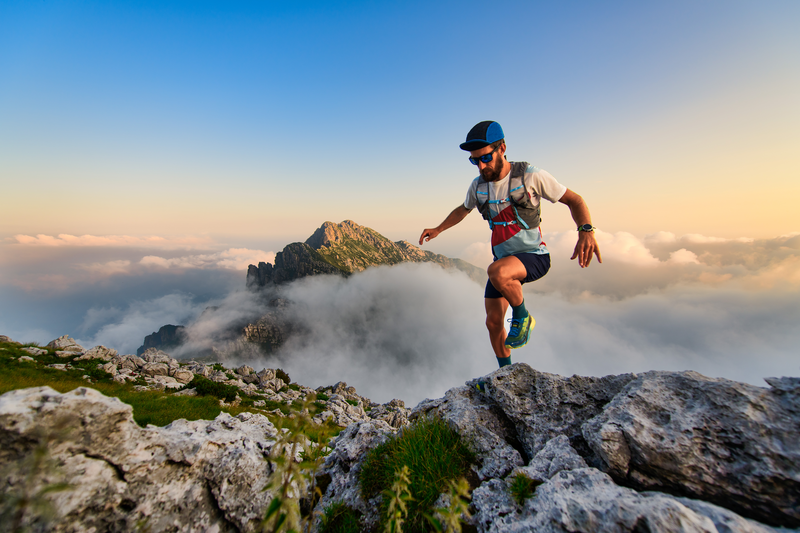
94% of researchers rate our articles as excellent or good
Learn more about the work of our research integrity team to safeguard the quality of each article we publish.
Find out more
ORIGINAL RESEARCH article
Front. Sustain. Food Syst. , 31 October 2022
Sec. Climate-Smart Food Systems
Volume 6 - 2022 | https://doi.org/10.3389/fsufs.2022.868479
This article is part of the Research Topic Climate Change, Land, Water and Food Security: Perspectives from Sub-Saharan Africa View all 14 articles
In rice production greenhouse gas emission (GHG) reduction is an important task for many countries, Tanzania included. Of global agricultural GHG emitted from rice fields, about 30 and 11% are represented by CH4 and N2O, respectively. For successful climate smart rice cultivation, rice management practices, including nitrogen fertilization are two key crucial components that need evaluation. The objective of this study was to evaluate the crop management practices and N fertilization on yield and greenhouse gases emission in paddy rice production, Experiments were designed in split-plot randomized complete block and replicated three times. Two rice management practices namely conventional practice (CP) and system of rice intensification (SRI) and six rates of nitrogen fertilizer (absolute control, 0, 60, 90,120 and 150 kg N ha−1) were applied in two consecutive seasons. The Source-selective and Emission-adjusted GHG CalculaTOR for Cropland (SECTOR) was used to calculate the GHG emission. Methane emission was in the range of 88.7–220.6 kg ha−1season−1, where higher emission was recorded in CP treatments (ABC, CP 0 and CP 120N) compared to SRI treatments. SRI reduced methane and carbon dioxide emission by 59.8% and 20.1% over CP, respectively. Seasonal nitrous oxide emissions was in the range of no detected amount to 0.0002 kgN2O ha−1 where SRI treatments recorded up to 0.0002 kgN2O ha−1 emissions while in CP treatment no amount of N2O was detected. The interaction of system of rice intensification and 90 kg N ha−1 (SRI90N) treatment recorded higher grains yield (8.1, 7.7 t ha−1) with low seasonal global warming potential (GWP) (3,478 and 3,517 kg CO2e ha−1) and low greenhouse gas intensity (0.42, 0.45 kg CO2e per kg paddy) compared to other treatments in wet and dry season, respectively. Therefore, SRI with 90 kg N was the treatment with mitigation potential and reduced GWP without compromising rice yield.
Global rice production is facing greatest challenge to meet an expected 34% increase in the world population by 2050 (Tilman et al., 2011; Alexandratos and Bruinsma, 2012; Tesfaye et al., 2021). Projected increases in the demand for rice will lead to increased application of fertilizers, particularly nitrogen-containing fertilizers and this will lead to increased greenhouse gas (GHG) emissions (van Beek et al., 2010; Van Groenigen et al., 2013; Arunrat et al., 2018).
