- 1Department of Geosciences and Natural Resource Management, University of Copenhagen, Frederiksberg, Denmark
- 2CIRAD (Centre de Coopération Internationale en Recherche Agronomique Pour de Développement), UMR DIADE, Montpellier, France
- 3DIADE, University of Montpellier, CIRAD, IRD, Montpellier, France
- 4Nicafrance Foundation, Finca La Cumplida, Matagalpa, Nicaragua
- 5Department of Food and Resource Economics, University of Copenhagen, Frederiksberg, Denmark
- 6IRD (Institut de Recherche pour le Développement), Montpellier, France
- 7CIRAD, University of Montpellier, UMR ABSYS, Montpellier, France
- 8UMR ABSYS, University of Montpellier, CIRAD, INRAE, SupAgro, Montpellier, France
- 9World Agroforestry, Vietnam Office, Hanoi, Vietnam
- 10UMR Eco&Sols, CIRAD, INRAE, SupAgro, IRD, Montpellier, France
- 11Plant Stress and Biodiversity Lab, Centro de Estudos Florestais (CEF), Instituto Superior Agronomia (ISA), Universidade de Lisboa (ULisboa), Oeiras, Portugal
- 12Unidade de Geobiociências, Geoengenharias e Geotecnologias (GeoBioTec), Faculdade de Ciências e Tecnologia (FCT), Universidade NOVA de Lisboa (UNL), Caparica, Portugal
- 13Agricultural Genetics Institute, Laboratoire Mixte International-Rice, Interactions & Coffee in Environment Phase II (LMI RICE2), Hanoi, Vietnam
Coffee is deemed to be a high-risk crop in light of upcoming climate changes. Agroforestry practices have been proposed as a nature-based strategy for coffee farmers to mitigate and adapt to future climates. However, with agroforestry systems comes shade, a highly contentious factor for coffee production in terms of potential yield reduction, as well as additional management needs and interactions between shade trees and pest and disease. In this review, we summarize recent research relating to the effects of shade on (i) farmers' use and perceptions, (ii) the coffee microenvironment, (iii) pest and disease incidence, (iv) carbon assimilation and phenology of coffee plants, (v) coffee quality attributes (evaluated by coffee bean size, biochemical compounds, and cup quality tests), (vi) breeding of new Arabica coffee F1 hybrids and Robusta clones for future agroforestry systems, and (vii) coffee production under climate change. Through this work, we begin to decipher whether shaded systems are a feasible strategy to improve the coffee crop sustainability in anticipation of challenging climate conditions. Further research is proposed for developing new coffee varieties adapted to agroforestry systems (exhibiting traits suitable for climate stressors), refining extension tools by selecting locally-adapted shade trees species and developing policy and economic incentives enabling the adoption of sustainable agroforestry practices.
Introduction
The coffee sector generates a global annual income exceeding $200 billion with a tendency for steady market growth of 2.2% every year (The Coffee Guide, 2021). There are more than 12.5 million coffee farms at the beginning of the coffee value chain, with ca. 60% belonging to smallholders, producing coffee on <5 ha (Enveritas, 2019). A large proportion of smallholder coffee farmers are living below the poverty line of $3.20 USD a day (The Coffee Guide, 2021).
As for several other economically important crops, ecological modeling approaches have predicted that coffee production will be threatened in the forthcoming years due to climate change (Chengappa et al., 2017; Asayehegn et al., 2018; Rahn et al., 2018; Grüter et al., 2022). The forecasted weather variation includes perturbations in intra- and inter-annual rainfall patterns, more frequent drought periods, elevated temperatures, as well as a shift in geographical coffee growing regions (Bunn et al., 2015a; de Sousa et al., 2019). In fact, the annual world coffee supply is already subjected to instability, mainly triggered by recurrent periods of drought accompanied by high temperature and irradiance as well as by episodes of frost (Caramori et al., 1996; Morais et al., 2006; Rigal et al., 2020a; Braga et al., 2021) affecting plant development, flowering, fruit set, and production (DaMatta and Ramalho, 2006). These impacts are believed to mainly affect Coffea arabica (Arabica) due to an inherent sensitivity to elevated temperatures, drought, pest, and diseases. Coffea canephora has previously been predicted to respond better to climate change than Arabica (Jayakumar et al., 2017; DaMatta et al., 2018). However, recent studies suggest that the alleged “heat tolerance” boasted by C. canephora (Robusta) plants could have been overestimated (Kath et al., 2020). Others point to elite Arabica genotypes which exhibit a heat and drought tolerance when simulated under climate change scenarios such as elevated air CO2 (eCO2) (Martins et al., 2014; Rodrigues et al., 2016; Ramalho et al., 2018; DaMatta et al., 2019; Avila et al., 2020a,b; Semedo et al., 2021). Still, impacts on both Arabica and Robusta plants are expected to occur, thus on-farm climate change mitigation and adaptation practices will be essential for a sustainable coffee management and productivity.
The cultivation of coffee under shaded agroforestry systems (AFS) (Figure 1) or intercropped with other trees/crops are among the agricultural practices able to improve microclimate conditions and mitigate the negative effects of climate change to coffee plants (Jaramillo et al., 2013; Partelli et al., 2014; Oliosi et al., 2016; Pham et al., 2019). Traditional coffee cultivation practices are often carried out by poorer farmers (Jha et al., 2014). These practices consist of growing coffee under native forest trees with a layered canopy. Generally, the higher the coffee cultivation intensity, the lower the layer complexity and plant diversity becomes. In its most intensified form (full-sun mono-crop systems), all plant diversity is lost. Since the late 90s, coffee farmers have moved toward intensive production systems, increasing inputs and decreasing the area of traditional shade-grown coffee. In new coffee growing regions (i.e., Southeast Asia), full-sun (FS) coffee cultivation systems dominate other forms (Jha et al., 2014), as is the case in Brazil, the world's largest coffee producer.
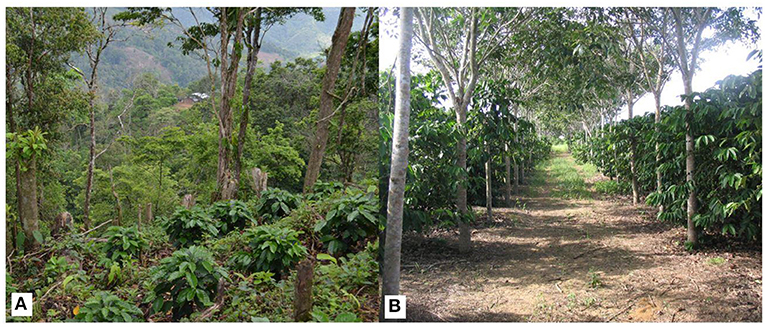
Figure 1. Different ways of cultivating coffee under shade. (A) Terraced Arabica coffee on an elevated site in amongst a mixture of trees species. (B) Experimental trial with C. canephora (Conilon cv.) in an agroforestry system intercropped with a single shade tree species. Photos © Benoit Bertrand and © Pierre Marraccini.
AFS enables several positive impacts such as buffering extreme temperatures and minimizing water loss by reducing soil evaporation and crop transpiration (DaMatta, 2004; Lin et al., 2008; Jha et al., 2014; Gomes et al., 2020; de Carvalho et al., 2021) as well as contributing to on-farm carbon sequestration (Ehrenbergerová et al., 2016). For this reason, AFS is today considered a nature-based solution for perennial crops which are sensitive to climate change. AFS has been shown to also reduce pests and diseases, e.g., by bolstering in-situ natural enemies of pests (Ratnadass et al., 2012). However, controversy surrounds the use of shade trees in coffee production due to their negative impacts on coffee growth and yield, as well as their potential to exacerbating biotic stressors (especially foliar disease such as coffee leaf rust—CLR) (Haggar et al., 2011; Avelino et al., 2020; Durand-Bessart et al., 2020; Gichuru et al., 2021).
The implications of AFS on coffee cup quality are also of the upmost importance, and the demand for specialty, high quality, and sustainably sourced coffee has skyrocketed over the last decade for both Arabica and Robusta coffee (van der Vossen et al., 2015). Industry and consumer demands call for a challenging feat—coffee cultivars/clones capable of coping with climate change combined with high yield and cup quality, produced under sustainable agricultural practices.
Coffee-AFS are living systems, highly variable to their given environments. A healthy coffee AFS should integrate localized, on-farm management practices including regulation of soil, water, tree, pest and disease to ensure ecological, economic, and social benefit of the system (Sebuliba et al., 2021). Given the documented variation within coffee-AFS, it is of the upmost importance for the coffee community to stay informed of the newest findings relating the interactions between shade and the coffee crop. For this reason, this literature review aims to bring together recent findings from studies (mainly performed in field trials) relating to the use of agroforestry compared to FS (open cultivated) systems in Arabica and Robusta coffee production. We evaluate recent work(s) in light of several important aspects associated with coffee management and future sustainability. This includes the effects of shade on (i) farmers' use and perceptions, (ii) the coffee microenvironment, (iii) pest and disease incidence, (iv) carbon assimilation and phenology of coffee plants, (v) coffee quality attributes, (vi) breeding of new Arabica coffee F1 hybrids for future AFS systems, and (vii) coffee production under climate change. The interactions between these key aspects can influence whether coffee farmers adopt the use of shade trees (Figure 2). Through this review, we begin to decipher whether shaded systems are a feasible, nature-based strategy for coffee farmers faced with climatic hazards through exploration of these key aspects.
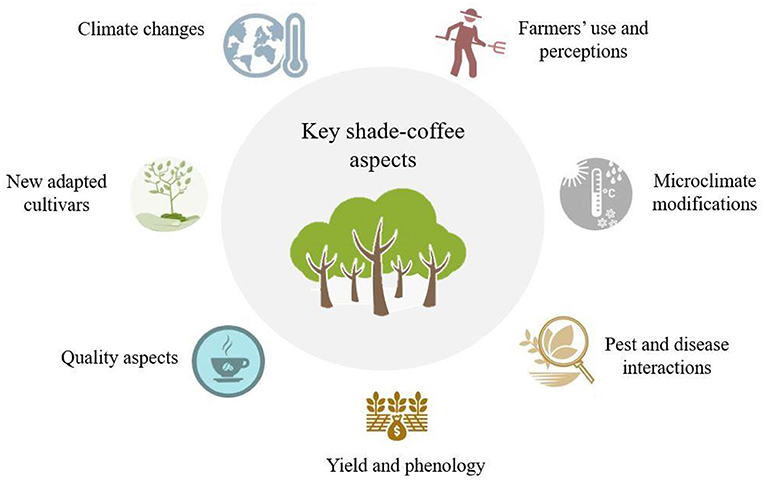
Figure 2. Key aspects relating to shaded-coffee systems. Any of the given factors may bear weight in the on-farm decision-making relating to the use and management of shaded-coffee systems.
Analysis of the Bibliography
In this work, we reviewed literature concerning coffee production under controlled field studies using shaded environments or within agroforestry-like systems. We based the review on database searches in Web of Science (WoS) and Scopus, as well as supplementary publications retrieved through other means (publication alerts, sent from colleagues etc.).
The core keywords used in the database searches were “agroforestry” AND “coffee” AND “climate.” This allowed for the inclusion of studies relating to both the C. arabica L. and C. canephora Pierre ex A. Froehner species. Additional key words were used in combination with the core keywords to cover each thematic area. These included (i) “farmer” AND “perception” OR “farmer” AND “use,” (ii) “microclimate” OR “aboveground,” (iii) “pest” OR “disease,” (iv) “phenology” OR “carbon” AND “assimilation,” (v) “quality” OR “biochemical” OR “organoleptic,” (vi) “hybrid,” and (vii) “climate change.” The date range was open for each search conducted and thus results spanned from 1976 to 2022 (including pre-prints). However, the majority of the studies found were dated between 2010 and 2022. Seven separate searches were conducted for each thematic area via WoS and Scopus on the 18th January 2022. In total 246 publications were reviewed across all thematic areas. The majority of the shade-related coffee research was found to be located in AFS-promoting countries such as in Latin America (e.g., Costa Rica, Colombia, Mexico, and Nicaragua). Given this, a geography bias was detected, with a large number of the work reviewed coming from these countries. This is likely connected to the long coffee cultivation history in Latin America and the rather recent commercial expansion into South East Asian countries (i.e., Vietnam) and Africa (i.e., Kenya, Tanzania, and Uganda). Despite this bias, many large coffee producing countries (e.g., Brazil, India, Kenya, Uganda, and Vietnam) are beginning to integrate AFS as a management approach. Therefore, some studies from these geographies were found (but to a lesser extent than the Latin American studies).
Coffee Farmers' Use and Perception of Shade Trees
There are no global data sets pertaining to the extent or share of coffee AFS compared to mono-crop or FS coffee to date. There is however, scientific consensus that coffee AFS is gradually diminishing in its share of total coffee area and is under threat from policies favoring intensive, highly productive systems (Jha et al., 2014; Albers et al., 2021; Harvey et al., 2021). According to the most recent attempt to quantify the share of AFS in coffee producing regions, Jha et al. (2014) found that <24% of the coffee area worldwide in 2010 was managed with traditional and diverse shade, 35% with sparse shade and 41% under FS. This study followed up on a 1996-study that reported massive conversions of shade coffee to intensively managed sun systems during the period from 1970 to 1990 in Latin America (Perfecto et al., 1996). This trend has continued until today, though at lower rates than previously recorded (Harvey et al., 2021). During the 1990's, coffee areas in Latin America and Africa have declined, while areas in Asia have increased, most notably in Vietnam, under intensive FS systems and thereby exacerbating the trend toward a lower share of shaded coffee (Jha et al., 2014). Key drivers to this trend were the national policies which targeted increasing yields to support greater exports. Breeding efforts have also traditionally focused on plant vigor and productivity (van der Vossen et al., 2015). However, the recent implementation of new agricultural policies, both public and private, and breeding efforts turned their attention to shaded coffee and intercropping systems, often as an adaptation strategy to climate change or as part of a certification process (Rice, 2018; Vanderhaegen et al., 2018; Bertrand et al., 2021). Recent efforts, notably public programs in Vietnam and China, have pushed for the conversion of large areas of full sun coffee into shaded systems (Rigal et al., 2018) and for the introduction of fruit trees intercropped with coffee trees (Thuy et al., 2019).
Coffee AFS vary in size and complexity, ranging from simple mixtures involving a few shade tree species planted at regular intervals within the rows of coffee trees to traditional or so-called “rustic systems,” where coffee is planted in plots of forests. In a rustic coffee AFS, numerous tree species can provide an almost complete shade cover through a multi-strata tree canopy (Somarriba et al., 2004). Shade tree abundance and diversity varies according to a number of farm and farmer characteristics, such as farmers' livelihood and diversification level, available space on the farm, and availability of workforce labor (Méndez et al., 2009; Rice, 2011; Lamond et al., 2016; Robbins et al., 2021).
The coffee crop under AFS is often less intensively managed than in FS systems, with fewer inputs in form of labor and expensive agrochemicals going into the production. Pruning of shade trees and leaf litter will reduce, to some extent, the need for chemical fertilizers (Bravo-Monroy et al., 2016). Additionally, the multi-strata agroforestry systems may reduce occurrences of certain pests and diseases (Soto-Pinto et al., 2002; Durand-Bessart et al., 2020) and thus the need for pesticide application. However, coffee AFS are not always the panacea for pest control, and these complex biotic networks were also reported to favor specific pests and pathogens depending on environmental factors (Allinne et al., 2016).
AFS use is common amongst small-scale coffee farmers, as labor is mainly family-based and used to manage all types of crops in the AFS of limited size. This is also associated with little access to agrochemical inputs (either due to market constraints and/or high costs), altogether resulting in lower management costs per hectare (Jezeer et al., 2017). On larger farms, labor may become the limiting factor for diverse shade systems, as found by Robbins et al. (2021) in India, where larger farms had higher abundance and diversity of shade trees than small farms due to better availability of hired labor. In a review of studies spanning 26 years across Latin America, Jezeer et al. (2017) reported that lower costs and supplementary income from shade tree products made shaded systems more profitable than non-shaded systems. Products from shade trees, such as fruits, firewood, timber and other materials, can make up a substantial part of the total income from coffee AFS (Rice, 2008; Souza et al., 2010; Thuy et al., 2019), and highly diverse coffee AFS increase the household's food and nutrition sources, especially in the shortage season (Jemal et al., 2021). Payment for ecosystem services can also generate additional source of revenues for AFS-coffee farmers (Cole, 2010; Thuy et al., 2021). Diversification of income from shade trees can be a safety net or gap-closing strategy especially important during times of low coffee prices (Gordon et al., 2007; Rice, 2011).
