- 1Chair of Plant Health, Institute of Agricultural and Environmental Sciences, Estonian University of Life Sciences, Tartu, Estonia
- 2Department of Plants and Crops, Laboratory of Agrozoology, Faculty of Bioscience Engineering, Ghent University, Ghent, Belgium
Approximately 84% of farms globally are <2 hectares; these and other smallholder farms collectively produce over one third of humanity's food. However, smallholder farms, particularly in developing countries, encounter difficulties in both production and profits due to their vulnerabilities. Sustainable intensification—increasing crop yield without significantly greater resource use—must be globally adopted in smallholder farming to achieve various Sustainable Development Goals (SDGs) endorsed by the United Nations (UN). While traditional techniques for conservation agriculture must be maintained and further promoted, new technologies will undoubtedly play a major role in achieving high yields in a sustainable and environmentally safe manner. RNA interference (RNAi) technology, particularly the use of transgenic RNAi cultivars and/or sprayable double-stranded RNA (dsRNA) pesticides, could accelerate progress in reaching these goals due to dsRNA's nucleotide sequence-specific mode of action against eukaryotic and viral pests. This sequence-specificity allows silencing of specific genetic targets in focal pest species of interest, potentially resulting in negligible effects on non-target organisms inhabiting the agroecosystem. It is our perspective that recent progress in RNAi technology, together with the UN's endorsement of SDGs that promote support in- and for developing countries, should facilitate an integrated approach to sustainable intensification of smallholder farms, whereby RNAi technology is used in combination with traditional techniques for sustainable intensification. However, the development of such approaches in developing countries will require developed countries to adhere to currently-defined socioeconomic SDGs.
Challenges to Sustainable Food Production, and Contemporary Role of Traditional Principles, in Smallholder Farms
As both arable land and water resources are becoming less abundant relative to dependent human populations, the United Nations (UN) has ambitiously set to achieve various Sustainable Development Goals (SDGs) by 2030 (United Nations, 2022). Each goal is relevant to a broader action plan for people, planet and prosperity; SDG 2—End hunger, achieve food security and improved nutrition, and promote sustainable agriculture—fully relates to sustainable food production. This goal attempts to mitigate the negative effects of resource scarcity on hunger, especially in developing countries where poverty and hunger present major problems within these communities.
Sustainable farming systems must be in place globally to counteract and prevent hunger in local, regional and foreign human populations. Of the ~570 million farms globally, most are small, 84% being under two hectares (Lowder et al., 2016). Smallholder farms represent a critical focus for achieving sustainable food production, given the global distribution of smallholder farms and their contribution to producing over a third of the world's food (Lowder et al., 2021). Furthermore, a recent evidence review and meta-analysis showed that smaller farms have, on average, higher yields and greater crop- and non-crop biodiversity than larger farms (Ricciardi et al., 2021).
Smallholder farms include some of the most vulnerable crop production systems, as they are often less- or insufficiently resourced for preventing crop damage (e.g., due to crop pests, farmland erosion, climate hazards). In addition, smallholder farms often have a more difficult time making profits from their labor, due to relatively small quantities of food produced, lack of social protection, dependency on less farm hands, and their disadvantage in supply chains. To provide for an exponentially growing human population, smallholder farming systems must undergo sustainable intensification, whereby crop yields are increased in a manner that minimizes environmental impacts without significantly greater expenditure of resources (e.g., water, land, labor costs). Traditional practices, together with newer technologies, have both been developed with the aim of aiding sustainable intensification in crop production; SDGs 1.4, 8.2, 9.4, and 9.5 (United Nations, 2022; Table 1) encourage greater adoption- and upgrades of technologies in developing countries. Indeed, sustainable intensification is likely to require a combination of both traditional- and biotechnological approaches to achieve the established SDGs.
