- 1Horticulture and Product Physiology, Wageningen University and Research, Wageningen, Netherlands
- 2Bioresource Technology and Veterinary Department, Vocational College, Universitas Gadjah Mada, Yogyakarta, Indonesia
- 3Food & Biobased Research, Wageningen University and Research, Wageningen, Netherlands
Effect of cultivation temperature during the phase of flowering and fruit development on tomato quality was investigated. Plants of two dwarf tomato cultivars “Ponchi Re” and “Tarzan,” were subjected to three different growth temperatures: 16, 22, or 28◦C, starting at the flowering phase. Mature green fruit was harvested and subjected to shelf life at 20◦C for 20 days or first stored at 4◦C for 15 days, and then placed under shelf life conditions. Fruit quality was determined through red color development, soluble solid content (SSC), softening, weight loss, and cold tolerance. Higher cultivation temperature increased development and production of fruit. Deviation from the 22◦C growth temperature led to increased soluble solid content in both cultivars, and smaller fruit diameter in “Tarzan.” Fruit grown at lower temperature had delayed color development during shelf life, and this was further delayed by prior cold storage. “Tarzan” showed more chilling injury (CI) symptoms than “Ponchi Re.” In our experiment, SSC can be manipulated by modulating cultivation temperature, but that it is not associated with CI tolerance. Delayed color formation at the lowest growth temperature observed in “Ponchi Re” tomatoes could be resulted in lower lycopene levels leads to lower ROS scavenging capacity. For “Tarzan” tomatoes, higher firmness at harvest, less softening, and lower weight loss during cold storage in fruit from the lowest cultivation temperature might positively correlated with increased membrane integrity, resulting in increased CI tolerance. This indicates that CI incidence depends on growth temperature and is cultivar dependent in dwarf tomato fruit.
Introduction
Tomato quality is a complex trait, governed by numerous processes at the plant and fruit level, which depend on the interplay between cultural practices, genetic, and environmental factors (Farneti et al., 2013). Tomato fruit quality is determined by color, texture, flavor, and absence of shriveling (Gautier et al., 2008). Those quality attributes are represented by lycopene content, firmness, volatile composition, the sugar to acid ratio, and weight loss (Bertin and Génard, 2018). Riga et al. (2008), found tomato quality properties depend more on the cumulative temperature over the course of the last 45 days before harvest than on photosynthetically active radiation (PAR). Growth temperature may also affect chilling tolerance of tomato. Chilling injury (CI) symptoms in tomato fruit are mostly visible during shelf life after prior storage below a certain, non-freezing temperature (Crisosto et al., 1999; Lurie and Crisosto, 2005; Liu et al., 2012; Biswas et al., 2016). Examples of CI symptoms are pitting, shriveling, mealiness, uneven or delayed ripening, and water-soaked areas (Ferguson et al., 1999; Lurie and Crisosto, 2005; Biswas et al., 2016). These symptoms considerably lower fruit quality and reduce its market value (Albornoz et al., 2019).
Moderate stresses such as high or low temperature, shortage or excess of water, stresses related to the intensity and quality of light perceived during cultivation, trigger plants to react by initiating immediate protection against the stressor (Sabehat et al., 1998; Neta-Sharir, 2005). Exposing plants to sub-optimal growth temperatures between 10 and 20◦C during 7–10 days induced chilling tolerance in tomato plants (Barrero-Gil et al., 2016); watermelon (Lu et al., 2020); and sweet pepper (Ferguson et al., 1999). Acquired chilling tolerance in plants exposed to low growth temperature is contributed to expression of C-repeat-binding factors (CBF) genes (Singh et al., 2011). In addition, accumulation of small heat shock proteins (Sabehat et al., 1996, 1998; Luengwilai et al., 2012) and/or abscisic acid (Daie and Campbell, 1981; Wang and Buta, 1994; Chen and Li, 2002) may induce tolerance to low temperatures. Elevated growth temperatures, particularly close to or at harvest, may also induce tolerance to low temperatures in fruits. Cucumber fruit grown at elevated greenhouse temperatures (32◦C) showed improved tolerance to chilling compared to fruit grown at standard growing temperature (21–22◦C), due to maintenance of higher firmness and enhanced activity of antioxidant enzymes such as superoxide dismutase (SOD) and catalase (CAT) (Kang et al., 2002). Therefore, it seems that deviation from preferred cultivation temperatures increases CI tolerance.
Tomato has become a model crop for both quality and CI research (Campos et al., 2010). Dwarf tomato cultivars offer benefits for studying pre-harvest to post-harvest relations due its compact size, short live cycle, and uniformity of the harvested fruit in terms of age and size (Meissner et al., 1997; Malacrida et al., 2006; Luengwilai et al., 2012; Tao et al., 2014). Mature green (MG) tomatoes are considered more sensitive to CI than red (R) tomatoes (Biswas et al., 2016). CI symptoms in MG tomatoes may appear as complete color inhibition after storage >10 d (Hobson, 1981; Whitaker, 1991; Lurie and Sabehat, 1997). To test the effect of cultivation temperature on tomato quality attributes, two dwarf tomato genotypes were cultivated at three temperatures (16, 22, or 28◦C). Several quality properties such as size, soluble solid content (SSC), color, firmness, weight loss, and chilling tolerance of green harvested fruit were analyzed from the fruit of these plants during shelf life with and without prior cold storage. We show that low growth temperature induced cold tolerance in “Tarzan,” but we found the opposite in the “Ponchi Re.” The physiological mechanisms that might explain how growth temperature affects quality properties and chilling tolerance during cold storage and subsequent shelf life were discussed.
Materials and Methods
Greenhouse Climate Conditions
“Ponchi Re” and “Tarzan” dwarf tomato plants were grown from seeds bought from Prudac (Enkhuizen, The Netherlands) in a greenhouse compartment of Wageningen University and Research in Wageningen, The Netherlands. Seeds were sown on September 11th, 2017 in a greenhouse with a photoperiod of 16 h per day with temperature settings at 21◦C (day and night), and a relative humidity of 75%. When daylight was insufficient, additional artificial lighting (Philips 400 watt Son-T natrium lamps, Eindhoven, The Netherlands) was employed. Plants were watered manually and supported with a plastic peg for stability during fruiting. Fertilizer was applied according to commercial growth practices.
Climate Chamber Conditions and Fruit Harvest
When plants were fully flowering, all flowers were removed, and the temperature treatments were started. The plants were divided randomly and placed in three climate cabinets (HPS1500S, Weiss Technik, Germany) set to either 16, 22, and 28◦C. For each temperature treatment, a corresponding relative humidity was set as to ensure a constant vapor pressure difference of 0.8 kPa for all treatments (Table 1). Every week, the position of the plants within a chamber was randomized. MG tomatoes were harvested twice, 1 week apart. Forty five MG tomatoes per cultivar were selected in each harvest and transported within 10 min to the Horticulture and product physiology laboratory, Wageningen University for further storage. Per temperature/cultivar combination, six extra tomatoes were harvested for immediate destructive measurements. Tomatoes of the first harvest were stored first at 4◦C for 15 d and then placed at 20◦C for 20 days (shelf life), whereas tomatoes from the second harvest were stored immediately at shelf life condition (20◦C).
