- 1Animal Science Department, Polytechnic of Agriculture and Animal Science Mapena, Tuban, Indonesia
- 2Department of Animal Products Technology, Faculty of Animal Science, Universitas Gadjah Mada, Yogyakarta, Indonesia
- 3Institute for Halal Industry and System, Universitas Gadjah Mada, Yogyakarta, Indonesia
Meatballs are processed meat products that are susceptible to adulteration. Indonesia enforces a halal product guarantee regulation so that all products in circulation are free from non-halal ingredients. This study aimed to detect with the PCR method the contamination by pork and chicken of commercial beef meatballs. The samples of commercial meatballs were obtained from 36 meatball shops in Bojonegoro Regency, East Java, and Boyolali Regency, Central Java Province, Indonesia. Reference meatballs as a positive control were prepared in the laboratory. DNA isolation was performed on commercial sample meatballs, reference meatballs, and 13 species of fresh meat for specificity tests. DNA concentrations were measured using a spectrophotometer, and visualization of the isolation and PCR results was conducted using agarose gel electrophoresis and a UV transilluminator. DNA isolates were amplified by PCR using gene targets 1.711B bovine repeat for identifying bovine species, CR1 SINE repeat element for identifying chicken species, and mitochondrial gene subunit ND1 for identifying pork species. The results showed that there was contamination by chicken meat in meatballs labeled as beef meatballs in 30 of 36 samples in Bojonegoro Regency and 33 of 36 samples in Boyolali Regency. The results also showed that 22 samples of commercial beef meatballs in the Boyolali Regency contained pork. The implementation of the PCR method using the three specific primers proved the presence of meat contamination that did not match the label on the meatballs circulating in the Indonesian market. The contamination by pork if beef meatballs has violated the regulation on halal product guarantees.
Introduction
Currently, there is an increase in public awareness of the need for healthy, halal, and nutritious food, especially for Muslims. Recently, there has been an increase in awareness and interest on the part of consumers, researchers, and the food industry in properly labeling the content from animal species in meat products and their derivatives (Sakaridis et al., 2013). Authentication of food products of animal origin is very important because it is directly related to public health, religion, and culture (Bottero and Dalmasso, 2011). This fact encourages the public, especially consumers in Indonesia, to prefer food that has a halal label, as determined by the Indonesian Ulama Council (MUI). This process shows how important it is to authenticate and verify food products regularly and periodically, especially processed meat products. The presence of pork-derived products in food products is a serious problem from a religious point of view. Some religions, such as Islam and Judaism, forbid their followers from consuming any food containing pork and its derivatives, while Hinduism forbids its followers from consuming beef (Ali et al., 2012).
Meatballs are among the processed meat food products from livestock that are very popular worldwide. They can be formulated from beef, chicken, pork, and/or fish meat, but beef meatballs are the most popular and widely available product on the market (Rohman et al., 2011). Halal product certification is rarely applied to meatball products sold in meatball stalls in the Indonesian market. Halal codes or labels are mostly applied to packaged meatball products marketed in supermarkets. Although the meatballs circulating on the market are mostly claimed to be beef meatballs, it is often found that the meat is counterfeited using other meats that are not desired by consumers. Hossain et al. (2017) reported that beef meatballs contained 80% buffalo meat, and as much as 20% had been completely replaced by buffalo meat. In 2013, 4.6% of beef was replaced with horse meat in European countries in 2013 (D'Amato et al., 2013). In addition to food products, counterfeiting also occurs in drug capsules. Sahilah et al. (2012) found that 37.3% or 42 of 113 samples of drug capsules had been identified as containing pork, which was not mentioned in the packaging. The FDA (2009) assumes that incorrect labeling is part of economically motivated adulteration (EMA), which is the intentional counterfeiting of products to gain more profit. The halal food certification process in Indonesia, based on Law of The Republic of Indonesia Number 33 (2014 year), requires a halal auditing process by a certified halal auditor. The halal auditor examines and reviews the materials used, product processing process, slaughter system, location of the products, research equipment, production room, and storage and examines the distribution and presentation of products. During the auditing process, if the auditor doubts the status of the ingredients or products, the auditor can recommend the Halal Agency Office for confirmatory laboratory analysis. Another study by Erwanto et al. (2014) also showed through the PCR-RFLP method that there were nine cases of positive pork contamination of meatball stalls around Yogyakarta Province. In addition to contamination with pork, there is a high possibility that beef meatballs can be adulterated using chicken meat. Kim and Kim (2019) found that the mixing of processed meat foods often occurs by mixing them with chicken meat because the price is lower than that of beef. Therefore, to detect contamination by pork of beef meatballs, it is also necessary to conduct research on contamination with chicken meat.
Various methods have been developed to detect species contamination of food products of animal origin, including protein-based methods, including high-performance liquid chromatography (HPLC) (Toorop et al., 1997), electrophoretic chromatographic techniques (Arun and Ugur, 2000), enzyme-linked immunosorbent assay (ELISA) (Doi et al., 2009), and Fourier transform infrared (FTIR) spectroscopy (Hashim et al., 2010). However, the protein-based method has a number of drawbacks, including having a detection limit, especially in identifying food that has undergone processing in the form of heat and pressure. In response to the limitations of protein-based methods for the identification of processed foods, DNA-based methods have been developed. The DNA molecule is relatively stable, allowing for the analysis of processed food products that have undergone heat processing (Karabasanavar et al., 2014).
Rahman et al. (2014) reported in a study that the conventional PCR method is an easy and reliable method for routine analysis of food products of animal origin. Because not all laboratories have the latest real-time PCR equipment, conventional PCR can be relied on for routine analysis, in line with the opinion of Karabasanavar et al. (2014) that, although real-time PCR is more sensitive than conventional PCR, in routine practice, it does not require very high sensitivity because the aggregation of several pig cells in a sample is sufficient to detect their presence. Therefore, conventional PCR using species specificity could be a choice for detecting species in meat products. Real-time PCR is more sensitive than conventional PCR, but it has a higher cost. Real-time PCR uses a more advanced tool, so using conventional PCR, which is already available in the laboratory, must be optimized for use. Previously, conventional PCR using restriction fragment length polymorphism (RFLP) and multiplex PCR were developed. Erwanto et al. (2014) reported that using PCR RFLP is an appropriate method to detect certain species in cooked meat. PCR RFLP requires the BseDI restriction enzyme in the process, which means that two steps are necessary to determine contamination. Multiplex PCR is one of the chance methods for the detection of multiple species targets in one PCR tube, but finding the optimal temperature among the primers requires many annealing optimizations with gradient temperatures. It seems that application in various commercial markets requires new optimization of the annealing temperature. A recent report showed that species-specific conventional PCR is appropriate to detect species in cooked meat (Abdel-Rahman et al., 2009; Karabasanavar et al., 2011) and gelatin products (Shabani et al., 2015). A reliable method of conventional PCR using species specificity could be a choice for detecting species in meat products. Real-time PCR is more sensitive than conventional PCR, but it has a higher cost. Real-time PCR uses a more advanced tool, so using conventional PCR that is already available in the laboratory is an effective and efficient option. The development of non-halal detection methods based on DNA technology has been previously reported in many scientific journals, but its application in the real market, such as meatball stalls, has not yet been performed for many commercial market samples, especially in Indonesia, which has a halal guarantee law. Therefore, to guarantee that the commercial meatball market has no mislabeling, this study focused on the detection of meat species adulteration using conventional PCR.
