- 1Department of Food Science and Technology, University of Energy and Natural Resources, Sunyani, Ghana
- 2Department of Food Science, University of Otago, Dunedin, New Zealand
- 3Department of Food Science and Technology, University for Development Studies, Tamale, Ghana
Traditional food fermentation is a practice that precedes human history. Acidic products such as yogurts and sourdoughs or alcoholic beverages produced through lactic acid or yeast fermentations, respectively, are widely described and documented. However, a relatively less popular group of fermented products known as alkaline fermented foods are common traditional products in Africa and Asia. These products are so called “alkaline” because the pH tends to increase during fermentation due to the formation of ammonia resulting from protein degradation by Bacillus species. Plant-based alkaline fermented foods (AFFs) are generally produced from legumes including soybean, non-soybean leguminous seeds, and other non-legume plant raw materials. Alkaline fermented food products such as natto, douchi, kinema, doenjang, chongkukjang, thua nao, meitauza, yandou, dawadawa/iru, ugba, kawal, okpehe, otiru, oso, ogiri, bikalga, maari/tayohounta, ntoba mbodi, cabuk, and owoh are produced at small industrial scale or household levels and widely consumed in Asia and Africa where they provide essential nutrients and health-promoting bioactive compounds for the population. Alkaline food fermentation is important for sustainable food security as it contributes to traditional dietary diversity, significantly reduces antinutritional components in raw plant materials thereby improving digestibility, improves health via the production of vitamins, and may confer probiotic and post-biotic effects onto consumers. In this review, we present currently available scientific information on plant-based AFFs and their role as sustainable sources of nutrients and bioactive compounds for improved health. Finally, we provide perspectives on research needs required to harness the full potential of AFFs in contributing to nutrition and health.
Overview of Traditional Alkaline Fermented Foods
Food fermentation is a practice that precedes human history and will continue to be with us far into the future as populations around the world today obtain a considerable portion of their nutritional needs and incomes through fermented foods processing (Hesseltine and Wang, 1980; Tamang et al., 2020). When applied to foods, fermentation has a much broader meaning as opposed the strict biochemical definition of “an ATP-generating process in which organic compounds act as both donors and acceptors of electrons” (Kim et al., 2012). Thus, fermented foods and beverages include “foods that are made through desired microbial growth and enzymatic conversions of food components” (Marco et al., 2021). It is estimated that over 5,000 varieties of fermented foods and beverages are produced and consumed around the world from diverse raw materials (substrates) such as cereals, roots and tubers, meat, dairy products, fish, fruits, vegetables and legumes (Owusu-Kwarteng et al., 2012, 2020; Akabanda et al., 2013; Tamang et al., 2016a, 2020; Agyei et al., 2020).
Traditional fermented foods and their associated microbes are diverse depending on the substrate and the methods used for the fermentations. Whether they are produced by uncontrolled natural fermentation or with controlled starter cultures, fermented foods attain their characteristic taste, flavor, consistency, improved nutritional, and functional properties through the effects of microbial assimilation, metabolites production, and enzymatic activities (Owusu-Kwarteng et al., 2015, 2020; Marco et al., 2021). Microbial communities in natural (spontaneous) fermented foods are principally determined by dispersal and selection. Thus, in traditional spontaneous fermentation of foods, naturally occurring autochthonous microorganisms adapt to the complex food substrate and the fermentation environment leading to competitive selection of the microbial species that are more effectively adapted and positively respond to the in-situ conditions (Ouoba et al., 2007; Illeghems et al., 2012; Walsh et al., 2016; Einson et al., 2018). Consequently, an active change in the microbial community and metabolic profile gets established, leading to a stable fermented food ecosystem with characteristic microbiota structure and composition, metabolome, organoleptic properties, and nutritional and health benefits (Giraffa, 2004; Wolfe and Dutton, 2015; Marco et al., 2021). However, the desired outcomes and final properties of fermented foods do not only depend on the microbial-led conversion of substrates but also on a range of physicochemical parameters such as water activity, temperature, pH, oxidation-reduction potential and substrate accessibility. Therefore, effective management of both the intrinsic and extrinsic parameters during fermentation is critical in achieving the desired characteristics and final properties of fermented food products (Terefe and Augustin, 2020).
Throughout the world, lactic acid fermented products such as yogurts and sourdoughs, acetic acid fermented vinegar or alcoholic beverages produced by lactic acid bacteria, acetic acid bacteria or yeast fermentations, respectively, are widely described and their suggested nutritional and health benefits documented. This has contributed, in part, to consumer interest and the popularity of these fermented food products (Dimidi et al., 2019; Staudacher and Nevin, 2019). However, a relatively less popular yet rich diverse group of fermented products known as alkaline fermented foods (AFFs) which form an essential part of the diets and culture of people in parts of Africa and Asia (Ouoba et al., 2004; Azokpota et al., 2006; Parkouda et al., 2009; Owusu-Kwarteng et al., 2020; Tamang et al., 2020) have received relatively little attention. Figure 1 shows a comparison of studies published on the Scopus database with the terms “lactic acid fermentation” and “alkaline fermentation” in title, abstract or keywords. Active research in the area of “alkaline food fermentation” only began to “take off” in the late 1980s, whereas lactic acid fermentation had benefited from detailed scientific research dated as far back as in 1950. Indeed, pioneering scientific research on African alkaline fermented foods only started in 1980s with the microbiology works on iru (Odunfa, 1981), dawadawa (Campbell-Platt, 1980), ogiri (Odunfa, 1983, 1985), and ugba (Odunfa and Oyewole, 1986).
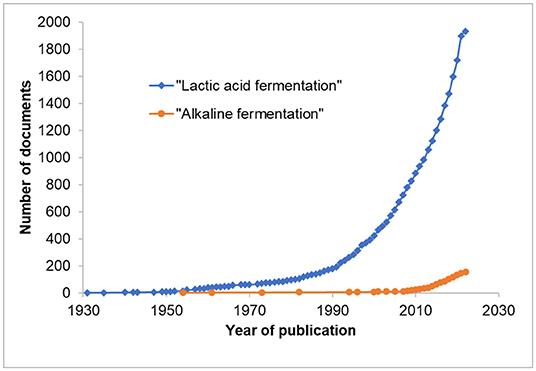
Figure 1. Comparison of cumulative publication outputs in Scopus database on the search terms “lactic acid fermentation” and “alkaline fermentation” (Search done on 23rd February 2022).
These so called “alkaline fermented foods” tends to have their pH increasing during fermentation. Thus, the predominant Bacillus spp. in these alkaline fermentations degrade the endogenous proteins in the raw plant into peptides and amino acids (Odunfa, 1985; Ouoba et al., 2003). As shown in equation 1, the free amino acids are further utilized by the Bacillus spp. as carbon and nitrogen sources to produce ammonia/ammonium hydroxide, resulting in the high pH values and the associated atypical odor of these fermented condiments (Ouoba et al., 2003, 2005; Parkouda et al., 2009; Amoa-Awua et al., 2014).
AFFs of Africa and Asia are produced from a range of protein-rich substrates such as Glycine max (soybeans), Parkia biglobosa (African locust bean) seeds and other non-leguminous plant parts. Plant-based AFFs of Asia are almost exclusively produced from soybean as the substrate, giving credence to the first domestication of soybean in Asia particularly in the eastern half of north China in 1,000 BC, and the importance and agricultural sustainability of soybean across the Asian region (Tamang et al., 2020). In Africa, however, several wild as well as cultivated legumes and non-leguminous plants are used as substrates to produce AFFs. Asian AFFs produced from soybean include natto from Japan (Kanno and Takamatsu, 1987), douchi from China (Fan et al., 2009), kinema and similar products from Nepal and neighboring countries such as Bengal, India, and Bhutan (Tamang et al., 2002), doenjang and chongkukjang from Korea (Park, 2000; Kim, 2002; Park and Jung, 2005; Baek et al., 2008; Park et al., 2010), thua nao from Thailand (Leejeerajumnean, 2000), and meitauza and yandou from China (Zhu et al., 2008; Xu et al., 2012; Qin et al., 2013). On the other hand, African locust bean [Parkia biglobosa (Jacq. Benth)] is a common substrate for AFF condiments in Africa under different local names such as dawadawa in Ghana and Nigeria, soumbala in Burkina Faso (Ouoba et al., 2004), afitin, iru or sonru in Benin (Azokpota et al., 2006), nététou in Senegal (N'Dir et al., 1994), kinda in Sierra Leone and iru among the Yoruba tribe of Southwestern Nigeria (Sanni et al., 2000). Similarly, roselle seeds [Hibiscus sabdariffa (Linn.)] is used to produce AFF condiment such as bikalga in Burkina Faso (Ouoba et al., 2008), yanyanku and ikpiru in Benin (Agbobatinkpo et al., 2013) and mbuja in Cameroon (Mohamadou et al., 2013). Other plant based AFFs in Africa include okpehe from Prosopis africana seeds (Achi, 1992; Oguntoyinbo et al., 2010), otiru from African yam bean (Jeff-Agboola, 2007), oso from Cathormion altissimum seeds (Popoola et al., 2004), ugba from Pentaclethra macrophylla seeds (Sanni et al., 2002; Ahaotu et al., 2013), maari and tayohounta from Baobab seed (Parkouda et al., 2010; Chadare et al., 2011; Kaboré et al., 2012), ogiri from melon/castor oil seeds (Odunfa, 1985; Ademola et al., 2018), owoh from cotton seeds (Gossypium hirsutum) seeds (Sanni and Ogbonna, 1991; Ezekiel et al., 2015), mantchoua and kantong from Kapok tree (Ceiba pentadra) seed (Kpikpi et al., 2014; Kere-Kando et al., 2020) and ntoba mbodi from cassava leaves (Louembe et al., 2003; Mbozo et al., 2017; Moutou-Tchitoula et al., 2018). A range of plant species used for the processing of AFFs in Asia and Africa are shown in Figure 2.
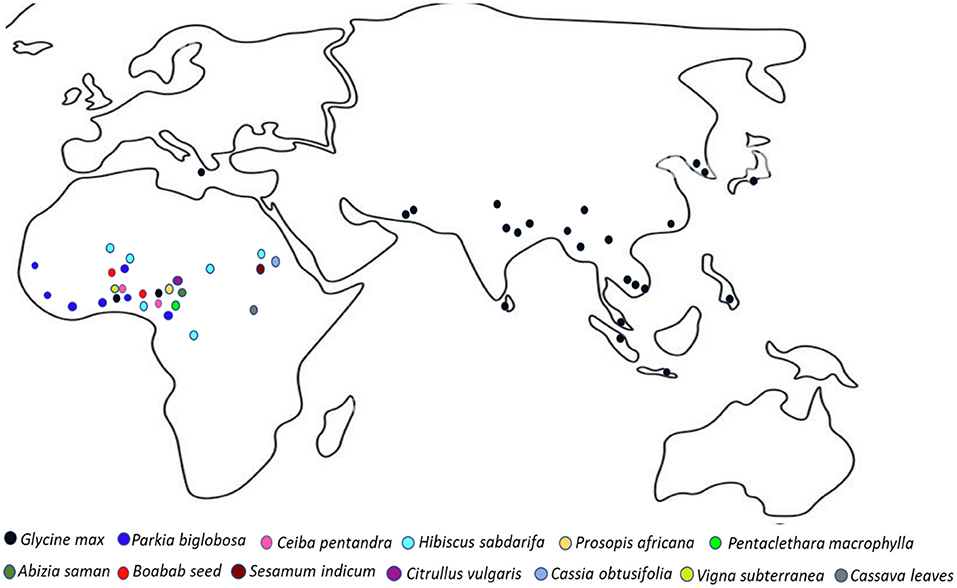
Figure 2. Diversity and distribution of plant species used for the production of alkaline fermented foods in Africa and Asia.
Being predominantly produced from locally cultivated and wild plants, AFFs and the microorganisms therein provide sustainable diets serving as sources of nutrients and health promoting bioactive molecules thereby contributing toward achieving the United Nations Sustainable Development Goals (SGD), specifically SGDs 2 and 3. In addition to the general benefits associated with fermentation such as improvement in shelf-life, organoleptic quality and safety, alkaline food fermentations play a significant role in nutritional intake of several traditional communities in Africa and Asia where these fermentation processes transforms somewhat inedible, unpalatable, potentially toxic and undigestible plant materials into diverse desirable food products that delivers essential nutrients such as proteins, amino acids, vitamins and minerals within otherwise marginal diets (Wang and Fung, 1996; Parkouda et al., 2009; Reddy et al., 2018). Furthermore, AFFs and their associated microorganisms have been associated with several putative health-promoting effects that can positively impact human health via mechanisms such as including nutritive alteration of raw components, biosynthesis of bioactive compounds, modification of the human gut microbiota, and development and modification of the immune system (Shin et al., 2001; Sarkar et al., 2002; Hosoi et al., 2003; Suzuki et al., 2004; Zhang et al., 2006; Wang et al., 2008; Tolhurst et al., 2012; Tamang et al., 2016a; Araki et al., 2020). However, except for natto which has received at least one Randomized Control Trial (RCT), evidence of nutritional and health promoting effects of AFFs or their inherent microorganisms have so far been limited to laboratory chemical analysis and animal/cell model studies in contrast to other fermented foods such as yogurts and cultured milk which have their health benefits reported through at least 20 RTCs for both healthy and patient population groups (Dimidi et al., 2019; Araki et al., 2020; PraŽnikar et al., 2020; Savaiano and Hutkins, 2021). In this review, we demonstrate based on reported scientific information, the potential contribution of plant-based AFFs toward achieving the UN-SDGs as these foods serve as a sustainable source of healthy and nutritious diets for many traditional communities in Africa and Asia. Finally, we present future perspective on the need to apply holistic, meta-omics methods (metagenomics, meta-transcriptomics, meta-proteomics and metabolomics) to characterize traditional AFFs as well as conduct properly designed large placebo-controlled RCTs that will provide a better understanding of the health benefits of traditional AFFs.
Predominant Microorganisms in AFFs
In order to appreciate the scope of AFFs in nutrition and health, it is necessary to acknowledge the diversity and origin of predominant microorganisms responsible for the fermentation of these products. In general, traditional AFFs of Africa and Asia are processed by spontaneous fermentation (depends on autochthonous or resident microorganism present in the raw substrate and/or surrounding environment) to initiate the fermentation processes in these products (Parkouda et al., 2009; Owusu-Kwarteng et al., 2020; Tamang et al., 2020). In assessing their microbial ecology, the gold standard culture dependent phenotypic approaches complemented by molecular tools such as internal transcribed spacer PCR (ITS-PCR), random amplification polymorphic DNA-PCR (RAPD-PCR), repetitive sequence-based PCR (rep-PCR), pulsed field gel electrophoresis (PFGE), as well as sequencing of 16S rRNA, gyrA, gyrB, and rpoB genes, have shown that plant-based AFFs of Africa and Asia are characterized by complex microbial communities. Despite this complexity, Bacillus subtilis and related species are the predominant functional microbiota in a wide-range of plant-based AFFs of Africa and Asia (Ouoba et al., 2004, 2010; Meerak et al., 2007, 2008; Oguntoyinbo et al., 2007; Parkouda et al., 2009, 2010; Chukeatirote et al., 2010; Kamada et al., 2015; Owusu-Kwarteng et al., 2020). Predominant microbial species associated with the fermentation of plant-based AFFs in Asia and Africa are shown in Table 1. Frequently identified species of Bacillus and related genera associated with plant-based AFFs are Bacillus subtilis, Bacillus cereus, Bacillus megaterium, Bacillus endophyticus, Bacillus licheniformis, Bacillus borstelensis, Bacillus pumilus, Bacillus coagulans, Bacillus circulans, Paenibacillus polymyxa, Lysinibacillus sphaericus, and Lysinibacillus fusiformis (Odunfa, 1981; Sarkar et al., 2002; Ouoba et al., 2004, 2010; Azokpota et al., 2007; Parkouda et al., 2009).