AFS must be locally tailored to answer farmers' constraints and needs, and to cope with local environmental conditions. The criteria established by each farmer to select shade trees are undoubtedly useful to other farmers, but one farm system can not necessarily be replicated to another site (Souza et al., 2010). For instance, the adequate degree of shade varies according to the elevation (Rahn et al., 2018). Coffee farmers in Kenya perceived dense shade as potentially problematic at high elevations, while they considered it beneficial at low elevations in regulating temperature, sun damages and pest incidences (Lamond et al., 2016). This may change in future scenarios with climate change and elevated atmospheric CO2, where 50% shade at high elevations will become beneficial as found in yield models by Rahn et al. (2018). In Uganda, coffee farmers selected perennial shade trees that were fast growing, with small leaves and wide crowns (Sebuliba et al., 2021). This tendency often results in the selection of exotic species over native trees (Graham et al., 2021), which no doubt alters local biodiversity and conservation aspects. Incompatible shade trees (having negative impacts on coffee) were often tolerated if they provided other benefits/services to the farming household (Graham et al., 2021). In their study in Brazil, Souza et al. (2010) found that farmers' first criterion for selecting tree species was compatibility with coffee, i.e., trees with deep roots and that would not bring sanitary problems to coffee trees, followed by biomass production, labor needed for tree management, and income diversification. In that study, shade itself was not a criterion for shade tree selection. Another study in southwest China found that farmers preferred trees with dense canopies and high economic returns despite their negative impact on coffee yield (Rigal et al., 2018). By contrast, a study in Indonesia found that coffee farmers selected tree species as a means of increasing coffee yield (Zaitunah and Ahmad, 2021). In Ethiopia, the decision to adopt AFS was found to be first determined by the provision of direct economic benefits and, to a lesser degree, by social factors such as gender, family size, educational level, and land tenure (Gebru et al., 2019). A study in Mexico found that farmers chose 40–80% shade cover to mitigate climate shocks, such as long heatwaves (Ruiz-García et al., 2021), and that the indigenous Huastec Mayan farming communities harbored a high number of edible plants in their coffee AFS (Heindorf et al., 2021). It is therefore essential to adapt AFS locally to answer farmers' needs in favor of the adoption and scaling up of shaded-coffee systems and must also fit with farmers' perceptions of shade trees. Nevertheless, providing information regarding shade tree benefits to farmers with limited experience with coffee AFS management is also important. Farmers near Mt. Elgon in Uganda, who had received training as part of a Trees of Food Security program, had a more positive view on the management costs and the benefits from shade trees compared to non-participating farmers (Buyinza et al., 2022).
Several studies have shown that farmers are aware of the trade-offs involved in introduction of shade tree species in coffee farms, and that they understand the impacts of agroforestry practices on coffee phenology and productivity (Cerdán et al., 2012; van der Wolf et al., 2016; Rigal et al., 2018; Dumont et al., 2019). Some of these studies even reveal in-depth knowledge of the complex causality between shade trees and agronomic services, such as soil conservation, nutrient cycling or pest, and disease management, as well as adverse competitive effects between plant species (Bagyaraj et al., 2015; Lamond et al., 2016; Liebig et al., 2016; van der Wolf et al., 2016; Nesper et al., 2017, 2018; Dumont et al., 2019). This extensive knowledge and its impact on farming practices is reflected in the farmers management of the percentage of shade throughout the year to regulate humidity, rainfall interception, light interception, and fungal diseases, such as CLR and American leaf spot (Cerdán et al., 2012). Farmers' willingness and ability to convert to and manage coffee AFS will depend on the economic performances of these systems and on the implementation of financial mechanisms to reward the adoption of more sustainable practices and the production of higher quality coffee (Borrella et al., 2015; Verburg et al., 2019). This transition will also require farmers' access to financial credit, as well as access to information and to adequate seedlings of both shade trees (Rigal et al., 2018), and coffee (van der Vossen et al., 2015). Furthermore, the adoption of shaded systems to coffee crops must be accompanied by the design of suitable AFS and their associated management practices. In traditional coffee growing areas, farmers' extensive local ecological knowledge can therefore be an asset in designing and scaling up locally tailored agroforestry practices (van der Wolf et al., 2016).
Shade and the Coffee Microenvironment
Shade trees can be beneficial in environments that are becoming increasingly less suitable for coffee cultivation (Ehrenbergerová et al., 2021). The presence of shade trees in coffee farming systems has generally been associated with favorable microclimate modifications such as lower air temperature fluctuations, increased air relative humidity, lower wind speed (see Table 1) and decreased frost damages (Figure 3). Research concerning ecosystem services by shade trees in coffee plantations have also noted an overall positive impact on soil fertility, total organic matter, and nutrient cycling, a reduced soil evaporation and soil erosion, as well as higher on-farm sequestration of carbon (Cannavo et al., 2011; Dubberstein et al., 2018; Guillemot et al., 2018; Padovan et al., 2018; De Giusti et al., 2019; Sarmiento-Soler et al., 2019; Jácome et al., 2020; Villarreyna et al., 2020; Zaro et al., 2020). An aspect relating to the belowground competition is weed control, with a significant reduction of weeds present under shaded coffee systems compared to full sun (Nestel and Altieri, 1992). Moreover, the weed species' commonly found in shaded systems (such as Commelinaceae spp.) tend to be less competitive with coffee plant for resources than those more prominently found in FS plantations such as Poaceae spp. and Compositae spp. (Staver et al., 2001).
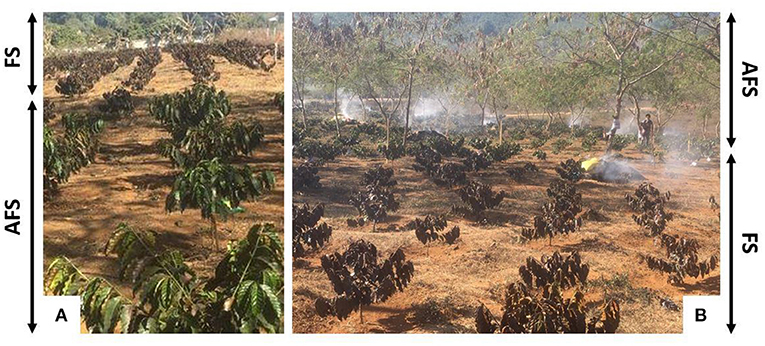
Figure 3. Frost protection of coffee plants by agroforestry practices. Young C. arabica plants (16 months old) in an experimental field of the Northern Mountainous Agriculture and Forestry Science (NOMAFSI) station of Mai Son (Son La province, Vietnam) under either agroforestry (AFS) with Leucaena leucocephala or full sun (FS) conditions and affected by frost (10th December 2019). AFS coffee plants were not damaged by frost (shown with green leaves). FS coffee plants damaged by frost are with brown and inclined leaves after a couple of hours and died within a day. (A) Photograph taken in the AFS trial showing the FS trial. (B) Photograph taken in the FS trial showing the AFS trial. Note: the smoke seen in photograph (B) was the result of controlled fires, which were lit the day after the first frost event. This was a short-term management practice intended to warm the coffee field microenvironment above 0 °C in subsequent nights. Photos © Philippe Vaast.
As a general rule, the selection and pruning of shade trees should favor the aboveground positive impacts while reducing the competition between coffee and shade trees for light, water and nutrients (Beer et al., 1998; Souza et al., 2010; van der Wolf et al., 2016). The selection of shade tree species with deeper root systems is especially important to ensure belowground complementarity rather than competition (Padovan et al., 2015; Rigal et al., 2020a). Here, we focus the review on the impact of shade trees on aboveground growing conditions, which will be directly impacted by climate change.
Temperatures
Changes in temperatures and intra- and inter-annual rainfall patterns will negatively impact the suitability of large areas traditionally suitable for coffee production (Bunn et al., 2015a; Semedo et al., 2018; de Sousa et al., 2019; Gomes et al., 2020). Although some areas might benefit from new climatic conditions (especially areas at high elevations which will see an increase in temperatures and a shift from sub-optimal to optimal conditions) (Ceballos-Sierra and Dall'Erba, 2021) overall coffee production is expected to decline due to global warming (Kath et al., 2020). In addition, climate change is expected to increase the frequency and severity of extreme temperature events, both for heatwaves or cold spells, which will further impact coffee production. Shade trees offer a mitigation strategy for these climatic hazards, with overall cooler daytime air temperatures, thus contributing to maintain suitable growing conditions at lower elevations (de Souza et al., 2012; Rahn et al., 2018; Gomes et al., 2020). A buffering impact of shade trees on air temperatures has been reported in numerous studies, with minimum night temperatures found to be 0.5–2°C higher than under FS, and maximum daytime temperatures 4–5°C lower compared to FS (Lin, 2007; Siles et al., 2010; Rigal et al., 2020a; Merle et al., 2022). This buffering impact offers the double asset of protecting coffee trees from climatic hazards such as frost (Figure 3) and lowering heat stress during peak temperatures in the dry season, therefore providing conditions more suitable for photosynthetic activity (van Kanten and Vaast, 2006).
Water-Use
Coffee was found to transpire more per unit leaf area in full sun than under shade, which indicates a higher level of environmental stress in non-shaded conditions, due to higher irradiance, wind speed, air temperature and vapor pressure deficit (VPD) in FS environment than in shaded conditions (van Kanten and Vaast, 2006; Lin, 2010; Coltri et al., 2019). Lower water use (due to reduced transpiration rates) could potentially become an important feature under future conditions of limited water availability namely due to more frequent and extended periods of drought (accompanied with greater high air temperatures) (DaMatta et al., 2018; Sarmiento-Soler et al., 2019; Byrareddy et al., 2021). However, the combined transpiration of shade trees and shaded coffee plants may contribute to a larger eco-system transpiration volume, with an overall reduction of water availability under shade compared to full sun (Sarmiento-Soler et al., 2019). This is especially relevant for coffee production areas where an extension of the dry season period is forecasted due to climate change (Cannavo et al., 2011). However, recent attempts to compare water loss between coffee-AFS and FS by a system of in-field sensors and pluviometers showed an overall higher water loss in unshaded environments (de Carvalho et al., 2021). The detrimental impact of competition for water by shade trees in AFS has been recently reported in cocoa systems (Abdulai et al., 2018). This study showed a direct link to cocoa tree mortality in the shaded systems, when facing extreme drought conditions, highlighting the importance of carefully selecting shade tree species capable of extracting water from deeper soil horizons compared to the accompanying crop plant/tree (Bayala and Prieto, 2020; Muñoz-Villers et al., 2020).
Pest and Disease Incidence Under Shade
The shaded-coffee environment within AFS hosts biological richness in terms of tree species, epiphytes, mammals, birds, reptiles, amphibians, and arthropods (Perfecto et al., 1996, 2005; Moguel and Toledo, 1999; Jezeer et al., 2019; Udawatta et al., 2021). Coffee-AFS provide ecological services, which in turn might benefit coffee production, for example by lowering dominance of pests through both direct and indirect competition (Kellermann et al., 2008; Perfecto et al., 2014). Moreover, the coffee plants themselves naturally attract a large range of natural enemies to pests and diseases, including lizards, ants, lady beetles, mites, predatory, and parasitoid wasps, and microorganisms such as entomopathogenic fungi (Perfecto et al., 2021; Venzon, 2021). Monoculture farming of coffee however, does not provide the adequate environment nor nutrition to maintain a high level of these natural enemies in the field.
The ecological interactions in coffee agroecosystems relating to prominent pests and diseases such as the coffee berry borer beetle (Hypothenemus hampei Ferrari), CLR (caused by the fungus Hemileia vastatrix Berk. & Broome), or the American leaf spot disease (caused by the fungus Mycena citricolor Berkeley & Curtis) are still being elucidated today (Avelino et al., 2020; Castillo et al., 2020; Cerda et al., 2020; Granados-Montero et al., 2020; Hajian-Forooshani et al., 2020; Merle et al., 2020).
Results reported on the incidence of pests and diseases vary according to site conditions (altitude etc.), farm management, shade tree species, density of shade, and as well as the presence of forest areas on and/or surrounding the farm (Karp et al., 2013). In fact, some studies showed a higher incidence of pest or disease infestation under shade vs. FS conditions (Soto-Pinto et al., 2002; Bosselmann et al., 2009; Avelino et al., 2012, 2020; Bukomeko et al., 2018; Durand-Bessart et al., 2020). By contrast, other reports showed pest and disease reduction in coffee under AFS due to the presence of more birds and ants, as well as microclimate modifications in the shade compared to the sun fields (Johnson et al., 2010).
Specific shade tree traits such as canopy openness and leaf area have been recently found to explain most microclimate conditions (Merle et al., 2022), and significantly relate to CLR incidence levels (Gagliardi et al., 2021). These findings may serve as a “missing link” to explain the AFS-CLR dynamics and shows the importance of shade tree selection in a coffee-AFS due to potential synergistic or antagonistic effects on these cropping systems. The Inga sp. is a common shade tree species in coffee-AFS and has been linked to an enhanced natural pest control for the coffee plant in proximity to its canopy due to its extra floral nectars (Rezende et al., 2014). Large and heavily shaded canopies of sap-exuding shade trees have been shown to have a dampening effect on the devastating Black Coffee Twig Borer (BCTB causal beetle: Xylosandrus compactus) (Bukomeko et al., 2018). However, this effect is lost under the shade tree Albizia chinensis (Wu, 2016; Bukomeko et al., 2018). A so-called “shelter effect” of rubber shade trees was recently revealed to allow for the persistence of Coffee Leaf Miner (CLM) on coffee leaves during the cold autumn and winter seasons (Righi et al., 2013). Although a higher number of leaves were mined in the shade, overall damage to the coffee plant by CLM was lower under the shade. These studies highlight the significant impacts of pests and diseases on coffee production and in turn on farmers' livelihood, and the importance of shade tree selection for their synergistic or antagonistic effects on key coffee pests or pathogens. Further research is needed to elucidate the interactions between pest and/or disease and coffee-shaded systems, especially along varied shade, temperature, and altitude gradients for better understanding and prediction of future outbreaks (Liebig et al., 2019).
Shade Effects on Carbon Assimilation and Phenology of Coffee Plants
Although the shade-modified microclimate may be overall favorable for the coffee plant, the associated reduction in solar irradiation in AFS is a cause of concern for many coffee-growers. Low light regime is the feature of shade-grown coffee most commonly associated with yield reductions, and a number of works corroborate this claim (Clemens and Zablah, 1993; Campanha et al., 2004; DaMatta, 2004). Due to the lack of a common “shade metric,” uncertainty lies in the levels of shade stated in the primary literature sources of the present review. Some researchers described shade in terms of a percentage of light interception at the coffee plant level (Bosselmann et al., 2009; Steiman et al., 2011; Partelli et al., 2014; Charbonnier et al., 2017). This indicator best characterizes homogeneous shade covers. Others report shade density in terms of the number of shade trees per hectare or distance from shade trees (Carvalho et al., 1961; Hernández Guerra, 1995; Baggio et al., 1997; Pilati, 2005; Siles et al., 2010; Virginio Filho et al., 2015; Araújo et al., 2016; Javier Lopez-Garcia et al., 2016), or in terms of shade tree leaf area index (Charbonnier et al., 2013; Rigal et al., 2020a), which might better describe AFS based on sparse shade trees or on fruit trees with low and thick canopy covers. A few studies were found to measure and/or simulate the level of shade cast by trees throughout the day (Charbonnier et al., 2013; Coltri et al., 2019). These approaches allowed for estimation of the amount of the radiation reaching coffee trees at different hours.
Natural shade trees provide an anisotropic delivery of light to their understory in terms of quantity and quality (e.g., assessed by the quantum ratio of red to far-red light; Vanderbilt and Grant, 1985; Chazdon et al., 1996; Lee et al., 1996). In tropical rainforests the average intensity of radiation on the forest floor ranges from 5 to 25 μmol photons m−2·s−1 (within the λ 400–700 nm range), which is equivalent to around 1–3% of sunlight delivered above the canopy (Chazdon et al., 1996; Lee et al., 1996). Spectral distributions of light within naturally shaded systems can also vary significantly compared to FS conditions, because leaves from the higher canopy strata will differentially absorb more red (R) and blue (B) light and reflect or transmit more far red (FR) light. This leads to altered light quality, with a low R:FR light conditions in the lower strata (where coffee will be growing), particularly in dense canopies. This may also have implications in plant resilience/response to other environmental biotic and abiotic stresses (Courbier and Pierik, 2019). The changed R:FR ratio may also trigger morphological and anatomical changes in the coffee crop. This is frequently observed by the development of shade leaves, which are thin but have larger area than leaves developed under FS. Shade trees within a coffee AFS will therefore provide a varying spectral quantity and quality over the course of the day/season due to sun orientation, over the course of their development and dependent on which shade-tree species are present (Vanderbilt and Grant, 1985). This temporal shading effect of natural shade trees may allow for light environments that reduce the over-excitation of the photosynthetic apparatus and the probability of photo-inhibition to occur at high irradiance periods in the day, particularly at noon, but also reduce the photosynthetic light amount needed for carbon assimilation. However, coffee is able to maintain net photosynthesis at levels similar to full sun at up to 55% light reduction, because photosynthetic light saturation are reached at irradiances quite below FS values in leaves acclimated to high irradiance exposure (Ramalho et al., 2000; Franck et al., 2007). Additionally, a recent study corroborates the idea of photosynthetic maintenance in shaded-coffee plants (Charbonnier et al., 2017). Despite a 60% reduction in irradiance below the canopy of the shade trees, coffee plants grown under shade increased their light-use efficiency by 50% and the overall aboveground net primary productivity (leaves, fruit, wood, etc.) was not statistically different to that of the FS-grown plants. This demonstrates how coffee plants can potentially compensate for the reduction in solar irradiation in a shaded environment by increasing their photosynthetic efficiency. Martins et al. (2013) supported this hypothesis by demonstrating a comparable level of net photosynthesis in coffee plants grown under 90% shade cover compared to FS, when calculated on a mass basis. This study also determined difference in the kinetics of photosynthesis, showing that the shade grown leaves exhibited faster photosynthetic induction compared with their sun counterparts, likely explained as an adaptive response to use the light energy from sun-flecks. Such increased photosynthetic performance observed in shade-grown coffee may allow the plant to maximize the potential for carbon assimilation in a low light environment, and is likely associated to the forest-understory evolutionary origin of C. arabica.