Materials and Methods
Preparation of Samples
Meatball sampling was conducted in Bojonegoro Regency, East Java Province, and Boyolali, Central Java Province, Indonesia. Meatballs were purchased randomly from approximately 10 percent of the population of meatball stalls that have permanent or semipermanent sales locations and that appeared crowded with visitors based on the observations of the researchers when obtaining meatball samples. Meatball samples were obtained as ready eat food which already heated around 90–100°C for 30–60 min. Each sample then was placed in thick plastic to avoid cross contamination. The total sample of meatballs taken was 36 samples from Bojonegoro Regency and 36 samples from Boyolali Regency. All of the samples were then stored at −20°C until analysis. Reference meatballs were made as a positive control in the laboratory. Positive control meatballs consisted of three types of meatballs: pork, chicken, and beef, prepared based on (Rohman et al., 2011) and containing ground beef at as much as 90% and the remaining 10% consisting of tapioca flour, table salt, garlic, and other necessary seasonings. For specificity testing, 13 types of fresh meat species used were native chicken (Gallus gallus domesticus); broiler chicken (Gallus gallus); layer chicken (Gallus gallus); Brahman cattle (Bos taurus); ongole crossbreed cattle (Bos indicus); pork (Sus sucrofa domesticus), quail (Cortunix cortunix); Manila duck (Cairina moschata); duck (Anas platyrhynchos domesticus); sheep (Ovis aries); goat (Capra aegagrus hircus); red tilapia (Oreochromis niloticus); and catfish (Clarias gariepinus).
Ethical Approval
No animals were used in the present study. Thus, there was no requirement for ethical approval.
DNA Isolation
Isolation was performed using a tissue DNA isolation kit from Favorgen Biotech Corp. Total DNA isolation was conducted by taking a 25-mg sample using a surgical blade, which was only used once for one sample to prevent cross-contamination between the collected samples. The DNA isolated solution was stored in a freezer (−20°C) until further analysis. Similarly, samples of meatballs and meat were stored at −20°C before DNA extraction, and analysis was performed on different days to prevent damage (Rahman et al., 2014).
Analysis of Isolated DNA and Consent Measurements
The results of DNA isolation were determined by agarose gel electrophoresis (qualitative) and measurement of the concentration and purity of DNA (quantitative). The agarose gel used was 0.8% (w/v). The DNA isolate was mixed with loading dye migrated from the negative pole to the positive pole using TBE 1 X buffer solution. Electrophoresis was performed with a voltage of 80 volts for 40 min. The results were visualized with a UV transluminator, and the images obtained were documented with the camera as data. The purity and concentration of the isolated DNA was measured by creating a 5-μL DNA solution (in 495 μL of ddH2O) at wavelengths of 260 and 280 nm. DNA purity was measured by comparing the values of the 260 and 280-nm wavelengths. The concentration of DNA isolation was calculated from the absorbance at a wavelength of 260 nm (λ260) multiplied by the dilution factor (100X) and the absorption constant (50 g/mL).
DNA Amplification With PCR
The mixture was prepared in a PCR microtube consisting of 10 μL of 5X Smobio PCR master mix, 37.5 μL of nuclease-free water, and 1 μL of each reverse and forward primer (primer was diluted from 10 μL of primer stock plus 90 μL of nuclease-free water). Then, 0.5 μL of DNA template were added to the solution mixture. Amplification was performed with pre-denaturation at 94°C for 2 min. Then, 35 amplification cycles were carried out as follows: 94°C for 30 s, annealing at 55°C (for chicken and beef primers); while for pig primers, it was annealing at 59°C for 30 s and extension at 72°C for 30 s and 72°C for 1 min and incubation at 4°C for 30 min. The primers used in the PCR process were chicken and beef primers, respectively: forward 5′ CTG GGT TGA AAA GGA CCA CAG T 3′ and reverse 5′ GTG ACG CAC TGA ACA GGT TG 3′ with amplification targets on repeat elements CR1 SINE and 169 bp amplicon length; and forward 5′ TTT CTT GTT ATA GCC CAC CAC AC 3′ and reverse 5′ TTT CTC TAA AGG TGG TTG GTC AG 3′ with an amplification target of 1.711B bovine repeat element and 98 bp amplicon length (Walker et al., 2003). Pork primers used the forward sequence 5′ AAA GGA CCC AAC GTT GTA GG 3′ and reverse sequence 5' TAG TGC TAG GGA TAA GGC TAG G 3′ with amplification targets on the mitochondrial ND1 subunit and 147 bp amplicon length (Hikmah et al., 2020). The species-specific primer was ordered from PT Genetics Science Indonesia, Tangerang, Banten. The results of the PCR amplicon were analyzed using agarose gel electrophoresis and were visualized by a UV transilluminator with a 100-bp DNA ladder as a marker.
PCR Optimization and Primer Specificity Testing
Optimizing of the annealing temperature was performed by testing each primer on the DNA template of cattle, chicken, and pig according to the primer used. The optimization temperature used was in the range of 54–59°C. After obtaining the optimal annealing temperature, a specificity test was applied to other species. Species used for specificity testing included Indonesian native chicken (Gallus gallus domesticus); broiler chicken (Gallus gallus); layer chicken (Gallus gallus); Brahman cattle (Boss taurus); ongole crossbreed cattle (Bos indicus); pork (Sus sucrofa domesticus); quail (Cortunix cortunix); Manila duck (Cairina moschata); duck (Anas platyrhynchos domesticus); sheep (Ovis aries); goat (Capra aegagrus hircus); red tilapia (Oreochromis niloticus); and catfish (Clarias gariepinus).
Variable and Data Analysis
The research included analysis of isolated DNA and observations of PCR amplification results with each primer. DNA analysis was performed on the results of DNA isolation from fresh meat for specificity tests, meatballs prepared as a positive control, and meatball samples from the field. Measurement of the concentration and purity of DNA, as well as observations of amplification of isolated DNA, was performed using the polymerase chain reaction (PCR) method. The data collected were analyzed descriptively and qualitatively.