Agriculture is the second major sector contributing to 24% of the global emissions next to the energy sector which contributes to 35% of GHG emission (Adounkpe et al., 2021; IPCC, 2014a). Agriculture contributes 14% of anthropogenic GHG emissions in the form of methane and nitrous oxide globally (IPCC, 2014a). At the same time agriculture can contribute to reduced net emission through bio-energy production and carbon sequestration. In Africa, between 1994 and 2014, the GHG emissions from agriculture increased at an average annual rate of between 2.9 and 3.1% (Tongwane and Moeletsi, 2018).
Paddy rice cultivation is one of the most important sources of anthropogenic emissions of greenhouse gases (GHGs), mainly nitrous oxide (N2O), methane (CH4), and carbon dioxide (CO2) (IPCC, 2014a; Arunrat et al., 2018) and is the major driving force for climate change (Smith et al., 2014). Rice (Oryza sativa L.) cultivation rank the second after enteric fermentation and is the leading agricultural sources of CH4, accounting for 22% of global anthropogenic agricultural emissions (Smartt et al., 2016). Paddy rice contributes 9–11% of the agricultural GHG emissions (IPCC, 2014a). Methane accounts for about 30% of the total global anthropogenic emissions (Gupta et al., 2021; Saunois et al., 2020). Eleven percent of global agricultural nitrous oxide (N2O) emissions come from rice fields (IPCC, 2007; Win et al., 2020; Zhang et al., 2021). Rice is the single crop grown under continuous flooded-soil conditions which contribute to the formation of the anoxic environment, this leads to the production and emission of CH4 (Smartt et al., 2016). CH4 and N2O are two major GHGs with a global warming potential (GWP) of 28 and 265 times that of CO2 in a 100-year time horizon, respectively (IPCC, 2014b). Global warming potential from rice cultivation has been reported to be 2.7 and 5.7 times greater than that of maize (Zea mays L.) and wheat (Triticum aestivum L.) systems, respectively, with CH4 specifically contributing more than 90% to the GWP of rice systems (Linquist et al., 2012).
Rice cultivation stimulates greenhouse gas (GHG) emissions from the soil into the atmosphere due to crop management practices such as irrigation water management, crop variety selection and fertilizer management, which in turn influences the biogeochemical processes of carbon and nitrogen in the soil (Islam et al., 2020). Methane is an end product of organic matter decomposition under anaerobic soil conditions (Linquist et al., 2012). Methane is produced by methanogens during organic matter decomposition, under an environment where the oxygen (O2) and sulfate (SO42−) are scarce and nitrous oxide is produced microbiologically during the an aerobic conditions of paddy soils (Bajgai et al., 2019). Large proportion of CH4 released from rice fields occurs through different ways such as aerenchyma tissues of rice plants, this transport mechanism contribute for about 90% of emissions, compared to 8.2% of emissions from ebullition and diffusion through the floodwater, respectively (Smartt et al., 2016).
Conventional practice (CP) coupled with continuous flooding irrigation and fertilizer application regimes, which is a common practice in Tanzania produces a huge amount of CH4 (Katambara et al., 2013; Boateng et al., 2019; Islam et al., 2020). This practice makes the soil environment anaerobic, by decreasing the redox potential (<-150 mV) there by results in the anaerobic degradation of complex organic substrates by methanogens and the production of CH4 (Islam et al., 2020). Various strategies for mitigating CH4 emission from rice cultivation include water management practices, particularly promoting intermittent drainage and alternate wetting and drying (AWD) (Minamikawa and Yagi, 2009), system of rice intensification (SRI) (Gathorne et al., 2013); improving organic management by composting; using rice cultivars with few unproductive tillers, high root oxidative activity and high harvest index (Zheng et al., 2014); application of fermented manure like biogas slurry (Petersen, 2018) and adopting direct-seeding of rice (DSR) (Susilawati et al., 2019). Methane emissions differ across agro climate (rice growing seasons), soil types, locations (due to difference in organic carbon) (Gaihre et al., 2011; Datta et al., 2013; Sun et al., 2013).