Nevertheless, there are cases in which the well-illuminated leaves from the upper part of the coffee canopy showed greater net carbon assimilation rate (A), associated with higher electron transport rate, as compared to self-shaded, lower-canopy coffee leaves in the same plant (Araujo et al., 2008). This study also concluded that there was no major difference in stomatal and mesophyll conductance between sun and shaded coffee leaves, which were similar regardless of leaf position. However, morphological (e.g., variations in specific leaf area and leaf inclination) or anatomical plasticity is likely of greater value in terms of acclimation to low-light environments. When considering leaf age, Campa et al. (2017) found that only mature coffee leaves were capable of acclimating to high-light conditions for C. arabica cv. Naryelis (grown under controlled conditions). The growing and juvenile leaves (under development) were found to have inefficient photo-protection mechanisms, scarce antioxidant protection, and a poor ability to export sucrose under increasing light conditions. These observations suggest that despite the fact that the C. arabica Naryelis cv. was selected for FS conditions, immature leaves of this cultivar are somehow sensitive to high light levels. These findings are in line with other reports showing a high acclimation plasticity of newly-matured coffee leaves after transition from deep shade to FS exposure, associated with the reinforcement of photosynthetic components, anti-oxidative and photo-protective mechanisms, as well with changes in the lipid profile of chloroplast membranes (Ramalho et al., 1997, 1998, 2000). Interestingly, these high irradiance stress responses, which potentially exacerbate oxidative stress conditions (as a secondary stress), constitute a common response in coffee leaves to other stresses such as heat (Rodrigues et al., 2016), cold (Ramalho et al., 2014), and drought (Dubberstein et al., 2020), thus confirming plasticity of some elite coffee genotypes to environmental constraints that should be explored in terms of breeding purposes.
Full-sun cultivation of coffee promotes a higher number of nodes and fruits per branch, leading to a greater competition between fruits, resulting in smaller beans, and biennial production due to heavy flowering followed by reduced flowering in the subsequent year (Cannell, 1974, 1976, 1985; DaMatta, 2004), although these problems can be mostly overcome with adequate fertilization and irrigation management under full sun cropping. In contrast, shaded-coffee production is synonymous with fewer nodes per branch, lower fruit loads and hence less competition between fruits, leading to larger beans, less alternate bearing pattern and less branch dieback (DaMatta, 2004). All these factors influence the annual yields and profitability of the coffee farm and are thus critical in the decision-making process regarding light/shade management. In particular, the flowering intensity has a direct influence on fruit loading in coffee plants (Franck et al., 2007). The Caturra cultivar of C. arabica was reported to produce a high variation in flower intensity across a spectrum of light, resulting in ca. 4,620, 3,052, 1,500, and 605 flowers per plant, under FS condition, 25, 50, and 75% light reduction, respectively. While shade has been reported to have a beneficial effect on bean size and density (Muschler, 2001, 2004; Morais et al., 2006; Vaast et al., 2006; Geromel et al., 2008; Bote and Struik, 2011; Somporn et al., 2012), the reduced flowering intensity under lowered irradiation in many cases leads to yield reduction (Vaast et al., 2006; Jaramillo-Botero et al., 2010). Therefore, coffee growers using shade-systems must balance the fine-line of negative effects (e.g., associated with flowering intensity) with the positive effects (e.g., lower need of water or fertilization inputs), to obtain a desired fruit load and bean quality. This is no easy feat and likely dependent on individual cultivar sensitivity to a low-light environment with respect to flowering, as well as to other environmental factors (i.e., whether the farm is operating under optimal/sub-optimal conditions of water availability and temperature, etc.) and/or the management the shade level through timely pruning of shade trees.
Shade Effects on Coffee Quality Attributes
There is little literature devoted to shade effects on cup quality or on the biochemical composition of coffee beans (Leroy et al., 2006). Here, we define coffee quality in the context of the bean size, biochemical composition, as well as organoleptic attributes mainly for C. arabica and for C. canephora (when available), with the main interactions between shade and coffee quality attributes shown in Table 2. As the farmers' choice of cultivar is a critical factor influencing quality, we also assess shade effects at the cultivar level (where feasible).
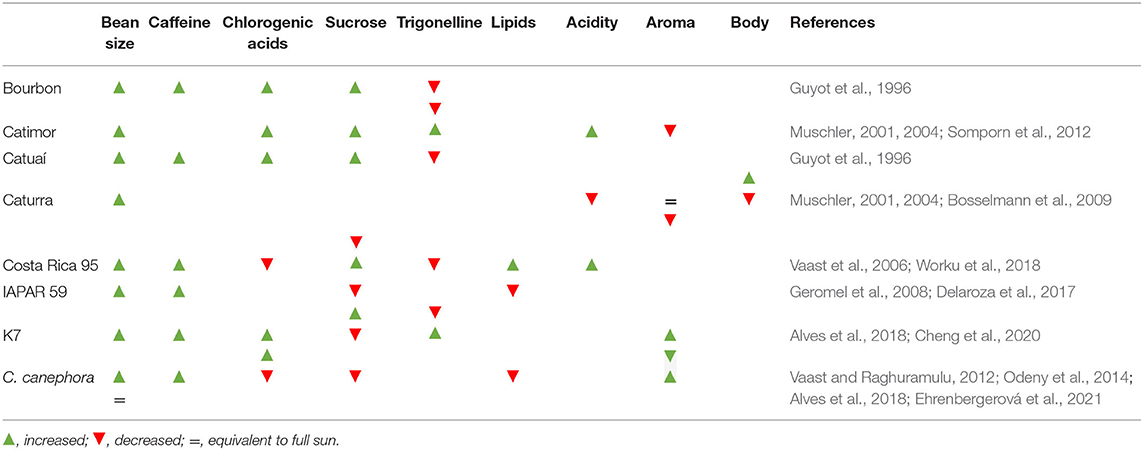
Table 2. The effects of shade on beans size, biochemical and organoleptic attributes associated with coffee quality of C. arabica cultivars and C. canephora species.
Shade Effects on Coffee Bean Size
Bean size is an important parameter defining coffee quality, with large beans being frequently associated with better cup quality and high prices in international markets (Vaast et al., 2006; Sanz-Uribe et al., 2017). For Arabica, high percentages of large coffee beans were reported under both natural and artificial shade (Table 2). The effects of shade on coffee bean size can be explained by the fewer branches produced under shade, with smaller number of nodes per branch, and fewer numbers of flowers per node. These shade impacts contribute to a reduced fruit load under shade (Cannell, 1976; Morais et al., 2006; Vaast et al., 2006; Jaramillo-Botero et al., 2010). Moreover, shade also lowers the tree stress (explaining lower biannual bearing pattern) (Fahl et al., 1994), and hence favors slow fruit ripening, better filling of beans which increases bean size, and ultimately cup quality (Morais et al., 2006; Vaast et al., 2006; Bote and Struik, 2011; da Silva Neto et al., 2018).
Compared to shade studies on C. arabica coffee, the impacts of shade on C. canephora are much scarcer. Vaast and Raghuramulu (2012) showed that the effects of shade on Robusta bean size were largely dependent on the shade trees selected and rainfall conditions. Under low rainfall conditions, Robusta intercropped with Artocarpus heterophyllus, Dalbergia latifolia, and Lagerstroemia microcarpa had a higher percentage of larger than normal bean size (coffee grading AA equivalent to >7 mm diameter) than those intercropped with Grevillea robusta. However, Robusta intercropped with A. heterophyllus or G. robusta provided lower AA bean percentage under high rainfall. Another study also reported that the AA bean percentage was strongly and positively influenced by the density of non-Grevillea shade trees but not by that of G. robusta (Boreux et al., 2016). In a more recent study performed across three Robusta plantations in Cambodia, Ehrenbergerová et al. (2021) showed that coffee bean size, as well as fruit ripening and yield, were not affected by shade trees.
Shade Effects on Coffee Bean Biochemical Composition
Although shade is widely documented to delay coffee bean maturity, knowledge of shade effects relating to coffee biochemical compounds is somewhat limited (Cheng et al., 2016). In green beans, coffee quality can be ascertained by quantifying some chemical compounds, namely sugars (e.g., sucrose), lipids, chlorogenic acids (CGA), and caffeine (Leroy et al., 2006). The light effects on sucrose are uncertain, since it was reported to increase both under FS (Vaast et al., 2006; Geromel et al., 2008; Delaroza et al., 2017; Worku et al., 2018), and shaded conditions (Guyot et al., 1996; Muschler, 2001, 2004; Somporn et al., 2012) (Table 2). A general negative relationship between fat and sucrose contents has been reported in both Arabica and C. canephora coffee species (Montagnon et al., 1998; Bertrand, 2002; Vaast et al., 2006). As sucrose is a precursor of polysaccharides and fat compounds (Bradbury and Halliday, 1990), Vaast et al. (2006) hypothesized that the high sucrose (as well CGAs and trigonelline) and low lipid contents (often observed under FS condition) pointed toward incomplete bean maturation, although a tendency to lower lipid content can result also from higher temperatures in mature beans (Ramalho et al., 2018).
The variation of bean biochemical contents observed under shaded vs. FS coffee beans is speculated to arise from differential enzymatic activities. In pericarp and perisperm tissues separated from C. arabica matured cherries, higher activities of sucrose synthase (EC 2.4.1.13) and sucrose-phosphate synthase (SPS: EC 2.4.1.14) were detected under shade as compared to full-sun (Geromel et al., 2006). These two enzymes also had high activity peaks in developing beans (endosperm) which could explain the sucrose reduction and the increase in reducing sugars observed under shade condition. By performing DPPH (1,1-Diphenyl-2-picrylhydrazyl) radical-scavenging activity assays, Somporn et al. (2011) reported that bean extracts of C. arabica cv. Catimor grown under shade had higher antioxidant activities than those from plants grown under FS.
In a more recent study on Robusta, Alves et al. (2018) analyzed the polyphenol oxidase (PPO) activity, an enzyme commonly considered as associated with good cup quality (Mazzafera, 1999). The results of sensorial analyses performed by these authors corresponded to PPO activities in beans with shade-grown coffee plants exhibiting lower PPO than those measured in beans harvested under FS condition.
The bean biochemical composition (and their associated cup quality) variation observed in shaded vs. FS cultivated coffee plants has been attributed to greater expression of genes involved in important metabolic pathways (de Castro and Marraccini, 2006; Cheng et al., 2020). For example, the elevated sucrose level observed in lower canopy (LC) beans compared to the upper canopy (UC) beans is likely the result of increased expression of a battery of genes (such as SPS1, SPS2.2, SUS2.1, CIN, VIN1, and VINI.2), previously reported to play a key role in coffee bean sugar metabolism (Geromel et al., 2006; Privat et al., 2008; Joët et al., 2009; Marraccini, 2020). Another example of this is the elevated expression of RD22 (a gene involved in plant responses to stress as reported by Yamaguchi-Shinozaki and Shinozaki, 1993) in beans from LC compared to UC, suggesting that the continued bean growth until the red stage in LC was facilitated by a stronger dehydration resistance and less chlorophyll degradation. Today, we are closer to characterize candidate genes (CGs) associated with the slow bean maturity process (Cheng et al., 2020). By selecting genotypes or environments (such as AFS) which are able to increase the expression of such CGs, higher quality coffee may be obtained, mitigating the adverse forecasted effects of climate change.
Shade Effects on Organoleptic Attributes
The previous section showed that shade influenced the biochemical composition of coffee beans. Among these compounds, it is well-known that sucrose, caffeine and trigonelline are essential flavor precursors able to form flavor components after roasting (Grosch, 2001; Homma, 2001). For example, caffeine is associated with the strength, body, and bitterness of coffee beverage and trigonelline is strongly correlated with high coffee quality (Farah et al., 2006; Janzen, 2010). The genetic origin (species and cultivar) of coffee plants can greatly influence the final cup quality (Bertrand et al., 2003, 2006; Leroy et al., 2006; Montagnon et al., 2012). The environmental conditions of coffee cultivation are also key drivers of cup quality (Bertrand et al., 2012). Positive quality attributes such as acidity, fruity character and flavor quality were found to be correlated and typical of coffees produced at cool climates and higher elevations (Bertrand et al., 2012). Therefore it is a great concern that increasing temperatures (associated with predicted climate change) will likely lead to negative impact on mid and lowland coffee quality. Worku et al. (2018) reported that the acidity of coffee grown under shade increased by 0.22 points for each 100 m increase in altitude, while no altitude effect on cup acidity was reported for coffee grown without shade. Acidity, trigonelline and total CQA content were reported to significantly increase in green beans under higher temperatures (Ramalho et al., 2018). Altogether, these findings suggest that the drift of these compounds under changing temperatures might be predominantly genotype-related. In a more recent study also performed in Ethiopia, C. arabica grown under diverse forests covers and densities (Tassew et al., 2021), also reported that cup quality organoleptic attributes (acidity, body, raw total, flavor, and cup total values) as well as grade were significantly and positively affected by shade and increasing elevation.
Several studies have highlighted the positive effects of shade on coffee cup quality (Table 2). On the contrary, a negative effect of natural shade on cup quality (fragrance, acidity, body, and sweetness) was reported for the C. arabica cv. Caturra grown at high altitudes (between 1,439 and 1,629 m.a.s.l.) in Southern Colombia (Bosselmann et al., 2009). This negative effect was likely due to the relatively high altitude of the study site, suggesting that the shaded environment promoted a lower than optimal air temperature for coffee production. Negative effects of shade on cup quality were also reported by Tolessa et al. (2017) when investigating the effects of both altitude and shade on the quality of Ethiopian specialty coffee. Their results suggested that the changes of quality scores driven by altitude, shade, and harvest period were small, but nonetheless led to re-classification of speciality coffee (Q1: SCAA note ≥85) to a lower classification (Q2: SCAA note 80–84.75) resulting in a lower international market price for farmers.
At the field level, the spatial distribution of both coffee and shade trees also influences the coffee bean biochemical composition (Delaroza et al., 2017) and consequently its cup quality. When studying the spatial distribution of different shade trees (Anadenanthera falcata, Albizia polycephala, and Cassia grandis), da Silva Neto et al. (2018) observed that the distance between coffee plants and shade trees affected the cup quality of beans from C. arabica cv. Obatã Vermelho. The best coffee cup quality was observed when coffee was harvested ~1 m from the trunk of the shade trees.
Shade effects on quality act through complex interactions with environmental (e.g., altitude/temperature), shade trees and processing (e.g., post-harvest treatments) factors, especially in sub-optimal zones (Walyaro, 1983; Joët et al., 2010; Geneti, 2019; Hameed et al., 2020). While positive effects of shade on coffee quality are now well-recognized for Arabica, few articles were dedicated to show the impact of shade on C. canephora cup quality. The shade effects on C. canephora coffee have recently been examined and shown to positively correlate with growth, yield, physiological, photosynthetic, ecological and microclimatic variables (Venancio et al., 2019; Piato et al., 2020). However, shade cover beyond 30% was associated with reduced beverage quality in the same review. When studying the effects of shade composition on Indian Robusta coffee quality, Vaast et al. (2011) reported that increasing the percentage of Grevillea robusta exotic species resulted in a decrease in cup quality as well as aroma and body. In a recent study, sensorial analyses performed by Alves et al. (2018) revealed that roasted-ripe beans of Brazilian C. canephora (known as “Conilon”) grown under rubber (Hevea brasiliensis) shade trees produced a lower cup quality than those grown under FS. The authors noticed the superior quality of sun-grown Conilon beans was also accompanied with higher lipid, total sugar contents, PPO activity and lesser membrane damages than under shade. Altogether, these observations led the authors to propose that shade-grown Conilon beans have undergone micro-organismal activity and/or undesired (or excessive) fermentation during cultivation, which resulted in lower coffee quality. Given the potential negative impact on cup quality, the use of densely shaded cultivation practices is not recommended for C. canephora. However, much work remains in order to characterize the cultivar and/or environmental dependency of bean quality changes, both in Arabica and, especially, in Robusta clones.
Breeding of New Arabica Coffee F1 Hybrids for Future Agroforestry Systems
Coffee cup quality clearly depends on coffee genotypes and on the genotype-environment interactions (Moschetto et al., 1996; Bertrand et al., 2006; Montagnon et al., 2012; Cheng et al., 2016). Historically, coffee breeding efforts have been mostly geared toward higher yield, as well as to pest and disease resistance (Lashermes et al., 2009; Bertrand et al., 2011), inadvertently at the expense of quality. Paradoxically, the demand for specialty, high quality, and sustainably sourced coffee has dramatically increased in the last decade (van der Vossen et al., 2015). These consumer trends call for coffee breeding efforts geared toward improving coffee quality and production under sustainable AFS conditions.
Over the last three decades, a new generation of Arabica coffee hybrids has been developed for a sustainable production of coffee (Bertrand et al., 2019). Unlike previous breeding programs, focused on high yielding varieties with CLR resistance (e.g., Lashermes et al., 2009; Bertrand et al., 2011), recent breeding strategies have extended the selection of Arabica hybrids to include high cup quality and adaptation to shaded systems (van der Vossen et al., 2015; Georget et al., 2019). These modern Arabica breeding programs widen the very narrow genetic base of cultivated American coffee cultivars by crossing them with wild Ethiopian germplasm (Engelmann et al., 2007; van der Vossen et al., 2015). Such crosses of cultivated lines (such as the Sarchimors) with wild pools often results in hybrid vigor where the offspring (F1-hybrids) exhibit higher vegetative growth and yield than its two parents (Fu et al., 2014). A quick recovery process of genes (in a single cross), which has also shown to be involved in adaptation to shade has recently been explored and proved to be successful when selecting ombrophilous Ethiopian individuals as the male parent to the F1 hybrids (van der Vossen et al., 2015; Georget et al., 2019).
Arabica F1 coffee hybrids have shown a better agronomic performance, yield and cup quality, and higher bean size compared to their cultivated Sarchimor parental lines (Bertrand et al., 2019; Marie et al., 2020). In Costa Rica, F1 hybrids produced between 11 and 26% more coffee yield compared to their parents and had an 8–10% increase in the 100-bean weight (Bertrand et al., 2005). Across a Central American network of 15 field trials combining FS and AFS systems, these hybrids demonstrated average yields that were 37% higher than the best commercial varieties, such as Caturra and Catimor, in both systems (Bertrand et al., 2006, 2011). Moreover, these high yields and quality parameters were more stable over varying environments, climates and production cycles, thus confirming their homeostasis and potential for acclimation to future climate conditions. When comparing performance under AFS conditions, the hybrids produced 50% more green beans than commercial pure line varieties (Bertrand et al., 2011). In culture chambers, F1 hybrids have also demonstrated a higher resistance to CLR especially when under low light conditions compared to commercial, pure line varieties, even when coupled with a low nitrogen supply as often observed in agroforestry conditions (Toniutti et al., 2017). It was recently proposed, that an altered circadian clock coupled with a higher photosynthetic efficiency could explain the superior agronomic performance of hybrids and their higher homeostasis (Toniutti et al., 2019; Breitler et al., 2020).