Results
The results of DNA isolation from fresh meat and positive control meatballs showed that DNA was successfully isolated. The concentration of isolated DNA varied from 320 to 1,800 ng/μL; however, it was sufficient for amplification using a PCR machine (Siswara, 2021).
The DNA isolation bands in Figures 1, 2 showed almost smear, the similar pattern also founded from Bojonegoro Regency (figure not shown). These indicating that the smears in the figures were due to the wide range of sizes of DNA fragments that were consequently in processed foods, such as meatballs, and the DNA was isolated after frozen storage. The results of the isolation of impure DNA did not interfere with the amplification process in PCR. Therefore, the results of the isolation still proceeded to the next stage, namely PCR, in accordance with the previous research of Erwanto et al. (2014), in which the results of DNA extraction from samples of meatballs on the market showed smears on the visualization but did not interfere with the PCR process (Siswara, 2021).
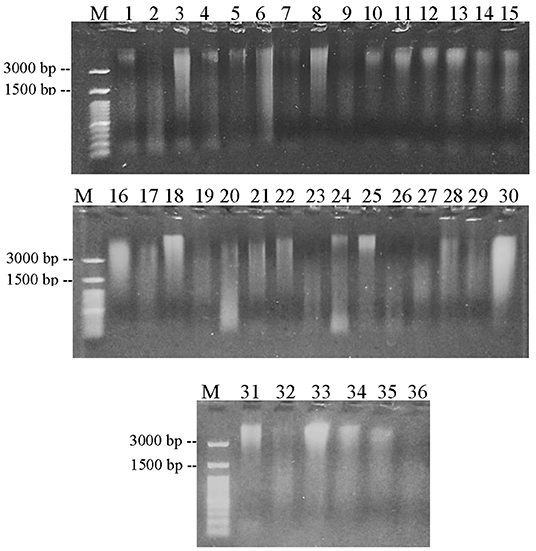
Figure 1. Visualization of DNA isolated from meatball samples in Boyolali Regency and electrophoresed on a 0.8% agarose gel. The samples were numbered L1–L36. (M) DNA marker 100 bp.
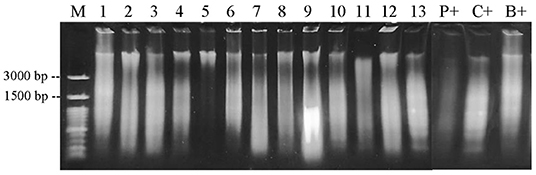
Figure 2. Visualization of DNA isolated from meat and control positive meatball samples, electrophoresed on 0.8% agarose gel. (M) DNA marker 100 bp. (1) Indonesia native chicken; (2) layer chicken; (3) broiler chicken; (4) Bos indicus cattle; (5) Bos taurus cattle; (6) pork; (7) quail; (8) Manila duck; (9) duck; (10) sheep; (11) goat; (12) red tilapia; (13) catfish; (P+) pork meatball; (C+) chicken meatball; (B+) beef meatball.
Prior to sample testing, each primer was optimized for annealing temperature. Based on Figure 3, there was one band of the thickest amplification results, indicating that the optimum temperature for annealing was reached. The optimum annealing temperature of bovine and chicken DNA primers was 55°C, while the optimum annealing temperature for pork DNA primers was 59°C. After determining the optimum temperature, each primer was tested for specificity on 13 types of fresh meat species. The results in Figure 4 show that the bovine primer only amplifies beef, both Bos taurus and Bos indicus. The chicken primer only amplified broiler, layer, and native chicken. Finally, the pig primer only amplified one species, namely pork.
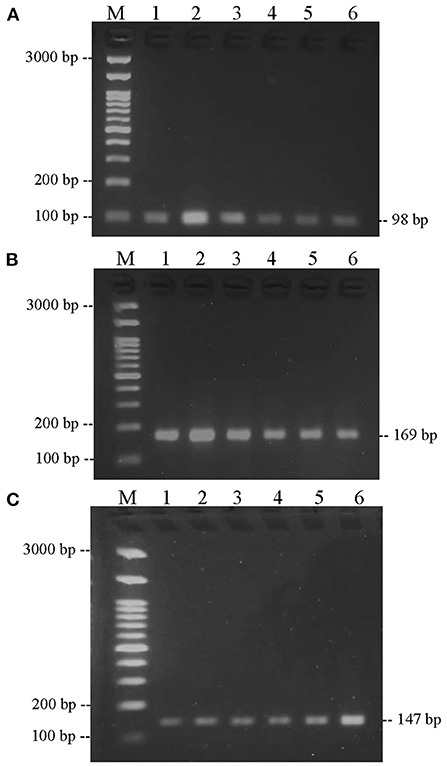
Figure 3. Optimization of annealing temperature. (A) Bovine primer; (B) chicken primer; and (C) pork primer at various temperatures, electrophoresed on 2% agarose gel. (M) DNA marker 100 bp. (1) Temperature 54°C; (2) temperature 55°C; (3) temperature 56°C; (4) temperature 57°C; (5) temperature 58°C; (6) temperature 59°C.
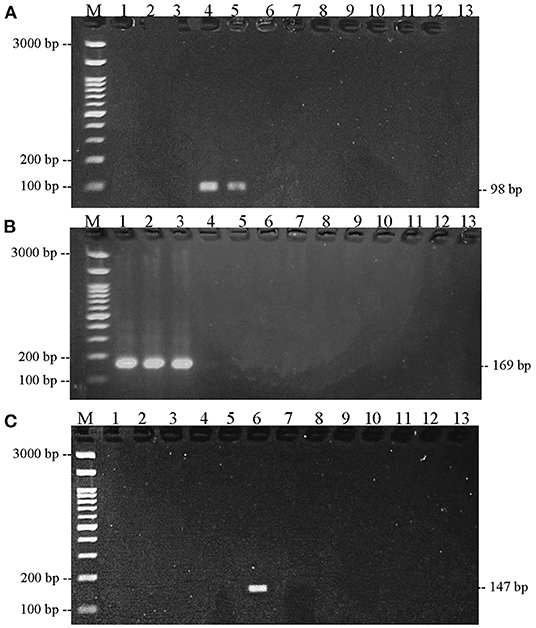
Figure 4. Results of primer specificity test. (A) Bovine primer; (B) chicken primer; (C) pork primer in various species, electrophoresed on 2% agarose gel. (1) Indonesia native chicken; (2) broiler chicken; (3) layer chicken; (4) Boss taurus cattle; (5) Bos indicus cattle; (6) pork; (7) quail; (8) Manila duck; (9) duck; (10) sheep; (11) goat; (12) red tilapia; (13) catfish.
