative data with a significant difference (p-value ≤ 0.05) were analyzed by Tukey's test, and the quantitative data with significant difference (p-value ≤ 0.05) provided by regression analysis. Linear and quadratic equation models were tested in the regression analysis as a function of storage time. The data were analyzed with the free version of the application software SAS® OnDemand for Academics.
The change in the particle size distribution (PSD) and the average particle diameter of mixed orange-carrot juice samples treated under different conditions were presented in Figures 1, 2. The PSD is mostly asymmetric concerning the average size of the particles, both for those with smaller diameters and those with larger dimensions. The control sample (untreated juice) showed a more symmetrical PSD, with size ranging from 486 to 6,540 nm. In this sample, only one peak was observed, representing 21% of the particles with an average size of 1,635 nm. The cumulative frequency for this treatment shows that ~20% of the particles are 1,000 nm or less in size. The PSD of the heat-treated juice was bimodal, that is, two peaks were observed at 687 nm (9.93%) and 2,750 nm (15.72%). The particle size was in the range between 409 and 4,620 nm, thus reduced with respect to that of the untreated sample.
Figure 1. Particle size distribution curves of orange-carrot juice submitted to different processing conditions. US, ultrasound. Heat treatment: 90°C/30 s.
Figure 2. Histograms of accumulated (black line) and frequency (gray column) of the particle size distribution of orange-carrot juice submitted to different processing conditions. US: ultrasound. Heat treatment: 90°C/30 s.
Regarding the PSD of the thermosonicated samples, no homogeneous effect was observed in the size of particles after ultrasound application compared to the control, but all the ultrasound-treated samples exhibited an increase in the number of smaller particles. The treatment at 40°C for 5 min in ultrasound reduced the variation in particle size, presenting particles between 144.5 and 3,890 nm. In this treatment condition, the highest size frequency observed was at 1,944 nm (14.28%) together with a small peak at 243 nm (1.97%). The increase in the treatment time (10 min) at this temperature promoted an increase of the values of the minimum (409 nm) and maximum (6,540 nm) particle size. A distinct PSD in a bimodal form, similar to the heat-treated sample, was observed after treatment at 40°C for 10 min, with peaks at 818 nm (5.68%) and 4,620 nm (29.28%). Similarly, when the ultrasound treatment was carried out at 50°C with increasing the processing time from 5 to 10 min a shift of the PSD was observed, the longer the time the higher the frequency of larger particles. With a further increase of the ultrasound treatment temperature at 60°C and by applying a processing time of 5 min, two peaks can be observed, the first at 578 (5.68%) nm and the second at 1,944 (19.9%) nm. When the ultrasound processing time at 60°C was prolonged up to 10 min, the size of more than 80% of the particles was equal or lower than 1,000 nm (Figure 1).
Analyzing all treatments in a superimposed mode (Figure 2), it is possible to observe that as the US treatment temperature increased from 40 to 50°C, regardless the treatment time, there was a shift toward the center of the curve, indicating an increase in the number of smaller particles. On the contrary, when ultrasound treatment temperatures of 50 and 60°C for 5 min were utilized, a smaller PSD variation was observed, with the minimum and maximum particles size ranging from 121.5 to 5,500 nm and from 243 to 3,270 nm, respectively. However, when the treatment time was increased up to 10 min an increasing number of larger particles is detected in the samples treated at 60°C.
The PSD consists of relating the frequency of solid particles in different size ranges, which can be presented in mass or volume depending on the different diameters observed in a given sample. Some studies obtained distinct observations after evaluating the effect of ultrasound on particle size under different treatment conditions (Bi et al., 2015; Rojas et al., 2016; Huang et al., 2018; Chen et al., 2020; Wang et al., 2020) and the results are mainly related to type of food, different conditions applied, particularly processing time.