Soluble Solid Content
The SSC was measured using a digital refractometer (Atago refractometer PR-32α, Fukaya-Shi, Saitama, Japan). Six tomatoes were measured for each treatment. The tomatoes were cut in half. Using one half per tomato, the juice was squeezed out and used to determine the SSC (◦Brix) using the refractometer. The other half of the fruit was used for dry matter content measurement. The refractometer and the knife used for cutting were cleaned with distilled water before each individual measurement. Results were expressed in ◦ Brix.
Dry Matter Content
The remaining halves of tomatoes from SSC measurement were weighed after cutting (fresh weight), and oven-dried at 70◦C until constant weight (24–48 h). Fresh and dry weight (in g) were measured using a balance (Mettler PM 480, Mettler-Toledo, Leicester, UK) and subsequently expressed as the percentage fresh to dry weight (Kaiser et al., 2019).
Fruit Diameter
The fruit size was determined by measuring the diameter (in mm) of the tomato along the equator, using a digital caliper.
Color Measurement
Color was assessed non-destructively by a hand-held photodiode array spectrophotometer (Pigment Analyzer PA1101, CP, Germany). Remittance was assessed at 570 (R570) and 780 (R780) nm by calculating the normalized different vegetative index (NDVI, Equation 1) and normalized anthocyanin index (NAI, Equation 2) which are normalized value between −1 and 1 (Affandi et al., 2020).
The measurement head of the pigment analyser was adapted by adding a small plastic cup on top of the measurement head to collect light for color measurements of dwarf tomatoes.
Firmness
Firmness was measured using a Zwick Z2.5/TS1S materials testing machine (Ulm, Germany). A probe (diameter 0.8 cm) was placed on the skin of the tomato and compressed the tomato 0.5 mm with the maximum force recorded and regarded as tomato firmness (Schouten et al., 2007). Each tomato was measured twice, and the average was taken. Measurement were taken on the equator of the fruit. After the first measurement the tomato was turned 45◦ for the second measurement. Results were expressed in Newton.
CI Indices and Weight Loss
Each tomato was judged for the degree of CI using the chilling injury index (CII) (Vega-García et al., 2010). Three CI symptoms were measured; uneven ripening and color development (U), pitting (P), and decay (D). The severity of each symptom is based on the percentage of affected tissue (0 = No injury 1 = <10%, 2 = 11–25%, 3 = 26–40%, 4 = >40%) with the CII score calculated as CII = (U+P+D)/3. Tomato weight loss over time was expressed as the percentage weight loss compared to the initial weight (Affandi et al., 2020).
Data Analysis
One-way ANOVA was employed to analyse data measured at harvest whereas data obtained over the course of shelf life were subjected to mixed ANOVA, applying SPSS ver.21 (SPSS, Chicago, USA) at P < 0.05. In the mixed ANOVA, growth temperature treatment and storage temperature serve as between subject factor whereas shelf life days as within subject factor. The Shapiro-Wilk was applied to test the normality of the variables. Mauchly's test of sphericity was carried out to test whether variances of the differences between all possible pairs of within-subject conditions were equal. Greenhouse-Geisser's correction was applied to calculate the degrees of freedom in the case that the sphericity assumption was not fulfilled. When mixed ANOVA resulted in a significant interaction, a pairwise comparison was carried out for each shelf life day with LSD (Least Significant Difference) values estimated.
Results
Growth Temperature Affected Plant Size and Start of the Fruiting Period
Tomato plants started flowering after 7 weeks after sowing. At this moment, they were transferred from the greenhouse compartment to the climate cabinet. Plant morphology was affected by the growth temperature: higher growth temperature during cultivation resulted in larger and darker leaves compared to plants grown at 22◦C. Whereas plants grown at 16◦C were smaller than that of 22◦C (Supplementary Figure 1). The fruiting period was also affected; tomatoes were ready to be harvested at mature green (MG) at 12, 13, and 14 weeks after sowing for tomatoes cultivated at 28, 22, and 16◦C, respectively. A higher growth temperature also correlated with increased fruit production (data not shown).
Growth Temperature Affected Soluble Solid Contents and Diameter
A growth temperature of 16 and 28◦C resulted in higher SSC (◦Brix) values at harvest for both “Ponchi Re” and “Tarzan” tomatoes, compared with tomatoes cultivated at 22◦C (Figure 1A). No differences with respect to growth temperature were found for fruit fresh weight and fruit dry matter content (data not shown). Diameter of the MG tomatoes was not affected by growth temperature for “Ponchi Re” tomatoes. “Tarzan” tomatoes grown at 22◦C, had a larger diameter than those grown at both 28 and 16◦C (Figure 1B).
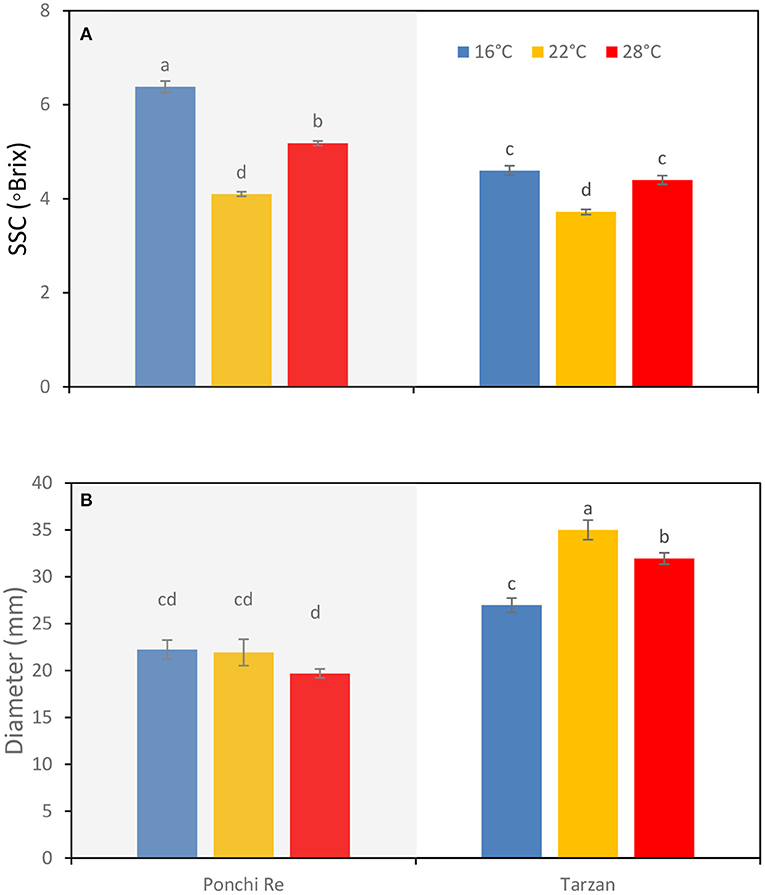
Figure 1. SSC (A) and diameter (B) of tomato grown at 16, 22, and 28◦C for “Ponchi Re” and “Tarzan” tomatoes at harvest. Error bars indicate standard error (n = 6). Different letters indicate significant difference at P < 0.05.
