- 1United Graduate School of Agricultural Sciences, Tottori University, Tottori, Japan
- 2Faculty of Agriculture, Tottori University, Tottori, Japan
- 3Arid Land Research Center, Tottori University, Tottori, Japan
- 4Agricultural Research Corporation, Wad Medani, Sudan
Narrow genetic diversity in the wheat gene pool restricts the improvement of wheat quality traits. Aegilops tauschii possesses valuable genetic diversity that can be used to improve not only biotic and abiotic stresses in arid regions but also wheat yield and quality. Our study, which used 392 multiple synthetic derivatives (MSD) panel developed with Ae. tauschii Coss. introgressions, had three main aims: to explore the genetic diversity of high-molecular-weight glutenin subunits (HMW-GS), to investigate the dough strength and the relationship between protein content and grain yield, and to identify lines with a good flour quality. A wide range of allelic diversity was observed at the Glu-D1 locus, reflecting the impact of the different introgressed portions of Ae. tauschii, and a wide variation was found in dough strength even between lines having the same composition of HMW-GS. We report a negative impact on dough strength of subunit 5t+10t from Ae. tauschii and a relatively positive impact of subunit 2t+12.1t. We identified four MSD lines with significantly enhanced flour quality. Regressing the grain yield of the MSD lines against protein content showed no correlation between the two traits and identified lines with comparable grain yield to the recurrent parent and higher protein content. The identified MSD lines could provide a valuable genetic resource for enhancing the end-use quality of flour without any loss in productivity.
Introduction
Genetic diversity is essential for crop adaptation to diverse and fluctuating environmental conditions. The genetic diversity of common wheat (Triticum aestivum L.) has narrowed due to a bottleneck effect during the polyploid evolution of common wheat and intensive selection during the breeding process in recent decades. This narrow genetic diversity often restricts the improvement of many traits in wheat (Kumar et al., 2019).
Grain yield and grain protein content are important factors affecting the economic value of common wheat. Many breeding programs aim to increase the grain protein content and simultaneously maintain a high grain yield. However, the well-documented negative relationship between grain protein content and grain yield is still a major challenge to producing lines that combine high yield and high protein content and hence good quality (Kibite and Evans, 1984; Cox et al., 1985; Gauer et al., 1992; Delzer et al., 1995; Marinciu and Suaulescu, 2008; Giancaspro et al., 2019; Taheri et al., 2021). In addition, the protein content and grain yield are strongly affected by environmental changes. One well-known example is that high temperatures after anthesis reduce grain yield because individual kernel weights are lower (Sofield et al., 1977; Tahir et al., 2006), and alter protein content and composition (Kolderup, 1975; Tahir et al., 2006). Wheat quality is essentially determined by both the composition and the amount of glutenin and gliadin, the two major components of gluten. The polymeric glutenins, comprising high-molecular-weight glutenin subunits (HMW-GS) and low-molecular-weight glutenin subunits (LMW-GSs), are the main determinant of the unique dough elasticity of wheat flour (Tatham et al., 1985; Payne, 1987; Shewry et al., 2003). The genes encoding HMW-GS are Glu-A1, Glu-B1, and Glu-D1 loci (Payne et al., 1982; Payne, 1987). The Glu-D1 locus has the strongest effect, followed by the Glu-B1 and Glu-A1 loci (Yang et al., 2013). Although HMW-GS constitute about 10% of seed storage proteins, about 80% of the variation in the Alveograph w value (which is a combined measure of dough strength and extensibility) can be attributed to variations in HMW-GS composition and protein content (Payne et al., 1988). Therefore, broadening the variation of HMW-GS alleles would potentially lead to increased options for developing wheat flour used in a variety of end-products. Several investigations and explorations have been undertaken in common wheat to find HMW-GS that have significant effects on dough strength. It has been proved that subunit 5+10 at Glu-D1 locus has the highest positive effect on dough strength (Payne, 1987) because the subunit 5 contains an extra cysteine residue at the beginning of the repetitive domain (Anderson et al., 1989). However, the number of excellent glutenin alleles is still limited in common wheat (Wang et al., 2012). Also, the germplasm available to breeders is not diverse enough to facilitate the selection of superior lines.
Many genes from Aegilops tauschii have been successfully transferred to common wheat using synthetic hexaploid wheat (SHW; Mujeeb-Kazi et al., 1996; Gill et al., 2008; Halloran et al., 2008; Ogbonnaya et al., 2013). The SHW has the same genome constitution as common wheat, so the chromosomes/genes introduced through crosses are stably transmitted to the offspring. Ae. tauschii, the D genome donor of common wheat, is a valuable resource of genetic diversity for the endosperm proteins gliadin and glutenin. Furthermore the SHW has a high yield potential compared to bread wheat (Lagudah and Halloran, 1988; Pflüger et al., 2001; Elbashir et al., 2017a; Kumar et al., 2019). Thus, Ae. tauschii can be used as a resource for increasing genetic variation and combining superior alleles for both grain yield and grain quality. However, expression of the genes that affect quality could be completely different when transferred into common wheat (Pflüger et al., 2001). Therefore, to evaluate the effects of these genes in the background of common wheat, a panel of multiple synthetic derivatives (MSD) has been developed using 43 Ae. tauschii accessions that represent the existing diversity in the entire natural habitat (Sohail et al., 2012; Tsujimoto et al., 2015; Gorafi et al., 2018). These 43 Ae. tauschii accessions (Supplementary Table 1) have been classified into three intraspecific lineages: TauL1, TauL2, and TauL3 (Matsuoka et al., 2013). The MSD makes it a powerful platform to detect and quantify the effect of the Ae. tauschii and that is why several studies could detect the impact of the A. tauschii segments on heat, drought, and seed shape characteristics (Elhadi et al., 2021; Itam et al., 2021b).
The objectives of this study were to explore and investigate the genetic diversity of HMW-GS from Ae. tauschii at the Glu-D1 locus, and to evaluate their expression and effects in the background of a common wheat cultivar regarding dough strength, protein content, and grain yield potential.
Materials and Methods
Plant Materials
This study used BC1F5 seeds harvested from 392 BC1F4 MSD panel (Elbashir et al., 2017b), which was developed through crossing and backcrossing of the Japanese common wheat cultivar “Norin 61” (hereafter referred to as N61) with 43 lines of SHW (Tsujimoto et al., 2015; Gorafi et al., 2018). The 43 lines of SHW were derived from crosses between 43 diverse genotypes of Ae. tauschii and T. turgidum var. durum cv. “Langdon” (LDN; Matsuoka and Nasuda, 2004; Kajimura et al., 2011).
We detected HMW-GS in the 43 lines of SHW and subsequently used the data to confirm HMW-GS in the MSD panel. To identify the HMW-GS' alleles in the 392 MSD panel and the 43 lines of SHW, the recurrent parent N61 and LDN were used in each electrophoresis assay.
Experimental Site, Design, and Cultural Practices
The 392 BC1F4 MSD panel was grown in season 2015/2016 in the field of the Arid Land Research Center, Tottori, Japan (35°32′N, 134°13′E, 11 m above sea level), where the soil contains 95% sand, 1.3% silt, and 3.7% clay (Fujiyama and Nagai, 1989). The field experiment was arranged in an augmented randomized complete block design with eight blocks and four replicated checks, one of which was the recurrent parent N61. The plot size was one row with five plants spaced 0.2 m apart. Before sowing, three types of fertilizers were used: Kumiai Fukugo PKN 366 at a rate of 60 kg ha−1 (MC FERTICOM Co., Ltd., Japan), Hitachi Fukugo 1 at a rate of 40 kg/ha (HITACHI CHEMICAL INDUSTRIES Co., Ltd., Japan), and granular carbonated magnesium lime at a rate of 100 kg/ha (SHIMIZU INDUSTRIAL Co., Ltd., Japan). At the tillering stage, the fertilizer Koudokasei 444 (Mitsubishi Shoji Agri-Service Co., Japan) was used at a rate of 500 kg/ha.
Identification of HMW-GS Composition
The composition of HMW-GS in the MSD panel was determined by sodium dodecyl sulfate-polyacrylamide gel electrophoresis (SDS-PAGE) according to the method used by Tanaka et al. (2003). The process of staining and decolorizing the gel was done according to that used by Dyballa and Metzger (2009). The gel was scanned using an image scanner (ES-2200, Seiko Epson Co., Japan).
Since the BC1F5 seeds in the MSD panel might still be genetically segregated, we investigated the composition of HMW-GS in three grains per line. We considered the MSD lines to have HMW-GS introduced from the SHW if at least one out of the three tested seeds had the HMW-GS of SHW.
The HMW-GS alleles at Glu-A1, Glu-B1, and Glu-D1 loci were identified based on the numbering system of Payne and Lawrence (1983). The subunits derived from Ae. tauschii were followed by the superscript designation “t” to refer to their origin. The nomenclature of subunits derived from Ae. tauschii is tentative in this paper because it is only based on electrophoresis and has not been confirmed by DNA analysis.
