- 1School of Biology, Faculty of Biological Sciences, University of Leeds, Leeds, United Kingdom
- 2Department of Health Studies and Centre for Ecology, Evolution and Behaviour, School of Life Sciences and the Environment, Royal Holloway, University of London, Egham, United Kingdom
- 3School of Life Sciences, Keele University, Keele, United Kingdom
- 4Zambia Agriculture Research Institute, Lusaka, Zambia
- 5Food, Agriculture and Natural Resources Policy Analysis Network (FANRPAN), Pretoria, South Africa
- 6International Centre of Insect Physiology and Ecology, Nairobi, Kenya
- 7Department of Sustainable Agriculture, Biodiversity and Ecosystem Management, School of Life Sciences and Bio Engineering, The Nelson Mandela African Institution of Science and Technology, Arusha, Tanzania
- 8Department of Zoology and Entomology, University of the Free State, Bloemfontein, South Africa
- 9Sustainability Research Institute, School of Earth and Environment, University of Leeds, Leeds, United Kingdom
- 10Lancaster Environment Centre, Lancaster University, Lancaster, United Kingdom
Agricultural productivity can be increased sustainably in sub-Saharan Africa (SSA) by reducing crop losses due to insect pest damage. As an alternative to environmentally-damaging chemical pesticides, biological control interventions and botanical pesticides show potential to achieve both high yields and profits. However, synthesized information of their performance and understanding of their adoption among smallholder farmers is limited. Here, 173 studies of biological control interventions and botanical pesticides of insect pests for 35 crops from 20 sub-Saharan countries from 2005 to 2021 were systematically reviewed. Drawing on published datasets, we found that cereals, particularly maize, were the most studied crop (59%). Research on botanical pesticides constituted 32% of the studies, followed by augmentation/introduction biocontrol (29%), and push-pull (21%). Studies evaluating the technical performance of biocontrol interventions dominated (73%), with a regional clustering of push-pull studies in Kenya. Few studies investigated each intervention on each crop type, across different farming contexts and scales, highlighting an urgent need for landscape-scale studies to elucidate land-use impacts on biocontrol effectiveness. Limited evidence also exists on the synergistic effects of biocontrol on multiple ecosystem services and on non-target/beneficial organisms. We found an absence of interdisciplinary studies that addressed the wider indirect benefits of not using chemical pesticides, the social-economic outcomes, and barriers to adoption by farmers, which we argue are necessary to identify pathways to greater adoption and to support policy advocacy of biocontrol interventions in SSA.
Introduction
By 2050 the population of sub-Saharan Africa is predicted to increase by 86%, with both native and invasive crop pests being one of the biggest threats to food production (Oerke and Dehne, 2004; Rosegrant et al., 2009). From 1970 to the present day biological invasions have cost sub-Saharan Africa's economy between $18.2 and $80 billion, incurred mainly by a small number of insects species including Chilo partellus (Spotted stem borer) Tuta absoluta (Tomato leafminer) and Spodoptera frugiperda (Fall armyworm) (Diagne et al., 2021). Among the 21 economically important lepidopteran stemborer species in Africa (Maes, 1997), indigenous species such as Busseola fusca (maize stalk borer) and Chilo partellus (Spotted stem borer) are highly devastating. The larval stages of stemborer pests feed on crop plants resulting in up to 80% yield loss depending on the crop type (Kfir et al., 2002). Recently, the fall armyworm has invaded Africa and spread rapidly to 21 countries (Stokstad, 2017), causing devastating damage to maize with estimated crop losses worth $3 billion a year (Stokstad, 2017). While smallholder farmers have always encountered significant crop losses due to native crop pests, the impact of this invasive pest has highlighted a critical need to develop effective and sustainable methods of pest control.
The most common option for pest control available to smallholder farmers is the application of chemical pesticides, which is an unsustainable solution. Chemical pesticides are often too expensive for smallholder farmers and the lack of access to protective equipment puts them at risk of harmful exposure to pesticides that are being increasingly restricted in the EU (Pesticides Action Network Europe, 2020). Furthermore, chemical pesticides have unintended negative impacts on beneficial non-target species, pesticide resistance is frequently observed (Hemingway et al., 2002), and environmental contamination (Nesser et al., 2016) puts people and livestock at risk from contaminated food and crop residues (Jepson et al., 2020).
In addition to the substantial scale of crop losses due to insect pests, SSA's economy is greatly dependent on agriculture, making the region most vulnerable to the impact of insect pest invasions (Paini et al., 2016). Therefore, there is an urgent need to reduce the impact of pests which would increase productivity and contribute to achieving a more sustainable and resilient food system. Increased agricultural productivity could benefit livelihoods and incomes of smallholder farmers and contribute to reducing hunger, thus playing a critical role in attaining sustainable development goals, such as food security (SDG2-3) and improved livelihoods (SDG1). Development of effective and sustainable interventions of pest control that are compatible with existing farming systems and adaptable to future challenges, including climate extremes and invasive pests in SSA, is needed.
Evidence reveals that biological control methods (hereafter biocontrol), defined as any practice that utilizes natural enemies of pests for the control of pest populations, have the potential to reduce the incidence of pests and enhance yields sustainably (Tembo et al., 2018). Historically, smallholder farmers in SSA have adopted indigenous pest management methods, such as intercropping and crop rotation, although methods employed are typically site-specific due to heterogeneous farm conditions (Abate et al., 2000). Numerous classical biological control interventions have been documented in Africa from the 1980s (Neuenschwander et al., 2003). For example, Phenacoccus manihoti (Cassava mealybug) has been successfully controlled by the introduction of Anagyrus lopezi (Encyrtid wasp), which reduced crop losses by over 90% (Neuenschwander, 2004). Other approaches, such as push-pull technology (Midega et al., 2018) and plant-derived botanical pesticides (Grzywacz et al., 2008), have been shown to be effective against a range of crop pests. However, there is little evidence of the wide-scale adoption of biocontrol innovations by the estimated 33 million smallholder farms in SSA, which contribute up to 70% of the food supply (IFAD, 2020).
There is a clear need to upscale the application of biocontrol strategies to replace chemical pesticides. However, despite recent calls for transdisciplinary and system thinking research on innovations for pest management and crop protection (Schut et al., 2014; Rodenburg et al., 2015), the biophysical and socio-economic constraints and implications on biocontrol adoption are unclear. Recent research in SSA (Smith et al., 2021) has argued for the need to move beyond narrow and simplistic metrics, and binary notions of adopters and non-adopters, to embrace innovation systems thinking, and incorporate ethnographic and qualitative methods to help better understand how farmers innovate and access beneficial outcomes from changes in agricultural practices.
However, to date, development and knowledge production about biocontrol is largely focused on a small number of research centers. For example, the International Centre of Insect Physiology and Ecology (ICIPE) and some other Consultative Group for International Agricultural Research (CGIAR) centers have strong connections with farmer communities, but these are regionally highly localized. Often, knowledge transfer and communication have been limited to scientific journals that remain disconnected from the intended beneficiaries (i.e., the smallholder farmers). There is even less clarity about the ways in which promoted biocontrol interventions interact with local farming knowledges, the multifaceted processes of on-farm decision making, and the wider innovation landscape within which farmers operate (Hermans et al., 2021; Smith et al., 2021).
A synthesis of the state of knowledge of biocontrol interventions in SSA is a crucial first step to understanding the pathways to upscaling biocontrol approaches. Here, we identify research needs that would further improve understanding of the efficacy of biocontrol methods by synthesizing knowledge for the following questions: (1) what evidence is available on the performance of biocontrol interventions and botanicals on all crop types and farming systems in sub-Saharan Africa?; (2) what evidence is available of their adoption by farming communities?; (3) how do ecological and socio-economical approaches compare in identifying what intervention approaches work, where, for whom and why?; and (4) what are the constraints to adoption and the opportunities for upscaling of biocontrol interventions and botanical pesticides in sub-Saharan Africa?
Methods
Search strategy
We systematically reviewed the scientific and grey literature to collate empirical studies that examined the adoption and performance of biological control interventions in sub-Saharan Africa. We focused on the sub-Saharan region which has a large population of smallholder farmers depending on local food production, with substantial incidence of insect pest outbreaks and crop damage threatening food security.
The literature searches were completed using electronic ISI Web of Science and Scopus in December 2020, with updates from the searches captured from 2005 until 30th April 2021. We followed standard systematic review protocols (Pullin and Stewart, 2006) and formulated the review questions by applying the PICO elements: Population (insect pests of crops), Intervention (biological control intervention/botanicals), Comparator(s) (absence of intervention, application of chemical pesticides), and Outcome (technical performance, measures of adoption, economic measures).
We used a combination of search terms based on the PICO elements and related them to a wide range of biological control techniques and insect pests (e.g., “biocontrol”, intercrop*”, “botanical pesticide*”, “armyworm”), agricultural settings (e.g., “agri*”, “farm*”) and the target geographical location (e.g., “sub-Saharan Africa”, “Southern Africa”). A wildcard (*) was used to capture multiple word endings (e.g., agri* to capture agriculture, agricultural) (Supplementary Table S1 for full search string). The search string was applied under Topic subject which covers Title, Abstract and Keywords. We captured grey literature by conducting additional searches on Google and Google Scholar and by searching websites of relevant institutions (Supplementary Table S2). Furthermore, we contacted relevant research groups to obtain unpublished data. We tested the performance of the search against 15 articles previously identified as relevant by the authors (Supplementary Table S3).
All results were exported into Mendeley 1.19.8. and a separate file was created for each database search. When searches were completed, the two database libraries were combined into one and the number of articles captured was recorded. All duplicates were removed using the automatic function in the Mendeley software and the test studies that were not retrieved by the systematic search were added to the final database. The queries in the two databases yielded 5,742 articles. We used the Reporting standards for Systematic Evidence Syntheses in environmental research (ROSES) to create the article database (Haddaway et al., 2018). We conducted four filtering phases: searching, screening, coding, and synthesis (Figure 1).