The collapse of the bubbles during ultrasound processing give rise to mechanical forces leading to particle size reductions due to the damages occurring on particles membrane (Amiri et al., 2018). However, an aggregation of small particles into larger ones can occur when higher ultrasound treatment powers is applied with higher temperatures, as observed by Shen et al. (2021). The sample subjected to ultrasound treatment may be more or less resistant to the process. The potential deviation in this mechanical resistance between the whole cells and its particles after ultrasound treatments can be explained by some mechanisms like the region exposed, the diverse compositions, the internal cell pressure, mechanical resistance of the intercellular liquid, and susceptibility to cavitation (Manzoor et al., 2021).
In the present study a reduced average PSD was detected in the blended juices with increasing the treatment time at a given temperature, suggesting that the increase of the treatment time has promoted the agglomeration of the smaller particles formed during sonication and, consequently, to the increase of particle size due to agglomeration phenomena. A similar behavior was observed on samples treated with ultrasound for 10 min at different temperatures. After ultrasound processing particles of different sizes formed, that might affect to different extent the turbidity and sedimentation process in blended juices.
The results of the PSD are consistent with the microstructure evaluation in which the disruption of cells by ultrasound treatment was well supported by optical microscopic photos (Figure 3), indicating that ultrasound promoted a reduction in particle size due to the high shear effect during cavitation process.
Figure 3. Effect of different treatments on orange-carrot juice microstructure: optical microscopy images using forty-fold increase. Black arrows indicate the orange structure in the middle of plant cells. The scale bar is 50 μm. US: ultrasound. Heat treatment: 90°C/30 s.
In the present study, microscopic characteristics were analyzed qualitatively from optical microscopy images. Juices are composed of pulp and serum, the latter consisting of water and intracellular soluble components such as sugars, acids, and minerals. The pulp contains fragments of plant tissues from preparation processes and cells. Preservation treatments can lead to the disintegration of cells which disperse in the serum (Campoli et al., 2018).
In the untreated and heat-treated samples, the cellular structures of the raw materials used in product processing can be observed denser, with integral cells and intact walls (Figure 3). In general, when ultrasound was applied it can be observed a gradual degradation of the cell, and as the temperature and time of the ultrasound treatments increased, the sample showed less dense particles and aggregates. The cells were more dispersed in the serum, possible due to an increase in the intracellular spaces after the cavitation process. Minimal structural changes were observed after ultrasound treatment at 40°C for 5 min, as well as pasteurization when compared to the untreated sample. Ultrasound treatments at 40°C for 10 min and 50°C for 5 and 10 min caused structural changes in the samples.
It is noteworthy that the samples sonicated at 60°C for 5 and 10 min showed more visible structural differences when compared to those subjected to the other treatments. In the most intense ultrasound condition (60°C/10 min), a different microscopic structure is observed; the particles were less compacted, with possible rupture of the vegetal cell membrane and release intracellular compounds from the pulp to serum. Furthermore, an orange-colored structure was clearly seen in the sample treated with ultrasound at 60°C for 10 min, differently from the other treatment conditions, which is more difficult to be explained (Figure 3).
According to Figure 3, it was confirmed that the cavitation process, generated by ultrasound, induced irreversible changes in the microstructure as well as the disruption of the vegetable tissues and an increase of porosity and intracellular spaces, as described previously (Rajewska and Mierzwa, 2017). This paper confirmed that structural changes caused by ultrasounds process, could promote a higher release of bioactive compounds, such as carotenoids, vitamins, and minerals, from plant cells. However, these compounds become more accessible to free radical oxidation reactions occurring during the cavitation process, which could hinder the quality of the treated juices (Rojas et al., 2016; Rajewska and Mierzwa, 2017; Campoli et al., 2018; Wang et al., 2020). Furthermore, ultrasound caused the formation of an increasing number of particles of small size, which resulted in a new structure that can improve the physical characteristics of the juice. Ultrasounds treatments contributed to obtain higher homogeneity and turbidity of samples and can delay the sedimentation of blended fruit and vegetable juices. Time and temperature could be factor associated to the modification of microscopic structures on juices samples (Ertugay and Başlar, 2014; Menelli et al., 2021). Longer ultrasound treatment times could determine higher damage and rupture observed in the cell structure (Rojas et al., 2016; Campoli et al., 2018; Huang et al., 2018; Wang et al., 2019, 2020; Zhang et al., 2019).