Previous studies have shown that effective fertilizer and water management practices under system of rice intensification coupled with alternate wetting and drying irrigation could reduce GHG emissions by 40% (Ku et al., 2017; Li et al., 2018; Islam et al., 2020; Ramesh and Rathika, 2020; Sander et al., 2020; Win et al., 2020). Alternate wetting and drying irrigation greatly enhances the diffusion of atmospheric oxygen (O2) into the soil, thus reducing the emission of CH4 (Yang et al., 2012; Xu et al., 2015; Islam et al., 2020). Slight increase N2O emission in AWD irrigation has been reported by Islam et al. (2020), Ku et al. (2017), Li et al. (2018) due to the increased nitrification of NH4+ during the dry episode and the subsequent denitrification of NO3- during re-wetting of dry soils, but it still reduces total GHG emissions from rice fields mainly due to reduced CH4 emissions. Reducing the emission of CH4 from the soil is the most effective way to mitigate the global warming potential in rice cultivation (Sander et al., 2014; Janz et al., 2019).
Mineral nitrogen fertilizer is very important and highest input in croplands, making almost half of global nitrogen input (Liu et al., 2010). Mineral nitrogen use is more widespread in West Africa compared with East and Southern Africa, which are the two major rice-producing regions in SSA (Tsujimoto et al., 2019). Surveys conducted on large-scale irrigation schemes, reported significantly low N application rates in Uganda (~2 kg ha−1), Mozambique (13–23 kg ha−1), and Tanzania (15–22 kg ha−1) compared to the rates in Burkina Faso, Mali, Niger, and Senegal (>100 kg ha−1) (Nakano et al., 2011; Nakano and Kajisa, 2013). Nhamo et al. (2014) also reported that fertilizer application rates were commonly 5–20 kg ha−1 for lowland rice production in East and Southern Africa. In 11 countries of west Africa a cross-sectional survey of 1,368 rice fields reported that mineral N fertilizer was used in 81% of irrigated lowland fields (with average application: 100 kg ha−1), 56% of rainfed lowland fields (65 kg ha−1), and 38% of rainfed upland fields (37 kg ha−1) (Niang et al., 2017). The average N application rate for the irrigated lowland fields in this survey is comparable with the average value for countries in Southeast Asia (FAO, 2002). Other studies have also reported relatively high N application rates in irrigated lowland fields in West Africa, for example, in a range of 72–112 kg ha−1 in Benin (Tanaka et al., 2013), 134–139 kg ha−1 in the Senegal River Valley (Tanaka et al., 2015), 37–251 kg N ha−1 in Mauritania (Haefele et al., 2001), and 73–147 kg ha−1 in Burkina Faso, Mali, and Senegal (Wopereis et al., 1999). The yield gap analysis verified equal or slightly greater yield potential and greater yield gaps of irrigated rice production, that is, large opportunities of yield increases with fertilizer inputs still remain in both Madagascar and Tanzania than most areas in West Africa (van Oort et al., 2015; Tanaka et al., 2017).
Though, only a portion of applied reactive nitrogen (N) is converted into food, the remaining is lost through various pathways like, denitrification, nitrate leaching and ammonia volatilization (Cassman et al., 2002; Tilman et al., 2002; Tesfaye et al., 2021). Various studies have reported that nitrous oxide (N2O) emissions are associated with nitrogen (N) fertilizer application and dry land conditions (Arunrat et al., 2018) while flooded fields are a significant source of methane (CH4) and contribute little to N2O emissions (Shang et al., 2011; Wang et al., 2011; Yao et al., 2012).