Molecular indicators and/or predictors, which can characterize shade-adapted coffee genotypes, are currently being identified. For example, it has been demonstrated that a high content of specific secondary metabolites such as chlorogenic acid 5-CQA and xanthone mangiferin indicates an adaptability to shade in coffee plants (Duangsodsri et al., 2020). Moreover, 5-CQA and mangiferin leaf contents, in full sun and shade, allowed for differentiating the genetic groups of Ethiopian wild accessions (higher contents) vs. cultivated American pure lines. This may be linked to the origin of the Ethiopian wild accessions, i.e., understory bushes in mesophilous forests (Sylvain, 1955) and that of American pure lines derived from the C. arabica “Yemen-Harare” group domesticated for cultivation in full sunlight (Scalabrin et al., 2020). The Arabica F1 hybrids had very similar concentrations of 5-CQA and mangiferin in the leaves of shaded and unshaded plants to those measured in their mother American pure lines, suggesting a significant maternal effect in adaptation to light growing conditions. This should be considered in future breeding programs. Such biochemical indicators will strengthen research around the potential for shade adaptability of coffee, allowing the development of physiological models of functioning under shade and can be exploited as potential markers to control the selection of more high-yielding varieties suitable for agroforestry. For this breeding purpose, all the genetic resources and the Arabica hybrids under selection must be placed under shade and this, from the juvenile stages in the nursery.
Using the new Arabica coffee F1 hybrids as a case study, the BREEDCAFS project [Breeding Coffee for Agroforestry Systems (2017–2021), https://www.breedcafs.eu/] has tested coffee varieties under AFS conditions. This project also characterized and identified the main response mechanisms of the coffee plant under several stressful conditions (e.g., heat, drought) and the potential mitigation role of eCO2 against stress impacts. Some of these new hybrids have been found to be better adapted and suited for climate change scenarios, while maintaining cup-quality and a robust defense system to biotic and abiotic stresses. The large-scale production and distribution of F1-hybrids is ongoing to provide farmers with high-quality and affordable seedlings. New horticultural solutions like mini-cuttings (Georget et al., 2017; Etienne et al., 2018) and seed gardens using mother plants with male sterility (Georget et al., 2019) are making the F1 hybrids more accessible to coffee farmer communities who often live in remote mountainous areas.
Unlike Arabica coffee, breeding programs geared toward C. canephora coffee currently do not select for shaded cultivation systems (Carvalho et al., 2019; Alkimim et al., 2021). Moreover, selection criteria for AFS were not clearly established until Bertrand et al. (2021) proposed a list of target traits including productivity, size of the beans, sensory score, and coffee tree volume allowing a high number of trees per hectare. Applying these criteria, they found hybrids capable of producing up to 22% more than the best pure line under full sun. Nevertheless, selecting for AFS requires years of phenotyping data and are quite time and money consuming. By combining extensive phenotyping with eco-physiological, metabolomic and transcriptomic studies, analytical, and predictive tools for coffee genomic selection have been recently developed (Mbebi et al., 2021). This has led to marker-aided rapid selection and a novel approach for breeding of perennial crops.
If climate change leads to extreme temperature increases, the extensive gene pool of the more than 120 wild species (Armarego-Marriott, 2021) may be researched and exploited in breeding programs aiming for more climate resilient coffee plants. Currently, only the two species C. arabica and C. canephora are cultivated. The idea was given recently by Davis et al. (2021) who reported that C. stenophylla, a wild species from Upper West Africa reveals a superior flavor and a sensory profile analogous to high-quality Arabica coffee. These authors demonstrated that this species grows at a mean annual temperature 6.2–6.8°C higher than Arabica coffee. Another example is C. racemosa Lour. (formerly known as C. ibo) which received a golden medal in a Lisbon fair in 1906 due to unique characteristics of taste and aroma, considered the best coffee of all Portuguese colonies (Vasconcellos, 1906), being present in lowlands (below 200 m) and areas of low water availability in Mozambique (Hallé and Faria, 1973). The valorization of these wild species could result in the selection of a large number of interspecific hybrids adapted to much hotter and drier climates as well as to shade.
Coffee Production Under Climate Change
Some climate model scenarios estimate a 50% decrease in the global area suitable for coffee production by 2050, which can reach up to 85% reduction in Brazil alone (Davis et al., 2012; Baca et al., 2014; Bunn et al., 2015a,b). This loss of adequate areas was estimated to be accompanied by aggravated incidence of pests and diseases (Magrach and Ghazoul, 2015), severe yield drops (van der Vossen et al., 2015), and even the extinction of a large number of wild coffee species (Davis et al., 2019). Still, negative climate impacts in coffee producing regions can be attenuated through climate adaptation measures. These include research, extension, and credit subsidies for improved coffee varieties, adequate irrigation, and the implementation of AFS management and ultimately considering the possibility of crop substitution (Koh et al., 2020). However, some coffee genotypes have demonstrated an intrinsic resilience to environmental constraints (Dubberstein et al., 2020; Semedo et al., 2021) than traditionally anticipated, but in line with the somewhat harsh environmental conditions endured by the plants under full sun conditions (DaMatta and Ramalho, 2006). Furthermore, a growing body of recent evidence shows that the increasing concentration of atmospheric CO2 (usually associated to global warming), can actually improve the coffee plant photosynthetic performance (Ramalho et al., 2013; Ghini et al., 2015), promote carbon investment in reproductive structures (Rakocevic et al., 2020), and boost yield under adequate rain fed (or irrigated) water supply (DaMatta et al., 2019; Pham et al., 2019). More importantly, eCO2 was shown to strengthen the resilience to supra-optimal temperatures on both C. arabica and C. canephora plants up to ~37°C or even 42°C (Martins et al., 2014, 2016; Rodrigues et al., 2016; Marques et al., 2021). This was associated with a reinforcement of photochemical efficiency, biochemical functioning, and protective mechanisms (Martins et al., 2016; Rodrigues et al., 2016; Scotti-Campos et al., 2019; Avila et al., 2020a,b). Although eCO2 can alter the content of some compounds in the coffee bean (Marcheafave et al., 2020; Rakocevic et al., 2021), it may also have a positive role in the preservation of bean quality under heat stress (Ramalho et al., 2018). Recent reports show the combined effects of high light and eCO2 in improving coffee growth and photosynthetic performance (Marçal et al., 2021). However, allometric and biomass partitioning of the coffee plants is affected by combined high light and eCO2 treatments, most commonly resulting in a higher root biomass-to-total leaf area and lower leaf area ratio (Avila et al., 2020a; Marçal et al., 2021). Other studies have attempted to mimic high atmospheric demand (such as elevated vapor pressure deficit) under non-limiting soil-water supply (Machado Filho et al., 2021). Genotypic variation was observed in root and stem hydraulic conductances and conductivity, as well as whole plant conductivity as a coping mechanism for elevated vapor pressure deficit in coffee. Studies such as these allow for physiological predictions of how elevated temperatures can affect coffee in irrigated farming systems.
Additionally, eCO2 was recently reported to have also marked implications on how coffee plants respond to soil water deficit, greatly attenuating drought impacts (even under severe water deficit conditions) regarding, among others, the photosynthetic performance, hydraulic conductance, and growth, as well as gene expression and metabolite profile (Avila et al., 2020a,b; Fernandes et al., 2021; Rodrigues et al., 2021; Semedo et al., 2021). When eCO2 is combined with a single drought episode, high genotypic heterogeneity has been observed in the primary metabolite responses of both C. arabica and C. canephora cultivars (Rodrigues et al., 2021). These findings stress the phenotypic plasticity of the Coffea genome and how it can be harnessed through targeted climate adaptation breeding programs. Moreover, improved modeling approaches (namely integrating the eCO2 “fertilization” effect) can constitute a powerful tool to assist coffee cultivation under climate change (Rahn et al., 2018). By designing shade management strategies (i.e., selecting the right tree species according to the local context and regulating shade level along an altitudinal or rainfall range) coffee systems can be adapted to climate change at landscape scale. Despite recent projections by Moat et al. (2017), showing an overall negative impact of climate change on the Ethiopian coffee sector, the recent findings on eCO2 and the use of elite coffee genotypes reviewed here support a less grim perspective than earlier forecasted by modeling approaches eluded to (largely based on temperature drifts) (DaMatta et al., 2019). In this context, continued breeding for improved shade tolerance and climate adaptability will likely further improve land-use prospects for coffee cultivation.
Concluding Remarks
We started out this work in order to shed light on whether shaded-coffee is a feasible, nature-based solution for climate change in coffee production. In order to answer this enquiry, we took a comprehensive look at the coffee farm, starting with the farmer's use of shade and global perceptions of the services of shade trees across the coffee belt. We then focused on the aboveground interactions of the shaded coffee microenvironment (including effects on the air temperature and water-use) as well as pest and disease incidence. The carbon assimilation and phenology of coffee plants was examined under shaded environments as these aspects directly relate to coffee yields. The development of coffee quality attributes under shade is highly contentious and thus also present in this evaluation of the coffee-AFS. Breeding trends and directions were considered in relation to Arabica F1 hybrids and Robusta clones under AFS. Finally, we evaluated the newest data pertaining to coffee production under climate change in order to determine the most relevant physiological limitations of the coffee plant under future climates.
Many smallholder coffee farmers demonstrate a sound expertise in shade management for coffee production throughout the seasons to optimize and counteract farm humidity, light interception and fungal pathogen attacks. By utilizing shade, coffee farmers may also reap the associated benefits including increased biodiversity, biological control of pests and diseases, climate-buffering services, as well as diversified incomes resulting from shade-tree products. While there has been a general decrease in coffee AFS over the last couple of decades, the growth in demand for specialty, certified coffee, combined with consumers' increased concern for sustainability and farmers' need to adapt to climate change, could reverse the trend, and favor the uptake of more coffee AFS in the future.
Shaded-coffee systems are shown to alter both the above- and belowground microenvironment, and impact the physiology, phenology (and therefore yield) as well as quality attributes of coffee. Recent work examining the shade effects (associated with AFS) on the coffee micro-environment tended to corroborate past studies which showed reductions in air temperatures, wind speeds, radiation levels, weeds, coffee water transpiration losses, and protection from frost events.
Regarding the physiology and phenology of coffee plants under shade, the photosynthetic performance of coffee plants can potentially compensate for the reduction in irradiation under shaded-environments; however, a lowered flowering intensity can lead to yield reductions if the shade level is too high. These physiological aspects could be taken into account also in future breeding efforts, in order to reduce negative shade effects in new genotypes.
In terms of the shade effects on coffee diseases and pests, many of the recent findings appear to be locally specific to their environments and/or the shade tree species used, hence a large variation was observed by a number of studies in this context. Pest or pathogen management advice should be as local as possible and linked to weather forecasting (especially precipitation and humidity) in order to be relevant to the coffee farming region.
Several cup quality and/or biochemical attributes of coffee were also shown to evolve positively under shade on C. arabica cultivars such as Catuaí, Catimors (especially CR95), and Sarchimors (especially IAPAR59), although for some cultivars no sensible advantages arise from shade implementation. Moreover, recent Arabica breeding programs have developed F1-hybrids able to maintain high yield and cup quality under shaded conditions. In the case of C. canephora coffee, preliminary research has pointed toward a decrease in quality aspects under shaded environments. Moreover, current breeding trends for C. canephora do not prioritize selection for shade, but could be oriented toward breeding for high-temperature-resistant clones.
Studies examining the impact of eCO2 on the coffee plant are quite new (within the last decade), but have already shown that eCO2 can improve coffee plant photosynthetic performance, promote carbon investment in reproductive structures, boost yield, and, especially important can increase plant vigor and its resilience to heat and drought constraints. However, the coffee plant is still highly vulnerable to the other detrimental impacts associated with climate change, thus new management practices must be considered for future production.
Overall, coffee AFS should match farmers' needs along with risk assessments of climatic hazards specific to the local environment. When AFS are site-specific in this way they can act as an adaptation strategy against climate change. The buffering effects, which AFS have on the coffee microclimate, serve as a mitigation against unfavorable environments or climate change events. Given this, coffee farmers may in fact be better off managing an AFS compared to intensive FS systems. Finally, the array of ecosystem services together with alternate revenue streams and increased cup qualities (for Arabica), provided by shade trees, may help compensate for potential yield losses under coffee AFS.
In order to correct the geography bias evident in the literature, an expansion of AFS-coffee studies is encouraged to take place in South East Asia (e.g., Vietnam and India) as well as in Africa (e.g., Kenya, Tanzania, and Uganda). This would allow for the examination of coffee-AFS across different cultivation environments and eventually lead to the implementation of management practices, which are both culturally and locally relevant. Future research should continue the development of coffee varieties adapted to agroforestry systems, in particular those which can maintain a high level of yield under shade. This can help to improve the overall profitability of coffee plantations in AFS. Research must continue to refine extension tools including the selection of locally-adapted shade tree species and mobilize their widespread use by coffee farmers. Other management practices can also be optimized along the coffee production cycle such especially concerning shade tree management (i.e., timely pruning). Finally, multi-faceted approaches which consider the market, social, and policy issues must also come into play in order to provide necessary recommendations to enable the adoption of AFS in coffee cultivation. An example of this is an incentivized scheme for the renovation of existing coffee farms using AFS together with new Arabica hybrid varieties bred for the AFS environment.
Author Contributions
Conceptualization was conducted by AK, PM, and AR. Literature review was conducted by AK and supplemented by all authors. Writing (original draft preparation) was conducted by AK, AB, CC, CR, HE, JR, MB, NT-G, PM, PV, and TS. All authors contributed to the writing (review and editing) and approved the final version of the manuscript.
Funding
This work was funded by and carried out in the context of the European Union's Horizon 2020 (H2020) research and innovation program by the BREEDCAFS (Breeding Coffee for Agroforestry Systems) project (2017–2021) under the grant agreement No. 727934 (www.breedcafs.eu). Portuguese national funding from Fundação para a Ciência e a Tecnologia (FCT), through the research units UIDB/00239/2020 (CEF) and UIDP/04035/2020 (GeoBioTec) are also acknowledged. TS benefits from a thesis scholarship jointly financed by CIRAD and ECOM trading.
Conflict of Interest
The authors declare that the research was conducted in the absence of any commercial or financial relationships that could be construed as a potential conflict of interest.
Publisher's Note
All claims expressed in this article are solely those of the authors and do not necessarily represent those of their affiliated organizations, or those of the publisher, the editors and the reviewers. Any product that may be evaluated in this article, or claim that may be made by its manufacturer, is not guaranteed or endorsed by the publisher.
Abbreviations
AFS, agroforestry systems; CLR, coffee leaf rust; eCO2, elevated air CO2; FS, full-sun; WoS, web of science.
References
Abdulai, I., Vaast, P., Hoffmann, M. P., Asare, R., Jassogne, L., Van Asten, P., et al. (2018). Cocoa agroforestry is less resilient to sub-optimal and extreme climate than cocoa in full sun. Glob. Change Biol. 24, 273–286. doi: 10.1111/gcb.13885
Albers, H. J., Brockmann, S., and Ávalos-Sartorio, B. (2021). Assessing policies to mitigate abandonment of shade-grown coffee production in forest systems amid low and uncertain prices. Environ. Dev. Econ. 26, 403–428. doi: 10.1017/S1355770X20000443
Alkimim, E. R., Caixeta, E. T., Sousa, T. V., Gois, I. B., Lopes da Silva, F., Sakiyama, N. S., et al. (2021). Designing the best breeding strategy for Coffea canephora: genetic evaluation of pure and hybrid individuals aiming to select for productivity and disease resistance traits. PLoS ONE 16, e0260997. doi: 10.1371/journal.pone.0260997
Allinne, C., Savary, S., and Avelino, J. (2016). Delicate balance between pest and disease injuries, yield performance, and other ecosystem services in the complex coffee-based systems of Costa Rica. Agr. Ecosyst. Environ. 222, 1–12. doi: 10.1016/j.agee.2016.02.001
Alves, A. L., Pessoa, M. S., de Souza, P. E. N., Partelli, F. L., Moscon, P. S., da Silva, E. C., et al. (2018). Influence of environmental and microclimate factors on the coffee beans quality (C. canephora): correlation between chemical analysis and stable free radicals. Agr. Sci. 9, 1173–1187. doi: 10.4236/as.2018.99082
Araújo, A. V., Partelli, F. L., Oliosi, G., and Pezzopane, J. R. M. (2016). Microclimate, development and productivity of robusta coffee shaded by rubber trees and at full sun. Rev. Cienc. Agron. 47, 700–709. doi: 10.5935/1806-6690.20160084
Araujo, W. L., Dias, P. C., Moraes, G. A., Celin, E. F., Cunha, R. L., Barros, R. S., et al. (2008). Limitations to photosynthesis in coffee leaves from different canopy positions. Plant Physiol. Biochem. 46, 884–890. doi: 10.1016/j.plaphy.2008.05.005
Armarego-Marriott, T. (2021). ‘Hot' coffee taste test. Nature Clim. Change 11, 378–378. doi: 10.1038/s41558-021-01051-4
Asayehegn, K., Temple, L., Vaast, P., and Iglesias, A. (2018). “Innovation systems to adapt to climate change: Lessons from the Kenyan coffee and dairy sectors,” in Handbook of Climate Change Resilience, ed W. L. Filho (Cham: Springer International Publishing), 2249–2272.