The results of PCR amplification of bovine DNA from meatball samples from Bojonegoro Regency are shown in Figure 5. The results showed that there were 35 of 36 samples containing beef, while one sample did not amplify. Figure 6 shows that the results of the amplification of meatball samples from Bojonegoro Regency with chicken DNA primer showed that there were 30 of 36 samples of meatballs containing chicken meat. Figure 7 shows the results of the amplification of meatball samples from Bojonegoro Regency with pork DNA primers and showed that there was no amplification in any of the samples.
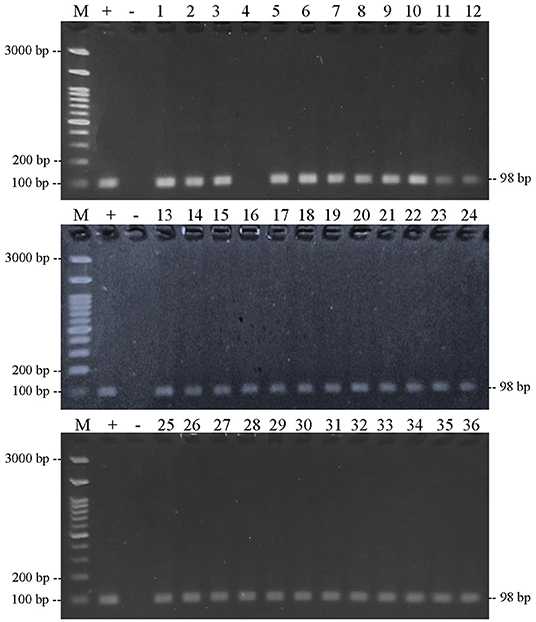
Figure 5. Results of PCR amplification of meatball samples in Bojonegoro Regency with bovine primers electrophoresed on a 2% agarose gel. (M) DNA marker 100 bp; (+) positive control meatballs; (–) negative control (no template control); samples were coded with numbers N1–N36.
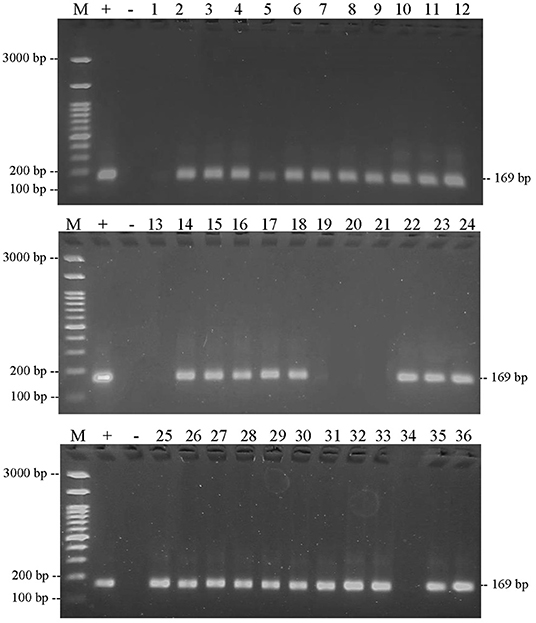
Figure 6. Results of PCR amplification of meatball samples in Bojonegoro Regency with chicken primers electrophoresed on a 2% agarose gel. (M) DNA marker 100 bp; (+) positive control meatballs; (–) negative control (no template control); samples were coded with numbers N1–N36.
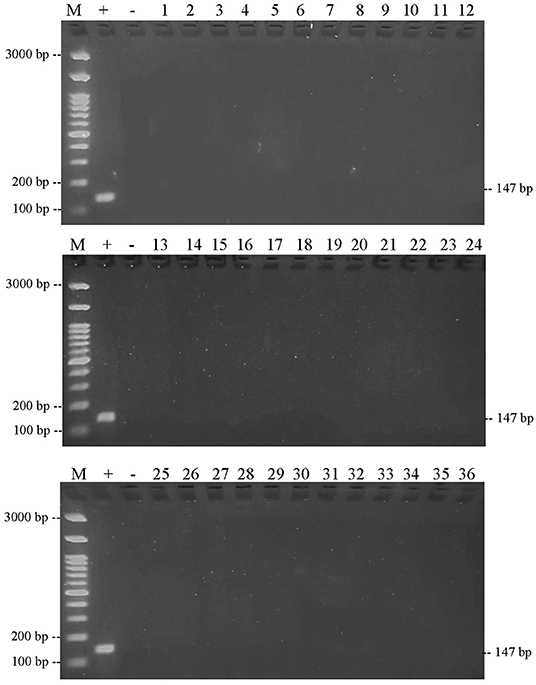
Figure 7. Results of PCR amplification of meatball samples in Bojonegoro Regency with pork primers electrophoresed on a 2% agarose gel. (M) DNA marker 100 bp; (+) positive control meatballs; (–) negative control (no template control); samples were coded with numbers N1–N36.
This result also showed that the three DNA-specific primers were amplified from meatball samples from the Boyolali Regency. Figure 8 shows the results of amplification of meatball samples from Boyolali Regency with bovine DNA primers. The results showed that all of the samples of meatballs contained beef. Furthermore, Figure 9 shows that there were 33 of 36 samples of meatballs from Boyolali Regency containing chicken meat. Figure 10 shows that 22 of 36 samples of meatballs from the Boyolali Regency contained pork. The results of PCR analysis of commercial meatballs from the Bojonegoro and Boyolali Regencies are summarized in Table 1.
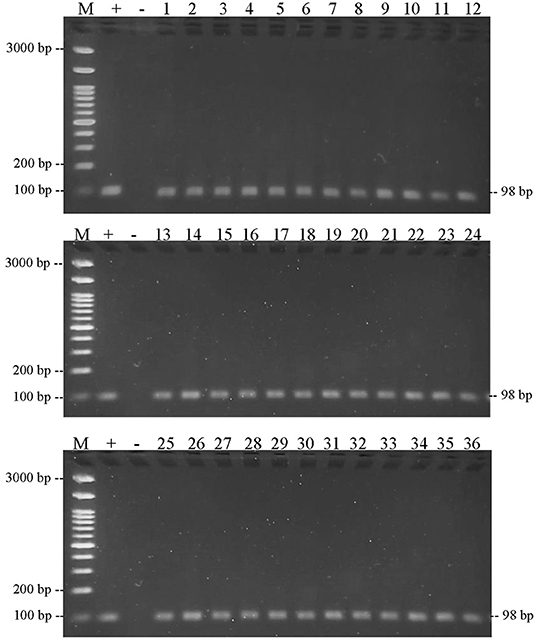
Figure 8. Results of PCR amplification of meatball samples in Boyolali Regency with bovine primers and electrophoresis on 2% agarose gels. (M) DNA marker 100 bp; (+) positive control meatballs; (–) negative control (no template control); samples were coded with numbers L1–L36.