Particle disruption by ultrasound technology is a complex phenomenon, which is affected different factors including the type of food undergoing the treatment and the processing conditions. The results reported in this work and the lack of data on mixed vegetable and fruit juices analysis in the current literature do not allow to draw conclusions on the utilization of ultrasounds for the stabilization of this kind of products. More work is needed with a special focus on the determination of physical characteristics of single juices after thermosonication.
The results of the sedimentation tests at 25°C are reported in Figure 4. The untreated sample showed an accelerated sedimentation with respect to the heat and ultrasound treated samples, being 50% of sedimentation reached in the first 24 h of storage. It was possible to observe a clear phase separation between the serum and the sediment (syneresis) in the first 48 h of storage (data not shown). The untreated juice presented also a more compact sediment at the bottom of the beaker.
Figure 4. Sedimentation index (% SI) of orange-carrot juice pulp submitted to different conservation treatments during 22 days of storage at 25°C. US: ultrasound. Heat treatment: 90°C/30 s.
Samples treated with ultrasounds showed a delayed sedimentation, the longer the processing time the slower the sedimentation process at 25°C observed in orange-carrot juice. In the most intense ultrasound treatment conditions (60°C/10 min), the phase separation occurred after 8 days of storage, and it was possible to identify smaller and more dispersed particles in the sediment. It is well known that ultrasound can cause damage to particles due to pressure gradient during cavitation, resulting in reduction of particle size, which consequently could delay the sedimentation over time (Ojha et al., 2018). According to Stokes' Law, the sedimentation rate and the shape of the sedimentation curves are related to particle diameter and density. Larger particles settle faster, while for smaller particles the sedimentation process is slower (Kubo et al., 2013). The sedimentation rate of samples treated with ultrasound varies according to the type of raw materials, being the plant cell wall more weak or resistant to structure damages during treatments (Kubo et al., 2013; Campoli et al., 2018).
The sedimentation of juices stored at 7°C was proved to be homogeneous in all treatments over the 22-day follow-up. For this reason, only the graph for storage at 25°C has been reported. It can be concluded that a reduction of the storage temperature allows the particles to remaining suspended for a longer time. The results of the present study are consistent with others previously reported since the lowest temperature of storage maintained the stability of juice samples over the days. Furthermore, this finding is a relevant point in juice processing since ultrasound treatment provided greater uniformity and stability of the orange-carrot juice. Our results are consistent with those of Fonteles et al. (2012) and Ertugay and Başlar (2014). These authors observed that samples treated with higher temperatures showed a lower sedimentation of the juice particles. Different behaviors in juice sedimentation are reported in other studies on the application of ultrasound technology, as they are related to the samples used. One reason for the faster sedimentation at 25°C observed in the present study is due to the different composition of the juice sample which is a blend of two food matrices (orange and carrot). Thus, it is suggested that carrot juice incorporation has accelerated the sedimentation process of the samples. Nonetheless, the results obtained indicated that ultrasound can be applied to reduce the sedimentation rate of juices, thus providing a better stability to the processed product. The dispersion of the particles is also related to their diameter. Changes of the particle size distribution may occur during ultrasound application, as already discussed, and can justify the findings on juice sedimentation.
Table 1 demonstrates the results for the turbidity and cloudiness of juice after different conditions of treatment on the first day. There was no difference in turbidity between the untreated sample and those treated with heat or ultrasound and the average value of this parameter was 99.99 ± 0.010 (p-value > 0.05). However, cloudiness was significantly affected by the different treatments applied and the storage time (p-value ≤ 0.05). During storage, no significant difference on cloudiness values of heat and ultrasound-treated samples was observed (p-value > 0.05).
Table 1. Turbidity and cloudiness assessment (mean ± standard deviation)* of untreated orange-carrot juice and samples submitted to different conservation treatments on the first day.