Avelino, J., Romero-Gurdián, A., Cruz-Cuellar, H. F., and Declerck, F. A. (2012). Landscape context and scale differentially impact coffee leaf rust, coffee berry borer, and coffee root-knot nematodes. Ecol. Appl. 22, 584–596. doi: 10.1890/11-0869.1
Avelino, J., Vílchez, S., Segura-Escobar, M. B., Brenes-Loaiza, M. A., Virginio Filho, E. D. M., and Casanoves, F. (2020). Shade tree Chloroleucon eurycyclum promotes coffee leaf rust by reducing uredospore wash-off by rain. Crop Prot. 129, 105038. doi: 10.1016/j.cropro.2019.105038
Avila, R. T., Almeida, W. L., Costa, L. C., Machado, K. L. G., Barbosa, M. L., Souza, R. P. B., et al. (2020a). Elevated air [CO2] improves photosynthetic performance and alters biomass accumulation and partitioning in drought-stressed coffee plants. Environ. Exp. Bot. 177, 104137. doi: 10.1016/j.envexpbot.2020.104137
Avila, R. T., Cardoso, A. A., Almeida, W. L., Costa, L. C., Machado, K. L. G., Barbosa, M., et al. (2020b). Coffee plants respond to drought and elevated [CO2] through changes in stomatal function, plant hydraulic conductance, and aquaporin expression. Environ. Exp. Bot. 177, 104148. doi: 10.1016/j.envexpbot.2020.104148
Baca, M., Läderach, P., Haggar, J., Schroth, G., and Ovalle, O. (2014). An integrated framework for assessing vulnerability to climate change and developing adaptation strategies for coffee growing families in Mesoamerica. PLoS ONE 9, e88463. doi: 10.1371/journal.pone.0088463
Baggio, A. J., Caramori, P. H., Androcioli Filho, A., and Montoya, L. (1997). Productivity of southern Brazilian coffee plantations shaded by different stockings of Grevillea robusta. Agroforest. Sys. 37, 111–120. doi: 10.1023/a:1005814907546
Bagyaraj, D. J., Thilagar, G., Ravisha, C., Kushalappa, C. G., Krishnamurthy, K. N., and Vaast, P. (2015). Below ground microbial diversity as influenced by coffee agroforestry systems in the Western Ghats, India. Agric. Ecosyst. Environ. 202, 198–202. doi: 10.1016/j.agee.2015.01.015
Bayala, J., and Prieto, I. (2020). Water acquisition, sharing and redistribution by roots: applications to agroforestry systems. Plant Soil 453, 17–28. doi: 10.1007/s11104-019-04173-z
Beer, J., Muschler, R., Kass, D., and Somarriba, E. (1998). Shade management in coffee and cacao plantations. Agroforest. Syst. 38, 139–164. doi: 10.1023/A:1005956528316
Bertrand, B. (2002). L'amélioration génétique de Coffea arabica L. en Amérique Centrale par la voie hybride F1 (Doctoral dissertation), ENSAM, Montpellier, France.
Bertrand, B., Alpizar, E., Lara, L., SantaCreo, R., Hidalgo, M., Quijano, J. M., et al. (2011). Performance of Coffea arabica F1 hybrids in agroforestry and full-sun cropping systems in comparison with American pure line cultivars. Euphytica 181, 147–158. doi: 10.1007/s10681-011-0372-7
Bertrand, B., Boulanger, R., Dussert, S., Ribeyre, F., Berthiot, L., Descroix, F., et al. (2012). Climatic factors directly impact the volatile organic compound fingerprint in green Arabica coffee bean as well as coffee beverage quality. Food Chem. 135, 2575–2583. doi: 10.1016/j.foodchem.2012.06.060
Bertrand, B., Breitler, J.-C., Georget, F., Penot, E., Bordeaux, M., Marraccini, P., et al. (2019). “New varieties for innovative agroforestry coffee systems,” in The Agroecological Transition of Agricultural Systems in the Global South, eds F. X. Côte, E. Poirier-Magona, S. Perret, P. Roudier, B. Rapidel, and M. C. Thirion (Versailles: Quae Editor), 161–176. doi: 10.35690/978-2-7592-3057-0
Bertrand, B., Etienne, H., Cilas, C., Charrier, A., and Baradat, P. (2005). Coffea arabica hybrid performance for yield, fertility and bean weight. Euphytica 141, 255–262. doi: 10.1007/s10681-005-7681-7
Bertrand, B., Guyot, B., Anthony, F., and Lashermes, P. (2003). Impact of Coffea canephora gene introgression on beverage quality of C. arabica. Theor. Appl. Genet. 107, 387–394. doi: 10.1007/s00122-003-1203-6
Bertrand, B., Vaast, P., Alpizar, E., Etienne, H., Davrieux, F., and Charmetant, P. (2006). Comparison of bean biochemical composition and beverage quality of Arabica hybrids involving Sudanese-Ethiopian origins with traditional varieties at various elevations in Central America. Tree Physiol. 26, 1239–1248. doi: 10.1093/treephys/26.9.1239
Bertrand, B., Villegas Hincapie, A. M., Marie, L., and Breitler, J.-C. (2021). Breeding for the main agricultural farming of arabica coffee. Front. Sustain. Food Syst. 5, 709901. doi: 10.3389/fsufs.2021.709901
Boreux, V., Vaast, P., Madappa, L. P., Cheppudira, K. G., Garcia, C., and Ghazoul, J. (2016). Agroforestry coffee production increased by native shade trees, irrigation, and liming. Agron. Sustain. Dev. 36, 1–9. doi: 10.1007/s13593-016-0377-7
Borrella, I., Mataix, C., and Carrasco-Gallego, R. (2015). Smallholder farmers in the speciality coffee industry: opportunities, constraints and the businesses that are making it possible. IDS Bull. 46, 29–44. doi: 10.1111/1759-5436.12142
Bosselmann, A. S., Dons, K., Oberthur, T., Olsen, C. S., Ræbild, A., and Usma, H. (2009). The influence of shade trees on coffee quality in small holder coffee agroforestry systems in Southern Colombia. Agr. Ecosyst. Environ. 129, 253–260. doi: 10.1016/j.agee.2008.09.004
Bote, A. D., and Struik, P. C. (2011). Effects of shade on growth, production and quality of coffee (Coffea arabica) in Ethiopia. J. Hortic. For. 3, 336–341. Available online at: https://edepot.wur.nl/192807.
Bradbury, A. G., and Halliday, D. J. (1990). Chemical structures of green coffee bean polysaccharides. J. Agr. Food Chem. 38, 389–392. doi: 10.1021/jf00092a010
Braga, G. B., Imbuzeiro, H. M. A., Pires, G. F., Oliveira, L. R. D., Barbosa, R. A., and Vilela, K. D. F. (2021). Frost risk and rural insurance in Brazil. Rev. Bras. Meteorol. 36, 703–711. doi: 10.1590/0102-7786360137
Bravo-Monroy, L., Potts, S. G., and Tzanopoulos, J. (2016). Drivers influencing farmer decisions for adopting organic or conventional coffee management practices. Food Policy 58, 49–61. doi: 10.1016/j.foodpol.2015.11.003
Breitler, J.-C., Djerrab, D., Léran, S., Toniutti, L., Guittin, C., Barrachina, C., et al. (2020). Coffea arabica clock entrainment by full moon light. BMC Plant Biol. 20, 24. doi: 10.1186/s12870-020-2238-4
Bukomeko, H., Jassogne, L., Kagezi, G. H., Mukasa, D., and Vaast, P. (2018). Influence of shaded systems on Xylosandrus compactus infestation in Robusta coffee along a rainfall gradient in Uganda. Agr. For. Entomol. 20, 327–333. doi: 10.1111/afe.12265
Bunn, C., Läderach, P., Ovalle Rivera, O., and Kirschke, D. (2015a). A bitter cup: climate change profile of global production of Arabica and Robusta coffee. Clim. Change 129, 89–101. doi: 10.1007/s10584-014-1306-x
Bunn, C., Läderach, P., Pérez Jimenez, J. G., Montagnon, C., and Schilling, T. (2015b). Multiclass classification of agro-ecologicalzones for Arabica coffee: an improved understanding of the impacts of climate change. PLoS ONE 10, e0140490. doi: 10.1371/journal.pone.0140490
Buyinza, J., Nuberg, I. K., Muthuri, C. W., and Denton, M. D. (2022). Farmers' knowledge and perceptions of management and the impact of trees on-farm in the Mt. Elgon region of Uganda. Small-Scale For. 21, 71–92. doi: 10.1007/s11842-021-09488-3
Byrareddy, V., Kouadio, L., Mushtaq, S., Kath, J., and Stone, R. (2021). Coping with drought: Lessons learned from robusta coffee growers in Vietnam. Clim. Serv. 22, 100229. doi: 10.1016/j.cliser.2021.100229
Campa, C., Urban, L., Mondolot, L., Fabre, D., Roques, S., Lizzi, Y., et al. (2017). Juvenile coffee leaves acclimated to low light are unable to cope with a moderate light increase. Front. Plant Sci. 8, 1126. doi: 10.3389/fpls.2017.01126
Campanha, M. M., Santos, R. H. S., De Freitas, G. B., Martinez, H. E. P., Garcia, S. L. R., and Finger, F. L. (2004). Growth and yield of coffee plants in agroforestry and monoculture systems in Minas Gerais, Brazil. Agroforest. Syst. 63, 75–82. doi: 10.1023/b:agfo.0000049435.22512.2d
Cannavo, P., Sansoulet, J., Harmand, J. M., Siles, P., Dreyer, E., and Vaast, P. (2011). Agroforestry associating coffee and Inga densiflora results in complementarity for water uptake and decreases deep drainage in Costa Rica. Agr. Ecosyst. Environ. 140, 1–13. doi: 10.1016/j.agee.2010.11.005
Cannell, M. G. R. (1974). Factors affecting arabica coffee bean size in Kenya. J. Hort. Sci. 49, 65–76. doi: 10.1080/00221589.1974.11514552
Cannell, M. G. R. (1976). Crop physiological aspects of coffee bean yield - a review. Kenya Coffee 41, 245–253.
Cannell, M. G. R. (1985). “Physiology of the coffee crop,” in Coffee, eds M. N. Clifford and K. C. Willson (Boston, MA: Springer), 108–134. doi: 10.1007/978-1-4615-6657-1_5
Caramori, P. H., Androcioli Filho, A., and Leal, A. C. (1996). Coffee shade with Mimosa scabrella Benth. for frost protection in southern Brazil. Agroforest. Syst. 33, 205–214. doi: 10.1007/BF00055423
Carvalho, A., Krug, C. A., Mendes, J. E. T., Antunes Filho, H., Junqueira, A. R., Aloisio Sobrinho, J., et al. (1961). Melhoramento do Cafeeiro: XXI-Comportamento regional de variedades. Linhagens e progênies de café ao sol e à sombra. Bragantia 20, 1045–1142. doi: 10.1590/s0006-87051961000100046
Carvalho, H. F., Silva, F. L. D., Resende, M. D. V. D., and Bhering, L. L. (2019). Selection and genetic parameters for interpopulation hybrids between kouilou and robusta coffee. Bragantia 78, 52–59. doi: 10.1590/1678-4499.2018124
Castillo, N. E. T., Melchor-Martínez, E. M., Sierra, J. S. O., Ramirez-Mendoza, R. A., Parra-Saldívar, R., and Iqbal, H. M. (2020). Impact of climate change and early development of coffee rust–an overview of control strategies to preserve organic cultivars in Mexico. Sci. Total Environ. 738, 140225. doi: 10.1016/j.scitotenv.2020.140225
Ceballos-Sierra, F., and Dall'Erba, S. (2021). The effect of climate variability on Colombian coffee productivity: a dynamic panel model approach. Agr. Syst. 190, 103126. doi: 10.1016/j.agsy.2021.103126
Cerda, R., Avelino, J., Harvey, C. A., Gary, C., Tixier, P., and Allinne, C. (2020). Coffee agroforestry systems capable of reducing disease-induced yield and economic losses while providing multiple ecosystem services. Crop Protect. 134, 105149. doi: 10.1016/j.cropro.2020.105149
Cerdán, C. R., Rebolledo, M. C., Soto, G., Rapidel, B., and Sinclair, F. L. (2012). Local knowledge of impacts of tree cover on ecosystem services in smallholder coffee production systems. Agr. Syst. 110, 119–130. doi: 10.1016/j.agsy.2012.03.014
Charbonnier, F., Le Maire, G., Dreyer, E., Casanoves, F., Christina, M., Dauzat, J., et al. (2013). Competition for light in heterogeneous canopies: application of MAESTRA to a coffee (Coffea arabica L.) agroforestry system. Agr. For. Meteorol. 181, 152–169. doi: 10.1016/j.agrformet.2013.07.010
Charbonnier, F., Roupsard, O., Le Maire, G., Guillemot, J., Casanoves, F., Lacointe, A., et al. (2017). Increased light-use efficiency sustains net primary productivity of shaded coffee plants in agroforestry system. Plant Cell Environ. 40, 1592–1608. doi: 10.1111/pce.12964
Chazdon, R. L., Pearcy, R. W., Lee, D. W., and Fetcher, N. (1996). “Photosynthetic responses of tropical forest plants to contrasting light environments,” in Tropical Forest Plant Ecophysiology, eds S. S. Mulkey, R. L. Chazdon, and A. P. Smith (Boston, MA: Springer). doi: 10.1007/978-1-4613-1163-8_1
Cheng, B., Furtado, A., Smyth, H. E., and Henry, R. J. (2016). Influence of genotype and environment on coffee quality. Trends Food Sci. Technol. 57, 20–30. doi: 10.1016/j.tifs.2016.09.003
Cheng, B., Smyth, H. E., Furtado, A., and Henry, R. J. (2020). Slower development of lower canopy beans produces better coffee. J. Exp. Bot. 71, 4201–4214. doi: 10.1093/jxb/eraa151
Chengappa, P. G., Devika, C. M., and Rudragouda, C. S. (2017). Climate variability and mitigation: perceptions and strategies adopted by traditional coffee growers in India. Clim. Dev. 9, 593–604. doi: 10.1080/17565529.2017.1318740
Clemens, H., and Zablah, J. S. (1993). Tecnología y desarrollo del sector cafetalero en Nicaragua: Caracterización del manejo del cultivo del café en la IV Región, ciclo 1990/91. Managua: Universidad Nacional Autónoma de Nicaragua, Facultad de Ciencias Económicas, Escuela de Economía Agrícola.