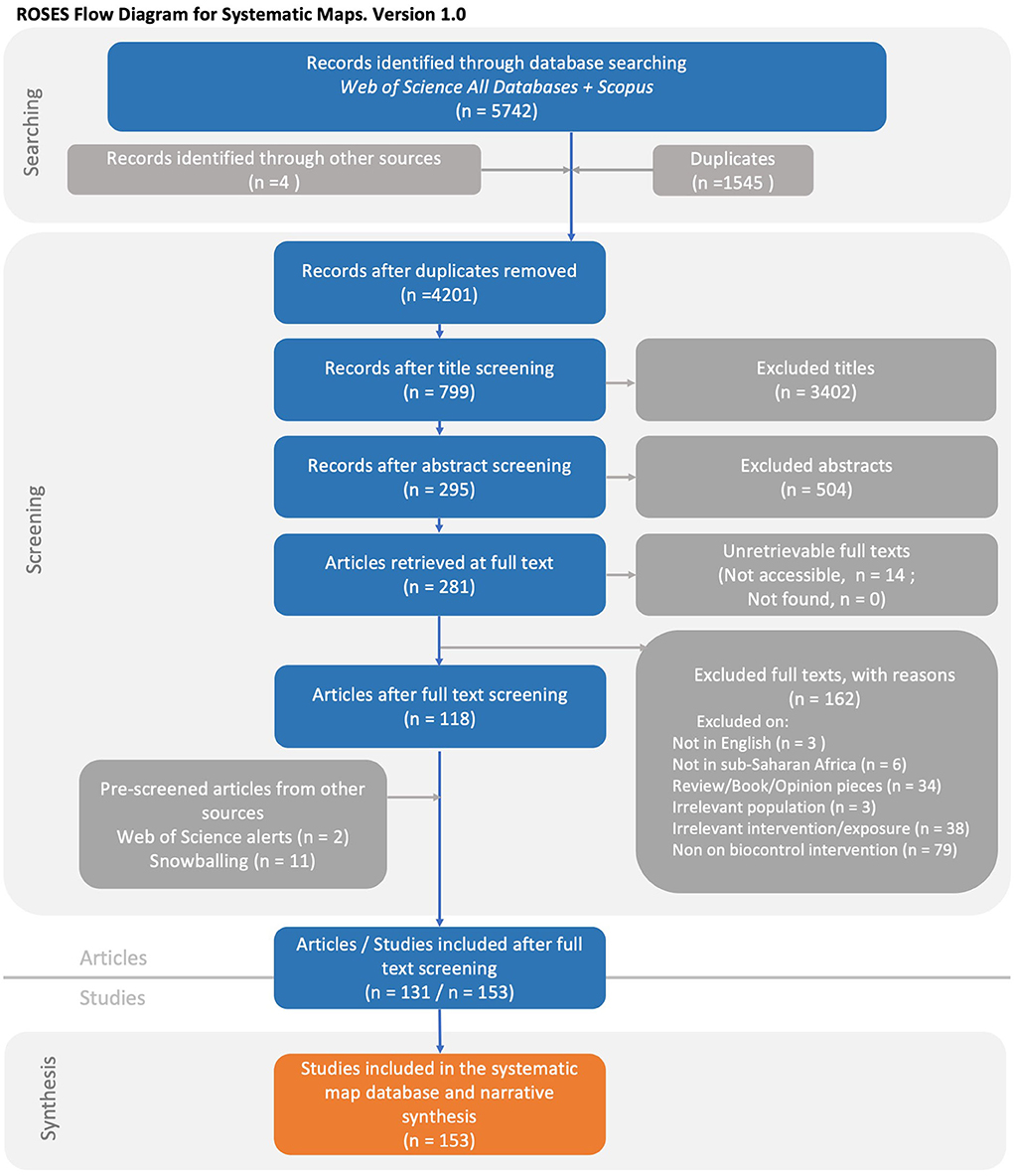
Figure 1. ROSES flow diagram of the literature included and excluded at each step of the systematic map process.
Article screening and eligibility criteria
The searching phase ensured the first filtering of the databases where duplicates (n = 1,545) were removed. The screening strategy was developed amongst all authors and was carried out at a title, abstract and full text level. The title and abstract screening consisted of an overview of an article's metadata including type of document, subject area, authors, and geographic location of studies. During this phase we identified and removed 3,902 articles at title level and 543 at abstract level. Additionally, we removed 14 articles for which we could not access the full text. To determine the consistency of the eligibility decision, we validated the agreement between authors using a Cohen's kappa coefficient (Cohen, 1960) at the title screening stage. Three authors analyzed a random sub-sample of 100 titles independently and agreement was tested using Cohen's coefficient. The Kappa Analysis showed a “moderate” agreement (kappa = 0.49), which was considered acceptable (Edwards et al., 2002).
The titles and abstracts were screened manually by the lead author in a MS Excel file with a separate sheet for each stage of the screening, using the eligibility criteria to determine which studies to include. At the abstract stage, the reasons for exclusion were recorded as: irrelevant subject (the study was not relevant to agriculture and/or biocontrol, e.g., biotechnologies), irrelevant population (the target pest of the study was a non-insect pest e.g., weeds, viruses, fungi, diseases, storage pests were also excluded as the focus of this review was on pre-harvest pests, which are the most common target of chemical pesticide applications), irrelevant sources (the study did not contain original research, was a review, a book or conference paper), irrelevant intervention (the study did not evaluate a field application of a biocontrol intervention, e.g., chemical pesticides or laboratory experiments), irrelevant geographic location (the study was carried out outside sub-Saharan Africa), or irrelevant language (the study was written in a language other than English). When there was uncertainty, the study was included at this stage for further analysis at full text level. A total of 305 studies were selected for full-text screening. All studies at the full text stage were screened against the following criteria:
a) Studies that evaluated a quantitative or qualitative measure (i.e., agroecological measurements, household interviews, economic evaluations) of the effectiveness and/or adoption of biocontrol interventions in agroecosystems were included. This included studies that measured either the effectiveness of biocontrol interventions in controlling pest populations and/or studies that investigated the perception, conditions or constraints to the adoption of biocontrol interventions.
b) A biological control intervention was defined as any practice that utilizes natural enemies of pests for the control of pest populations. These include augmentation, introduction, or inoculation with natural enemies (i.e., predators, parasitoids, entomopathogenic fungi, nematodes, viruses, bacteria), and conservation biological control. The latter, defined as the manipulation of habitat to enhance reproduction, survival and efficacy of natural enemies (Amoabeng et al., 2020), was also included and divided into the subcategories of intercropping, field margins and push-pull. We included any intercropped plants including beans, cereal, vegetables and roots. Push-pull was defined as a type of intercropping strategy using repellent “push” plants and trap “pull” plants to manipulate the distribution and abundance of insect pests and/or natural enemies (Khan and Pickett, 2008). Botanical pesticides, defined as substances derived from natural materials (i.e. plant extracts) (Sporleder and Lacey, 2013), were included. Studies exclusively focusing on the evaluation of chemical pesticides were excluded. Studies that explored the effect of distance to natural habitat, non-crop habitat and/or landscape complexity on the delivery of biocontrol ecosystem services to the crop were included and coded as “landscape effect”.
c) We included only studies on interventions targeting insect pests in sub-Saharan Africa due to their widespread presence and the substantial crop and economic damage they cause in this food insecure region. Studies focusing on crop diseases were excluded.
d) Studies that investigated post-harvest/storage pests were excluded to maintain a manageable scope of the study. Studies performed in a laboratory were also excluded as they did not provide contextual information useful for informing strategic ways toward upscaling and did not allow an analysis of the socio-economic dimensions of adoption.
e) Only studies published in the English language and performed in any country in the sub-Saharan region were included. Purely descriptive publications, such as opinion pieces, conference abstracts and book chapters, were excluded. Relevant reviews were not included in the map, but were screened for primary studies that met the inclusion criteria.
f) Studies that did not provide a clear definition of biocontrol practice (either a technical/agronomic definition or farmer/landowner's own definition) were excluded.
g) To identify the current constraints and opportunities to the adoption of biological control interventions, a date restriction was set to the year 2005 and later.
Screening at all levels was performed by the lead author to ensure consistency in the application of the inclusion/exclusion criteria. After reading the article the decision was recorded in the appropriate MS Excel sheet and the reasons for exclusion were recorded. When there was uncertainty on the inclusion of a study, this was discussed with at least one of the co-authors.
Data coding strategy and data synthesis
The data coding strategy was developed by all authors and tested on the studies in the test list. For all the articles that passed the three screening stages, key wording was used to describe, categorize, and code the studies. These included bibliographic and geographic information, such as author(s), title, affiliation, publication name, year, research discipline, country, latitude and longitude, site name and numbers, and duration of the study. We recorded the affiliation of the lead author as either “university” or “non-university research institute”. Research disciplines were defined as “agroecological” “social” “economic” or a combination of these disciplines. All the coded variables extracted from the studies and their definitions are listed in Supplementary Table S4. Furthermore, we screened the text for information that could answer one or all of the three broad questions: (a) What is biological control (i.e., is the practice clearly defined and described)?, (b) What biological control and botanical pesticide approaches do or do not work (i.e., how biological control effectiveness/adoption is measured)?, (c) Where do biological control interventions work, why and for whom (i.e., the context or determinant factors for the success or adoption of biological control approaches are considered or discussed)?. This information was recorded narratively.
We extracted 533 measurements of effectiveness of biocontrol interventions from 109 studies. Effectiveness was measured as pest incidence, pest damage, yield, natural enemy abundance, parasitism intensity and infestation rate. This also included the measurement of a negative control (i.e., compared with untreated/monocropping) and/or positive control (i.e., compared with chemical pesticides). Means were pooled and confidence limits (95%) were calculated for these pooled means.
Results
Of the 5742 articles identified, 149 met the inclusion criteria for the final systematic map (Supplementary Table S5). Fifteen of these articles had multiple study sites and/or investigated multiple biocontrol interventions and were included as separate records, hereafter referred to as “studies.” This resulted in a total of 173 studies included in the final map (Figure 1).
Overview of evidence
All studies came from peer reviewed journal articles with the majority being published in Crop Protection (50%). The countries in which most studies were carried out were Kenya, Ethiopia, Nigeria, Tanzania, and Uganda, with Kenya accounting for 25% of the studies (Figure 2). The remaining studies were clustered in Western Africa (e.g., Benin, Ghana, Cameroon, and Niger) and Southern Africa (e.g., Zambia, Zimbabwe, Malawi and South Africa) (Figure 2). Studies were predominantly affiliated with non-academic research institutions (57%) including ICIPE, the International Crop Research Institute for the Semi-Arid Tropics (ICRISAT), the International Institute of Tropical Agriculture (IITA), Centre for Ecological Research and Forestry Applications (CREAF) and governmental agriculture departments. The remaining 43% were affiliated with African and international universities.