During the Paris Climate Agreement of 2015, countries agreed to limit global temperature increase to below 1.5°C by reducing GHG emissions and are responsible to report their nationally determined contributions (NDCs) (UNFCCC, 2015; Gyanchandani, 2016; Elkahwagy et al., 2017). Like other countries in the world, Tanzania is looking for the best GHG mitigation options across all sectors, including paddy rice cultivation in agriculture sector. Although the Tanzanian Government is committed to (UNFCCC) and desires to minimize GHG emissions while promoting irrigated rice production, data on GHG emissions continue to be a challenge although this information is required in the Nationally Determined Contributions (NDCs) and climate change mitigation options. Indeed, the measurement lapse is a challenge to many developing countries especially in Africa (Nyamadzawo et al., 2013; Rosenstock et al., 2016; Boateng et al., 2017; Pelster et al., 2017; Zheng et al., 2019; Bigaignon et al., 2020). Current understanding of GHG emissions in sub Saharan Africa (SSA) is particularly limited when compared to the potential of the continent (Nyamadzawo et al., 2013; Kim et al., 2015; Boateng et al., 2017, 2020; Tongwane and Moeletsi, 2018; Bigaignon et al., 2020; Owino et al., 2020). This indicates that more research is thus needed in this regard to investigate the effects of crop management practices and nitrogen fertilization levels on greenhouse gas emissions from rice field. In line with this, it is hypothesized that the combination of system of rice intensification and optimum nitrogen would reduce greenhouse gas emissions and improve rice yield in irrigated lowland rice. To test this hypothesis, a study was conducted to evaluate the crop management practices and N fertilization on yield and greenhouse gases emission in paddy rice production.
The field experiments were conducted at Mkindo farmer managed irrigation scheme located in Mkindo village in Hembeti Ward, Mvomero District, Morogoro Region, Eastern Tanzania. The district is located between latitude 6°16' and 6°18'South, and longitude 37°32' and 37°36' East and its altitude ranges between 345 to 365 m amsl. The experimental site located at latitude 6°15'13” south and longitude 37°32'19”. Mkindo farmer managed irrigation scheme is about 85 km from Morogoro municipality (Gowele et al., 2020). The scheme was constructed in the period between 1980 and 1983. The scheme started producing rice in 1985 with only 17 ha under cultivation. Rice is the only crop produced in the scheme which serves as food and income generation. The scheme has arable area of 740 ha, with only 300 ha under rice cultivation and a near future expansion of about 620 ha is expected. The climate is tropical with two distinct seasons, dry and wet seasons. The average monthly maximum temperature at the experimental site ranges between 35.1 to 28.5°C for February and June while the average monthly minimum temperature ranges between 20.4 to 15.8°C for January, March and July, respectively. The average temperature and rainfall of Mkindo Climatic conditions for 21 years (1999–2020) has been reported in Table 1.
Portions of the soil samples were dried, ground and sieved through a 2 mm sieve for physico-chemical characterization. Parameters measured were soil pH, particle size distribution, organic carbon and extractable phosphorus (AvP). Other parameters included total nitrogen, basic cations such as calcium(Ca), magnesium, potassium and sodium and micronutrients namely zinc (Zn), copper (Cu), manganese (Mn) and iron (Fe). Cation exchange capacity (CEC) and electrical conductivity (EC) were also determined for all the samples and the analysis followed the standard procedures as shown in Table 2 and results are shown in Table 3.
Table 3. Selected soil chemical properties of Mkindo Irrigation scheme at 0–20 cm of soil used in the study.
Each season, the experiment was arranged in a split-plot randomized complete block design in triplicate with two factors (crop management practices in main plots and nitrogen rates in sub-plots). The main plot was then divided into six subplots of size 16 m2 plots were surrounded by consolidated bunds, and a 2 m buffer strips were left between main plots and 1 m for sub plots to provide access pathways and more importantly to minimize lateral movement of irrigation water and fertilizers between plots. The treatment details are shown in Table 4. Fertilizer treatments comprised six nitrogen rates; these include absolute control treatment (ABC) which did not receive any kind of fertilizer. The absolute control treatment intended to evaluate rice response under natural soil fertility. The N fertilizer treatments included a control treatment (N0) without any nitrogen fertilizer application but received P and K fertilizers, this treatment is required to assess crop response to nitrogen fertilizer application and to calculate fertilizer use efficiency. The amount of 120 kg N ha−1, represents the current blanket recommendation for rice grown in Mkindo. The nitrogen fertilizer source was Urea (CON2H4, total nitrogen 46% N) and was applied in two splits, that is half 2 weeks after transplanting and the rest half at 55 days. Sources of full dose of phosphorus 60 kg ha−1 was triple super phosphate (45% P2O5) and potassium 60 kg ha−1 from muriate of potash (60% K2O). Phosphorus and potassium fertilizers were applied at same rate to all plots during transplanting.