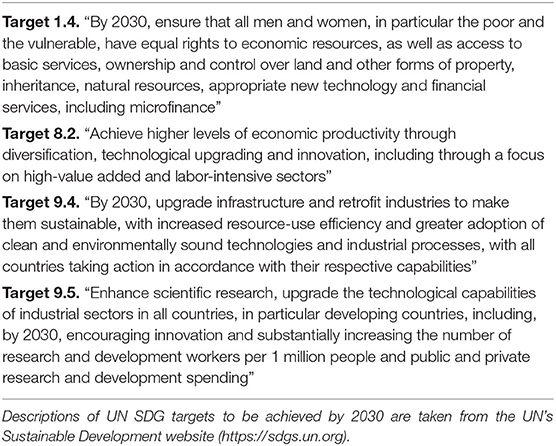
Table 1. Sustainable Development Goal (SDG) targets, endorsed by the United Nations (UN), relevant to adopting RNAi crop technology in developing countries.
Diversified cropping systems, as well as landscape heterogeneity, represent long-held approaches to sustainable food production. Such approaches for diversification aim to enhance sustainable production via promoting ecological diversity at both field- and landscape scales. Cropping systems such as inter-, under- and cover cropping, maintaining crop diversity, preserving and restoring natural and seminatural habitats, and implementation of diverse agroforestry practices can enhance both above- and belowground ecosystem services in smallholder farms, allowing reductions in external inputs, thereby promoting healthy viable agroecosystems; they can also benefit food- and nutrition security and encourage diverse diets, without reducing crop yields (Chai et al., 2021; Drinkwater et al., 2021; Jemal et al., 2021; Priyadarshana et al., 2021; Rodriguez et al., 2021). However, diversified cropping systems continue to raise both economic and social challenges that must be overcome, and smallholder farmers understand that crop diversification is not a standalone sustainable solution (Rodriguez et al., 2021). Furthermore, ecosystem services are not explicitly considered to be key factors for achieving SDGs in developing countries (Knight, 2021). Some principles of conservation agriculture may even limit crop productivity. For example, in an analysis of field trials across 48 crops and 68 countries, yield declines with an overall mean of 10% were observed when no-tillage was applied in the absence of other sustainable intensification practices (Pittelkow et al., 2015). Thus, traditional techniques for sustainable intensification should not be implemented alone, but rather alongside other field- and landscape scale measures.
Given the variability of environmental and socioeconomic conditions surrounding smallholder farming, sustainable intensification interventions must be adapted to local contexts (Reich et al., 2021). Furthermore, traditional techniques for sustainable resource management (e.g., intercropping, cover cropping, crop rotation, organic farming, agroforestry) should be applied using advanced technologies for efficient use of resources (Imoro et al., 2021). Indeed, while building upon the knowledge of indigenous communities is expected to be crucial for reaching SDGs (United Nations Environment Programme Food Agriculture Organization of the United Nations, 2020), the integration of new technologies can be pivotal in reaching these goals. While smallholder farmers may view new biotechnological applications as high-risk, the push to globally achieve SDGs requires that we explore the benefits of an interdisciplinary path to reach these goals. The current momentum in technological development, especially with regard to crop protection biotechnologies, also allows the development and assessment of more target-specific pesticides that potentially provide the most ecologically sustainable way to control crop pests.
Prospective Role of RNAi Technology in Sustainable Intensification
Crop pests represent a dynamic, highly unpredictable and often difficult-to-control problem for farmers globally, and cautious pesticide use has become an important pillar of integrated pest management (Barzman et al., 2015). Pesticides are considered irreplaceable to farmers in situations where natural biological control is inadequate in regulating pests of cash crops, which renders such farms vulnerable without the integration of pesticides. Here we consider a biosafe pesticide technology: the use of transgenic RNA interference (RNAi) cultivars and/or sprayed double-stranded RNA (dsRNA), representing approaches that have made significant progress in crop protection research and are currently under development by several biotechnology companies (Rank and Koch, 2021; Willow and Veromann, 2021). The attraction to the use of RNAi as a crop protection technology is due to dsRNA's nucleotide sequence-specific mode of action against eukaryotes and viruses. This specificity allows remarkably target-specific control of crop pests, via the evolutionarily conserved mechanism of RNAi (Rank and Koch, 2021), potentially leaving beneficial non-target organisms (e.g., crop pollinators, biological control agents, soil decomposers) unharmed. This is in stark contrast to the observed effects of non-RNA pesticide technology on non-target organisms (Sgolastra et al., 2018; Simon-Delso et al., 2018; Calvo-Agudo et al., 2019; Willow et al., 2019; Schulz et al., 2021). Thus, SDGs 3.9, 12.4, 14.1, 15.5, and 15.9 (United Nations, 2022; Table 2) may be better achieved through the use of RNAi pesticide technology, rather than conventional pesticides which currently dominate the market.
