- 1Institute for Epidemiology and Pathogen Diagnostics, Julius Kühn-Institute—Federal Research Centre for Cultivated Plants, Braunschweig, Germany
- 2Ecological Plant Protection Group, University of Kassel, Witzenhausen, Germany
Legumes are important drivers of soil fertility, however, their frequent use in rotations fosters long-lived soil-borne pathogens that can seriously compromise legume root health and nitrogen fixation. To overcome this, the current study aims at improving the general soil suppressiveness toward pests and diseases by agroecosystem management that can be predicted by nematode-based bioindicators. Two long-term organically managed agroecosystems comparing plow and shallow non-inversion tillage were analyzed for free-living nematode communities. Soils out of these agroecosystems were evaluated further in a greenhouse assay for their ability to suppress pea root rot caused by Didymella pinodella, Fusarium avenaceum, and F. redolens. There was a general trend for higher levels of pea root rot disease severity following inoculations with single pathogens, however, this effect was heterogeneous among experiments and tillage systems. This was mainly due to an already very high resident population of D. pinodella in soil and the presence of seed-borne F. oxysporum determined by their high incidence in pea roots irrespective of the soil and inoculated pathogens. Additional inoculation with D. pinodella, for example, resulted in only 8.5% biomass reduction compared to the non-inoculated control, in both tillage systems. Similar biomass losses were recorded in non-inversion tilled soils inoculated with F. redolens. When analyzed across inoculation treatments, the pea root rot disease severity was only slightly reduced in non-inversion tilled soils when compared to the plough systems (11% in Exp 1 and, 9% in Exp 2), however in both experiments non-inversion tillage resulted in greater pea biomass (33 and 19% in Exp1 and 2, respectively). Furthermore, the metabolic, enrichment, and bacterivore carbon footprints of nematodes were 88, 81, and 97% higher, respectively, in the non-inversion tilled soils compared to the plough. The metabolic carbon footprint of nematodes correlated negatively with pea root rot disease severity (rho = −0.71, p = 0.047). Hence, non-inversion tillage was effective in controlling pea root rot. The use of nematode metabolic footprints for predicting soil health should be extended for various agroecosystems aiming for its general use in evaluating effects of agroecosystem management through researchers and potentially farm management advisors.
Introduction
The development of more sustainable agro-ecosystems is indispensably linked with higher cropping frequencies of legumes. Their capability to fix nitrogen as well as their great use in animal nutrition allows for increased nutrient cycling on the farm level and decreased dependency on external fertilizer and pesticides (Finckh et al., 2015; Böhm et al., 2020). However, long-lived soil-borne pathogens can seriously compromise legume root health, and by this nitrogen fixation, limiting their frequent use in crop rotations (Le May et al., 2014; Baćanović-Šišić et al., 2018; Šišić et al., 2018b). Among the more than 20 different species involved in legume root rot (Baćanović-Šišić et al., 2018), Didymella pinodella, Fusarium avenaceum, and F. redolens are the most important pathogens of peas (Pisum sativum L.) and faba beans (Vicia faba L.) in Germany (Pflughöft et al., 2012; Baćanović-Šišić et al., 2018; Šišić and Finckh, 2021; Šišić et al., 2021). These pathogens not only have a broad host range and can occur asymptomatically on crops but also survive long periods in soil either saprophytically or as chlamydospores limiting the effectiveness of crop rotations (Finckh et al., 2015; Šišić et al., 2021).
Fostering soil life and, thus, general soil suppressiveness toward pests and diseases could be the only sustainable solution for adequate soil-borne disease suppression. In general, soils are considered suppressive when a plant shows low or even no disease even if the responsible pathogen is present in soil and also environmental conditions are suitable for plant infection (Cook and Baker, 1983). Drivers of soil suppressiveness are the structure, activity, and functions of the microbial communities. Their analysis is, therefore, key for determining soil suppressiveness (Mazzola, 2004; Creamer et al., 2022). Microorganisms interact with higher trophic levels of the micro-, meso-, and macrofauna and together form the soil food web. A positive correlation of the number of trophic links in the soil food web and the suppressive potential of soils has been suggested (Creamer et al., 2022). However, on the one hand, assessment of all individual links in the soil food web to determine soil suppressiveness is unfeasible considering the labor, time, and material expenses needed for the analyses. Therefore, assessments have to focus on limited parts of the soil food web that allow a clear indication of the suppressive properties of a given soil. Conversely, biotests are still indispensable for assessing the suppressive potential of soils as well as for validation of the links between soil food web and soil suppressiveness (Sánchez-Moreno and Ferris, 2007; Steel and Ferris, 2016; Zwetsloot et al., 2022) and there is a need to identify reliable indicators of soil suppressiveness.
Nematodes are an important part of the soil food web. Their communities comprise multiple trophic levels by which they build the intersection between the soil microflora and the meso- and macrofauna (Neher, 2010) and are easier to identify (Bongers, 1994; Andrássy, 2005) and to quantify (Hallmann and Subbotin, 2018) than bacteria and fungi. Therefore, it is not surprising that nematodes were recently cited as indicators of disease and pest regulation (Zwetsloot et al., 2022).
Several nematode based indices have been developed based on life-cycle, colonization speed, and sensitivity traits that allow to derive info on important soil functions related to soil disturbance, dominance of bacterial or fungal mediated soil processes, and soil nutrient enrichment (Bongers, 1990; Ferris et al., 2001; Ferris, 2010a) allowing for the determination of various “footprints” with respect to the carbon cycle (Table 1) (Du Preez et al., 2022). Individual nematode data (feeding types, body weight, etc.) are collected in one repository, the NEMAPLEX web page of the UC Davis (http://nemaplex.ucdavis.edu/), and single nematode based indicators can be automatically calculated by using the web platform (NINJA, https://shiny.wur.nl/ninja/) (Sieriebriennikov et al., 2014). This allows for a broad and universal use of nematodes as indicators of soil functions (Table 1) even though a certain amount of expert knowledge is needed to identify free-living nematodes at least to the family level. However, studies that correlate nematode based bioindicators with soil suppressiveness are rare and focus on nematode control (Sánchez-Moreno and Ferris, 2007; Steel and Ferris, 2016) while other soil-borne diseases so far were not considered.
Recently, we observed soil suppressiveness in two organically managed long-term experiments (LTEs) that were established in adjacent fields in 2010 and 2011 comparing a minimum tillage system combined with regular organic mulch application with a ploughed unmulched system (Junge et al., 2020; Schmidt et al., 2020). A massive increase of free-living nematodes already 4 years after conversion to minimum tillage was documented when one of the two LTEs was sampled (Schmidt et al., 2017). Biotests and nematode assessments 4 years later revealed positive correlations of bacterivorous nematodes and soil fertility in both LTEs (Schmidt et al., 2020). It is widely accepted that conservation agriculture based on the simultaneous application of minimum tillage, residue retention, and crop rotation, can have positive feedbacks on soil health and crop production (van Bruggen et al., 2006; Govaerts et al., 2008; Paulitz et al., 2010; Schlatter et al., 2017; Krauss et al., 2020). However, studies that link soil health and soil suppressiveness with soil chemical, biological, and physical properties still remain rare (Bünemann et al., 2018).
We investigated the two LTEs in 1 year, representing two different rotational positions for soil disease suppressiveness toward pathogenic fungi and in parallel quantified the various trophic levels of nematodes in the food web to determine whether nematode-based bioindicators are potentially useful to predict soil disease suppressiveness. The study specifically addressed the question of whether a crop rotation that includes cover crops and mulch applications in combination with non-inversion tillage can improve legume root health and by this legume biomass production. Therefore, in 2019, we collected soils from the long-term experiments from the plough-based system (25 cm) and the non-inversion tillage system (max. 15 cm) that includes application of transferred dead mulch to potatoes (Schmidt et al., 2020) either during grass clover (experiment 1) or after potato cropping (experiment 2). Soils were planted to field pea (Pisum sativum L.) and inoculated with pea pathogens under controlled conditions to assure disease infestation. Furthermore, links between soil nematode identities and quantities and the derived bioindicators with pea root rot severity and biomass production were explored to find suitable indicators for soil suppressiveness. We hypothesized that (i) pea root rot can be reduced and pea biomass increased by non-inversion tillage in combination with organic mulch application compared with a plough-based inversion tillage system without mulch, (ii) the abundance of nematode families and, thus, also indicators computed out of nematode community compositions respond to changes in the agro-ecosystem management (crop rotation, tillage/ organic mulch application), and (iii) nematode-based indicators correlate positively with pea biomass and negatively with pea root rot.