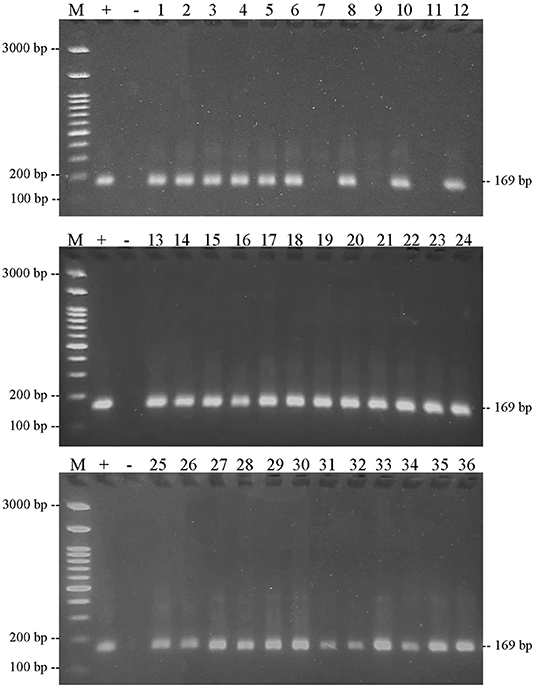
Figure 9. Results of PCR amplification of meatball samples in Boyolali Regency with chicken primers and electrophoresis on 2% agarose gels. (M) DNA marker 100 bp; (+) positive control meatballs; (–) negative control (no template control); samples were coded with numbers L1–L36.
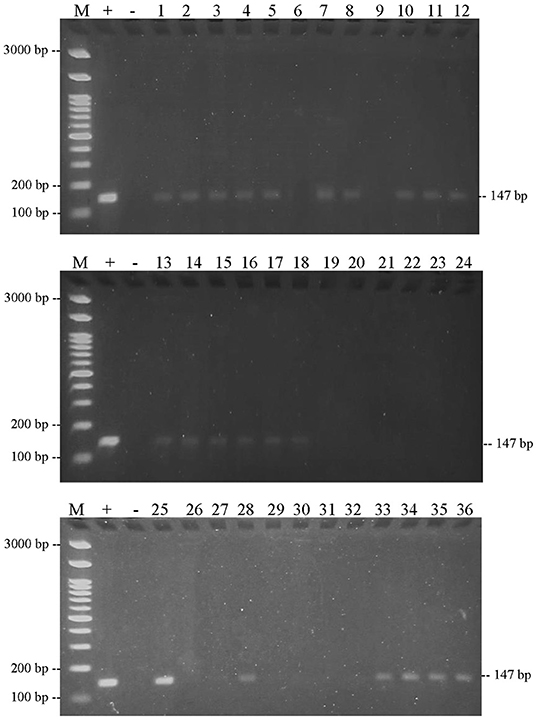
Figure 10. Results of PCR amplification of meatball samples in Boyolali Regency with pork primers and electrophoresis on 2% agarose gels. (M) DNA marker 100 bp; (+) positive control meatballs; (–) negative control (no template control); samples were coded with numbers L1–L36.
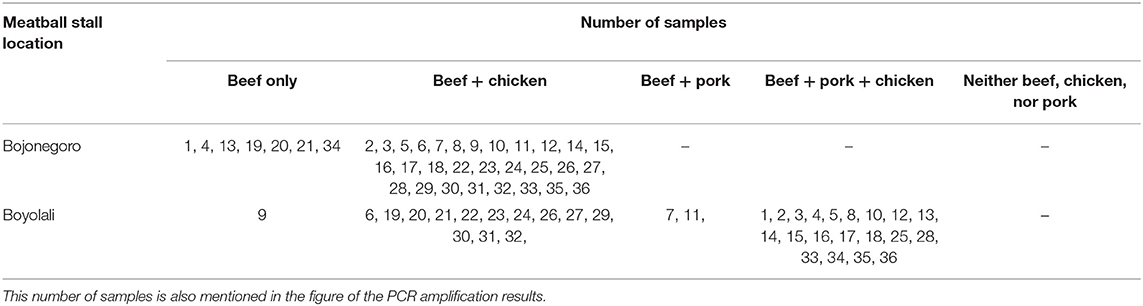
Table 1. Summary of meat species components in commercial meatballs from Bojonegoro and Boyolali Regencies.
Discussion
The concentrations of DNA isolated were quite different, presumably due to the different ingredients used for making meatballs from each sample. Previous research has reported that, in samples that were heat-processed (100 and 120°C), low yields of DNA were detected with almost all extraction approaches. The typical smear pattern of nucleic acid degradation of DNA extracted from heat-treated meats was observed (Piskata et al., 2019). Ghatak et al. (2013) also reported that isolation of DNA from frozen stored buccal swabs and urine samples exhibited bands with some degree of DNA degradation (low-intensity bands) and concomitant smearing in the lane. A similar performance was also found in previous research (Erwanto et al., 2012; Piskata et al., 2019). DNA extracted from processed food was assumed to be fragmented and appeared as a greatly expanded smear.
Primers designed to amplify short target DNA fragments have better sensitivity (Mendoza-romero et al., 2004). The amplicon target of 149 bp is relatively small and has little impact on the high heating process (Yin et al., 2020). Coinciding data resulted from this research; the target amplicon used in the pork DNA primer and bovine DNA primer in this study were suitable for detecting DNA in meatballs. The amplicon target in the pork DNA primer used in this study was 147 bp, and the bovine DNA primer was 98 bp. Hossain et al. (2017) stated that a small amplicon target length is more stable for heat treatment than a longer amplicon target, making it suitable for the amplification process in processed food products, such as meatballs.
Several research reports have found mixing of high-priced meat products with lower-value meat species. Adulteration of meat or its derivative products can occur by replacing higher-value meat with less expensive meat (Mohamad et al., 2013). A study of yak meat (Wang et al., 2013) reported that there had been adulteration of the meat with beef and pork to earn more profits. In addition to causing economic losses, the mixing of unlabeled animal species has a negative impact on people with allergies to certain foodstuffs (Kim et al., 2016). The content of meat species from mammals in products labeled as containing only poultry is a major problem for people with meat allergies because hives, asthma, or even anaphylactic shock can occur (Restani et al., 2009).