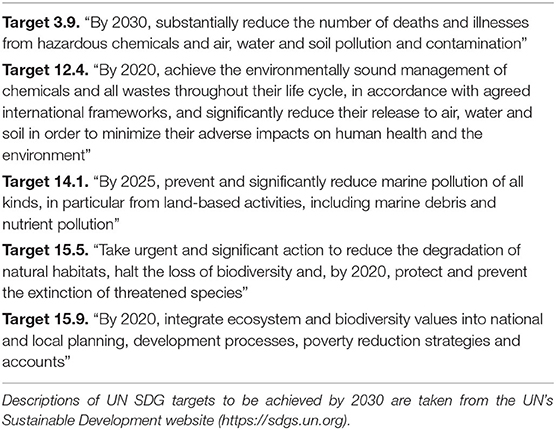
Table 2. Sustainable Development Goal (SDG) targets, endorsed by the United Nations (UN), that may be better achieved through the use of RNAi crop technology, rather than conventional pesticides.
RNAi technology represents a promising solution for controlling outbreaks of pests in various cropping systems (Baum et al., 2007; Koch et al., 2016; Mitter et al., 2017; Cagliari et al., 2019; Worrall et al., 2019; Petek et al., 2020; Rank and Koch, 2021). Harnessing RNAi technology for crop protection holds great potential for compatibility, and perhaps synergy, with conservation biological control services in agroecosystems, as a result of dsRNA's nucleotide sequence-specific mode of action (potentially conferring no toxicity to non-target organisms). Furthermore, dsRNA can be used to prevent plant virus transmission/vectoring by crop pests (Bahrami Kamangar et al., 2019; Worrall et al., 2019), as well as target non-essential mechanisms, thereby reducing pest population fitness while maintaining pest presence for specialized biological control agents (e.g., specialist predators, parasitoids) to sustain their own populations (Willow et al., 2021b). Rapid degradation of dsRNA in soils and waterbodies (Parker et al., 2019; Bachman et al., 2020) also presents important benefits over other types of pesticide molecules. However, as both dsRNA uptake and RNAi efficiency can vary between- and within taxa (Wytinck et al., 2020; Willow and Veromann, 2021), potentially necessitating the use of nanoparticles as co-formulants (Wytinck et al., 2020), the environmental fate of sprayed dsRNA must be examined under various formulation scenarios.
Both dsRNA production and transgenic biotechnologies have steadily increased in cost-efficiency over recent years. For example, with the advent of large-scale cell-free dsRNA production, the cost of dsRNA has dropped to < $0.50 per gram (Rank and Koch, 2021). Since exogenously-applied (sprayed) dsRNA can be specifically designed to base-pair with endogenous messenger RNA (mRNA) that codes for a specific gene, this enables a multitude of molecular targets in any eukaryotic or viral pest with available transcriptome sequence information (necessary for designing a template for dsRNA synthesis). While this information is not currently available for most agricultural pests, current costs of obtaining whole-sequence information are no longer considered prohibitive, and promising initiatives are underway to mitigate this knowledge gap (Lewin et al., 2018; The Earth BioGenome Project, 2018; i5k, 2022).