Irrespective of raw material or geographic location of production, B. subtilis remains the dominant functional species in most AFFs in Africa and Asia. B. subtilis is reported as the predominant species in the fermentation of dawadawa and soy-daddawa in Ghana and Nigeria (Dakwa et al., 2005; Amoa-Awua et al., 2006; Ezeokoli et al., 2016), soumbala, bikalga, maari and mantchoua in Burkina-Faso (Bengaly, 2001; Ouoba et al., 2004, 2007, 2010; Parkouda et al., 2010; Kaboré et al., 2012; Kere-Kando et al., 2020), kawal in Sudan and Chad (Mbaiguinam et al., 2005), soumbara in Côte d'Ivoire (Adjoumani et al., 2019), afitin, sonru, and iru in Benin (Azokpota et al., 2007), tayohounta, yanyanku, and ikpiru in Benin (Chadare et al., 2011; Agbobatinkpo et al., 2013), mbuja in Cameroon (Mohamadou et al., 2013), kinda in Sierra Leone (Meerak et al., 2008), and okpehe, oso, ugba, iru, and owoh in Nigeria (Sanni et al., 2000, 2002; Popoola et al., 2004; Oguntoyinbo et al., 2010; Adewumi et al., 2013, 2014; Ahaotu et al., 2013; Ezekiel et al., 2015; Ademola et al., 2018). Similarly, Asian AFFs produced from soybean such as kinema produced in Nepal, thua nao in Thailand, chongukukjang and doenjang in Korea, meitauza in China, and natto in Japan are produced by B. subtilis (Tamang et al., 2016b).
The B. subtilis species complex is a tight assemblage of related species that includes B. subtilis subsp. subtilis BEST195, the starter strain used for natto fermentation which is thought to have been isolated from a traditionally prepared natto and was therefore originally named Bacillus natto. However, Bacillus natto was re-classified and included in B. subtilis in 1970 supported by phylogenetic analyses using 16S rRNA genes (Tamang et al., 2002; Kubo et al., 2011). Since its isolation, further characterization including entire genome information (Kiuchi et al., 1987; Sulistyo et al., 1988; Nishito et al., 2010) have led to the development of B. subtilis subsp. subtilis BEST195 starter strain used for natto production in Japan. Natto starter cultivation generally targets genes regulating the metabolic pathways of secondary metabolites that affect texture, aroma, sensitivity to bacteriophages, spore formation and germination, and high productivity of ⋎PGA via the pgs operon (Ashiuchi and Misono, 2002; Beckett, 2009; Kubo et al., 2011) which imparts a sticky texture onto natto. In plant-based African AFFs, B. subtilis play technological and functional roles such as Production of Aroma/flavor compounds (Owens et al., 1997; Ouoba et al., 2005; Nwokeleme and Ugwuanyi, 2015; Akanni et al., 2018), amino acids synthesis (Ouoba et al., 2003; Azokpota et al., 2006), degradation of carbohydrates and oils (Kiers et al., 2000; Ouoba et al., 2003, 2007; Azokpota et al., 2006), production of antimicrobial peptides (Ouoba et al., 2007; Savadogo et al., 2011; Kaboré et al., 2012, 2013; Compaore et al., 2013), and reduction of anti-nutritional factors and toxic compounds (Okafor, 1977; Odunfa, 1985; Abban et al., 2013).
Being produced predominantly by spontaneous fermentation processes, the microbial ecology of traditional plant-based AFFs of Africa and Asia are generally characterized by mixed populations of different species of bacteria, yeasts and molds (Dirar et al., 1985; Sarkar et al., 1994; Dakwa et al., 2005; Jeff-Agboola, 2007; Parkouda et al., 2009, 2010; Sohliya et al., 2009; Ouoba et al., 2010; Kim et al., 2011a; Rashad et al., 2011; Tamang et al., 2012; Adewumi et al., 2013; Owusu-Kwarteng et al., 2020). Thus, in addition to Bacillus and related species, other groups of microorganisms including other aerobic endospore-forming bacteria (AEB), lactic acid bacteria (LAB), yeasts and molds have been frequently identified as members of the microbial consortia of traditional plant-based AFFs. However, the contribution of these so-called “secondary microbiota” to technological and functional properties of AFFs have been sparsely reported. Lactic acid bacterial may enhance the safety of AFFs through the production of antimicrobial compounds (Kaboré et al., 2012). Yeasts such as Candida parapsilosis and Geotrichum candidum have also been shown to significantly lower the levels of free fatty acids, while contributing to the production of free amino acids as well as enhance the formation of flavor compounds in traditional AFFs (Sarkar et al., 1996; Kim et al., 2011b; Rashad et al., 2011).
Nutritional and Dietary Value of Plant-Based AFFs
Plant-based AFFs play significant roles in the nutritional intake and are important in achieving dietary sufficiency in several traditional communities where they are produced and consumed. Two main features of alkaline fermentation process are particularly significant in relation to national and dietary impacts of AFFs. Firstly, enzymes produced by predominant bacteria during alkaline fermentation hydrolyses macronutrient molecules i.e., complex carbohydrates, proteins, and fats, thereby enhancing nutrient bioavailability and digestibility of the fermented product compared with the unfermented substrate (Wang and Fung, 1996). For example, B. subtilis fermentation has been shown to effectively hydrolyse soybean proteins and polysaccharides, resulting in low-molecular-weight, water-soluble products that require little further degradation by gastrointestinal enzymes (Kiers et al., 2000). Secondly, enzymatic degradation during fermentation significantly reduces naturally occurring toxic components, allergens, and antinutritional components in the raw food substrate, thereby transforming otherwise inedible, difficult to digest or potentially toxic raw materials into palatable and culturally desirable food products that deliver essential nutrients. In Africa, several of such alkaline fermented food products are produced in each country or region, thus contributing to a complex rich traditional dietary diversity, with important food security and sustainability implications (Iwuoha and Eke, 1996; Parkouda et al., 2009).
Soybean, the most common plant substrate for AFFs produced in Asia, is nutritious with high levels of protein and fat (Bouchenak and Lamri-Senhadji, 2013). However, unprocessed soybean contains high levels of complex oligosaccharides and antinutrients (Reddy and Pierson, 1994), as well as potential allergens (Phromraksa et al., 2008). The presence of antinutritional factors such as tannins, trypsin inhibitors and phytic acid tend to limit the digestibility and bioavailability of essential nutrients in unprocessed soy products (Ghavidel and Prakash, 2007). Therefore, in order to liberate the essential nutrients from soybean for human digestion and absorption, some form of processing is necessary. Fermentation has proven to reduce antinutrient levels, improving the nutritional and organoleptic properties of fermented food products (Osman, 2004; Eltayeb et al., 2007; Atuna et al., 2022).
During the production of soy-based natto (Figure 3A), biochemical changes occurring as a result of fermentation has been shown to improve the content of protein, lipids and minerals such as iron, zinc and calcium (Table 2). Hu et al. (2010) reported 1.1-fold increase in protein content of natto after fermentation. It has been suggested that microorganisms increase the protein content of samples on which they grow due to microbial synthesis of protein (Hu et al., 2010). Crude fat and total ash contents of natto ranges from 18–24.7 to 4.7–4.6%, respectively (Table 2). Although fermentation is reported to have little effect on the crude fat content of natto, there appears to be strain-specific influence by the fermenting microorganism which may result in increased or decreased fat content. For example, the lipid content of natto decreased by 1.1-times from 25.41% in raw soybeans inoculated with the “Itobiki” strain of B. natto while the NRRL B-3383 strain of B. natto increased lipid content by 1.1-fold (Wei and Chang, 2004). Thus, different B. natto strains have different capabilities to synthesize lipid or utilize carbohydrates during fermentation (Wei and Chang, 2004). Another notable compositional change in natto is the significant increase in vitamin K content after fermentation. The extraordinary increase in vitamin K in natto has been attributed to vitamin K2 (menaquinone-7 or MK-7) synthesis by B. subtilis natto (Kouris-Blazos and Belski, 2016). Epidemiological studies have also shown that increased consumption of Japanese natto is positively correlated with bone density among Japanese females as a result of increases in serum vitamin K and ⋎-carboxylated osteocalcin, both of which facilitate bone mineralization (Tsukamoto et al., 2000; Katsuyama et al., 2002). For water-soluble vitamins, fermentation was reported to increase thiamine and riboflavin contents by 3-folds and a 5-fold increase in vitamin B12 content compared to the raw substrate (Reddy et al., 1983).
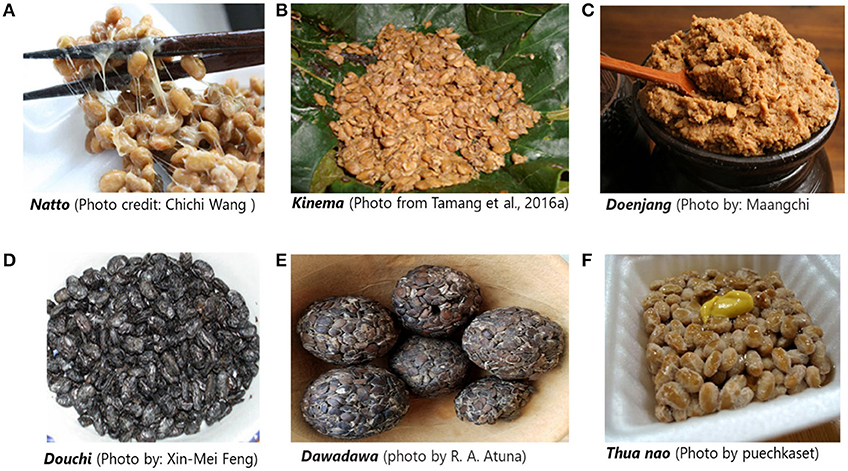
Figure 3. Examples of traditional AFFs in Africa and Asia. (A) Natto (Photograph credit: Chichi Wang), (B) Kinema (Photograph from Tamang et al., 2016a), (C) Doenjang (Photograph by Maangchi), (D) Douchi (Photograph by Xin-Mei Fang), (E) Dawadawa (Photograph by R. A. Atuna), and (F) Thua nao (Photograph by puechkaset).
Kinema (Figure 3B), a fermented-soy condiment, is a traditional delicacy produced in Nepal and parts of India. Kinema not only possesses exceptional flavor but has a significant dietary importance as a relatively cheap substitute for meat (Sarkar et al., 1994). Like other soy-based fermented products, kinema is a rich source of protein (43.4–48.7%), fat (16.1–22.7%), carbohydrates (27.4–29.6%), and minerals (Table 1). The nutrient composition of kinema has previously been reported (Sarkar and Tamang, 1995; Sarkar et al., 1997b, 1998). Total protein content of kinema increases by about 1% while free amino acid content increases by 60-fold compared to raw soybeans. However, for its dietary role as meat substitute, tryptophan, cysteine, and methionine are the main limiting amino acids in kinema, although protein quality has been reported to be comparable to animal source protein (Sarkar et al., 1994, 1997b). B-vitamins in kinema varies from the raw soybean substrate with general increases in levels of riboflavin and niacin, but a decrease in thiamine (Sarkar et al., 1998). The minerals content of kinema was also reported to be lower than raw soybean. The decline in the mineral level in kinema has been attributed to the preparation process where soybeans are soaked, cooked, and the water discarded (Sarkar et al., 1998).
Doenjang (Figure 3C) is an indigenous alkaline fermented soybean paste which serves as an important protein source and seasoning agent in the traditional diets of the people of Korea. Doenjang is considered a nutritious food that provides essential amino acids, fatty acids, minerals, and vitamins, associated with various nutritional benefits. Proximate composition of doenjang include 54.7% water, 13.8% crude protein, 8.0% crude lipid, 14.4% (Park and Jung, 2005). Amino acids composition of doenjang include glutamic acid, leucine, alanine, histidine, lysine, proline, and valine (Park and Jung, 2005). Fermentation of doenjang for 80 days results in relative increases in methionine and threonine levels compared to raw soybeans. However, these amino acids are still limiting in doenjang and therefore other dietary strategies such as complementation would ensure adequate intake of essential amino acids (Namgung et al., 2010). Like doenjang, chongkukjang is another traditional Korean soy-based fermented food, similar to Japanese natto, but different in usage (Chukeatirote, 2015). Chongkukjang, commonly consumed for its perceived health-promoting properties, is also regarded as a rich source of proteins, lipids, amino acids, vitamins, and minerals (Ali et al., 2018). Fermentation of soybean into chongkukjang with higher concentrations (5–7%) of B. subtilis enhances the minerals content of the product (Ali et al., 2018). The significant improvement of minerals content has been attributed to microbial involvement and interaction with metabolites breakdown (Ali et al., 2018). Fermentation is also reported to degrade antinutritional factors during chongkukjang processing, invariably improving the bioavailability of minerals (Ali et al., 2018). The microorganisms' strains in the fermentation process significantly influence the product's value. The total mineral contents in chongkukjang fermented with Bacillus amyloliquefaciens RWL-1 increased by about 33% compared with the quantity obtained from the conventional B. subtilis (Shahzad et al., 2020).
Douchi (Figure 3D), a nutrient-rich fermented soy-based food product, is widely used seasoning in China and other Asian countries to enhance appetite (Li and Ma, 2014). During douchi production, a series of complex biochemical reactions enhance chemical and nutritional components in the raw soybeans. During fermentation, proteins are converted to peptides and amino acids, lipid to fatty acids, starch to reducing-sugars and ethanol, and various aroma components by salt-tolerant microorganisms (Li and Ma, 2014). The crude protein and fat content of douchi ranges from 35.9–40.4 to 19.9–23.9%, respectively (Table 1). The crude protein content of pre-fermented douchi slightly increased and then decreased by 1.2-fold after four (4) weeks of ripening (Wang et al., 2016). The fermentation process is also reported to increase the product's mineral content and bio-accessibility (Liu et al., 2020).
Meitauza is produced from okara (a by-product from the production of soybean foods such as soymilk and tofu) in China. Crude protein content of meitauza declines by almost 6% due to the interaction between microbes and the fermenting media. However, total free amino acids significantly increased during the fermentation process (Xu et al., 2012). Strong-tasting amino acids such as glutamic acid and glycine increase by over 10-folds; and aspartic acid, alanine and arginine increased several-fold. Similarly, amino acid with sweet taste: leucine, valine, serine, and methionine significantly increase during fermentation, improving the taste and overall nutritional value of the fermented product. Although okara contains high moisture content (>80%), the water is linked to dietary fiber, resulting in the pasty texture of the by-product (Redondo-Cuenca et al., 2008). The fiber, largely insoluble cellulose and hemicellulose, form a more significant part of the dry matter content (40–60%). Fermentation can increase the soluble fiber level in okara, improving its nutritional quality and processibility (Sharma et al., 2020). It also induces a significant decrease in lipid and lignin contents in okara. These compositional changes have led to a pleasant and delicate taste, as well as a smooth and rubbery texture (Colletti et al., 2020).