Evaluation of Flour Quality
Grain yield (g) per plant was calculated from an average of five plants. Whole wheat flour was obtained by grinding 4 g from each line of the MSD panel using a UDY cyclone sample mill (UDY Co., USA) equipped with a 1-mm screen. The protein content of the samples was measured as a percentage of the total weight by near-infrared spectroscopy (NIR composition analyzer KJT-270, Kett Electric Laboratory Co. Ltd., Japan). To assess the gluten quantity and quality, we measured the SDS sedimentation volume in 1 g of flour, using the method according to Takata et al. (1999). The sedimentation volume is highly correlated with bread loaf volume (Axtord et al., 1979), where dough strength is the main factor. For lines that derived their HMW-GS from Ae. tauschii, specific sedimentation values, which are highly correlated with dough strength, were assessed as an index of gluten quality by dividing the SDS sedimentation volume (mL) by protein content (%), because protein content is reported to be highly correlated with sedimentation volume (Moonen et al., 1982; Tanaka and Tsujimoto, 2012).
Statistical Analysis
Data were tested for normality and homogeneity of variance before analysis using the Shapiro–Wilk Test and Levene's Test, respectively. Analysis of variance (ANOVA) was performed for dough strength and protein content of the MSD lines using the GenStat Software program (18th edition). The least significant difference (0.05) was used for mean separation. Duncan's Multiple Range Test was used to compare the mean dough strength of the different HMW-GS combinations using SPSS Software (version 25.0.1). ANOVA for the field experiment was performed using Plant Breeding Tools v. 1.4 software (International Rice Research Institute, http://bbi.irri.org/products). Regression analysis was conducted using Microsoft Excel 2019.
Results
Identification and Frequency of HMW-GS in the MSD Panel
At Glu-A1 locus, 307 lines possessed subunit 2* inherited from N61, and 85 lines had a null allele derived from the LDN genome in SHW (Table 1). At Glu-B1 locus, 288 lines had the subunit pair 7+8 from N61, and 104 lines possessed the subunit pair 6+8 from LDN. As for the Glu-D1 locus, 289 lines inherited the 2.2+12 subunit pair from N61, whereas 103 lines inherited their Glu-D1 subunit pair from SHW harboring Ae. tauschii.
Given the nature of the MSD development method, the expected segregation ratio was 75% from N61 and 25% from SHW. Our result for the frequency of HMW-GS pairs at Glu-A1, Glu-B1, and Glu-D1 loci in the MSD panel fitted to the Mendelian expected ratio of 3:1. This indicates that no special selection occurred for any of the alleles during the development of the MSD panel and that no identical alleles with that of N61 (2.2+12) have been found in Ae. tauschii.
In the 103 lines harboring HMW-GS at Glu-D1 derived from Ae. tauschii, three subunits were x-type (2.1t, 2t, and 5t) and three were y-type (10t, 12t, and 12.1t) (Figure 1). Subunit 12.1t had slightly smaller molecular weight and faster mobility than subunit 12 in N61, whereas subunit 2.1t had slower mobility than subunit 2 in N61. The HMW-GS were found in the form of five different haplotypes, 2t+10t, 2t+12t, 5t+10t, 2.1t+12t, and 2t +12.1t. The most frequent pair of HMW-GS was 2t+12t (42 lines), followed by 5t+10t (30 lines) (Table 2). The subunit pair 2t+12.1t was found in 21 lines, 2t+10t in nine lines, and 2.1t+12t in only one line.
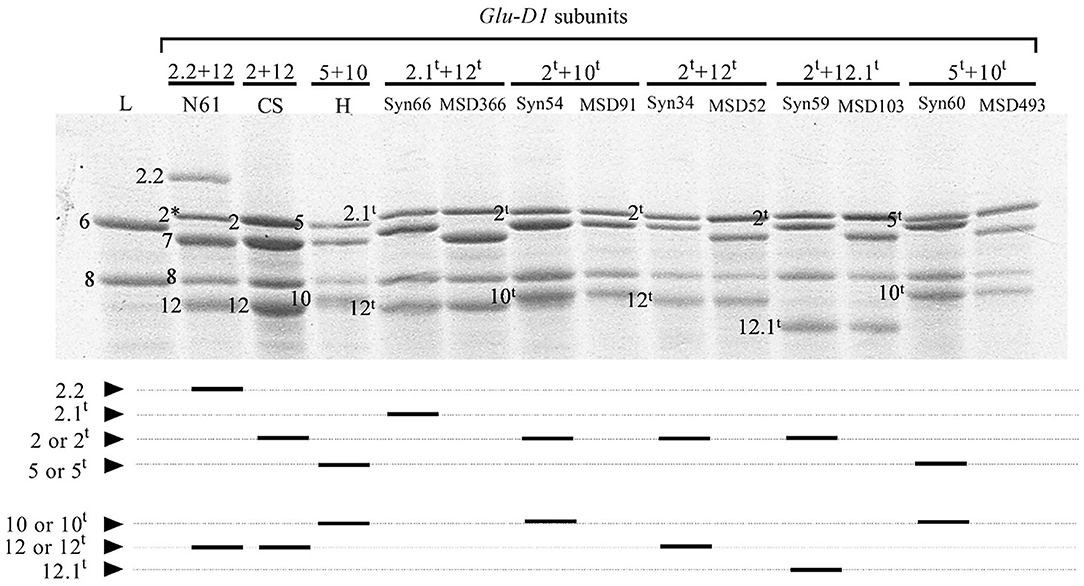
Figure 1. Expression of HMW-GS derived from Aegilops tauschii in (upper panel) SDS-PAGE and (lower panel) a schematic diagram. N61, Norin 61; L, Langdon; CS, Chinese spring; H, Haruhikari; Syn, synthetic hexaploid line; MSD, multiple synthetic derivatives.
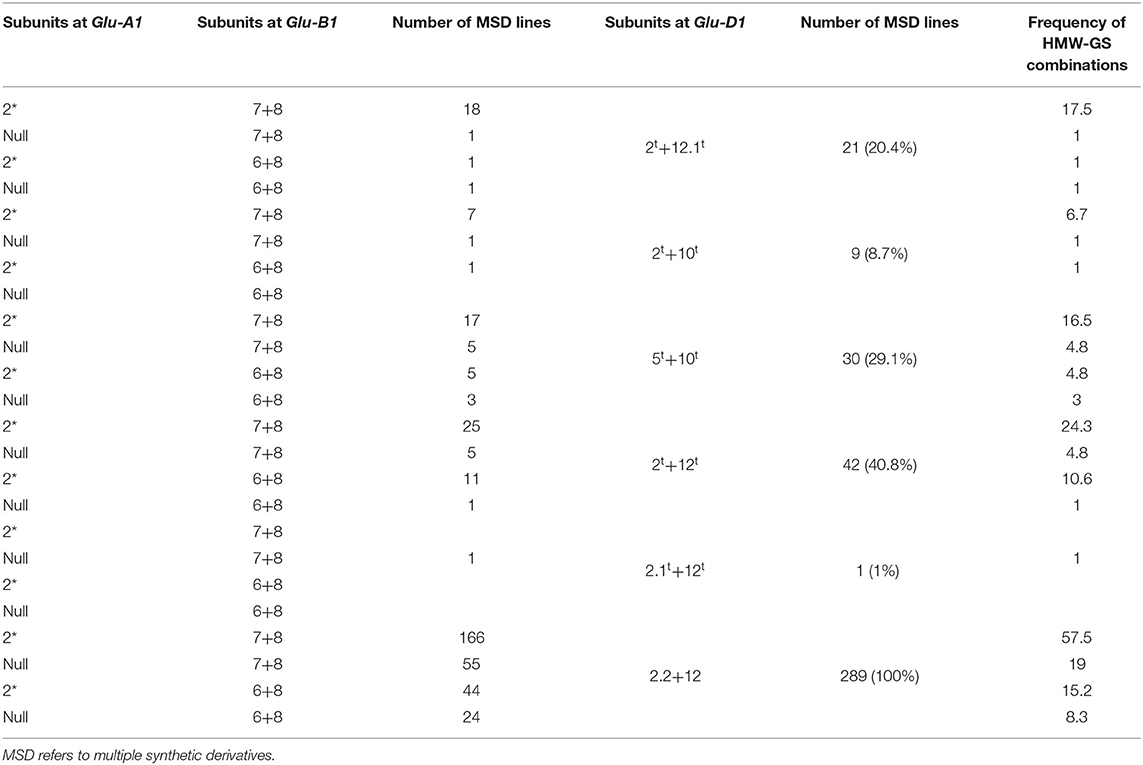
Table 2. Different subunit pairs, combinations, and gene frequency of HMW-GS encoded at Glu-A1, Glu-B1, and Glu-D1 loci in the MSD lines with Glu-D1 derived from Ae. tauschii (103) and N61 (289).
In all, 16 combinations of HMW-GS at the three Glu loci were distinguished in the 103 MSD lines. The most frequent combination was 2*, 7+8, 2t+12t, which was observed in 25 lines, followed by 2*, 7+8, 2t+12.1t, and 2*, 7+8, 5t+10t which were observed in 18 and 17 lines, respectively (Table 2).
Relationship Between HMW-GS in MSD Lines and Ae. tauschii Intraspecific Lineages
The MSD lines used in this study originated from three lineages of Ae. tauschii. The 60 MSD lines from TauL2 Ae. tauschii contained all combinations of HMW-GS except 2.1t+12t (Figure 2). The most common subunit pair was 2t+12t, which was found in 27 MSD lines in TauL2; the subunit pair 2t+12.1t was exclusively found in 21 lines from TauL2; and subunit pairs 5t+10t and 2t+10t were found in seven and five lines, respectively. All 15 lines of the TauL3 lineage contained the subunit pair 2t+12t. In TauL1, the 28 lines possessed three pairs of HMW-GS (5t+10t, 2t+10t, and 2.1t+12t); the most common subunit pair was 5t+10t (23 lines), followed by the subunit pair 2t+10t (four lines), and the subunit pair 2.1t+12t was found in only a single line from TauL1. TauL1 had no lines that possessed subunit pair 2t+12t, although it is the most abundant subunit in MSD lines (Table 2).