Cole, R. J. (2010). Social and environmental impacts of payments for environmental services for agroforestry on small-scale farms in southern Costa Rica. Int. J. Sustain. Dev. World Ecol. 17, 208–216. doi: 10.1080/13504501003729085
Coltri, P. P., Pinto, H. S., do Valle Gonçalves, R. R., Junior, J. Z., and Dubreuil, V. (2019). Low levels of shade and climate change adaptation of Arabica coffee in south eastern Brazil. Heliyon 5, e01263. doi: 10.1016/j.heliyon.2019.e01263
Courbier, S., and Pierik, R. (2019). Canopy light quality modulates stress responses in plants. iScience 22, 441–452. doi: 10.1016/j.isci.2019.11.035
da Silva Neto, F. J., Gomes Morinigo, K. P., de França Guimarães, N., de Souza Gallo, A., Bispo de Souza, M. D., Stolf, R., et al. (2018). Shade trees spatial distribution and its effect on grains and beverage quality of shaded coffee trees. J. Food Qual. 8, 7909467. doi: 10.1155/2018/7909467
DaMatta, F. M. (2004). Ecophysiological constraints on the production of shaded and unshaded coffee: a review. Field Crops Res. 86, 99–114. doi: 10.1016/j.fcr.2003.09.001
DaMatta, F. M., Avila, R. T., Cardoso, A. A., Martins, S. C., and Ramalho, J. C. (2018). Physiological and agronomic performance of the coffee crop in the context of climate change and global warming: a review. J. Agr. Food Chem. 66, 5264–5274. doi: 10.1021/acs.jafc.7b04537
DaMatta, F. M., Rahn, E., Läderach, P., Ghini, R., and Ramalho, J. C. (2019). Why could the coffee crop endure climate change and global warming to a greater extent than previously estimated? Clim. Change 152, 167–178. doi: 10.1007/s10584-018-2346-4
DaMatta, F. M., and Ramalho, J. C. (2006). Impacts of drought and temperature stress on coffee physiology and production: a review. Braz. J. Plant. Physiol. 18, 55–81. doi: 10.1590/s1677-04202006000100006
Davis, A. P., Chadburn, H., Moat, J., O'Sullivan, R., Hargreaves, S., and Lughadha, E. N. (2019). High extinction risk for wild coffee species and implications for coffee sector sustainability. Sci. Adv. 5, eaav3473. doi: 10.1126/sciadv.aav3473
Davis, A. P., Gole, T. W., Baena, S., and Moat, J. (2012). The impact of climate change on indigenous Arabica coffee (Coffea arabica): predicting future trends and identifying priorities. PLoS ONE 7, e47981. doi: 10.1371/journal.pone.0047981
Davis, A. P., Mieulet, D., Moat, J., Sarmu, D., and Haggar, J. (2021). Arabica-like flavour in a heat-tolerant wild coffee species. Nat. Plants 7, 413–418. doi: 10.1038/s41477-021-00891-4
de Carvalho, A. F., Fernandes-Filho, E. I., Daher, M., de Carvalho Gomes, L., Cardoso, I. M., Fernandes, R. B. A., et al. (2021). Microclimate and soil and water loss in shaded and unshaded agroforestry coffee systems. Agroforest. Syst. 95, 119–134. doi: 10.1007/s10457-020-00567-6
de Castro, R. D., and Marraccini, P. (2006). Cytology, biochemistry and molecular changes during coffee fruit development. Braz. J. Plant Physiol. 18, 175–199. doi: 10.1590/s1677-04202006000100013
De Giusti, G., Kristjanson, P., and Rufino, M. C. (2019). Agroforestry as a climate change mitigation practice in smallholder farming: evidence from Kenya. Clim. Change 153, 379–394. doi: 10.1007/s10584-019-02390-0
de Sousa, K., van Zonneveld, M., Holmgren, M., Kindt, R., and Ordoñez, J. C. (2019). The future of coffee and cocoa agroforestry in a warmer Mesoamerica. Sci. Rep. 9, 8828. doi: 10.1038/s41598-019-45491-7
de Souza, H. N., de Goede, R. G., Brussaard, L., Cardoso, I. M., Duarte, E. M., Fernandes, R. B., et al. (2012). Protective shade, tree diversity and soil properties in coffee agroforestry systems in the Atlantic Rainforest biome. Agr. Ecosyst. Environ. 146, 179–196. doi: 10.1016/j.agee.2011.11.007
Delaroza, F., Rakocevic, M., Malta, G. B., Sanchez, P. M., Bruns, R. E., and Scarminio, I. S. (2017). Factorial design effects of plant density, pattern and light availability on the caffeine, chlorogenic acids, lipids, reducing sugars and ash contents of Coffea arabica L. beans and leaves. Anal. Methods 9, 3612–3618. doi: 10.1039/c7ay00721c
Duangsodsri, T., Villain, L., Vestalys, I. R., Michalet, S., Abdallah, C., Breitler, J.-C., et al. (2020). 5-CQA and mangiferin, two leaf biomarkers of adaptation to full sun or shade conditions in Coffea arabica L. Metabolites 10, 383. doi: 10.3390/metabo10100383
Dubberstein, D., Lidon, F. C., Rodrigues, A. P., Semedo, J. N., Marques, I., Rodrigues, W. P., et al. (2020). Resilient and sensitive key points of the photosynthetic machinery of Coffea spp. to the single and superimposed exposure to severe drought and heat stresses. Front. Plant Sci. 11, 1049. doi: 10.3389/fpls.2020.01049
Dubberstein, D., Rodrigues, W. P., Semedo, J. N., Rodrigues, A. P., Pais, I. P., Leitão, A. E., et al. (2018). “Chapter 4: Mitigation of the negative impact of warming on the coffee crop – the role of increased air [CO2] and management strategies,” in Climate Resilient Agriculture - Strategies and Perspectives, eds C. Srinivasarao, A. K. Shanker, and C. Shanker (London: Intech), 57–85. doi: 10.5772/intechopen.72374
Dumont, E. S., Gassner, A., Agaba, G., Nansamba, R., and Sinclair, F. (2019). The utility of farmer ranking of tree attributes for selecting companion trees in coffee production systems. Agroforest. Syst. 93, 1469–1483. doi: 10.1007/s10457-018-0257-z
Durand-Bessart, C., Tixier, P., Quinteros, A., Andreotti, F., Rapidel, B., Tauvel, C., et al. (2020). Analysis of interactions amongst shade trees, coffee foliar diseases and coffee yield in multistrata agroforestry systems. Crop Protect. 133, 105137. doi: 10.1016/j.cropro.2020.105137
Ehrenbergerová, L., Cienciala, E., Kučera, A., Guy, L., and Habrov,á, H. (2016). Carbon stock in agroforestry coffee plantations with different shade trees in Villa Rica, Peru. Agroforest. Syst. 90, 433–445. doi: 10.1007/s10457-015-9865-z
Ehrenbergerová, L., Klimková, M., Cano, Y. G., Habrová, H., Lvončík, S., Volarík, D., et al. (2021). Does shade impact coffee yield, tree trunk, and soil moisture on Coffea canephora plantations in Mondulkiri, Cambodia? Sustainability 13, 13823. doi: 10.3390/su132413823
Engelmann, F., Dulloo, M. E., Astorga, C., Dussert, S., and Anthony, F. (2007). Conserving Coffee Genetic Resources. IRD Editions.
Enveritas (2019). Available online at: https://carto.com/blog/enveritas-coffee-poverty-visualization/ (accessed February 3, 2022).
Etienne, H., Breton, D., Breitler, J.-C., Bertrand, B., Déchamp, E., Awada, R., et al. (2018). Coffee somatic embryogenesis: how did research, experience gained and innovations promote the commercial propagation of elite clones from the two cultivated species? Front. Plant Sci. 9, 1630. doi: 10.3389/fpls.2018.01630
Fahl, J. I., Carelli, M. C., Vega, J., and Magalhaes, A. C. (1994). Nitrogen and irradiance levels affecting net photosynthesis and growth of young coffee plants (Coffea arabica L.). J. Hort. Sci. 69, 161–169. doi: 10.1080/14620316.1994.11515262
Farah, A., Monteiro, M., Calado, V., Franca, A. S., and Trugo, L. C. (2006). Correlation between cup quality and chemical attributes of Brazilian coffee. Food Chem. 98, 373–380. doi: 10.1016/j.foodchem.2005.07.032
Fernandes, I., Marques, I., Paulo, O. S., Batista, D., Partelli, F. L., Lidon, F. C., et al. (2021). Understanding the impact of drought in Coffea genotypes: transcriptomic analysis supports a common high resilience to moderate water deficit but a genotype dependent sensitivity to severe water deficit. Agronomy 11, 2255. doi: 10.3390/agronomy11112255
Franck, N., Vaast, P., and Dauzat, J. (2007). “Coffee a shade-adapted plant: Implications on its carbon balance and consequences on coffee yield and quality in agroforestry systems,” in 21st International Conference on Coffee Science, 1023–1031. Available online at: https://agritrop.cirad.fr/540102/ (accessed February 15, 2022).
Fu, D., Xiao, M., Hayward, A., Fu, Y., Liu, G., Jiang, G., et al. (2014). Utilization of crop heterosis: a review. Euphytica 197, 161–173. doi: 10.1007/s10681-014-1103-7
Gagliardi, S., Avelino, J., Virginio Filho, E. D. M., and Isaac, M. E. (2021). Shade tree traits and microclimate modifications: implications for pathogen management in biodiverse coffee agroforests. Biotropica 53, 1356–1367. doi: 10.1111/btp.12984
Gebru, B. M., Wang, S. W., Kim, S. J., and Lee, W. K. (2019). Socio-ecological niche and factors affecting agroforestry practice adoption in different agroecologies of southern Tigray, Ethiopia. Sustainability 11, 3729. doi: 10.3390/su11133729
Geneti, D. (2019). Review on genetic, environment and their interaction (G x E) for stability of coffee quality. J. Biol. Agr. Healthc. 9, 23–28. doi: 10.7176/jbah/9-21-04
Georget, F., Courtel, P., Garcia, E. M., Hidalgo, M., Alpizar, E., Breitler, J.-C., et al. (2017). Somatic embryogenesis-derived coffee plantlets can be efficiently propagated by horticultural rooted mini-cuttings: a boost for somatic embryogenesis. Sci. Hortic. 216, 177–185. doi: 10.1016/j.scienta.2016.12.017
Georget, F., Marie, L., Alpizar, E., Courtel, P., Bordeaux, M., Hidalgo, J. M., et al. (2019). Starmaya: the first Arabica F1 coffee hybrid produced using genetic male sterility. Front. Plant Sci. 10, 1344. doi: 10.3389/fpls.2019.01344
Geromel, C., Ferreira, L. P., Davrieux, F., Guyot, B., Ribeyre, F., Scholz, M. B. S., et al. (2008). Effects of shade on the development and sugar metabolism of coffee (Coffea arabica L.) fruits. Plant Physiol. Biochem. 46, 569–579. doi: 10.1016/j.plaphy.2008.02.006
Geromel, C., Ferreira, L. P., Guerreiro, S. M. C., Cavalari, A. A., Pot, D., Pereira, L. F., et al. (2006). Biochemical and genomic analysis of sucrose metabolism during coffee (Coffea arabica) fruit development. J. Exp. Bot. 57, 3243–3258. doi: 10.1093/jxb/erl084
Ghini, R., Torre-Neto, A., Dentzien, A. F. M., Guerreiro-Filho, O., Lost, R., Patrício, F. R. A., et al. (2015). Coffee growth, pest and yield responses to free-air CO2 enrichment. Clim. Change 132, 307–320. doi: 10.1007/s10584-015-1422-2
Gichuru, E., Alwora, G., Gimase, J., and Kathurima, C. (2021). Coffee leaf rust (Hemileia vastatrix) in Kenya - a review. Agronomy 11, 2590. doi: 10.3390/agronomy11122590
Gomes, L. C., Bianchi, F. J. J. A., Cardoso, I. M., Fernandes, R. B. A., Fernandes Filho, E. I., and Schulte, R. P. O. (2020). Agroforestry systems can mitigate the impacts of climate change on coffee production: a spatially explicit assessment in Brazil. Agr. Ecosyst. Environ. 294, 106858. doi: 10.1016/j.agee.2020.106858
Gordon, C., Manson, R., Sundberg, J., and Cruz-Angón, A. (2007). Biodiversity, profitability, and vegetation structure in a Mexican coffee agroecosystem. Agr. Ecosyst. Environ. 118, 256–266. doi: 10.1016/j.agee.2006.05.023
Graham, S., Ihli, H. J., and Gassner, A. (2021). Agroforestry, indigenous tree cover and biodiversity conservation: a case study of Mount Elgon in Uganda. Eur. J. Dev. Res. 1–19. doi: 10.1057/s41287-021-00446-5
Granados-Montero, M., Avelino, J., Arauz-Cavallini, F., Castro-Tanzi, S., and Ureña, N. (2020). Leaf litter and Mycena citricolor inoculum on the American leaf spot epidemic. Agr. Mesoam. 31, 77–94. doi: 10.15517/am.v31i1.36614
Grosch, W. (2001). Volatile compounds,” in Coffee: Recent Developments, eds R. J. Clarke and O. G. Vitzthum (Oxford: Blackwell Science), 68–89. doi: 10.1002/9780470690499.ch3
Grüter, R., Trachsel, T., Laube, P., and Jaisli, I. (2022). Expected global suitability of coffee, cashew and avocado due to climate change. PLoS ONE 17, e0261976. doi: 10.1371/journal.pone.0261976
Guillemot, J., Le Maire, G., Munishamappa, M., Charbonnier, F., and Vaast, P. (2018). Native coffee agroforestry in the Western Ghats of India maintains higher carbon storage and tree diversity compared to exotic agroforestry. Agr. Ecosyst. Environ. 265, 461–469. doi: 10.1016/j.agee.2018.06.002
Guyot, B., Gueule, D., Manez, J.-C., Perriot, J.-J., Giron, J., and Villain, L. (1996). Influence de l'altitude et de l'ombrage sur la qualité des cafés Arabica. Plant. Rech. Dév. 3, 272–283.
Haggar, J., Barrios, M., Bolaños, M., Merlo, M., Moraga, P., Munguia, R., et al. (2011). Coffee agroecosystem performance under full sun, shade, conventional and organic management regimes in Central America. Agroforest. Syst. 82, 285–301. doi: 10.1007/s10457-011-9392-5
Hajian-Forooshani, Z., Vandermeer, J., and Perfecto, I. (2020). Insights from excrement: invasive gastropods shift diet to consume the coffee leaf rust and its mycoparasite. Ecology 101, e02966. doi: 10.1002/ecy.2966
Hallé, N., and Faria, M. T. (1973). Le Coffea racemosa lour. Agronomia Moçambicana Lourenço Marques 7, 243–250.
Hameed, A., Hussain, S. A., and Suleria, H. A. R. (2020). “Coffee Bean-Related' Agroecological factors affecting the coffee,” in Co-Evolution of Secondary Metabolites. Reference Series in Phytochemistry, eds J. M. Mérillon and K. Ramawat (Cham: Springer), 645–650. doi: 10.1007/978-3-319-96397-6_21
Harvey, C. A., Pritts, A. A., Zwetsloot, M. J., Jansen, K., Pulleman, M. M., Armbrecht, I., et al. (2021). Transformation of coffee-growing landscapes across Latin America. A review. Agron. Sustain. Dev. 41, 62. doi: 10.1007/s13593-021-00712-0
Heindorf, C., Reyes-Agüero, J. A., Fortanelli-Martínez, J., and van't Hooft, A. (2021). More than maize, bananas, and coffee: the inter–and intraspecific diversity of edible plants of the Huastec mayan landscape mosaics in Mexico. Econom. Bot. 75, 158–174. doi: 10.1007/s12231-021-09520-9
Hernández Guerra, O. R. (1995). Yield and financial analysis of an agroforestry system with coffee (Coffea arabica cv caturra) and poro (Erythrina poeppigiana) under different densities of laurel (Cordia alliodora) (No. Thesis H557re), CATIE, Turrialba, Costa Rica. Available online at: http://www.sidalc.net/cgi-bin/wxis.exe/?IsisScript=orton.xisandmethod=postandformato=2andcantidad=1andexpresion=mfn=060124 (accessed February 15, 2022).