Dawadawa (Figure 3E) is the most important protein-rich food condiment in West and Central African, primarily used as a meat substitute or to enhance the organoleptic qualities of traditional staple foods. Dawadawa is known by different local names depending on the country of production; dawadawa in Ghana, iru (Nigeria), nététu (Senegal), kinda (Sierra Leone), afitin, iru, or sonru (Benin) and soumbala (Burkina Faso). Varying nutritional composition have been reported for dawadawa because a range of raw materials including locust bean, bambara groundnuts [Vigna subterranean (L.) Verdc.], and soybean serve as substrates for the fermentation (Achi, 2005). In general, locust bean is inedible and could be toxic to humans (Wang and Fung, 1996). However, a significant reduction in the anti-nutrient content and enhanced amino acid profile has been reported for alkaline fermented locust bean (Ijarotimi and Keshinro, 2012). Generally, dawadawa produced from African locust beans contain about 49.7% protein, 26.9% lipids, 4.3% total ash, and 7.4% carbohydrates (Appiah et al., 2012). Alkaline fermentation of African locust bean resulted in an increase in total protein content and an improvement in the amino acid profile (Urua et al., 2013). This increase in free amino acids levels due to the proteolytic activities by the fermentative microorganisms have been reported for dawadawa (Odunfa, 1981; Akabanda et al., 2018). Additionally, fermentation of locust bean results in a 6-fold increase in thiamine and 3-fold increase in riboflavin contents, but a reduction (30%) in niacin content. Similar reduction in niacin content was reported for ugba, fermented condiment from African oil bean seeds, corresponding to progressive reduction in cyanide, phytate, tannin and oxalate contents (Ogueke et al., 2013). Sulfur-containing amino acids are generally limiting in locust bean dawadawa (Campbell-Platt, 1980) and ogiri produced by alkaline fermentation of melon seeds, but these could be complemented through the consuming the AFFs with cereal-based staples (Wang and Fung, 1996). For soy-dawadawa, fermentation protein, carbohydrates and fat contents are reported to be 43.2–47.4, 16.2, and 19.9–26.7%, respectively (Table 2). The major biological changes occurring during soy-dawadawa processing include hydrolyses of protein, fat, and carbohydrate, with the fermentation leading to increased contents of protein and amino acids such as lysine, arginine, proline, phenylalanine, isoleucine, and leucine (Dakwa et al., 2005; Terlabie et al., 2006).
In Congo and parts of central Africa, cassava (Manihot esculenta Crantz) leaves are fermented to produce ntoba mbodi (Louembe et al., 2003). Ntoba mbodi is relatively rich in protein although a slight reduction in free nitrogen occurs during fermentation, probably due to liberation of hydrogen cyanide. Extensive cellulase activity, through fermentation, further enhances the bioavailability of carbohydrates and increases magnesium, calcium, and potassium contents in notba nbodi compared to raw cassava leaves. However, methionine and phenylalanine are the limiting amino acids in ntoba nbodi (Mokemiabeka et al., 2011). Another product similar to ntoba nbodi is kawal produced by the fermentation of sickle pod [Senna obttusifolia (L.) H.S/Irwin & Barneby] leaves in Chad and Sudan where it serves as meat substitute and flavoring agent soups and stews (Dirar, 1984; Harper and Collins, 1992). While Dirar (1984) reported a decrease in crude protein content of after fermentation, Harper and Collins (1992) reported increased crude protein content of kawal. Although about 50% protein loss during the fermentation of kawal has been observed, the loss is overshadowed by the substantial increase in digestibility and palatability of the fermented product. Limiting amino acids in kawal include cysteine, histidine, and threonine. However, kawal is consumed with cereals foods which may provide complementary proteins (Mbaiguinam et al., 2005).
Health Promoting Effects of AFFs
Production of Vitamins
All vitamins are essential for human growth—meaning human cannot synthesize adequate quantities of vitamins de novo, if at all. Therefore, human diet must contain adequate quantities of these vitamins in order to maintain normal growth and bodily functions. These vitamins act as co-factors or pre-cursors to important metabolic processes and enzymes in living organisms. Fermentation is touted as a natural process for the in-situ biosynthesis of vitamins in food materials (Odunfa, 1986). As shown in Table 3, examples of vitamins whose levels have been significantly increased after fermentation are Vitamin K and the B-vitamins (e.g., thiamine (B1), riboflavin (B2), niacin (B3), pantothenic acid (B5), pyridoxal (B6), biotin (B7), folate (B9), and cobalamins (B12).
The biosynthetic pathways for the production of vitamins B2 (Burgess et al., 2009), B12 (Burgess et al., 2009), B9 (Rossi et al., 2011), and other B-type vitamins have been widely reported in lactic acid bacteria (LeBlanc et al., 2011). Among alkaline-fermenting bacterial strains, B. subtilis (Bacher et al., 1980; Bretzel et al., 1999; Li et al., 2013; Man et al., 2014), B. tequilensis (Abdulla et al., 2016), B. abortus (García-Angulo, 2017), B. amyloliquefaciens (Vitreschak et al., 2002), and B. halodurans (Averianova et al., 2020) are the most studied species.
Most food-grade microorganisms, including those found in alkaline fermented foods have the genetic wherewithal to synthesize vitamins during growth on organic materials. The class of genes needed for the biosynthetic of these vitamins differ for each vitamin but can be intricately linked, as is the case for vitamin B2, B9, and B12 (Gu and Li, 2016). In B. subtilis, the production of Vitamin B2 begins with the expression of ribA gene which encodes for the bifunctional enzyme guanosine 5′-triphosphate (GTP) cyclohydrolase II/3,4-dihydroxy-2-butanone 4-phosphate synthase responsible for the conversion of GTP to 5-amino-6-ribityl-amino-2,4(1H,3H) pyrimidinedione (ArP), and ribulose 5-phosphate (Ribu5P) to 3,4-dihydroxy-2-butanone-4-phosphate (DHBP). ArP and DHBP are the two substrates of riboflavin (Vitamin B2) (Averianova et al., 2020). Plant-based food products whose riboflavin levels have been significantly improved via alkaline fermentation include dawadawa (Oguntoyinbo et al., 2016), kinema (Sarkar et al., 1998), and tempe (Mani and Ming, 2017).
The production of folate also begins with GTP which acts as a precursor for 6-hydroxymethyl-7,8-dihydropterin pyrophosphate (DHPPP) through a series of four enzymatic conversion steps. Another important substrate for folate production is para-aminobenzoic acid (pABA) which is produced from erythrose 4-phosphate and phosphoenolpyruvate through the shikimate pathway (Bermingham and Derrick, 2002). The coupling together of DHPP and pABA, and subsequent glutamylation of the final product gives polyglutamate forms of dihydrofolate (DHF), and tetrahydrofolate (THF) (Rossi et al., 2011).
Cobalamin (Vitamin B12) has an intricate and complex structure. It is no wonder that as many as 30 genes are required for its biosynthesis (Gu and Li, 2016). One of the few Bacillus species known to synthesis Vitamin B12 de novo is B. megaterium where the synthesis occurs through an anaerobic route (Fang et al., 2017). B. megaterium is considered a producer of Vitamin 12 at the industrial scale (Vary, 1994; Mohammed et al., 2014). In this species, uroporphyrinogen III is first converted to precorrin-2 and then subsequently to cobyric acid. The transformation of precorrin-2 to cobyric acid occurs through a series of reactions involving eight methylations, six amidations, insertions of cobalt, and decarboxylation and contraction of the porphyrin ring (Moore and Warren, 2012). Cobyric acid is converted to cobinamide, and then to cobalamin.
Kwak et al. (2008) reported the Vitamin B12 contents of traditionally fermented Doenjang and Chungkookjang, two Korean soybean-based fermented foods, and found levels of 1.85 mg/100 g and 0.69 mg/100 g, respectively. These levels were significantly higher than those found in factory-made versions of the products which had 0.04–0.86 mg/100 g and 0.06–0.15 mg/100 g, respectively. The high levels of Vitamin B12 in the traditional fermentation process could be due to the fact that the traditional process to prepare Doenjang and Chungkookjang is relatively long (~ about 10 months) and uses “wild-type” multiple microorganisms found in nature (Kwak et al., 2010).
Vitamin B12 production to levels of about 91.43 mg/100 dw in in fermented soybean, Thua nao (Figure 3F) using mixed culture fermentation using mixed cultures of B. amyloliquefaciens. and Klebsiella spp. have been reported (Yongsmith et al., 2016). The levels of Vitamin B12 in tempeh (i.e., 0.7–8.0 mg/100 g), (Watanabe et al., 2014). The levels of Vitamin B12 in natto has been reported to be over five times that in cooked soybeans (Wang and Fung, 1996).
The fact that the aforementioned vitamins are abundant in alkaline fermented plant-based foods suggest that fermentation can be considered as suitable chemical-free production route for these vitamins, for use in supplements and food fortification programs (Gu and Li, 2016). Further, in some cases (such as in riboflavin synthesis) production of these vitamins via fermentation leads to products with higher chemical purity, compared with chemically synthesized alternatives (Bretzel et al., 1999).
Improved Digestibility
In general, plant-based foods are comparatively less digestible than animal-based foods, when subjected to the human digestive system. The low digestibility of plant materials is due to factors such as the presence of cellulose cell wall that decrease access by enzymes to target molecules (Holland et al., 2020), the inability of human gastro intestinal enzymes to digest cellulose (Cummings, 1984), restrictive structural features of target molecules (e.g., hydrophobicity and folding patterns of plant proteins), presence of “antinutritive” compounds or secondary metabolites that inhibit human gastric enzyme activities (Sarwar Gilani et al., 2012), complexation of target molecules with other compound (e.g., starch-lipid conjugates) (Qin et al., 2019), among others. All of these aforementioned phenomena decrease the digestion, bioaccessibility, and bioavailability of nutrients in plant-based foods. The ability of alkaline fermentation to improve the digestibility of plant foods therefore strongly depends largely on microbial metabolic processes that affects one or more of the above-mentioned factors. The description (with examples) of two pathways through which fermentation increases the digestibility of certain alkaline-fermented foods is given below.
Partial Hydrolysis and Release of Hydrolytic Products and Their Metabolites
The action of alkaline fermentation leads to the generation of a host of factors that directly or indirectly causes partial hydrolysis or softening of plant matrix. For example, microbial enzymes produced in situ during fermentation can utilize biomolecules in plant matrices as substrates, leading to the generation of partially-hydrolysed plant microstructures and release of hydrolysis products. Case in point, proteolysis is considered the most important metabolic activity in alkaline fermentation (Parkouda et al., 2009), and increased protease activity during alkaline fermentation is well-reported (Ouoba et al., 2003; Owusu-Kwarteng et al., 2020). Proteolysis is mostly caused by Bacillus spp. (Ouoba et al., 2003), and this biochemical process leading to the formation of peptides and free amino acids that improve the nutritional qualities of the fermented product. The levels of essential amino acids such as methionine, cysteine, leucine, tyrosine, phenylalanine, and lysine have been reported to increase after alkaline fermentation (Ouoba et al., 2003; Owusu-Kwarteng et al., 2020). The released free amino acids are further metabolized by the microorganisms to ammonia, giving rise to the characteristic pungent “ammonia-like” odors of some traditional fermented foods such as dawadawa (Parkouda et al., 2009). High pH is known to degrade cellulose and lignocellulose (Glaus and Van Loon, 2008). Therefore, the production of ammonia and accompanying high pH during alkaline fermentation can be expected to indirectly “soften” plant compounds, making them more susceptible to the actions of subsequent food processing and gastrointestinal enzymes.
Reduction in Levels of Antinutritional Factors and Flatus-Forming Oligosaccharides
Plant materials are rich in antinutritional factors such as lectins, tannins, saponins, protease inhibitors, oxalic acid, cyanogenic glycosides, and phytic acid. These antinutritional factors can reduce the bioavailability of key nutrients (such as vitamins and minerals), or interfere with the activities of gastro-intestinal digestion. Some antinutritional compounds (e.g., hydrocyanic acid released from cyanogenic glycosides) can be toxic to humans.
The seeds of African locust bean (Parkia biglobosa), used in the preparation of dawadawa contains antinutrients such as nitrates, tannins, cyanide, phytates, and oxalates (Termote et al., 2022). Urua et al. (2013), in their work on the effect of processing on three accessions of African locust bean reported that fermentation reduced the levels of phytic acid and oxalates respectively by up to 44.4 and 50.9% each; whereas boiling reduced the levels of these antinutrient by up to 33.3 and 4.4%, respectively. The oxalate contents in two of the accessions actually increased by 140.8 and 78.1%, respectively (Urua et al., 2013). In another study, trypsin inhibitor activity, tannins, and phytic acids in African locust bean seed were reduced significantly by 89.0, 59.8, and 62.5%, respectively after 72 h of alkaline fermentation (Esenwah and Ikenebomeh, 2008). In other reports, alkaline fermentation of soy beans into kinema has been shown to reduce the levels of tannins, phytic acids, trypsin inhibitor activity, and haemagglutinating activity by 100, 61, 71, and 100%, respectively (Sharma et al., 2015). Kobawila et al. (2005) have demonstrated that the cyanogenic glycoside content reduced significantly by 70–75% during alkaline fermentation of cassava leaves (Kobawila et al., 2005). These examples demonstrate that alkaline fermentation degrades antinutritional compounds, thereby reducing or eliminating them from the fermented food.
Improved Health Benefits via the Actions of Probiotics and Post-biotics
Many of the Bacillus strains predominant in alkaline fermented foods are also used commercially as probiotics. Some of these strains include B. cereus, B. clausii, B. coagulans, B. licheniformis, B. polyfermenticus, B. pumilus, and B. subtilis—all of which exhibit probiotic activities in both spores and vegetative forms (Lee et al., 2019). Compared to probiotic lactic acid bacteria, Bacillus probiotic strains have better survival rates and stability to processing conditions such heat, cold, moisture, dehydration, as well as gastric conditions (Lee et al., 2019). This feature of Bacillus probiotics is largely due to their ability to form spores (Nicholson et al., 2000). In fact, the ability of Bacillus spores to germinate and grow in the gastro-intestinal tract has been reported (Hoa et al., 2000). This means that fermented pant-based foods could be used as carriers for the delivery of Bacillus probiotics into the mammalian digestive systems.
The influence of probiotics on human gut microbiome is well-reported (Thursby and Juge, 2017; Kawai et al., 2018), but the focus of has mostly been on lactic acid bacteria probiotics. Whereas, the impact of individual Bacillus strains on gut microbiome of some animal model (e.g., chicken and pigs) have been studied (Poulsen et al., 2018; Jacquier et al., 2019), little is known on how specific Bacillus strains influence the human gut microbiome. It is however known that, as probiotics, Bacillus strains stimulate antimicrobial, anticancer, antihypertensive, antioxidant, fibrinolytic, and immunomodulating activities in vitro and in vivo (Lee et al., 2019). These biological properties can be stimulated by the metabolic process of probiotics and post-biotics in alkaline fermenting foods.
Post-biotics are the bioactive soluble by-products released following either through the metabolic processes of probiotics or through lysis of probiotics (Wegh et al., 2019). A technical definition of post-biotics, as proposed by The International Scientific Association of Probiotics and Pre-biotics (ISAPP) is “preparation of inanimate microorganisms and/or their components that confers a health benefit on the host” (Salminen et al., 2021).