Both RNAi cultivars and dsRNA sprays present certain advantages over each other. RNAi cultivars constantly produce the target-specific dsRNA in the plant's tissues, allowing the target pest to be constantly exposed to the pesticide, as long as the pest feeds on the transgenic crop. In some instances, chronic feeding on dsRNA can significantly enhance RNAi efficacy, compared to short-term feeding on dsRNA (Willow et al., 2021a). Thus, transgenic approaches to RNAi-based pest management may be necessary in many cases, supporting the use of RNAi cultivars over dsRNA sprays. On the other hand, dsRNA sprays can be altered (e.g., co-formulants, target gene) for adaptive management, and in cases of pest polyphagy, multiple crop species can be treated simultaneously to target the same organism, reducing the pest's ability to successfully take refuge on its secondary or tertiary host plant. Furthermore, dsRNA, when sprayed, is less persistent in the environment compared to that for RNAi cultivars (which constantly produce the target-specific dsRNA in the plant's tissues throughout the growing period); this reduced persistency tightens the period of potential exposure to dsRNA, lessening the chance of resistance development in target organisms, resistance development being a potential hurdle for RNAi implementation (Khajuria et al., 2018; Mishra et al., 2021). Finally, there is the possibility of efficient RNAi-based control via topical contact to sprayed dsRNA; this has been observed in aphids (Niu et al., 2019; Zheng et al., 2019; Yan et al., 2020), and is likely to be an effective method of control for additional insect pests, even though oral consumption of treated plant parts is the typical aim when spraying dsRNA for management of insect pests.
Integrating RNAi-Based Management in Smallholder Farms
Whether considering traditional techniques for sustainable intensification, the use of new sustainable technologies, or a combination of both approaches, social and financial constraints to implementing RNAi are evident, especially in the context of smallholder farmers in developing countries. However, with the rapidly decreasing costs of both dsRNA production and transgenic biotechnologies, together with the increasing number of agriculturally relevant pest species with available genetic sequence information, the prospect of integrating these techniques to work toward sustainable resource management in smallholder farming systems is becoming increasingly realistic. Indeed, RNAi techniques have already been introduced into smallholder farming systems, with great success. For example, in 1998, the U.S. government allowed about 200 smallholder papaya farmers to begin planting a papaya ringspot virus (PRSV)-resistant cultivar, as PRSV had resulted in nearly 40% production loss. This RNAi cultivar, commercialized as Rainbow papaya, produced immediate positive results, not only halting the rapid decline of Hawaii's papaya industry, but returning production to levels similar to before PRSV invasion. Today this RNAi-based papaya dominates the Hawaiian papaya market (Kuo and Falk, 2020). More recently, in 2021, the Kenyan National Biosafety Authority has approved the environmental release of an RNAi cassava cultivar resistant to cassava brown steak virus (CBSV) and Ugandan cassava brown streak virus (UCBSV), both of which result in cassava brown streak disease (CBSD). This cultivar, known as 4046 cassava, shows a positive food- and feed safety profile compared to its non-transgenic parental cultivar (Wagaba et al., 2021). National performance trials currently being conducted are optimistic, and this cultivar is expected to soon be available to Kenyan cassava farmers (Cassava Plus, 2021). RNAi-based control of such viral diseases enhances sustainable intensification not only by increasing crop yields, but potentially also by reducing or eliminating the need to use insecticidal interventions against crop disease vectors (e.g., whiteflies, in the case of both CBSV and UCBSV). Using both tobacco and cowpea plants, Worrall et al. (2019) recently demonstrated the disruption of aphid-mediated virus transmission between plants, via exogenous application of dsRNA targeting bean common mosaic virus. Together these case studies suggest that both transgenic and dsRNA spray approaches can be mobilized to protect crops from viral diseases and potentially reduce the need to directly manage disease vectors.