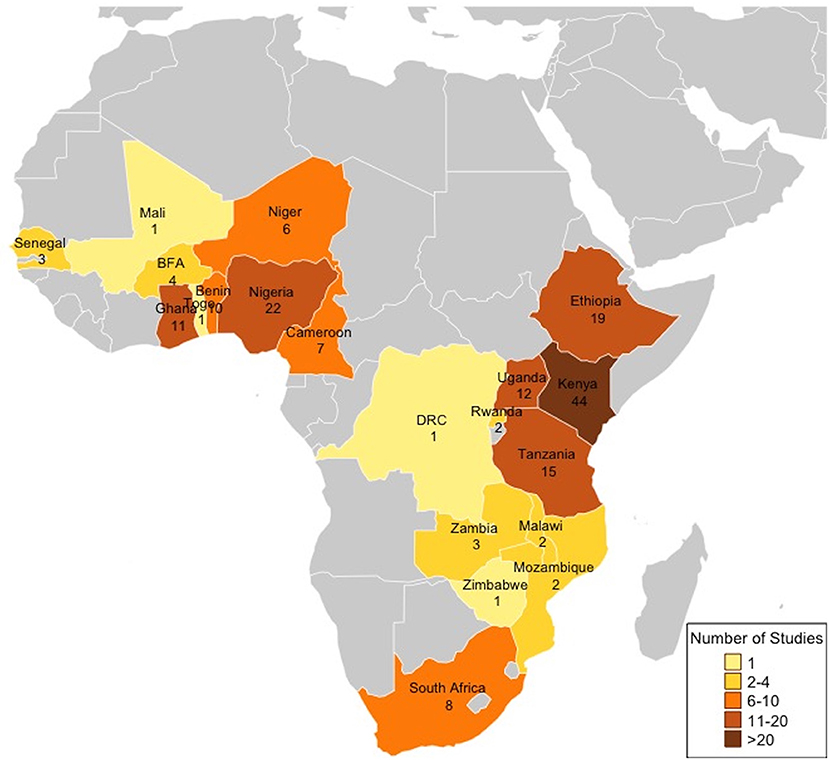
Figure 2. Distribution map of the countries included in the systematic map; color coded by number of studies recorded per country.
Studies were performed across a variety of spatial scales, though mostly on multiple farms (40%). The most common combination was “multiple farm” and “within field”, i.e., the study evaluated a within-field specific biocontrol intervention and compared the results across several farms in different areas (25%). Thirty-six multiple-farm studies (20%) were also coded as “landscape scale” as they assessed the efficacy of biocontrol interventions across agroecological or climatic regions of a country. We found only eight “whole farm” scale studies, where the effect of a biocontrol intervention was measured on pest populations across the whole farm. Most studies concerned smallholder farms, representative of large parts of the SSA agricultural system, while 32% of studies were carried out on research farms.
Interventions studied
Biocontrol interventions were largely studied in cereal crops (59%), specifically maize (39%) (Figure 3A; Supplementary Table S6), followed by vegetable crops (25%), particularly cabbage, fruit crops (14%), comprising mostly mango and tomato, and legumes (13%). Cash crops (e.g., coffee) and nuts were least studied (Figure 3A). Geographically, Kenya accounted for most studies on push-pull (53%), where through ICIPE the push-pull technique was developed and most actively trialed.
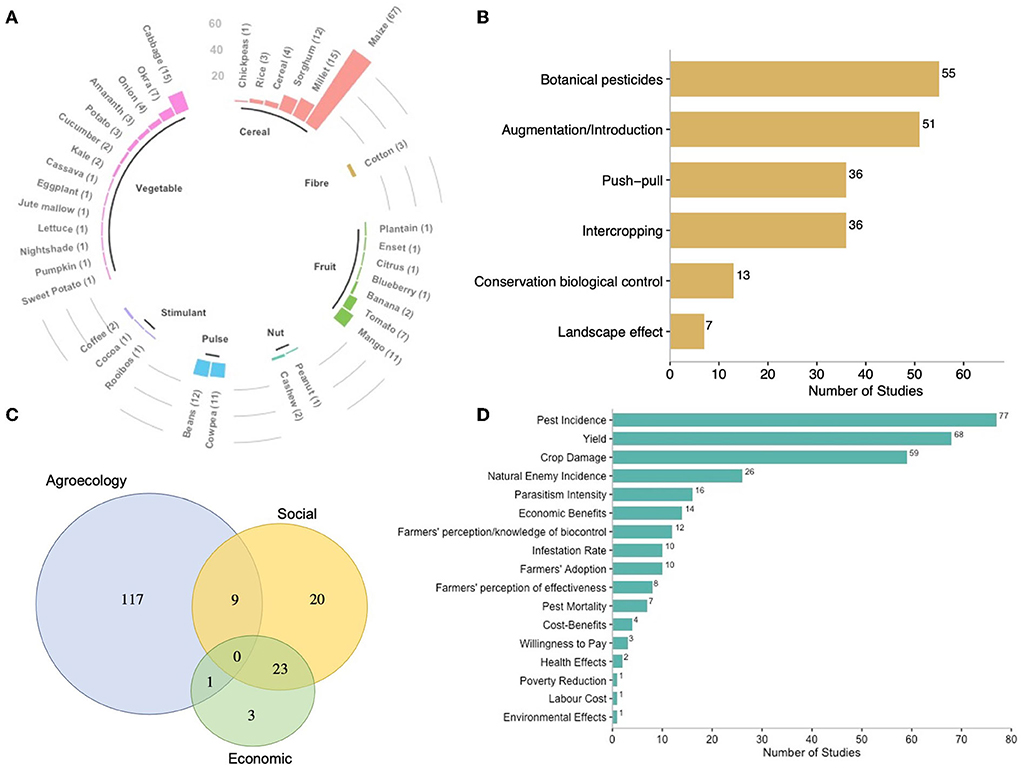
Figure 3. Overview of the 173 studies on biocontrol intervention in SAA. Number of studies by (A) crop type, (B) biocontrol intervention, (C) discipline, and (D) measure of outcome. The total number of studies is at the end of each column. The overall number exceeds 139 as some studies evaluated more than one biocontrol intervention, in more than one country and/or more target pests. Note that “Conservation biological control” refers to studies that examined those biocontrol practices as a broad umbrella of interventions. “Landscape effect” refers to studies that explored the effect of distance to natural habitat and/or landscape complexity on the delivery of biocontrol ecosystem services to the crop.
Amongst all interventions tested, botanical pesticides dominated (32%), followed by augmentation/introduction biocontrol (29%). Push-pull technology and intercropping were each assessed in 21% of studies (Figure 3B), followed by the inclusion of field margins as a potential source of biocontrol agents (8%). Studies exploring the effect of distance to natural habitat, non-crop habitat and/or landscape complexity on the delivery of biocontrol (“landscape effect”) constituted just 4% of total studies. A summary of the interventions tested, and their definitions is available in Table 1.
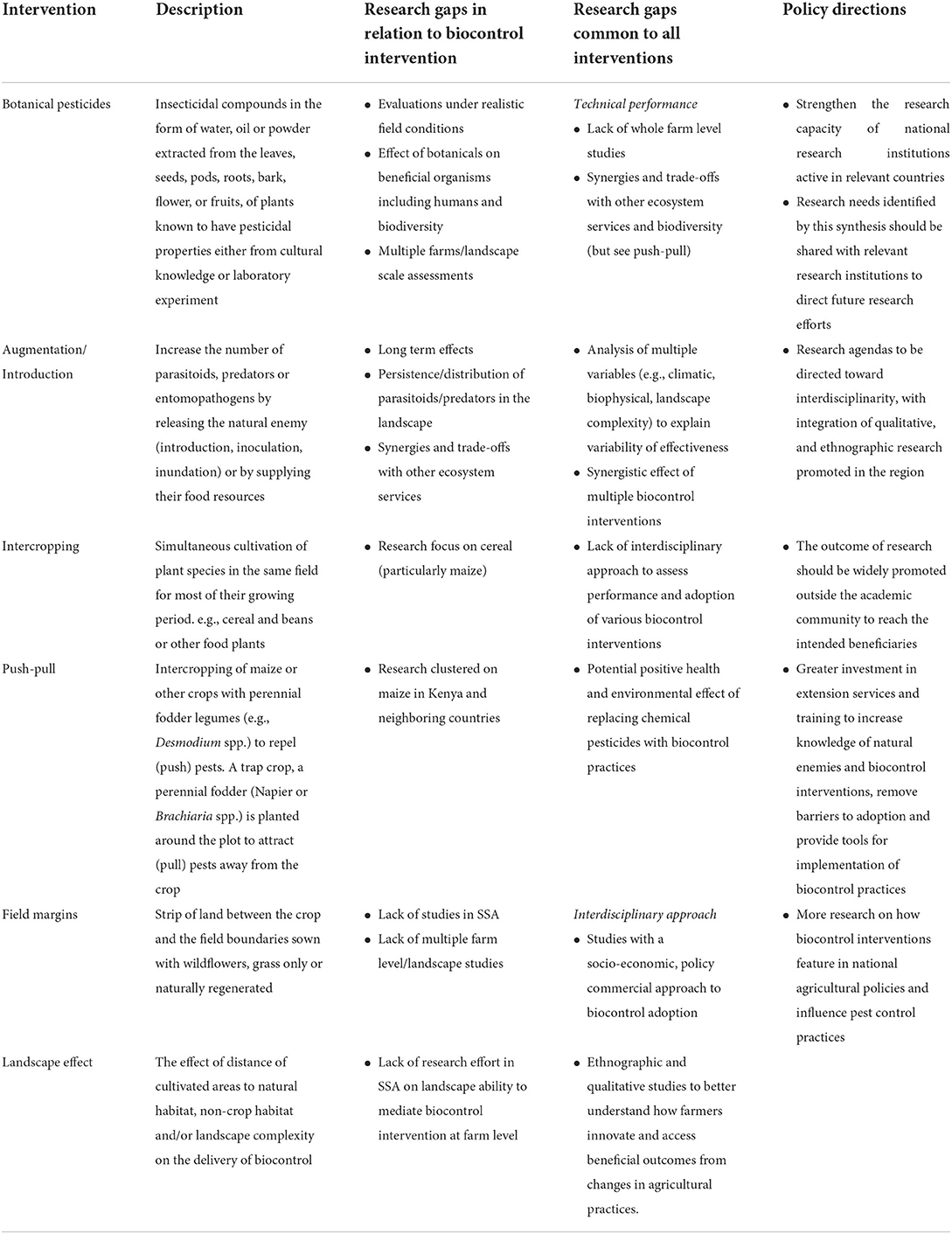
Table 1. List of biocontrol interventions reviewed for sub-Saharan Africa and research gaps identified in this review.