Unwanted contamination of meat products by other species can occur intentionally or unintentionally (Hertanto et al., 2017). Contaminated meat products can occur due to cross-contamination during processing using the same equipment on different species. Various amplified bands are formed, and there are thick and thin bands. A thin band indicates a relatively small amount of pork contamination, while a thick band indicates relatively large contamination of meatball samples (Okuma and Hellberg, 2015). In order to profit consideration, the presence of pork in meat products that is relatively small or <0.1% can be said to be unintentional contamination during the production process because, at very low levels, it is not very profitable for producers (Al-Kahtani et al., 2017). The location for grinding meatballs is usually in a traditional market, so several traders grind meatballs in the same location. The bands formed tend to be in samples with locations that are close to each other, thus strengthening the suspicion that contamination might occur in milling machines used together. In addition, the high pig population in the Boyolali Regency is a factor in the distribution of pork in that regency. This fact could make it easier for meatball traders to obtain pork, and the possibility of pork contamination is high when there is a large amount of pork circulating on the market or many pork traders in the Boyolali district. Based on data from the Statistical Central Bureau, Republic of Indonesia, Bojonegoro Regency had no pig population, and Boyolali Regency had a pig population of 6,767 in 2019.
Based on the Law (UU) of the Republic of Indonesia, Number 33 (2014), all food and beverage products distributed in the area of the Republic of Indonesia are mandatory to be halal by 2024. Therefore, a halal product guarantee is needed, namely legal certainty about the halalness of a product as evidenced by a halal certificate. However, to date, there are still limited meatball stalls in Indonesia that have halal certificates. Based on the Regulation of the Minister of Religion of the Republic of Indonesia Number 26 (2019), Chapter 25, paragraph 2, pork is classified as haram food. Haram food ingredients are those that are prohibited from being included in halal food. In addition, pork is designated as a forbidden food ingredient, as also stated in Law Number 33 (2014) on Halal Products Guarantee in Chapter 18, paragraph 1. Laws and government regulations about halal foods in Indonesia have clear statements that pork is a type of unallowed ingredient. Although the rules are clear, adulteration of commercial meatballs has been found in Boyolali Regency. Chicken is a halal food, but chicken found in beef meatballs is also a type of mislabeling that is not in accordance with consumer rights. The adulteration of meat raises concerns in terms of religion and the economy (Ballin, 2010). Contamination with pork, either in small or large quantities, is not justified in foodstuffs, especially meatballs. Ali et al. (2015) stated that cows and pigs are species that are important to religious issues. In 139 samples of processed food, 37% contained pork, and 23% contained chicken, neither of which was mentioned on the label. In addition, 57% errors in food labeling in the Italian meat market have been reported (Di Pinto et al., 2014). In Indonesia, there has also been reported contamination of meatballs with pork. Erwanto et al. (2014) reported that nine samples of meatballs contaminated with pork were collected from around Yogyakarta.
The contamination of food of animal origin with unspecified meat species also has an impact on health. The USDA (2015) stated that 75% of the emergence of infectious diseases in humans comes from animals. There are several animal species that are capable of transmitting disease (zoonosis) to humans, in line with the case of the global pandemic due to the 2019 coronavirus disease (COVID-19). Apart from zoonotic cases, pork contamination can cause some health problems for consumers. Based on research reports, people who consumed larger amounts of processed meat and pork had a higher risk of high-stage prostate cancer (Michaud et al., 2001; Rohrmann et al., 2007). Pork meat and bacon are associated with the risk of oral cancer because these foods are rich in fat content (Toporcov et al., 2004). Continuous intake of pork at 25 g/d elevated the risk for rectal cancer (Aykan, 2015). Research from Yuan et al. (1995) reported that Chinese women who have a diet with high pork intake and low vegetable intake have a propensity to develop breast cancer. A high risk of various types of cancer has a positive association with fat intake from pork.
Based on the study of research results and references, a fast, sensitive, effective, and simple method for identifying meat species is urgently needed (Abbas et al., 2018). The study used the PCR method, which was considered to meet these requirements. PCR has proved to be an adequate technique for detecting small amounts of DNA and can be successfully performed using species-specific primers for highly processed meat products, even gelatin from beef and pork (Shabani et al., 2015). Species-specific PCR has the greatest advantages of all DNA-based methods in terms of speed and specificity, and it is a new method for species identification (Yin et al., 2020). The small size of the target amplicon also has advantages for the primer used. By performing proper detection, the content of ingredients in food of animal origin can be determined. The meat used in food of animal origin must be correctly declared in accordance with its origin so as not to violate government regulations and laws related to halal quality assurance, food, and consumer protection.
Conclusions
The contamination by chicken and pork of beef meatballs circulating on the Indonesian market has violated the provisions of laws and government regulations related to halal quality assurance in Indonesia. The PCR method proved to be a robust and reliable method to confirm the contamination of mislabeled commercial food products.
Data Availability Statement
The original contributions presented in the study are included in the article/supplementary material, further inquiries can be directed to the corresponding author.
Author Contributions
HS: preparing data for manuscript, analysis of the sample, and data analysis. YE: research idea and design, manuscript preparation, and manuscript editing. ES: data analysis, English editing, and assistance with the experimental analysis. All authors contributed to the article and approved the submitted version.
Funding
This research was part of the thesis recognition project funding (RTA) under directorate of research, Universitas Gadjah Mada, contract number 3143/UN1.P.III/DIT-LIT/PT/2021 and a World Class Research grant, Ministry of Education, Culture, Research and Technology with contract number 4496/UN1/DITLIT/DIT-LIT/PT/2021.
Conflict of Interest
The authors declare that the research was conducted in the absence of any commercial or financial relationships that could be construed as a potential conflict of interest.
Publisher's Note
All claims expressed in this article are solely those of the authors and do not necessarily represent those of their affiliated organizations, or those of the publisher, the editors and the reviewers. Any product that may be evaluated in this article, or claim that may be made by its manufacturer, is not guaranteed or endorsed by the publisher.
Acknowledgments
The authors express thanks for the valuable assistance during the experiment by Mr. Ari Surya Sukarno and Mr. Rifqi.