As far as the results of turbidity measurements are concerned, it is noteworthy to underline that this parameter is measured on samples which did not undergo centrifugation. Therefore, since the precipitation of the particles did not occur, the absorbance values of the treated and untreated sample did not differ. Bhat and Goh (2017) treated strawberry juice and analyzed turbidity using the same methodology. The authors also did not observe significant differences in turbidity when comparing treated samples and the control group.
As far as cloudiness mean values are concerned, this parameter in ultrasound treated juices increased by an average of 24% (p-value ≤ 0.05). Untreated juice exhibited the lowest mean (1.375 ± 0.279). The heat-treated sample showed an average value of cloudiness (1.492 ± 0.175) statistically equal to untreated juice. Juices treated with ultrasound showed cloudiness values themselves (mean = 1.637 ± 0.277) statistically similar, whatever were the processing conditions used, and higher than those of untreated and heat-treated (p ≤ 0.05). Although heat can also cause particle breakage in smaller structures, the increase in turbidity in the pasteurized sample was lower than in ultrasound treatments. Concerning the storage time, there was a reduction of this parameter over the 22 days (cloudiness = 1.720–0.007858445 * time of storage; R2 = 0.7256; p-value = 0.0028). The mean decreased from 1.748 ± 0.110 to 1.539 ± 1.77 between the first and last day of storage, respectively.
Turbidity, or cloudiness, is a desirable quality parameter in fruit and vegetable juices. It is related to the presence of cellulose, hemicellulose, and pectin particles, as well as organic acids and sugar, which contribute to improving the color and aroma of the product. Also, it is a feature that is directly linked to product homogeneity and consistency. The present results of post-effect and storage coincide with those previously reported in other publications for fruits and vegetable juices such as grapefruit, apple, peach, strawberry, guava, carrot, and kiwifruit (Aadil et al., 2013; Ertugay and Başlar, 2014; Rojas et al., 2016; Bhat and Goh, 2017; Campoli et al., 2018; Chen et al., 2019; Wang et al., 2020).
The increase of this parameter in ultrasound post-processing effect is attributed to cavitation, which is the formation, growth, and collapse of bubbles. Also, the combination of mechanical and chemical effects of ultrasound favored the results obtained in the present and other studies. The pressure exerted during collapse promotes particle breakage in smaller structures, thereby increasing the number of particles suspended in the sample (Aadil et al., 2013; Campoli et al., 2018). Smaller particles tend to remain in suspension after centrifugation (which is always performed with the same acceleration), increasing the values of absorbance and turbidity. Also, enzyme inactivation and reduction of particle size influence cloud stability. The high-pressure gradient results in structural modifications on pectin molecules and can enhance the cloud stability of juices (Tiwari et al., 2009).
The reduction observed over the storage time can be attributed to electrostatic or Van der Waals forces that induce the formation of small particle aggregates, which precipitate more easily after centrifugation (Kubo et al., 2013).
All the results in this study indicated that ultrasound treatment can considerably affect and improve stability parameters of orange-carrot juice after processing and during storage with respect to heat treatment. High-intensity ultrasound processing, especially the treatment at 60°C for 10 min, significantly increased the disruption of cell structures of juice and retarded pulp sedimentation. Despite this, the particle size distribution showed an increase in smaller particles and a reduction in larger ones in all ultrasound treatments. These changes resulted in the improvement of cloudiness. Therefore, ultrasound processing can be considered as a potential novel processing technique for improving the quality of juices, but further studies must be conducted to better understand the ultrasound effect on physical, rheological, and chemical parameters of mixed juices.
The data that support the findings of this study will be available upon request to the corresponding author.
BL, ASa, ASp, PD, and JS: conceptualization, writing—original draft, and investigation. BL and JS: data curation, formal analysis, and writing—review and editing. BL, ASa, ASp, and PD: methodology. JS: project administration. All authors contributed to the article and approved the submitted version.
This work was supported by scholarship of Higher Education Improvement Coordination (Coordenação de Aperfeiçoamento de Pessoal de Nível Superior) granted to BL and financial support of Foundation for the Support of Research and Innovation of Espírito Santo (Fundação de Amparo à Pesquisa e Inovação do Espírito Santo-FAPES) for project number 554/2015 that allowed the acquisition of the ultrasound equipment used in this research.