We found that all interventions targeted a wide range of important insect pests, although the most targeted were those that were pests of staple crops, including the fall armyworm (Spodoptera frugiperda) (18%), Diamondback moth (Plutella xylostella) (6%), Crambid cereal stemborer (Chilo partellus) (6%) and Maize stemborer (Busseola fusca) (5%), followed by fruit flies (8%) and aphids (7%) (Supplementary Table S7).
Biocontrol and botanicals performance: What is the evidence that the intervention works?
We considered the performance of biocontrol and botanicals in both agronomic (e.g., technical performance, farm economics) and socioeconomic terms (e.g., intensity of adoption, farmers' perception of performance, economic benefits).
Technical performance
Agroecological studies performing manipulative experiments to measure the effectiveness of biocontrol interventions dominated the literature (73%) (Figure 3C). The most common measures of success were pest incidence, measured in 45% of all studies captured, followed by crop yield (39%) and pest damage incidence (34%) (Figure 3D). Other quantitative metrics of biocontrol efficacy included parasitism intensity (9%), pest infestation rate (6%) and pest mortality (4%). The incidence of natural enemies was measured in 15% of studies, mostly in combination with other ecological metrics to quantify the impact of botanical pesticides, field margins and intercropping.
Across all studies, biocontrol interventions reduced pest damage in comparison to untreated crops (Figure 4A). For example, in intercropped fields pest damage was on average 9% as opposed to 14% in untreated crops. All biocontrol interventions achieved greater yield than untreated crops or monocropping (Figure 4B). Chemical pesticides appeared to perform better against most biocontrol interventions, but with some exceptions. Augmentation/Introduction of natural enemies showed a greater performance than both negative and positive controls (i.e., chemical pesticides) with 44, 56, and 66% pest damage respectively (Figure 4A). Augmentative interventions also produced on average 8 t/ha compared to 2 t/ha following chemical pesticide applications (Figure 4B). Chemical pesticides were not consistently measured against biocontrol across the literature, and for some interventions (e.g., push-pull) we found no evidence of how they compared to synthetic pesticides. Conversely, we found 95 yield performance comparisons between botanical and chemical pesticides, which showed similar yields across most crop types (Figure 4C). Furthermore, compared to chemical pesticides, no study examined the indirect impacts of biocontrol interventions, such as the potential for reduced environmental contamination and limited effects on non-target species.
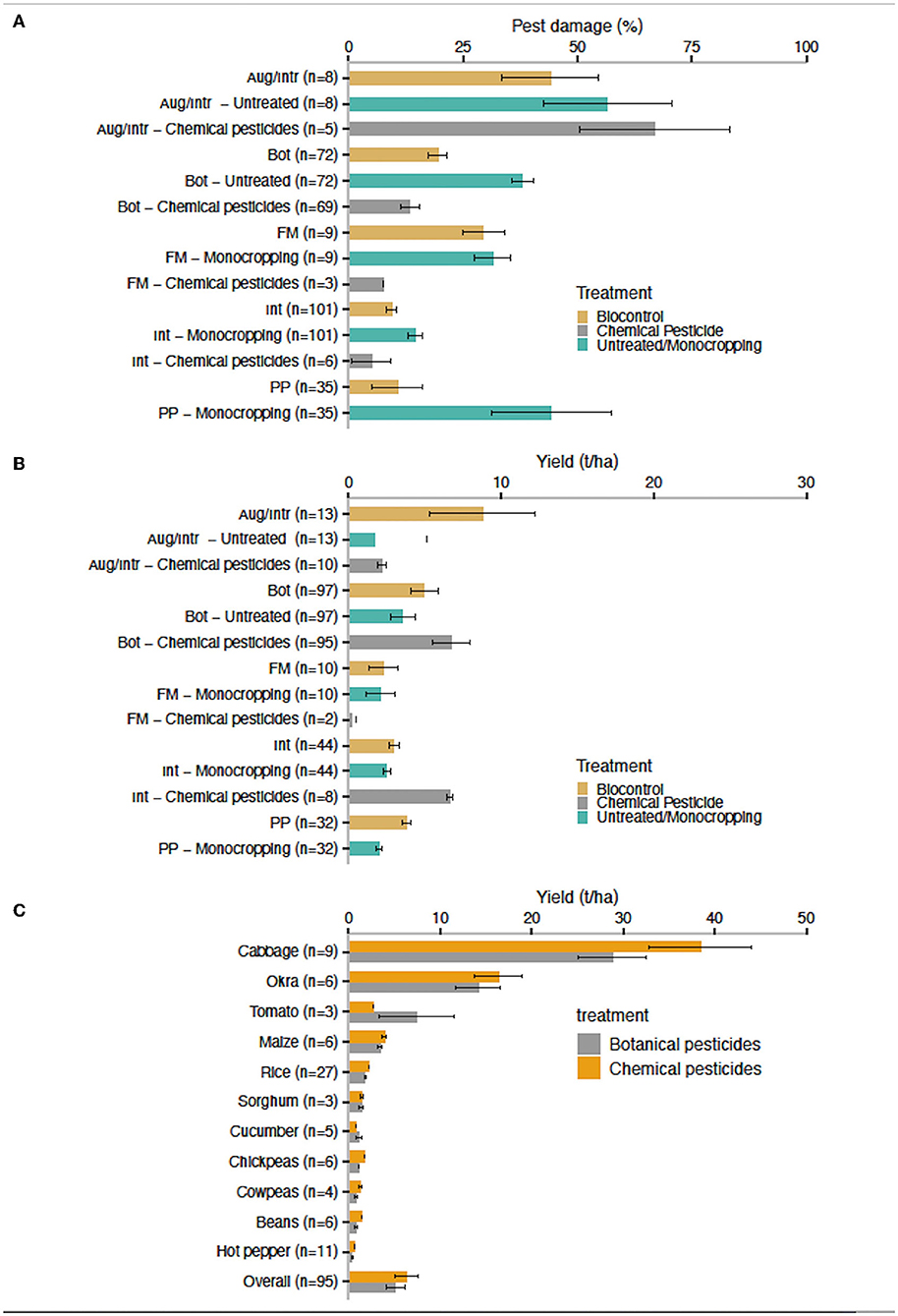
Figure 4. Comparative changes in (A) pest damage, (B) yield across biocontrol intervention, and (C) comparative changes in yield between botanical and chemical pesticides. The means, 95% confidence intervals and n (that is the number of times that intervention was found in the 149 articles) are presented. Biocontrol performances are dependent on numerous factors, including within study conditions, which can result in heterogeneity between studies.
Socioeconomic performance
Very few studies focused on either the social (17%) or socioeconomic (13%) performance of biocontrol interventions, and only three studies focused entirely on economic benefits (Figure 3C). Social studies used structured questionnaires and interviews to measure farmers' knowledge of natural enemies and biocontrol interventions (7%), farmers' perception of biocontrol effectiveness (5%), and adoption intensity (6%) (Figure 3C). For example, one study (Tambo et al., 2020) conducted interviews with farmers to assess their perception of the effectiveness of a number of biocontrol interventions against the fall armyworm. They found that about 80% of farmers across Ghana, Rwanda, Uganda, Zambia and Zimbabwe perceived that management options such as intercropping and biopesticides worked against this serious pest. Studies that documented economic benefits (11%) often measured this as the increase in economic revenue due to the reduction in pest damage, cost-benefit analysis (7%), poverty reduction (3%) and willingness to pay for biocontrol (3%). We found only one study measuring both the health and environmental impact of adopting biocontrol interventions amongst mango farmers in Kenya (Mwungu et al., 2020).
Interdisciplinary evidence
Although a wide variety of agroecological and socio-economic metrics were used as indicators of biocontrol success, quantitative methods in agroecological disciplines dominated the literature and were seldom integrated with social evaluations. Out of 173 studies, only nine studies from five articles evaluated both biophysical and social measures (Figure 3C). One study (Ba et al., 2013) combined an agroecological assessment of the augmentative release of parasitoids to control the millet head miner with structured questionnaires to farmers, which assessed their perception and knowledge of biocontrol before and after the study. Improved knowledge of pests by farmers and consistent willingness to implement augmentative interventions were observed following the implementation on their farms. This shows the potential of involving farmers throughout the process to increase their awareness of pests and their natural enemies, as well as their likelihood to adopt biocontrol interventions.
Two studies (Khan et al., 2008b; Midega et al., 2018) provided examples of the few cases where the impact of implementing a push-pull technique for managing stemborer was evaluated on maize using both biophysical and survey data. The farmers' perception of push-pull effectiveness was evaluated in Kenya, Tanzania and Uganda. Such interdisciplinary studies provide a more holistic picture of the adoption of biocontrol interventions in SSA, by measuring success not only in terms of pest control, but also by identifying adopters' characteristics that may influence their decision to implement a biocontrol intervention.
Biocontrol success: Where does it work, for whom and why?
A variety of variables were reported across the reviewed studies to describe the environmental, socio-economic and demographic contexts in which the studies were performed. Biophysical variables included were climatic (i.e., rainfall patterns, average temperatures), agricultural (such as cropping systems), and landscape characteristics (such as distance to natural habitat and landscape composition). These were often reported in the study site description to provide context, mostly in agroecological studies, but they rarely featured as predictors in the analysis (5%). Biocontrol performance was predominantly analyzed with one-way Analysis of Variance (ANOVA), using biocontrol practice as the sole factor (biocontrol compared with untreated control/chemical pesticides) predicting the measure of success. Landscape attributes, including landscape composition within a given radius, were tested as predictors of biocontrol effectiveness in 5% of studies and distance from natural habitat in 1%. Landscape complexity and proximity to natural habitat showed a positive correlation with natural enemy populations and predation (Henri et al., 2015; Milligan et al., 2016; Kebede et al., 2018; Soti et al., 2019) and pest infestation levels (Kebede et al., 2019). Two studies (Midega et al., 2014; Kebede et al., 2018) found that decreased landscape complexity reduced the performance of the push-pull system on maize in Ethiopia and Kenya.