References
Abbas, O., Zadravec, M., Baeten, V., Mikuš, T., Lešić, T., Vulić, A., et al. (2018). Analytical methods used for the authentication of food of animal origin. Food Chem. 246, 6–17. doi: 10.1016/j.foodchem.2017.11.007
Abdel-Rahman, S. M., El-Saadani, M. A., Ashry, K. M., and Haggag, A. S. (2009). Detection of adulteration and identification of cat's, dog's, donkey's and horse's meat using species-specific PCR and PCR-RFLP techniques. Aust. J. Basic Appl. Sci. 3, 1716–1719. Available online at: http://www.ajbasweb.com/old/ajbas/2009/1716-1719.pdf
Ali, M. E., Hashim, U., Mustafa, S., Che Man, Y. B., Dhahi, T. S., Kashif, M., et al. (2012). Analysis of pork adulteration in commercial meatballs targeting porcine-specific mitochondrial cytochrome b gene by TaqMan probe real-time polymerase chain reaction. Meat Sci. 91, 454–459. doi: 10.1016/j.meatsci.2012.02.031
Ali, M. E., Razzak, M. A., Hamid, S. B. A., Rahman, M. M., Amin, M., al Rashid, N. R. A., et al. (2015). Multiplex PCR assay for the detection of five meat species forbidden in Islamic foods. Food Chem. 177, 214–224. doi: 10.1016/j.foodchem.2014.12.098
Al-Kahtani, H. A., Ismail, E. A., and Asif Ahmed, M. (2017). Pork detection in binary meat mixtures and some commercial food products using conventional and real-time PCR techniques. Food Chem. 219, 54–60. doi: 10.1016/j.foodchem.2016.09.108
Arun, O., and Ugur, M. (2000). Animal species determination in sausages using an SDS-PAGE technique. Archive Für Lebensmittelhygiene 51, 33–56. Available online at: http://nek.istanbul.edu.tr:4444/ekos/MAKALE/M6758.pdf
Aykan, N. F. (2015). Red meat and colorectal cancer. Oncol. Rev. 9, 38–44. doi: 10.4081/oncol.2015.288
Ballin, N. Z. (2010). Authentication of meat and meat products. Meat Sci. 86, 577–587. doi: 10.1016/j.meatsci.2010.06.001
Bottero, M. T., and Dalmasso, A. (2011). Animal species identification in food products: evolution of biomolecular methods. Vet. J. 190, 34–38. doi: 10.1016/j.tvjl.2010.09.024
D'Amato, M. E., Alechine, E., Cloete, K. W., Davison, S., and Corach, D. (2013). Where is the game? Wild meat products authentication in South Africa: a case study. Investig. Genet. 4, 1–13. doi: 10.1186/2041-2223-4-6
Di Pinto, A., Bottaro, M., Bonerba, E., Bozzo, G., Ceci, E., Marchetti, P., et al. (2014). Occurrence of mislabeling in meat products using DNA-based assay. J. Food Sci. Technol. 52, 2479–2484. doi: 10.1007/s13197-014-1552-y
Doi, H., Watanabe, E., Shibata, H., and Tanabe, S. (2009). A reliable enzyme linked immunosorbent assay for the determination of bovine and porcine gelatin in processed foods. J. Agric. Food Chem. 57, 1721–1726. doi: 10.1021/jf802733y
Erwanto, Y., Abidin, M. Z., Muslim, E. Y. P., Sugiyono, S., and Rohman, A. (2014). Identification of pork contamination in meatballs of Indonesia local market using polymerase chain reaction-restriction fragment length polymorphism (PCR-RFLP) analysis. Asian Aust. J. Anim. Sci. 27, 1487–1492. doi: 10.5713/ajas.2014.14014
Erwanto, Y., Abidin, M. Z., and Sismindari, Rohman, A. (2012). Pig species identification in meatballs using polymerase chain reaction-restriction fragment length polymorphism for Halal authentication. Int. Food Res. J. 19, 901–906. Available online at: http://www.ifrj.upm.edu.my/19%20(03)%202012/(17)%20IFRJ%2019%20(03)%202012%20Rohman.pdf
Food and Drug Administration (FDA). (2009). Economically motivated adulteration; public meeting; request for comment. Federal Register. 74, 15497–15499. Available online at: http://edocket.access.gpo.gov/2009/pdf/E9-7843.pdf
Ghatak, S., Muthukumaran, R. B., and Nachimuthu, S. K. (2013). A simple method of genomic DNA extraction from human samples for PCR-RFLP analysis. J. Biomol. Tech. 24, 224–231. doi: 10.7171/jbt.13-2404-001
Hashim, D. M., Man, Y. B. C., Norakasha, R., Shuhaimi, M., Salmah, Y., and Syahariza, Z. A. (2010). Potential use of Fourier transform infrared spectroscopy for differentiation of bovine and porcine gelatins. Food Chem. 118, 856–860. doi: 10.1016/j.foodchem.2009.05.049
Hertanto, B. S., Fitra, R. A., Kartikasari, L. R., and Cahyadi, M. (2017). Authentication of raw chicken meat from pork contamination using gene Cyt-B with duplex-polymerase chain reaction analysis. Buletin Anim. Sci. 41, 113–118. doi: 10.21059/buletinpeternak.v41i2.13376
Hikmah, N., Rumiyati, R., Sismindari, S., and Rohman, A. (2020). Simultaneous detection of pork and wild boar meat in chicken sausages using the combination of a single primer and real-time polymerase chain reaction (qPCR). Pharmaciana 10, 11. doi: 10.12928/pharmaciana.v10i1.14771
Hossain, M. A. M., Ali, M. E., Hamid, S. B. A., Asing, M.ustafa, S., Desa, M. N. M., and Zaidul, I. S. M. (2017). Targeting double genes in multiplex PCR for discriminating bovine, buffalo and porcine materials in food chain. Food Control 73, 175–184. doi: 10.1016/j.foodcont.2016.08.008
Karabasanavar, N. S., Singh, S. P., Kumar, D., and Shebannavar, S. N. (2014). Detection of pork adulteration by highly-specific PCR assay of mitochondrial D-loop. Food Chem. 145, 530–534. doi: 10.1016/j.foodchem.2013.08.084
Karabasanavar, N. S., Singh, S. P., Umapathi, V., Kumar, D., Patil, G., and Shebannavar, S. N. (2011). A highly specific PCR assay for identification of raw and heat treated mutton (Ovis aries). Small Ruminant Res. 100, 153–158. doi: 10.1016/j.smallrumres.2011.07.009
Kim, M., Yoo, I., Lee, S. Y., Hong, Y., and Kim, H. Y. (2016). Quantitative detection of pork in commercial meat products by TaqMan® real-time PCR assay targeting the mitochondrial D-loop region. Food Chem. 210, 102–106. doi: 10.1016/j.foodchem.2016.04.084