Low Growth Temperature Resulted in a Delay in Red Color Formation After Harvest
After harvest all green harvested tomatoes matured to red ripe, values were measured non-destructively by the pigment analyser (Figure 2). NAI and NDVI values are representative for lycopene and chlorophyll levels in the tomato pericarp, respectively (Schouten et al., 2014). The point in time where the NAI and NDVI values cross (cross point, CP), is an indication of the synchronization of the chlorophyll decay and lycopene formation. In “Ponchi Re,” the CP was ~14, 12, and 3 days after the start of shelf life without cold storage for tomatoes cultivated at 16, 22 and 28◦C, respectively. For prior cold stored “Ponchi Re,” the CP was 17, 9, and 7 days (Figures 2A–F). For non-cold stored “Tarzan” tomatoes the delay in color formation due to growth temperature was less pronounced (Figures 2G–L): 10, 8, and 5 days without storage, and 12, 7, and 9 days with prior cold storage for cultivation at 16, 22, and 28◦C, respectively. “Ponchi Re” tomatoes showed a difference in the CP of ~11 days between the lowest and highest growth temperature without cold storage (Figures 2A–C). This difference is approximately only 5 days for “Tarzan” tomatoes (Table 2). In fruit cultivated at 16 and 28◦C, prior cold storage resulted in a delay in the start of color development. This delay is about 2–4 days for tomatoes of both cultivars (Figure 2). Interestingly the CP was shorter for prior cold stored compared to non-cold stored tomatoes for especially “Ponchi Re” tomatoes (3 days) when cultivated at 22◦C (Figures 2B,E).
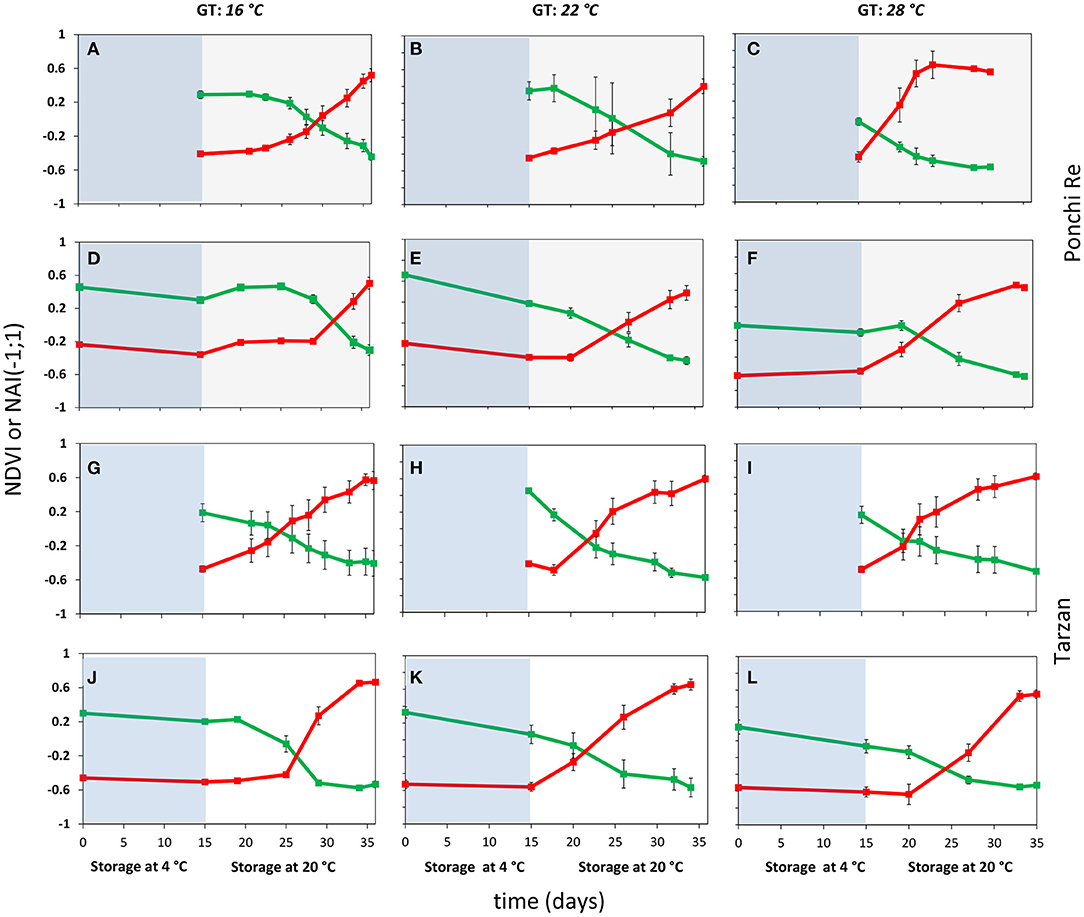
Figure 2. Color development as indicated by NDVI (green lines) and NAI (red lines) values during cold storage at 4◦C (blue area) and during shelf life (white area) of “Ponchi Re” (A–F) and “Tarzan” (G–L) tomatoes cultivated at a GT (growth temperature) of 16, 22, and 28◦C. Error bars indicate standard error (n = 15).
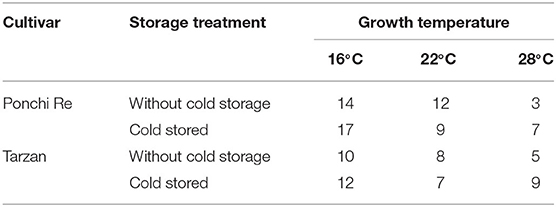
Table 2. Crossing points (CP) in days during shelf life at 20◦C of cold stored and without cold storage “Ponchi Re” and “Tarzan” tomatoes.
Growth Temperature Affects Firmness at Harvest and Subsequent Softening Behavior
Firmness at harvest was lower for tomatoes cultivated at 22◦C (Figures 3A,B). Cold storage resulted in less softening for tomatoes cultivated at 16◦C (Figures 3C,D). Softening during shelf life without prior cold storage was faster for “Ponchi Re” tomatoes cultivated at 28◦C than tomatoes cultivated at 16 and 22◦C (Figure 3A). Softening for non-cold stored “Tarzan” tomatoes was not affected by growth temperature (Figure 3B). Cold storage had no effect on the speed of softening in Ponchi Re. However, the reduced softening of Tarzan fruit grown at 16◦C during cold storage was compensated by an increased softening during shelf life, to end at the same firmness as the other fruit, after 20 days of shelf life.
