- Functional Ecology and Environment Engineering Laboratory, Faculty of Science and Technology, Sidi Mohamed Ben Abdellah University, Fez, Morocco
Developing bioactive food packaging, capable of extending the shelf life of fruits, has received increasing attention in recent years. The present study highlights the interest in post-harvest treatment for strawberries with chitosan as a preservation solution. Chitosan extraction was carried out from shrimp shells (Parapenaeus longirostris), composed of chitin, using citric acid during the demineralization step. Extracted chitosan was characterized by Fourier transform infrared (FTIR) spectroscopy, X-ray diffraction (XRD), and scanning electron microscopy (SEM). The presence of amino group (-NH2) in the obtained chitosan was confirmed by infrared (IR) spectral data. Deacetylation degree (DD), which has a value of 80.86%, was determined by FTIR spectra. X-ray diffraction pattern (XRD) showed two peaks of crystalline character, characteristic of extracted chitosan, approximately at 20° and 30° (2θ). Extracted chitosan morphology was studied by scanning electron microscopy (SEM) and showed a relatively smooth top surface and fibrous structures. Chitosan, acetic acid, and their interaction effects were evaluated on Aspergillus niger mycelial growth strain isolated from spoiled strawberries. Chitosan revealed a strong anti-fungal activity, dose-dependent (from 0 to 3%), on Aspergillus niger mycelial growth, while acetic acid showed moderate anti-fungal activity against the Aspergillus niger strain. Agri-food application was carried out using chitosan solubilized in acetic acid as a post-harvest treatment tool for the prolongation of shelf life of strawberries (by using an experimental design). Coating, with the developed preservative solution, significantly reduced microbial spoilage in strawberries. Treated strawberries retained their initial pigmentation for a longer period when compared to untreated strawberries. The treatment carried out maintained the cellular structures of treated strawberries during the storage period and thus extended the shelf life of strawberries which is considered very susceptible to reduce post-harvest losses.
Introduction
Fruits are biodegradable and are subjected to enzymatic and microbial alteration due to physiological and biochemical changes that occur after harvest. Several pathogens, such as fungi and bacteria, are responsible for post-harvest spoilage in fruits and vegetables (Singh and Sharma, 2018). Some fruits are very susceptible to rot and perish, due to over-ripening, softening, and dislocation. This limits their potential for storage, handling, and transportation. Post-harvest losses reduce the income of producers and distributors, limit organic farming development, and increase the final selling product price, with negative consequences on consumers (Lurol et al., 2019).
Strawberries are fragile fruits that spoil quickly after harvest and are so considered highly perishable due to their susceptibility to spoilage, mechanical damage, physiological disorders, and fungal decay (Trinetta et al., 2020). They are susceptible to post-harvest losses and therefore have a very limited shelf life. Post-harvest treatments of fruits are of great importance in order to improve the shelf life of these harvested products, while minimizing losses and maximizing profits.
Several methods have been proposed to combat post-harvest fruit losses, such as low-temperature and controlled atmosphere storage (Anese et al., 2020), and usage of edible coatings (Cazón and Vázquez, 2019). The use of bioactive compounds such as chitosan, to control post-harvest fungal attacks, has received much attention due to future problems with chemical agents, which include increasing public resistance to fungicide-treated products and increase fungicide-tolerant pathogens (Betchem et al., 2019).
Chitosan is the only natural alkaline polysaccharide that has good biological inter-miscibility and biodegradable properties (Liang et al., 2017). This non-toxic active biopolymer, most abundant after cellulose, is generally found in crustacean exoskeletons (Harkin et al., 2019) and is obtained by the partial deacetylation of chitin. Chitosan exhibits antimicrobial activity against a wide range of foodborne filamentous fungi, yeasts, and bacteria. The antimicrobial properties and the ability to form films make chitosan the first reference polymer for developing active food packaging, capable of inhibiting the growth of microorganisms and improving food safety (Cazón and Vázquez, 2019). However, with recent developments in chitosan, it is now a promising way to control post-harvest rot. Fruit coating has become one of the most popular methods for extending the shelf life of many fruits by delaying ripening, water loss, and rotting (Betchem et al., 2019).
Morocco is one of the countries that produce strawberries to export in a very competitive market. Quality remains an objective that justifies the procedural and technological improvements introduced to reduce post-harvest losses and facilitate management logistics. Strawberries have physical–chemical characteristics that limit the growth of most bacteria but remain vulnerable to the invasion of molds that are well-adapted to their acidic character. Thus, the losses are a consequence of the proliferation of molds. In this study, we used the Aspergillus niger strain as a model to develop a biological, less expensive, and effective anti-fungal treatment. The present study aims at prolonging the post-harvest preservation of strawberries. This study relates to chitosan extracted by citric acid and then solubilized in acetic acid to protect the cellular structures of strawberries against microbiological and biochemical alterations. This study concerns the use of organic acid for the demineralization of shrimp shells. The use of organic acids does not harm the environment, reduces the cost of production, and increases the bioavailability of biological activities, namely, antimicrobial activity and antioxidant activity.
The present study highlights the post-harvest treatment of strawberries with chitosan as a preservative solution. Chitosan extraction was carried out from shrimp shells (Parapenaeus longirostris) commercialized in Morocco. It was characterized by Fourier transform infrared (FTIR) spectrometry, X-ray diffraction (XRD), and scanning electron microscopy (SEM). Furthermore, the effect of chitosan and acetic acid and their interaction were evaluated on mycelial Aspergillus niger growth strain isolated from spoiled strawberries. The last part of this work was devoted to the optimization effects of chitosan and acetic acid on Aspergillus niger, by experimental design (22 = 4 experiments), in the post-harvest conservation of strawberries.
Materials and methods
Biological materials
Chitosan extraction was carried out from shrimp shells (Parapenaeus longirostris) marketed in Morocco. Anti-fungal activities of chitosan and acetic acid were evaluated against Aspergillus niger mycelial growth. These fungal strains are isolated from spoiled strawberries. Strawberries with lesions were disinfected with 1% sodium hypochlorite. Then, strawberries were placed in Petri dishes containing potato sucrose agar (PSA) and incubated for 7 days in the dark at 24°C. Optical microscopic examination of the different cultures allowed for the determination of fungal species using identification keys.
Chitosan extraction
Chitosan extraction was performed after removing loosened tissues from shrimp shells. Subsequently, shells were washed, dried, and ground to obtain a dry powder. Deproteinization process was carried out with 30 g of powdered shrimp shell using sodium hydroxide (NaOH) at 5% (1.25 N) at a ratio of 1 g:8 ml (w/v), all refluxed at 70°C for 3 h. The product was collected and washed until a clear solution was obtained, and then dried under vacuum. Discoloration was carried out with pure acetone for 24 h. Product was recovered and washed until neutral, then dried (Kandile et al., 2018). Decolorized product was demineralized using 1% citric acid at a ratio of 1 g:10 ml (w/v) for 24 h at room temperature. Obtained chitin was collected and washed to obtain a light brown powder. The deacetylation of the purified chitin was carried out using sodium hydroxide (NaOH) at 55% (12.5 N) with a ratio of 1 g:5 ml (w/v) at 100°C for 12 h. Obtained chitosan was washed with distilled water and completely dried (Kandile et al., 2018).
Extracted chitosan characterization
The prepared chitosan was characterized by Fourier transform infrared (FTIR) spectroscopy, X-ray diffraction (XRD) technique, and scanning electron microscopy (SEM). These analyzes were carried out at the Regional University Center of Interface (CURI) attached to Sidi Mohamed Ben Abdellah University (USMBA) in Fez.