There is a growing number of studies reporting the health properties of post-biotics (Wegh et al., 2019; Zółkiewicz et al., 2020; Salminen et al., 2021) but these studies do not discriminate the source of these post-biotics, i.e., whether from acid or alkaline fermentation. However, examples of post-biotics that can be expected to be produced following alkaline fermentation of plant-based foods are soluble proteins, protein hydrolysates, bacteriocins, free amino acids, vitamins, soluble dietary fiber, exopolysaccharides, non-viable cells, and cell fragments. As presented earlier (see Sections Nutritional and Dietary Value of Plant-Based AFFs), alkaline fermentation leads to the production of several probiotics-derived biomolecules, many of which can be expected to have bioactive properties. Some of the mechanisms through which post-biotics mediate health in hosts includes mediating growth and metabolic activities of gut microbiota, modulating systemic metabolism, mediating signaling in nervous system, enhancing epithelial barrier function, modulating immune responses, triggering bioactive properties such as anti-adhesion, anti-biofilm, antidiabetic, antiviral, immunomodulatory, antihypertensive, hypocholesterolemic, and antioxidant effects (Tangyu et al., 2019; Wegh et al., 2019; Nataraj et al., 2020; Zółkiewicz et al., 2020; Salminen et al., 2021). A schematic of the nutrition and health impact of alkaline fermentation on plant-based foods is shown in Figure 4.
Conclusion and Future Outlook
As shown in the preceding discussion, alkaline fermentation of plant-based foods offers several sensorial, nutritional, and health benefits. For example, guided selection of starter cultures and probiotic strains could be used to stimulate the in situ biofortification of plant-based foods through alkaline fermentation as a strategy to prevent deficiencies in nutrients such as vitamins, minerals, and proteins. However, as demonstrated in this review, the scientific underpinnings of the microbial metabolic factors and health-promoting effects of AFFs are not as well-studied as other fermented foods produced via lactic acid fermentation. For example, a careful search of the literature shows that only a few Bacillus species have been studied for their vitamin production potential, with B. subtilis being the most studied. Even then, most of the fundamental scientific studies on vitamin-producing alkaline fermenters were performed at laboratory scale using genetically modified strains, and in chemically defined media. The production of vitamins by alkaline fermenters in situ in plant-based foods needs more investigation.
Moreover, perspectives for improving the traditional production processes of AFFs to ensure consistency in safety and quality should be researched. Research is needed to unravel the genome sequences of microorganisms in AFFs. This will help with proper identification and taxonomic classification of species responsible for the generation of desired properties. Genomic sequences of microbiota in AFFs will also allow the selection of genes that can be targeted and altered by metabolic engineering strategies, to improve desired traits such as the production of post-biotics (e.g., enzymes, vitamins, bacteriocins, and exopolysaccharides), or to isolate and exclude genes responsible for the production of biogenic amines and antibiotic resistance genes. Another important area that needs attention is the generation of clinical evidence on how the composite microbial species in AFFs alter human gut microbiome. For reasons such as relatively low cost of production and the aforementioned nutritional and health promoting benefits, AFFs are expected to play a huge role in meeting the food, dietary, and health requirements of consumers, going forward.
Author Contributions
JO-K designed the manuscript. JO-K and DA critically revised and corrected the manuscript. JO-K, DA, FA, RA, and FKA wrote the manuscript. All authors contributed to the article and approved the submitted version.
Conflict of Interest
The authors declare that the research was conducted in the absence of any commercial or financial relationships that could be construed as a potential conflict of interest.
Publisher's Note
All claims expressed in this article are solely those of the authors and do not necessarily represent those of their affiliated organizations, or those of the publisher, the editors and the reviewers. Any product that may be evaluated in this article, or claim that may be made by its manufacturer, is not guaranteed or endorsed by the publisher.
References
Abban, S., Brimer, L., Abdelgadir, W. S., Jakobsen, M., and Thorsen, L. (2013). Screening for Bacillus subtilis group isolates that degrade cyanogens at pH 4.5-5.0. Int. J. Food Microbiol. 161, 31–35. doi: 10.1016/j.ijfoodmicro.2012.11.019
Abdulla, M., Khalil Bagy, M., Nafady, N. A., Morsy, F. M., and Mahmoud, G. (2016). Activation of riboflavin production by Bacillus subtilis (KU559874) and Bacillus tequilensis (KU559876). EC Bacteriol Virol Res. 2, 131–150.
Achi, O. K. (1992). Microorganisms associated with natural fermentation of Prosopis africana seeds for the production of okpiye. Plant Foods Hum. Nutr. 42, 297–304. doi: 10.1007/BF02194090
Achi, O. K. (2005). Traditional fermented protein condiments in Nigeria. Afr. J. Biotechnol. 4, 1612–1621.
Ademola, O., Adeyemi, T., Ezeokoli, O., Ayeni, K., Obadina, A., Somorin, Y., et al. (2018). Phylogenetic analyses of bacteria associated with the processing of iru and ogiri condiments. Lett. Appl. Microbiol. 67, 354–362. doi: 10.1111/lam.13040
Adewumi, G. A., Oguntoyinbo, F. A., Keisam, S., and Jeyaram, K. (2013). Combination of culture-independent and culture-dependent molecular methods for the determination of bacterial community of iru, a fermented Parkia biglobosa seeds. Front. Microbiol. 3, 436. doi: 10.3389/fmicb.2012.00436
Adewumi, G. A., Oguntoyinbo, F. A., Romi, W., Singh, T. A., and Jeyaram, K. (2014). Genome subtyping of autochthonous Bacillus species isolated from Iru, a fermented Parkia biglobosa seed. Food Biotechnol. 28, 250–268. doi: 10.1080/08905436.2014.931866
Adjoumani, K. Y., Ouattara, G. H., Germain, K. T., and Zeze, G. K. (2019) Molecular identification species diversity of the microbiota associated with soumbara, a traditional fermented food commonly consumed in Côte d'Ivoire. Res. J. Food Sci. Nutr. 4, 48–57. doi: 10.31248/RJFSN2019.068
Agbobatinkpo, P. B., Thorsen, L., Nielsen, D. S., Azokpota, P., Akissoe, N., Hounhouigan, J. D., et al. (2013). Biodiversity of aerobic endospore-forming bacterial species occurring in Yanyanku and Ikpiru, fermented seeds of Hibiscus sabdariffa used to produce food condiments in Benin. Int. J. Food Microbiol. 163, 231–238. doi: 10.1016/j.ijfoodmicro.2013.02.008
Agyei, D., Owusu-Kwarteng, J., Akabanda, F., and Akomea-Frempong, S. (2020). Indigenous African fermented dairy products: processing technology, microbiology and health benefits. Crit. Rev. Food Sci. Nutr. 60, 991–1006. doi: 10.1080/10408398.2018.1555133
Ahaotu, I., Anyogu, A., Njoku, O., Odu, N., Sutherland, J., and Ouoba, L. (2013). Molecular identification and safety of Bacillus species involved in the fermentation of African oil beans (Pentaclethra macrophylla Benth) for production of Ugba. Int. J. Food Microbiol. 162, 95–104. doi: 10.1016/j.ijfoodmicro.2013.01.001
Ahn, B.-Y. (2018). Optimization of vitamin D 2 production using enoki mushroom powder and the preparation of Doenjang using the powder. J. East Asian Soc. Diet. Life 28, 88–100. doi: 10.17495/easdl.2018.2.28.1.88
Akabanda, F., Owusu-Kwarteng, J., Tano-Debrah, K., Glover, R. L. K., Nielsen, D. S., and Jespersen, L. (2013). Taxonomic and molecular characterization of lactic acid bacteria and yeasts in nunu, a Ghanaian fermented milk product. Food Microbiol. 34, 277–283. doi: 10.1016/j.fm.2012.09.025
Akabanda, F., Parkouda, C., Suurbaar, J., Donkor, A., and Owusu-Kwarteng, J. (2018). Effects of mechanical dehulling on microbiological characteristics and chemical changes during processing of Parkia biglobosa seeds into dawadawa, a West African alkaline fermented condiment. J. Ghana Sci. Assoc. 17, 13–19.
Akanni, G. B., de Kock, H. L., Naude, Y., and Buys, E. M. (2018). Volatile compounds produced by Bacillus species alkaline fermentation of bambara groundnut (Vigna subterranean (L. Verdc) into a dawadawa-type African food condiment using headspace solid-phase microextraction and GCxGC-TOFMS. Int. J. Food. Prop. 21, 930–42. doi: 10.1080/10942912.2018.1460757
Ali, M. W., Shahzad, R., Bilal, S., Adhikari, B., Kim, I.-D., Lee, J.-D., et al. (2018). Comparison of antioxidants potential, metabolites, and nutritional profiles of Korean fermented soybean (Cheonggukjang) with Bacillus subtilis KCTC 13241. J. Food Sci. Technol. 55, 2871–2880. doi: 10.1007/s13197-018-3202-2
Amadi, E., Barimalaa, I., and Omosigho, J. (1999). Influence of temperature on the fermentation of bambara groundnut (Vigna subterranea) to produce a dawadawa-type product. Plant Foods Hum. Nutr. 54, 13–20. doi: 10.1023/A:1008003118374
Amoa-Awua, W. K., Awusi, B., Owusu, M., Appiah, V., Ofori, H., Thorsen, L., et al. (2014). Reducing the atypical odour of dawadawa: Effect of modification of fermentation conditions and post-fermentation treatment on the development of the atypical odour of dawadawa. Food Control 42, 335–342. doi: 10.1016/j.foodcont.2014.02.016
Amoa-Awua, W. K., Terlabie, N. N., and Sakyi-Dawson, E. (2006). Screening of 42 Bacillus isolates for ability to ferment soybeans into dawadawa. Int. J. Food Microbiol. 106, 343–347. doi: 10.1016/j.ijfoodmicro.2005.08.016
Appiah, F., Oduro, I., Ellis, W., and Adu, G. (2012). Comparative assessment of the performance of Parkia biglobosa, Glycine max and Treculia africana in the production of a local condiment (dawadawa) in Ghana. Afr. J. Food Sci. 6, 111–116. doi: 10.5897/AJFS12.007
Araki, R., Fujie, K., Yuine, N., Watabe, Y., Maruo, K., Suzuki, H., et al. (2020). The possibility of suppression of increased postprandial blood glucose levels by gamma-polyglutamic acid-rich natto in the early phase after eating: a randomized crossover pilot study. Nutrients 12, 915. doi: 10.3390/nu12040915
Ashiuchi, M., and Misono, H. (2002). Biochemistry and molecular genetics of poly-γ-glutamate synthesis. Appl. Microbiol. Biotechnol. 59, 9–14. doi: 10.1007/s00253-002-0984-x
Atuna, R. A., Ametei, P. N., Bawa, A.-A., and Amagloh, F. K. (2022). Traditional processing methods reduced phytate in cereal flour, improved nutritional, functional and rheological properties. Sci. Afr. 15, e01063. doi: 10.1016/j.sciaf.2021.e01063
Averianova, L. A., Balabanova, L. A., Son, O. M., Podvolotskaya, A. B., and Tekutyeva, L. A. (2020). Production of vitamin B2 (Riboflavin) by microorganisms: an overview [review]. Front. Bioeng. Biotechnol. 8, 570828. doi: 10.3389/fbioe.2020.570828
Azokpota, P., Hounhouigan, D., and Nago, M. (2006). Microbiological and chemical changes during the fermentation of African locust bean (Parkia biglobosa) to produce afitin, iru and sonru, three traditional condiments produced in Benin. Int. J. Food Microbiol. 107, 304–309. doi: 10.1016/j.ijfoodmicro.2005.10.026
Azokpota, P., Moller, P., Houghouigan, J., and Jakobson, M. (2007). Biodiversity of predominant Bacillus isolated from afitin, iru and sonru at different fermentation time. Int. J. Biol. Chem. Sci. 1, 211–222. doi: 10.4314/ijbcs.v1i3.39716
Bacher, A., Baur, R., Eggers, U., Harders, H. D., Otto, M. K., and Schnepple, H. (1980). Riboflavin synthases of Bacillus subtilis. Purification and properties. J. Biol. Chem. 255, 632–637. doi: 10.1016/S0021-9258(19)86223-3
Baek, L.-M., Park, L.-Y., Park, K.-S., and Lee, S.-H. (2008). Effect of starter cultures on the fermentative. Kor. J. Food Sci. Technol. 40, 400–405.
Balogun, M. A., and Oyeyiola, G. P. (2011). Microbiological and chemical changes during the production of okpehe from Prosopis africana seeds. J. Asian Sci. Res. 1, 390–398. Available online at: https://archive.aessweb.com/index.php/5003/article/view/3310
Barimalaa, I. S., Achinewhu, S. C., Yibatama, I., and Amadi, E. N. (1994). Studies on the solid substrate fermentation of bambara groundnut (Vigna subterranea (L) Verde). J. Sci. Food Agri. 66, 443–446. doi: 10.1002/jsfa.2740660404
Beckett, D. (2009). Biotin sensing at the molecular level. J. Nutri. 139, 167–170. doi: 10.3945/jn.108.095760
Bengaly, M. D. (2001). Etude microbiologique et valeur nutritionelle d'un condiment traditionnel riche en protéines, obtenu par fermentation naturelle des graines d'Hibiscus sabdariffa. Mémoire de Doctorat. UFR-SVT, Université de Ouagadougou, Burkina Faso
Bermingham, A., and Derrick, J. P. (2002). The folic acid biosynthesis pathway in bacteria: evaluation of potential for antibacterial drug discovery. Bioessays 24, 637–648. doi: 10.1002/bies.10114
Bouchenak, M., and Lamri-Senhadji, M. (2013). Nutritional quality of legumes, and their role in cardiometabolic risk prevention: a review. J. Med. Food 16, 185–198. doi: 10.1089/jmf.2011.0238
Bretzel, W., Schurter, W., Ludwig, B., Kupfer, E., Doswald, S., Pfister, M., et al. (1999). Commercial riboflavin production by recombinant Bacillus subtilis: down-stream processing and comparison of the composition of riboflavin produced by fermentation or chemical synthesis. J. Indust. Microbiol. Biotechnol. 22, 19–26. doi: 10.1038/sj.jim.2900604
Burgess, C. M., Smid, E. J., and van Sinderen, D. (2009). Bacterial vitamin B2, B11 and B12 overproduction: an overview. Int. J. Food Microbiol. 133, 1–7. doi: 10.1016/j.ijfoodmicro.2009.04.012
Campbell-Platt, G. (1980). African locust bean (Parkia species) and its West African fermented food product, dawadawa. Ecol. Food Nutr. 9, 123–132. doi: 10.1080/03670244.1980.9990590
Chadare, F. J., Jonkman, J., Wolkers-Rooijackers, J., Nout, M. J., Hounhouigan, J. D., and Zwietering, M. H. (2011). Microbiota of Tayohounta, a fermented baobab flavour food of Benin. Afr. J. Biotechnol. 10, 15607–15615. doi: 10.5897/AJB11.1770
Chantawannakul, P., Oncharoen, A., Klanbut, K., Chukeatirote, E., and Lumyong, S. (2002). Characterization of proteases of Bacillus subtilis strain 38 isolated from traditionally fermented soybean in Northern Thailand. Sci. Asia 28, 241–245. Available online at: http://scienceasia.org/2002.28.n3/v28_241_245.pdf
Chen, T., Jiang, S., Xiong, S., Wang, M., Zhu, D., and Wei, H. (2012). Application of denaturing gradient gel electrophoresis to microbial diversity analysis in Chinese Douchi. J. Sci. Food Agri. 92, 2171–2176. doi: 10.1002/jsfa.5604
Chen, T., Wang, M., Jiang, S., Xiong, S., Zhu, D., and Wei, H. (2011a). Investigation of the microbial changes during koji?making process of Douchi by culture-dependent techniques and PCR?DGGE. Int. J. Food Sci. Technol. 46, 1878–1883. doi: 10.1111/j.1365-2621.2011.02696.x
Chen, T., Xiong, S., Jiang, S., Wang, M., Wu, Q., and Wei, H. (2011b). Molecular identification of microbial community in Chinese douchi during post-fermentation process. Food Sci. Biotechnol. 20, 1633–1638. doi: 10.1007/s10068-011-0225-0
Chettri, R., and Tamang, J. P. (2008). Microbiological evaluation of maseura, an ethnic fermented legume-based condiment of Sikkim. J. Hill Res. 21, 1–7.