Homma, S. (2001). “Non-volatile compounds, part II,” in Coffee: Recent Developments, eds R. J. Clarke and O. G. Vitzthum (Oxford: Blackwell Science), 50–67. doi: 10.1002/9780470690499.ch2
Jácome, M. G. O., Mantovani, J. R., Silva, A. B. D., Rezende, T. T., and Landgraf, P. R. C. (2020). Soil attributes and coffee yield in an agroforestry system. Coffee Sci. 15, e151676. doi: 10.25186/.v15i.1676
Janzen, S. O. (2010). Comprehensive Natural Products II. Chemistry and Biology Chemistry of Coffee. Amsterdam: Elsevier Applied Science. doi: 10.1021/ja105512f
Jaramillo, J., Setamou, M., Muchugu, E., Chabi-Olaye, A., Jaramillo, A., Mukabana, J., et al. (2013). Climate change or urbanization? Impacts on a traditional coffee production system in East Africa over the last 80 years. PLoS ONE 8, e51815. doi: 10.1371/journal.pone.0051815
Jaramillo-Botero, C., Santos, R. H. S., Martinez, H. E. P., Cecon, P. R., and Fardin, M. P. (2010). Production and vegetative growth of coffee trees under fertilization and shade levels. Sci. Agr. 67, 639–645. doi: 10.1590/s0103-90162010000600004
Javier Lopez-Garcia, F., Escamilla-Prado, E., Zamarripa-Colmenero, A., and Guillermo Cruz-Castillo, J. (2016). Yield and quality of coffee cultivars (Coffea arabica L.) in Veracruz, Mexico. Rev. Fitotec. Mex. 39, 297–304. doi: 10.35196/rfm.2016.3.297-304
Jayakumar, M., Rajavel, M., Surendran, U., Gopinath, G., and Ramamoorthy, K. (2017). Impact of climate variability on coffee yield in India—with a micro-level case study using long-term coffee yield data of humid tropical Kerala. Clim. Change 145, 335–349. doi: 10.1007/s10584-017-2101-2
Jemal, O. M., Callo-Concha, D., and van Noordwijk, M. (2021). Coffee agroforestry and the food and nutrition security of small farmers of south-western Ethiopia. Front. Sustain. Food Syst. 5, 608868. doi: 10.3389/fsufs.2021.608868
Jezeer, R. E., Santos, M. J., Verweij, P. A., Boot, R. G., and Clough, Y. (2019). Benefits for multiple ecosystem services in Peruvian coffee agroforestry systems without reducing yield. Ecosyst. Serv. 40, 101033. doi: 10.1016/j.ecoser.2019.101033
Jezeer, R. E., Verweij, P. A., Santos, M. J., and Boot, R. G. (2017). Shaded coffee and cocoa–double dividend for biodiversity and small-scale farmers. Ecol. Econ. 140, 136–145. doi: 10.1016/j.ecolecon.2017.04.019
Jha, S., Bacon, C. M., Philpott, S. M., Ernesto Méndez, V., Läderach, P., and Rice, R. A. (2014). Shade coffee: update on a disappearing refuge for biodiversity. BioScience 64, 416–428. doi: 10.1093/biosci/biu038
Joët, T., Laffargue, A., Salmona, J., Doulbeau, S., Descroix, F., Bertrand, B., et al. (2009). Metabolic pathways in tropical dicotyledonous albuminous seeds: Coffea arabica as a case study. New Phytol. 182, 146–162. doi: 10.1111/j.1469-8137.2008.02742.x
Joët, T., Salmona, J., Laffargue, A., Descroix, F., and Dussert, S. (2010). Use of the growing environment as a source of variation to identify the quantitative trait transcripts and modules of co-expressed genes that determine chlorogenic acid accumulation. Plant Cell Environ. 33, 1220–1233. doi: 10.1111/j.1365-3040.2010.02141.x
Johnson, M. D., Kellermann, J. L., and Stercho, A. M. (2010). Pest reduction services by birds in shade and sun coffee in Jamaica. Anim. Conserv. 13, 140–147.doi: 10.1111/j.1469-1795.2009.00310.x
Karp, D. S., Mendenhall, C. D., Sandí, R. F., Chaumont, N., Ehrlich, P. R., Hadly, E. A., et al. (2013). Forest bolsters bird abundance, pest control and coffee yield. Ecol. Lett. 16, 1339–1347. doi: 10.1111/ele.12173
Kath, J., Byrareddy, V. M., Craparo, A., Nguyen-Huy, T., Mushtaq, S., Cao, L., et al. (2020). Not so robust: Robusta coffee production is highly sensitive to temperature. Glob. Change Biol. 26, 3677–3688. doi: 10.1111/gcb.15097
Kellermann, J. L., Johnson, M. D., Stercho, A. M., and Hackett, S. C. (2008). Ecological and economic services provided by birds on Jamaican Blue Mountain coffee farms. Conserv. Biol. 22, 1177–1185. doi: 10.1111/j.1523-1739.2008.00968.x
Koh, I., Garrett, R., Janetos, A., and Mueller, N. D. (2020). Climate risks to Brazilian coffee production. Environ. Res. Lett. 15, 104015. doi: 10.1088/1748-9326/aba471
Lamond, G., Sandbrook, L., Gassner, A., and Sinclair, F. L. (2016). Local knowledge of tree attributes underpins species selection on coffee farms. Exp. Agric. 55, 35–49. doi: 10.1017/s0014479716000168
Lashermes, P., Bertrand, B., and Etienne, H. (2009). “Breeding coffee (Coffea arabica) for sustainable production,” in Breeding Plantation Tree Crops: Tropical Species, eds S. M. Jain and P. M. Priyadarshan (New York, NY: Springer), 525–543. doi: 10.1007/978-0-387-71201-7_14
Lee, D. W., Baskaran, K., Mansor, M., Mohamad, H., and Yap, S. K. (1996). Irradiance and spectral quality affect Asian tropical rain forest tree seedling development. Ecology 77, 568–580. doi: 10.2307/2265631
Leroy, T., Ribeyre, F., Bertrand, B., Charmetant, P., Dufour, M., Montagnon, C., et al. (2006). Genetics of coffee quality. Braz. J. Plant Physiol. 18, 229–242. doi: 10.1590/s1677-04202006000100016
Liebig, T., Jassogne, L., Rahn, E., Läderach, P., Poehling, H. M., Kucel, P., et al. (2016). Towards a collaborative research: a case study on linking science to farmers' perceptions and knowledge on Arabica coffee pests and diseases and its management. PLoS ONE 11, e0159392. doi: 10.1371/journal.pone.0159392
Liebig, T., Ribeyre, F., Läderach, P., Poehling, H. M., van Asten, P., and Avelino, J. (2019). Interactive effects of altitude, microclimate and shading system on coffee leaf rust. J. Plant Inter. 14, 407–415. doi: 10.1080/17429145.2019.1643934
Lin, B. B. (2007). Agroforestry management as an adaptive strategy against potential microclimate extremes in coffee agriculture. Agr. Forest Meteor 144, 85–94. doi: 10.1016/j.agrformet.2006.12.009
Lin, B. B. (2010). The role of agroforestry in reducing water loss through soil evaporation and crop transpiration in coffee agroecosystems. Agr. For. Meteor. 150, 510–518. doi: 10.1016/j.agrformet.2009.11.010
Lin, B. B., Perfecto, I., and Vandermeer, J. (2008). Synergies between agricultural intensification and climate change could create surprising vulnerabilities for crops. Bioscience 58, 847–854. doi: 10.1641/B580911
Machado Filho, J. A., Rodrigues, W. P., Baroni, D. F., Pireda, S., Campbell, G., Souza, G. A. R., et al. (2021). Linking root and stem hydraulic traits to leaf physiological parameters in Coffea canephora clones with contrasting drought tolerance. J. Plant Physiol. 258–259, 153355. doi: 10.1016/j.jplph.2020.153355
Magrach, A., and Ghazoul, J. (2015). Climate and pest-driven geographic shifts in global coffee production: Implications for forest cover, biodiversity and carbon storage. PLoS ONE 10, e0133071. doi: 10.1371/journal.pone.0133071
Marçal, D. M. S., Avila, R. T., Quiroga-Rojas, L. F., de Souza, R. P. B., Gomes Junior, C. C., Ponte, L. R., et al. (2021). Elevated [CO2] benefits coffee growth and photosynthetic performance regardless of light availability. Plant Physiol. Biochem. 158, 524–535. doi: 10.1016/j.plaphy.2020.11.042
Marcheafave, G. G., Pauli, E. D., Tormena, C. D., Ortiz, M. C. V., de Almeida, A. G., Rakocevic, M., et al. (2020). Factorial design fingerprint discrimination of Coffea arabica beans under elevated carbon dioxide and limited water conditions. Talanta 209, 120591. doi: 10.1016/j.talanta.2019.120591
Marie, L., Abdallah, C., Campa, C., Courtel, P., Bordeaux, M., Navarini, L., et al. (2020). G × E interactions on yield and quality in Coffea arabica: new F1 hybrids outperform American cultivars. Euphytica 216, 78. doi: 10.1007/s10681-020-02608-8
Marques, I., Fernandes, I., Paulo, O. S., Lidon, F. C., DaMatta, F. M., Ramalho, J. C., et al. (2021). A transcriptomic approach to understanding the combined impacts of supra-optimal temperatures and CO2 revealed different responses in the polyploid Coffea arabica and its diploid progenitor C. canephora. Int. J. Mol. Sci. 22, 3125. doi: 10.3390/ijms22063125
Marraccini, P. (2020). “Gene expression in coffee,” in Progress in Botany, Vol. 42, eds F. M. Cánovas, U. Lüttge, M. C. Risueño, and H. Pretzsch (Springer: Cham), 43–111. doi: 10.1007/124_2020_42
Martins, L. D., Tomaz, M. A., Lidon, F. C., DaMatta, F. M., and Ramalho, J. C. (2014). Combined effects of elevated [CO2] and high temperature on leaf mineral balance in Coffea spp. plants. Clim. Change 126, 365–379. doi: 10.1007/s10584-014-1236-7
Martins, M. Q., Rodrigues, W. P., Fortunato, A. S., Leitão, A. E., Rodrigues, A. P., Pais, I. P., et al. (2016). Protective response mechanisms to heat stress in interaction with high [CO2] conditions in Coffea spp. Front. Plant Sci. 7, 947. doi: 10.3389/fpls.2016.00947
Martins, S. C. V., Detmann, K. C., Reis, J. V. D., Pereira, L. F., Sanglard, L. M. V. P., Rogalski, M., et al. (2013). Photosynthetic induction and activity of enzymes related to carbon metabolism: insights into the varying net photosynthesis rates of coffee sun and shade leaves. Theor. Exp. Plant Physiol. 25, 62–69. doi: 10.1590/s2197-00252013000100008
Mazzafera, P. (1999). Chemical composition of defective coffee beans. Food Chem. 64, 547–554. doi: 10.1016/S0308-8146(98)00167-8
Mbebi, A. J., Tong, H., and Nikoloski, Z. (2021). L2,1-norm regularized multivariate regression model with applications to genomic prediction. Bioinformatics 37, 2896–2904. doi: 10.1093/bioinformatics/btab212
Méndez, V. E., Shapiro, E. N., and Gilbert, G. S. (2009). Cooperative management and its effects on shade tree diversity, soil properties and ecosystem services of coffee plantations in western El Salvador. Agroforest. Syst. 76, 111–126. doi: 10.1007/s10457-009-9220-3
Merle, I., Pico, J., Granados, E., Boudrot, A., Tixier, P., Virginio Filho, E. D. M., et al. (2020). Unraveling the complexity of coffee leaf rust behavior and development in different Coffea arabica agroecosystems. Phytopathol. 110, 418–427. doi: 10.1094/phyto-03-19-0094-r
Merle, I., Villarreyna-Acuña, R., Ribeyre, F., Roupsard, O., Cilas, C., and Avelino, J. (2022). Microclimate estimation under different coffee-based agroforestry systems using full-sun weather data and shade tree characteristics. Eur. J. Agr. 132, 126396. doi: 10.1016/j.eja.2021.126396
Moat, J., Williams, J., Baena, S., Wilkinson, T., Gole, T. W., Challa, Z. K., et al. (2017). Resilience potential of the Ethiopian coffee sector under climate change. Nat. Plants 3, 17081. doi: 10.1038/nplants.2017.81
Moguel, P., and Toledo, V. M. (1999). Biodiversity conservation in traditional coffee systems of Mexico. Conserv. Biol. 13, 11–21. doi: 10.1046/j.1523-1739.1999.97153.x
Montagnon, C., Guyot, B., Cilas, C., and Leroy, T. (1998). Genetic parameters of several biochemical compounds from green coffee, Coffea canephora. Plant Breed. 117, 576–578. doi: 10.1111/j.1439-0523.1998.tb02211.x
Montagnon, C., Marraccini, P., and Bertrand, B. (2012). “Breeding for coffee quality,” in Specialty Coffee: Managing Quality, eds T. Oberthür, P. Läderach, H. A. J. Pohlan, and J. Cock (Penang: IPNI), 93–122.
Morais, H., Caramori, P. H., Ribeiro, A. M. D. A., Gomes, J. C., and Koguishi, M. S. (2006). Microclimatic characterization and productivity of coffee plants grown under shade of pigeon pea in Southern Brazil. Pesqui. Agropecu. Bras. 41, 763–770. doi: 10.1590/s0100-204x2006000500007
Moschetto, D., Montagnon, C., Guyot, B., Perriot, J.-J., Leroy, T., and Eskes, A. (1996). Studies on the effect of genotype on cup quality of Coffea canephora. Trop. Sci. 36, 18–31.
Muñoz-Villers, L. E., Geris, J., Alvarado-Barrientos, M. S., Holwerda, F., and Dawson, T. (2020). Coffee and shade trees show complementary use of soil water in a traditional agroforestry ecosystem. Hydr. Earth Syst. Sci. 24, 1649–1668. doi: 10.5194/hess-24-1649-2020
Muschler, R. G. (2001). Shade improves coffee quality in a sub-optimal coffee-zone of Costa Rica. Agroforest. Syst. 51, 131–139. doi: 10.1023/A:1010603320653
Muschler, R. G. (2004). “Shade management and its effect on coffee growth and quality,” in Coffee: Growing, Processing, Sustainable Production, eds J. N. Wintgens (Weinheim: WILEY-VCH Verlag GmbH Co. KGaA), 391–418. doi: 10.1002/9783527619627.ch14
Nesper, M., Kueffer, C., Krishnan, S., Kushalappa, C. G., and Ghazoul, J. (2017). Shade tree diversity enhances coffee production and quality in agroforestry systems in the Western Ghats. Agric. Ecosyst. Environ. 247, 172–181. doi: 10.1016/j.agee.2017.06.024
Nesper, M., Kueffer, C., Krishnan, S., Kushalappa, C. G., and Ghazoul, J. (2018). Simplification of shade tree diversity reduces nutrient cycling resilience in coffee agroforestry. J. Appl. Ecol. 56, 119–131. doi: 10.1111/1365-2664.13176
Nestel, D., and Altieri, M. A. (1992). The weed community of Mexican coffee agroecosystems: effect of management upon plant biomass and species composition. Acta Ocol. 13, 715–726.
Odeny, D. A., Chemining'wa, G. N., and Shibairo, S. I. (2014). “Beverage quality and biochemical components of shaded coffee,” in 25th International Conference on Coffee Science, Association for Science and Information on Coffee (ASIC) 2014 (Brooklyn, NY). doi: 10.19026/ajfst.12.3063
Oliosi, G., Giles, J. A. D., Rodrigues, W. P., Ramalho, J. C., and Partelli, F. L. (2016). Microclimate and development of Coffea canephora cv. Conilon under different shading levels promoted by Australian cedar (Toona ciliata M. Roem. var. Australis). Austr. J. Crop Sci. 10, 528–538. doi: 10.21475/ajcs.2016.10.04.p7295x
Padovan, M. D. P., Brook, R. M., Barrios, M., Cruz-Castillo, J. B., Vilchez-Mendoza, S. J., Costa, A. N., et al. (2018). Water loss by transpiration and soil evaporation in coffee shaded by Tabebuia rosea Bertol. and Simarouba glauca dc. compared to unshaded coffee in sub-optimal environmental conditions. Agr. For. Meteor. 248, 1–14. doi: 10.1016/j.agrformet.2017.08.036
Padovan, M. D. P., Cortez, V. J., Navarrete, L. F., Navarrete, E. D., Deffner, A. C., Centeno, L. G., et al. (2015). Root distribution and water use in coffee shaded with Tabebuia rosea Bertol. and Simarouba glauca DC. compared to full sun coffee in sub-optimal environmental conditions. Agroforest. Syst. 89, 857–868. doi: 10.1007/s10457-015-9820-z
Partelli, F. L., Araújo, A. V., Vieira, H. D., Dias, J. R. M., Menezes, L. F. T. D., and Ramalho, J. C. (2014). Microclimate and development of 'Conilon' coffee intercropped with rubber trees. Pesqui. Agropecu. Bras. 49, 872–881. doi: 10.1590/s0100-204x2014001100006
Perfecto, I., Hajian-Forooshani, Z., White, A., and Vandermeer, J. (2021). Ecological complexity and contingency: ants and lizards affect biological control of the coffee leaf miner in Puerto Rico. Agr. Ecosyst. Environ. 305, 107104. doi: 10.1016/j.agee.2020.107104
Perfecto, I., Rice, R. A., Greenberg, R., and Van der Voort, M. E. (1996). Shade coffee: a disappearing refuge for biodiversity: shade coffee plantations can contain as much biodiversity as forest habitats. BioScience 46, 598–608. doi: 10.2307/1312989
Perfecto, I., Vandermeer, J., Mas, A., and Pinto, L. S. (2005). Biodiversity, yield, and shade coffee certification. Ecol. Econom. 54, 435–446. doi: 10.1016/j.ecolecon.2004.10.009
Perfecto, I., Vandermeer, J., and Philpott, S. M. (2014). Complex ecological interactions in the coffee agroecosystem. Ann. Rev. Ecol. Evol. Syst. 45, 137–158. doi: 10.1146/annurev-ecolsys-120213-091923
Pham, Y., Reardon-Smith, K., Mushtaq, S., and Cockfield, G. (2019). The impact of climate change and variability on coffee production: a systematic review. Clim. Change 156, 609–630. doi: 10.1007/s10584-019-02538-y
Piato, K., Lefort, F., Subía, C., Caicedo, C., Calderón, D., Pico, J., et al. (2020). Effects of shade trees on robusta coffee growth, yield and quality. A meta-analysis. Agron. Sustain. Dev. 40, 38. doi: 10.1007/s13593-020-00642-3
Pilati, A. (2005). Evaluación de tres diferentes tipologías de manejo agronómico, sobre la estructura de crecimiento, de productividad y calidad del café (Coffea arabica L.), en la zona del Pacífico Sur de Nicaragua (Doctoral dissertation), Universidad Nacional Agraria, UNA. Available online at: http://repositorio.una.edu.ni/id/eprint/1983 (accessed February 15, 2022).