Fourier transform infrared (FTIR) spectroscopy
Chitosan, extracted for potential upgrading, was characterized by Fourier transform infrared (FTIR) spectroscopy using KBr pellets in the scanning range of 400–4,000 cm−1. The FTIR spectra of chitosan were measured (Bruker Vertex 70) with a resolution of 4 cm−1.
Degree of deacetylation (DD) determination
The FTIR spectra of chitosan extracted by citric acid were obtained in a frequency range of 400–4,000 cm−1. The degree of deacetylation (DD) of the extracted chitosan was calculated from the FTIR spectra using the method proposed by Khan et al. (2002).
• A1655: Absorbance of chitosan at 1,655 cm−1
• A3450: Absorbance of chitosan at 3,450 cm−1
X-ray diffraction (XRD)
The chitosan extracted with an organic acid was characterized by an X-ray diffractometer (X'Pert-Pro) with CuKa radiation (voltage = 40 kV, intensity = 30 mA). The X-ray diffractometer includes a 2θ goniometer.
Scanning electron microscopy (SEM)
The morphology of the extracted chitosan was investigated by a scanning electron microscope (SEM) (JEOL JSM-IT500HR) at a magnification range of × 600.000, an accelerating voltage of 0.5–30.0 kV, and a maximum sample size of 200 mm in diameter and 75 mm in height.
Effect of chitosan and acetic acid on Aspergillus niger growth
Anti-fungal activity of chitosan and acetic acid on Aspergillus niger mycelial growth was evaluated on the malt agar solid medium. Aspergillus niger inoculation was done by deposing aseptically culture of (5 mm) on a culture medium containing increasing concentrations of chitosan (from 0 to 3%). The same test was carried out on a culture medium containing increasing concentrations of acetic acid (from 0 to 3%). Growth kinetics was monitored by evaluating mycelial diameters' growth. Aspergillus niger growth rates (μ) were calculated by the following formula:
• μ: Aspergillus niger growth rate
• D2 and D1: Mycelia growth diameters
• Δt: Time Unit (t2-t1)
Optimization of the effect of chitosan and acetic acid on Aspergillus niger growth in the post-harvest preservation of strawberries
The strategy for optimizing the effect of chitosan and acetic acid effect Aspergillus niger in the post-harvest treatment of strawberries was carried out by the two-level experimental design method (22 = 4 experiments). The two factors X1 (acetic acid) and X2 (chitosan) levels are shown in Table 1. Table 2 shows the experimental design developed to study the effects of the two factors X1 (acetic acid) and X2 (chitosan) and their interaction (XX2), on the post-harvest conservation of strawberries. Experiments were done on batches of 20 kg and repeated three times.
• Yaa: Response function relating to anti-fungal activity against (Aspergillus niger) strain contaminating strawberries
• Ypig: Pigmentation response function of strawberries
• Yfi : Firmness response function of strawberries

Table 2. Experimental design developed to study the effects of factors (X1: acetic acid and X2: chitosan) and their interaction (XX2) on the shelf life extension of strawberries.
In this part, we carried out the post-harvest treatment of strawberries with chitosan dissolved in acetic acid. First, strawberries were washed and well-drained strawberries (15–30 min) were treated by soaking in a preservation solution. The solution was prepared by dissolving chitosan powder, extracted with citric acid, in acetic acid solution, according to the concentrations elaborated in the experimental design (Table 2). After draining for 5 min, treated strawberries and untreated strawberries (control batch) were stored for 15 days at room temperature.
Mathematical study of factors and their interaction
Experimental design matrix analysis (Table 2) leads to response functions presenting coded factors effects (X1 and X2) and their interaction which has the following formula:
• a0: Model coefficient
• a1 and a2: Coefficients of the linear part of the mathematical model
• a1, 2: Interaction coefficient of the two factors
Mathematical analysis interactions between factors (X1 and X2) present response surfaces and allows the identification of areas where there is a maximum or minimum response of studied response functions (Yaa, Ypig, and Yfi) in the experimental domain.
Response functions
Anti-fungal activity against Aspergillus niger
The effect of treatment on prolonging the storage of post-harvest strawberries was studied. The percentages of contamination by Aspergillus niger were calculated using the following formula:
• NContaminated: Number of strawberries contaminated by Aspergillus niger
• NTotal: Total number of strawberries processed
Pigmentation of strawberries
Pigmentation of treated and untreated strawberries was evaluated by measuring the concentration of anthocyanin pigments by using the spectroscopic method (Giusti and Wrolstad, 2001).
Firmness of strawberries
The firmness of processed strawberries was taken into account the hardness of fruits. This parameter was evaluated by making microscopic observations of strawberry tissue sections. Firmness is a variable that mainly affects the final fruit appearance. A quantitative assessment was developed by a jury based on a questionnaire.
Statistical analyses
The statistical analyses were performed by analysis of variance (ANOVA) and means ± were compared using Duncan's multiple range tests at P ≤ 0.05 with the SPSS statistical software. The mathematical models obtained were also developed with SPSS software. Experimental design (22 = 4 experiments) was done by using JMP 4 software. Response surfaces of experimental design response functions (Yaa, Ypig, and Yfi) were done by Maple 9 software. The effect of chitosan and acetic acid on Aspergillus niger growth and the effect of experimental design factors (X1 and X2) were done in Microsoft excel.
Results and discussion
Chitosan extraction
Crustacean chitin is usually found in a complex matrix with proteins, minerals, and pigments (Kaur and Dhillon, 2015; Shamshina et al., 2016). The most commonly followed protocol for the extraction of chitin from crustacean exoskeletons includes steps such as deproteinization and demineralization, while decolorization is optional (Srinivasan et al., 2018). The main procedure for obtaining chitosan is based on alkaline chitin deacetylation. In the present study, chitosan extraction involved an organic acid (citric acid) for the demineralization of shrimp shells. The use of an organic acid is more environmentally adequate and greatly reduces the production cost, unlike mineral acid application. It is desirable to develop a biological demineralization process durably applicable in the food industry, less expensive, less harmful to environment, and promising for many industries.
Characterization of extracted chitosan
Fourier transform infrared (FTIR) spectroscopy
The final extracted product was characterized by Fourier transform infrared (FTIR) spectroscopy. The later was used to identify functional groups in extracted chitosan. The assignment of absorption bands is based on the literature data (Shigemasa et al., 1996; Duarte et al., 2002; Kumirska et al., 2010).
The FTIR spectral data of chitosan extracted, by citric acid, revealed the following peaks (Figure 1): Peak at 3328.21 cm−1 is attributed to OH stretching vibrations, while peaks at 2919.99 and 2870.06 cm−1 are attributed to the CH-stretching aliphatic vibration in the -CH and -CH2 groups. Amide frequencies consist of N-H bond stretching of amide I and N-H straining vibrations of -NH2 groups are observed at 1649.55 and 1584.17 cm−1, respectively. Peak at 1374.14 cm−1 is attributed to the symmetric deformation of C-H in the -CH3 group. The 1318.70 cm−1 peak is attributed to the vibration modes of amide III. Absorption bands at 1149.69 cm−1 correspond to anti-symmetric stretching of the C-O-C bridge. Stretching vibrations are also observed at 1060.58 and 1022.79 cm−1 for the C-O stretching vibration of alcohol groups. Many authors have observed similar FTIR bands (Dimzon and Knepper, 2015; De Queiroz Antonino et al., 2017; Kumari et al., 2017).