The authors thank the Multi-User Laboratory of Histotechniques of the Federal University of Espírito Santo for allowing the performance of microscopy analyses and to the Research Laboratory of Physico-Chemistry and Analytical Chemistry of the Federal Institute of Espírito Santo, Campus Aracruz, for allowing the analysis of particle size distribution.
The authors declare that the research was conducted in the absence of any commercial or financial relationships that could be construed as a potential conflict of interest.
All claims expressed in this article are solely those of the authors and do not necessarily represent those of their affiliated organizations, or those of the publisher, the editors and the reviewers. Any product that may be evaluated in this article, or claim that may be made by its manufacturer, is not guaranteed or endorsed by the publisher.
Aadil, R. M., Zeng, X. A., Han, Z., and Sun, D. W. (2013). Effects of ultrasound treatments on quality of grapefruit juice. Food Chem. 141, 3201–3206. doi: 10.1016/j.foodchem.2013.06.008
Alvarenga, P. D. L., Vasconcelos, C. M., and São José, J. F. B. (2020). Application of ultrasound combined with acetic acid and peracetic acid: microbiological and physicochemical quality of strawberries. Molecules 26, 1–16. doi: 10.3390/molecules26010016
Amiri, A., Mousakhani-Ganjeh, A., Torbati, S., Ghaffarinejhad, G., and Esmaeilzadeh Kenari, R. (2018). Impact of high-intensity ultrasound duration and intensity on the structural properties of whipped cream. Int. Dairy J. 78, 152–158. doi: 10.1016/j.idairyj.2017.12.002
Bhat, R., and Goh, K. M. (2017). Sonication treatment convalesce the overall quality of hand-pressed strawberry juice. Food Chem. 215, 470–476. doi: 10.1016/j.foodchem.2016.07.160
Bi, X., Hemar, Y., Balaban, M. O., and Liao, X. (2015). The effect of ultrasound on particle size, color, viscosity and polyphenol oxidase activity of diluted avocado puree. Ultrason. Sonochem. 27, 567–575. doi: 10.1016/j.ultsonch.2015.04.011
Campoli, S. S., Rojas, M. L., do Amaral, J. E. P. G., Canniatti-Brazaca, S. G., and Augusto, P. E. D. (2018). Ultrasound processing of guava juice: Effect on structure, physical properties and lycopene in vitro accessibility. Food Chem. 268, 594–601. doi: 10.1016/j.foodchem.2018.06.127
Chen, L., Bi, X., Guo, D., Xing, Y., and Che, Z. (2019). The effect of high-power ultrasound on the quality of carrot juice. Food Sci. Technol. Int. 25, 394–403. doi: 10.1177/1082013219825736
Chen, L., Chen, L., Zhu, K., Bi, X., Xing, Y., and Che, Z. (2020). The effect of high-power ultrasound on the rheological properties of strawberry pulp. Ultrason. Sonochem. 67, 1–10. doi: 10.1016/j.ultsonch.2020.105144
Ertugay, M. F., and Başlar, M. (2014). The effect of ultrasonic treatments on cloudy quality-related quality parameters in apple juice. Innov. Food Sci. Emerg. Technol. 26, 226–231. doi: 10.1016/j.ifset.2014.06.013
Fonteles, T. V., Costa, M. G. M., de Jesus, A. L. T., de Miranda, M. R. A., Fernandes, F. A. N., and Rodrigues, S. (2012). Power ultrasound processing of cantaloupe melon juice: effects on quality parameters. Food Res. Int. 48, 41–48. doi: 10.1016/j.foodres.2012.02.013
Huang, B., Zhao, K., Zhang, Z., Liu, F., Hu, H., and Pan, S. (2018). Changes on the rheological properties of pectin-enriched mango nectar by high intensity ultrasound. LWT - Food Sci. Technol. 91, 414–422. doi: 10.1016/j.lwt.2018.01.062