A common variety TXD 305 was used. The trial rice variety takes 120–130 days to maturity under rainfed or irrigated ecologies. In establishing system of rice intensification SRI crop management practice plots, during transplanting a square grid pattern was created on the soil's surface using a wooden marker that demarcated distances of 25 × 25 cm between perpendicular lines. A 10-days-old seedlings were uprooted from nursery and transplanted one seedling per hill within 30 min of uprooting in both seasons. During weeding rotary (cono-weeder) and hand were used. Based on results in an earlier studies with the same rice variety under same area (Mkindo Irrigation scheme condition) conducted by Kahimba et al. (2013), Reuben et al. (2016) we decided to adopt the SRI recommended principles of spacing, seedling age, weeding and irrigation interval. In conventional practice (CP) crop management a 25-days-old seedlings were transplanted in puddled field at 20 × 20 cm spacing keeping three seedlings hill−1, hand weeding was used in weed management as shown in Table 5.
Application of continuous flooding irrigation was based mainly on local farmers' practice in CP plots. For the first 14 days after transplanting, a 3–5 cm water depth was maintained under both irrigation regimes to facilitate seedling recovery. Thereafter, SRI and CP plots were managed differently. Plots under CP were continuously flooded with a 3–10 cm water level until 10 days before harvest. After the first 14 days of transplanting the SRI plots were kept with a layer of 2 cm of water until 14 days after panicle initiation stage, and during the rest of the growing cycle, plots were maintained without standing water for 3–5 days before re-irrigation. Thereafter, the SRI plots were re-irrigated to 2 cm when water depth dropped to 15 cm below the soil; this took 2–3 days interval (Kahimba et al., 2013). The soil water depths were measured and monitored in each SRI plot using PVC pipe installed in the plots at a 15 cm depths as described by Lampayan et al. (2015). The water depth was measured at 8:00 am and 14:00 pm each day using a 101 p7 flat tape water level meter (Solinst Canada Ltd., Geogetown, Ontario Canada). PVC pipes installed in SRI plots, with perforated holes with a diameter of about 0.5 cm each and spaced about 2 cm away from one another. The tube was buried vertically 15 cm into the soil and half of its length protruding above the soil surface. Pipes were installed near to the bund for easy water monitoring. After burying the PVC pipes the soil inside the tubes was removed so as bottom level is visible. After tube installation the water level inside the tube was checked and was the same the outside. Each of the main plots was irrigated separately. Irrigation water was provided from an irrigation canal and measured by a plastic ruler inserted into the plot.
At physiological maturity, grain yields were determined from a (2 × 2 m) = 4 m2 crop cut at the center of each field leaving a border rows. The rice plants in each plot were manually harvested and threshed separately. The harvested samples were threshed, cleaned, and sun dried for 2–3 days to a constant weight to obtain their dry weight. The moisture content of the dried grains was measured using a grain moisture meter (8988N Xiamen Hyhoo Imp. & Exp. Co., Ltd., Fujian, China). Finally rice grain yields were calculated based on standard moisture (14%) for rice storage.
Intergovernmental Panel on Climate Change (IPCC) guidelines are applied through GHG calculators during assessment of GHG at national and subnational levels. Such calculators include SECTOR tool (Wassmann et al., 2019; Lai et al., 2021), EXACT tool (Grewer et al., 2013, 2016, 2018), the Cool Farm Tool (Hillier et al., 2011, 2013; Vetter et al., 2018).