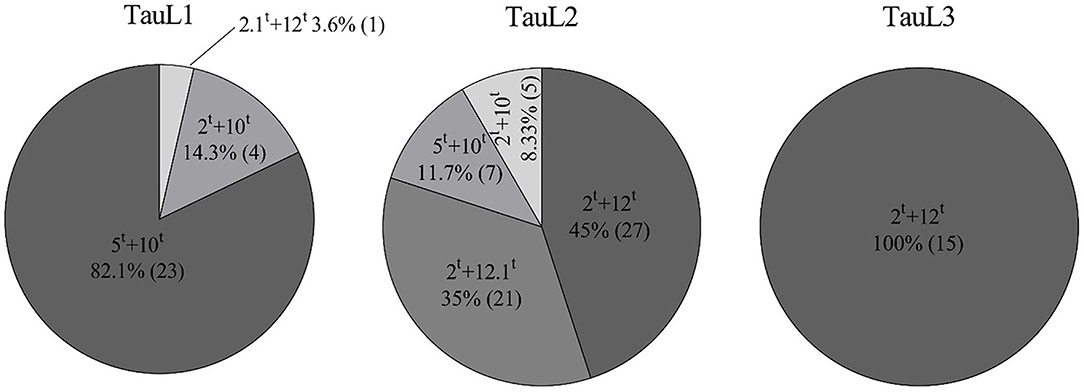
Figure 2. Frequency of the different subunit pairs derived from three lineages of Ae. tauschii: TauL1, TauL2, and TauL3. Numbers in parentheses refer to the number of MSD lines in which the subunit pair appeared.
Evaluation of Dough Strength in the 103 MSD Lines
Highly significant (P < 0.001) differences for dough strength were found among the 103 MSD lines that derived their HMW-GS from Ae. tauschii (Table 3 and Figure 3). Moreover, dough strength for MSD lines showed a normal distribution according to the Shapiro–Wilk normality test (P < 0.05). The variation in dough strength among MSD lines ranged from weak to strong (0.232–0.732 mL/%). In comparison with N61, 3.9% of MSD lines (4 lines, viz. MSD272, MSD363, MSD219, and MSD61) (Supplementary Table 2) showed dough strength significantly higher than N61. A total of 42 MSD lines (40.8%) showed dough strength comparable with that of N61, whereas 55.3% of MSD lines (57 lines) showed significantly lower dough strength than N61.

Table 3. ANOVA and heritability for protein content (%), grain yield/plants (g), and dough strength (mL/%).
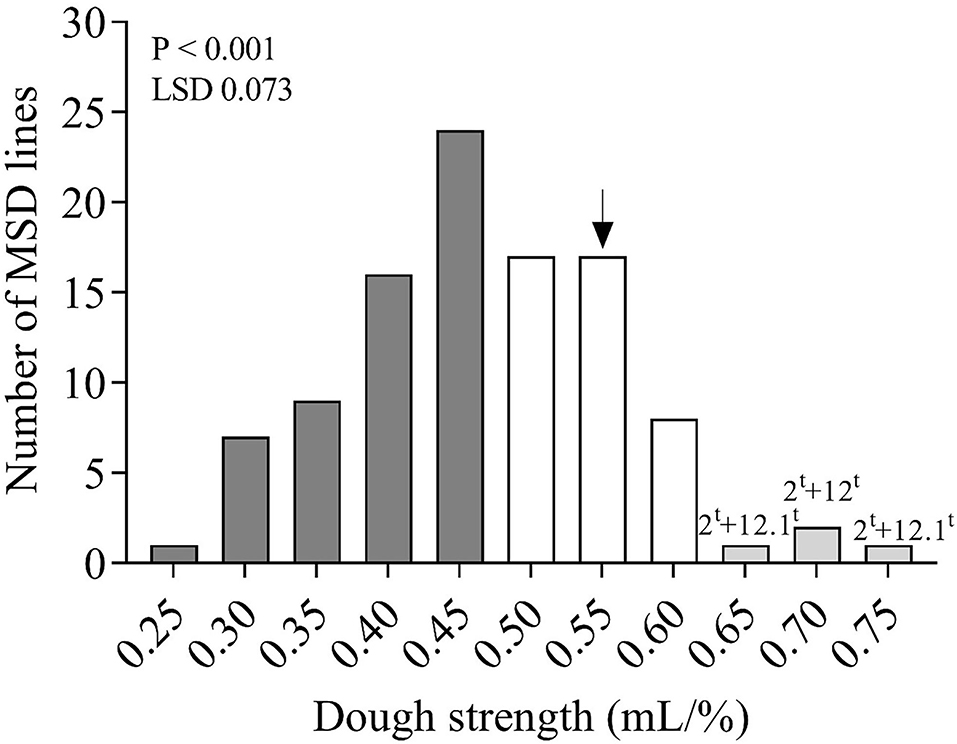
Figure 3. Distribution of the mean dough strength (mL/%) of 103 MSD lines that derived their HMW-GS from Ae. tauschii and the recurrent parent N61, shown by a black arrow. Dark gray, white, and light gray columns indicate the number of lines in the MSD panel that had significantly lower, comparable, or higher dough strength than N61, respectively. Numbers and letters above the columns indicate the important subunit pairs in the MSD lines that had dough strength superior to N61. LSD stands for least significant difference.
Variation and Evaluation in Dough Strength Within the Five HMW-GS Haplotypes Derived From Ae. tauschii
MSD lines with subunit pair 2t+12t exhibited the widest variation in dough strength (0.31–0.68 mL/%) followed by those that carried subunit pairs 5t+10t, 2t+12.1t, and 2t+10t (Figure 4).
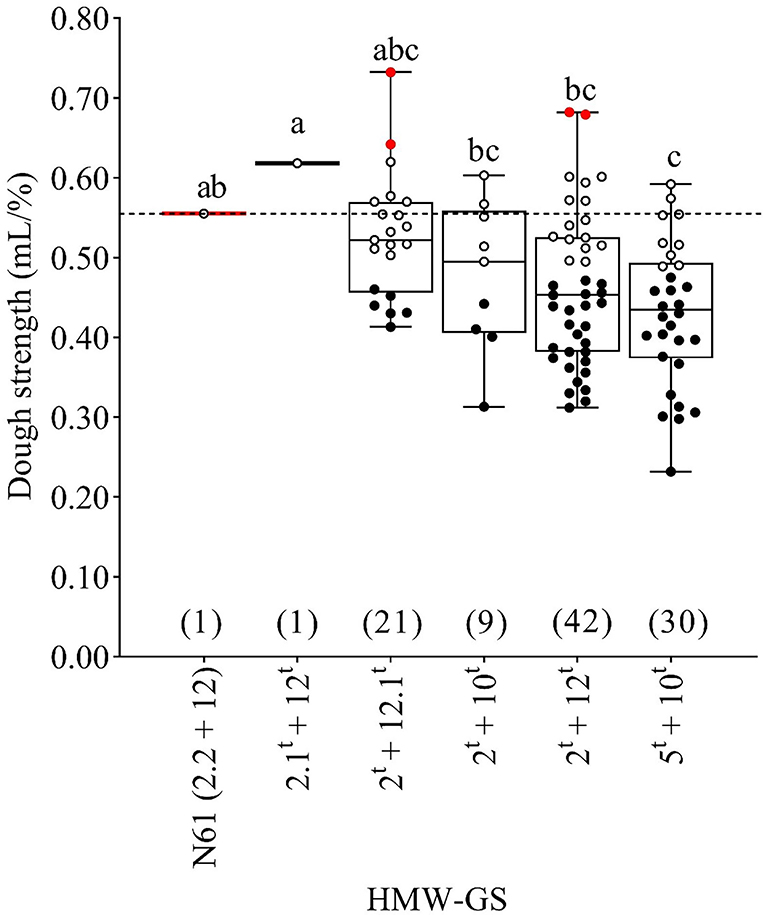
Figure 4. Boxplot for the dough strength (mL/%) as affected by the HMW-GS pairs derived from Ae. tauschii and the recurrent parent N61. Similar lower case letters indicate that the means for HMW-GS pairs are not significantly different according to Duncan's Multiple Range Test at P < 0.05. A horizontal dashed line compares the N61 value with the values of other MSD lines. Red, white, and black dots indicate the MSD lines that were significantly higher, comparable, or lower than N61, respectively, according to the least significant difference (0.05). Numbers in parentheses refer to the number of MSD lines having each HMW-GS pair.
The mean dough strength of the recurrent parent N61 was significantly higher than the mean dough strength for lines possessing subunit pair 5t+10t which exhibited the lower dough strength average among all subunits derived from Ae. tauschii. The dough strength of the single line that carried subunit pair 2.1t+12t was notably higher than the means of the other HMW-GS pairs derived from Ae. tauschii (2t+12.1t, 2t+10t, 2t+12t, and 5t+10t), and was also higher than that of the recurrent parent N61 although this difference was not significant. There was variation inside each subunit pair where some lines were comparable, and others were significantly lower or higher than N61. For example, although the means dough strength for the subunits 2t+12.1t and 2t+12t was comparable to N61, there were two lines from both subunits that were significantly higher than N61 and 13 and 14 comparable, six and 24 lines were significantly lower than N61 respectively (Figure 4).