A few agroecological studies provided suggestions of the optimal conditions for successful biocontrol implementation, yet these were not always empirically tested. For example, the augmentative release of predators or parasitoids was found to be more effective when combined with other practices, such as netting (Kungu et al., 2019), manure and mineral fertilizers (Mutisya et al., 2015), and reduced use of synthetic pesticides (Kahuthia-Gathu et al., 2017). Climatic conditions, such as humidity and UV levels, were highlighted as critical for parasitoid establishment (Kabore et al., 2017; Agboyi et al., 2020) suggesting that this intervention may not be possible in some agro-climatic regions. The importance of landscape-scale implementation of biocontrol programs was also highlighted (Kabore et al., 2017), as pests could migrate to neighboring fields that were not inoculated with parasitoids. Natural enemies could also move across the landscape if suitable habitat was available.
The success of botanical pesticide applications were mostly related to the plant species used to develop the compound (Supplementary Table S8), their concentration and spray timing (Alao and Adebayo, 2015; Ezena and Akotsen-Mensah, 2016). Studies assessing intercropping and push-pull identified suitable conditions for successful implementation and integration with other climate smart farming practices, such as strip tillage and insecticides (Abdallah et al., 2018), combining with mulching and/or wood ash (Demissie et al., 2019), earthing-up and harvesting time (Fite et al., 2014). The effect of biocontrol on multiple ecosystem services was evaluated explicitly for push-pull technology, which was shown to improve weed control (Khan et al., 2008b, 2009) and increase soil fertility (Khan et al., 2011; Kumela et al., 2019a).
About 20% of studies reported demographic and socioeconomic factors, which were tested in 12% of the total studies for their potential effect on perception or degree of biocontrol adoption. Those factors tested were sex, age, marital status and education level of farmers, farming experience, access to extension services and group membership, farming system, farm size, household size and livelihood strategies. The low number of studies identified in this review does not allow us to generate a comprehensive picture of why and for whom biocontrol control worked, but it allowed us to characterize some of the facilitators and barriers to biocontrol adoption, which are presented in Table 2.
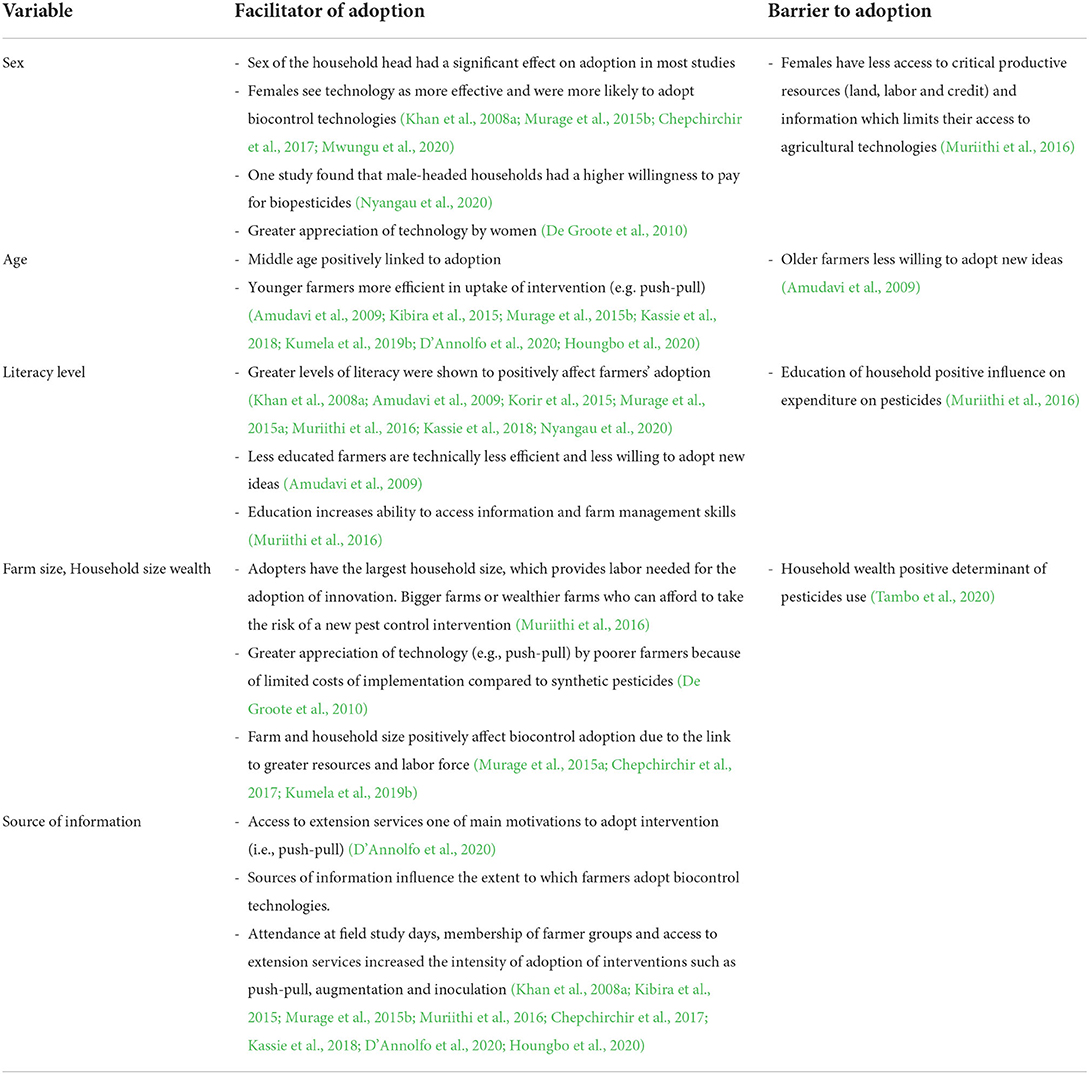
Table 2. Contextual factors affecting the adoption of biocontrol interventions by farmers in sub-Saharan African.
Constraints and opportunities for upscaling
Only 11% of the studies examined either the technical or socio-economic factors affecting the adoption and upscaling of biocontrol interventions.
The laborious process for extracting botanical pesticides, the need for several applications and the high volume of water required to produce aqueous extract were highlighted as negatively affecting adoption (Adda et al., 2011). We found that 50% of studies on botanicals were conducted in research farms or university campuses, which highlights that the effectiveness of botanical extracts has been rarely evaluated under farm conditions (Mkindi et al., 2017), and may reduce farmers' adoption due to little agroecological evidence of effectiveness. Farmers appeared less keen to adopt some botanicals (e.g., neem oil) due to non-availability of products and/or the high cost (Togbé et al., 2015). In some countries, such as Benin, synthetic pesticides are subsidized by the government, which may have encouraged smallholder farmers who are typically resource-poor, to use chemical pesticides due to prohibitive costs of alternative methods (Togbé et al., 2015). Emerging studies are calling for subsidies to be reallocated to biopesticides as lower risk options for the control of insect pests (Tambo et al., 2020).
Farmers were less likely to adopt biopesticides (bacteria/viruses/fungi and botanicals) because they take more time to be effective and do not entirely eradicate the pest (Constantine et al., 2020). Furthermore, the availability of biopesticides is not consistent as they may not be registered in a country or not stocked by the retailer where farmers buy synthetic pesticides (Constantine et al., 2020). Finally, a common source of advice for farmers is from pesticide dealers, who are more likely to recommend a product that they stock (Constantine et al., 2020).
The reluctance to adopt biocontrol interventions such as intercropping and push-pull was mainly due to the reluctance of farmers to use up cultivatable land, fear of taking risks with an unknown technology, and the labor demand required for establishment (Ifeany-obi et al., 2018; Kumela et al., 2019a). Challenges to the adoption of push-pull also included lack of information and shortage of inputs (e.g., availability of Desmodium seeds for push-pull) (Khan et al., 2014).
Discussion
This study summarized 173 studies that investigated biocontrol interventions, their performance and adoption for 35 crops across 20 countries in SSA from 2005 to 2021. Pest control interventions in cereal crops (particularly maize) dominated, while studies in other crop types such as vegetables, fruits and nuts were sparse. The lack of studies on social and economic outcomes highlighted the need for more interdisciplinary and participatory approaches to biocontrol evaluation that goes beyond the technical performance.
We found that there has been a consistent research focus in the past 15 years on the need to reduce the reliance on conventional pest control in favor of biocontrol interventions, yet this has not increased steadily over time (Supplementary Figure S1) according to the studies included in this review. However, there are still many open questions about how to improve the performance of biocontrol against sub-Saharan crop pests. There are relatively few studies investigating each intervention on each crop type, and we revealed regional clustering of some interventions (e.g., push-pull in Kenya). More research is needed to allow robust conclusions to be drawn for biocontrol interventions across different crop types, contexts, and scales (Table 1).
Crucially, our review highlighted a paucity of studies addressing the indirect health and environmental impacts of biocontrol interventions, and the co-benefits of not using chemical inputs, which is essential given the substantial risks that chemical inputs pose to human health and the environment in SSA (Macharia, 2015).
Despite established knowledge that the impact of local farm management is mediated by the wider landscape context (Smith et al., 2020), evidence is lacking on the interactive effect of landscape context and biocontrol practices on yield and other agronomic attributes. This research gap may lead to an underestimation of the variability of biocontrol effectiveness across different local management and landscapes contexts. Crucially, one study in Ethiopia (Kebede et al., 2019) showed that landscape context (i.e., proportion of maize in the landscape) was positively associated with pest damage in maize crops and overrode field-level management, when those were considered together. More research is needed to elucidate the role of the surrounding landscape, including natural habitat and neighboring farm management, on the capacity of biocontrol interventions to reduce pest damage.
There is a clear need to further our understanding of the potential synergistic effects of biocontrol interventions on multiple ecosystem services. Push-pull technologies have been shown to indirectly improve weed management and soil fertility, which could contribute to enhancing food security and food system resilience for smallholder farmer communities. Overall, our review found a paucity of evidence of the potential effects of biocontrol management on multiple ecosystem services.