Chettri, R., and Tamang, J. P. (2015). Bacillus species isolated from tungrymbai and bekang, naturally fermented soybean foods of India. Int. J. Food Microbiol. 197, 72–76. doi: 10.1016/j.ijfoodmicro.2014.12.021
Cho, K. M., and Seo, W. T. (2007). Bacterial diversity in a Korean traditional soybean fermented foods (doenjang and ganjang) by 16S rRNA gene sequence analysis. Food Sci. Biotechnol. 16, 320–324.
Chukeatirote, E. (2015). Thua nao: Thai fermented soybean. J. Ethn. Foods 2, 115–118. doi: 10.1016/j.jef.2015.08.004
Chukeatirote, E., Dajanta, K., and Apichartsrangkoon, A. (2010). Thua nao, indigenous Thai fermented soybean: a review. J. Biol. Sci. 10, 581–583. doi: 10.3923/jbs.2010.581.583
Colletti, A., Attrovio, A., Boffa, L., Mantegna, S., and Cravotto, G. (2020). Valorisation of by-products from soybean (Glycine max (L.) Merr.) processing. Molecules 25, 2129. doi: 10.3390/molecules25092129
Compaore, C. S., Nielsen, D. S., Ouoba, L. I. I., Berner, T. S., Nielsen, K. F., Sawadogo-Lingani, H., et al. (2013). Co-production of surfactin and a novel bacteriocin by Bacillus subtilis subsp. subtilis H4 isolated from Bikalga, an African alkaline Hibiscus sabdariffa seed fermented condiment. Int. J. Food Microbiol. 162, 297–307. doi: 10.1016/j.ijfoodmicro.2013.01.013
Dahal, N. R., Karki, T. B., Swamylingappa, B., Li, Q. I., and Gu, G. (2005). Traditional foods and beverages of Nepal-a review. Food Rev. Int. 21, 1–25. doi: 10.1081/FRI-200040579
Dakwa, S., Sakyi-Dawson, E., Diako, C., Annan, N. T., and Amoa-Awua, W. K. (2005). Effect of boiling and roasting on the fermentation of soybeans into dawadawa (soy-dawadawa). Int. J. Food Microbiol. 104, 69–82. doi: 10.1016/j.ijfoodmicro.2005.02.006
Dihingia, A., Bordoloi, J., Dutta, P., Kalita, J., and Manna, P. (2018). Hexane-Isopropanolic extract of tungrymbai, a North-East Indian fermented soybean food prevents hepatic steatosis via regulating AMPK-mediated SREBP/FAS/ACC/HMGCR and PPARα/CPT1A/UCP2 pathways. Sci. Rep. 8, 10021. doi: 10.1038/s41598-018-27607-7
Dimidi, E., Cox, S. R., Rossi, M., and Whelan, K. (2019). Fermented foods: definitions and characteristics, impact on the gut microbiota and effects on gastrointestinal health and disease. Nutrients 11, 1806. doi: 10.3390/nu11081806
Dirar, H. A. (1984). Kawal, meat substitute from fermentedCassia obtusifolia leaves. Econom. Bot. 38, 342–349. doi: 10.1007/BF02859013
Dirar, H. A., Harper, D. B., and Collins, M. A. (1985). Biochemical and microbiological studies on kawal, a meat substitute derived by fermentation of Cassia obtusifolia leaves. J. Sci. Food Agric. 36, 881–892. doi: 10.1002/jsfa.2740360919
Einson, J. E., Rani, A., You, X., Rodriguez, A. A., Randell, C. L., Barnaba, T., et al. (2018). A vegetable fermentation facility hosts distinct microbiomes reflecting the production environment. Appl. Environ. Microbiol. 84, e01680–e01618. doi: 10.1128/AEM.01680-18
Eltayeb, M. M., Hassan, A. B., Sulieman, M. A., and Babiker, E. E. (2007). Effect of processing followed by fermentation on antinutritional factors content of pearl millet (Pennisetum glaucum L.) cultivars. Pak. J. Nutr. 6, 463–467. doi: 10.3923/pjn.2007.463.467
Esenwah, C. N., and Ikenebomeh, M. J. (2008). Processing effects on the nutritional and anti-nutritional contents of african locust bean (Parkia biglobosa Benth.) seed. Pak. J. Nutr. 7, 214–217. doi: 10.3923/pjn.2008.214.217
Ezekiel, O. O., Ogunshe, A. A. O., and Jegede, D. E. (2015). Controlled fermentation of cotton seeds (Gossypium hirsutum) for owoh production using bacteria starter cultures. Nigerian Food J. 33, 54–60. doi: 10.1016/j.nifoj.2015.04.013
Ezeokoli, O. T., Gupta, A. K., Mienie, C., Popoola, T. O., and Bezuidenhout, C. C. (2016). PCR-denaturing gradient gel electrophoresis analysis of microbial community in soy-daddawa, a Nigerian fermented soybean (Glycine max (L.) Merr.) condiment. Int. J. Food Microbiol. 220, 58–62. doi: 10.1016/j.ijfoodmicro.2016.01.003
Fan, J., Zhang, Y., Chang, X., Saito, M., and Li, Z. (2009). Changes in the radical scavenging activity of bacterial-type douchi, a traditional fermented soybean product, during the primary fermentation process. Biosci. Biotechnol. Biochem. 73, 2749–2753. doi: 10.1271/bbb.90361
Fang, H., Kang, J., and Zhang, D. (2017). Microbial production of vitamin B(12): a review and future perspectives. Microb. Cell Fact. 16, 15–15. doi: 10.1186/s12934-017-0631-y
García-Angulo, V. A. (2017). Overlapping riboflavin supply pathways in bacteria. Crit. Rev. Microbiol. 43, 196–209. doi: 10.1080/1040841X.2016.1192578
Ghavidel, R. A., and Prakash, J. (2007). The impact of germination and dehulling on nutrients, antinutrients, in vitro iron and calcium bioavailability and in vitro starch and protein digestibility of some legume seeds. LWT Food Sci. Technol. 40, 1292–1299. doi: 10.1016/j.lwt.2006.08.002
Giraffa, G. (2004). Studying the dynamics of microbial populations during food fermentation. FEMS Microbiol. Rev. 28, 251–260. doi: 10.1016/j.femsre.2003.10.005
Glaus, M. A., and Van Loon, L. R. (2008). Degradation of cellulose under alkaline conditions: new insights from a 12 years degradation study. Environ. Sci. Technol. 42, 2906–2911. doi: 10.1021/es7025517
Gu, Q., and Li, P. (2016). “Biosynthesis of vitamins by probiotic bacteria, probiotics and prebiotics in human nutrition and health,” in Probiotics and Prebiotics in Human Nutrition and Health, eds V. Rao and L. G. Rao (Rijeka: IntechOpen), 135–148. doi: 10.5772/63117
Harper, D. B., and Collins, M. A. (1992). “Leaf and seed fermentations of Western Sudan,” in Applications of Biotechnology in Traditional Fermented Foods (Washington, DC: National Academies Press), 105–113.
Hesseltine, C., and Wang, H. L. (1980). The importance of traditional fermented foods. Bioscience 30, 402–404. doi: 10.2307/1308003
Hoa, N. T., Baccigalupi, L., Huxham, A., Smertenko, A., Van, P. H., Ammendola, S., et al. (2000). Characterization of Bacillus species used for oral bacteriotherapy and bacterioprophylaxis of gastrointestinal disorders. Appl. Environ. Microbiol. 66, 5241–5247. doi: 10.1128/AEM.66.12.5241-5247.2000
Holland, C., Ryden, P., Edwards, C. H., and Grundy, M. M. L. (2020). Plant cell walls: impact on nutrient bioaccessibility and digestibility. Foods 9, 201. doi: 10.3390/foods9020201
Hong, S. W., Choi, J. Y., and Chung, K. S. (2012). Culture-based and denaturing gradient gel electrophoresis analysis of the bacterial community from chungkookjang, a traditional Korean fermented soybean food. J. Food Sci. 77, M572–M578. doi: 10.1111/j.1750-3841.2012.02901.x
Hosoi, T., Hirose, R., Saegusa, S., Ametani, A., Kiuchi, K., and Kaminogawa, S. (2003). Cytokine responses of human intestinal epithelial-like Caco-2 cells to the nonpathogenic bacterium Bacillus subtilis (natto). Int. J. Food Microbiol. 82, 255–264. doi: 10.1016/S0168-1605(02)00311-2
Hu, Y., Ge, C., Yuan, W., Zhu, R., Zhang, W., Du, L., et al. (2010). Characterization of fermented black soybean natto inoculated with Bacillus natto during fermentation. J. Sci. Food Agric. 90, 1194–1202. doi: 10.1002/jsfa.3947
Ijarotimi, O. S., and Keshinro, O. O. (2012). Comparison between the amino acid, fatty acid, mineral and nutritional quality of raw, germinated and fermented African locust bean (Parkia biglobosa) flour. Acta Sci. Polonorum Technol. Aliment. 11, 151–165.
Illeghems, K., De Vuyst, L., Papalexandratou, Z., and Weckx, S. (2012). Phylogenetic analysis of a spontaneous cocoa bean fermentation metagenome reveals new insights into its bacterial and fungal community diversity. PLoS ONE 7, e38040. doi: 10.1371/journal.pone.0038040
Inatsu, Y., Nakamura, N., Yuriko, Y., Fushimi, T., Watanasiritum, L., and Kawamoto, S. (2006). Characterization of Bacillus subtilis strains in Thua nao, a traditional fermented soybean food in northern Thailand. Lett. Appl. Microbiol. 43, 237–242. doi: 10.1111/j.1472-765X.2006.01966.x
Isu, N. R., and Njoku, H. O. (1997). An evaluation of the microflora associated with fermented African oil bean (Pentaclethra macrophylla Bentham) seeds during ugba production. Plant Food Hum. Nutr. 51, 145–157. doi: 10.1023/A:1007906413195
Isu, N. R., and Ofuya, C. O. (2000). Improvement of the traditional processing and fermentation of African oil bean (Pentaclethra macrophylla Bentham) into a food snack-‘ugba'. Int. J. Food Microbiol. 59, 235–239. doi: 10.1016/S0168-1605(00)00318-4
Iwuoha, C. I., and Eke, O. S. (1996). Nigerian indigenous fermented foods: their traditional process operation, inherent problems, improvements and current status. Food Res. Int. 29, 527–540. doi: 10.1016/0963-9969(95)00045-3
Jacquier, V., Nelson, A., Jlali, M., Rhayat, L., Brinch, K. S., and Devillard, E. (2019). Bacillus subtilis 29784 induces a shift in broiler gut microbiome toward butyrate-producing bacteria and improves intestinal histomorphology and animal performance. Poult. Sci. 98, 2548–2554. doi: 10.3382/ps/pey602
Jamir, B., and Deb, C. R. (2018). Nutritional assessment and molecular identification of microorganisms from Akhuni/Axone: a soybean based fermented food of Nagaland, India. J. Adv. Biol. 11, 2170–2179. doi: 10.24297/jab.v11i1.7118
Jayani, R. S., Saxena, S., and Gupta, R. (2005). Microbial pectinolytic enzymes: a review. Process Biochem. 40, 2931–2944. doi: 10.1016/j.procbio.2005.03.026
Jeff-Agboola, Y. (2007). Microorganisms associated with natural fermentation of African yam bean (Sphenostylis sternocarpa Harms) seeds for the production of otiru. Res. J. Microbiol. 2, 816–823. doi: 10.3923/jm.2007.816.823
Jeyaram, K., Singh, W. M., Premarani, T., Devi, A. R., Chanu, K. S., Talukdar, N. C., et al. (2008). Molecular identification of dominant microflora associated with ‘Hawaijar'-a traditional fermented soybean (Glycine max (L.)) food of Manipur, India. Int. J. Food Microbiol. 122, 259–268. doi: 10.1016/j.ijfoodmicro.2007.12.026
Kaboré, D., Nielsen, D. S., Sawadogo-Lingani, H., Diawara, B., Dicko, M. H., Jakobsen, M., et al. (2013). Inhibition of Bacillus cereus growth by bacteriocin producing Bacillus subtilis isolated from fermented baobab seeds (maari) is substrate dependent. Int. J. Food Microbiol. 162, 114–119. doi: 10.1016/j.ijfoodmicro.2012.12.027
Kaboré, D., Thorsen, L., Nielsen, D. S., Berner, T. S., Sawadogo-Lingani, H., Diawara, B., et al. (2012). Bacteriocin formation by dominant aerobic sporeformers isolated from traditional maari. Int. J. Food Microbiol. 154, 10–18. doi: 10.1016/j.ijfoodmicro.2011.12.003
Kamada, M., Hase, S., Fujii, K., Miyake, M., Sato, K., Kimura, K., et al. (2015). Whole-genome sequencing and comparative genome analysis of bacillus subtilis strains isolated from non-salted fermented soybean foods. PLoS ONE 10, e0141369. doi: 10.1371/journal.pone.0141369
Kang, K. J., Jeoung, J. H., and Cho, J. I. (2000). Inhibition of aflatoxin-producing fungi with antifungal compound produced by Bacillus subtilis. J. Fd. Hyg. Safety 15, 122–127.