Privat, I., Foucrier, S., Prins, A., Epalle, T., Eychenne, M., Kandalaft, L., et al. (2008). Differential regulation of grain sucrose accumulation and metabolism in Coffea arabica (Arabica) and Coffea canephora (Robusta) revealed through gene expression and enzyme activity analysis. New Phytol. 178, 781–797. doi: 10.1111/j.1469-8137.2008.02425.x
Rahn, E., Vaast, P., Läderach, P., van Asten, P., Jassogne, L., and Ghazoul, J. (2018). Exploring adaptation strategies of coffee production to climate change using a process-based model. Ecol. Model. 371, 76–89. doi: 10.1016/j.ecolmodel.2018.01.009
Rakocevic, M., Batista, E. R., Pazianotto, R. A. A., Scholz, M. B. S., Souza, G. A. R., Campostrini, E., et al. (2021). Leaf gas exchange and bean quality fluctuations over the whole canopy vertical profile of Arabic coffee cultivated under elevated CO2. Funct. Plant Biol. 48, 469–482. doi: 10.1071/FP20298
Rakocevic, M., Braga, K. S. M., Batista, E. R., Maia, A. H. N., Scholz, M. B. S., and Filizola, H. F. (2020). The vegetative growth assists to reproductive responses of Arabic coffee trees in a long-term FACE experiment. Plant Growth Regul. 91, 305–316. doi: 10.1007/s10725-020-00607-2
Ramalho, J. C., Campos, P. S., Teixeira, M., and Nunes, M. A. (1998). Nitrogen dependent changes in antioxidant system and in fatty acid composition of chloroplast membranes from Coffea arabica L. plants submitted to high irradiance. Plant Sci. 135, 115–124. doi: 10.1016/s0168-9452(98)00073-9
Ramalho, J. C., DaMatta, F. M., Rodrigues, A. P., Scotti-Campos, P., Pais, I., Batista-Santos, P., et al. (2014). Cold impact and acclimation response of Coffea spp. plants. Theor. Exp. Plant Physiol. 26, 5–18. doi: 10.1007/s40626-014-0001-7
Ramalho, J. C., Pais, I. P., Leitão, A. E., Guerra, M., Reboredo, F. H., Máguas, C. M., et al. (2018). Can elevated air [CO2] conditions mitigate the predicted warming impact on the quality of coffee bean? Front. Plant Sci. 9, 287. doi: 10.3389/fpls.2018.00287
Ramalho, J. C., Pons, T. L., Groeneveld, H. W., Azinheira, H. G., and Nunes, M. A. (2000). Photosynthetic acclimation to high light conditions in mature leaves of Coffea arabica L.: role of xanthophylls, quenching mechanisms and nitrogen nutrition. Funct. Plant Biol. 27, 43–51. doi: 10.1071/pp99013
Ramalho, J. C., Pons, T. L., Groeneveld, H. W., and Nunes, M. A. (1997). Photosynthetic responses of Coffea arabica leaves to a short-term high light exposure in relation to N availability. Physiol. Plant. 101, 229–239. doi: 10.1034/j.1399-3054.1997.1010130.x
Ramalho, J. C., Rodrigues, A. P., Semedo, J. N., Pais, I. P., Martins, L. D., Simões-Costa, M. C., et al. (2013). Sustained photosynthetic performance of Coffea spp. under long-term enhanced [CO2]. PLoS ONE 8, e82712. doi: 10.1371/journal.pone.0082712
Ratnadass, A., Fernandes, P., Avelino, J., and Habib, R. (2012). Plant species diversity for sustainable management of crop pests and diseases in agroecosystems: a review. Agron. Sustain. Dev. 32, 273–303. doi: 10.1007/s13593-011-0022-4
Rezende, M. Q., Venzon, M., Perez, A. L., Cardoso, I. M., and Janssen, A. (2014). Extrafloral nectaries of associated trees can enhance natural pest control. Agr. Ecosyst. Environ 188, 198–203. doi: 10.1016/j.agee.2014.02.024
Rice, R. A. (2008). Agricultural intensification within agroforestry: the case of coffee and wood products. Agr. Ecosyst. Environ. 128, 212–218. doi: 10.1016/j.agee.2008.06.007
Rice, R. A. (2011). Fruits from shade trees in coffee: how important are they? Agroforest. Syst. 83, 41–49. doi: 10.1007/s10457-011-9385-4
Rice, R. A. (2018). Coffee in the crosshairs of climate change: agroforestry as abatis. Agroecol. Sustain. Food Syst. 42, 1058–1076. doi: 10.1080/21683565.2018.1476428
Rigal, C., Vaast, P., and Xu, J. (2018). Using farmers' local knowledge of tree provision of ecosystem services to strengthen the emergence of coffee-agroforestry landscapes in southwest China. PLoS ONE 13, e0204046. doi: 10.1371/journal.pone.0204046
Rigal, C., Xu, J., Hu, G., Qiu, M., and Vaast, P. (2020b). Coffee production during the transition period from monoculture to agroforestry systems in near optimal growing conditions, in Yunnan Province. Agr. Syst. 177, 102696. doi: 10.1016/j.agsy.2019.102696
Rigal, C., Xu, J., and Vaast, P. (2020a). Young shade trees improve soil quality in intensively managed coffee systems recently converted to agroforestry in Yunnan Province, China. Plant Soil 453, 119–137. doi: 10.1007/s11104-019-04004-1
Righi, C. A., Campoe, O. C., Bernardes, M. S., Lunz, A. M. P., Piedade, S. M. S., and Pereira, C. R. (2013). Influence of rubber trees on leaf-miner damage to coffee plants in an agroforestry system. Agroforest. Syst. 87, 1351–1362. doi: 10.1007/s10457-013-9642-9
Robbins, P., Tripuraneni, V., Karanth, K. K., and Chhatre, A. (2021). Coffee, trees, and labor: political economy of biodiversity in commodity agroforests. Ann. Amer. Ass. Geogr. 111, 1046–1061. doi: 10.1080/24694452.2020.1803726
Rodrigues, A. M., Jorge, T., Osorio, S., Pott, D. M., Lidon, F. C., DaMatta, F. M., et al. (2021). Primary metabolite profile changes in Coffea spp. promoted by single and combined exposure to drought and elevated CO2 concentration. Metabolites 11, 427. doi: 10.3390/metabo11070427
Rodrigues, W. P., Martins, M. Q., Fortunato, A. S., Rodrigues, A. P., Semedo, J. N., Simões-Costa, M. C., et al. (2016). Long-term elevated air [CO2] strengthens photosynthetic functioning and mitigates the impact of supra-optimal temperatures in tropical Coffea arabica and Coffea canephora species. Glob. Change Biol. 22, 415–431. doi: 10.1111/gcb.13088
Ruiz-García, P., Conde-Álvarez, C., Gómez-Díaz, J. D., and Monterroso-Rivas, A. I. (2021). Projections of local knowledge-based adaptation strategies of Mexican coffee farmers. Climate 9, 60. doi: 10.3390/cli9040060
Sanz-Uribe, J. R., Yusianto Menon, S. N., Peñuela, A., Oliveros, C., Husson, J., et al. (2017). “Postharvest processing- revealing the green bean,” in The Craft and Science of Coffee, B. Folmer (Elsevier), 51–76. doi: 10.1016/b978-0-12-803520-7.00003-7
Sarmiento-Soler, A., Vaast, P., Hoffmann, M. P., Rötter, R. P., Jassogne, L., Van Asten, P. J., et al. (2019). Water use of Coffea arabica in open versus shaded systems under smallholder's farm conditions in Eastern Uganda. Agr. Forest Meteorol. 266, 231–242. doi: 10.1016/j.agrformet.2018.12.006
Scalabrin, S., Toniutti, L., Di Gaspero, G., Scaglione, D., Magris, G., Vidotto, M., et al. (2020). A single polyploidization event at the origin of the tetraploid genome of Coffea arabica is responsible for the extremely low genetic variation in wild and cultivated germplasm. Sci. Rep. 10, 4642. doi: 10.1038/s41598-020-61216-7
Scotti-Campos, P., Pais, I. P., Ribeiro-Barros, A. I., Martins, L. D., Tomaz, M. A., Rodrigues, W. P., et al. (2019). Lipid profile adjustments may contribute to warming acclimation and to heat impact mitigation by elevated [CO2] in Coffea spp. Environ. Exp. Bot. 167, 103856. doi: 10.1016/j.envexpbot.2019.103856
Sebuliba, E., Majaliwa, J. G., Isubikalu, P., Turyahabwe, N., Eilu, G., and Ekwamu, A. (2021). Characteristics of shade trees used under Arabica coffee agroforestry systems in Mount Elgon Region, Eastern Uganda. Agroforest. Syst. 96, 65–77. doi: 10.1007/s10457-021-00688-6
Semedo, J. N., Rodrigues, A. P., Lidon, F. C., Pais, I. P., Marques, I., Gouveia, D., et al. (2021). Intrinsic non-stomatal resilience to drought of the photosynthetic apparatus in Coffea spp. can be strengthened by elevated air CO2. Tree Physiol. 41, 708–727. doi: 10.1093/treephys/tpaa158
Semedo, J. N., Rodrigues, W. P., Dubberstein, D., Martins, M. Q., Martins, L. D., Pais, I. P., et al. (2018). “Coffee responses to drought, warming and high [CO2] in a context of future climate change scenarios,” in Theory and Practice of Climate Adaptation (Cham: Springer), 465–477.
Siles, P., Harmand, J. M., and Vaast, P. (2010). Effects of Inga densiflora on the microclimate of coffee (Coffea arabica L.) and overall biomass under optimal growing conditions in Costa Rica. Agroforest. Syst. 78, 269–286. doi: 10.1007/s10457-009-9241-y
Somarriba, E., Harvey, C. A., Samper, M., Anthony, F., González, J., Staver, C., et al. (2004). “Biodiversity conservation in neotropical coffee (Coffea arabica) plantations,” in Agroforestry and Biodiversity Conservation in Tropical Landscapes (Washington, DC: Island Press), 198–226.
Somporn, C., Kamtuo, A., Theerakulpisut, P., and Siriamornpun, S. (2011). Effects of roasting degree on radical scavenging activity, phenolics and volatile compounds of Arabica coffee beans (Coffea arabica L. cv. Catimor). Int. J. Food Sci. 46, 2287–2296. doi: 10.1111/j.1365-2621.2011.02748.x
Somporn, C., Kamtuo, A., Theerakulpisut, P., and Siriamornpun, S. (2012). Effect of shading on yield, sugar content, phenolic acids and antioxidant property of coffee beans (Coffea arabica L. cv. Catimor) harvested from north-eastern Thailand. J. Sci. Food Agr. 92, 1956–1963. doi: 10.1002/jsfa.5568
Soto-Pinto, L., Perfecto, I., and Caballero-Nieto, J. (2002). Shade over coffee: its effects on berry borer, leaf rust and spontaneous herbs in Chiapas, Mexico. Agroforest. Syst. 55, 37–45. doi: 10.1023/A:1020266709570
Souza, H. N., Cardoso, I. M., Fernandes, J. M., Garcia, F. C. P., Bonfim, V. R., Santos, A. C., et al. (2010). Selection of native trees for intercropping with coffee in the Atlantic Rainforest biome. Agroforest. Syst. 80, 1–16. doi: 10.1007/s10457-010-9340-9
Staver, C., Guharay, F., Monterroso, D., and Muschler, R. G. (2001). Designing pest-suppressive multistrata perennial crop systems: shade-grown coffee in Central America. Agroforest. Syst. 53, 151–170. doi: 10.1023/a:1013372403359
Steiman, S., Idol, T., Bittenbender, H. C., and Gautz, L. (2011). Shade coffee in Hawaii–exploring some aspects of quality, growth, yield, and nutrition. Sci. Hortic. 128, 152–158. doi: 10.1016/j.scienta.2011.01.011
Tassew, A. A., Yadessa, G. B., Bote, A. D., and Obso, T. K. (2021). Influence of location, elevation gradients, processing methods, and soil quality on the physical and cup quality of coffee in the Kafa biosphere reserve of SW Ethiopia. Heliyon 7, e07790. doi: 10.1016/j.heliyon.2021.e07790
The Coffee Guide (2021). Available online at: https://www.thecoffeeguide.org/ (accessed February 3, 2022).
Thuy, P. T., Niem, L. D., Ho, T. M. H., Burny, P., and Lebailly, P. (2019). Economic analysis of perennial crop systems in Dak Lak province, Vietnam. Sustainability 11, 81. doi: 10.3390/su11010081
Thuy, P. T., Thuy, L. T. T., Kdam, T. H. N., Truong, P. V., Kdam, T. P. H. N., Dung, T. T., et al. (2021). Impacts of payment for forest ecosystem services in protecting forests in Dak Lak province, Vietnam. Forests 12, 1383. doi: 10.3390/f12101383
Tolessa, K., D'heer, J., Duchateau, L., and Boeckx, P. (2017). Influence of growing altitude, shade and harvest period on quality and biochemical composition of Ethiopian specialty coffee. J. Sci. Food Agr. 97, 2849–2857. doi: 10.1002/jsfa.8114
Toniutti, L., Breitler, J.-C., Etienne, H., Campa, C., Doulbeau, S., Urban, L., et al. (2017). Influence of environmental conditions and genetic background of arabica coffee (C. arabica L) on leaf rust (Hemileia vastatrix) pathogenesis. Front. Plant Sci. 8, 2025. doi: 10.3389/fpls.2017.02025
Toniutti, L., Breitler, J.-C., Guittin, C., Doulbeau, S., Etienne, H., Campa, C., et al. (2019). An altered circadian clock coupled with a higher photosynthesis efficiency could explain the better agronomic performance of a new coffee clone when compared with a standard variety. Int. J. Mol. Sci. 20, 736. doi: 10.3390/ijms20030736
Udawatta, R. P., Rankoth, L. M., and Jose, S. (2021). “Agroforestry for biodiversity conservation,” in Agroforestry and Ecosystem Services, eds R. P. Udawatta and S. Jose (Cham: Springer), 245–259. doi: 10.1007/978-3-030-80060-4_10
Vaast, P., Bertrand, B., Perriot, J.-J., Guyot, B., and Génard, M. (2006). Fruit thinning and shade improve bean characteristics and beverage quality of coffee (Coffea arabica L.) under optimal conditions. J. Sci. Food Agr. 86, 197–204. doi: 10.1002/jsfa.2338
Vaast, P., and Raghuramulu, Y. (2012). Coffee quality in coffee agro-forestry systems of Kodagu, western ghats of India. Ind. Coffee 76, 10–11.
Vaast, P., Raghuramulu, Y., and Menon, S. (2011). “Effects of shade tree composition on the coffee quality in agroforestry systems of the Kodagu district, South-Western India,” in Proceedings of the 23rd International Conference on Coffee Science (Bali), 971–976.
Vaast, P., Van Kanten, R., Siles, P., Dzib, B., Franck, N., Harmand, J. M., et al. (2005). “Shade: a key factor for coffee sustainability and quality,” in Colloque Scientifique International Sur Le Café (Nairobi: Association for Science and Information on Coffee (ASIC)). Available online at: https://agritrop.cirad.fr/529372/ (accessed February 15, 2022).
van der Vossen, H., Bertrand, B., and Charrier, A. (2015). Next generation variety development for sustainable production of arabica coffee (Coffea arabica L.): a review. Euphytica 204, 243–256. doi: 10.1007/s10681-015-1398-z
van der Wolf, J., Jassogne, L., Gram, G. I. L., and Vaast, P. (2016). Turning local knowledge on agroforestry into an online decision-support tool for tree selection in smallholders' farms. Exp. Agr. 55, 50–66. doi: 10.1017/s001447971600017x
van Kanten, R., and Vaast, P. (2006). Transpiration of arabica coffee and associated shade tree species in sub-optimal, low-altitude conditions of Costa Rica. Agroforest. Syst. 67, 187–202. doi: 10.1007/s10457-005-3744-y
Vanderbilt, V. C., and Grant, L. (1985). Plant canopy specular reflectance model. IEEE Tran. Geosci. Remote Sens. GE-23, 722–730. doi: 10.1109/tgrs.1985.289390
Vanderhaegen, K., Akoyi, K. T., Dekoninck, W., Jocqu,é, R., Muys, B., Verbist, B., et al. (2018). Do private coffee standards ‘walk the talk' in improving socio-economic and environmental sustainability? Glob. Environ. Change 51, 1–9. doi: 10.1016/j.gloenvcha.2018.04.014
Vasconcellos, E. (1906). “Exposição Colonial de Algodão, Borracha, Cacau e Café, in CATÁLOGO (Lisboa: Sociedade de Geografia de Lisboa), 104.
Venancio, L. P., do Amaral, J. F. T., Cavatte, P. C., Vargas, C. T., dos Reis, E. F., and Dias, J. R. (2019). Vegetative growth and yield of robusta coffee genotypes cultivated under different shading levels. Biosci. J. 35, 1490–1503. doi: 10.14393/bj-v35n5a2019-45039
Venzon, M. (2021). Agro-ecological management of coffee pests in Brazil. Front. Sustain. Food Syst. 5, 721117. doi: 10.3389/fsufs.2021.721117
Verburg, R., Rahn, E., Verweij, P., van Kuijk, M., and Ghazoul, J. (2019). An innovation perspective to climate change adaptation in coffee systems. Environ. Sci. Policy 97, 16–24. doi: 10.1016/j.envsci.2019.03.017
Villarreyna, R., Avelino, J., and Cerda, R. (2020). Ecosystem-based adaptation: effect of shade trees on ecosystem services in coffee plantations. Agron. Mesoamer. 31, 499–516. doi: 10.15517/am.v31i2.37591
Virginio Filho, E. D. M., Casanoves, F., Haggar, J., Staver, C., Soto, G., Avelino, J., et al. (2015). “La productividad útil, la materia orgánica y el suelo en los primeros 10 años de edad en sistemas de producción de café a pleno sol y bajo varios tipos de sombra y niveles de insumos orgánicos y convencionales en Costa Rica,” in Sistemas Agroforest, 131–159. Available online at: https://agris.fao.org/agris-search/search.do?recordID=FR2017102414 (accessed February 15, 2022).
Walyaro, D. J. A. (1983). Considerations in breeding for improved yield and quality in Arabica coffee (Coffea arabica L.) (Doctoral dissertation), Wageningen University, Wageningen, Netherlands.
Worku, M., De Meulenaer, B., Duchateau, L., and Boeckx, P. (2018). Effect of altitude on biochemical composition and quality of green arabica coffee beans can be affected by shade and postharvest processing method. Food Res. Int. 105, 278–285. doi: 10.1016/j.foodres.2017.11.016
Wu, L. (2016). Infestation and Management of the Black Coffee Twig Borer in Uganda: And the Potential Impact of the Leguminous Tree Albizia chinensis on Robusta Coffee. Uppsala: Independent project/Degree project / SLU, Department of Ecology.
Yamaguchi-Shinozaki, K., and Shinozaki, K. (1993). The plant hormone abscisic acid mediates the drought-induced expression but not the seed-specific expression of rd22, a gene responsive to dehydration stress in Arabidopsis thaliana. Mol. Gen. Genet. 238, 17–25. doi: 10.1007/bf00279525
Zaitunah, A., and Ahmad, A. G. (2021). “Analysis of agroforestry tree species composition and coffee cultivation production tropical highlands, Aceh Tengah-Indonesia,” in IOP Conference Series: Earth and Environmental Science, Vol. 886 (Makassar: IOP Publishing), 012048. doi: 10.1088/1755-1315/886/1/012048
Zaro, G. C., Caramori, P. H., Yada Junior, G. M., Sanquetta, C. R., Androcioli Filho, A., Nunes, A. L. P., et al. (2020). Carbon sequestration in an agroforestry system of coffee with rubber trees compared to open-grown coffee in southern Brazil. Agroforest. Syst. 94, 799–809. doi: 10.1007/s10457-019-00450-z
Keywords: agroforestry, bean quality, climate change, Coffea, crop management, hybrid, microclimate, shade
Citation: Koutouleas A, Sarzynski T, Bordeaux M, Bosselmann AS, Campa C, Etienne H, Turreira-García N, Rigal C, Vaast P, Ramalho JC, Marraccini P and Ræbild A (2022) Shaded-Coffee: A Nature-Based Strategy for Coffee Production Under Climate Change? A Review. Front. Sustain. Food Syst. 6:877476. doi: 10.3389/fsufs.2022.877476
Received: 16 February 2022; Accepted: 27 March 2022;
Published: 28 April 2022.
Edited by:
Paulo Mazzafera, State University of Campinas, BrazilReviewed by:
Priscila Coltri, University of Campinas, BrazilGoetz Schroth, Independent Researcher, Santarém, Brazil
Lenka Ehrenbergerová, Mendel University in Brno, Czechia
Copyright © 2022 Koutouleas, Sarzynski, Bordeaux, Bosselmann, Campa, Etienne, Turreira-García, Rigal, Vaast, Ramalho, Marraccini and Ræbild. This is an open-access article distributed under the terms of the Creative Commons Attribution License (CC BY). The use, distribution or reproduction in other forums is permitted, provided the original author(s) and the copyright owner(s) are credited and that the original publication in this journal is cited, in accordance with accepted academic practice. No use, distribution or reproduction is permitted which does not comply with these terms.
*Correspondence: Athina Koutouleas, YXRrQGlnbi5rdS5kaw==