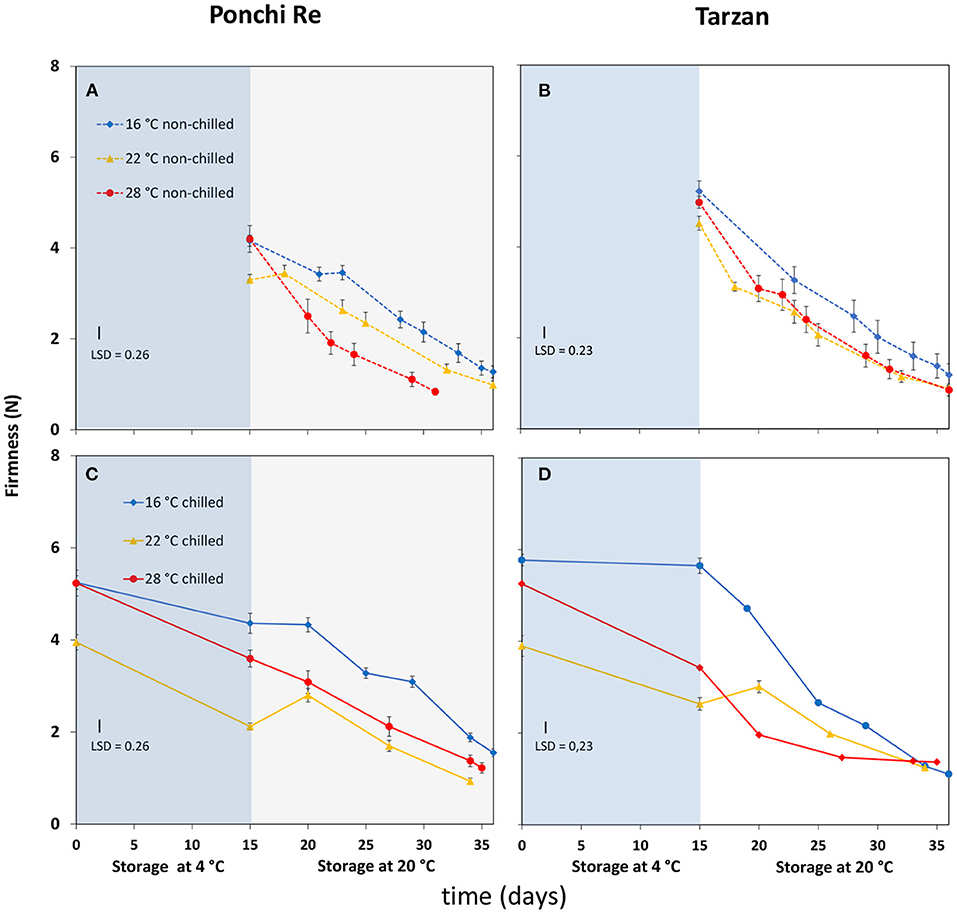
Figure 3. Firmness (N) values during cold storage at 4◦C (blue area) and during shelf life (white area) of “Ponchi Re” (A,C) and “Tarzan” (B,D) tomatoes cultivated at a GT (growth temperature) of 16, 22, and 28◦C. Error bars indicate standard error (n = 15).
Lower Growth Temperature Reduced Weight Loss
Weight loss during storage and shelf life was generally correlated with cultivation temperature. Weight loss was lowest at 16◦C, and increased for tomatoes of both cultivars cultivated at 22 and 28◦C (Figure 4). Weight loss during shelf life of prior cold stored tomatoes was similar as for non-cold stored tomatoes, with the exception of “Ponchi Re” fruit cultivated at 22◦C (Figure 4C). Reduced weight loss for “Ponchi Re” grown at 16 and 28◦C compared with 22◦C, could be considered a positive effect of cultivation stress on quality.
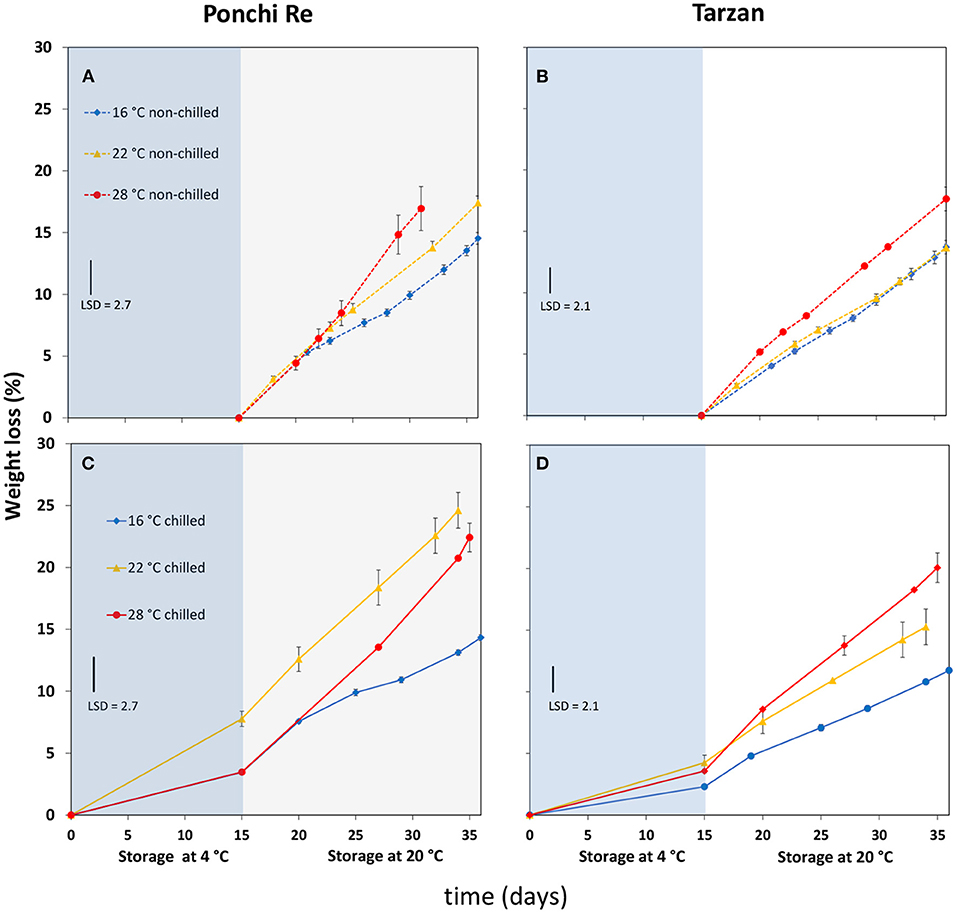
Figure 4. Weight loss percentage values during cold storage at 4◦C (blue area) and during shelf life (white area each plot) of “Ponchi Re” (A,C) and “Tarzan” (B,D) tomatoes cultivated at a GT (growth temperature) of 16, 22, and 28◦C. Error bars indicate standard error (n = 15).