We found a wealth of studies on botanical pesticides, testing extracts from plants known to have insecticidal properties (e.g., the exotic neem tree, Azadirachta indica), but predominantly on research farms. We found evidence gaps in their evaluation under a different range of smallholder farm conditions and practices to assess their efficacy and their benefits to farmers (Isman and Grieneisen, 2014). More research is needed to provide robust and updated recommendations on botanical use and effects, particularly focusing on plants extracts that are locally available to smallholder farmers to integrate and build on local knowledge.
Furthermore, evidence is sparse and inconsistent on the impact of botanical pesticides on beneficial organisms and other non-target species, which is one of the main drawbacks of synthetic chemical pesticides. It has been shown that neem contributed to a decrease in the size of predatory Coccinellidae and spider populations (Amera et al., 2017), although another study found that botanicals are less toxic to non-targets than synthetic pesticides (Tembo et al., 2018). It is crucial that future research focusses on elucidating the impact of botanicals not only on pest populations, but on the invertebrate communities on farms and surrounding habitats. Table 2 summarizes critical research gaps on performance of biocontrol interventions.
Our review may have missed some context-specific studies due to selecting only studies reported in English, which reflected the expertise of the authors. However, while we may have missed some context-specific details, the overall patterns in our research questions were nevertheless still captured within the English language literature, which spanned multiple countries, crops, pests and agro-ecological contexts.
Policy implications and future directions
Our synthesis takes the first step toward understanding the pathways to upscaling biological control adoption in SSA, and informing future research agendas that will provide recommendations for the integration of biocontrol practices into national agricultural policies. Key research gaps identified in our analysis could identify priority implementation areas and help strengthen the capacity of national research institutions already active in the region. Ultimately, future efforts should aim to address the disconnect between scientific research and the intended beneficiaries to enable policy recommendations that support sustainable pest control. Key strategies to overcome constraints of biocontrol adoption in SSA require the inclusion of these innovations in national agricultural policies, and the creation of clear regulatory frameworks, on the implementation, production, and distribution, for example of botanical pesticides.
A list of critical evidence gaps and recommendations of policy directions is presented in Table 1.
Crucially, our synthesis revealed only 55 social studies and 27 economic studies, compared to 127 studies focusing on agroecology, which highlighted the limited amount of evidence on socio-economic, policy and commercial aspects of biocontrol implementation. While it is essential to measure the ability of biocontrol technologies to reduce the negative effects of insect pests on crop production, there are also real opportunities to develop more nuanced understanding of adoption, innovation, and upscaling processes through interdisciplinary and transdisciplinary research. Our review indicates that these processes are currently being analyzed in a narrow sense, predominantly through multivariate analyses of the socio-economic determinants of adoption and non-adoption.
Most studies with an agroecological focus lacked participation from the potential beneficiaries (e.g., farmers), which would provide opportunities for knowledge sharing and the identification of opportunities and challenges of biocontrol implementation on the ground. Direct engagement with potential beneficiaries of biocontrol, as well as policy actors, may provide insights into how biocontrol innovations contribute to the well-being of different groups of people. This is critical to inform decision making in an equitable and inclusive way that delivers outcomes relevant to people across different contexts and scales (Mandle et al., 2021). Participatory community-based approaches involving local farmer groups are being increasingly used in order to identify pathways for achieving positive agro-ecological outcomes (González-Chang et al., 2020). Such approaches would increase farmers' knowledge and could lead to greater biocontrol uptake.
Increasing stakeholder engagement would also help to address key constraints to adoption identified in this review. This could be achieved by intensifying the use of training programs such as farmers field days, which have been shown to contribute to dissipating farmers' misconceptions about biocontrol effectiveness and overcome reluctance to adopt due to the perceived risk of an unknown technology (Giram et al., 2017; Emerick and Dar, 2021). Furthermore, future efforts should aim to make practical support more readily available to smallholder farmers by using technology, such as a recently-developed push-pull smartphone app (Agape Innovation, 2021) that provides an easy stepwise guide on how to set up this farming system. These simple, practical solutions could help extend knowledge of biocontrol innovations that is informed by science to potentially millions of sub-Saharan farmers.
Our evidence-based analysis demonstrates that an interdisciplinary and participatory approach exploring the technical, social and economic outcomes of biocontrol adoption is necessary to support wider uptake, inform policy and assist investments to reduce pest damage at a continental scale.
Data availability statement
The original contributions presented in the study are included in the article/Supplementary material, further inquiries can be directed to the corresponding author.
Author contributions
FR implemented the study, carried out the systematic review, and analyzed the data. All authors conceived and co-designed the study, contributed ideas that informed the structure and narrative of the paper, they provided editorial support, and have agreed to the published version of the manuscript.
Funding
This work was funded by the Engineering and Physical Sciences Research Council (EPSRC) project Scaling-up Biocontrol Innovations in Africa (Grant Number EP/T024410/1), which was a UKRI Global Challenges Research Fund Clusters award to SS, TB, GC, ZK, AM, SSl, SW, and KW. The funder had no role in the design of the study, the collection, interpretation of data, writing the manuscript, or in the decision to publish the results.
Conflict of interest
The authors declare that the research was conducted in the absence of any commercial or financial relationships that could be construed as a potential conflict of interest.
Publisher's note
All claims expressed in this article are solely those of the authors and do not necessarily represent those of their affiliated organizations, or those of the publisher, the editors and the reviewers. Any product that may be evaluated in this article, or claim that may be made by its manufacturer, is not guaranteed or endorsed by the publisher.
Supplementary material
The Supplementary Material for this article can be found online at: https://www.frontiersin.org/articles/10.3389/fsufs.2022.883975/full#supplementary-material
References
Abate, T., van Huis, A., and Ampofo, J. K. O. (2000). Pest management strategies in traditional agriculture: an African perspective. Annu. Rev. Entomol. 45, 631–659. doi: 10.1146/annurev.ento.45.1.631
Abdallah, M., Mwatawala, M. W., Kudra, A. B., Urio, N. A., and Mtakwa, P. W. (2018). Damage and control of the invasive African black beetle Heteronychus arator F. (Coleoptera: Scarabaeidae) in Southern highlands of Tanzania. Int. J. Pest Manag. 64, 88–93. doi: 10.1080/09670874.2017.1335913
Adda, C., Atachi, P., Hell, K., Tam,ò, M., and Tamo, M. (2011). Potential use of the Bushmint, Hyptis suaveolens, for the control of infestation by the pink stalk borer, Sesamia calamistis on maize in Southern Benin, West Africa. J. Insect Sci. 11, 1–13. doi: 10.1673/031.011.0133
Agboyi, L. K., Ketoh, G. K., Douro Kpindou, O. K., Martin, T., Glitho, I. A., Tam, M., et al. (2020). Improving the efficiency of Beauveria bassiana applications for sustainable management of Plutella xylostella (Lepidoptera: Plutellidae) in West Africa. Biol. Control 144, 0–2. doi: 10.1016/j.biocontrol.2020.104233
Alao, F. O., and Adebayo, T. A. (2015). Comparative efficacy of Tephrosia vogelii and Moringa oleifera against insect pests of watermelon (Citrullus lanatus Thumb). Int. Lett. Nat. Sci. 35. doi: 10.18052/www.scipress.com/ILNS.35.71
Amera, T., Mensah, R. K., and Belay, A. (2017). Integrated pest management in a cotton-growing area in the Southern Rift Valley region of Ethiopia: development and application of a supplementary food spray product to manage pests and beneficial insects. Int. J. Pest Manag. 63, 185–204. doi: 10.1080/09670874.2016.1278084
Amoabeng, B. W., Stevenson, P. C., Mochiah, B. M., Asare, K. P., and Gurr, G. M. (2020). Scope for non-crop plants to promote conservation biological control of crop pests and serve as sources of botanical insecticides. Sci. Rep. 10, 1–15. doi: 10.1038/s41598-020-63709-x
Amudavi, D. M., Khan, Z. R., Wanyama, J. M., Midega, C. A. O., Pittchar, J., Nyangau, I. M., et al. (2009). Assessment of technical efficiency of farmer teachers in the uptake and dissemination of push-pull technology in Western Kenya. Crop Prot. 28, 987–996. doi: 10.1016/j.cropro.2009.04.010
Ba, M. N., Baoua, I. B., N'Diaye, M., Dabire-Binso, C., Sanon, A., and Tamo, M. (2013). Biological control of the millet head miner Heliocheilus albipunctella in the Sahelian region by augmentative releases of the parasitoid wasp Habrobracon hebetor: effectiveness and farmers' perceptions. Phytoparasitica 41, 569–576. doi: 10.1007/s12600-013-0317-x
Chepchirchir, R. T., Macharia, I., Murage, A. W., Midega, C. A. O., and Khan, Z. R. (2017). Impact assessment of push-pull pest management on incomes, productivity and poverty among smallholder households in Eastern Uganda. Food Secur. 9, 1359–1372. doi: 10.1007/s12571-017-0730-y
Cohen, J. (1960). A coefficient of agreement for nominal scales. Educ. Psychol. Meas. 20, 37–46. doi: 10.1177/001316446002000104
Constantine, K. L., Kansiime, M. K., Mugambi, I., Nunda, W., Chacha, D., Rware, H., et al. (2020). Why don't smallholder farmers in Kenya use more biopesticides? Pest Manag. Sci. 76, 3615–3625. doi: 10.1002/ps.5896
D'Annolfo, R., Gemmill-Herren, B., Amudavi, D., Shiraku, H. W., Piva, M., Garibaldi, L. A., et al. (2020). The effects of agroecological farming systems on smallholder livelihoods: a case study on push–pull system from Western Kenya. Int. J. Agric. Sustain. 0, 1–15. doi: 10.1080/14735903.2020.1822639
De Groote, H., Rutto, E., Odhiambo, G., Kanampiu, F., Khan, Z., Coe, R., et al. (2010). Participatory evaluation of integrated pest and soil fertility management options using ordered categorical data analysis. Agric. Syst. 103, 233–244. doi: 10.1016/j.agsy.2009.12.005
Demissie, G., Mendesil, E., Diro, D., and Tefera, T. (2019). Effect of crop diversification and mulching on termite damage to maize in western Ethiopia. Crop Prot. 124. doi: 10.1016/j.cropro.2019.01.029
Diagne, C., Turbelin, A. J., Moodley, D., Novoa, A., Leroy, B., Angulo, E., et al. (2021). The economic costs of biological invasions in africa: a growing but neglected threat? NeoBiota 67, 11–40. doi: 10.3897/neobiota.67.59132
Edwards, P., Clarke, M., DiGuiseppi, C., Pratap, S., Roberts, I., and Wentz, R. (2002). Identification of randomized controlled trials in systematic reviews: Accuracy and reliability of screening records. Stat. Med. 21, 1635–1640. doi: 10.1002/sim.1190
Emerick, K., and Dar, M. H. (2021). Farmer field days and demonstrator selection for increasing technology adoption. Rev. Econ. Stat. 103, 680–693. doi: 10.1162/rest_a_00917
Ezena, G. N., and Akotsen-Mensah, C. (2016). Exploiting the insecticidal potential of the invasive siam weed, Chromolaena odorata L. (Asteraceae) in the management of the major pests of cabbage and their natural enemies in Southern Ghana. Adv. Crop Sci. Technol. 4:e1000230. doi: 10.4172/2329-8863.1000230
Fite, T., Getu, E., and Sori, W. (2014). Integrated management of sweetpotato weevil, Cylas puncticollis (Boheman) (Coleoptera: Curculionidae) in Eastern Ethiopia. J. Entomol. 11, 225–237. doi: 10.3923/je.2014.225.237
Giram, H., Khan, Z. R., Pittchar, J. O., and Ochatum, N. (2017). Impact of Field Days on Farmers' Knowledge and Intent to Adopt Push Pull Technology in Uganda. Int. J. Agr. Ext. 2, 131–143. Available online at: http://search.ebscohost.com/login.aspx?direct=trueanddb=lbhandAN=20183042817andlogin.asp?custid=magn1307andsite=ehost-liveandcustid=magn1307%0Ahttp://www.cabi.org/cabdirect/showpdf.aspx?PAN=http://www.cabi.org/cabdirect/showpdf.aspx?PAN=20183042817%0Ahttp://escijou (accessed June 10, 2022).