There is an urgent need for developing and validating reliable indicators of soil health that are less dependent on labor and time consuming biotests. For the first time, we could show that metabolic footprints of nematodes positively correlate with suppression of soil-borne diseases of pea and that organic conservation agriculture was promoting this disease suppressiveness.
Materials and Methods
The two long-term experiments originally started in 2010 (experiment 1) and 2011 (experiment 2) in adjacent fields located on the organic experimental farm of the University of Kassel (Germany) in Neu-Eichenberg (51°22′51″N, 9°54′44″E, 231 m ASL with an eastern incline of 3%). The soil type is a Haplic Luvisol with 3.3% sand, 83.4% silt and 13.3% clay (USDA classification Zc). Liming (CaCO3) was applied to all treatments at 2 t ha−1 in August/September 2019.
Experimental designs and crop rotations including a range of cash and cover crops were similar in both field experiments and described in detail in Schmidt et al. (2017; 2020). In brief, both experiments consist of a split-plot design with four replicates and tillage as main factor (12 × 60 m2) with: (1) minimum tillage by chisel ploughing or shallow rototilling (5–15 cm) including the application of dead mulch to potatoes vs. (2) conventional moldboard plough tillage (20–25 cm) without mulch application. The dead mulch applied to potatoes under minimum tillage was typically obtained from rye/pea or triticale/vetch cover crop mixtures (C/N ratios of 20–25) that were chopped (<10 cm) and applied at 10–26 t ha−1 DM on average with an adapted manure spreader. Each tillage main plot (12 × 60 m2) was split into eight 6 × 15 m2 sub-plots of which four of the subplots received ~5 t dry matter (ha yr)−1 of a high-quality yard waste compost that was applied manually. The remaining four sub-plots received potassium (K2SO4) and phosphorus (rock phosphate) fertilizer equivalent to their amounts in the respective composts. For details of the compost used between 2012 and 2015, see Schmidt et al. (2017). Composts used thereafter were of similar quality. In total, 16 plots were investigated (two field experiments ×4 field replicates × two tillage treatments). The tillage treatments were either plough tillage without mulch and with mineral phosphorus and potassium fertilization or minimum tillage combined with mulch application and compost fertilization. Treatments were chosen for differences in pea biomass production as observed previously (Schmidt et al., 2020).
In September 2019, about 110 soil cores were taken randomly from each plot center (4.5 × 10 m) with an Edelmann corer (5 cm diameter on average, 15 cm soil depth). Hence, soils were sampled during grass-clover cropping in experiment 1 and after potato harvest in experiment 2. Soils were sieved subsequently to 1 cm and stored in aerated plastic containers at 12°C until processing.
Soil Suppressiveness Bioassay
The susceptible pea variety 'Santana' was used as a model plant to evaluate soil health and soil suppressive capacity against three major pea root rot pathogens, namely F. avenaceum, F. redolens and D. pinodella. A mixture of six isolates of each pathogen species was used for inoculation of peas (see below). The isolates originated from the Internal Culture Collection of the Ecological Plant Protection Department at University of Kassel, and their taxonomic identity was previously confirmed morphologically and molecularly (Šišić et al., 2018b, 2021). Briefly, Fusarium isolates for inoculum production were grown on Synthetic Nutrient-Poor Agar (SNA) (Nirenberg, 1976; Nylander, 2004) whereas D. pinodella isolates were cultured on Coons medium (Coons, 1916). The plates were incubated at 20–23°C under constant cycles of 12 h BLB fluorescent light. After ~20 days of incubation, spores were scraped off from the agar surface with 15 mL of sterile distilled water and enumerated in the suspension with a Fuchs Rosenthal hemocytometer (Paul Marienfeld GmbH & Co. KG, Lauda-Königshofen, Germany).
The 16 soil sources collected from both field experiments (see above, two experiments × four field replicates × two tillage/fertilizer treatments) were transferred into 600 ml pots (~400 g dry soil pot−1). Five surface-sterilized (70% ethanol for 5 min) and pre-germinated (2 days) pea seeds were transplanted in each pot. Following transplanting, pots were inoculated with isolate mixtures of each pathogen at a concentration of 4 × 103 spores g−1 soil. Control pots were left non-inoculated. Additional pots containing autoclaved sand representing non-inoculated and pathogen inoculated treatments were included in the experiment as negative and positive controls, respectively, for determination of pea germination and pathogenicity of inoculated fungi (data not shown). Four replicates were used per treatment (256 pots in total) and blocked by field replicates along the microclimatic gradients in the greenhouse. Experimental plants were kept in the greenhouse at 21/18°C day/night temperatures. Pots were watered to approximately 40% water-holding capacity every 2–3 days. Plants were harvested during flowering (BBCH 65; ~8 weeks of growing) and the roots were separated from the aboveground biomass. Above ground plant parts of each pot were weighed and dried at 105°C until constant weight was achieved. Roots were washed under running tap water and the root rot disease severity (DI) was assessed and transformed into an index (0–100) according to Šišić et al. (2018b).
To confirm that artificial inoculations were successful and to identify any additional fungal pathogens in pea roots, the fungi were re-isolated from the roots using the protocol described in Šišić et al. (2018a, 2021). Briefly, four randomly selected roots from each treatment were surface-sterilized for 10 s in 1% NaOCl, thoroughly washed in distilled water and placed on filter paper under a laminar flow hood for 1 h to dry. Subsequently, the roots were cut into ~1 cm long fragments, placed in Petri dishes containing Coons medium, and incubated under alternating cycles of 12 h BLB fluorescent light and 12 h darkness. After 10–15 days of incubation, pure cultures of the resulting Fusarium and Didymella colonies were generated and morphologically identified following the methods described by Boerema (2004) and Leslie and Summerell (2006), respectively.
Nematode Assessment
Active nematode communities were extracted from 250 ml soil aliquots using an Oostenbrink elutriator (Hallmann and Subbotin, 2018). Nematodes were collected on three mounted 45 μm sieves, washed into beakers, and transferred onto Oostenbrink dishes to obtain clean samples. After incubation at room temperature for 48 h, the nematodes in the Oostenbrink dishes were collected on 20 μm sieves and transferred to plastic tubes, which were then filled up to 30 ml volume with tap water. Nematode abundances were counted from three 1 ml independent aliquots at 40-fold magnification under a compound microscope. The final nematode abundances were always calculated on 100 ml fresh soil. After transferring aliquots of the suspension to permanent mass slides, nematodes were heat killed and morphologically classified to the family level (about 100 individuals per sample) using the key of Bongers (1994). Nematode-based indicators (Table 1) were calculated with identified family abundances using the nematode indicator joint analysis web tool (Sieriebriennikov et al., 2014) (https://shiny.wur.nl/ninja/). The computed calculations were done for each plot separately by using the standard settings of the web tool. Details regarding the single calculations are published elsewhere (Bongers, 1990; Ferris et al., 2001; Ferris and Bongers, 2009; Ferris, 2010b). Soil nutrients were much less in the CT system without mulch compared to the MT system with mulch (Schmidt et al., 2020) indicating clear fertilization effects. The seven nematode indices used in our study (Table 1) are considered useful to study such effects (Du Preez et al., 2022).
Statistics
Statistical analyses were performed with R version 4.0.2 (R Core Team, 2020) using the packages “nlme” (Pinheiro et al., 2020) for analyses via linear mixed models and “emmeans” (Lenth, 2020) for linear contrasts of non-inversion and inversion tillage systems and calculation of standard error of the differences between means (SED). Furthermore, the treatment vs. control function was used to analyze effects of inoculation of pathogenic fungi on pea biomass production and disease severity in comparison to the control. The “car” package via Levene tests (Fox and Weisberg, 2019) and boxplots of model residuals were used to test the applied models for their variance homogeneity. In case of violations of variance homogeneity, linear mixed-effects models (lme) were adjusted with the weighting function “varIdent” to use individual standard errors for each factor level (Zuur et al., 2009).
Fixed factors were experiment, tillage system and fungal inoculation (the latter only for bioassay data). For nematode analysis, random factors were experiment and field replicate, the latter nested in the preceding factor to account for the split-block design. For the bioassay, field plots were used as random factor nested in the field replicates to account for the two-phased experiment with pseudo-replicates (replicates that were made of single field plots).
Thus, the random term for the data analysis of the biotest was “random = ~1|field experiment/field replicate/ field plot”.
Spearman's ρ correlations were used to study the relation of nematode-based bioindicators with pea biomass production and DI (on field plot level averaged over the tillage and inoculation treatments) by using the “rcorr” function of the R-package “Hmisc” (Harrell F. E. Jr., 2020). Results were visualized for each field experiment separately using the R function “corrplot” of the “Hmisc” package based on the p < 0.05 significance level.