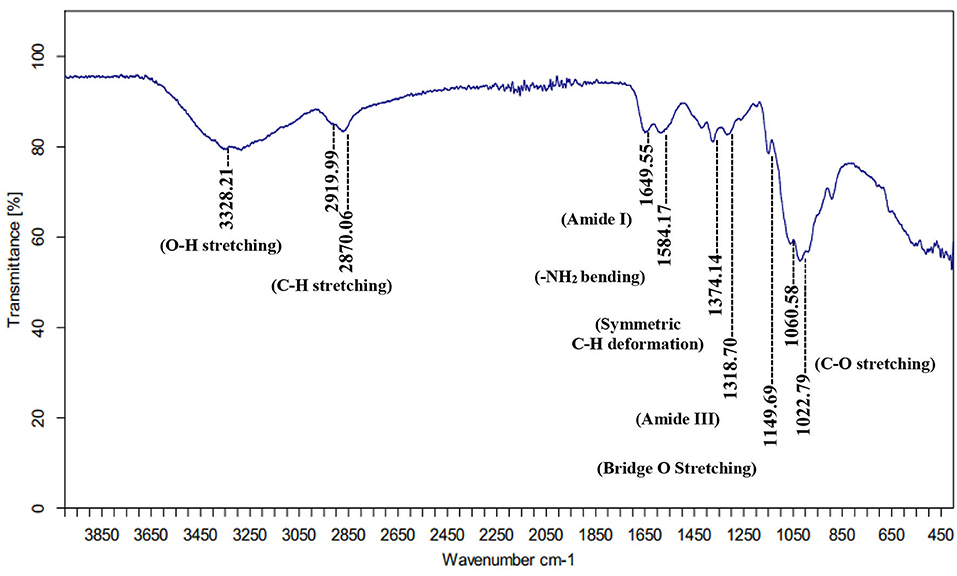
Figure 1. Infrared (IR) spectral data of extracted chitosan identified by Fourier transform infrared (FTIR) spectroscopy.
Deacetylation degree (DD) of chitosan
Deacetylation degree (DD) of extracted chitosan was calculated from the FTIR spectra. In FTIR spectra, different absorption bands represent different functional groups present in chitosan. The determination of DD was done by two absorption bands, a characteristic band that represents the amide group of N-acetyl-D-glucosamine and a reference band that represents a group present in both N-acetyl-D-glucosamine and D-glucosamine (Dimzon and Knepper, 2015).
In the present work, the deacetylation degree of chitosan extracted by citric acid is 80.86%. This parameter is very important because it influences the physical, chemical, and biological properties of this biopolymer. Deacetylation degrees of the chitosan samples, obtained from the shells of white shrimp, were 94.23 and 92.45% when the chitin samples were converted into chitosan by the removal of the acetyl group with sodium hydroxide (NaOH) at 40% (w/v) and 60% (w/v), respectively (Kuyyogsuy, 2020).
X-ray diffraction (XRD)
Chitosan extracted from organic acid was characterized by the X-ray diffraction technique (Figure 2). This later made it possible to analyze the crystallinity of the extracted chitosan. X-ray diffraction pattern (XRD) showed two broad and characteristic diffraction peaks at 2θ around 20° and 30° which are typical fingerprints of semi-crystalline chitosan character. Kuyyogsuy (2020) reported that the XRD pattern of chitosan obtained, from white shrimp shells, revealed characteristic diffraction peaks at 2θ around 10° and 20° corresponding to the crystalline nature of chitosan. Samples of chitosan synthesized from different sources such as fish, shrimp, and crab shells were analyzed by X-ray diffraction (XRD) and diffraction peaks were observed at different values of 2θ such as 20°, 30°, 45°, and 65° (Kumari et al., 2017).
Scanning electron microscopy (SEM)
Morphological characteristics of extracted chitosan were studied by scanning electron microscopy (SEM). Figure 3 shows the surface morphology of chitosan extracted by citric acid at 100 and 5 μm. Chitosan micrographs showed a relatively smooth top surface and fibrous structures. The figure (5 μm) reveals the fibrous nature of extracted chitosan.
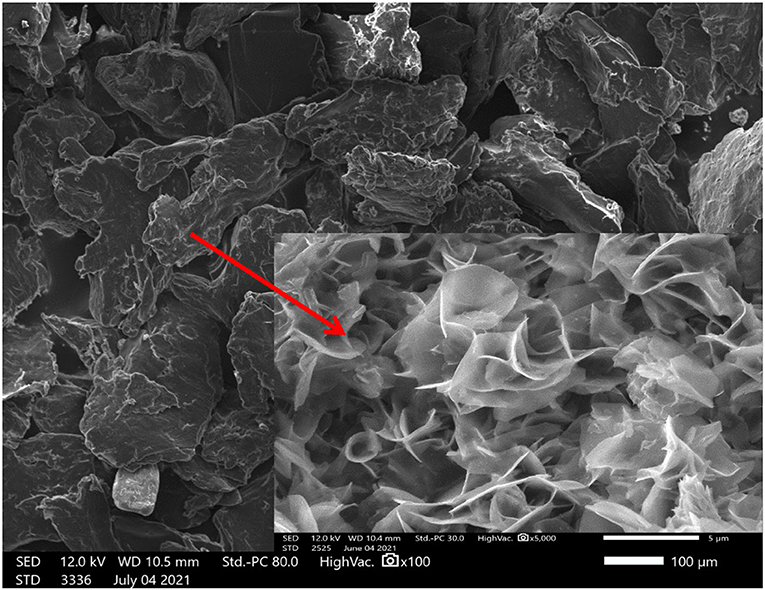
Figure 3. Extracted chitosan morphology, at 100 and 5 μm, identified by scanning electron microscopy (SEM).
Effect of chitosan and acetic acid on Aspergillus niger growth
Anti-fungal activities of chitosan and acetic acid on Aspergillus niger mycelial growth strain were evaluated. Figure 4 shows the growth rates (μ in day−1) of Aspergillus niger according to the concentrations of chitosan and acetic acid.
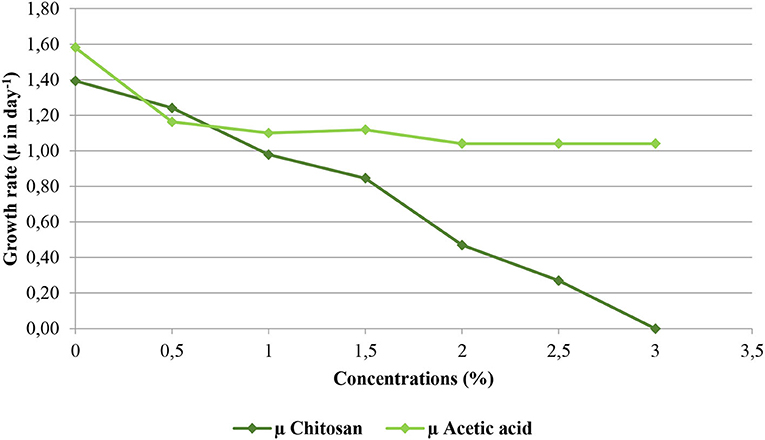
Figure 4. Growth rate (μ) of Aspergillus niger according to the concentrations of chitosan and acetic acid.
The kinetics in Figure 4 initially allowed screening to determine the experimental area studied in Table 3, which shows the effect of interactions between chitosan and acetic acid on microbial load, pigmentation stabilization, and cell structures. These concentrations (Figure 4) allowed us to determine the experimental domain limits of the factors covered by the experimental design.