Growth Temperature Affected Chilling Injury Incidence Depending on Cultivar
Chilling injury was judged by observing three symptoms; uneven ripening and color development, pitting, and decay (Affandi et al., 2020). For “Ponchi Re” tomatoes, increasing growth temperature resulted in lower CI incidence (P < 0.0001) while the opposite occurred for “Tarzan” tomatoes (Figure 5). “Tarzan” tomatoes, cultivated at 28◦C, experienced higher CI index values due to higher decay and pitting. In contrast, CI index of “Ponchi Re” cultivated at 16◦C was mainly due to higher uneven coloring and decay (data not shown).
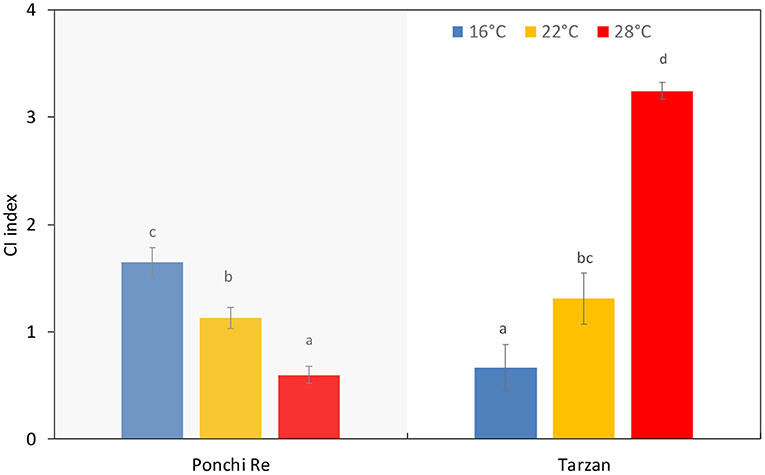
Figure 5. CI index of tomato grown at 16, 22, and 28◦C for “Ponchi Re” and “Tarzan” tomatoes at the end of shelf life following storage at 4◦C for 15 d. Error bars indicate standard error (n = 15). Different letters in each plot indicates significant differences at P < 0.05.
Discussion
Higher SSC Is Not Always Associated With Chilling Tolerance
A lower (16◦C) than optimum growth temperature increased SSC of “Ponchi Re” tomatoes (Figure 1). This is in line with the findings of Klopotek and Kläring (2014), who found increased accumulation of soluble sugars when tomato plants are exposed to growth temperature between 14–16◦C. Increased levels of soluble sugars, especially glucose, induce the ascorbate–glutathione cycle that scavenges H2O2 (Shao et al., 2013). Soluble sugars are also thought to act as osmoprotectant that confers freezing tolerance in plants (Ruelland et al., 2009). In addition, higher soluble sugars are associated with better cold tolerance such as in tomato (Liu et al., 2012; Zhang et al., 2019), loquat (Shao et al., 2013) and nectarines (Zhao et al., 2019). Higher SSC at harvest for 16◦C cultivated “Ponchi Re” tomatoes was, however, associated with higher CI incidence (Figure 5). This indicates that SSC is here likely not associated with chilling tolerance. In addition, higher SCC values for 16◦C cultivated “Ponchi Re” tomatoes softened at the same rate, indicating that this higher SSC was not related to difference in developmental stage. On the contrary, firmness at harvest was generally higher for 16◦C cultivated “Ponchi Re” tomatoes (Figure 3). It is possible that a higher SSC may have an osmotic effect resulting in increased water containment causing higher firmness (Shackel et al., 1991; Farneti et al., 2013; Lahaye et al., 2013). “Ponchi Re” fruits cultivated at higher than optimum (28◦C) also had higher SSC, and in this case, a lower CI index was observed. Plants cultivated at the lowest growth temperature showed smaller plants and less number of fruit and leaves (Supplementary Figure 1), it is likely that the plants grown at 16◦C were able to allocate more assimilates to fruit which caused higher SSC (Heuvelink, 1997; Beckles, 2012).
Low Growth Temperature Delayed Red Color Development, Especially for “Ponchi Re” Tomatoes
The crossing point (CP) indicates the level of synchronization of pigment degradation and synthesis. Cold storage was reported to delay and reduce lycopene accumulation in MG tomatoes despite uninterrupted chlorophyll degradation during shelf life (Affandi et al., 2020). The CP could therefore be regarded as indicator of chilling sensitivity. “Ponchi Re” tomatoes showed a larger difference in CP between the lowest and highest growth temperature with or without cold storage than “Tarzan” tomatoes (Figure 2). This indicates that color development is delayed by lower growth temperatures for especially “Ponchi Re” tomatoes. NAI and NDVI behavior is highly synchronized by growth temperature, with and without cold storage. This might indicate that a system that synchronizes chlorophyll decay and lycopene synthesis is dependent on growth temperature. Such a system might be under the regulation SGR (STAY-GREEN) protein. SGR genes induces chlorophyll breakdown and simultaneously blocks lycopene synthesis until SGR levels decrease to a threshold that allows synthesis of lycopene to start (Hu et al., 2011; Luo et al., 2013; Sánchez-González et al., 2016). Possibly, synthesis and breakdown of SGR in “Ponchi Re” tomatoes is more temperature dependent than in “Tarzan” tomatoes.
Prior cold stored showed a similar delay with the non-cold stored tomatoes in the start of the color development for both cultivars when cultivated at 16 or 28◦C (Figure 2). The opposite was shown for tomatoes cultivated at 22◦C. This means that cold storage benefitted the start of color development for tomatoes when cultivated at 22◦C. Likely, cold storage induced the accumulation of lycopene precursors, but only when cultivated at 22◦C. It is currently unclear why this happens. But it is clear that growth temperature affects color development. For instance, Hernandez et al. (2015) showed that a high (32◦C) growth temperature decreased and later increased the lycopene content when the number of days of high temperature exposure was increased.
Cold Tolerance Might Be Related to Membrane Integrity, Whereas Cold Sensitivity Might Be Related to Lack of ROS Scavenging Capacity
A lower growth temperature resulted in less softening during cold storage for both cultivars (Figures 3C,D). In addition, tomatoes cultivated at 16◦C showed less weight loss in general (Figure 4). Firmness retention during chilling and shelf life is associated with higher structural cell wall integrity and reduced decay (Mirdehghan et al., 2007; Rodoni et al., 2010; Gang et al., 2015). Lower weight loss is thought to be related to higher membrane integrity leading to less decay (Cohen et al., 1994; Ali et al., 2019; Ahmed et al., 2021). Finally, a higher SSC could also lead to increased osmolarity, retaining more water resulting in higher firmness (Shackel et al., 1991; Farneti et al., 2013; Lahaye et al., 2013).
Higher firmness retention and lower weight loss in low temperature cultivated “Tarzan” tomatoes than the control and higher temperature was associated with lower CI incidence (Figure 5). This might indicate that the cold tolerance of “Tarzan” from low temperature cultivation is, at least partly, based on increased membrane integrity. Increased cold tolerance in terms of ability to restore development after prior exposure to low temperature (4◦C) was also found in tomato plants (Barrero-Gil et al., 2016), watermelon (Lu et al., 2020), and sweet pepper (Ferguson et al., 1999) when cultivated at lower temperatures. There could also be a genetic component. It is possible that “Tarzan” may have a better regulation of the components of the chilling tolerance inducing CBF pathway (Singh et al., 2011) or produce small heat shock proteins (Luengwilai et al., 2012) when cultivated at lower cultivation temperatures.