Results
Fusarium avenaceum, F. redolens, and D. pinodella inoculated at 4,000 spores g substrate−1 to sterile sand controls reduced pea biomass by 28, 19, and 42%, respectively, compared to the non-inoculated control. D. pinodella inoculated at 16,000 spores g substrate−1 reduced pea biomass by 72% compared to the control. The root rot severity of peas (DI) was 60, 75, 76, 81, and 95 respectively for the non-inoculated control, F. avenaceum, F. redolens, D. pinodella (all at 4,000 spores g substrate−1), and D. pinodella (16,000 spores g substrate−1) treatments. The dominating pathogens isolated from roots of the control were F. oxysporum (63%) and D. pinodella (25%). The recovery rate of inoculated pathogens from pea roots in the sterile system was always 100% (all data not shown).
Soil Health in Biotests After Artificial Inoculation With Pathogenic Fungi
Shortly after transplanting pea seedlings, seedling mortality was observed in all experimental runs and in all treatments. The significance of seedling mortality due to damping-off (Figure 1) was greater in experiment 1 (22.5%) than experiment 2 (11.4%) (t9 = 3.9, p = 0.003). Although not statistically significant, seedling mortality was particularly high in CT pots (12 pots with ≥60% seedling mortality compared to 3 pots in the MT system) but was unaffected by artificial pathogen inoculation (Figure 1). Therefore, pots (pseudo-replicates) with ≥60% seedling mortality that accounted for 6% of the total number of pots were removed from analysis.
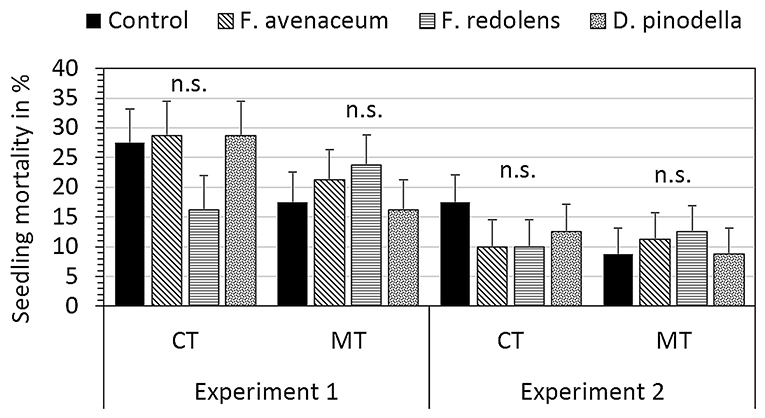
Figure 1. Seedling mortality and standard errors for each inoculation treatment (F. avenaceum, Fusarium avenaceum; F. redolens, Fusarium redolens, D. pinodella, Didymella pinodella, Control, non-inoculated natural soil) within each field experiment and tillage treatment (CT = plough tillage without organic mulch; MT = minimum tillage with organic mulch application to potatoes). Standard errors derived from linear mixed effects models and estimated marginal means.
Mean root rot disease severity (DI) averaged over inoculation treatments was 10–13% greater in CT soils compared to MT soils in both years (t6 = 3.56, p = 0.012 and t6 = 2.59, p = 0.041 for experiment 1 and 2, respectively) (Figure 2A). Lower DI in MT soils resulted also in general trend of greater pea biomass in comparison to CT soils (33 and 19% in soils from experiment 1 and 2, respectively), but this difference was statistically significant for soils from field experiment 1 only (t6 = 3.99, p = 0.007) (Figure 2B). Among individual pathogen treatments, increased DI in comparison to the corresponding non-inoculated control occurred only when roots were infested with F. redolens in MT soils of experiment 2 (+19%), whereas significant reductions in pea biomass were observed only in D. pinodella inoculated CT soils collected from experiment 1 (−15%, Figure 2B). No differences in DI and pea biomass were found for the remaining treatments. Isolations made from arbitrarily selected plant roots indicated very high resident population of D. pinodella and F. oxysporum resulting in 69 and 61% recovery of these pathogens, respectively, out of all roots. F. avenaceum, F. redolens, and D. pinodella were recovered from 25, 50, and 81% of roots, respectively (data not shown).
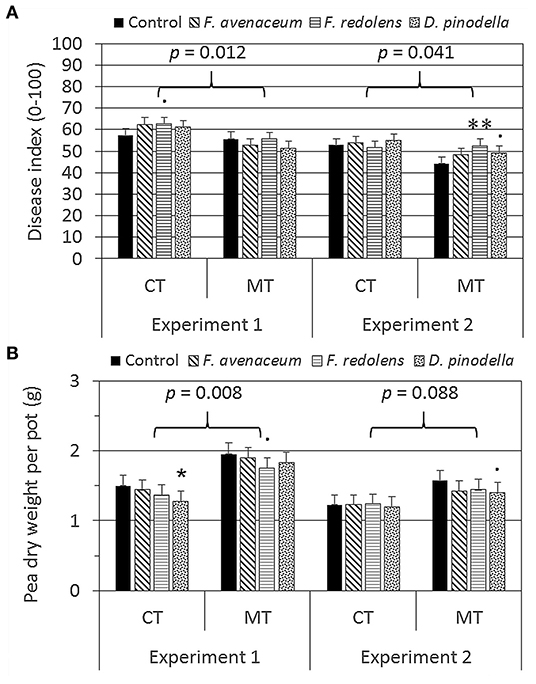
Figure 2. Means and standard errors of pea disease index (A) and dry weight (B) in soils managed with plough tillage without organic mulch (CT) and minimum tillage with organic mulch application to potatoes (MT) (df = 6) after inoculation with three pathogenic fungi compared to the Control (F. avenaceum, Fusarium avenaceum; F. redolens, Fusarium redolens, D. pinodella, Didymella pinodella, Control, non-inoculated natural soil) (df = 197). Effects were separated for experiment 1 (during grass-clover cropping) and experiment 2 (after potato harvest). Asterisks (p < 0.05*, p < 0.01**) and dots (p < 0.1) indicate significant differences of inoculated treatments from the control (linear mixed effects models and estimated marginal means).
Nematode Communities
Relative abundances of nematode feeding types varied between field experiments and management systems (Figure 3). In soils collected from experiment 1, relative frequencies of bacterivorous, fungivorous, herbivorous, and omnivorous nematodes made 50, 16, 31, and 3% of the total nematode community, respectively. Respective relative frequencies in experiment 2 were 29, 18, 42, and 10% of the total nematode community. Carnivorous nematodes were overall rare and represented <1% of the total nematode community. Total numbers of herbivorous and omnivorous nematodes were similar in both field experiments (Figure 3). In contrast, the total number of bacterivorous nematodes was 454 and 1,378 nematodes 100 ml soil−1 in experiment 1 and 2, respectively. The total number of fungivorous nematodes was 282 and 397 nematodes 100 ml soil−1, in experiment 1 and 2, respectively.
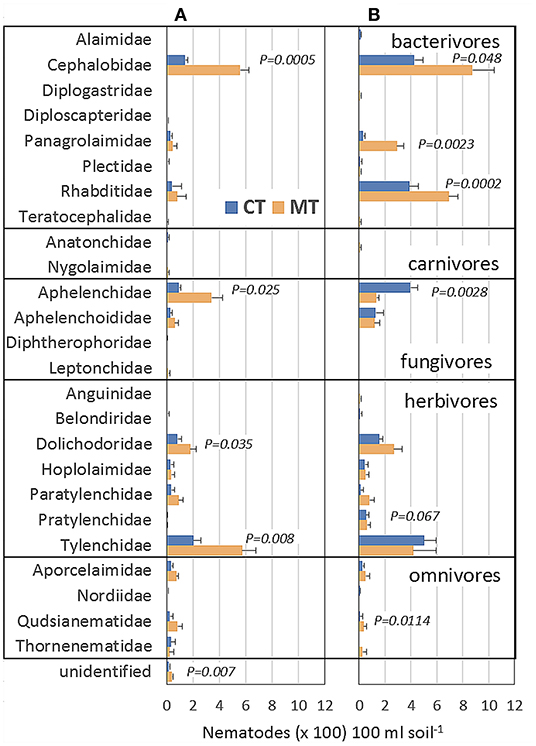
Figure 3. Morphologically identified frequency of nematodes per family in 100 ml soil for the first experiment during grass-clover cropping (A) and second experiment after potato harvest (B) under conventional ploughing (CT) and minimum tillage including application of organic mulches (MT). Nematodes are sorted alphabetically within each feeding type. Standard errors and exact p-values (p < 0.05, df = 6) are shown and result from linear-mixed effects models and contrasts of estimated marginal means. Tillage systems do not differ significantly for any nematode family that lacks a p-value (p > 0.05).