Aspergillus niger growth rate decreases significantly where chitosan concentration increase. At a 3% chitosan concentration, we observe a total inhibition of mycelial growth. Acetic acid reveals a moderate anti-fungal activity against Aspergillus niger strain because Aspergillus niger can assimilate partially acetic acid, and beyond a concentration of 2% of acetic acid growth rate stabilizes and therefore with the increase in the concentration of acetic acid, toxicity of the latter does not increase.
Chitosan reveals a negative effect on the Aspergillus niger mycelial growth which agree with results reported by other authors. Dewi and Muhammad Nur (2018) reported that chitosan had an inhibitory effect on Aspergillus niger mycelial colonies growth. Higher the concentration of chitosan, lower the average diameter of the mycelial colonies and the higher percentage of inhibition. Chitosan treatment with a concentration of 1.5% inhibited more than 50% of Aspergillus niger growth. Many studies have clearly indicated that chitosan (positively charged) can bind to fungi or bacteria (negatively charged) cell surface, producing plasma membrane permeabilization thereby causing leakage of intracellular materials (Verlee et al., 2017; Xing et al., 2017; Meng et al., 2020).
Optimization of the effect of chitosan and acetic acid on Aspergillus niger in the post-harvest preservation of strawberries
The effect of post-harvest treatment on prolonging shelf life of strawberries was studied. Obtained results from the agro-food application of chitosan extracted by citric acid dissolved in acetic acid as a post-harvest treatment tool for strawberries are shows in Table 3. Values correspond to the average of three repetitions.
• Yaa: Response function relating to anti-fungal activity against germs contaminating strawberries (Aspergillus niger)
• Ypig: Pigmentation response function of strawberries
• Yfi : Firmness response function of strawberries
According to the results obtained in Table 3, we find that the treatment carried out massively reduced microbial load in strawberries during the storage period when compared to untreated fruits (control batch). During the first experiment, 75% of treated strawberries were contaminated with Aspergillus niger, while, during experiment 4, 92% of treated strawberries inhibited mycelial growth. Strawberries treated with the preservative solution are characterized by a bright coloring compared to the control strawberries which have a pale coloring of low luster, and therefore the treatment has a significant effect on the stabilization of pigmentation. In experiment 4, the treatment ensured anthocyanin pigments stability with a percentage of 87.5%. On the one hand, the treatment carried out made it possible to maintain the cellular integrity of the treated strawberries. On the other hand, untreated strawberries softened over time. The firmness of strawberries is significantly improved by the treatment applied. Ripening of treated fruits is thus delayed by a few days compared to the control batch (Figure 5), an important period for the competitiveness of the product. The strawberries treated in experiment 4 maintained their firmness with a percentage of 90%.
The effect of chitosan treatment reveals interesting characteristics to extend the storage time of fruits considered to be very sensitive to post-harvest losses (Figure 5). In this present study, the application of chitosan as a preservative solution for strawberries addressed two main spoilage factors: microbial growth and increased oxidation, thereby extending the shelf life of treated strawberries. These results are related to the functional properties of this polymer, namely, antioxidant, antimicrobial, and film-forming activities. Therefore, this gives promising results as active food packaging. Acetic acid contributed as a solvent in the stabilization of the pigmentation and in the preservation of the cellular structures of the treated strawberries.
The effect of studied factors and their interaction on the microbial load reduction of strawberries
Natural variables (acetic acid and chitosan) coding into synthetic variables (X1 and X2) allowed us to develop an experimental design type 22 (4 experiments). Results of variance analysis of experimental design are shown in Table 4. Table 4 validates the mathematical model and Table 5 validates the coefficients of the model.
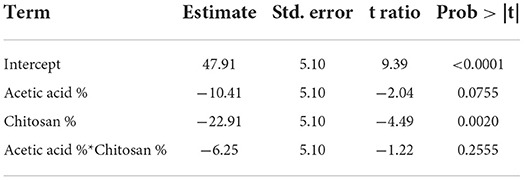
Table 5. Mathematical model parameters estimation in the experimental design related to the microbial load of fruits.
Obtained F (8.6) value is higher than the critical one F′ (4.07) illustrated in the Fisher Snedecor table, which confirms the mathematical model validity illustrated in Table 5.
Response function mathematical model (Equation 1) related to anti-fungal activity against Aspergillus niger obtained is as follows:
• a0 = 47.91 coefficient model
• a1 = −10.41 coefficient related to acetic acid activity
• a2= −22.91 coefficient related to chitosan activity
• a1, 2= −6.25 coefficient representing the interaction effect of the two factors
Student test performed on the coefficients (Table 5) shows that they have a significant effect and consequently we retained all of them. Coefficients analysis sign shows that studied factors and their interactions have a negative effect on the microbial load of strawberries (i.e., its reduction). This is consistent with results obtained from the study of the chitosan effect on Aspergillus niger, where the latter shows strong anti-fungal activity on Aspergillus niger mycelial growth. Effects of studied factors (X1 and X2) and their interaction (XX2) are shown in Figure 6.
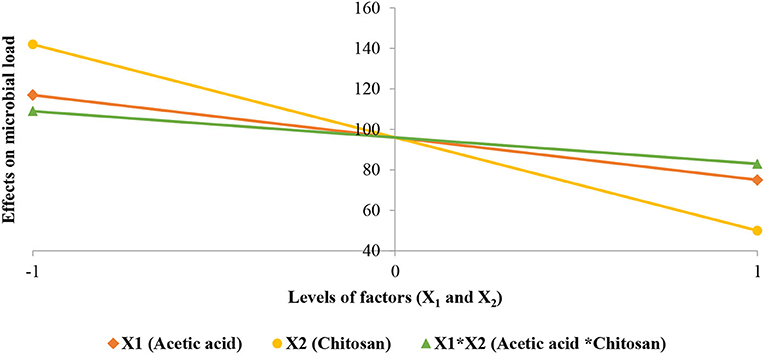
Figure 6. Effects of studied factors (X1 and X2) and their interaction on the microbial load reduction of strawberries.
The interaction of the studied factors is shown in Figure 7 where we observe the response surface according to coded variables (acetic acid and chitosan), which delimits the experimental domain. This figure shows that anti-fungal activity against Aspergillus niger is maximal when acetic acid and chitosan are at the highest level (1.5 and 3%, respectively). Thus, the contamination of strawberries by Aspergillus niger is maximal when both factors X1 and X2 are at the lowest levels (0.5 and 1%, respectively).
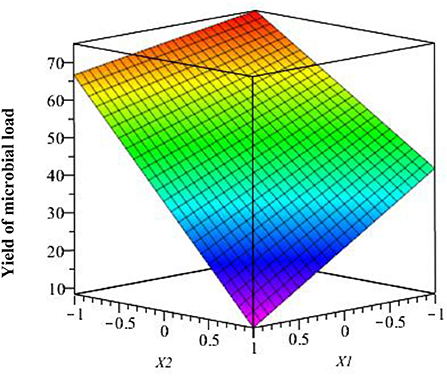
Figure 7. Effect of studied factors (X1 and X2) and their interaction on the response surface microbial load.
The effect of studied factors and their interaction on the pigmentation stabilization of strawberries
Natural variables (acetic acid and chitosan) coding into synthetic variables (X1 and X2) allowed us to develop an experimental design type 22 (4 experiments) illustrated in the Materials and methods section. Variance analysis of experimental design results is shown in Table 6. Table 6 validates the mathematical model and Table 7 validates the coefficients of the model.
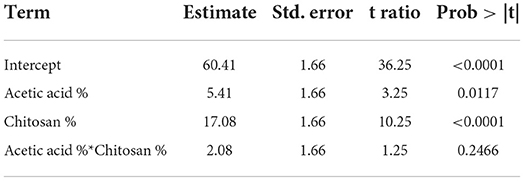
Table 7. Estimation of mathematical model parameters of the experimental design related to pigmentation stability of fruits.