It is paramount that progress in crop protection biotechnology does not exclude smallholder farmers in developing countries. This would require dependable socioeconomic support from local and foreign stakeholders and mid- or downstream sectors of food supply chains. It may also require dependable educational support from the scientific community and relevant expert practitioners, given the potential for smallholder farmers to view RNAi crop biotechnology as high-risk. Thus, financial support/investments and transfer of knowledge are both crucial to allow smallholder farmers to integrate sequence-specific biosafe pesticide technology into pest management strategies. Such aims can be facilitated by local and foreign adherence to defined targets in SDGs 1, 2, 4, 7, 8, 9, 10, 11, 12, 13, 15, and 17 (United Nations, 2022), which should be expected to be fully endorsed, in practice, by all able UN member states; the transfer of support from developed- to developing countries is particularly relevant here. Furthermore, the integration of new and traditional crop protection techniques are likely to facilitate a beneficial two-way transfer of knowledge regarding the use of sustainable intensification techniques.
It is our perspective that RNAi technology could be of great value to sustainable intensification of smallholder farms, including in developing countries, where there is currently the least infrastructure in place for implementing this technology. It must also be considered that achieving SDGs in agricultural and ecological sustainability innately requires adherence to socioeconomic SDGs designed to provide support for smallholder farmers in developing countries. Given the potential for ecologically sustainable crop protection via the use of RNAi technology, it remains vital to fill in the existing gaps regarding: (1) ensuring smallholder farmers satisfactory support; (2) obtaining transcriptome sequences of important crop pests globally; and (3) determining methods for cost-effective RNAi applications in pest taxa for which RNAi-based management is considered a possible solution.
Data Availability Statement
The original contributions presented in the study are included in the article/supplementary material, further inquiries can be directed to the corresponding author/s.
Author Contributions
JW conceived the manuscript and wrote the original draft. All authors made comments/suggests toward revising the original draft, and approved the final version of the manuscript.
Funding
This work was funded by the Personal Research Funding project No. PRG1056 of the Estonian Research Council.
Conflict of Interest
The authors declare that the research was conducted in the absence of any commercial or financial relationships that could be construed as a potential conflict of interest.
Publisher's Note
All claims expressed in this article are solely those of the authors and do not necessarily represent those of their affiliated organizations, or those of the publisher, the editors and the reviewers. Any product that may be evaluated in this article, or claim that may be made by its manufacturer, is not guaranteed or endorsed by the publisher.
Acknowledgments
The authors acknowledge Ana Isabel Silva, as well as our reviewer and editor, for providing comments and suggestions that helped to improve this manuscript.
References
Bachman, P., Fischer, J., Song, Z., Urbanczyk-Wochniak, E., and Watson, G. (2020). Environmental fate and dissipation of applied dsRNA in soil, aquatic systems, and plants. Front. Plant Sci. 11, 21. doi: 10.3389/fpls.2020.00021
Bahrami Kamangar, S., Christiaens, O., Taning, C. N. T., De Jonghe, K., and Smagghe, G. (2019). The cuticle protein MPCP2 is involved in Potato virus Y transmission in the green peach aphid Myzus persicae. J. Plant Dis. Prot. 126, 351–357. doi: 10.1007/s41348-019-00232-w
Barzman, M., Bàrberi, P., Birch, A. N. E., Boonekamp, P., Dachbrodt-Saaydeh, S., Graf, B., et al. (2015). Eight principles of integrated pest management. Agron. Sustain. Dev. 35, 1199–1215. doi: 10.1007/s13593-015-0327-9
Baum, J. A., Bogaert, T., Clinton, W., Heck, G. R., Feldmann, P., Ilagan, O., et al. (2007). Control of coleopteran insect pests through RNA interference. Nat. Biotechnol. 25, 1322–1326. doi: 10.1038/nbt1359
Cagliari, D., Dias, N. P., Galdeano, D. M., dos Santos, E. Á., Smagghe, G., and Zotti, M. J. (2019). Management of pest insects and plant diseases by non-transformative RNAi. Front. Plant Sci. 10, 1319. doi: 10.3389/fpls.2019.01319
Calvo-Agudo, M., González-Cabrera, J., Picó, Y., Calatayud-Vernich, P., Urbaneja, A., Dicke, M., et al. (2019). Neonicotinoids in excretion product of phloem-feeding insects kill beneficial insects. Proc. Natl Acad. Sci. U. S. A. 116, 16817–16822. doi: 10.1073/pnas.1904298116
Cassava Plus. (2021). GMO Cassava. Available online at: https://cassavaplus.org/news/kenya-citizentv-gmo-cassava (accessed March 20, 2022).