Bacterivores were dominated by the families of Cephalobidae, Rhabditidae, and Panagrolaimidae. These were about twice as abundant in the MT than in the CT system in soils of both field experiments (Figure 3). Similarly, the total number of herbivores was 144 and 12% greater in the MT system than in the CT system in experiment 1 and 2, respectively. Within this feeding type, the families of Dolichodoridae (t6 = 2.81, p = 0.03) and Tylenchidae (t6 = 3.91, p = 0.02) were 40–88% more frequent in the MT than in the CT system in experiment 1. Fungivorous nematode frequencies were similar in both field experiments but strongly affected by field experiment × management system interactions. Hence, total numbers of the family Aphelenchidae in the MT system were 250% greater than those in the CT system in experiment 1 in grass-clover (t6 = 2.97, p = 0.025). In contrast, the total number of Aphelenchidae was 100% greater in the CT system compared to the MT system in experiment 2 after potatoes (t6 = 4.87, p = 0.003).
The different nematode community structures in soils from both experiments and management systems were also reflected in multiple nematode-based indicators (Table 2). For example, the channel index (CI) for the MT system was 48 in experiment 1 and much lower in experiment 2 (CI = 6). Vice versa was the situation for the enrichment index (EI) which was very high for the MT system in experiment 2 (EI = 78.7) but only moderate in experiment 1 (EI = 48.2) indicating a higher nutrient status in experiment 2 under MT. In contrast, EI were similar among the two experiments under CT (EIs = 60 and 69) resulting in a reversal of the relations between MT and CT in the two experiments. Nematode footprints are reflected and summarized by the different abundances of nematode feeding types shown in Figure 3. For example, the metabolic footprint (MFP) was 135% larger in experiment 2 than experiment 1. Within each experiment, the MFP, enrichment footprint (EFP), and bacterivore footprint (BFP) were 43–57% lower in the CT system than in the MT system (t6 < −2.7, p <0.035). The fungivore footprint (FFP) was 71% lower in the CT system compared to the MT system in experiment 1 (t6 = −3.51, p = 0.013) but 126% greater in experiment 2 (t6 = −2.87, p = 0.028).
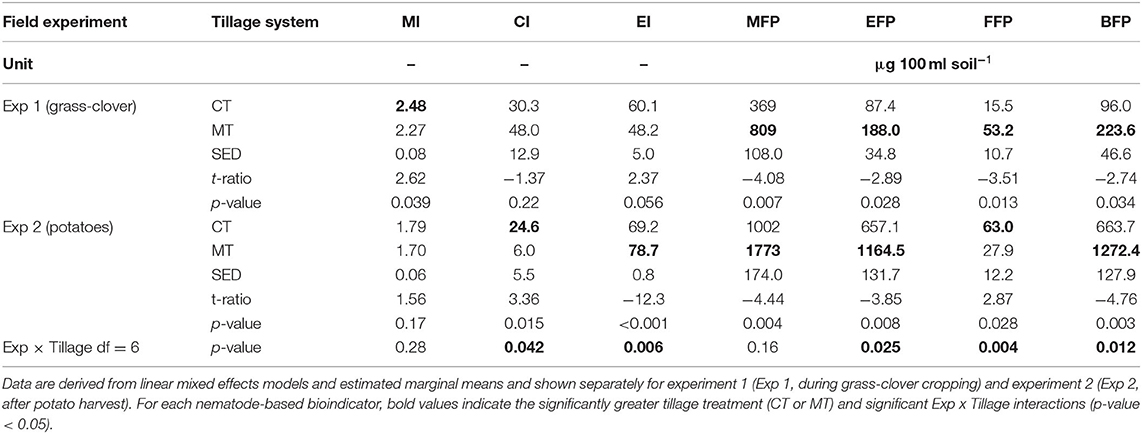
Table 2. Means and standard errors of the difference (SED) for plough tillage plus mineral fertilizer application and minimum tillage including mulch and compost application on the maturity index (MI), channel index (CI), enrichment index (EI), metabolic footprints (MFP), enrichment footprints (EFP), fungivore footprints (FFP), and bacterivore footprints (BFP).
Nematode-Based Indicators and Soil Disease Suppressiveness
There were negative and positive correlations of several nematode-based bioindicators with the DI of peas (Figure 4), i.e., pea plants were either healthier (red circles) or more diseased (blue circles) when these indicator increased. The MFP correlated negatively with the disease index (DI) in both experiments (rho = −0.71, p = 0.047, both experiments). The MFP further correlated significantly with the BFP in both experiments (rho > 0.88, p < 0.004). While no other significant correlations with DI occurred in experiment 2, the EI and MI correlated positively (rho = 0.79, p = 0.02, both indices) and the FFP negatively (rho = 0.88, p = 0.004) with the DI in experiment 1. Neither of the nematode-based bioindicators correlated with pea biomass production (rho = −0.62 to 0.57, p > 0.1).
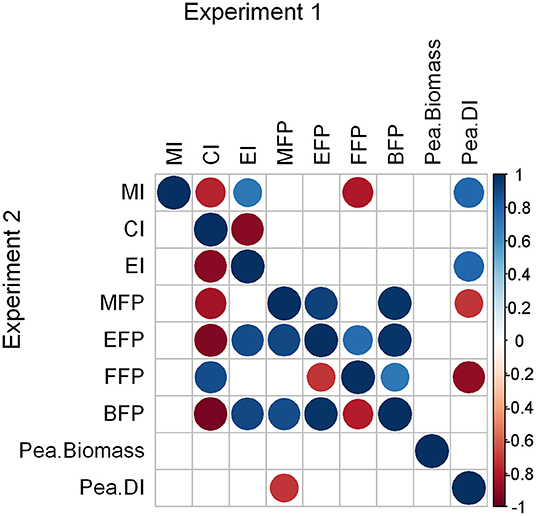
Figure 4. Spearman's rho correlation coefficients of nematode based bioindicators as well as pea biomass and disease indices independent of applied treatments. Correlations within experiments 1 and 2 are shown in the upper and lower diagonal, respectively. Positive correlations are displayed in blue and negative correlations in red color. Color intensity and the size of the circle are proportional to the correlation coefficients. In the right side of the correlogram, the legend color shows the correlation coefficients and the corresponding colors. Correlations with p-values > 0.05 (n = 8) are considered insignificant and were left blank. Abbreviations: maturity index (MI), channel index (CI), enrichment index (EI), metabolic footprint (MFP), enrichment footprint (EFP), fungivore footprint (FFP), bacterivore footprint (BFP), pea dry matter yield (Pea.Biomass) and disease index of peas (Pea.DI). The latter two indicators were assessed in a separate greenhouse experiment.
Discussion
Conservation Agriculture Improves Soil Suppressiveness
The DI in both experiments was high and indicated severe infestations with native soil- and seed-borne pathogens such as with the frequently isolated pathogens D. pinodella (69%) and F. oxysporum (61%) out of pea roots. Both fungi had been re-isolated frequently out of leguminous roots taken from agricultural fields throughout Germany (Pflughöft et al., 2012; Baćanović-Šišić et al., 2018; Šišić et al., 2018b). The fact that both fungi are also seed-borne (Finckh et al., 2015) and that both occurred on the control plants in the sterile sand system suggests their responsibility for the high general disease severity. In another field nearby (100 m distance), Wille et al. (2021) identified Aphanomyces euteiches and Fusarium solani via quantitative PCR as dominating pea root rot pathogens in soils, indicating additional actors possibly involved in the pea root rot complex.
The DI was greater in the first experiment than in the second experiment likely due to the different crops in both fields. While potatoes in experiment 2 are generally non-hosts for many legume root rot pathogens, clover in experiment 1 shares a number of similar if not identical pathogens with pea, including D. pinodella and many Fusarium spp. (Le May et al., 2014; Šišić et al., 2018b). This concurs with a massively increased DI on pea that was grown after Vicia faba in comparison to other non-leguminous crops in Dutch fields (Oyarzun et al., 1994, 1998).