Obtained F (39.06) value is higher than the critical one F′ (4.07) illustrated in the Fisher Snedecor table, which confirms mathematical model validity as shown in Table 7.
Response function mathematical model (Equation 2) related to strawberries pigmentation stabilization obtained is as follows:
• a0 = 60.41 coefficient model
• a1 = 5.41 coefficient related to acetic acid activity
• a2 = 17.08 coefficient related to chitosan activity
• a1, 2 = 2.08 coefficient representing the interaction effect of the two factors
Student test performed on the coefficients (Table 7) shows that they have a significant effect and consequently we retained all of them. Coefficients analysis sign shows that studied factors and their interactions have a positive effect on the pigmentation stabilization of strawberries. Chitosan protected anthocyanin pigments from degradation during storage due to its antioxidant properties. Acetic acid contributed as a solvent in stabilizing the pigmentation of treated strawberries. Effects of studied factors (X1 and X2) and their interaction (XX2) are shown in Figure 8.
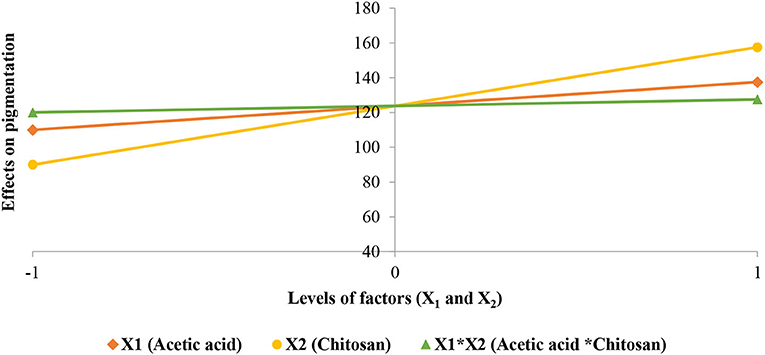
Figure 8. Effects of studied factors (X1 and X2) and their interaction on the pigmentation stabilization of strawberries.
The interaction of studied factors is shown in Figure 9 where we observe the response surface according to coded variables (acetic acid and chitosan), which delimits the experimental domain. This figure shows that pigmentation stabilization is maximal when acetic acid and chitosan are at the highest level (1.5 and 3%, respectively).
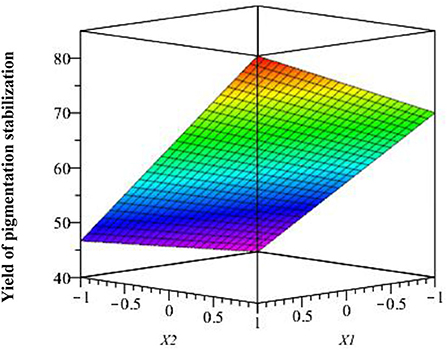
Figure 9. Effect of studied factors (X1 and X2) and their interaction on response surface pigmentation stabilization.
The effect of studied factors and their interaction on the firmness improvement of strawberries
Natural variables (acetic acid and chitosan) coding into synthetic variables (X1 and X2) allowed us to develop an experimental design type 22 (4 experiments) illustrated in the Materials and methods section. Variance analysis of experimental design results is shown in Table 8. Table 8 validates the mathematical model and Table 9 validates the coefficients of the model.
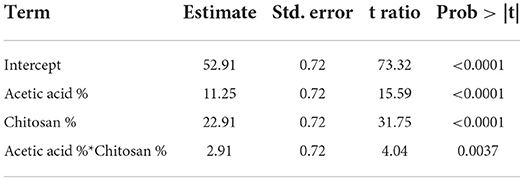
Table 9. Estimation of mathematical model parameters of the experimental design related to the firmness of the fruits.
Obtained F (422.55) value is higher than the critical one F′ (4.07) illustrated in the Fisher Snedecor table, which confirms mathematical model validity as shown in Table 9.
Response function mathematical model (Equation 3) related to strawberries firmness improvement obtained is as follows:
• a0 = 52.91 coefficient model
• a1 = 11.25 coefficient related to acetic acid activity
• a2 = 22.91 coefficient related to chitosan activity
• a1, 2= 2.91 coefficient representing the interaction effect of the two factors
Student test performed on the coefficients (Table 9) shows that they have a significant effect and consequently we retained all of them. Coefficient analysis sign shows that the studied factors and their interactions have a positive effect on the firmness improvement of strawberries. Chitosan preserved the cellular structures of fruits as a post-harvest treatment due to its functional properties. This polymer regulates parameters responsible for the ripening of fruits during the storage period. Acetic acid contributes as a chitosan vector in the preservation of cellular structures of strawberries, by inhibiting oxidative enzymes such as polyphenol oxidases. Factors effects (X1 and X2) and their interaction (XX2) are shown in Figure 10.
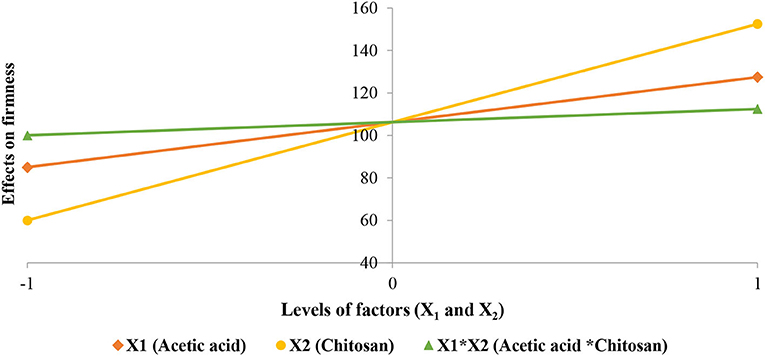
Figure 10. Effects of studied factors (X1 and X2) and their interaction on the firmness improvement of strawberries.
The interaction of studied factors is shown in Figure 11 where we observe the response surface according to the coded variables (acetic acid and chitosan), which delimits the experimental domain. This figure shows that firmness improvement is maximal when acetic acid and chitosan are at the highest level (1.5 and 3%, respectively).
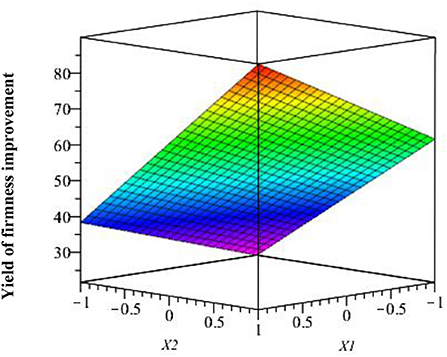
Figure 11. Effect of studied factors (X1 and X2) and their interaction on response surface firmness improvement.
According to obtained results, we find that edible coating made from chitosan extracted with organic acid (citric acid) significantly reduced microbial load of deterioration in strawberries. Treated strawberries retained their initial pigmentation for a longer duration when compared to untreated strawberries. Maturation of treated strawberries is thus delayed by a few days when compared to batch control and therefore treatment carried out has maintained cellular integrity in treated strawberries during the storage period. Acetic acid contributed as a solvent in stabilizing pigmentation and preserving cellular structures in treated strawberries. Soaking strawberries in an elaborated preservative solution extends the storage life of fruits and is considered to be very sensitive to post-harvest losses.