Kanno, A., and Takamatsu, H. (1987). Changes in the volatile components of “natto” during manufacturing and storage studies on“ natto”, part IV. Nippon Shokuhin Kogyo Gakkaishi 34, 330–335. doi: 10.3136/nskkk1962.34.5_330
Katsuyama, H., Ideguchi, S., Fukunaga, M., Saijoh, K., and Sunami, S. (2002). Usual dietary intake of fermented soybeans (natto) is associated with bone mineral density in premenopausal women. J. Nutr. Sci. Vitaminol. 48, 207–215. doi: 10.3177/jnsv.48.207
Kawai, K., Kamochi, R., Oiki, S., Murata, K., and Hashimoto, W. (2018). Probiotics in human gut microbiota can degrade host glycosaminoglycans. Sci. Rep. 8, 10674. doi: 10.1038/s41598-018-28886-w
Kere-Kando, C., Greve-Johansen, P., Compaoré, C. S., Sawadogo-Lingani, H., Ouédraogo, G. A., Diawara, B., et al. (2020). Predominance of bacillus spp. During the production of mantchoua, a traditional kapok seed fermented condiment from Burkina Faso. J. Microbiol. Biotechnol. Food Sci. 9, 1009–1015. doi: 10.15414/jmbfs.2020.9.5.1009-1015
Khan, M. M. A., Kumar, A., and Bala, K. L. (2016). Effect of storage conditions on physio-chemical characteristics of tray and solar dehydrated health functionality indigenous fermented soybean hawaijar. Int. J. Food Ferment. Technol. 6, 137. doi: 10.5958/2277-9396.2016.00036.2
Kiers, J. L., Van Laeken, A. E. A., Rombouts, F. M., and Nout, M. J. R. (2000). In vitro digestibility of Bacillus fermented soya bean. Int. J. Food Microbiol. 60, 163–169. doi: 10.1016/S0168-1605(00)00308-1
Kim, J. -Y., Yeo, S. -H., Baek, S. -Y., and Choi, H. -S. (2011b). Molecular and morphological identification of fungal species isolated from bealmijang meju. J. Microbiol. Biotechnol. 21, 1270–1279. doi: 10.4014/jmb.1105.05013
Kim, M.-H., Han, S.-Y., Ko, J.-M., and Kim, Y.-S. (2012). Degradation characteristics of proteins in cheonggukjang (fermented unsalted soybean paste) prepared with various soybean cultivars. Food Sci. Biotechnol. 21, 9–18. doi: 10.1007/s10068-012-0002-8
Kim, T.-W., Lee, J.-H., Kim, S.-E., Park, M.-H., Chang, H. C., and Kim, H.-Y. (2009). Analysis of microbial communities in doenjang, a Korean fermented soybean paste, using nested PCR-denaturing gradient gel electrophoresis. Int. J. Food Microbiol. 131, 265–271. doi: 10.1016/j.ijfoodmicro.2009.03.001
Kim, Y. -S., Kim, M. -C., Kwon, S. -W., Kim, S. -J., Park, I. -C., Ka, J. -O., et al. (2011a). Analyses of bacterial communities in meju, a Korean traditional fermented soybean bricks, by cultivation-based and pyrosequencing methods. J. Microbiol. 49, 340–348. doi: 10.1007/s12275-011-0302-3
Kiuchi, K., Funane, K., Taya, N., and Sulistyo, J. (1987). Isolation and Identification of Natto Bacteria From Market-Sold Natto Starters. Tokyo: Report of National Food Research Institute.
Kiuchi, K., and Watanabe, S. (2004) “Industrialization of Japanese natto,” in Industrialization of Indigenous Fermented Foods, ed K. Steinkraus (New York, NY: CRC press), 193–238.
Kobawila, S., Louembe, D., Keleke, S., Hounhouigan, J., and Gamba, C. (2005). Reduction of the cyanide content during fermentation of cassava roots and leaves to produce bikedi and ntoba mbodi, two food products from Congo. Afr. J. Biotechnol. 4, 689–696. doi: 10.5897/AJB2005.000-3128
Kouris-Blazos, A., and Belski, R. (2016). Health benefits of legumes and pulses with a focus on Australian sweet lupins. Asia Pac. J. Clin. Nutr. 25, 1–17.
Kpikpi, E. N., Thorsen, L., Glover, R., Dzogbefia, V. P., and Jespersen, L. (2014). Identification of Bacillus species occurring in kantong, an acid fermented seed condiment produced in Ghana. Int. J. Food Microbiol. 180, 1–6. doi: 10.1016/j.ijfoodmicro.2014.03.028
Kronenberg, H. J., and Hang, Y. D. (1984). Biochemical changes in Okara during metauza fermentation. Nutr. Rep. Int. 30, 439–443.
Kubo, Y., Rooney, A. P., Tsukakoshi, Y., Nakagawa, R., Hasegawa, H., and Kimura, K. (2011). Phylogenetic analysis of Bacillus subtilis strains applicable to natto (fermented soybean) production. Appl. Environ. Microbiol. 77, 6463–6469. doi: 10.1128/AEM.00448-11
Kuswanto, K. R. (1988). Studies on the Semayi Indonesian traditional fermented food. Mem. Tokyo Univ. Agri. 30, 189–287.
Kwak, C. S., Hwang, J. Y., Watanabe, F., and Park, S. C. (2008). Vitamin B12 Contents in Some Korean Fermented Foods and Edible Seaweeds. Kor. J. Nutr. 41, 439–447. Available online at: https://www.koreamed.org/SearchBasic.php?RID=2265673
Kwak, C. S., Lee, M. S., Oh, S. I., and Park, S. C. (2010). Discovery of novel sources of vitamin B12 in traditional korean foods from nutritional surveys of centenarians. Curr. Gerontol. Geriatr. Res. 2010, 374897. doi: 10.1155/2010/374897
LeBlanc, J. G., Laiño, J. E., del Valle, M. J., Vannini, V., van Sinderen, D., Taranto, M. P., et al. (2011). B-Group vitamin production by lactic acid bacteria – current knowledge and potential applications. J. Appl. Microbiol. 111, 1297–1309. doi: 10.1111/j.1365-2672.2011.05157.x
Lee, A. R., Kim, G. M., Park, J. Y., Jo, H. D., Cha, J., Song, Y. S., et al. (2010). Characterization of a 27 kDa fibrinolytic enzyme from Bacillus amyloliquefaciens CH86-1 isolated from cheonggukjang. Korean J. Appl. Microbiol. Biotechnol. 53, 56–61. doi: 10.3839/jksabc.2010.010
Lee, I.-J., Haw, K., and Kim, S.-I. (1959). Biochemical studies on korean fermented foods. VIII studies on vitamin B12 contents of the fermented foods in Korea. Yakhak Hoeji 4, 50–52.
Lee, N.-K., Kim, W.-S., and Paik, H.-D. (2019). Bacillus strains as human probiotics: characterization, safety, microbiome, and probiotic carrier. Food Sci. Biotechnol. 28, 1297–1305. doi: 10.1007/s10068-019-00691-9
Leejeerajumnean, A. (2000). Bacillus fermentation of soybeans: Characterization of traditional thua nao manufacture (Doctoral dissertation). University of Reading, Reading, United Kingdom
Li, L.-T., Yin, L.-J., and Saito, M. (2004). Function of traditional foods and food culture in China. Jpn. Agric. Res. Q. 38, 213–220. doi: 10.6090/jarq.38.213
Li, L. -T., and Ma, Y. -L. (2014) “Douchi,” in Handbook of Indigenous Foods Involving Alkaline Fermentation, eds P. K. Sarkar M. R. Nout (London; New York, NY: CRC Press), 18–33.
Li, Z. X., Yin, G. M., and Chen, T. (2013). Optimization of riboflavin production by recombinant bacillus subtilis X42 using statistical designs. Adv. Mater. Res. 634, 1031–1036. doi: 10.4028/www.scientific.net/AMR.634-638.1031
Liu, C.-J., Gong, F.-M., Li, X.-R., Li, H.-Y., Zhang, Z.-H., Feng, Y., et al. (2012). Natural populations of lactic acid bacteria in douchi from Yunnan Province, China. J. Zhejiang Univer. Sci. B 13, 298–306. doi: 10.1631/jzus.B1100221
Liu, L., Chen, X., Hao, L., Zhang, G., Jin, Z., Li, C., et al. (2020). Traditional fermented soybean products: processing, flavor formation, nutritional and biological activities. Crit. Rev. Food Sci. Nutr. 62, 1971–1989. doi: 10.1080/10408398.2020.1848792
Louembe, D., Kobawila, S., Bouanga-Kalou, G., and Kéléké, S. (2003). Etude microbiologique des feuilles fermentées de manioc:≪ntoba mbodi≫. Tropicultura 21, 106–111.
Man, Z.-W., Rao, Z.-M., Cheng, Y.-P., Yang, T.-W., Zhang, X., Xu, M.-J., et al. (2014). Enhanced riboflavin production by recombinant Bacillus subtilis RF1 through the optimization of agitation speed. World J. Microbiol. Biotechnol. 30, 661–667. doi: 10.1007/s11274-013-1492-0
Mani, V., and Ming, L. C. (2017). “Chapter 19 - tempeh and other fermented soybean products rich in isoflavones,” in Fermented Foods in Health and Disease Prevention, eds J. Frias, C. Martinez-Villaluenga, and E. Peñas (London: Academic Press), 453–474. doi: 10.1016/B978-0-12-802309-9.00019-4
Marco, M. L., Sanders, M. E., Gänzle, M., Arrieta, M. C., Cotter, P. D., De Vuyst, L., et al. (2021). The international scientific association for probiotics and prebiotics (ISAPP) consensus statement on fermented foods. Nat. Rev. Gastroenterol. Hepatol. 18, 196–208. doi: 10.1038/s41575-020-00390-5
Mbaiguinam, M., Mahmout, Y., Tarkodjiel, M., Delobel, B., and Bessiere, J.-M. (2005). Constituents of Kawal, fermented Cassia obtusifolia leaves, a traditional food from Chad. Afr. J. Biotechnol. 4, 1080–1083.
Mbajunwa, O. K., Akingbala, J. O., Mulongoy, K., and Oguntimein, G. (1998). Starter culture evaluation for the production of ugba from African oil bean seed Pentaclethra macrophylla. J. Sci. Food Agri. 77, 127–132. doi: 10.1002/(SICI)1097-0010(199805)77:1<127::AID-JSFA17>3.0.CO;2-4
Mbozo, A. B. V., Kobawila, S. C., Anyogu, A., Awamaria, B., Louembe, D., Sutherland, J. P., et al. (2017). Investigation of the diversity and safety of the predominant Bacillus pumilus sensu lato and other Bacillus species involved in the alkaline fermentation of cassava leaves for the production of Ntoba Mbodi. Food Control 82, 154–162. doi: 10.1016/j.foodcont.2017.06.018
Meerak, J., Iida, H., Watanabe, Y., Miyashita, M., Sato, H., Nakagawa, Y., et al. (2007). Phylogeny of γ-polyglutamic acid-producing Bacillus strains isolated from fermented soybean foods manufactured in Asian countries. J. Gen. Appl. Microbiol. 53, 315–323. doi: 10.2323/jgam.53.315
Meerak, J., Yukphan, P., Miyashita, M., Sato, H., Nakagawa, Y., and Tahara, Y. (2008). Phylogeny of γ-polyglutamic acid-producing Bacillus strains isolated from a fermented locust bean product manufactured in West Africa. J. Gen. Appl. Microbiol. 54, 159–166. doi: 10.2323/jgam.54.159
Mohamadou, B.-A., Mbofung, C. M., and Thouvenot, D. (2008). Bacterial fermentation induced mineral dynamics during the production of Mbuja from Hibiscus sabdariffa seeds in earthen-ware pots. Ann. Microbiol. 58, 447. doi: 10.1007/BF03175542
Mohamadou, B. A., Mbofung, C. M., and Barbier, G. (2013). Genotypic and phenotypic diversity among Bacillus species isolated from Mbuja, a Cameroonian traditional fermented condiment. Afr. J. Biotechnol. 12, 1335–1343.
Mohammadou, B. A., Mbofung, C. M., Mounier, J., and Coton, E. (2018). Use of selected Bacillus spp. strains for directed fermentation of Hibiscus sabdariffa seeds into Mbuja. Asian Food Sci. J. 2, 1–9. doi: 10.9734/AFSJ/2018/41317
Mohammed, Y., Lee, B., Kang, Z., and Du, G. (2014). Development of a two-step cultivation strategy for the production of vitamin B12 by Bacillus megaterium. Microb. Cell Fact. 13, 102. doi: 10.1186/s12934-014-0102-7
Mokemiabeka, S., Dhellot, J., Kobawila, S., Diakabana, P., Loukombo, R. N., Nyanga-Koumou, A., et al. (2011). Softening and mineral content of cassava (Manihot esculenta Crantz) leaves during the fermentation to produce ntoba mbodi. Adv. J. Food Sci. Technol. 3, 418–423.
Moore, S. J., and Warren, M. J. (2012). The anaerobic biosynthesis of vitamin B12. Biochem. Soc. Trans. 40, 581–586. doi: 10.1042/BST20120066
Moutou-Tchitoula, D. P., Nguimbi, E., Mora, P., Kobawila, S. C., and Miambi, E. (2018). Assessment of dominant bacterial strains isolated from Ntoba mbodi, an indigenous African alkaline-fermented food, and their potential enzyme activities. Afr. J. Microbiol. Res. 12, 779–787. doi: 10.5897/AJMR2018.8875
Musa, D. A., Omale, J., and Daikwo, A. M. (2011). Fungal contamination of fermented prosopis africana (okpehe) and toxicity screening of the crude extracts in albino rats (rattus novergicus). Adv. Appl. Sci. Res. 2, 108–113.
Nam, Y. -D., Lee, S. -Y., and Lim, S. -I. (2012). Microbial community analysis of Korean soybean pastes by next-generation sequencing. Int. J. Food Microbiol. 155, 36–42. doi: 10.1016/j.ijfoodmicro.2012.01.013
Namgung, H. J., Park, H. J., Cho, I. H., Choi, H. K., Kwon, D. Y., Shim, S. M., et al. (2010). Metabolite profiling of doenjang, fermented soybean paste, during fermentation. J. Sci. Food Agric. 90, 1926–1935. doi: 10.1002/jsfa.4036
Nataraj, B. H., Ali, S. A., Behare, P. V., and Yadav, H. (2020). Postbiotics-parabiotics: the new horizons in microbial biotherapy and functional foods. Microb. Cell Fact. 19, 168. doi: 10.1186/s12934-020-01426-w
N'dir, B., Gningue, R. D., Keita, N'. D. G., Souane, M., Laurent, L., Cornelius, C, et al. (1997) Caractéristiques microbiologiques et organoleptiques du nététu du commerce. Cahiers Agri. 6, 299–304.
N'Dir, B., Hbid, C. L., Cornelius, C., Roblain, D., Jacques, P., Vanhentenryck, F., et al. (1994). Proptiétés antifongiques de la microflora sporulée du nététu. Cahiers Agric. 3, 23–30. Available online at: https://revues.cirad.fr/index.php/cahiers-agricultures/article/view/29819/29579
Nicholson, W. L., Munakata, N., Horneck, G., Melosh, H. J., and Setlow, P. (2000). Resistance of Bacillus endospores to extreme terrestrial and extraterrestrial environments. Microbiol. Mol. Biol. Rev. 64, 548–572. doi: 10.1128/MMBR.64.3.548-572.2000
Nishito, Y., Osana, Y., Hachiya, T., Popendorf, K., Toyoda, A., Fujiyama, A., et al. (2010). Whole genome assembly of a natto production strain Bacillus subtilis natto from very short read data. BMC Genom. 11, 1–12. doi: 10.1186/1471-2164-11-243
Nwokeleme, C. O., and Ugwuanyi, J. O. (2015). Evolution of volatile flavour compounds during fermentation of African oil bean (Pentaclethra macrophylla Benth) seeds for “Ugba” production. Int. J. Food Sci. 706328. doi: 10.1155/2015/7063282015
Odunfa, S. (1985). Biochemical changes in fermenting African locust bean (Parkia biglobosa) during ‘iru' fermentation. Int. J. Food Sci. Technol. 20, 295–303. doi: 10.1111/j.1365-2621.1985.tb00379.x
Odunfa, S., and Oyewole, O. (1986). Identification of Bacillus species from ‘iru', a fermented African locust bean product. J. Basic Microbiol. 26, 101–108. doi: 10.1002/jobm.3620260212
Odunfa, S. A. (1981). Microorganisms associated with fermentation of African locust bean (Parkia filicoidea) during iru preparation. J. Plant Foods 3, 245–250. doi: 10.1080/0142968X.1981.11904236
Odunfa, S. A. (1983). Carbohydrate changes in fermenting locust bean (Parkia filicoidea) during iru preparation. Plant Foods Hum. Nutr. 32, 3–10. doi: 10.1007/BF01093924
Odunfa, S. A. (1986). Microbiological assay of vitamin B and biotin in some Nigerian fermented foods. Food Chem. 19, 129–136. doi: 10.1016/0308-8146(86)90106-8
Ogueke, C. C., Okoli, A. I., Owuamanam, C. I., Ikechukwu, A. P., and Iwouno, J. O. (2013) Production of soup condiment (ogiri ugu) from fluted pumpkin seeds using bacillus subtilis. Int. J. Life Sci. 2, 113–120.