In this study Source-selective and Emission-adjusted greenhouse gas CalculaTOR for Cropland (SECTOR) was used to calculate the emission of greenhouse gases. SECTOR is a greenhouse gas (GHG) calculator for cropland based on the values calculated from Intergovernmental Panel on Climate Change (IPCC) Tier 2 approach approved methodology (I. P. O., 2006) and (IPCC, 2019) refinement (Wassmann et al., 2019). SECTOR is guided by Tier 2 requirements and approach as shown in Table 6. This tool was developed by the International Rice Research Institute's (IRRI) GHG Mitigation in Rice Platform. Presently this tool is available in excel and requires inputs from the user on cropping area, yield, and management practices. SECTOR has been developed in response to increasing interest in mitigation studies in cropland, in particular rice production. These include the farm-diary data (Tables 7, 8) recorded such as:
a) Pre-season water management (number of days of flooding prior to crop establishment).
b) Number of days of crop growth (starting at transplanting stage).
c) Number and duration of drying events (the number of times when the water depth falls at least 10 cm below the soil surface; or the number of times in which the soil dries to the point of light cracking).
d) Total nitrogen input.
e) Water management before and during the growing season.
f) Residuals management.
The tool offers a high range of flexibility in terms of sourcing emission and activity data as well as selecting a range of scales for aggregation. Moreover, SECTOR provides a streamlined framework for accelerated data input that will facilitate rapid assessments of multiple scenarios for domains with many spatial units. Also SECTOR can easily be adjusted to incorporate new emission factors and calculation procedures expected in forthcoming revisions of the IPCC Guidelines. This tool is available as an XLS file and can be downloaded jointly with its manual from http://climatechange.irri.org/SECTOR.
Table 6. Overview of specific Tier 2 requirements for GHG calculations in cropland within mitigation projects and approach in SECTOR.
Table 8. Yield, season length, nitrogen rate and water management used as input values in SECTOR tool.
In this study IPCC factors were used to calculate the combined GWP for 100 years [GWP = (CH4 x 25 + N2O x 298), kg ] equivalents ha−1 from methane and nitrous oxide.
The Greenhouse gas intensity (GHGI) was calculated by dividing global warming potential (GWP) by grain yield (Ali et al., 2019).
Analysis of variance was performed on yield for all treatments over the total growth period of both seasons using the Genstart software 14th version. Global warming potential (GWP) of CH4 and N2O was calculated in mass of CO2 equivalent (kg CO2 eq ha−1) over 100-yr time horizon. A radiative forcing potential relative to CO2 of 25 for CH4 and 298 for N2O (Myhre et al., 2013; Ali et al., 2019) was used. Anova tables are presented in Supplementary materials. Greenhouse gas intensity/yield-scaled global warming potential (GHGI/GWPY) was calculated by taking the ratio of GWP and corresponding grain yield for each treatment. These results were generated direct from the SECTOR calculator.
Methane emission in this study was in the range of 88.7–220.6 kg ha−1 season−1, where higher emission recorded in conventional treatments (CP -ABC, CP- 0N and CP- 120 N) (Table 9). SRI reduced methane emission by 59.8% over CP. Relatively low amount of CH4 emission from SRI treatments was due to partially aerobic soil conditions because of alternate wetting and drying water management cycles employed during experiment. Alternate wetting and drying irrigation water management under SRI greatly enhances the diffusion of atmospheric oxygen (O2) into the soil, thus reducing the activity of CH4 producing bacteria (Yang et al., 2012; Xu et al., 2015; Islam et al., 2020). Aeration makes the soil environment oxic, which results in the oxidation of CH4 by the methanotrophs, causing a decrease in CH4 emission. It is reported that up to 80% of the CH4 produced during the rice-growing season is oxidized by the methanotrophs (Islam et al., 2020).
Table 9. Seasonal methane and carbon dioxide emission as influenced by crop management practices and nitrogen fertilization rates.