The delay in lycopene formation in “Ponchi Re” tomatoes cultivated at the lowest growth temperatures (Figure 2) is probably associated with the increased CI incidence at lowest growth temperature (Figure 5). Reduced lycopene formation might result in less ROS scavenging capacity as lycopene and lycopene precursors, such as phytoene and phytofluene, scavenge singlet oxygen and peroxyl radicals generated by chilling stress (Engelmann et al., 2012; Lado et al., 2016; Rey et al., 2020). This delay might trigger a cascade of oxidative processes that lead to chilling damage (Hodges et al., 2004; Martínez et al., 2014). Cold stored “Tarzan” tomatoes cultivated at 16◦C, also experienced a delay in color development, although to a lesser extent (Figure 2). However, this delay did not result in more CI symptoms (Figure 5). This implies that the development of CI symptoms depends not only on the ROS scavenging capacity but also on other factors, undoubtedly dependent on genotypical differences between the two cultivars used in this study, such as membrane integrity, cell wall integrity, and transpiration rate of the fruit (Cohen et al., 1994; Rodoni et al., 2010; Gang et al., 2015).
Conclusion
This study assessed the effect of growth temperature during fruiting on quality properties such as SSC, color, firmness, weight loss, and chilling injury development in two dwarf tomato cultivars. Results exhibited that cultivation at low temperature delayed color development in “Ponchi Re” and to a lesser extent in “Tarzan” tomatoes. This delay probably contributed to the higher incidence of chilling injury symptoms in “Ponchi Re” compared to “Tarzan.” The lack of lycopene formation presumably results in less ROS scavenging capacity and more CI symptoms in “Ponchi Re.” Our study also demonstrated that low growth temperature increased firmness at harvest and decreased softening during cold storage in both cultivars. This could possibly be attributed to increased membrane integrity and higher osmolarity. Because “Tarzan” tomatoes had higher chilling tolerance when cultivated at the lower growth temperature, it is likely that higher membrane integrity was a component inducing higher chilling tolerance. This indicates that chilling injury incidence depends on growth temperature and genotype in the fruit of dwarf tomato plants.
Data Availability Statement
The raw data supporting the conclusions of this article will be made available by the authors, without undue reservation.
Author Contributions
RS and EW contributed to conception and design of the study. FYA and CP carried out the experiments, performed the statistical analysis, wrote the first draft of the manuscript, and wrote sections of the manuscript. FYA and RS contributed to data interpretation and discussion. EW and JV critically revised and approved the manuscript. All authors contributed to the article and approved the submitted version.
Funding
FYA acknowledge the Indonesian Endowment Fund for Education (LPDP) (Grant No. PRJ-2348/LPDP/2015) for the fellowship to conduct doctoral study.
Conflict of Interest
The authors declare that the research was conducted in the absence of any commercial or financial relationships that could be construed as a potential conflict of interest.
Publisher's Note
All claims expressed in this article are solely those of the authors and do not necessarily represent those of their affiliated organizations, or those of the publisher, the editors and the reviewers. Any product that may be evaluated in this article, or claim that may be made by its manufacturer, is not guaranteed or endorsed by the publisher.
Supplementary Material
The Supplementary Material for this article can be found online at: https://www.frontiersin.org/articles/10.3389/fsufs.2022.876597/full#supplementary-material
References
Affandi, F. Y., Verdonk, J. C., Ouzounis, T., Ji, Y., Woltering, E. J., and Schouten, R. E. (2020). Far-red light during cultivation induces postharvest cold tolerance in tomato fruit. Postharvest Biol. Technol. 159:111019. doi: 10.1016/j.postharvbio.2019.111019
Ahmed, Z. F., Alblooshi, S. S., Kaur, N., Maqsood, S., and Schmeda-Hirschmann, G. (2021). Synergistic effect of preharvest spray application of natural elicitors on storage life and bioactive compounds of date palm (Phoenix dactylifera L., cv. Khesab). Horticulturae 7:145. doi: 10.3390/horticulturae7060145
Albornoz, K., Cantwell, M. I., Zhang, L., and Beckles, D. M. (2019). Integrative analysis of postharvest chilling injury in cherry tomato fruit reveals contrapuntal spatio-temporal responses to ripening and cold stress. Sci. Rep. 9:2795. doi: 10.1038/s41598-019-38877-0
Ali, S., Khan, A. S., Anjum, M. A., Nawaz, A., Naz, S., Ejaz, S., et al. (2019). Aloe vera gel coating delays post-cut surface browning and maintains quality of cold stored lotus (Nelumbo nucifera Gaertn.) root slices. Sci. Hortic. 256:108612. doi: 10.1016/j.scienta.2019.108612
Barrero-Gil, J., Huertas, R., Rambla, J. L., Granell, A., and Salinas, J. (2016). Tomato plants increase their tolerance to low temperature in a chilling acclimation process entailing comprehensive transcriptional and metabolic adjustments. Plant Cell Environ. 39, 2303–2318. doi: 10.1111/pce.12799
Beckles, D. M (2012). Factors affecting the postharvest soluble solids and sugar content of tomato (Solanum lycopersicum L.) fruit. Postharvest Biol. Technol. 63, 129–140. doi: 10.1016/j.postharvbio.2011.05.016
Bertin, N., and Génard, M. (2018). Tomato quality as influenced by preharvest factors. Sci. Hortic. 233, 264–276. doi: 10.1016/j.scienta.2018.01.056
Biswas, P., East, A. R., Hewett, E. W., and Heyes, J. A. (2016). Chilling injury in tomato fruit. Hortic. Rev. 44, 229–278.