Ogunshe, A. A., Omotosho, M. O., and Ayansina, A. D. V. (2007). Microbial studies and biochemical characteristics of controlled fermented afiyo-a Nigerian fermented food condiment from Prosopis africana (Guill and Perr.) Taub. Pakistan J. Nutr. 6, 620–627.
Oguntoyinbo, F., Sanni, A., Franz, C., and Holzapfel, W. (2007). Phenotypic diversity and technological properties of Bacillus subtilis species isolated from okpehe, a traditional fermented condiment. World J. Microbiol. Biotechnol. 23, 401–410. doi: 10.1007/s11274-006-9238-x
Oguntoyinbo, F. A., Fusco, V., Cho, G.-S., Kabisch, J., Neve, H., Bockelmann, W., et al. (2016). Produce from Africa's Gardens: potential for leafy vegetable and fruit fermentations. Front. Microbiol. 7, 981. doi: 10.3389/fmicb.2016.00981
Oguntoyinbo, F. A., Huch, M., Cho, G.-S., Schillinger, U., Holzapfel, W. H., Sanni, A. I., et al. (2010). Diversity of Bacillus species isolated from okpehe, a traditional fermented soup condiment from Nigeria. J. Food Prot. 73, 870–878. doi: 10.4315/0362-028X-73.5.870
Oguntoyinbo, F. A., and Oni, O. M. (2004). Incidence and characterization of Bacillus cereus isolated from traditional fermented meals in Nigeria. J. Food Protect. 67, 2805–2808. doi: 10.4315/0362-028X-67.12.2805
Ojinnaka, M. T. C., and Ojimelukwe, P. C. (2013). Study of the volatile compounds and amino acid profile in Bacillus fermented castor oil bean condiment. J. Food Res. 2, 191-198. doi: 10.5539/jfr.v2n1p191
Okafor, N. D. U. K. A. (1977). Microorganisms associated with cassava fermentation for gari production. J. Appl. Bacteriol. 42, 279–284. doi: 10.1111/j.1365-2672.1977.tb00693.x
Omafuvbe, B. O., Abiose, S. H., and Adaraloye, O. O. (1999). The production of ‘Kpaye'-a fermented condiment from Prosopis africana (Guill and Perr) Taub. Seeds. Int. J. Food Microbiol. 51, 183–186. doi: 10.1016/S0168-1605(99)00088-4
Omafuvbe, B. O., Falade, O. S., Osuntogun, B. A., and Adewusi, S. R. (2004). Chemical and biochemical changes in African locust bean (Parkia biglobosa) and melon (Citrullus vulgaris) seeds during fermentation to condiments. Pakistan J. Nutr. 3, 140–145.
Osman, M. A. (2004). Changes in sorghum enzyme inhibitors, phytic acid, tannins and in vitro protein digestibility occurring during Khamir (local bread) fermentation. Food Chem. 88, 129–134. doi: 10.1016/j.foodchem.2003.12.038
Ouoba, L., Diawara, B., Annan, N., Poll, L., and Jakobsen, M. (2005). Volatile compounds of soumbala, a fermented African locust bean (Parkia biglobosa) food condiment. J. Appl. Microbiol. 99, 1413–1421. doi: 10.1111/j.1365-2672.2005.02722.x
Ouoba, L., Nyanga-Koumou, C., Parkouda, C., Sawadogo, H., Kobawila, S., Keleke, S., et al. (2010).. Genotypic diversity of lactic acid bacteria isolated from African traditional alkaline-fermented foods. J. Appl. Microbiol. 108, 2019–2029. doi: 10.1111/j.1365-2672.2009.04603.x
Ouoba, L., Parkouda, C., Diawara, B., Scotti, C., and Varnam, A. (2008). Identification of Bacillus spp. from Bikalga, fermented seeds of Hibiscus sabdariffa: phenotypic and genotypic characterization. J. Appl. Microbiol. 104, 122–131. doi: 10.1111/j.1365-2672.2007.03550.x
Ouoba, L. I. I., Cantor, M. D., Diawara, B., Traore, A. S., and Jakobsen, M. (2003). Degradation of African locust bean oil by Bacillus subtilis and Bacillus pumilus isolated from soumbala, a fermented African locust bean condiment. J. Appl. Microbiol. 95, 868–873. doi: 10.1046/j.1365-2672.2003.02063.x
Ouoba, L. I. I., Diawara, B., Christensen, T., Dalgaard Mikkelsen, J., and Jakobsen, M. (2007). Degradation of polysaccharides and non-digestible oligosaccharides by Bacillus subtilis and Bacillus pumilus isolated from Soumbala, a fermented African locust bean (Parkia biglobosa) food Condiment. Euro. Food Res. Technol. 224, 689–694. doi: 10.1007/s00217-006-0359-0
Ouoba, L. I. I., Diawara, B., Kofi Amoa-Awua, W., Traoré, A. S., and Møller, P. L. (2004). Genotyping of starter cultures of Bacillus subtilis and Bacillus pumilus for fermentation of African locust bean (Parkia biglobosa) to produce Soumbala. Int. J. Food Microbiol. 90, 197–205. doi: 10.1016/S0168-1605(03)00302-7
Owens, J. D., Allagheny, N., Kipping, G., and Ames, J. M. (1997). Formation of volatile compounds during Bacillus subtilis fermentation of soya beans. J. Sci. Food Agri. 74, 132–40. doi: 10.1002/(SICI)1097-0010(199705)74:1<132::AIDJSFA779>3.0.CO
Owusu-Kwarteng, J., Akabanda, F., Nielsen, D. S., Tano-Debrah, K., Glover, R. L., and Jespersen, L. (2012). Identification of lactic acid bacteria isolated during traditional fura processing in Ghana. Food Microbiol. 32, 72–78. doi: 10.1016/j.fm.2012.04.010
Owusu-Kwarteng, J., Parkouda, C., Adewumi, G. A., Ouoba, L. I. I., and Jespersen, L. (2020). Technologically relevant Bacillus species and microbial safety of West African traditional alkaline fermented seed condiments. Crit. Rev. Food Sci. Nutr. 62, 871–888. doi: 10.1080/10408398.2020.1830026
Owusu-Kwarteng, J., Tano-Debrah, K., Akabanda, F., and Jespersen, L. (2015). Technological properties and probiotic potential of Lactobacillus fermentum strains isolated from West African fermented millet dough. BMC Microbiol. 15, 261. doi: 10.1186/s12866-015-0602-6
Pakwan, C., Chitov, T., Chantawannakul, P., Manasam, M., Bovonsombut, S., and Disayathanoowat, T. (2020). Bacterial compositions of indigenous Lanna (Northern Thai) fermented foods and their potential functional properties. PLoS ONE. 15, e0242560. doi: 10.1371/journal.pone.0242560
Park, K., and Jung, K. (2005). “Fermented soybean products as functional foods: functional properties of doenjang (fermented soybean paste),” in Asian Functional Foods, eds J. Shi, C.-T. Ho, and F. Shahidi (London: CRC press), 555–596. doi: 10.1201/9781420028119.ch20
Park, M. K., Cho, I. H., Lee, S., Choi, H.-K., Kwon, D.-Y., and Kim, Y.-S. (2010). Metabolite profiling of Cheonggukjang, a fermented soybean paste, during fermentation by gas chromatography-mass spectrometry and principal component analysis. Food Chem. 122, 1313–1319. doi: 10.1016/j.foodchem.2010.03.095
Park, S.-K. (2000). Quality assessment of commercial doenjang prepared by traditional method. J. Korean Soc. Food Sci. Nutr. 29, 211–217.
Parkouda, C., Nielsen, D. S., Azokpota, P., Ivette Irène Ouoba, L., Amoa-Awua, W. K., Thorsen, L., et al. (2009). The microbiology of alkaline-fermentation of indigenous seeds used as food condiments in Africa and Asia. Crit. Rev. Microbiol. 35, 139–156. doi: 10.1080/10408410902793056
Parkouda, C., Thorsen, L., Compaoré, C. S., Nielsen, D. S., Tano-Debrah, K., Jensen, J. S., et al. (2010). Microorganisms associated with Maari, a Baobab seed fermented product. Int. J. Food Microbiol. 142, 292–301. doi: 10.1016/j.ijfoodmicro.2010.07.004
Peng, Y., Huang, Q., Zhang, R. H., and Zhang, Y. Z. (2003). Purification and characterization of a fibrinolytic enzyme produced by Bacillus amyloliquefaciens DC-4 screened from douchi, a traditional Chinese soybean food. Comp. Biochem. Physiol. Part B: Biochem. Mole. Biol. 134, 45–52. doi: 10.1016/S1096-4959(02)00183-5
Phromraksa, P., Nagano, H., Boonmars, T., and Kamboonruang, C. (2008). Identification of proteolytic bacteria from Thai traditional fermented foods and their allergenic reducing potentials. J. Food Sci. 73, 189–195. doi: 10.1111/j.1750-3841.2008.00721.x
Popoola, T., Jolaoso, A., and Afolabi, R. (2004). Microbiology of the production of Oso, a condiment made by fermenting seeds of Cathormion altissimum. Trop. Sci. 44, 187–189. doi: 10.1002/ts.166
Popoola, T., Jolaoso, A., and Akintokun, A. (2005). An assessment of the nutritional value of oso-a condiment made by fermenting seeds of Cathormion altissimum. J. Food Technol. 3, 149–151.
Popoola, T. O. S., Jolaoso, A. A., Afolabi, R. O., and Akintokun, A. K. (2007). Microbiological studies on the production of Oso: A condiment made from fermenting seeds of Cathormion altissimum. J. Herb. Spice. Med. Plant. 12, 129–138. doi: 10.1300/J044v12n01_12
Poulsen, A. R., Jonge, N., Nielsen, J. L., Højberg, O., Lauridsen, C., Cutting, S. M., et al. (2018). Impact of Bacillus spp. spores and gentamicin on the gastrointestinal microbiota of suckling and newly weaned piglets. PLoS ONE 13, e0207382. doi: 10.1371/journal.pone.0207382
PraŽnikar, Z. J., Kenig, S., Vardjan, T., Bizjak, M. C., and Petelin, A. (2020). Effects of kefir or milk supplementation on zonulin in overweight subjects. J. Dairy Sci. 103, 3961–3970. doi: 10.3168/jds.2019-17696
Qin, H., Yang, H., Qiao, Z., Gao, S., and Liu, Z. (2013). Identification and characterization of a Bacillus subtilis strain HB-1 isolated from Yandou, a fermented soybean food in China. Food Control 31, 22–27. doi: 10.1016/j.foodcont.2012.10.004
Qin, R., Yu, J., Li, Y., Copeland, L., Wang, S., and Wang, S. (2019). Structural changes of starch–lipid complexes during postprocessing and their effect on in vitro enzymatic digestibility. J. Agric. Food Chem. 67, 1530–1536. doi: 10.1021/acs.jafc.8b06371
Qu, Z., Jiang, A. M., Chen, M. T., Ockerman, H. W., and Basu, L. (2012). Potential starter culture isolated from traditionally fermented YangJiang lobster sauce and protease characteristics research. Food Sci. Technol. Res. 18, 585–591. doi: 10.3136/fstr.18.585
Rashad, M. M., Mahmoud, A. E., Abdou, H. M., and Nooman, M. U. (2011). Improvement of nutritional quality and antioxidant activities of yeast fermented soybean curd residue. Afr. J. Biotechnol. 10, 5504–5513.
Reddy, N., and Pierson, M. (1994). Reduction in antinutritional and toxic components in plant foods by fermentation. Food Res. Int. 27, 281–290. doi: 10.1016/0963-9969(94)90096-5
Reddy, N., Pierson, M., Sathe, S. K., Salunkhe, D., and Beuchat, L. R. (1983). Legume-based fermented foods: their preparation and nutritional quality. Crit. Rev. Food Sci. Nutr. 17, 335–370. doi: 10.1080/10408398209527353
Reddy, N., Pierson, M. D., and Salunkhe, D. (2018). Legume-Based Fermented Foods. London: CRC Press. doi: 10.1201/9781351074001
Redondo-Cuenca, A., Villanueva-Suárez, M. J., and Mateos-Aparicio, I. (2008). Soybean seeds and its by-product okara as sources of dietary fibre. Measurement by AOAC and englyst methods. Food Chem. 108, 1099–1105. doi: 10.1016/j.foodchem.2007.11.061
Rossi, M., Amaretti, A., and Raimondi, S. (2011). Folate production by probiotic bacteria. Nutrients 3, 118–134. doi: 10.3390/nu3010118
Salminen, S., Collado, M. C., Endo, A., Hill, C., Lebeer, S., Quigley, E. M. M., et al. (2021). The international scientific association of probiotics and prebiotics (ISAPP) consensus statement on the definition and scope of postbiotics. Nat. Rev. Gastroenterol. Hepatol. 18, 649–667. doi: 10.1038/s41575-021-00440-6
Sanni, A., Ayernor, G., Sakyi-Dawson, E., and Sefa-Dedeh, S. (2000). Aerobic spore-forming bacteria and chemical composition of some Nigerian fermented soup condiments. Plant Foods Hum. Nutr. 55, 111–118. doi: 10.1023/A:1008147120526
Sanni, A., and Ogbonna, D. (1991). The production ofowoh—a Nigerian fermented seasoning agent from cotton seed (Gossypium hirsutum L.). Food Microbiol. 8, 223–229. doi: 10.1016/0740-0020(91)90054-6
Sanni, A. I., Onilude, A., Fadahunsi, I., Ogunbanwo, S., and Afolabi, R. (2002). Selection of starter cultures for the production of ugba, a fermented soup condiment. Euro. Food Res. Technol. 215, 176–180. doi: 10.1007/s00217-002-0520-3
Sarkar, P., Hasenack, B., and Nout, M. (2002). Diversity and functionality of Bacillus and related genera isolated from spontaneously fermented soybeans (Indian Kinema) and locust beans (African Soumbala). Int. J. Food Microbiol. 77, 175–186. doi: 10.1016/S0168-1605(02)00124-1
Sarkar, P., Tamang, J., Cook, P., and Owens, J. (1994). Kinema—a traditional soybean fermented food: proximate composition and microflora. Food Microbiol. 11, 47–55. doi: 10.1006/fmic.1994.1007
Sarkar, P. K., Jones, L. J., Craven, G. S., and Somerset, S. M. (1997a). Oligosaccharide profiles of soybeans during kinema production. Lett. Appl. Microbiol. 24, 337–339. doi: 10.1046/j.1472-765X.1997.00035.x
Sarkar, P. K., Jones, L. J., Craven, G. S., Somerset, S. M., and Palmer, C. (1997b). Amino acid profiles of kinema, a soybean-fermented food. Food Chem. 59, 69–75. doi: 10.1016/S0308-8146(96)00118-5
Sarkar, P. K., Jones, L. J., Gore, W., Craven, G. S., and Somerset, S. M. (1996). Changes in soya bean lipid profiles during kinema production. J. Sci. Food Agric. 71, 321–328. doi: 10.1002/(SICI)1097-0010(199607)71:3<321::AID-JSFA587>3.0.CO;2-J
Sarkar, P. K., Morrison, E., Tinggi, U., Somerset, S. M., and Craven, G. S. (1998). B-group vitamin and mineral contents of soybeans during kinema production. J. Sci. Food Agric. 78, 498–502. doi: 10.1002/(SICI)1097-0010(199812)78:4<498::AID-JSFA145>3.0.CO;2-C
Sarkar, P. K., and Tamang, J. P. (1995). Changes in the microbial profile and proximate composition during natural and controlled fermentations of soybeans to produce kinema. Food Microbiology, 12, 317–325. doi: 10.1016/S0740-0020(95)80112-X
Sarwar Gilani, G., Wu Xiao, C., and Cockell, K. A. (2012). Impact of antinutritional factors in food proteins on the digestibility of protein and the bioavailability of amino acids and on protein quality. Br. J. Nutr. 108 (Suppl. 2), S315–332. doi: 10.1017/S0007114512002371
Savadogo, A., Tapi, A., Chollet, M., Wathelet, B., Traore, A. S., and Jacques, P. (2011). Identification of surfactin producing strains in Soumbala and Bikalga fermented condiments using polymerase chain reaction and matrix assisted laser desorption/ionization-mass spectrometry methods. Int. J. Food Microbiol. 151, 299–306. doi: 10.1016/j.ijfoodmicro.2011.09.022
Savaiano, D. A., and Hutkins, R. W. (2021). Yogurt, cultured fermented milk, and health: a systematic review. Nutr. Rev. 79, 599–614. doi: 10.1093/nutrit/nuaa013
Shahzad, R., Shehzad, A., Bilal, S., and Lee, I.-J. (2020). Bacillus amyloliquefaciens RWL-1 as a new potential strain for augmenting biochemical and nutritional composition of fermented soybean. Molecules 25, 2346. doi: 10.3390/molecules25102346
Sharma, A., Kumari, S., Wongputtisin, P., Nout, M. J., and Sarkar, P. K. (2015). Optimization of soybean processing into kinema, a bacillus-fermented alkaline food, with respect to a minimum level of antinutrients. J. Appl. Microbiol. 119, 162–176. doi: 10.1111/jam.12826
Sharma, R., Garg, P., Kumar, P., Bhatia, S. K., and Kulshrestha, S. (2020). Microbial fermentation and its role in quality improvement of fermented foods. Fermentation 6, 106. doi: 10.3390/fermentation6040106
Shin, D., and Jeong, D. (2015). Korean traditional fermented soybean products: Jang. J. Ethn. Foods 2, 2–7. doi: 10.1016/j.jef.2015.02.002
Shin, K.-E., Choi, S.-K., Kim, D.-S., and Kim, H.-Y. (2012). Antioxidant compounds and activities of short-term green Gochujang. J. East Asian Soc. Diet. Life 22, 657–666.