In this study, we used the strain Aspergillus niger as a model to develop a biological, less expensive, and effective anti-fungal treatment. The treatment elaborated by the biological chitosan and the acetic acid limits the proliferation of the molds which produce hydrolytic enzymes which degrade the cellular structures (firmness) and the pigments (anthocyanin). The stabilization of the cellular strucures limits or reduces cellular self-destruction. Strawberries attacked by molds ripen and spoil rapidly as fungal hydrolases degrade the polymers in strawberries into monomers that are assimilated by the bacteria that take hold afterward. The elaborate treatment ensures normal or slightly delayed ripening because the tissue structures are more stable. The prolongation of the conservation of strawberries lasts several days (7–8 days), a justified time sufficient to manage well the marketing of these very fragile fruits. This invention will give great competitiveness in the national and international market. The management of the sales of the strawberries treated by this process will be easier following prolonged conservation which allows the producers to manage time and space at the level of competitiveness.
The chitosan coating can be used to maintain the quality of strawberries by reducing growth and consequently enhancing the shelf life during cold storage compared to the uncoated treatment (Jiang et al., 2020). In addition, the chitosan (61 kDa) coating was more effective in delaying the pH increase and could better delay the reduction of strawberry flavonoids during storage. A study done by Abdipour et al. (2020) reported that the application of chitosan (1%) edible coating and UV irradiation maintained the post-harvest quality and reduced the weight loss during the storage of treated sweet cherry fruit. The chitosan coating on the strawberries' surface acted as a semi-permeable barrier to gases and water, thus reducing respiration and water loss and neutralizing dehydration and shrinkage of the fruit (Jongsri et al., 2016; Cazón and Vázquez, 2019). Chitosan coating has good mechanical strength on the surface of strawberries and could slow down texture degradation (Jiang et al., 2020). In addition, Petriccione et al. (2015) reported that chitosan treatment significantly reduced water loss and delayed the qualitative changes in the color of post-harvest strawberries. Furthermore, changes in the total polyphenol, anthocyanin, and flavonoid contents and the antioxidant capacity of chitosan-coated strawberries were delayed. In vivo bioassays showed that strawberries immersed in chitosan (7.5, 10, and 15 mg mL−1) displayed significant inhibition of mycelial growth and spore germination of post-harvest fungal strawberry pathogens (Arceo-Martínez et al., 2019).
Strawberries are highly perishable fruits that degrade quickly post-harvest, which explains their short shelf life. Fungal deterioration is one of the main causes of post-harvest losses of these fruits considered to be fragile. Storing strawberries at low temperatures is the most commonly applied method to improve their shelf life (Saleem et al., 2021). However, this technique without the use of other combined durable treatments is not considered appropriate due to the highly perishable nature of strawberries once released to the market. Other post-harvest techniques have been reported to extend the shelf life of strawberries, namely, edible coatings (Qamar et al., 2018; Quintana et al., 2021). These improve the visual appearance and maintain the post-harvest quality of coated fruits and vegetables. Chitosan coating has been applied post-harvest to many fruits and vegetables such as strawberries (Ban et al., 2018; Trinetta et al., 2020).
In various studies, chitosan has been proven to possess broad-spectrum antimicrobial activity, which can inhibit a huge variety of fungi and bacteria growth. This biopolymer is a potential antimicrobial substance due to its good biocompatibility, biodegradability, non-toxicity, and availability. The electrostatic interaction between chitosan and amine groups on fungi cell surface is the key that gives it ability to bind, alter or destroy the microbial cell surface (Qin et al., 2020). This explains the anti-fungal activity of chitosan against Aspergillus niger in strawberries in our study. Chitosan exhibits key anti-fungal properties that inhibited the growth of the mycelial fungus recognized as a food pathogen and spoilage microorganism. Aspergillus genus strains often contaminate products in the food industry. This currently encourages manufacturers to overcome this problem by using natural ingredients that meet food safety standards. Aspergillus niger is by far the most common Aspergillus species responsible for the post-harvest decay of fresh fruits, namely, apples, pears, peaches, citrus, grapes, figs, strawberries, mangoes, and melons (Pitt and Hocking, 2009). Thus, chitosan is a marine biological polysaccharide that has attracted much interest for antimicrobial applications (Chuan et al., 2019; Shariatinia, 2019).
Chitosan is considered a biopolymer with excellent antioxidant properties due to the presence of the amine group and two hydroxyl groups that give the polymer the ability to scavenge free radicals (Anraku et al., 2018). The use of antioxidants as food additives protects foodstuffs against oxidation, which generally affects food quality. Food attractive color suggests freshness and quality to consumers and influences purchasing decisions. For this reason, food coloring is an important parameter to consider in the food industry. Anthocyanins are natural pigments sensitive to degradation by exogenous and endogenous factors (Ge et al., 2018). Coatings and films are alternative methods to extend and protect these pigments from degradation, due to their bioactive properties, so chitosan has been used in many of these applications (Harkin et al., 2019). In this study, chitosan treatment ensured anthocyanin pigments stability in strawberries during the storage period. Acetic acid contributed as a solvent and reagent against oxidation enzymes (polyphenol oxidase) in stabilizing the pigmentation of treated strawberries.
As an active food packaging, chitosan can be applied to food as an edible thin film or an edible coating. The coating is a thin layer formed as a covering on a food product, which is applied in liquid form, by dipping the product in a chitosan solution (or spraying the product with chitosan solution) (Muxika et al., 2017). Chitosan has a wide spectrum of biological actions such as antioxidant activity, antimicrobial function, anti-fungal properties, film-forming properties, and food additive properties, which could be used in the food industry to improve safety, quality, and food shelf life (Kulawik et al., 2019). In this study, a coating made with chitosan improved post-harvest stability of strawberries for a long time by keeping their initial pigmentation, reducing the microbial load of deterioration, and preserving cellular structures when compared to control strawberries, and as a result, the treatment realized extended shelf life of strawberries.
Applying chitosan film or coating can improve perishable fruits and shelf life of vegetables as a post-harvest treatment. Overall, chitosan regulates gas exchange (CO2 and O2), post-harvest fruit respiration rate, and ethylene production rate during the storage period (Cazón and Vázquez, 2019). Furthermore, studies show that applying chitosan has a beneficial effect on maintaining firmness, titratable acidity, moisture content, and color retention throughout storage in treated vegetables and fruits (Leceta et al., 2015). In our study, acetic acid contributed as a chitosan vector in preserving the cellular structures of strawberries.
The results of this work can help the decision-makers (legislation and law in force) to elaborate organic quality standards according to the official bulletin which forms a schedule of conditions to be respected and followed by the producers. This contribution is double-edged, elimination of toxic acids for public health and protection for the environment. Thus, this work is a milestone that contributes to sustainable development.
Today's consumers demand less use of chemicals to extend the shelf life of food products. For this reason, there has been a growing interest in recent times to develop materials with film-forming ability and antimicrobial and antioxidant properties, which help improve food safety and shelf life. Due to its functional properties, chitosan is considered a natural alternative to synthetic antimicrobials and antioxidants. This biodegradable polymer is gaining more attention in the development of new active food packaging materials.
Conclusion
Strawberries are very perishable fruits that degrade quickly after harvest, hence the interest in developing bioactive food packaging capable of extending their shelf life. Chitosan extracted by an organic acid (citric acid) during demineralization showed strong anti-fungal activity on the mycelial growth of Aspergillus niger known as a food pathogen and post-harvest spoilage microorganism. This biopolymer can be used as an edible coating on certain food products so that the intensity of Aspergillus niger contamination will be reduced or eliminated. The coating of strawberries by soaking in chitosan solubilized in acetic acid extended the shelf life (7–8 days) of these fruits which are considered to be very sensitive to post-harvest losses, by reducing deterioration microbial load, stabilizing pigmentation, and preserving cellular structures of treated strawberries. Today's consumer is influenced by the organic trend. This is currently prompting researchers and manufacturers to overcome this problem by using natural ingredients such as biodegradable polymers, proteins, and essential oils in active packaging that respects food safety standards.