Conservation agriculture, i.e., the simultaneous application of minimum tillage, residue retention, and crop rotation has often been credited with positive feedbacks on soil health and crop production (van Bruggen et al., 2006; Govaerts et al., 2008; Paulitz et al., 2010; Schlatter et al., 2017; Krauss et al., 2020). This study agrees because there was greater pea biomass production and decreased pea DI in the biotest in the non-inversion tillage mulch system than in the plough-based system. Moreover, the consistent reduction of pea DI independent of the rotational crop and artificially inoculated root rot pathogens suggests a stable general suppressiveness which is considered a “practical goal” for farming strategies (Schlatter et al., 2017). This concurs with the common understanding of soil suppressiveness being achieved by organic matter management (Bailey and Lazarovits, 2003; Mazzola, 2004; Bonanomi et al., 2018). For example, integrating minimum tillage, crop rotations, and organic amendments reduced root-knot nematodes and disease severity due to Fusarium oxysporum in a ginger farming system (Stirling et al., 2012). Our results confirm results of an experiment conducted with the same field soil 5 months earlier with focus on plant-parasitic nematodes (Schmidt et al., 2020). The authors likewise observed a lower pea DI (−5 to −12%) in the non-inversion tillage system with compost compared to the plough system with mineral fertilization. Therefore, our study confirms the first part of the first hypothesis that non-inversion tillage in combination with mulch fosters soil suppressiveness in general.
However, the conservation agriculture system used here failed to suppress artificially inoculated pathogens consistently in both field experiments. The direct parasitism through seed-borne F. oxysporum and D. pinodella, as indicated by the biotest in sterile sand, likely inhibited a direct suppression by the soil microbiome and caused similar disease severities in inoculated and non-inoculated plants. Soil inoculation with D. pinodella also failed to significantly raise the DI of winter peas in comparison to the control in three consecutive field trials (Baćanović-Šišić et al., 2018). The 4,000 spores per g of substrate inoculated to pots in our study was likely insufficient to raise the DI above the general level caused by the seed-borne pathogens. Oyarzun et al. (1998) showed that pea DI after inoculation with different pathogens depends on pathogen species, its inoculated density as well as crop rotation. For example, inoculation of F. solani at 1,000 spores per g substrate was sufficient to increase pea DI significantly independent of the preceding crop. In contrast, inoculation with A. euteiches at 10–100 spores per g substrate almost killed the pea in soils following Vicia faba cropping, while more than 1,000 spores per g substrate were needed to increase DI after maize and flax. Hence, a more detailed experiment with stepwise increased inoculation densities and/or the use of different preceding crops may contribute to our knowledge of pea root rot suppressiveness in our soils.
Soil Nematode Communities and Derived Indicators Reflect Soil Management and Rotational Position
Nematode trophic groups in this study reflected those assessed on a global scale (van den Hoogen et al., 2019) and those present in Polish agroecosystems (Wasilewska, 1979). The rare occurrence of omnivorous and carnivorous nematodes was likely due to their low reproduction rates in combination with low stress tolerance, e.g., frequent soil tillage, quick alternations between nutrient rich and depleted soil conditions as well as unstable food supply that are common in agroecosystems (Ferris et al., 2001; Schmidt et al., 2020). Furthermore, nematode extraction procedures may bias nematode community retrieval. According to Neher et al. (1995), elutriation techniques, such as used in our study, retrieve less omnivorous and carnivorous nematodes than Cobb's sifting and gravity method. However, such bias can be neglected here due to the great differences in nematode communities among the applied treatments.
With respect to the individual nematode families and derived nematode-based indicators, large differences were observed among management systems and rotational position. This was likely due to the quick response of soil nematodes and their derived indicators on alternating environmental conditions (Landi et al., 2018) which is the main reason for their usefulness in describing soil fertility, food web stability, and detecting soil toxicity (Korthals et al., 1996; Ferris et al., 2001; Neher, 2001; Ferris, 2010a).
With respect to the differences among the field experiments, soils from experiment 2 were greatly disturbed by potato cropping and harvesting, either through hilling (plough system) or through mulch application and subsequent incorporation by the harvester (minimum tillage system). Consequently, bacterivorous nematodes with short generation cycles (Panagrolaimidae, Rhabditidae) quickly responded on the resulting bacterial blooming and built up large populations. Over time, as nitrogen rich food sources decrease, fungal mediated break down of crop residues becomes more important (Grzyb et al., 2020) accompanied by increased densities of fungivorous nematodes (Bongers and Bongers, 1998). Such a situation likely occurred under grass-clover in experiment 1, 16 months after the last mulch application and 12 months after the last tillage operation. Furthermore, in these situations, nematodes of the families of Rhabditidae and Panagrolaimidae form dauer larvae, a resting stage that enables them to survive periods of food scarcity (Bongers and Bongers, 1998). Extraction of these immobile resting stages fails when using Oostenbrink elutriators followed by Oostenbrink dish extractions where the nematode yield depends on active nematode movement (Hallmann and Subbotin, 2018). Hence, it is not surprising that both nematode families had lower densities in experiment 1 than in experiment 2 (Figure 1) which is further reflected by higher MI and lower BFP in grass-clover (experiment 1) compared to after potatoes (experiment 2, Table 2). The higher CI indicating an increased significance of fungal degradation pathways and the lower enrichment index indicating a lower level of food resources (Ferris et al., 2001) in experiment 1 compared to experiment 2 confirmed this (Table 2).
The non-inversion tillage system used here is indispensably linked with the mulch application to potatoes that had been applied twice since the start of the long-term experiments (Schmidt et al., 2020). In organic farming systems, slow soil warming is a great challenge in temperate non-inversion tillage systems during spring and often accompanied by yield losses due to reduced nutrient mineralization (Berry et al., 2002; Vincent-Caboud et al., 2017; Junge et al., 2020). Significantly larger pea biomass yields in the biotest (Figure 1) and generally higher nutrient contents in the mulch system (Schmidt et al., 2020) indicated the potential for compensation of reduced soil warming effects under field conditions. For no-till systems, Neher (2010) described a greater predominance of fungivorous nematodes compared to plough systems. The reason is that hyphal networks remain more intact in MT and fungi have better access to crop residues on the soil surface and are, therefore, dominating drivers of organic matter decay. However, we found greater populations of the bacterivorous families of Rhabditidae, Panagrolaimidae, and Cephalobidae in the non-inversion tillage system compared to the plough system independent of the rotational crops. The shallow tillage in the non-inversion tillage system initiates contact of mulch and other aboveground residues with the soil and, therefore, is the most likely reason for a bacterial dominated break-down of crop residues in both tillage systems (Neher, 2010). Furthermore, the bacterivore:fungivore ratio is driven by resource quality and the low C:N ratio of the mulch likely fostered bacterivorous nematodes (Neher, 1999). Although the effect of the tillage and mulch systems on nematodes was similar in both field experiments, two major interactions were found. The populations of Aphelenchidae and Tylenchidae were greater in the plough system than in the minimum tillage system after potatoes (experiment 2) but the situation was vice versa in the grass clover crop (experiment 1) albeit at much lower densities. While the greater population of the fungivorous Aphelenchidae could be explained by the above discussed reduction of residue quality that led to an increased residue break-down through fungi, the situation is more complex for the Tylenchidae. Nematodes of this family belong to the least studied taxa and although they are generally considered to be herbivores, a number of studies clearly demonstrated fungivorous behavior of the genus Filenchus within this family (Okada et al., 2005; Qing and Bert, 2017, 2019). As the family of Tylenchidae is highly diverse, we cannot exclude the presence of additional genera within this family that feed on other resources than herbs, such as algae, mosses, and lichens (Yeates et al., 1993).
The alternating resource quality also caused interactions among the CI, EI, and FFP between the non-inversion tillage system and the plough system. While CI and EI remained relatively stable in the plough tillage system, extreme changes occurred in the non-inversion tillage/mulch system and for FFP a complete reversal occurred among the systems (Table 2). For the latter system, the impact of the alternating resource quality on the CI has been described above. However, the situation is more complex for the EI which is roughly calculated by dividing the bacterivorous enrichment opportunists (e.g., Rhabditidae, Panagrolaimidae) by the basal bacterivorous and fungivorous fauna (Cephalobidae, Aphelenchidae, Aphelenchoididae) (Ferris et al., 2001). Hence, the lower densities of Rhabditidae and Panagrolaimidae and the greater densities of fungivorous nematodes in the non-inversion/mulch system caused the reduction of the EI in experiment 1 compared to experiment 2 and, therefore, were responsible for the tillage by experiment interactions (Table 2). The reversed interactions between tillage systems for the FFP but not for any other footprints used in this study reflected the pattern already observed for the CI. After potatoes (experiment 2), microbial biomass was stimulated by hilling and harvesting in the plough system (Schmidt et al., 2020), thus, leading to generally greater footprints compared to the grass-clover soil (experiment 1, Table 2). The mulch in the non-inversion/mulch system provided food for fungi also 16 month after application (grass-clover, experiment 1) after easy accessible carbohydrates had been degraded by bacteria directly after its application (potatoes, experiment 2). In contrast, food availability in the plough system was scarce in the grass-clover and the FFP, therefore, declined. All other footprints used here were greater significantly in the non-inversion/mulch system compared to the plough system. This significant impact is not surprising as these specific indicators were suggested by Du Preez et al. (2022) for studies on fertilization experiments. Maize straw mulching in a long-term zero tillage system in China increased footprints of fungivorous and bacterivorous nematodes irrespective of the mulch quantity (Luo et al., 2021) confirming our results. Zero tillage in combination with legume-dominated cover crops also increased bacterivore footprints by 8–20% compared to a standard tillage system in California, USA (Zhang et al., 2017). As this is far less than the 77–115% increase observed under non-inversion tillage system in our study, we assume that the mulch application had the greatest effect on footprints. This is confirmed by about 20–45% increased enrichment and structure footprints after application of 15 t ha−1 banana and passion fruit mulch to zero, reduced and plough-based tillage systems (Zhong et al., 2017).