Impact of Glu-D1 Locus on Dough Strength
To explore the impact of the Glu-D1 locus on dough strength and exclude the effects of Glu-A1 and Glu-B1 loci, we calculated the mean dough strength of the lines that had the same subunits at Glu-A1 and Glu-B1 loci but different subunits at Glu-D1 locus (Figure 5). Accordingly, lines were divided into four groups: (i) Group 1 consisted of lines with subunit combination of 2* and 7+8 at Glu-A and Glu-B loci, respectively, and four subunit pairs at Glu-D1 locus: 2t+12.1t, 2t+12t, 2t+10t, and 5t+10t; (ii) Group 2 comprised of lines with subunit combination of 2* and 6+8 at Glu-A and Glu-B loci, respectively, together with four subunit pairs at Glu-D1 locus: 2t+12.1t, 2t+12t, 2t+10t, and 5t+10t; (iii) Group 3 consisted of lines showing null subunits and 7+8 at Glu-A and Glu-B loci, respectively, and five different subunit pairs at Glu-D1 locus: 2.1t+12t, 2t+12t, 2t+12.1t, 2t+10t, and 5t+10t; (iv) Group 4 consisted of subunits null and 6+8 at Glu-A and Glu-B locus, respectively, and three different subunit pairs at Glu-D1 locus: 2t+12t, 2t+12.1t, and 2t+10t (Figure 5).
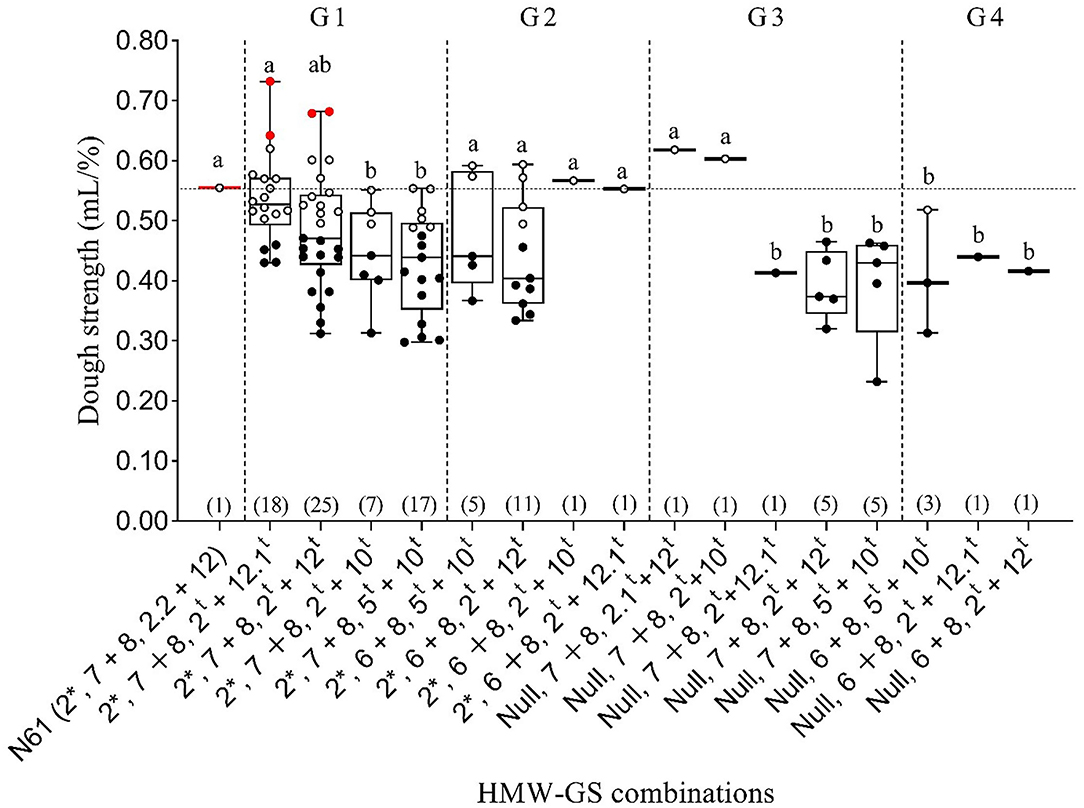
Figure 5. Boxplot of dough strength (mL/%) for different subunit combinations at three Glu-1 loci in 103 MSD lines that derived their HMW-GS from Ae. tauschii and the recurrent parent N61. The horizontal dotted line compares the N61 value with those of MSD lines carrying different subunit combinations, which are separated by dashed vertical lines into groups G1–G4. Within a group, the same letter indicates that the means are not significantly different at the 5% probability level according to Duncan's Multiple Range Test. Red, white and black dots indicate the MSD lines that had significantly higher, comparable, or lower dough strength, respectively, than N61, according to least significant difference (0.05). Numbers in parentheses refer to the number of MSD lines having each HMW-GS combination.
We found that MSD lines with the same subunit combination at Glu-A1 and Glu-B1 loci showed a wide variation in their dough strength values when the subunit pair at Glu-D1 was different. In Group 1, N61 and the MSD lines that had subunit pair 2t+12.1t at Glu-D1 locus had significantly higher mean dough strength of than lines with subunit pairs 2t+10t and 5t+10t (Figure 5). In Group 2, no significant differences were observed in the dough strength of the MSD lines. In Group 3, N61 and two MSD lines that had subunit pairs 2.1t+12t and 2t+10t at Glu-D1 locus had significantly higher mean dough strength than those with subunit pairs 2t+12.1t, 2t+12t, and 5t+10t. In Group 4, no significant differences were observed in the dough strength of the MSD lines, but all had significantly lower dough strength than that of N61. When results in Group 1 were considered in detail, two MSD lines with the subunit combination of 2*, 7+8, 2t+12.1t showed significantly higher dough strength than that of N61, whereas 12 lines were comparable and 4 lines were significantly lower than N61 respectively. Interestingly, two lines out of 4 lines that were significantly lower than N61 were developed from the same Ae. tauschii accession of the superior lines (MSD272 and MSD219) of the same combination (2*, 7+8, 2t+12.1t). For the subunit combination of 2*, 7+8, 2t+12t, two lines were significantly higher than N61, 10 were comparable and 13 lines were significantly lower than N61 respectively. Also, out of the 13 lines that were significantly lower than N61 in this combination there was three and two lines were developed from the same Ae. tauschii accession of the superior lines MSD61 and MSD363 respectively. On the other hand, all lines with the subunit combinations of null, 7+8, 5t+10t and null, 7+8, 2t+12tshowed significantly lower dough strength than N61 (Figure 5).
Relationship Between Protein Content and Grain Yield/Plant for the MSD Lines
To explore the impact of introgressions from Ae. tauschii on the yield and the quality, we performed a regression analysis for protein content and grain yield/plant (Figure 6). Results showed no relationship between the two traits (r = 0.046; P < 0.6438). The grain yield/plant in all MSD lines did not differ from N61 (according to the least significant difference of 0.05) (Table 3). For the protein content, 69 lines were significantly higher (Supplementary Table 2), 20 lines were significantly lower, and 15 were comparable to N61. Thus, we separated the MSD lines into three categories (A), (B), and (C) based on significant differences in protein content.
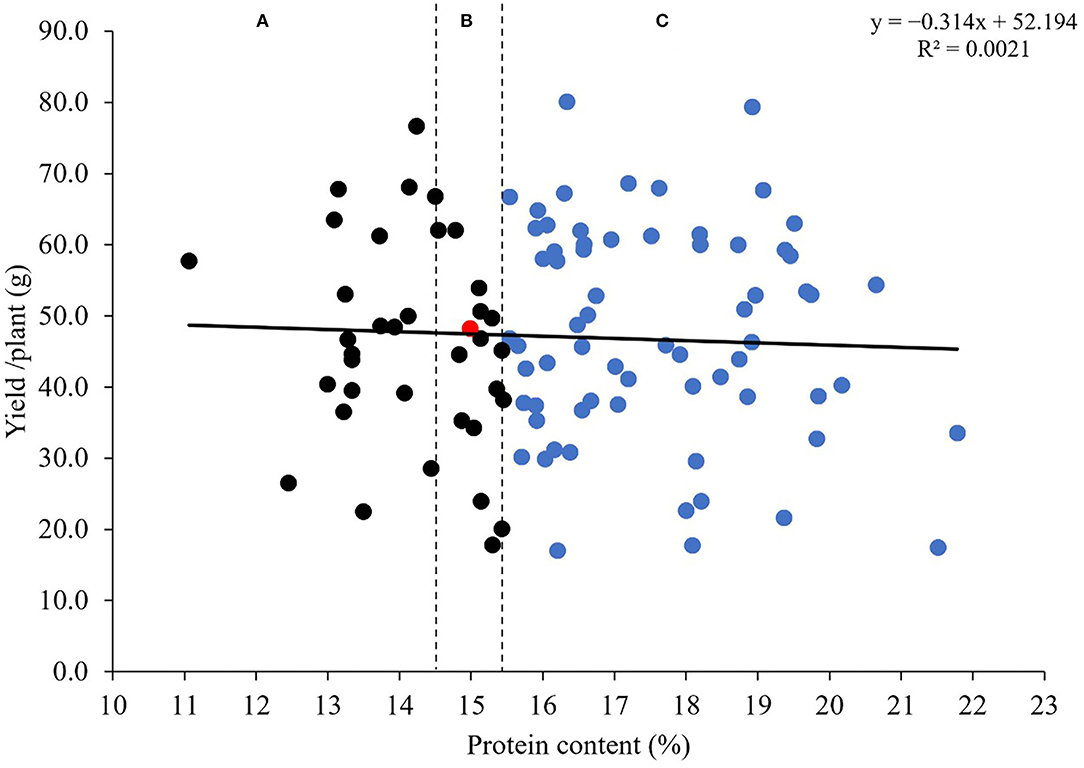
Figure 6. Regression analysis of the relationship between protein content (%) and grain yield/plant (g) for 103 MSD lines that derived their HMW-GS from Ae. tauschii and the recurrent parent N61, shown by a red dot. Vertical dotted lines classify results of comparable grain yield/plant into those with (A) a lower protein content than N61, (B) a comparable protein content than N61, and (C) a higher protein content than N61 (indicated with blue dots).