Campos, M. L., Carvalho, R. F., Benedito, V. A., and Peres, L. E. P. (2010). Small and remarkable. Plant Signal. Behav. 5, 267–270. doi: 10.4161/psb.5.3.10622
Chen, W. P., and Li, P. H. (2002). Membrane stabilization by abscisic acid under cold aids proline in alleviating chilling injury in maize (Zea mays L.) cultured cells. Plant Cell Environ. 25, 955–962. doi: 10.1046/j.1365-3040.2002.00874.x
Cohen, E., Shapiro, B., Shalom, Y., and Klein, J. D. (1994). Water loss: a nondestructive indicator of enhanced cell membrane permeability of chilling-injured citrus fruit. J. Am. Soc. Hortic. Sci. 119, 983–986. doi: 10.21273/JASHS.119.5.983
Crisosto, C. H., Mitchell, F. G., and Ju, Z. (1999). Susceptibility to chilling injury of peach, nectarine, and plum cultivars grown in California. HortScience 34, 1116–1118. doi: 10.21273/HORTSCI.34.6.1116
Daie, J., and Campbell, W. F. (1981). Response of tomato plants to stressful temperatures: increase in abscisic acid concentrations. Plant Physiol. 6, 26–29. doi: 10.1104/pp.67.1.26
Engelmann, N. J., Clinton, S. K., and Erdman, J. W. Jr. (2012). Nutritional aspects of phytoene and phytafluene, carotenoid precursors to lycopene (vol 2, pg 51, 2010). Adv. Nutr. 3, 255–255. doi: 10.3945/an.110.000075
Farneti, B., Schouten, R. E., Qian, T., Dieleman, J. A., Tijskens, L. M. M., and Woltering, E. J. (2013). Greenhouse climate control affects postharvest tomato quality. Postharvest Biol. Technol. 86, 354–361. doi: 10.1016/j.postharvbio.2013.07.004
Ferguson, I., Volz, R., and Woolf, A. (1999). Preharvest factors affecting physiological disorders of fruit. Postharvest Biol. Technol. 15, 255–262. doi: 10.1016/S0925-5214(98)00089-1
Gang, C., Li, J., Chen, Y., Wang, Y., Li, H., Pan, B., et al. (2015). Synergistic effect of chemical treatments on storage quality and chilling injury of honey peaches. J. Food Process. Preservat. 39, 1108–1117. doi: 10.1111/jfpp.12325
Gautier, H., Diakou-Verdin, V., Bénard, C., Reich, M., Buret, M., Bourgaud, F., et al. (2008). How does tomato quality (sugar, acid, and nutritional quality) vary with ripening stage, temperature, and irradiance? J. Agric. Food Chem. 56, 1241–1250. doi: 10.1021/jf072196t
Hernandez, V., Hellín, P., Fenoll, J., and Flores, P. (2015). Increased temperature produces changes in the bioactive composition of tomato, depending on its developmental stage. J. Agric. Food Chem. 63, 2378–2382. doi: 10.1021/jf505507h
Heuvelink, E (1997). Effect of fruit load on dry matter partitioning in tomato. Sci. Hortic. 69, 51–59. doi: 10.1016/S0304-4238(96)00993-4
Hobson, G. E (1981). The short-term storage of tomato fruit. J. Hortic. Sci. 56, 363–368. doi: 10.1080/00221589.1981.11515014
Hodges, D. M., Lester, G. E., Munro, K. D., and Toivonen, P. M. A. (2004). Oxidative stress: Importance for postharvest quality. HortScience 39, 924–929. doi: 10.13140/2.1.3929.1526
Hu, Z. L., Deng, L., Yan, B., Pan, Y., Luo, M., Chen, X., et al. (2011). Silencing of the LeSGR1 gene in tomato inhibits chlorophyll degradation and exhibits a stay-green phenotype. Biol. Plant. 55, 27–34. doi: 10.1007/s10535-011-0004-z
Kaiser, E., Ouzounis, T., Giday, H., Schipper, R., Heuvelink, E., and Marcelis, L. F. M. (2019). Adding blue to red supplemental light increases biomass and yield of greenhouse-grown tomatoes, but only to an optimum. Front. Plant Sci. 7:1275. doi: 10.3389/fpls.2018.02002
Kang, H. M., Park, K. W., and Saltveit, M. E. (2002). Elevated growing temperatures during the day improve the postharvest chilling tolerance of greenhouse-grown cucumber (Cucumis sativus) fruit. Postharvest Biol. Technol. 24, 49–57. doi: 10.1016/S0925-5214(01)00129-6
Klopotek, Y., and Kläring, H. P. (2014). Accumulation and remobilisation of sugar and starch in the leaves of young tomato plants in response to temperature. Sci. Hortic. 180, 262–267. doi: 10.1016/j.scienta.2014.10.036
Lado, J., Rodrigo, M. J., López-Climent, M., Gómez-Cadenas, A., and Zacarías, L. (2016). Implication of the antioxidant system in chilling injury tolerance in the red peel of grapefruit. Postharvest Biol. Technol. 111, 214–223. doi: 10.1016/j.postharvbio.2015.09.013
Lahaye, M., Devaux, M. F., Poole, M., Seymour, G. B., and Causse, M. (2013). Pericarp tissue microstructure and cell wall polysaccharide chemistry are differently affected in lines of tomato with contrasted firmness. Postharvest Biol. Technol. 76, 83–90. doi: 10.1016/j.postharvbio.2012.09.009
Liu, C., Jahangir, M. M., and Ying, T. (2012). Alleviation of chilling injury in postharvest tomato fruit by preconditioning with ultraviolet irradiation. J. Sci. Food Agric. 92, 3016–3022. doi: 10.1002/jsfa.5717
Lu, J., Nawaz, M. A., Wei, N., Cheng, F., and Bie, Z. (2020). Suboptimal temperature acclimation enhances chilling tolerance by improving photosynthetic adaptability and osmoregulation ability in watermelon. Hortic. Plant J. 6, 49–60. doi: 10.1016/j.hpj.2020.01.001
Luengwilai, K., Beckles, D. M., and Saltveit, M. E. (2012). Chilling-injury of harvested tomato (Solanum lycopersicum L.) cv. Micro-Tom fruit is reduced by temperature pre-treatments. Postharvest Biol. Technol. 63, 123–128. doi: 10.1016/j.postharvbio.2011.06.017
Luo, Z., Zhang, J., Li, J., Yang, C., Wang, T., Ouyang, B., Li, H., Giovannoni, J. J., and Ye, Z. (2013). A STAY-GREEN protein SlSGR1 regulates lycopene and ß-caroteneaccumulation by interacting directly with SlPSY1 during ripening processes in tomato. New Phytol. 198, 442–452. doi: 10.1111/nph.12175
Lurie, S., and Crisosto, C. H. (2005). Chilling injury in peach and nectarine. Postharvest Biol. Technol. 37, 195–208. doi: 10.1016/j.postharvbio.2005.04.012
Lurie, S., and Sabehat, A. (1997). Prestorage temperature manipulations to reduce chilling injury in tomatoes. Postharvest Biol. Technol. 11, 57–62. doi: 10.1016/S0925-5214(97)01411-7
Malacrida, C., Valle, E. M., and Boggio, S. B. (2006). Postharvest chilling induces oxidative stress response in the dwarf tomato cultivar Micro-Tom. Physiol. Plant. 127, 10–18. doi: 10.1111/j.1399-3054.2005.00636.x
Martínez, A., Stinco, C. M., and Melendez-Martinez, A. J. (2014). Free radical scavenging properties of phytofluene and phytoene isomers as compared to lycopene: a combined experimental and theoretical study. J. Phys. Chem. B 118, 9819–9825. doi: 10.1021/jp503227j