Chai, Q., Nemecek, T., Liang, C., Zhao, C., Yu, A., Coulter, J. A., et al. (2021). Integrated farming with intercropping increases food production while reducing environmental footprint. Proc. Natl Acad. Sci. U. S. A. 118, e2106382118. doi: 10.1073/pnas.2106382118
Drinkwater, L. E., Midega, C. A. O., Awuor, R., Nyagol, D., and Khan, Z. R. (2021). Perennial legume intercrops provide multiple belowground ecosystem services in smallholder farming systems. Agric. Ecosyst. Environ. 320, 107566. doi: 10.1016/j.agee.2021.107566
i5k (2022). i5k: Sequencing Five Thousand Arthropod Genomes. i5K. Available online at: http://i5k.github.io/ (accessed January 10, 2022).
Imoro, Z. A., Imoro, A. Z., Duwiejuah, A. B., and Abukari, A. (2021). Harnessing indigenous technologies for sustainable management of land, water, and food resources amidst climate change. Front. Sustain. Food Syst. 5, 282. doi: 10.3389/fsufs.2021.691603
Jemal, O. M., Callo-Concha, D., and van Noordwijk, M. (2021). Coffee agroforestry and the food and nutrition security of small farmers of south-western Ethiopia. Front. Sustain. Food Syst. 5, 293. doi: 10.3389/fsufs.2021.608868
Khajuria, C., Ivashuta, S., Wiggins, E., Flagel, L., Moar, W., Pleau, M., et al. (2018). Development and characterization of the first dsRNA-resistant insect population from western corn rootworm, Diabrotica virgifera LeConte. PLoS ONE 13, e0197059. doi: 10.1371/journal.pone.0197059
Knight, J. (2021). Environmental services: a new approach toward addressing sustainable development goals in sub-Saharan Africa. Front. Sustain. Food Syst. 5, 241. doi: 10.3389/fsufs.2021.687863
Koch, A., Biedenkopf, D., Furch, A., Weber, L., Rossbach, O., Abdellatef, E., et al. (2016). An RNAi-based control of Fusarium graminearum infections through spraying of long dsRNAs involves a plant passage and is controlled by the fungal silencing machinery. PLoS Pathog. 12, e1005901. doi: 10.1371/journal.ppat.1005901
Kuo, Y.-W., and Falk, B. W. (2020). RNA interference approaches for plant disease control. Biotechniques 69, 469–477. doi: 10.2144/btn-2020-0098
Lewin, H. A., Robinson, G. E., Kress, W. J., Baker, W. J., Coddington, J., Crandall, K. A., et al. (2018). Earth BioGenome Project: sequencing life for the future of life. Proc. Natl Acad. Sci. U. S. A. 115, 4325–4333. doi: 10.1073/pnas.1720115115
Lowder, S. K., Sánchez, M. V., and Bertini, R. (2021). Which farms feed the world and has farmland become more concentrated? World Dev. 142, 105455. doi: 10.1016/j.worlddev.2021.105455
Lowder, S. K., Skoet, J., and Raney, T. (2016). The number, size, and distribution of farms, smallholder farms, and family farms worldwide. World Dev. 87, 16–29. doi: 10.1016/j.worlddev.2015.10.041
Mishra, S., Dee, J., Moar, W., Dufner-Beattie, J., Baum, J., Dias, N. P., et al. (2021). Selection for high levels of resistance to double-stranded RNA (dsRNA) in Colorado potato beetle (Leptinotarsa decemlineata Say) using non-transgenic foliar delivery. Sci Rep. 11, 6523. doi: 10.1038/s41598-021-85876-1