Discussion
Identification and Frequency of HMW-GS in the MSD Panel
In this study, we reported a large variation in HMW-GS and flour quality in MSD lines derived from 43 Ae. tauschii accessions in the background of the Japanese wheat cultivar N61.
Our investigation at Glu-D1 locus revealed five types of HMW-GS pairs derived from Ae. tauschii. Sixteen subunit combinations at Glu-1 loci were distinguished in the 103 MSD lines. This result is considered representative of the wide diversity in Ae. tauschii across their natural habitat.
Our result on the frequency of HMW-GS at Glu-A1, Glu-B1, and Glu-D1 loci in the MSD panel (Table 1) indicated that the SHW genome was successfully introgressed into the recurrent parent (N61) through self-pollinating and backcrossing, and that no identical alleles with that of N61 (2.2+12) have been found in Ae. tauschii. The subunit 2.2 (x-type subunit) has not been observed in any previous study of Ae. tauschii, which supports the hypothesis of Payne et al. (1983) that this subunit arose within hexaploid wheat by a rare unequal crossing-over with another HMW-GS gene. Since there is a tight link between x-type and y-type subunits in bread wheat (Lawrence and Shepherd, 1980), there is no possibility that some lines can simultaneously derive subunit 12 from N61 (which is a y-type subunit) and x-type subunits from Ae. tauschii. This result agrees with Gorafi et al. (2018) who analyzed the crossing over status of MSD individuals and found the result consistent with the expected ratio after one backcross event.
In our study, we used SDS-PAGE analysis, the traditional standard for distinguishing HMW-GS; thus, the identification of the five subunits that derived from Ae. tauschii was based on previously reported electrophoresis mobility results. For instance, William et al. (1993), Rasheed et al. (2012), and Tariq et al. (2018) reported similar SDS-PAGE mobility of 5t+10t and 2t+12t derived from Ae. tauschii accessions. Yan et al. (2003) observed similar SDS-PAGE mobility of 2t+10t and 2t+12t derived from Ae. tauschii accessions. Similarly, the subunit pair 2.1t+12t has been identified according to its SDS-PAGE mobility (Pflüger et al., 2001; Rasheed et al., 2012; Tariq et al., 2018). Our finding was in agreement with Lagudah et al. (1987), who documented the presence of subunit pairs 5t+10t and 2t+12t, which were equivalent to the SDS-PAGE bands of common wheat, and they also found subunit 2.1t in conjunction with subunit 10.1t. Gianibelli et al. (2001) reported for the first time the presence of subunit 2t+12.1t by SDS-PAGE in Ae. tauschii accessions. Our results contrasted with the findings of Delorean et al. (2021), who documented the absence of the 5+10 wheat haplotype in 273 sequenced Ae. tauschii accessions at both the molecular level and also by SDS-PAGE mobility.
The most frequent subunit pair in our study was 2t+12t (40.8%), which is the most abundant pair in common wheat as well (Payne, 1987), followed by subunit pair 5t+10t (29.1%) (Table 2). The same pattern of frequency for these two pairs (2t+12t and 5t+10t) has been observed in primary SHW derived from 52 Ae. tauschii accessions (Tariq et al., 2018). Rasheed et al. (2012) reported a lower frequency for the subunit pair 2t+12t and a higher frequency for 5t+10t in 95 selected synthetic lines developed by the International Maize and Wheat Improvement Center. Subunit pairs 2t+10t and 2.1t+12t were found in 9 lines (8.7%) and only one line (1%), respectively. In contrast to our result, the subunit pair 2t+10t has been found at the higher frequency of 12.63% in 198 Ae. tauschii accessions (Yan et al., 2003), and 2.1t+12t has been reported in six accessions in a 92 accessions of Ae. tauschii accessions (Pflüger et al., 2001) and at a frequency of 16.8% in a 95 synthetic hexaploid accessions (Rasheed et al., 2012).
Relationship Between HMW-GS in the MSD Lines and Ae. tauschii Intraspecific Lineages
TauL2 exhibited the widest diversity at the Glu-D1 locus, compared to TauL1 and TauL3. MSD lines that belonged to TauL2 contained all types of HMW-GS derived from Ae. tauschii except the subunit 2.1t+12t. All MSD lines belonging to TauL2 originated from Iran. This diversity at Glu-D1 alleles in TauL2 matched well with literature that considers Iran to be the center of genetic variation of Ae. tauschii (Dudnikov and Goncharov, 1993). Also, Delorean et al. (2021) evaluated the Glu-D1 diversity relative to the geographic origin of Ae. tauschii accessions and found that the greatest concentration of haplotype diversity was located along the shores of the Caspian Sea in Iran. Similarly, Lagudah and Halloran (1988) reported that the northeastern region of Iran exhibited a wide diversity of the Glu-D1 subunits. Therefore, identifying geographical areas where the progenitor species of existing SHW were collected would assist in guiding future collection missions (Ogbonnaya et al., 2013).
Subunit 12.1t exclusively belongs to the Ae. tauschii genome and does not exist at the Glu-D1 locus in common wheat (Tahernezhad et al., 2013). In our study, we found the subunit pair 2t+12.1t exclusive to TauL2, which might indicate that this subunit pair has a unique origin, but further studies are needed to confirm this. All lines that belonged to TauL3, which originated from Georgia, carried subunit pair 2t+12t and were genetically similar to TauL2 (Matsuoka et al., 2013). Delorean et al. (2021) studied gene-level phylogeny at Glu-D1 for 273 sequenced Ae. tauschii accessions and showed that a unique group of Glu-D1 alleles belonging to Lineage 3 accessions was found within a narrow clade with Lineage 2.
The most common 2t+12t subunit pair was not found in TauL1. This may indicate that Ae. tauschii genotypes belonging to this TauL lineage may not have been involved in the evolution of common wheat. Indeed, it appears that TauL2 and TauL3 are closer to common wheat than TauL1 because they contain the most common HMW-GS allele prevalent in common wheat (2t+12t) (Matsuoka et al., 2013). Moreover, Delorean et al. (2021) demonstrated that the superior subunit pair 5+10 was found to be clustered very tightly with TauL3, whereas the wheat subunit pair 2+12 was found to be clustered with TauL2, indicating the contribution of these two lineages (TauL2 and TauL3) to the current wheat genome.
Evaluation of Dough Strength of the MSD Lines
We evaluated the dough strength of the 103 MSD lines that derived their HMW-GS from Ae. tauschii to explore the effect of the wild gene in the background of N61. The significant genotypic differences (P < 0.001) observed in dough strength indicated high genetic diversity among the MSD lines. This variation has been attributed mainly to different introgression segments of Ae. tauschii in the MSD lines (Itam et al., 2021a). Itam et al. (2021b) and Elbashir et al. (2017a) also found high genetic diversity in the MSD lines for different traits and have attributed these variations to Ae. tauschii.
We calculated the mean dough strength for each of the five pairs of HMW-GS derived from Ae. tauschii. In our study, the subunit 2.1t exhibited the strongest dough strength average in combination with subunit 12t, and this pair was significantly higher than 5t+10t, 2t+10t, and 2t+12t. This indicates the positive impact of this subunit when combined with subunit 12t; however, it has been reported to have a weak contribution to specific rheological characteristics when associated with subunit 10.1t in SHW (Lagudah et al., 1987). The subunit pair 2t+12.1t also showed relatively strong dough strength. Two MSD lines possessing this subunit pair showed significantly stronger dough compared to N61, indicating the positive impact of this subunit pair on dough strength. To the best of our knowledge, this is the first time that the effect of subunit pair 2t+12.1t in Ae. tauschii on wheat quality has been studied in the background of a wheat cultivar.