Data availability statement
The original contributions presented in the study are included in the article/supplementary material, further inquiries can be directed to the corresponding author.
Author contributions
AE-a, LE, and FE have made a substantial contribution to the work and approved it for publication. All authors contributed to the article and approved the submitted version.
Funding
This work was supported by the PRIMA project (Enhancing Mediterranean Fresh Produce Shelf-Life using Sustainable Preservative Technologies and Communicating Knowledge on Dynamic Shelf-Life using Food Cloud Services and Predictive Modeling BIOFRESHCLOUD).
Conflict of interest
The authors declare that the research was conducted in the absence of any commercial or financial relationships that could be construed as a potential conflict of interest.
Publisher's note
All claims expressed in this article are solely those of the authors and do not necessarily represent those of their affiliated organizations, or those of the publisher, the editors and the reviewers. Any product that may be evaluated in this article, or claim that may be made by its manufacturer, is not guaranteed or endorsed by the publisher.
References
Abdipour, M., Sadat Malekhossini, P., Hosseinifarahi, M., and Radi, M. (2020). Integration of UV irradiation and chitosan coating: a powerful treatment for maintaining the postharvest quality of sweet cherry fruit. Sci. Hortic. 264, 109197. doi: 10.1016/j.scienta.2020.109197
Anese, R., de, O., Brackmann, A., Thewes, F. R., Schultz, E. E., Ludwig, V., et al. (2020). Impact of dynamic controlled atmosphere storage and 1-methylcyclopropene treatment on quality and volatile organic compounds profile of ‘Galaxy' apple. Food Packag. Shelf Life 23, 100443. doi: 10.1016/j.fpsl.2019.100443
Anraku, M., Gebicki, J. M., Iohara, D., Tomida, H., Uekama, K., Maruyama, T., et al. (2018). Antioxidant activities of chitosans and its derivatives in in vitro and in vivo studies. Carbohydr. Polym. 199, 141–149. doi: 10.1016/j.carbpol.2018.07.016
Arceo-Martínez, M. T., Jiménez-Mejía, R., Salgado-Garciglia, R., Santoyo, G., López-Meza, J. E., and Loeza-Lara, P. D. (2019). In vitro and in vivo anti-fungal effect of chitosan on post-harvest strawberry pathogens. Agrociencia 53, 1297–1311.
Ban, Z., Yan, J., Wang, Y., Zhang, J., Yuan, Q., and Li, L. (2018). Effects of postharvest application of chitosan-based layer-by-layer assemblies on regulation of ribosomal and defense proteins in strawberry fruit (Fragaria × ananassa). Sci. Hortic. 240, 293–302. doi: 10.1016/j.scienta.2018.06.035
Betchem, G., Johnson, N. A. N., and Wang, Y. (2019). The application of chitosan in the control of post-harvest diseases: a review. J. Plant Dis. Protect. 126, 495–507. doi: 10.1007/s41348-019-00248-2
Cazón, P., and Vázquez, M. (2019). “Applications of chitosan as food packaging materials,” in Sustainable Agriculture Reviews 36: Chitin and Chitosan: Applications in Food, Agriculture, Pharmacy, Medicine and Wastewater Treatment, Vol. 36, eds G. Crini and E. Lichtfouse (Cham: Springer International Publishing), 81–123. doi: 10.1007/978-3-030-16581-9_3
Chuan, D., Jin, T., Fan, R., Zhou, L., and Guo, G. (2019). Chitosan for gene delivery: methods for improvement and applications. Adv. Colloid Interface Sci. 268, 25–38. doi: 10.1016/j.cis.2019.03.007
De Queiroz Antonino, R. S. C. M., Lia Fook, B. R. P., De Oliveira Lima, V. A., De Farias Rached, R. Í., Lima, E. P. N., Da Silva Lima, R. J., et al. (2017). Preparation and characterization of chitosan obtained from Shells of Shrimp (Litopenaeus vannamei Boone). Mar. Drugs 15, 141. doi: 10.3390/md15050141
Dewi, R., and Muhammad Nur, R. (2018). Antifungal activity of chitosan on Aspergillus spp. Int. J. Bioeng. Biotechnol. 2, 24–30.
Dimzon, I. K. D., and Knepper, T. P. (2015). Degree of deacetylation of chitosan by infrared spectroscopy and partial least squares. Int. J. Biol. Macromol. 72, 939–945. doi: 10.1016/j.ijbiomac.2014.09.050
Duarte, M. L., Ferreira, M. C., Marvão, M. R., and Rocha, J. (2002). An optimised method to determine the degree of acetylation of chitin and chitosan by FTIR spectroscopy. Int. J. Biol. Macromol. 31, 1–8. doi: 10.1016/S0141-8130(02)00039-9
Ge, J., Yue, P., Chi, J., Liang, J., and Gao, X. (2018). Formation and stability of anthocyanins-loaded nanocomplexes prepared with chitosan hydrochloride and carboxymethyl chitosan. Food Hydrocoll. 74, 23–31. doi: 10.1016/j.foodhyd.2017.07.029
Giusti, M. M., and Wrolstad, R. E. (2001). Characterization and measurement of anthocyanins by UV-visible spectroscopy. Curr. Protoc. Food Analytical Chem. 00, F1.2.1–F1.2.13. doi: 10.1002/0471142913.faf0102s00
Harkin, C., Mehlmer, N., Woortman, D. V., Brück, T. B., and Brück, W. M. (2019). “Nutritional and additive uses of chitin and chitosan in the food industry,” in Sustainable Agriculture Reviews 36: Chitin and Chitosan: Applications in Food, Agriculture, Pharmacy, Medicine and Wastewater Treatment, eds G. Crini and E. Lichtfouse (Cham: Springer International Publishing), 1–43. doi: 10.1007/978-3-030-16581-9_1
Jiang, Y., Yu, L., Hu, Y., Zhu, Z., Zhuang, C., Zhao, Y., et al. (2020). The preservation performance of chitosan coating with different molecular weight on strawberry using electrostatic spraying technique. Int. J. Biol. Macromol. 151, 278–285. doi: 10.1016/j.ijbiomac.2020.02.169
Jongsri, P., Wangsomboondee, T., Rojsitthisak, P., and Seraypheap, K. (2016). Effect of molecular weights of chitosan coating on postharvest quality and physicochemical characteristics of mango fruit. LWT 73, 28–36. doi: 10.1016/j.lwt.2016.05.038
Kandile, N. G., Zaky, H. T., Mohamed, M. I., Nasr, A. S., and Ali, Y. G. (2018). Extraction and characterization of chitosan from shrimp shells. Open J. Organic Polymer Mater. 8, 33–42. doi: 10.4236/ojopm.2018.83003
Kaur, S., and Dhillon, G. S. (2015). Recent trends in biological extraction of chitin from marine shell wastes: a review. Crit. Rev. Biotechnol. 35, 44–61. doi: 10.3109/07388551.2013.798256
Khan, T. A., Peh, K. K., and Ch'ng, H. S. (2002). Reporting degree of deacetylation values of chitosan: the influence of analytical methods. J. Pharm. Pharmaceut. Sci. 5, 205–212.