Meissner, R., Jacobson, Y., Melamed, S., Levyatuv, S., Shalev, G., Ashri, A., et al. (1997). A new model system for tomato genetics. Plant J. 12, 1465–1472. doi: 10.1046/j.1365-313x.1997.12061465.x
Mirdehghan, S. H., Rahemi, M., Martínez-Romero, D., Guillén, F., Valverde, J. M., Zapata, P. J., et al. (2007). Reduction of pomegranate chilling injury during storage after heat treatment: role of polyamines. Postharvest Biol. Technol. 44, 19–25. doi: 10.1016/j.postharvbio.2006.11.001
Neta-Sharir, I (2005). Dual role for tomato heat shock protein 21: protecting photosystem II from oxidative stress and promoting color changes during fruit maturation. Plant Cell 17, 1829–1838. doi: 10.1105/tpc.105.031914
Rey, F., Zacarías, L., and Rodrigo, M. J. (2020). Carotenoids, vitamin C, and antioxidant capacity in the peel of mandarin fruit in relation to the susceptibility to chilling injury during postharvest cold storage. Antioxidants 9:1296. doi: 10.3390/antiox9121296
Riga, P., Anza, M., and Garbisu, C. (2008). Tomato quality is more dependent on temperature than on photosynthetically active radiation. J. Sci. Food Agric. 88, 158–166. doi: 10.1002/jsfa.3065
Rodoni, L., Casadei, N., Concellon, A., Chaves Alicia, A. R., and Vicente, A. R. (2010). Effect of short-term ozone treatments on tomato (Solanum lycopersicum L.) fruit quality and cell wall degradation. J. Agric. Food Chem. 58, 594–599. doi: 10.1021/jf9029145
Ruelland, E., Vaultier, M.-N., Zachowski, A., and Hurry, V. (2009). Cold signalling and cold acclimation in plants. Adv. Bot. Res. 49, 35–150. doi: 10.1016/S0065-2296(08)00602-2
Sabehat, A., Lurie, S., and Weiss, D. (1998). Expression of small heat-shock proteins at low temperatures. A possible role in protecting against chilling injuries. Plant Physiol. 117, 651–658. doi: 10.1104/pp.117.2.651
Sabehat, A., Weiss, D., and Lurie, S. (1996). The correlation between heat-shock protein accumulation and persistence and chilling tolerance in tomato fruit. Plant Physiol. 110, 531–537. doi: 10.1104/pp.110.2.531
Sánchez-González, M. J., Schouten, R. E., Tijskens, L. M. M., Sánchez-Guerrero, M. C., Medrano, E., del Rio-Celestino, M., et al. (2016). Salinity and ripening on/off the plant effects on lycopene synthesis and chlorophyll breakdown in hybrid Raf tomato. Sci. Hortic. 211, 203–212. doi: 10.1016/j.scienta.2016.08.030
Schouten, R. E., Huijben, T. P. M., Tijskens, L. M. M., and van Kooten, O. (2007). Modelling quality attributes of truss tomatoes: linking colour and firmness maturity. Postharvest Biol. Technol. 45, 298–306. doi: 10.1016/j.postharvbio.2007.03.011
Schouten, R. E., Farneti, B., Tijskens, L. M. M., Alarcón, A. A., and Woltering, E. J. (2014). Quantifying lycopene synthesis and chlorophyll breakdown in tomato fruit using remittance VIS spectroscopy. Postharvest Biol. Technol. 96, 53–63. doi: 10.1016/j.postharvbio.2014.05.007
Shackel, K. A., Greve, C., Labavitch, J. M., and Ahmadi, H. (1991). Cell turgor changes associated with ripening in tomato pericarp tissue. Plant Physiol. 97, 814–816. doi: 10.1104/pp.97.2.814
Shao, X., Zhu, Y., Cao, S., Wang, H., and Song, Y. (2013). Soluble sugar content and metabolism as related to the heat-induced chilling tolerance of loquat fruit during cold storage. Food Bioproc. Technol. 6, 3490–3498. doi: 10.1007/s11947-012-1011-6
Singh, S., Rathore, M., Goyary, D., Singh, R. K., Anandhan, S., Sharma, D. K., et al. (2011). Induced ectopic expression of At-CBF1 in marker-free transgenic tomatoes confers enhanced chilling tolerance. Plant Cell Rep. 30, 1019–1028. doi: 10.1007/s00299-011-1007-0
Tao, F., Zhang, L., McCarthy, M. J., Beckles, D. M., and Saltveit, M. (2014). Magnetic resonance imaging provides spatial resolution of chilling injury in Micro-Tom tomato (Solanum lycopersicum L.) fruit. Postharvest Biol. Technol. 97, 62–67. doi: 10.1016/j.postharvbio.2014.06.005
Vega-García, M. O., López-Espinoza, G., Chávez Ontiveros, J., Caro-Corrales, J. J., Delgado Vargas, F., and López-Valenzuela, J. A. (2010). Changes in protein expression associated with chilling injury in tomato fruit. J. Am. Soc. Hortic. Sci. 135, 83–89. doi: 10.21273/JASHS.135.1.83
Wang, C. Y., and Buta, J. G. (1994). Methyl jasmonate reduces chilling injury in Cucurbita pepo through its regulation of abscisic acid and polyamine levels. Environ. Exp. Bot. 34, 427–432. doi: 10.1016/0098-8472(94)90025-6
Whitaker, B. D (1991). Changes in lipids of tomato fruit stored at chilling and non-chilling temperatures. Phytochemistry 30, 757–761. doi: 10.1016/0031-9422(91)85247-W
Zhang, W. F., Gong, Z. H., Wu, M. B., Chan, H., Yuan, Y. J., Tang, N., et al. (2019). Integrative comparative analyses of metabolite and transcript profiles uncovers complex regulatory network in tomato (Solanum lycopersicum L.) fruit undergoing chilling injury. Sci. Rep. 9:4470. doi: 10.1038/s41598-019-41065-9
Keywords: temperature stress, dwarf tomatoes, ripening, cold tolerance, tomato quality
Citation: Affandi FY, Pijnenburg C, Verdonk JC, Woltering EJ and Schouten RE (2022) Growth Temperature Influences Postharvest Quality and Cold Tolerance of Green Harvested Dwarf Tomatoes During Storage. Front. Sustain. Food Syst. 6:876597. doi: 10.3389/fsufs.2022.876597
Received: 15 February 2022; Accepted: 27 March 2022;
Published: 18 April 2022.
Edited by:
Ghulam Khaliq, Lasbela University of Agriculture, Water and Marine Sciences, PakistanReviewed by:
Mahmood Ul Hasan, University of Agriculture, Faisalabad, PakistanYahya Faqir, Southwest University of Science and Technology, China
Copyright © 2022 Affandi, Pijnenburg, Verdonk, Woltering and Schouten. This is an open-access article distributed under the terms of the Creative Commons Attribution License (CC BY). The use, distribution or reproduction in other forums is permitted, provided the original author(s) and the copyright owner(s) are credited and that the original publication in this journal is cited, in accordance with accepted academic practice. No use, distribution or reproduction is permitted which does not comply with these terms.
*Correspondence: Fahrizal Yusuf Affandi, ZmFocml6YWx5dXN1Zi5hZmZhbmRpQHVnbS5hYy5pZA==