Payne (1987) proved that the subunit pair 5+10 at Glu-D1 locus has the highest positive effect on dough strength and a higher Glu-1 score compared to 2+12. This explains its frequent association with dough characterized by stronger elasticity and superior end-use qualities for bread making. Results in our study showed that lines carrying subunit 5t+10t exhibited the lowest dough strength values among all subunits at Glu-D1 and were significantly lower than N61. For the first time, the impact on dough strength of subunit pair 5t+10t inherited from Ae. tauschii in the background of a wheat cultivar is reported as poor. Previous studies documented that the subunit pair 5t+10t, which has mobility in SDS-PAGE typical to bands of 5+10 in wheat derived from Ae. tauschii, is associated with good bread-making quality (Lagudah et al., 1987; Hsam et al., 2001). We suggest that the decreased dough strength values in this study of lines carrying subunit pair 5t+10t could be due to the lack of the extra cysteine in 1Dx5. The absence of an additional cysteine in subunit 1Dx5t derived from Ae. tauschii has been reported in previous studies (Pflüger et al., 2001). It is also possible that the subunit pair (5t+10t) present in our materials might be a different pair of the same size—and therefore with the same mobility in SDS-PAGE—as the subunits 5+10 in T. aestivum. Delorean et al. (2021) used haplotype molecular sequence diversity and SDS-PAGE to explore whether the electrophoresis mobility would reflect the differences visible at the molecular level, and observed that in some cases genes showed a difference in SDS-PAGE mobility, but the alleles looked identical at the molecular level. Similarly, haplotypes were seen to be different at the molecular level but identical in SDS-PAGE mobility. Interestingly, the presence of an Ae. tauschii haplotype identical to that of wheat subunit pair 2+12 and the absence, even by SDS-PAGE mobility, of the exact wheat 5+10 haplotype in the Ae. tauschii accession has been documented (Delorean et al., 2021). Likewise, the difference in basic isoelectric value between subunit 5t+10t derived from Ae. tauschii and the same subunit pair in common wheat has been confirmed by a two-dimensional method (isoelectric focusing with SDS-PAGE), although these subunit pairs had identical electrophoretic mobility (Lagudah and Halloran, 1988). Mackie et al. (1996) reported that the subunits Dy10t and Dy12t from Ae. tauschii were more hydrophobic than those from T. aestivum. Yan et al. (2003) reported a difference in relative mobility by A-PAGE between subunits Dx5t and Dy10t from Ae. tauschii and those of common wheat, although they showed the same mobility under SDS-PAGE. Thus, the unexpectedly poor impact of subunit 5t+10t from Ae. tauschii on dough strength could be attributed to the lack of an extra cysteine or to difficulties in interpreting SDS-PAGE mobility. More confirmatory investigations are needed.
Impact of Glu-D1 Locus on Dough Strength
The same subunit may play varied roles on wheat quality in different pairs of HMW-GS (Zhao et al., 2020). Our result showed a wide variation even between the same HMW-GS pair. This variation in the same subunit pair, and even between sister lines with the same subunit pair, indicates that there might be other factors/genes that affect dough strength. It might be due to the different recombinant portions from SHW (introgressed segments) across the 21 chromosomes. The presence of different introgressed segments from SHW in MSD lines, including sister lines, has been documented (Itam et al., 2021a). These different genomic segments have been found to cause a variation in physio-agronomic traits between MSD lines (Itam et al., 2021a). In further study, a genome-wide association study will need to be performed to find factors/genes other than HMW-GS. Furthermore, LMW-GS and gliadin, which were not investigated in this study, are known to contribute markedly to flour quality, sometimes even more so than the HMW-GS (Pogna et al., 1982; Gupta et al., 1989). Therefore, revealing the allelic compositions of LMW-GS and gliadin is very important to better understand the observed variations in wheat quality.
Two lines (MSD61 and MSD363) (Supplementary Table 2 and Figure 4) that carried 2t+12t exhibited good dough strength despite the fact that this subunit pair is frequently associated with weak dough strength (Payne, 1987). This might be attributed to their high proportion of total HMW-GS at the Glu-D1 locus, as has been reported earlier (Horvat et al., 2006).
We observed that two lines that carried the null allele at the Glu-A1 locus showed dough strength values higher than the recurrent parent N61. This suggested that the introgression of the Glu-D1 locus from Ae. tauschii compensated for the negative impact of the null allele in these lines, where most of the studies reported a significant negative effect of null allele and its association with lower values of gluten strength (Ruiz and Carrillo, 1993; Raciti et al., 2003). Thus, those lines could be used in breeding programs to improve the quality and overcome the negative impact of the null allele.
Relationship Between Protein Content and Grain Yield in the MSD Lines
Although the negative relationship between grain protein content and grain yield is well-known (Kibite and Evans, 1984; Cox et al., 1985; Gauer et al., 1992; Delzer et al., 1995; Marinciu and Suaulescu, 2008; Giancaspro et al., 2019; Taheri et al., 2021), our findings showed no relationship between the two traits in MSD lines. Moreover, most of the MSD lines had higher or lower protein content with comparable grain yield values to the recurrent parent. This may indicate that the increase or decrease in protein content that occurred due to the introgression of the D genome is independent of the grain yield in MSD lines. Also, it may have increased the variation in protein content to such an extent that it counteracts the generally known negative relationship between protein content and grain yield. Although our finding is based on a homogenous grain yield and protein content of five independent plants evaluated for one season under optimum condition, it is very promising and pave the way for more detailed investigation and validation. The regression analysis used was powerful and allowed the classification of the MSD lines in different groups considering their protein content and grain yield. Some lines in group C had a clear good comparable grain yield and high protein content compared to N61, these lines could be a target for more detailed analysis and evaluation to elucidate the basis of the positive or no correlation between the grain yield and protein content especially that breaking the negative relationship between these traits is an important aspect for wheat breeding to increase the grain yield and maintain the quality characteristics. The identified MSD lines could provide a valuable genetic resource for enhancing the end-use quality without any loss in productivity.
Conclusion
This study found that the MSD lines derived all the allelic variations at Glu-D1 locus that existed in their ancestor Ae. tauschii accessions. Five subunit pairs (2.1t+12t, 2t+12.1t, 2t+12t, 2t+10t, and 5t+10t) were identified with different frequency in 103 MSD lines. These subunit pairs may offer different options in breeding programs for different end-use products. The MSD lines also exhibited a wide variation in dough strength even in lines with the same HMW-GS composition, and even between sister lines with the same HMW-GS composition. Since dough strength (elasticity) is a critical factor determining the end-use quality of wheat flour, the variation that the MSD lines showed on dough strength (from strong to weak) could be used in breeding programs for different purposes, not only for improving bread-making quality. We documented the poor impact of subunit pair 5t+10t from Ae. tauschii on dough strength in contrast to the well-documented positive impact of this subunit pair on dough strength. However, we found the subunit pair 2t+12.1t to have a positive impact on dough strength.
We identified four MSD lines that significantly enhanced the flour quality, MSD219, MSD363, MSD272, and MSD61, which carried two different alleles at the Glu-D1 locus (2.1t+12t and 2t+12t) derived from Ae. tauschii. These lines are promising and could serve as a good source to improve wheat flour quality in the breeding programs. A total of 69 MSD lines were identified with comparable grain yield and significantly higher protein content than the recurrent parent N61. These MSD lines could be used in breeding programs to improve wheat quality without any concern about the deterioration in grain yields.
Data Availability Statement
The original contributions presented in the study are included in the article/Supplementary Material, further inquiries can be directed to the corresponding author.
Author Contributions
HTs, HTa, YG, and IT constructed the study concept. HTsu provided the materials. IM, HO, NK, YG, and HTa performed the experiments. IM wrote the 1st draft of the manuscript. HO, NK, HM, YG, IT, HTs, and HTa contributed to analysis, data interpretation, and assisted in manuscript preparation. All authors reviewed the manuscript and approved the final version.
Funding
This research was supported by the Science and Technology Research Partnership for Sustainable Development (SATREPS). Japan Science and Technology Agency (JST, JPMJSA1805)/Japan International Cooperation Agency (JICA). JSPS KAKENHI Grant Number JP20K05983 and the Marginal Region Agriculture Project of Tottori University.
Conflict of Interest
The authors declare that the research was conducted in the absence of any commercial or financial relationships that could be construed as a potential conflict of interest.
The reviewer HA declared a past co-authorship with one of the authors IT to the handling editor.
Publisher's Note
All claims expressed in this article are solely those of the authors and do not necessarily represent those of their affiliated organizations, or those of the publisher, the editors and the reviewers. Any product that may be evaluated in this article, or claim that may be made by its manufacturer, is not guaranteed or endorsed by the publisher.
Acknowledgments
The excellent technical assistance of the staff of the Arid Land Research Center, Tottori University, is warmly acknowledged.
Supplementary Material
The Supplementary Material for this article can be found online at: https://www.frontiersin.org/articles/10.3389/fsufs.2022.887795/full#supplementary-material
Abbreviations
HMW-GS, high-molecular-weight glutenin subunits; MSD, multiple synthetic derivatives; SDS-PAGE, sodium dodecyl sulfate-polyacrylamide gel electrophoresis; SHW, synthetic hexaploid wheat; N61, Norin 61; LDN, Langdon.
References
Anderson, O. D., Greene, F. C., Yip, R. E., Halford, N. G., Shewry, P. R., and Malpica-Romero, J. M. (1989). Nucleotide sequences of the two high-molecular-weight glutenin genes from the D-genome of a hexaploid bread wheat, Triticum aestivum L. cv Cheyenne. Nucl. Acids Res. 17:461. doi: 10.1093/nar/17.1.461
Axtord, D. W., McDermott, E. E., and Redman, D. G. (1979). Note on the sodium dodecyl sulfate test of bread making quality: comparision with pelschenke and Zeleny test. Cereal Chem 56, 582–584.