Nematode Footprints Predict Soil Disease Suppressiveness But Not Fertility
Nematodes have been broadly used to study soil processes under various environmental conditions, but have never been investigated in relation to soil suppressiveness to soil-borne fungi. One reason may be the long-term (management) processes that render a soil suppressive. The long-term experiments used in this study were supposed to have an already stabilized food web that should simplify correlations of nematode-based bioindicators and disease suppressiveness. However, we found that nematode food webs were predominantly depending on the actual crop and the associated soil disturbance in the rotations including fertilizer/ mulch inputs (Figure 3; Table 2) which had been described recently as main drivers of nematode communities (Neher et al., 2019; Schmidt et al., 2020). Notwithstanding, we found significant negative correlations among DI and MFP (Figure 4) in both field experiments indicating a potential usefulness of MFP as indicator for suppressiveness of soil borne diseases even in variable environments. This is likely due to the direct link of the MFP to the nematode biomass which predominantly depends on available prey (bacteria, fungi, plants, other nematodes). Greater microbial activity and biomass in the soil also increases the nematode abundance (Schmidt et al., 2020) and, thus, the MFP which is driven by bacterivorous nematodes in this experiment (Figure 4). Both microbial parameters are known indicators of soil health (Stirling, 2014) and the MFP, therefore, combines both to one major indicator, that can be easily assessed using simple nematode extraction and identification methods. The value of MFPs as general indicator is also expressed in its low sensitivity to nematode dominance structures. For example, bacterivorous and herbivorous nematodes generally dominate in agricultural farming systems (Yeates et al., 1997; Luo et al., 2021) while in forests, fungivorous nematodes can be of similar or even greater importance than bacterivores and herbivores (Neher et al., 2004; Sánchez-Moreno and Ferris, 2007; Zhang et al., 2015; McQueen and Treonis, 2020).
In contrast to a previous study with the same soil (Schmidt et al., 2020), we did not find any correlation of nematode-based indicators and pea biomass production (Figure 4). This was surprising as pea yields in the biotest of our study were similar to those observed previously (Schmidt et al., 2020). One reason could be that the number of pairs for the correlation was lower (n = 8) in the current study than in the experiment by Schmidt et al. (2020) (n = 16). The irregular reactions of pea toward artificial inoculation with fungal pathogens could also play a role (Figure 2). For example, significant negative correlations for DI and pea biomass occurred only in soils inoculated with F. redolens (r = −0.78, p = 0.02) in experiment 1 and in soils inoculated with F. avenaceum (r = −0.87, p = 0.006) but not within the other three inoculation treatments including the control in either experiment (data not shown).
This study demonstrates the usefulness of nematode assessments and the derivation of indicators out of these results to evaluate the soils' suppressive potential toward diseases. Especially the non-inversion tillage system combined with frequent mulch and compost applications not only increased the soil disease suppressiveness but also pea yields in biotests and, therefore, provides an option for biological systems management. Our study further highlights the importance of increasing soil organic matter to achieve disease control caused by soil-borne fungi. Nevertheless, this work can be seen only as a start of series of similar experiments that are conducted by researchers on a global scale and whose data are needed to confirm the predictive ability of the MFP for disease suppressive soils. New studies should consider different climatic regions as well as various soil conditions, preferentially in long-term experiments with greater stability of soil building processes compared to short-term field trials. Lastly, there is a great demand for more studies that accompany single (soil) biological assessments by agronomic measurements that allow economical evaluation of ecosystem services. The metabolic carbon footprint of nematodes, in particular, appears promising for such research questions.
Data Availability Statement
The original contributions presented in the study are included in the article/supplementary materials and the complete raw datasets for this study are published in the Open Agrar repository: https://doi.org/10.5073/20220321-151638. Further inquiries can be directed to the corresponding author/s.
Author Contributions
JS, MF, and AŠ: conceptualization and methodology. JS, LT, and AŠ: investigation. JS: formal analysis and writing—original draft. AŠ, LT, MF, and JS: writing—review and editing. JS and MF: funding acquisition. MF: resources. All authors have read and agreed to the published version of the manuscript.
Funding
This research was funded by the Deutsche Forschungsgemeinschaft (DFG, German Research Foundation)—Project no. 420414676: The self-regulatory potential of agro-ecosystems: Using nematodes as indicators for legume disease suppressive soils, by the European Union FP7 Project n.289277: OSCAR (Optimizing Subsidiary Crop Applications in Rotations), and by the German BMBF Project no. FKZ 031A350C: INSUSFAR (Innovative approaches to optimizing genetic diversity for sustainable farming systems of the future).
Conflict of Interest
The authors declare that the research was conducted in the absence of any commercial or financial relationships that could be construed as a potential conflict of interest.
Publisher's Note
All claims expressed in this article are solely those of the authors and do not necessarily represent those of their affiliated organizations, or those of the publisher, the editors and the reviewers. Any product that may be evaluated in this article, or claim that may be made by its manufacturer, is not guaranteed or endorsed by the publisher.
Acknowledgments
The authors would like to thank Matthias von Ahn for his excellent assistance in nematode extraction and counting, as well as for his thorough maintenance of the greenhouse experiments.
References
Andrássy, I. (2005). Free-Living Nematodes of Hungary: Nematoda Errantia. Budapest: Hungarian Natural History Museum : Systematic Zoology Research Group of the Hungarian Academy of Sciences.
Baćanović-Šišić, J., Šišić, A., Schmidt, J. H., and Finckh, M. R. (2018). Identification and characterization of pathogens associated with root rot of winter peas grown under organic management in Germany. Eur. J. Plant Pathol. 151, 745–755. doi: 10.1007/s10658-017-1409-0
Bailey, K. L., and Lazarovits, G. (2003). Suppressing soil-borne diseases with residue management and organic amendments. Soil Tillage Res. 72, 169–180. doi: 10.1016/S0167-1987(03)00086-2
Berry, P. M., Sylvester-Bradley, R., Philipps, L., Hatch, D. J., Cuttle, S. P., Rayns, F. W., et al. (2002). Is the productivity of organic farms restricted by the supply of available nitrogen? Soil Use Manag. 18, 248–255. doi: 10.1079/SUM2002129
Boerema, G. H., (ed). (2004). Phoma Identification Manual: Differentiation of Specific and Infra-Specific Taxa in Culture. Wallingford, Oxfordshire; Cambridge, MA: CABI Pub.
Böhm, H., Dauber, J., Dehler, M., Gallardo, D. A. A., Witte, T., de Fuß, R., et al. (2020). Fruchtfolgen mit und ohne leguminosen: ein review. JFK 72, 489–509. doi: 10.5073/JfK.2020.10-11.01
Bonanomi, G., Lorito, M., Vinale, F., and Woo, S. L. (2018). Organic amendments, beneficial microbes, and soil microbiota: Toward a unified framework for disease suppression. Ann. Rev. Phytopathol. 56, 1.1–1.20. doi: 10.1146/annurev-phyto-080615-100046
Bongers, T. (1990). The maturity index: an ecological measure of environmental disturbance based on nematode species composition. Oecologia 83, 14–19. doi: 10.1007/BF00324627
Bongers, T. (1994). De nematoden van Nederland: een identificatietabel voor de in Nederland aangetroffen zoetwater- en bodembewonende nematoden. 2. druk. Utrecht: Stichting Uitg. KNNV.
Bongers, T., and Bongers, M. (1998). Functional diversity of nematodes. Appl. Soil Ecol. 10, 239–251. doi: 10.1016/S0929-1393(98)00123-1
Bünemann, E. K., Bongiorno, G., Bai, Z., Creamer, R. E., De Deyn, G., de Goede, R., et al. (2018). Soil quality – A critical review. Soil Biol. Biochem. 120:105–125. doi: 10.1016/j.soilbio.2018.01.030
Cook, R. J., and Baker, K. F. (1983). The Nature and Practice of Biological Control of Plant Pathogens. St. Paul, MA: APS Press.