Kentish, S. E. (2017). “Engineering principles of ultrasound technology,” in Ultrasound: Advances in Food Processing and Preservation (London, UK: Elsevier Inc.), 1–13. doi: 10.1016/B978-0-12-804581-7.00001-4
Kubo, M. T. K., Augusto, P. E. D., and Cristianini, M. (2013). Effect of high pressure homogenization (HPH) on the physical stability of tomato juice. Food Res. Int. 51, 170–179. doi: 10.1016/j.foodres.2012.12.004
Lee, H. S., and Coates, G. A. (2003). Effect of thermal pasteurization on Valencia orange juice color and pigments. LWT - Food Sci. Technol. 36, 153–156. doi: 10.1016/S0023-6438(02)00087-7
Leite, T. S., Augusto, P. E. D., and Cristianini, M. (2015). Using high pressure homogenization (HPH) to change the physical properties of cashew apple juice. Food Biophys. 10, 169–180. doi: 10.1007/s11483-014-9385-9
Manzoor, M. F., Siddique, R., Hussain, A., Ahmad, N., Rehman, A., Siddeeg, A., et al. (2021). Thermosonication effect on bioactive compounds, enzymes activity, particle size, microbial load, and sensory properties of almond (Prunus dulcis) milk. Ultrason. Sonochem. 78, 1–9. doi: 10.1016/j.ultsonch.2021.105705
Martínez-Flores, H. E., Garnica-Romo, M. G., Bermúdez-Aguirre, D., Pokhrel, P. R., and Barbosa-Cánovas, G. V. (2015). Physico-chemical parameters, bioactive compounds and microbial quality of thermo-sonicated carrot juice during storage. Food Chem. 172, 650–656. doi: 10.1016/j.foodchem.2014.09.072
Menelli, G. S., Fracalossi, K. L., Lepaus, B. M., and de São José, J. F. B. (2021). Effects of high-intensity ultrasonic bath on the quality of strawberry juice. CYTA – J. Food 19, 501–510. doi: 10.1080/19476337.2021.1918768
Nadeem, M., Ubaid, N., Qureshi, T. M., Munir, M., and Mehmood, A. (2018). Effect of ultrasound and chemical treatment on total phenol, flavonoids and antioxidant properties on carrot-grape juice blend during storage. Ultrason. Sonochem. 45, 1–6. doi: 10.1016/j.ultsonch.2018.02.034
Ojha, K. S., Tiwari, B. K., and O'Donnell, C. P. (2018). “Effect of ultrasound technology on food and nutritional quality,” in Advances in Food and Nutrition Research (Vol. 84) (London, UK: Academic Press Inc.), 207–240. doi: 10.1016/bs.afnr.2018.01.001
Ordóñez-Santos, L. E., Martínez-Girón, J., and Arias-Jaramillo, M. E. (2017). Effect of ultrasound treatment on visual color, vitamin C, total phenols, and carotenoids content in Cape gooseberry juice. Food Chem. 233, 96–100. doi: 10.1016/j.foodchem.2017.04.114
Pokhrel, P. R., Bermúdez-Aguirre, D., Martínez-Flores, H. E., Garnica-Romo, M. G., Sablani, S., Tang, J., et al. (2017). Combined effect of ultrasound and mild temperatures on the inactivation of E. coli in fresh carrot juice and changes on its physicochemical characteristics. J. Food Sci. 82, 2343–2350. doi: 10.1111/1750-3841.13787
Priyadarshini, A., and Priyadarshini, A. (2018). “Market dimensions of the fruit juice industry,” in Fruit Juices: Extraction, Composition, Quality and Analysis (London: Elsevier Inc.), 15–32. doi: 10.1016/B978-0-12-802230-6.00002-3
Rajauria, G., and Tiwari, B. K. (2018). “Fruit juices: an overview,” in Fruit Juices: Extraction, Composition, Quality and Analysis (London: Elsevier Inc.), 3–13. doi: 10.1016/B978-0-12-802230-6.00001-1
Rajewska, K., and Mierzwa, D. (2017). Influence of ultrasound on the microstructure of plant tissue. Innov. Food Sci. Emerg. Technol. 43, 117–129. doi: 10.1016/j.ifset.2017.07.034