Cox, M. C., Qualset, C. O., and Rains, D. W. (1985). Genetic variation for nitrogen assimilation and translocation in wheat. I. Dry matter and nitrogen accumulation. Crop Sci. 25, 430–435. doi: 10.2135/cropsci1985.0011183X002500030002x
Delorean, E., Gao, L., Cervantes Lopez, J. F., Wulff, B. B. H., Ibba, M. I., and Poland, J. (2021). High molecular weight glutenin gene diversity in Aegilops tauschii demonstrates unique origin of superior wheat quality 2 3. Commun. Biol. 4:1242. doi: 10.1038/s42003-021-02563-7
Delzer, B. W., Busch, R. H., and Hareland, G. A. (1995). Recurrent selection for grain protein in hard red spring wheat. Crop Sci. 35, 730–735. doi: 10.2135/cropsci1995.0011183X003500030014x
Dudnikov, A. J., and Goncharov, N. P. (1993). Allozyme variation in Aegilops squarrosa. Hereditas 119, 117–122. doi: 10.1111/j.1601-5223.1993.00117.x
Dyballa, N., and Metzger, S. (2009). Fast and sensitive colloidal coomassie G-250 staining for proteins in polyacrylamide gels. J. Vis. Exp. 2009:e1431. doi: 10.3791/1431
Elbashir, A. A. E., Gorafi, Y. S. A., Tahir, I. S. A., Elhashimi, A. M. A., Abdalla, M. G. A., and Tsujimoto, H. (2017a). Genetic variation in heat tolerance-related traits in a population of wheat multiple synthetic derivatives. Breed. Sci. 67, 483–492. doi: 10.1270/jsbbs.17048
Elbashir, A. A. E., Gorafi, Y. S. A., Tahir, I. S. A., Kim, J.-S., and Tsujimoto, H. (2017b). Wheat multiple synthetic derivatives: a new source for heat stress tolerance adaptive traits. Breed. Sci. 2017:16204. doi: 10.1270/jsbbs.16204
Elhadi, G. M. I., Kamal, N. M., Gorafi, Y. S. A., Yamasaki, Y., Takata, K., Tahir, I. S. A., et al. (2021). Exploitation of tolerance of wheat kernel weight and shape-related traits from aegilops tauschii under heat and combined heat-drought stresses. Int. J. Mol. Sci. 22, 1–21. doi: 10.3390/ijms22041830
Fujiyama, H., and Nagai, T. (1989). Studies on responses of plants grown on sand dune soil to a nutrient solution applied by drip irrigation. I. Relation between behavior of nutrients in the soil and nutrient uptake by tomatoes. Soil Sci. Plant Nutr. 35, 55–61. doi: 10.1080/00380768.1989.10434736
Gauer, L. E., Grant, C. A., Bailey, L. D., and Gehl, D. T. (1992). Effects of nitrogen fertilization on grain protein content, nitrogen uptake, and nitrogen use efficiency of six spring wheat (Triticum aestivum L.) cultivars, in relation to estimated moisture supply. Can. J. Plant Sci. 72, 235–241. doi: 10.4141/cjps92-026
Giancaspro, A., Giove, S. L., Zacheo, S. A., Blanco, A., and Gadaleta, A. (2019). Genetic variation for protein content and yield-related traits in a durum population derived from an inter-specific cross between hexaploid and tetraploid wheat cultivars. Front. Plant Sci. 10:1509. doi: 10.3389/fpls.2019.01509
Gianibelli, M. C., Gupta, R. B., Lafiandra, D., Margiotta, B., and Macritchie, F. (2001). Polymorphism of high Mr. glutenin subunits in Triticum tauschii : characterisation by chromatography and electrophoretic Methods 33, 39–52. doi: 10.1006/jcrs.2000.0328
Gill, B. S., Huang, L., Kuraparthy, V., Raupp, W. J., Wilson, D. L., and Friebe, B. (2008). Alien genetic resources for wheat leaf rust resistance, cytogenetic transfer, and molecular analysis. Aust. J. Agric. Res. 59, 197–205. doi: 10.1071/AR07315
Gorafi, Y. S. A., Kim, J.-S., Elbashir, A. A. E., and Tsujimoto, H. (2018). A population of wheat multiple synthetic derivatives: an effective platform to explore, harness and utilize genetic diversity of Aegilops tauschii for wheat improvement. Theor. Appl. Genet. 131, 1615–1626. doi: 10.1007/s00122-018-3102-x
Gupta, R. B., Singh, N. K., and Shepherd, K. W. (1989). The cumulative effect of allelic variation in LMW and HMW glutenin subunits on dough properties in the progeny of two bread wheats. Theor. Appl. Genet. 77, 57–64. doi: 10.1007/BF00292316
Halloran, G. M., Ogbonnaya, F. C., and Lagudah, E. S. (2008). Triticum (Aegilops) tauschii in the natural and artificial synthesis of hexaploid wheat. Aust. J. Agric. Res. 59, 475–490. doi: 10.1071/AR07352
Horvat, D., Drezner, G., Jurković, Z., Šimić, G., Magdić, D., and Dvojković, K. (2006). The importance of high-molecular-weight glutenin subunits for wheat quality evaluation. Poljoprivreda 12, 53–57.
Hsam, S. L. K., Kieffer, R., and Zeller, F. J. (2001). Significance of Aegilops tauschii glutenin genes on breadmaking properties of wheat. Cereal Chem. 78, 521–525. doi: 10.1094/CCHEM.2001.78.5.521
Itam, M. O., Gorafi, Y. S. A., Tahir, I. S. A., and Tsujimoto, H. (2021b). Genetic variation in drought resilience-related traits among wheat multiple synthetic derivative lines: insights for climate resilience breeding. Breed. Sci. 2021:20162. doi: 10.1270/jsbbs.20162
Itam, M. O., Mega, R., Gorafi, Y. S. A., Yamasaki, Y., Tahir, I. S. A., Akashi, K., et al. (2021a). Genomic analysis for heat and combined heat–drought resilience in bread wheat under field conditions. Theor. Appl. Genet. 2021:3969. doi: 10.1007/s00122-021-03969-x
Kajimura, T., Murai, K., and Takumi, S. (2011). Distinct genetic regulation of flowering time and grain-filling period based on empirical study of D-genome diversity in synthetic hexaploid wheat lines. Breed. Sci. 61, 130–141. doi: 10.1270/jsbbs.61.130
Kibite, S., and Evans, L. E. (1984). Causes of negative correlations between grain yield and grain protein concentration in common wheat. Euphytica 33, 801–810. doi: 10.1007/BF00021906
Kolderup, F. (1975). Effects of temperature, photoperiod, and light quantity on protein production in wheat grains. J. Sci. Food Agric. 94, 583–592. doi: 10.1002/jsfa.2740260504
Kumar, A., Kapoor, P., Chunduri, V., Sharma, S., and Garg, M. (2019). Potential of Aegilops sp. for improvement of grain processing and nutritional quality in wheat (Triticum aestivum). Front. Plant Sci. 10, 1–19. doi: 10.3389/fpls.2019.00308
Lagudah, E. S., and Halloran, G. M. (1988). Phylogenetic relationships of Triticum tauschii the D genome donor to hexaploid wheat. Theor. Appl. Genet. 75, 592–598. doi: 10.1007/BF00289125
Lagudah, E. S., Macritchie, F., and Halloran, G. M. (1987). The influence of high-molecular-weight subunits of glutenin from Triticum tauschii on flour quality of synthetic hexaploid wheat. J. Cereal Sci. 5, 129–138. doi: 10.1016/S0733-5210(87)80016-4
Lawrence, G. J., and Shepherd, K. W. (1980). Variation in glutenin protein subunits of wheat. Austr. J. Biol. Sci. 33, 221–233. doi: 10.1071/BI9800221
Mackie, A. M., Lagudah, E. S., Sharp, P. J., and Lafiandra, D. (1996). Molecular and biochemical characterisation of HMW glutenin subunits from T. tauschii and the D genome of hexaploid wheat. J. Cereal Sci. 23, 213–225. doi: 10.1006/jcrs.1996.0022
Marinciu, C., and Suaulescu, N. N. (2008). Cultivar effects on the relationship between grain protein concentration and yield in winter wheat. Rom. Agric. Res. 25, 19–27.
Matsuoka, Y., and Nasuda, S. (2004). Durum wheat as a candidate for the unknown female progenitor of bread wheat: an empirical study with a highly fertile F1 hybrid with Aegilops tauschii Coss. Theor. Appl. Genet. 109, 1710–1717. doi: 10.1007/s00122-004-1806-6
Matsuoka, Y., Nasuda, S., Ashida, Y., Nitta, M., Tsujimoto, H., Takumi, S., et al. (2013). Genetic basis for spontaneous hybrid genome doubling during allopolyploid speciation of common wheat shown by natural variation analyses of the paternal species. PLoS ONE 8, 1–17. doi: 10.1371/journal.pone.0068310
Moonen, J. H. E., Scheepstra, A., and Graveland, A. (1982). Use of the SDS-sedimentation test and SDS-polyacrylamidegel electrophoresis for screening breeder's samples of wheat for bread-making quality. Euphytica 31, 677–690. doi: 10.1007/BF00039206
Mujeeb-Kazi, A., Rosas, V., and Roldan, S. (1996). Conservation of the genetic variation of Triticum tauschii (Coss.) Schmalh.(Aegilops squarrosa auct. non L.) in synthetic hexaploid wheats (T. turgidum L. s. lat. x T. tauschii; 2n= 6x= 42, AABBDD) and its potential utilization for wheat improvement. Genet. Resour. Crop Evol. 43, 129–134. doi: 10.1007/BF00126756
Ogbonnaya, F. C., Abdalla, O., Mujeeb-Kazi, A., Kazi, A. G., Xu, S. S., Gosman, N., et al. (2013). Synthetic hexaploids: harnessing species of the primary gene pool for wheat improvement. Plant Breed. Rev. 37, 35–122. doi: 10.1002/9781118497869.ch2
Payne, P. I. (1987). Genetics of wheat storage proteins and the effect of allelic variation on bread-making quality. Annu. Rev. Plant Physiol. 38, 141–153. doi: 10.1146/annurev.pp.38.060187.001041
Payne, P. I., Holt, L. M., Krattiger, A. F., and Carrillo, J. M. (1988). Relationships between seed quality characteristics and HMW glutenin subunit composition determined using wheats grown in Spain. J. Cereal Sci. 7, 229–235. doi: 10.1016/S0733-5210(88)80004-3
Payne, P. I., Holt, L. M., and Lawrence, G. J. (1983). Detection of a novel high molecular weight subunit of glutenin in some Japanese hexaploid wheats. J. Cereal Sci. 1, 3–8. doi: 10.1016/S0733-5210(83)80003-4
Payne, P. I., Holt, L. M., Worland, A. J., and Law, C. N. (1982). Structural and genetical studies on the high-molecular-weight subunits of wheat glutenin: part 3. Telocentric mapping of the subunit genes on the long arms of the homoeologous group 1 chromosomes. Theor. Appl. Genet. 63, 129–138. doi: 10.1007/BF00303695
Payne, P. I., and Lawrence, G. J. (1983). Catalogue of alleles for the complex gene loci, Glu-A1, Glu-B1, and Glu-D1 which code for high-molecular-weight subunits of glutenin in hexaploid wheat. Cereal Res. Commun. 1983, 29–35.