Mitter, N., Worrall, E. A., Robinson, K. E., Li, P., Jain, R. G., Taochy, C., et al. (2017). Clay nanosheets for topical delivery of RNAi for sustained protection against plant viruses. Nat. Plants 3, 1–10. doi: 10.1038/nplants.2016.207
Niu, J., Yang, W.-J., Tian, Y., Fan, J.-Y., Ye, C., Shang, F., et al. (2019). Topical dsRNA delivery induces gene silencing and mortality in the pea aphid. Pest Manag. Sci. 75, 2873–2881. doi: 10.1002/ps.5457
Parker, K. M., Barragán Borrero, V., van Leeuwen, D. M., Lever, M. A., Mateescu, B., and Sander, M. (2019). Environmental fate of RNA interference pesticides: adsorption and degradation of double-stranded RNA molecules in agricultural soils. Environ. Sci. Technol. 53, 3027–3036. doi: 10.1021/acs.est.8b05576
Petek, M., Coll, A., Ferenc, R., Razinger, J., and Gruden, K. (2020). Validating the potential of double-stranded RNA targeting Colorado potato beetle mesh gene in laboratory and field trials. Front. Plant Sci. 11, 1250. doi: 10.3389/fpls.2020.01250
Pittelkow, C. M., Liang, X., Linquist, B. A., van Groenigen, K. J., Lee, J., Lundy, M. E., et al. (2015). Productivity limits and potentials of the principles of conservation agriculture. Nature 517, 365–368. doi: 10.1038/nature13809
Priyadarshana, T. S., Lee, M.-B., Ascher, J. S., Qiu, L., and Goodale, E. (2021). Crop heterogeneity is positively associated with beneficial insect diversity in subtropical farmlands. J. Appl. Ecol. 58, 2747–2759. doi: 10.1111/1365-2664.14005
Rank, A. P., and Koch, A. (2021). Lab-to-field transition of RNA spray applications – How far are we? Front. Plant Sci. 12, 2243. doi: 10.3389/fpls.2021.755203
Reich, J., Paul, S. S., and Snapp, S. S. (2021). Highly variable performance of sustainable intensification on smallholder farms: a systematic review. Glob. Food Sec. 30, 100553. doi: 10.1016/j.gfs.2021.100553
Ricciardi, V., Mehrabi, Z., Wittman, H., James, D., and Ramankutty, N. (2021). Higher yields and more biodiversity on smaller farms. Nat. Sustain. 4, 651–657. doi: 10.1038/s41893-021-00699-2
Rodriguez, C., Dimitrova Mårtensson, L.-M., Zachrison, M., and Carlsson, G. (2021). Sustainability of diversified organic cropping systems—Challenges identified by farmer interviews and multi-criteria assessments. Front. Agron. 3, 75. doi: 10.3389/fagro.2021.698968
Schulz, R., Bub, S., Petschick, L. L., Stehle, S., and Wolfram, J. (2021). Applied pesticide toxicity shifts toward plants and invertebrates, even in GM crops. Science 372, 81–84. doi: 10.1126/science.abe1148
Sgolastra, F., Arnan, X., Cabbri, R., Isani, G., Medrzycki, P., Teper, D., et al. (2018). Combined exposure to sublethal concentrations of an insecticide and a fungicide affect feeding, ovary development and longevity in a solitary bee. Proc. R. Soc. B 285, 20180887. doi: 10.1098/rspb.2018.0887
Simon-Delso, N., Martin, G. S., Bruneau, E., and Hautier, L. (2018). Time-to-death approach to reveal chronic and cumulative toxicity of a fungicide for honeybees not revealed with the standard ten-day test. Sci. Rep. 8, 7241. doi: 10.1038/s41598-018-24746-9
The Earth BioGenome Project (2018). Earth BioGenome Project. Available online at: https://www.earthbiogenome.org (accessed December 16, 2021).