González-Chang, M., Wratten, S. D., Shields, M. W., Costanza, R., Dainese, M., Gurr, G. M., et al. (2020). Understanding the pathways from biodiversity to agro-ecological outcomes: a new, interactive approach. Agric. Ecosyst. Environ. 301, 107053. doi: 10.1016/j.agee.2020.107053
Grzywacz, D., Mushobozi, W. L., Parnell, M., Jolliffe, F., and Wilson, K. (2008). Evaluation of Spodoptera exempta nucleopolyhedrovirus (SpexNPV) for the field control of African armyworm (Spodoptera exempta) in Tanzania. Crop Prot. 27, 17–24. doi: 10.1016/j.cropro.2007.04.005
Haddaway, N. R., Macura, B., Whaley, P., and Pullin, A. S. (2018). ROSES RepOrting standards for systematic evidence syntheses: pro forma, flow-diagram and descriptive summary of the plan and conduct of environmental systematic reviews and systematic maps. Environ. Evid. 7, 7. doi: 10.1186/s13750-018-0121-7
Hemingway, J., Field, L., and Vontas, J. (2002). An overview of insecticide resistance. Science 298, 96–97. doi: 10.1126/science.1078052
Henri, D. C., Jones, O., Tsiattalos, A., Thebault, E., Seymour, C. L., van Veen, F. J. F. F., et al. (2015). Natural vegetation benefits synergistic control of the three main insect and pathogen pests of a fruit crop in southern Africa. J. Appl. Ecol. 52, 1092–1101. doi: 10.1111/1365-2664.12465
Hermans, T. D. G., Whitfield, S., Dougill, A. J., and Thierfelder, C. (2021). Why we should rethink ‘adoption' in agricultural innovation: empirical insights from Malawi. Land Degrad. Dev. 32, 1809–1820. doi: 10.1002/ldr.3833
Houngbo, S., Zannou, A., Aoudji, A., Sossou, H. C., Sinzogan, A., Sikirou, R., et al. (2020). Farmers' knowledge and management practices of fall armyworm, Spodoptera frugiperda (J.E. Smith) in Benin, West Africa. Agriculture-Basel 10, 430. doi: 10.3390/agriculture10100430
Ifeany-obi, C., Angba, A. O., and Nnawuihe, P. (2018). Environmentally sustainable farm management strategies adopted by compound farmers in Mbaitoli Local Government Area, Imo State Nigeria. J. Agric. Ext. 22, 97–107. doi: 10.4314/jae.v22i1.11S
Isman, M. B., and Grieneisen, M. L. (2014). Botanical insecticide research : many publications, limited useful data. Trends Plant Sci. 19, 140–145. doi: 10.1016/j.tplants.2013.11.005
Jepson, P. C., Murray, K., Bach, O., Bonilla, M. A., and Neumeister, L. (2020). Selection of pesticides to reduce human and environmental health risks: a global guideline and minimum pesticides list. Lancet Planet. Heal. 4, e56–e63. doi: 10.1016/S2542-5196(19)30266-9
Kabore, A., Ba, N. M., Dabire-Binso, C. L., and Sanon, A. (2017). Field persistence of Habrobracon hebetor (Say) (Hymenoptera: Braconidae) following augmentative releases against the millet head miner, Heliocheilus albipunctella (de Joannis) (Lepidoptera: Noctuidae), in the Sahel. Biol. Control 108, 64–69. doi: 10.1016/j.biocontrol.2017.03.001
Kahuthia-Gathu, R., Nyambo, B., and Subramanian, S. (2017). Impact of introduced parasitoid Cotesia vestalis (Hymenoptera: Braconidae) on Plutella xylostella (Lepidoptera: Plutellidae) and its parasitoid guild on kale in semi-arid areas in Kenya. Int. J. Trop. Insect Sci. 37, 163–175. doi: 10.1017/S1742758417000091
Kassie, M., Stage, J., Diiro, G., Muriithi, B., Muricho, G., Ledermann, S. T., et al. (2018). Push-pull farming system in Kenya: implications for economic and social welfare. Land Use Policy 77, 186–198. doi: 10.1016/j.landusepol.2018.05.041
Kebede, Y., Bianchi, F., Baudron, F. F., Abraham, K., de Valenca, A., Tittonell, P., et al. (2018). Implications of changes in land cover and landscape structure for the biocontrol potential of stemborers in Ethiopia. Biol. Control 122, 1–10. doi: 10.1016/j.biocontrol.2018.03.012
Kebede, Y., Bianchi, F. J., Baudron, F., and Tittonell, P. (2019). Landscape composition overrides field level management effects on maize stemborer control in Ethiopia. Agric. Ecosyst. Environ. 279, 65–73. doi: 10.1016/j.agee.2019.04.006
Kfir, R., Overholt, W. A., Khan, Z. R., and Polaszek, A. (2002). Biology and management of economically important lepidopteran cereal stem borers in Africa. Annu. Rev. Entomol. 47, 701–731. doi: 10.1146/annurev.ento.47.091201.145254
Khan, Z., Midega, C., Pittchar, J., Pickett, J., and Bruce, T. (2011). Push-pull technology: a conservation agriculture approach for integrated management of insect pests, weeds and soil health in Africa. Int. J. Agric. Sustain. 9, 162–170. doi: 10.3763/ijas.2010.0558
Khan, Z. R., Amudavi, D. M., Midega, C. A. O., Wanyarna, J. M., and Pickett, J. A. (2008a). Farmers' perceptions of a ‘push-pull' technology for control of cereal stemborers and Striga weed in western Kenya. Crop Prot. 27, 976–987. doi: 10.1016/j.cropro.2007.12.001
Khan, Z. R., Midega, C. A. O., Amudavi, D. M., Hassanali, A., and Pickett, J. A. (2008b). On-farm evaluation of the ‘push-pull' technology for the control of stemborers and striga weed on maize in western Kenya. Food Crop. Res. 106, 224–233. doi: 10.1016/j.fcr.2007.12.002
Khan, Z. R., Midega, C. A. O., Wanyama, J. M., Amudavi, D. M., Hassanali, A., Pittchar, J., et al. (2009). Integration of edible beans (Phaseolus vulgaris L.) into the push-pull technology developed for stemborer and Striga control in maize-based cropping systems. Crop Prot. 28, 997–1006. doi: 10.1016/j.cropro.2009.05.014
Khan, Z. R., Midega, C. A. O. O., Pittchar, J. O., Murage, A. W., Birkett, M. A., Bruce, T. J. A. A., et al. (2014). Achieving food security for one million sub-Saharan African poor through push-pull innovation by 2020. Philos. Trans. R. Soc. B-Biol. Sci. 369, 284. doi: 10.1098/rstb.2012.0284
Khan, Z. R., and Pickett, J. A. (2008). “Push-pull strategy for insect pest management,” in Encyclopedia of Entomology, ed. J. L. Capinera (Dordrecht: Springer Netherlands), 3074–3082. doi: 10.1007/978-1-4020-6359-6_3253
Kibira, M., Affognon, H., Njehia, B., Muriithi, B., Mohamed, S., and Ekesi, S. (2015). Economic evaluation of integrated management of fruit fly in mango production in Embu County, Kenya. Afric. J. Agric. Resour. Econ. 10, 343–353 doi: 10.22004/ag.econ.229815
Korir, J. K., Affognon, H. D., Ritho, C. N., Kingori, W. S., Irungu, P., Mohamed, S. A., et al. (2015). Grower adoption of an integrated pest management package for management of mango-infesting fruit flies (Diptera: Tephritidae) in Embu, Kenya. Int. J. Trop. Insect Sci. 35, 80–89. doi: 10.1017/S1742758415000077
Kumela, T., Mendesil, E., Enchalew, B., Kassie, M., and Tefera, T. (2019a). Effect of the Push-Pull Cropping System on Maize Yield, Stem Borer Infestation and Farmers' Perception. Agronomy-Basel 9, 452. doi: 10.3390/agronomy9080452
Kumela, T., Simiyu, J., Sisay, B., Likhayo, P., Mendesil, E., Gohole, L., et al. (2019b). Farmers' knowledge, perceptions, and management practices of the new invasive pest, fall armyworm (Spodoptera frugiperda) in Ethiopia and Kenya. Int. J. PEST Manag. 65, 1–9. doi: 10.1080/09670874.2017.1423129
Kungu, M., Deletre, E., Subramanian, S., Fiaboe, K. K. M. M., Gitonga, L., Lagat, Z. O., et al. (2019). A new mite IPM strategy: predator avoidance behaviour resulting from the synergetic effects of predator release and acaricide-treated nets. PEST Manag. Sci. 75, 979–985. doi: 10.1002/ps.5203
Macharia, I. (2015). Pesticides and health in vegetable production in Kenya. Biomed Res. Int. 2015, 241516. doi: 10.1155/2015/241516
Maes, K. (1997). The taxonomy of the lepidopteran cereal stemborers of Africa. Insect Sci. Its Appl. 17, 9–12. doi: 10.1017/S1742758400022128
Mandle, L., Shields-Estrada, A., Chaplin-Kramer, R., Mitchell, M. G. E., Bremer, L. L., Gourevitch, J. D., et al. (2021). Increasing decision relevance of ecosystem service science. Nat. Sustain. 4, 161–169. doi: 10.1038/s41893-020-00625-y