These results are in agreement with those of Corton et al. (2000), who conducted 9 experiments for 5years and found the CH4 emission at a given treatments was higher during the wet season by 2 to 3 times the emission during the dry season. The methane emission was in the range of 67–120 kg CH4-C ha−1 in dry season and 200–389 kg CH4-C ha−1 in wet season. According to studies conducted by Hidayah et al. (2009) in Indonesia and Jain et al. (2014) in India the SRI methods reduced the methane emission up to 60 and 64%, respectively compared to conventional puddled transplanted rice.
Increased methane emission in CP treatments was due to formation of anoxic condition due to flooding moisture condition. Anoxic condition results in decreasing redox potential (−150 mV), which leads to the anaerobic decomposition of complex organic substrates by methanogens that finallydrive CH4 production (Islam et al., 2020). Higher methane emission in CP may also be due to availability of organic substrate from root exudates and the reducing condition in the rice rhizosphere (Jain et al., 2014). The available organic carbon from root exudates increases the population of methanogen in flooding condition (Kumaraswamy et al., 2000).
Researchers around the global have reported different amount of methane emission from rice cultivation; this could be due to different in climate, soils, water management, verities, cultivars, fertilizer management and others. A study by Kim et al. (2012) reported the low seasonal methane emission of 126.8 from SRI plots compared to 458.4 kg C ha−1 from conventional flooding. According to Jain et al. (2014), the cumulative emission of CH4 during the cropping period was lowest (8.16 kg ha−1) in the SRI and the highest (22.59 kg ha−1) in conventional method of transplanting method.
Seasonal carbon dioxide emissions ranged from 0.0 to 1,034 kg ha−1season−1 in both season and was significantly affected by treatment interaction (Table 9).CPN0 and CP120N had the highest seasonal cumulative flux and was significantly different from all other treatments. Except the treatment, CP60N recorded no seasonal flux for CO2. System of rice intensification reduced CO2 emission by 25%. Yearly CO2 emission was higher in conventional treatments compared to SRI treatments. Carbon dioxide emissions are influenced by the crop residue and litter content, root activities, and microbial processes because the soil carbon pool is converted into CO2 by the action of soil microorganisms. In the availability of water and urease enzymes, urea fertilizer applied in the fields converted to , OH–, and HCO3, and this bicarbonate finally evolves into CO2 and water (Hussain et al., 2015; Gupta et al., 2021).
Seasonal nitrous oxide emission was in the range of no detected amount to 0.0002 kgN2O ha−1 (Table 10). There were similar trends in emissions in both seasons where the emission of up to 0.0002 kgN2O ha−1 was recorded in SRI treatments. Whereas SRI ABC and SRI0 treatments no amount of nitrous oxide captured by the tool. The tool captured the zero amounts (0.0000 kg ha−1) in the CP treatment. Results are in agreement with that of Karki et al. (2021) in their study reported that N2O emissions are generally low in flooded rice fields as most of the nitrogen is lost as N2 rather than N2O.
Table 10. Seasonal nitrous oxide emission (kg N ha−1 season−1) as affected by management practices and nitrogen rates.
Zero N2O emissions in conventional practice could be contributed by immobilization and retention of N fertilizer in soil (Fuhrmanna et al., 2018). The zero N2O fluxes could also be due to some of the nitrogen being lost through leaching thus reducing amount of nitrogen substrate available for N2O emissions. Owino et al. (2020) in their study in Kenya also observed insignificant N2O emissions during rice growing season when the soil was flooded. This could be due to formation of anoxic conditions in the flooded paddies which create suitable conditions for denitrification with major product of this process being nitrogen gas (N2).
In this study the relative amount of N2O (0.0002 kgN2O ha−1) was recorded in SRI treatments, this could be due to the effect of alternate wetting water management regime that allow the introduction of oxygen when the field is free from flooded water, aerobic soil conditions significantly reduce CH4 emission (Linquist et al., 2015; Lagomarsino et al., 2016; Jiang et al., 2019; Karki et al., 2021).