United Nations (2022). Sustainable Development. Available online at: https://sdgs.un.org/ (accessed January 18, 2022).
United Nations Environment Programme Food Agriculture Organization of the United Nations (2020). The UN Decade on Ecosystem Restoration 2021-2030. Available online at: https://wedocs.unep.org/bitstream/handle/20.500.11822/30919/UNDecade.pdf?sequence=11 (accessed January 17, 2022).
Wagaba, H., Kuria, P., Wangari, P., Aleu, J., Obiero, H., Beyene, G., et al. (2021). Comparative compositional analysis of cassava brown streak disease resistant 4046 cassava and its non-transgenic parental cultivar. GM Crops Food 12, 158–169. doi: 10.1080/21645698.2020.1836924
Willow, J., Silva, A., Veromann, E., and Smagghe, G. (2019). Acute effect of low-dose thiacloprid exposure synergised by tebuconazole in a parasitoid wasp. PLoS ONE 14, e0212456. doi: 10.1371/journal.pone.0212456
Willow, J., Soonvald, L., Sulg, S., Kaasik, R., Silva, A. I., Taning, C. N. T., et al. (2021a). RNAi efficacy is enhanced by chronic dsRNA feeding in pollen beetle. Commun. Biol. 4, 1–8. doi: 10.1038/s42003-021-01975-9
Willow, J., Taning, C. N. T., Cook, S. M., Sulg, S., Silva, A. I., Smagghe, G., et al. (2021b). RNAi targets in agricultural pest insects: advancements, knowledge gaps, and IPM. Front. Agron. 3, 99. doi: 10.3389/fagro.2021.794312
Willow, J., and Veromann, E. (2021). Highly variable dietary RNAi sensitivity among Coleoptera. Front. Plant Sci. 12, 2914. doi: 10.3389/fpls.2021.790816
Worrall, E. A., Bravo-Cazar, A., Nilon, A. T., Fletcher, S. J., Robinson, K. E., Carr, J. P., et al. (2019). Exogenous application of RNAi-inducing double-stranded RNA inhibits aphid-mediated transmission of a plant virus. Front. Plant Sci. 10, 265. doi: 10.3389/fpls.2019.00265
Wytinck, N., Manchur, C. L., Li, V. H., Whyard, S., and Belmonte, M. F. (2020). dsRNA uptake in plant pests and pathogens: insights into RNAi-based insect and fungal control technology. Plants 9, 1780. doi: 10.3390/plants9121780
Yan, S., Qian, J., Cai, C., Ma, Z., Li, J., Yin, M., et al. (2020). Spray method application of transdermal dsRNA delivery system for efficient gene silencing and pest control on soybean aphid Aphis glycines. J. Pest Sci. 93, 449–459. doi: 10.1007/s10340-019-01157-x
Keywords: sustainable intensification, conservation agriculture, dsRNA, spray-induced gene silencing, transgenic, pesticide, integrated pest management, developing countries
Citation: Willow J and Veromann E (2022) Integrating RNAi Technology in Smallholder Farming: Accelerating Sustainable Development Goals. Front. Sustain. Food Syst. 6:868922. doi: 10.3389/fsufs.2022.868922
Received: 03 February 2022; Accepted: 30 March 2022;
Published: 25 April 2022.
Edited by:
Subhasis Mandal, Central Soil Salinity Research Institute (ICAR), IndiaReviewed by:
Durgesh K. Jaiswal, Savitribai Phule Pune University, IndiaCopyright © 2022 Willow and Veromann. This is an open-access article distributed under the terms of the Creative Commons Attribution License (CC BY). The use, distribution or reproduction in other forums is permitted, provided the original author(s) and the copyright owner(s) are credited and that the original publication in this journal is cited, in accordance with accepted academic practice. No use, distribution or reproduction is permitted which does not comply with these terms.
*Correspondence: Jonathan Willow, jonathan@emu.ee