Midega, C. A. O., Pittchar, J. O., Pickett, J. A., Hailu, G. W., and Khan, Z. R. (2018). A climate-adapted push-pull system e ff ectively controls fall armyworm, Spodoptera frugiperda (J E Smith), in maize in East Africa. Crop Prot. 105, 10–15. doi: 10.1016/j.cropro.2017.11.003
Midega, C. A. O. O., Jonsson, M., Khan, Z. R., and Ekbom, B. (2014). Effects of landscape complexity and habitat management on stemborer colonization, parasitism and damage to maize. Agric. Ecosyst. Environ. 188, 289–293. doi: 10.1016/j.agee.2014.02.028
Milligan, M. C., Johnson, M. D., Garfinkel, M., Smith, C. J., Njoroge, P., Gar, M., et al. (2016). Quantifying pest control services by birds and ants in Kenyan coffee farms. Biol. Conserv. 194, 58–65. doi: 10.1016/j.biocon.2015.11.028
Mkindi, A., Mpumi, N., Tembo, Y., Stevenson, P. C., Ndakidemi, P. A., Mtei, K., et al. (2017). Invasive weeds with pesticidal properties as potential new crops. Ind. Crops Prod. 110, 113–122. doi: 10.1016/j.indcrop.2017.06.002
Murage, A. W., Midega, C. A. O., Pittchar, J. O., Pickett, J. A., and Khan, Z. R. (2015a). Determinants of adoption of climate-smart push-pull technology for enhanced food security through integrated pest management in eastern Africa. FOOD Secur. 7, 709–724. doi: 10.1007/s12571-015-0454-9
Murage, A. W., Pittchar, J. O., Midega, C. A. O. O., Onyango, C. O., and Khan, Z. R. (2015b). Gender specific perceptions and adoption of the climate-smart push-pull technology in eastern Africa. Crop Prot. 76, 83–91. doi: 10.1016/j.cropro.2015.06.014
Muriithi, B. W., Affognon, H. D., Diiro, G. M., Kingori, S. W., Tanga, C. M., Nderitu, P. W., et al. (2016). Impact assessment of integrated pest management (IPM) strategy for suppression of mango-infesting fruit flies in Kenya. Crop Prot. 81, 20–29. doi: 10.1016/j.cropro.2015.11.014
Mutisya, D. L., El-Banhawy, E. M., Khamala, C. P. M. M., and Kariuki, C. W. (2015). Management of cassava green mite Mononychellus progresivus (Acari: Tetranychidae) in different agro-ecological zones of Kenya. Syst. Appl. Acarol. 20, 39–50. doi: 10.11158/saa.20.1.5
Mwungu, C. M., Muriithi, B., Ngeno, V., Affognon, H., Githiomi, C., Diiro, G., et al. (2020). Health and environmental effects of adopting an integrated fruit fly management strategy among mango farmers in Kenya. African J. Agric. Resour. Econ. 15, 14–26. doi: 10.53936/afjare.2020.15(1).02
Nesser, G. A. A., Abdelbagi, A. O., Hammad, A. M. A., Tagelseed, M., and Laing, M. D. (2016). Levels of pesticides residues in the White Nile water in the Sudan. Environ. Monit. Assess. 188, 374. doi: 10.1007/s10661-016-5367-3
Neuenschwander, P. (2004). Harnessing nature in Africa Biological pest control can benefit the pocket, health and the environment. Nature 432, 801–802. doi: 10.1038/432801a
Neuenschwander, P., Borgemeister, C., and Langewald, J. (2003). Biological Control in IPM Systems in Africa. Wallingford, CT: CAB International.
Nyangau, P., Muriithi, B., Diiro, G., Akutse, K. S., and Subramanian, S. (2020). Farmers' knowledge and management practices of cereal, legume and vegetable insect pests, and willingness to pay for biopesticides. Int. J. PEST Manag. 0, 1–13. doi: 10.1080/09670874.2020.1817621
Oerke, E.-C., and Dehne, H.-W. (2004). Safeguarding production—losses in major crops and the role of crop protection. Crop Prot. 23, 275–285. doi: 10.1016/j.cropro.2003.10.001
Paini, D. R., Sheppard, A. W., Cook, D. C., De Barro, P. J., Worner, S. P., and Thomas, M. B. (2016). Global threat to agriculture from invasive species. Proc. Natl. Acad. Sci. USA. 113, 7575–7579. doi: 10.1073/pnas.1602205113
Pesticides Action Network Europe (2020). Banned and Hazardous Pesticides in European. Available online at: https://www.pan-europe.info/resources/reports/2020/09/banned-and-hazardous-pesticides-european-food
Pullin, A. S., and Stewart, G. B. (2006). Guidelines for systematic review in conservation and environmental management. Conserv. Biol. 20, 1647–1656. doi: 10.1111/j.1523-1739.2006.00485.x
Rodenburg, J., Schut, M., Demont, M., Klerkx, L., Gbèhounou, G., Lansink, A. O., et al. (2015). Systems approaches to innovation in pest management: reflections and lessons learned from an integrated research program on parasitic weeds in rice. Int. J. Pest Manag. 61, 329–339. doi: 10.1080/09670874.2015.1066042
Rosegrant, M. R., Ringler, C., Sulser, T. B., Ewing, M., Palazzo, A., Zhu, T., et al. (2009). Agriculture and Food Security Under Global Change: Prospects for 2025/2050. Washington, DC: IFPRI. Available online at: http://alliance.cgxchange.org/documentation-for-the-development-of-the-cgiar-strategy-and-mega-programs/SRF_IMPACT10-10-09c.pdf
Schut, M., Rodenburg, J., Klerkx, L., van Ast, A., and Bastiaans, L. (2014). Systems approaches to innovation in crop protection. A systematic literature review. Crop Prot. 56, 98–108. doi: 10.1016/j.cropro.2013.11.017
Smith, H. E., Sallu, S. M., Whitfield, S., Gaworek-Michalczenia, M. F., Recha, J. W., Sayula, G. J., et al. (2021). Innovation systems and affordances in climate smart agriculture. J. Rural Stud. 87, 199–212. doi: 10.1016/j.jrurstud.2021.09.001
Smith, O. M., Cohen, A. L., Reganold, J. P., Jones, M. S., Orpet, R. J., Taylor, J. M., et al. (2020). Landscape context affects the sustainability of organic farming systems. Proc. Natl. Acad. Sci. USA. 117, 2870–2878. doi: 10.1073/pnas.1906909117
Soti, V., Thiaw, I., Debaly, Z. M., Sow, A., Diaw, M., Fofana, S., et al. (2019). Effect of landscape diversity and crop management on the control of the millet head miner, Heliocheilus albipunctella (Lepidoptera: Noctuidae) by natural enemies. Biol. Control 129, 115–122. doi: 10.1016/j.biocontrol.2018.10.006
Sporleder, M., and Lacey, L. A. (2013). “Chapter 16 - Biopesticides,” in Insect Pests of Potato, eds. A. Alyokhin, C. Vincent, and P. Giordanengo (San Diego: Academic Press), 463–497. doi: 10.1016/B978-0-12-386895-4.00016-8
Stokstad, E. (2017). New crop pest takes Africa at lightning speed. Science 356, 473 LP−474. doi: 10.1126/science.356.6337.473
Tambo, J. A., Kansiime, M. K., Mugambi, I., Rwomushana, I., Kenis, M., Day, R. K., et al. (2020). Understanding smallholders' responses to fall armyworm (Spodoptera frugiperda) invasion: evidence from five African countries. Sci. Total Environ. 740, 140015. doi: 10.1016/j.scitotenv.2020.140015
Tembo, Y., Mkindi, A. G., Mkenda, P. A., Mpumi, N., Mwanauta, R., Stevenson, P. C., et al. (2018). Pesticidal plant extracts improve yield and reduce insect pests on legume crops without harming beneficial arthropods. Front. Plant Sci. 9, 1425. doi: 10.3389/fpls.2018.01425
Keywords: biocontrol, Africa, crop pests, push-pull, sustainable agriculture, innovation, adoption
Citation: Ratto F, Bruce T, Chipabika G, Mwamakamba S, Mkandawire R, Khan Z, Mkindi A, Pittchar J, Chidawanyika F, Sallu SM, Whitfield S, Wilson K and Sait SM (2022) Biological control interventions and botanical pesticides for insect pests of crops in sub-Saharan Africa: A mapping review. Front. Sustain. Food Syst. 6:883975. doi: 10.3389/fsufs.2022.883975
Received: 25 February 2022; Accepted: 05 July 2022;
Published: 28 July 2022.
Edited by:
Celia A. Harvey, Monteverde Institute, Costa RicaReviewed by:
Kris A. G. Wyckhuys, Chrysalis Consulting, VietnamAlbert Thembinkosi Modi, University of KwaZulu-Natal, South Africa
Copyright © 2022 Ratto, Bruce, Chipabika, Mwamakamba, Mkandawire, Khan, Mkindi, Pittchar, Chidawanyika, Sallu, Whitfield, Wilson and Sait. This is an open-access article distributed under the terms of the Creative Commons Attribution License (CC BY). The use, distribution or reproduction in other forums is permitted, provided the original author(s) and the copyright owner(s) are credited and that the original publication in this journal is cited, in accordance with accepted academic practice. No use, distribution or reproduction is permitted which does not comply with these terms.
*Correspondence: Fabrizia Ratto, Fabrizia.Ratto@rhul.ac.uk