Kulawik, P., Jamróz, E., and Özogul, F. (2019). “Chitosan for seafood processing and preservation,” in Sustainable Agriculture Reviews 36: Chitin and Chitosan: Applications in Food, Agriculture, Pharmacy, Medicine and Wastewater Treatment, eds G. Crini and E. Lichtfouse (Cham: Springer International Publishing). doi: 10.1007/978-3-030-16581-9_2
Kumari, S., Kumar Annamareddy, S. H., Abanti, S., and Kumar Rath, P. (2017). Physicochemical properties and characterization of chitosan synthesized from fish scales, crab and shrimp shells. Int. J. Biol. Macromol. 104, 1697–1705. doi: 10.1016/j.ijbiomac.2017.04.119
Kumirska, J., Czerwicka, M., Kaczyński, Z., Bychowska, A., Brzozowski, K., Thöming, J., et al. (2010). Application of spectroscopic methods for structural analysis of chitin and chitosan. Mar. Drugs 8, 1567–1636. doi: 10.3390/md8051567
Kuyyogsuy, A. (2020). Preparation and characterization of chitosan obtained from pacific white shrimp shells and its in vitro antifungal activity. Asian J. Chem. 32, 2515–2519. doi: 10.14233/ajchem.2020.22779
Leceta, I., Peñalba, M., Arana, P., Guerrero, P., and de la Caba, K. (2015). Ageing of chitosan films: effect of storage time on structure and optical, barrier and mechanical properties. Eur. Polym. J. 66, 170–179. doi: 10.1016/j.eurpolymj.2015.02.015
Liang, J., Yan, H., Puligundla, P., Gao, X., Zhou, Y., and Wan, X. (2017). Applications of chitosan nanoparticles to enhance absorption and bioavailability of tea polyphenols: a review. Food Hydrocoll. 69, 286–292. doi: 10.1016/j.foodhyd.2017.01.041
Lurol, S., Vernin, X., Bébin, A., Husson, P.h., Baros, C., Brachet, M.-L., et al. (2019). D2BIOFRUITS - développement de méthodologies d'évaluation des pertes post-récolte et étude de l'efficacité de procédés compatibles avec l'agriculture biologique pour désinfecter et désinsectiser les fruits. Innov. Agronomiques 71, 225–245. doi: 10.15454/R0SZIM
Meng, D., Garba, B., Ren, Y., Yao, M., Xia, X., Li, M., et al. (2020). Antifungal activity of chitosan against Aspergillus ochraceus and its possible mechanisms of action. Int. J. Biol. Macromol. 158, 1063–1070. doi: 10.1016/j.ijbiomac.2020.04.213
Muxika, A., Etxabide, A., Uranga, J., Guerrero, P., and de la Caba, K. (2017). Chitosan as a bioactive polymer: processing, properties and applications. Int. J. Biol. Macromol. 105, 1358–1368. doi: 10.1016/j.ijbiomac.2017.07.087
Petriccione, M., Mastrobuoni, F., Pasquariello, M. S., Zampella, L., Nobis, E., Capriolo, G., et al. (2015). Effect of chitosan coating on the postharvest quality and antioxidant enzyme system response of strawberry fruit during cold storage. Foods 4, 501–523. doi: 10.3390/foods4040501
Qamar, J., Ejaz, S., Anjum, M. A., Nawaz, A., Hussain, S., Ali, S., et al. (2018). Effect of aloe vera gel, chitosan and sodium alginate based edible coatings on postharvest quality of refrigerated strawberry fruits of cv. Chandler. J. Horticult. Sci. Technol. 1, 8–16. doi: 10.46653/jhst180101008
Qin, Y., Li, P., and Guo, Z. (2020). Cationic chitosan derivatives as potential antifungals: a review of structural optimization and applications. Carbohydr. Polym. 236, 116002. doi: 10.1016/j.carbpol.2020.116002
Quintana, S. E., Llalla, O., García-Risco, M. R., and Fornari, T. (2021). Comparison between essential oils and supercritical extracts into chitosan-based edible coatings on strawberry quality during cold storage. J. Supercrit. Fluids 171, 105198. doi: 10.1016/j.supflu.2021.105198
Saleem, M. S., Anjum, M. A., Naz, S., Ali, S., Hussain, S., Azam, M., et al. (2021). Incorporation of ascorbic acid in chitosan-based edible coating improves postharvest quality and storability of strawberry fruits. Int. J. Biol. Macromol. 189, 160–169. doi: 10.1016/j.ijbiomac.2021.08.051
Shamshina, J. L., Barber, P. S., Gurau, G., Griggs, C. S., and Rogers, R. D. (2016). Pulping of crustacean waste using ionic liquids: to extract or not to extract. ACS Sustain. Chem. Eng. 4, 6072–6081. doi: 10.1021/acssuschemeng.6b01434
Shariatinia, Z. (2019). Pharmaceutical applications of chitosan. Adv. Colloid Interface Sci. 263, 131–194. doi: 10.1016/j.cis.2018.11.008
Shigemasa, Y., Matsuura, H., Sashiwa, H., and Saimoto, H. (1996). Evaluation of different absorbance ratios from infrared spectroscopy for analyzing the degree of deacetylation in chitin. Int. J. Biol. Macromol. 18, 237–242. doi: 10.1016/0141-8130(95)01079-3
Singh, D., and Sharma, R. R. (2018). “Postharvest diseases of fruits and vegetables and their management,” in Postharvest Disinfection of Fruits and Vegetables (Cambridge: Academic Press), 1–52.
Srinivasan, H., Kanayairam, V., and Ravichandran, R. (2018). Chitin and chitosan preparation from shrimp shells Penaeus monodon and its human ovarian cancer cell line, PA-1. Int. J. Biol. Macromol. 107, 662–667. doi: 10.1016/j.ijbiomac.2017.09.035
Trinetta, V., McDaniel, A. G., Batziakas, K., Yucel, U., Nwadike, L., et al. (2020). Antifungal packaging film to maintain quality and control postharvest diseases in strawberries. Antibiotics 9, 618. doi: 10.3390/antibiotics9090618
Verlee, A., Mincke, S., and Stevens, C. V. (2017). Recent developments in antibacterial and antifungal chitosan and its derivatives. Carbohydr. Polym. 164, 268–283. doi: 10.1016/j.carbpol.2017.02.001
Keywords: active food packaging, antifungal activity, Aspergillus niger, chitosan, citric acid, post-harvest treatment, strawberries
Citation: El-araby A, El Ghadraoui L and Errachidi F (2022) Usage of biological chitosan against the contamination of post-harvest treatment of strawberries by Aspergillus niger. Front. Sustain. Food Syst. 6:881434. doi: 10.3389/fsufs.2022.881434
Received: 22 February 2022; Accepted: 08 July 2022;
Published: 08 August 2022.
Edited by:
I. Made Joni, Universitas Padjadjaran, IndonesiaReviewed by:
Emma Rochima, Universitas Padjadjaran, IndonesiaFerry Faizal, Universitas Padjadjaran, Indonesia
Copyright © 2022 El-araby, El Ghadraoui and Errachidi. This is an open-access article distributed under the terms of the Creative Commons Attribution License (CC BY). The use, distribution or reproduction in other forums is permitted, provided the original author(s) and the copyright owner(s) are credited and that the original publication in this journal is cited, in accordance with accepted academic practice. No use, distribution or reproduction is permitted which does not comply with these terms.
*Correspondence: Abir El-araby, YWJpci5lbGFyYWJ5QHVzbWJhLmFjLm1h
†ORCID: Abir El-araby orcid.org/0000-0001-5500-0153
Faouzi Errachidi orcid.org/0000-0001-6596-4108