Pflüger, L. A., D'Ovidio, R., Margiotta, B., Peña, R., Mujeeb-Kazi, A., and Lafiandra, D. (2001). Characterisation of high- and low-molecular weight glutenin subunits associated to the D genome of Aegilops tauschii in a collection of synthetic hexaploid wheats. Theor. Appl. Genet. 103, 1293–1301. doi: 10.1007/s001220100704
Pogna, N. E., Boggini, G., Corbellini, M., Cattaneo, M., and Peruffo, A. D. B. (1982). Association between gliadin electrophoretic bands and quality in common wheat. Can. J. Plant Sci. 62, 913–918. doi: 10.4141/cjps82-135
Raciti, C. N., Doust, M. A., Lombardo, G. M., Boggini, G., and Pecetti, L. (2003). Characterization of durum wheat mediterranean germplasm for high and low molecular weight glutenin subunits in relation with quality. Eur. J. Agron. 19, 373–382. doi: 10.1016/S1161-0301(02)00091-6
Rasheed, A., Safdar, T., Gul-Kazi, A., Mahmood, T., Akram, Z., and Mujeeb-Kazi, A. (2012). Characterization of HMW-GS and evaluation of their diversity in morphologically elite synthetic hexaploid wheats. Breed. Sci. 62, 365–370. doi: 10.1270/jsbbs.62.365
Ruiz, M., and Carrillo, J. M. (1993). Linkage relationships between prolamin genes on chromosomes 1A and 1B of durum wheat. Theor. Appl. Genet. Int. J. Plant Breed. Res. 87, 353–360. doi: 10.1007/BF01184923
Shewry, P. R., Halford, N. G., Tatham, A. S., Popineau, Y., Lafiandra, D., and Belton, P. S. (2003). The high molecular weight subunits of wheat glutenin and their role in determining wheat processing properties. Adv. Food Nutr. Res. 45, 219–302. doi: 10.1016/S1043-4526(03)45006-7
Sofield, I., Evans, L. T., Cook, M. G., and Wardlaw, I. F. (1977). Factors influencing the rate and duration of grain filling in wheat. Funct. Plant Biol. 4, 785–797. doi: 10.1071/PP9770785
Sohail, Q., Shehzad, T., Kilian, A., Eltayeb, A. E., Tanaka, H., and Tsujimoto, H. (2012). Development of diversity array technology (DArT) markers for assessment of population structure and diversity in Aegilops tauschii. Breed. Sci. 62, 38–45. doi: 10.1270/jsbbs.62.38
Taheri, A., Abad, H. H. S., Nourmohammadi, G., and Ardabili, M. S. (2021). Investigating quantitative and qualitative performance of bread wheat genotypes under different climatic conditions. Gesunde Pflanz 73, 229–238. doi: 10.1007/s10343-021-00547-5
Tahernezhad, Z., Musavi, Z., alabedin, Zamani, M. J., Jafar Aghaei, M., and Rostam Foroudi, B. (2013). Allelic diversity of high molecular weight glutenin subunits (HMW-GS) in Iranian Aegilops tauschii Coss. accessions by sodium dodecyl sulphate polyacrylamide gel electrophoresis (SDS-PAGE). Genet. Resour. Crop Evol. 60, 905–911. doi: 10.1007/s10722-012-9887-6
Tahir, I. S. A., Nakata, N., Ali, A. M., Mustafa, H. M., Saad, A. S. I., Takata, K., et al. (2006). Genotypic and temperature effects on wheat grain yield and quality in a hot irrigated environment. Plant Breed. 125, 323–330. doi: 10.1111/j.1439-0523.2006.01236.x
Takata, K., Yamauchi, H., Iriki, N., and Kuwabara, T. (1999). Prediction of bread-making quality by prolonged swelling SDS-sedimentation test [in soft wheat]. Breed. Sci. 66, 244–259. doi: 10.1270/jsbbs.49.221
Tanaka, H., Tomita, M., Tsujimoto, H., and Yasumuro, Y. (2003). Limited but specific variations of seed storage proteins in Japanese common wheat (Triticum aestivum L.). Euphytica 132, 167–174. doi: 10.1023/A:1024638616507
Tanaka, H., and Tsujimoto, H. (2012). Positive or negative effects on dough strength in large-scale group-1 chromosome deletion lines of common wheat (Triticum aestivum L.). Euphytica 186, 57–65. doi: 10.1007/s10681-011-0489-8
Tariq, M. J., Shah, M. K. N., Hassan, M. U., Sajjad, M., Jamil, M., Ali, N., et al. (2018). Prevelance of higher glutenin variation in synthetic wheat germplasm. J. Anim. Plant Sci. 28, 568–575.
Tatham, A. S., Miflin, B. J., and Shewry, P. R. (1985). The beta-turn conformation in wheat gluten proteins: relationship to gluten elasticity. Cereal Chem. 62, 405–412.
Tsujimoto, H., Sohail, Q., and Matsuoka, Y. (2015). “Broadening the genetic diversity of common and durum wheat for abiotic stress tolerance breeding,” in Advances in Wheat Genetics: From Genome to Field (Tokyo: Springer), 233–238. doi: 10.1007/978-4-431-55675-6_25
Wang, K., An, X. L., Pan, L. P., Dong, K., Gao, L. Y., Wang, S. L., et al. (2012). Molecular characterization of HMW-GS 1Dx3 t and 1Dx4 t genes from Aegilops tauschii and their potential value for wheat quality improvement. Hereditas 149, 41–49. doi: 10.1111/j.1601-5223.2011.02215.x
William, M., Pena, R. J., and Mujeeb-Kazi, A. (1993). Seed protein and isozyme variations in Triticum tauschii (Aegilops squarrosa). Theor. Appl. Genet. 87, 257–263. doi: 10.1007/BF00223774
Yan, Y., Hsam, S. L. K., Yu, J., Jiang, Y., and Zeller, F. J. (2003). Allelic variation of the HMW glutenin subunits in Aegilops tauschii accessions detected by sodium dodecyl sulphate (SDS-PAGE), acid polyacrylamide gel (A-PAGE) and capillary electrophoresis. Euphytica 130, 377–385. doi: 10.1023/A:1023062316439
Yang, Y., Li, S., Zhang, K., Dong, Z., Li, Y., An, X., et al. (2013). Efficient isolation of ion beam-induced mutants for homoeologous loci in common wheat and comparison of the contributions of Glu-1 loci to gluten functionality. Theor. Appl. Genet. 127, 359–372. doi: 10.1007/s00122-013-2224-4
Keywords: Aegilops tauschii, Triticum aestivum L., multiple synthetic derivative panel, high molecular-weight glutenin subunits, wheat flour quality, grain yield, genetic diversity
Citation: Mohamed IES, Oe H, Kamal NM, Mustafa HM, Gorafi YSA, Tahir ISA, Tsujimoto H and Tanaka H (2022) Enhancing Wheat Flour Quality Through Introgression of High-Molecular-Weight Glutenin Subunits From Aegilops tauschii Accessions. Front. Sustain. Food Syst. 6:887795. doi: 10.3389/fsufs.2022.887795
Received: 02 March 2022; Accepted: 13 April 2022;
Published: 16 May 2022.
Edited by:
Athanasios Tsivelikas, International Center for Agricultural Research in the Dry Areas (ICARDA), MoroccoReviewed by:
Uday Chand Jha, Indian Institute of Pulses Research (ICAR), IndiaHafid Aberkane, Nestle R&D Tours, France
Copyright © 2022 Mohamed, Oe, Kamal, Mustafa, Gorafi, Tahir, Tsujimoto and Tanaka. This is an open-access article distributed under the terms of the Creative Commons Attribution License (CC BY). The use, distribution or reproduction in other forums is permitted, provided the original author(s) and the copyright owner(s) are credited and that the original publication in this journal is cited, in accordance with accepted academic practice. No use, distribution or reproduction is permitted which does not comply with these terms.
*Correspondence: Hiroyuki Tanaka, aHRhbmFrYUB0b3R0b3JpLXUuYWMuanA=