Coons, G. H. (1916). Factors involved in the growth and the pycnidium formation of Plenodomus fuscomaculans. J. Agric. Res. 5, 713–769.
Creamer, R. E., Barel, J. M., Bongiorno, G., and Zwetsloot, M. J. (2022). The life of soils: Integrating the who and how of multifunctionality. Soil Biol. Biochem. 166, 108561. doi: 10.1016/j.soilbio.2022.108561
Du Preez, G., Daneel, M., De Goede, R., Du Toit, M., Ferris, H., Fourie, H., et al. (2022). Nematode-based indices in soil ecology: application, utility and future directions. Soil Biol. Biochem. 169:108640. doi: 10.1016/j.soilbio.2022.108640
Ferris, H. (2010a). Contribution of nematodes to the structure and function of the soil food web. J. Nematol. 42, 63–67.
Ferris, H. (2010b). Form and function: metabolic footprints of nematodes in the soil food web. Eur. J. Soil Biol. 46, 97–104. doi: 10.1016/j.ejsobi.2010.01.003
Ferris, H., and Bongers, T. (2009). “Indices developed specifically for analysis of nematode assemblages,” in Nematodes as Environmental Indicators, eds Wilson, M. J., and Kakouli-Duarte, T. (Wallingford; Cambridge, MA: CABI), 124–145.
Ferris, H., Bongers, T., and de Goede, R. G. M. (2001). A framework for soil food web diagnostics: extension of the nematode faunal analysis concept. Agric. Ecosyst. Environ. Appl. Soil Ecol. 18, 13–29. doi: 10.1016/S0929-1393(01)00152-4
Finckh, M. R., Yli-Mattila, T., Nykänen, A., Kurki, P., and Hannukkala, A. (2015). “Organic temperate legume disease management,” in Plant Diseases and their Management in Organic Agriculture, eds Finckh Maria, R., van Bruggen, A. H. C., Tamm, L. (St. Paul, MN: American Phytopathological Society), 293–310.
Fox, J., and Weisberg, S. (2019). An R Companion to Applied Regression. 3rd Edn. Thousand Oaks, CA: SAGE Publications.
Govaerts, B., Mezzalama, M., Sayre, K. D., Crossa, J., Lichter, K., Troch, V., et al. (2008). Long-term consequences of tillage, residue management, and crop rotation on selected soil micro-flora groups in the subtropical highlands. Appl. Soil Ecol. 38, 197–210. doi: 10.1016/j.apsoil.2007.10.009
Grzyb, A., Wolna-Maruwka, A., and Niewiadomska, A. (2020). Environmental factors affecting the mineralization of crop residues. Agronomy. 10, 1951. doi: 10.3390/agronomy10121951
Hallmann, J., and Subbotin, S. A. (2018). “Methods for extraction, processing and detection of plant and soil nematodes,” in Plant Parasitic Nematodes in Subtropical and Tropical Agriculture, 3rd Edn, eds Sikora, R. A., Coyne, D., Hallmann, J., and Timper, P. (Boston, MA: CABI), 87–119.
Harrell, F. E. Jr. (2020). Hmisc: Harrell Miscellaneous. Available online at: https://CRAN.R-project.org/package=Hmisc (accessed March 20, 2020).
Junge, S. M., Storch, J., Finckh, M. R., and Schmidt, J. H. (2020). “Developing organic minimum tillage farming systems for Central and Northern European conditions,” in No-Till Farming Systems for Sustainable Agriculture: Challenges and Opportunities, eds Dang, Y. P., Dalal, R. C., and Menzies, N. W. (Cham: Springer), 173–192.
Korthals, G. W., Bongers, T., Kammenga, J. E., Alexiev, A. D., and Lexmond, T. M. (1996). Long-term effects of copper and pH on the nematode community in an agroecosystem. Environ. Toxicol. Chem. 15, 979–985. doi: 10.1002/etc.5620150621
Krauss, M., Berner, A., Perrochet, F., Frei, R., Niggli, U., and Mäder, P. (2020). Enhanced soil quality with reduced tillage and solid manures in organic farming – a synthesis of 15 years. Sci. Rep. 10, 4403. doi: 10.1038/s41598-020-61320-8
Landi, S., Papini, R., d'Errico, G., Barzanti, G. P., and Roversi, P. F. (2018). Nematode indicators as integrative measures of soil condition during conversion from conventional to organic rice production in Italy: a case study. Biol. Agric. Hortic. 34, 141–153. doi: 10.1080/01448765.2017.1399166
Le May, C., Guibert, M., Baranger, A., and Tivoli, B. (2014). A wide range of cultivated legume species act as alternative hosts for the pea aschochyta blight fungus, Didymella pinodes. Plant Pathol. 63, 877–887. doi: 10.1111/ppa.12154
Lenth, R. (2020). emmeans: Estimated Marginal Means, Aka Least-Squares Means. Available online at: https://CRAN.R-project.org/package=emmeans(accessed February 10, 2020).
Leslie, J. F., and Summerell, B. A. (2006). The Fusarium Laboratory Manual. Ames, IA: Blackwell Publishing.
Luo, J., Zhang, X., Kou, X., Xie, H., Bao, X., Mahamood, M., et al. (2021). Effects of residue mulching amounts on metabolic footprints based on production and respiration of soil nematodes in a long-term no-tillage system. Land Degrad. Dev. 32, 2383–2392. doi: 10.1002/ldr.3918
Mazzola, M. (2004). Assessment and management of soil microbial community structure for disease suppression. Annu. Rev. Phytopathol. 42, 35–59. doi: 10.1146/annurev.phyto.42.040803.140408
McQueen, J. P., and Treonis, A. M. (2020). Cacao agroforestry in Belize: effects on soil nematode community structure. Agroforest Syst. 94, 1123–1132. doi: 10.1007/s10457-019-00477-2
Neher, D. A. (1999). Nematode communities in organically and conventionally managed agricultural soils. J. Nematol. 31, 142–154.
Neher, D. A. (2001). Role of nematodes in soil health and their use as indicators. J. Nematol. 33, 161–168.
Neher, D. A. (2010). Ecology of plant and free-living nematodes in natural and agricultural soil. Annu. Rev. Phytopathol. 48:371–394. doi: 10.1146/annurev-phyto-073009-114439
Neher, D. A., Nishanthan, T., Grabau, Z. J., and Chen, S. Y. (2019). Crop rotation and tillage affect nematode communities more than biocides in monoculture soybean. Agric. Ecosyst. Environ. Appl. Soil Ecol. 140:89–97. doi: 10.1016/j.apsoil.2019.03.016
Neher, D. A., Peck, S. L., Rawlings, J. O., and Campbell, C. L. (1995). Measures of nematode community structure and sources of variability among and within agricultural fields. Plant Soil. 170, 167–181. doi: 10.1007/BF02183065
Neher, D. A., Weicht, T. R., Moorhead, D. L., and Sinsabaugh, R. L. (2004). Elevated CO2 alters functional attributes of nematode communities in forest soils. Funct. Ecol. 18, 584–591. doi: 10.1111/j.0269-8463.2004.00866.x
Nirenberg, H. (1976). Untersuchungen über die morphologische und biologische Differenzierung in der Fusarium-Sektion Liseola. Berlin-Dahlem: Biologischen Bundesanstalt für Land- und Forstwirtschaft.
Nylander, J. (2004). MrModeltest V2. Program distributed by the author. Bioinformatics 24, 581–583. doi: 10.1093/bioinformatics/btm388
Okada, H., Harada, H., and Kadota, I. (2005). Fungal-feeding habits of six nematode isolates in the genus Filenchus. Soil Biol. Biochem. 37, 1113–1120. doi: 10.1016/j.soilbio.2004.11.010
Oyarzun, P. J., Dijst, G., and Maas PWTh. (1994). Determination and analysis of soil receptivity to Fusarium solani f. sp. pisi causing dry root rot of peas. Phytopathology 84, 834–842. doi: 10.1094/Phyto-84-834
Oyarzun, P. J., Gerlagh, M., and Zadoks, J. C. (1998). Factors associated with soil receptivity to some fungal root rot pathogens of peas. Appl. Soil Ecol. 10, 151–169. doi: 10.1016/S0929-1393(98)00042-0
Paulitz, T. C., Schroeder, K. L., and Schillinger, W. F. (2010). Soilborne pathogens of cereals in an irrigated cropping system: effects of tillage, residue management, and crop rotation. Plant Dis. 94, 61–68. doi: 10.1094/PDIS-94-1-0061
Pflughöft, O., Merker, C., Tiedemann, A., and Schäfer, B. C. (2012). Zur Verbreitung und Bedeutung von Pilzkrankheiten in Körnerfuttererbsen (Pisum sativum L.) in Deutschland. Gesunde Pflanzen. 64, 39–48. doi: 10.1007/s10343-011-0270-x
Pinheiro, J., Bates, D., DebRoy, S., Sarkar, D., and R Core Team (2020). nlme: Linear and Nonlinear Mixed Effects Models. Available online at: https://CRAN.R-project.org/package=nlme (accessed October 15, 2020).