Rojas, M. L., Leite, T. S., Cristianini, M., Alvim, I. D., and Augusto, P. E. D. (2016). Peach juice processed by the ultrasound technology: changes in its microstructure improve its physical properties and stability. Food Res. Int. 82, 22–33. doi: 10.1016/j.foodres.2016.01.011
São José, J. F. B., Medeiros, H. S., De Andrade, N. J., Ramos, A. M., and Vanetti, M. C. D. (2018). Effect of ultrasound and chemical compounds on microbial contamination, physicochemical parameters and bioactive compounds of cherry tomatoes. Italian J. Food Sci. 30, 467–486. doi: 10.14674/IJFS-1015
Shen, Y., Zhu, D., Xi, P., Cai, T., Cao, X., Liu, H., et al. (2021). Effects of temperature-controlled ultrasound treatment on sensory properties, physical characteristics and antioxidant activity of cloudy apple juice. LWT 142, 111030. doi: 10.1016/j.lwt.2021.111030
Silva, V. M., Sato, A. C. K., Barbosa, G., Dacanal, G., Ciro-Velásquez, H. J., and Cunha, R. L. (2010). The effect of homogenisation on the stability of pineapple pulp. Int. J. Food Sci. Technol. 45, 2127–2133. doi: 10.1111/j.1365-2621.2010.02386.x
Tiwari, B. K., Muthukumarappan, K., O'Donnell, C. P., and Cullen, P. J. (2009). Inactivation kinetics of pectin methylesterase and cloud retention in sonicated orange juice. Innov. Food Sci. Emerg. Technol. 10, 166–171. doi: 10.1016/j.ifset.2008.11.006
Wang, J., Wang, J., Vanga, S. K., and Raghavan, V. (2020). High-intensity ultrasound processing of kiwifruit juice: Effects on the microstructure, pectin, carbohydrates and rheological properties. Food Chem. 313, 126121. doi: 10.1016/j.foodchem.2019.126121
Wang, J., Wang, J., Ye, J., Vanga, S. K., and Raghavan, V. (2019). Influence of high-intensity ultrasound on bioactive compounds of strawberry juice: profiles of ascorbic acid, phenolics, antioxidant activity and microstructure. Food Control 96, 128–136. doi: 10.1016/j.foodcont.2018.09.007
Keywords: thermosonication, mixed juices, rheological properties, ultrasound, preservation
Citation: Lepaus BM, Santos AKPdO, Spaviero AF, Daud PS and de São José JFB (2022) Stability parameters during refrigerated storage and changes on the microstructure of orange-carrot blend juice processed by high-power ultrasound. Front. Sustain. Food Syst. 6:891662. doi: 10.3389/fsufs.2022.891662
Received: 08 March 2022; Accepted: 18 October 2022;
Published: 10 November 2022.
Edited by:
Giovanna Ferrari, University of Salerno, ItalyReviewed by:
Debabandya Mohapatra, Central Institute of Agricultural Engineering (ICAR), IndiaCopyright © 2022 Lepaus, Santos, Spaviero, Daud and de São José. This is an open-access article distributed under the terms of the Creative Commons Attribution License (CC BY). The use, distribution or reproduction in other forums is permitted, provided the original author(s) and the copyright owner(s) are credited and that the original publication in this journal is cited, in accordance with accepted academic practice. No use, distribution or reproduction is permitted which does not comply with these terms.
*Correspondence: Jackline Freitas Brilhante de São José, amFja2xpbmUuam9zZUB1ZmVzLmJy
Disclaimer: All claims expressed in this article are solely those of the authors and do not necessarily represent those of their affiliated organizations, or those of the publisher, the editors and the reviewers. Any product that may be evaluated in this article or claim that may be made by its manufacturer is not guaranteed or endorsed by the publisher.
Research integrity at Frontiers
Learn more about the work of our research integrity team to safeguard the quality of each article we publish.