Shin, Z.-I., Yu, R., Park, S.-A., Chung, D. K., Ahn, C.-W., Nam, H.-S., et al. (2001). His-His-Leu, an angiotensin I converting enzyme inhibitory peptide derived from Korean soybean paste, exerts antihypertensive activity in vivo. J. Agric. Food Chem. 49, 3004–3009. doi: 10.1021/jf001135r
Singh, T. A., Devi, K. R., Ahmed, G., and Jeyaram, K. (2014). Microbial and endogenous origin of fibrinolytic activity in traditional fermented foods of Northeast India. Food Res. Int. 55, 356–362. doi: 10.1016/j.foodres.2013.11.028
Sohliya, I., Joshi, S., Bhagobaty, R. K., and Kumar, R. (2009). Tungrymbai-A traditional fermented soybean food of the ethnic tribes of Meghalaya. Ind. J. Trad. Knowle. 8, 559-561.
Staudacher, H. M., and Nevin, A. N. (2019). Fermented foods: fad or favourable addition to the diet? Lancet Gastroenterol. Hepatol. 4, 19. doi: 10.1016/S2468-1253(18)30392-3
Sulistyo, J., Taya, N., Funane, K., and Kiuchi, K. (1988). Production of natto starter. Nippon Shokuhin Kogyo Gakkaishi. 35, 278–283.
Sumino, T., Endo, E., Kageyama, A. S., Chihihara, R., and Yamada, K. (2003). Various Components and Bacteria of Furu (Soybean Cheese). J. Cookery Sci. Japan 36, 157–163. doi: 10.11402/cookeryscience1995.36.2_157
Suparthana, I. P., Duniaji, A. S., and Hashimoto, M. (2018). Investigation to traditionally produced soybean fermented food (sere kedele) in gianyar region-bali and molecular identification of bacteria involved in their spontaneous fermentation. Media Ilmiah Teknologi Pangan (Scientific Journal of Food Technology), 5, 66–72.
Suzuki, H., Watabe, J., Takeuchi, H., Tadano, Y., Masuda, S., and Maruta, K. (2004). Effect of Bacillus subtilis C-3102 intakes on the composition and metabolic activity of fecal microflora of humans. J. Intest. Microbiol. 18, 93–99.
Tamang, J. P. (2012). “Plant-based fermented foods and beverages of Asia,” in Handbook of Plant-Based Fermented Food and Beverage Technology, eds Y. H. Hui and E. Özgül Evranuz (London: CRC Press), 49–90.
Tamang, J. P., Cotter, P. D., Endo, A., Han, N. S., Kort, R., Liu, S. Q., et al. (2020). Fermented foods in a global age: East meets west. Compr. Rev. Food Sci. Food Saf. 19, 184–217. doi: 10.1111/1541-4337.12520
Tamang, J. P., Shin, D.-H., Jung, S.-J., and Chae, S.-W. (2016a). Functional properties of microorganisms in fermented foods. Front. Microbiol. 7, 578. doi: 10.3389/fmicb.2016.00578
Tamang, J. P., Tamang, N., Thapa, S., Dewan, S., Tamang, B. M., Yonzan, H., et al. (2012). Microorganisms and nutritional value of ethnic fermented foods and alcoholic beverages of North East India. Indian J. Trad. Know. 11, 7–25.
Tamang, J. P., Thapa, S., Dewan, S., Jojima, Y., Fudou, R., and Yamanaka, S. (2002). Phylogenetic analysis of Bacillus strains isolated from fermented soybean foods of Asia: kinema, chungkokjang and natto. J. Hill Res. 15, 56–62.
Tamang, J. P., Watanabe, K., and Holzapfel, W. H. (2016b). Diversity of microorganisms in global fermented foods and beverages. Front. Microbiol. 7, 377. doi: 10.3389/fmicb.2016.00377
Tangyu, M., Muller, J., Bolten, C. J., and Wittmann, C. (2019). Fermentation of plant-based milk alternatives for improved flavour and nutritional value. Appl. Microbiol. Biotechnol. 103, 9263–9275. doi: 10.1007/s00253-019-10175-9
Tarvainen, M., Fabritius, M., and Yang, B. (2019). Determination of vitamin K composition of fermented food. Food Chem. 275, 515–522. doi: 10.1016/j.foodchem.2018.09.136
Terefe, N. S., and Augustin, M. A. (2020). Fermentation for tailoring the technological and health related functionality of food products. Crit. Rev. Food Sci. Nutr. 60, 2887–2913. doi: 10.1080/10408398.2019.1666250
Terlabie, N. N., Sakyi-Dawson, E., and Amoa-Awua, W. K. (2006). The comparative ability of four isolates of Bacillus subtilis to ferment soybeans into dawadawa. Int. J. Food Microbiol. 106, 145–152. doi: 10.1016/j.ijfoodmicro.2005.05.021
Termote, C., Odongo, N. O., Dreyer, B. S., Guissou, B., Parkouda, C., and Vinceti, B. (2022). Nutrient composition of Parkia biglobosa pulp, raw and fermented seeds: a systematic review. Crit. Rev. Food Sci. Nutr. 62, 119–144. doi: 10.1080/10408398.2020.1813072
Thursby, E., and Juge, N. (2017). Introduction to the human gut microbiota. Biochem. J. 474, 1823–1836. doi: 10.1042/BCJ20160510
Tolhurst, G., Heffron, H., Lam, Y. S., Parker, H. E., Habib, A. M., Diakogiannaki, E., et al. (2012). Short-chain fatty acids stimulate glucagon-like peptide-1 secretion via the G-protein–coupled receptor FFAR2. Diabetes 61, 364–371. doi: 10.2337/db11-1019
Tsukamoto, Y., Ichise, H., Kakuda, H., and Yamaguchi, M. (2000). Intake of fermented soybean (natto) increases circulating vitamin K2 (menaquinone-7) and γ-carboxylated osteocalcin concentration in normal individuals. J. Bone Miner. Metab. 18, 216–222 doi: 10.1007/s007740070023
Uaboi-Egbenni, P., Okolie, P., Sobande, A., Alao, O., Teniola, O., and Bessong, P. (2009). Identification of subdominant lactic acid bacteria in dawadawa (a soup condiment) and their evolution during laboratory-scale fermentation of Parkia biglobosa (African locust beans). Afr. J. Biotechnol. 8, 7241–7248.
Urua, I. S., Uyoh, E. A., Ntui, V. O., and Okpako, E. C. (2013). Effect of processing on proximate composition, anti-nutrient status and amino acid content in three accessions of African locust bean [Parkia biglobosa (jacq.) benth]. Int. J. Food Sci. Nutr. 64, 94–102. doi: 10.3109/09637486.2012.704903
Vary, P. S. (1994). Prime time for Bacillus megaterium. Microbiology 140 (Pt. 5), 1001–1013. doi: 10.1099/13500872-140-5-1001
Visessanguan, W., Benjakul, S., Potachareon, W., Panya, A., and Riebroy, S. (2005). Accelerated proteolysis of soy proteins during fermentation of thua-nao inoculated with Bacillus subtilis. J. Food Biochem. 29, 349–366. doi: 10.1111/j.1745-4514.2005.00012.x
Vitreschak, A. G., Rodionov, D. A., Mironov, A. A., and Gelfand, M. S. (2002). Regulation of riboflavin biosynthesis and transport genes in bacteria by transcriptional and translational attenuation. Nucleic Acids Res. 30, 3141–3151. doi: 10.1093/nar/gkf433
Walsh, A. M., Crispie, F., Kilcawley, K., O'Sullivan, O., O'Sullivan, M. G., Claesson, M. J., et al. (2016). Microbial succession and flavor production in the fermented dairy beverage kefir. Msystems 1, e00052–e00016. doi: 10.1128/mSystems.00052-16
Wang, D., Wang, L.-,j., Zhu, F.-,x., Zhu, J.-,y., Chen, X. D., Zou, L., et al. (2008). In vitro and in vivo studies on the antioxidant activities of the aqueous extracts of Douchi (a traditional Chinese salt-fermented soybean food). Food Chem. 107, 1421–1428. doi: 10.1016/j.foodchem.2007.09.072
Wang, H., Meng, F., Yin, L., Cheng, Y., Lu, A., and Wang, J. (2016). Changes of composition and angiotensin i-converting enzyme-inhibitory activity during douchi fermentation. Int. J. Food Propert. 19, 2408–2416. doi: 10.1080/10942912.2015.1040122
Wang, J., and Fung, D. Y. (1996). Alkaline-fermented foods: a review with emphasis on pidan fermentation. Crit. Rev. Microbiol. 22, 101–138. doi: 10.3109/10408419609106457
Watanabe, F., Yabuta, Y., Bito, T., and Teng, F. (2014). Vitamin B12-containing plant food sources for vegetarians. Nutrients 6, 1861–1873. doi: 10.3390/nu6051861
Wegh, C. A. M., Geerlings, S. Y., Knol, J., Roeselers, G., and Belzer, C. (2019). Postbiotics and their potential applications in early life nutrition and beyond. Int. J. Mol. Sci. 20, 4673. doi: 10.3390/ijms20194673
Wei, Q., and Chang, S. K. (2004). Characteristics of fermented natto products as affected by soybean cultivars. J. Food Proc. Preserv. 28, 251–273. doi: 10.1111/j.1745-4549.2004.23047.x
Wolfe, B. E., and Dutton, R. J. (2015). Fermented foods as experimentally tractable microbial ecosystems. Cell 161, 49–55. doi: 10.1016/j.cell.2015.02.034
Xu, X., Liu, H., and Zhou, Y. (2012). “Study on the meitauza production from okara by Actinomucor elegans and Zymomonas mobilis,” in Information Technology and Agricultural Engineering, eds E. Zhu and S. Sambath (Berlin; Heidelberg: Springer), 329–336.
Yongsmith, B., Kitpreechavanich, V., Tangjitjaroenkun, J., and Krusong, W. (2016). “Bioenrichment of Vitamin B 12 in Fermented Foods,” in Functional Properties of Traditional Foods (Boston, MA: Springer), 17–37. doi: 10.1007/978-1-4899-7662-8_3
Yoo, S. K., Cho, W. H., Kang, S. M., and Lee, S. H. (1999). Isolation and identification of microorganisms in Korean traditional soybean paste and soybean sauce. Korean J. Appl. Microbiol. Biotechnol. 27, 113–117.
Zhang, J.-H., Tatsumi, E., Ding, C.-H., and Li, L.-T. (2006). Angiotensin I-converting enzyme inhibitory peptides in douchi, a Chinese traditional fermented soybean product. Food Chem. 98, 551–557. doi: 10.1016/j.foodchem.2005.06.024
Zhu, Y., Fan, J., Cheng, Y., and Li, L. (2008). Improvement of the antioxidant activity of Chinese traditional fermented okara (Meitauza) using Bacillus subtilis B2. Food Control 19, 654–661. doi: 10.1016/j.foodcont.2007.07.009
Keywords: sustainability, Bacillus spp., nutrition, legume, fermentation, Africa, Asia
Citation: Owusu-Kwarteng J, Agyei D, Akabanda F, Atuna RA and Amagloh FK (2022) Plant-Based Alkaline Fermented Foods as Sustainable Sources of Nutrients and Health-Promoting Bioactive Compounds. Front. Sustain. Food Syst. 6:885328. doi: 10.3389/fsufs.2022.885328
Received: 28 February 2022; Accepted: 28 April 2022;
Published: 14 June 2022.
Edited by:
Santosh Kumar, Central Institute of Technology Kokrajhar, IndiaReviewed by:
Siddhartha Singha, Indian Institute of Technology Guwahati, IndiaAvik Mukherjee, Central Institute of Technology, Kokrajhar, India
Copyright © 2022 Owusu-Kwarteng, Agyei, Akabanda, Atuna and Amagloh. This is an open-access article distributed under the terms of the Creative Commons Attribution License (CC BY). The use, distribution or reproduction in other forums is permitted, provided the original author(s) and the copyright owner(s) are credited and that the original publication in this journal is cited, in accordance with accepted academic practice. No use, distribution or reproduction is permitted which does not comply with these terms.
*Correspondence: James Owusu-Kwarteng, amFtZXMub3d1c3Uta3dhcnRlbmdAdWVuci5lZHUuZ2g=