Qing, X., and Bert, W. (2017). Redefinition of genus Malenchus Andrássy, 1968 (Tylenchomorpha: Tylenchidae) with additional data on ecology. J. Nematol. 49, 189–206. doi: 10.21307/jofnem-2017-064
Qing, X., and Bert, W. (2019). Family Tylenchidae (Nematoda): an overview and perspectives. Org. Divers. Evol. 19, 391–408. doi: 10.1007/s13127-019-00404-4
R Core Team (2020). R: A Language and Environment for Statistical Computing. Vienna: R Foundation for Statistical Computing. Available online at: https://www.r-project.org/ (accessed March 24, 2016).
Sánchez-Moreno, S., and Ferris, H. (2007). Suppressive service of the soil food web: Effects of environmental management. Agric. Ecosyst. Environ. 119, 75–87. doi: 10.1016/j.agee.2006.06.012
Schlatter, D., Kinkel, L., Thomashow, L., Weller, D., and Paulitz, T. (2017). Disease suppressive soils: new insights from the soil microbiome. Phytopathology 107, 1284–1297. doi: 10.1094/PHYTO-03-17-0111-RVW
Schmidt, J. H., Finckh, M. R., and Hallmann, J. (2017). Oilseed radish/black oat subsidiary crops can help regulate plant-parasitic nematodes under non-inversion tillage in an organic wheat-potato rotation. Nematology 19, 1135–1146. doi: 10.1163/15685411-00003113
Schmidt, J. H., Hallmann, J., and Finckh, M. R. (2020). Bacterivorous nematodes correlate with soil fertility and improved crop production in an organic minimum tillage system. Sustainability 12, 6730. doi: 10.3390/su12176730
Sieriebriennikov, B., Ferris, H., and de Goede, R. G. M. (2014). NINJA: An automated calculation system for nematode-based biological monitoring. Eur. J. Soil Biol. 61, 90–93. doi: 10.1016/j.ejsobi.2014.02.004
Šišić, A., Baćanović-Šišić, J., Al-Hatmi, A. M. S., Karlovsky, P., Ahmed, S. A., Maier, W., et al. (2018a). The ‘forma specialis' issue in Fusarium: a case study in Fusarium solani f. sp. pisi. Sci. Rep. 8, 1252. doi: 10.1038/s41598-018-19779-z
Šišić, A., Baćanović-Šišić, J., Karlovsky, P., Wittwer, R., Walder, F., Campiglia, E., et al. (2018b). Roots of symptom-free leguminous cover crop and living mulch species harbor diverse Fusarium communities that show highly variable aggressiveness on pea (Pisum sativum). PLoS ONE 13, e0191969. doi: 10.1371/journal.pone.0191969
Šišić, A., and Finckh, M. R. (2021). Extension and Agronomic Evaluation Practice Surveys and Investigations in the Context of Exemplary Demonstration Networks Soya and Lupine of the Protein Crop Strategy. Witzenhausen: Universität Kassel. Available online at: https://orgprints.org/id/eprint/39709/ (accessed January 7, 2022).
Šišić, A., Oberhänsli, T., Baćanović-Šišić, J., Hohmann, P., and Finckh, M. R. (2021). A novel real time PCR method for the detection and quantification of Didymella pinodella in symptomatic and asymptomatic plant hosts. J. Fungi 8, 41. doi: 10.3390/jof8010041
Steel, H., and Ferris, H. (2016). Soil nematode assemblages indicate the potential for biological regulation of pest species. Acta Oecol. 73, 87–96. doi: 10.1016/j.actao.2016.03.004
Stirling, G. R. (2014). Biological Control of Plant-parasitic Nematodes: Soil Ecosystem Management in Sustainable Agriculture, 2nd Edn. Wallingford: CAB International.
Stirling, G. R., Smith, M. K., Smith, J. P., Stirling, A. M., and Hamill, S. D. (2012). Organic inputs, tillage and rotation practices influence soil health and suppressiveness to soilborne pests and pathogens of ginger. Aust. Plant Pathol. 41, 99–112. doi: 10.1007/s13313-011-0096-0
van Bruggen, A. H. C., van Semenov, A. M., van Diepeningen, A. D., van Vos, O. J., and de Blok, W. J. (2006). Relation between soil health, wave-like fluctuations in microbial populations, and soil-borne plant disease management. Eur. J. Plant Pathol. 115, 105–122. doi: 10.1007/s10658-005-1250-8
van den Hoogen, J., Geisen, S., Routh, D., Ferris, H., Traunspurger, W., Wardle, D. A., et al. (2019). Soil nematode abundance and functional group composition at a global scale. Nature 572, 194–198. doi: 10.1038/s41586-019-1418-6
Vincent-Caboud, L., Peigné, J., Casagrande, M., and Silva, E. (2017). Overview of organic cover crop-based no-tillage technique in Europe: farmers' practices and research challenges. Agriculture 7, 42. doi: 10.3390/agriculture7050042
Wasilewska, L. (1979). The structure and function of soil nematode communities in natural ecosystems and agrocenoses. Pol. Ecol. Stud. 5, 97–146.
Wille, L., Kurmann, M., Messmer, M. M., Studer, B., and Hohmann, P. (2021). Untangling the pea root rot complex reveals icrobial markers for plant health. Front. Plant Sci. 12, 737820. doi: 10.3389/fpls.2021.737820
Yeates, G. W., Bardgett, R. D., Cook, R., Hobbs, P. J., Bowling, P. J., and Potter, J. F. (1997). Faunal and microbial diversity in three Welsh grassland soils under conventional and organic management regimes. J. Appl. Ecol. 34, 453–470. doi: 10.2307/2404890
Yeates, G. W., Bongers, T., De Goede, R. G. M., Freckman, D. W., and Georgieva, S. S. (1993). Feeding habits in soil nematode families and genera—an outline for soil ecologists. J. Nematol. 25, 315–331.
Zhang, X., Ferris, H., Mitchell, J., and Liang, W. (2017). Ecosystem services of the soil food web after long-term application of agricultural management practices. Soil Biol. Biochem. 111, 36–43. doi: 10.1016/j.soilbio.2017.03.017
Zhang, Z. Y., Zhang, X. K., Jhao, J. S., Zhang, X. P., and Liang, W. J. (2015). Tillage and rotation effects on community composition and metabolic footprints of soil nematodes in a black soil. Eur. J. Soil Biol. 66, 40–48. doi: 10.1016/j.ejsobi.2014.11.006
Zhong, S., Zeng, H., and Jin, Z. (2017). Influences of different tillage and residue management systems on soil nematode community composition and diversity in the tropics. Soil Biol. Biochem. 107, 234–243. doi: 10.1016/j.soilbio.2017.01.007
Zuur, A., Leno, E. N., Walker, N., Saveliev, A. A., and Smith, G. M. (2009). Mixed Effects Models and Extensions in Ecology With R. New York, NY: Springer-Verlag.
Keywords: long-term experiment, nematode populations, organic agriculture, non-inversion tillage, nematode-based bioindicators
Citation: Schmidt JH, Theisgen LV, Finckh MR and Šišić A (2022) Increased Resilience of Peas Toward Root Rot Pathogens Can Be Predicted by the Nematode Metabolic Footprint. Front. Sustain. Food Syst. 6:881520. doi: 10.3389/fsufs.2022.881520
Received: 22 February 2022; Accepted: 04 April 2022;
Published: 10 May 2022.
Edited by:
Khurram Shahzad, Lasbela University of Agriculture, Water and Marine Sciences, PakistanReviewed by:
Deborah A. Neher, University of Vermont, United StatesSubhan Danish, Bahauddin Zakariya University, Pakistan
Copyright © 2022 Schmidt, Theisgen, Finckh and Šišić. This is an open-access article distributed under the terms of the Creative Commons Attribution License (CC BY). The use, distribution or reproduction in other forums is permitted, provided the original author(s) and the copyright owner(s) are credited and that the original publication in this journal is cited, in accordance with accepted academic practice. No use, distribution or reproduction is permitted which does not comply with these terms.
*Correspondence: Jan H. Schmidt, amFuLWhlbnJpay5zY2htaWR0JiN4MDAwNDA7anVsaXVzLWt1ZWhuLmRl