Kim, M. J., and Kim, H. Y. (2019). A fast multiplex real-time PCR assay for simultaneous detection of pork, chicken, and beef in commercial processed meat products. LWT 114, 108390. doi: 10.1016/j.lwt.2019.108390
Mendoza-romero, L., Verkaar, E. L. C., Savelkoul, P. H., Catsburg, A., Aarts, H. J. M., Buntjer, J. B., et al. (2004). Real-time PCR detection of ruminant DNA. J. Food Prot. 67, 550–554. doi: 10.4315/0362-028X-67.3.550
Michaud, D. S., Augustsson, K., Rimm, E. B., Stampfer, M. J., Willett, W. C., and Giovannucci, E. (2001). A prospective study on intake of animal products and risk of prostate cancer. Cancer Causes Control 12, 556–567. doi: 10.1023/A:1011256201044
Mohamad, N. A., El Sheikha, A. F., Mustafa, S., and Mokhtar, N. F. K. (2013). Comparison of gene nature used in real-time PCR for porcine identification and quantification: a review. Food Res. Int. 50, 330–338. doi: 10.1016/j.foodres.2012.10.047
Okuma, T. A., and Hellberg, R. S. (2015). Identification of meat species in pet foods using a real-time polymerase chain reaction (PCR) assay. Food Control 50, 9–17. doi: 10.1016/j.foodcont.2014.08.017
Piskata, Z., Servusova, E., Babak, V., Nesvadbova, M., and Borilova, G. (2019). The quality of DNA isolated from processed food and feed via different extraction procedures. Molecules 24, 1188. doi: 10.3390/molecules24061188
Rahman, M. M., Ali, M. E., Hamid, S. B. A., Mustafa, S., Hashim, U., and Hanapi, U. K. (2014). Polymerase chain reaction assay targeting cytochrome b gene for the detection of dog meat adulteration in meatball formulation. Meat Sci. 97, 404–409. doi: 10.1016/j.meatsci.2014.03.011
Restani, P., Ballabio, C., Tripodi, S., and Fiocchi, A. (2009). Meat allergy. Curr Opin Allergy Clin Immunol. 9, 265–269. doi: 10.1097/ACI.0b013e32832aef3d
Rohman, A., Sismindari, E., Y, and Che Man, Y. B. (2011). Analysis of pork adulteration in beef meatball using Fourier transform infrared (FTIR) spectroscopy. Meat Sci. 88, 91–95. doi: 10.1016/j.meatsci.2010.12.007
Rohrmann, S., Platz, E. A., Kavanaugh, C. J., Thuita, L., Hoffman, S. C., and Helzlsouer, K. J. (2007). Meat and dairy consumption and subsequent risk of prostate cancer in a US cohort study. Cancer Causes Control 18, 41–50. doi: 10.1007/s10552-006-0082-y
Sahilah, A. M., Fadly, M. L., Norrakiah, A. S., Aminah, A., Wan Aida, W., Ma'aruf, A. G., et al. (2012). Halal market surveillance of soft and hard gel capsules in pharmaceutical products using PCR and southern-hybridization on the biochip analysis. Int. Food Res. J. 19, 371–375. Available online at: http://www.ifrj.upm.edu.my/19%20(01)%202011/(50)IFRJ-2011-199%20Sahilah.pdf
Sakaridis, I., Ganopoulos, I., Argiriou, A., and Tsaftaris, A. (2013). A fast and accurate method for controlling the correct labeling of products containing buffalo meat using High Resolution Melting (HRM) analysis. Meat Sci. 94, 84–88. doi: 10.1016/j.meatsci.2012.12.017
Shabani, H., Mehdizadeh, M., Mohammad, S., and Ansari, E. (2015). Halal authenticity of gelatin using species-specific PCR. Food Chem. 184, 203–206. doi: 10.1016/j.foodchem.2015.02.140
Siswara, H. N. (2021). Deteksi unsur babi dan ayam pada bakso sapi dengan metode polymerase chain reaction di Kabupaten Bojonegoro dan Boyolali (master's thesis). Universitas Gadjah Mada, Yogyakarta, Indonesia.
Toorop, R. M., Murch, S. J., and Ball, R. O. (1997). Development of a rapid and accurate method for separation and quantification of myofibrillar proteins in meat. Food Res. Int. 30, 619–627. doi: 10.1016/S0963-9969(98)00012-X
Toporcov, T. N., Antunes, J. L. F., and Tavares, M. R. (2004). Fat food habitual intake and risk of oral cancer. Oral Oncol. 40, 925–931. doi: 10.1016/j.oraloncology.2004.04.007
United States Department of Agriculture (USDA). (2015). Zoonotic Diseases of Sheep and Goats. Available online at: https://www.aphis.usda.gov/aphis/ourfocus/animalhealth/sa_animal_disease_information/sheep-goat/zoonotic (accessed April 13, 2021).
Walker, J. A., Hughes, D. A., Anders, B. A., Shewale, J., Sinha, S. K., and Batzer, M. A. (2003). Quantitative intra-short interspersed element PCR for species-specific DNA identification. Anal. Biochem. 316, 259–269. doi: 10.1016/S0003-2697(03)00095-2
Wang, P., Hu, Y., Yang, H., Han, J., Zhao, Y., and Chen, Y. (2013). DNA-based authentication method for detection of yak (Bos grunniens) in meat products. J. AOAC Int. 96, 142–147. doi: 10.5740/jaoacint.11-398
Yin, R., Sun, Y., Wang, K., Feng, N., Zhang, H., and Xiao, M. (2020). Development of a PCR-based lateral flow strip assay for the simple, rapid, and accurate detection of pork in meat and meat products. Food Chem. 318, 126541. doi: 10.1016/j.foodchem.2020.126541
Keywords: meatball, meat adulteration, halal, DNA-based methods, polymerase chain reaction
Citation: Siswara HN, Erwanto Y and Suryanto E (2022) Study of Meat Species Adulteration in Indonesian Commercial Beef Meatballs Related to Halal Law Implementation. Front. Sustain. Food Syst. 6:882031. doi: 10.3389/fsufs.2022.882031
Received: 23 February 2022; Accepted: 10 June 2022;
Published: 05 July 2022.
Edited by:
Serap Cosansu, Sakarya University, TurkeyReviewed by:
Ian Jenson, Meat & Livestock Australia, AustraliaMuhammad Cahyadi, Sebelas Maret University, Indonesia
Copyright © 2022 Siswara, Erwanto and Suryanto. This is an open-access article distributed under the terms of the Creative Commons Attribution License (CC BY). The use, distribution or reproduction in other forums is permitted, provided the original author(s) and the copyright owner(s) are credited and that the original publication in this journal is cited, in accordance with accepted academic practice. No use, distribution or reproduction is permitted which does not comply with these terms.
*Correspondence: Yuny Erwanto, yunyer@ugm.ac.id