Studies have reported increased nitrous oxide emission from SRI treatments due to the general relationship between N2O and CH4, that when fields are saturated CH4 increases, CO2 and N2O decreases. However, when fields become drier, CH4 emissions decrease and CO2 and N2O emissions increase. Slight increase N2O emission in field managed under alternate wetting and drying irrigation has been documented (Ku et al., 2017; Li et al., 2018; Islam et al., 2020). This is due to the increased nitrification of ammonium during the dry period and the subsequent denitrification of NO3- during re-wetting of dry soils, but the GHG emission still reduced due to reduction in methane emission. Jain et al. (2014) reported increase of emission of N2O–N by an average of 22.5% in SRI methods over conventional transplanted method.
Variable range of nitrous oxide emission in rice ecosystems has been reported by scholars this could be due to different in climate, management practices, different in soils, fertilization programs, varieties and other factors.
Seasonal nitrous oxide emission of 0.000028 kg N2O ha−1 from conventional plots and 0.074 kgN2O ha−1 from SRI plots was reported by Kim et al. (2012) in Korea under nine season experiments. According to Jain et al. (2014) the seasonal integrated fluxes of N2O– N were 0.69 and 0.90 kg ha−1 from conventional transplanted rice and SRI planting methods, respectively. Boateng et al. (2020) conducted a study in Ghana and reported the seasonal N2O emissions ranged from 1.61 to 58.08 kg N2O ha−1, and Gitonga (2020) conducted a study in Kenya and found the seasonal N2O emissions ranged from 0.18 to 1.29 kgN2O ha−1. Hadi et al. (2010) in Indonesia reported the average N2O emissions of 1.97 from intermittently drained plots and −19.7 kgN2O ha−1 from continuously flooded plots respectively.
Global warming potential (GWP) was significantly p < 0.001 affected by combined treatments of crop management practices and nitrogen fertilization rates and was high in CP treatments in both seasons (Table 11). The GWPs in this study are in range of 3,114.0–7,843 kg CO2-e ha−1season−1, these range are within the range reported in other areas. Higher amount of 7,843, followed by 7,780 kg CO2e ha−1 season−1 was recorded in CP120N and CP NO respectively. Low GWP of 3,114.0 and 3,367 kg CO2e ha−1 season−1 was recorded in CP 60N and SRIABC treatments. SRI lowered the GWP significantly due to low methane emission compared to the CP method. The reduction of GWP of up to 57.1% was recorded in SRI treatments over CP treatments. These results confirm that the total GWP in rice fields is solely determined by CH4 emission. However the radiative forcing of N2O is much higher than CH4, but the magnitude of N2O emissions is very small. Thus, CH4 is the major contributor of GWP in rice cultivation, representing over 90% of the total GWP (Sander et al., 2014; Janz et al., 2019; Islam et al., 2020). The reduction of GWP has been reported in SRI methods by Jain et al. (2014) reported the reduction of 29% in SRI methods over the transplanted puddled rice method.
Table 11. Global warming potential, grain yield and greenhouse gas intensity as affected by management practices and nitrogen.
Pramono et al. (2020) reported the highest GWP of 8,270 kg of CO2-e ha−1season−1 and lowest GWP of 4,240 kg ha−1 season−1. Hadi et al. (2010) reported Seasonal Global warming potential ranged from 10,162–38,381 GWP (kg C-CO2 eq ha−1 season−1).
Greenhouse gas intensity (GHGI) was in the range of 0.42–2.04 kg CO2e kg−1 paddy, these are within the range reported by Ali et al. (2019). SRI 90 and SRI 150 treatments recorded low amount (0.42 kg CO2e kg−1 paddy) and CP ABC recorded higher GHGI of 2.04 kg CO2e kg−1 paddy. SRI lowered the GHGI significantly due to low methane emission compared to the CP method.
These results are in agreement with Win et al. (2020) who reported significant the range of GHGI values (1.4–7.4 kg CO2e kg−1 paddy) under continuous flooding than under alternate to wetting and drying irrigation. Zhang et al. (2016) reported the GHGIs (kg CO2 eq. t−1 grain) ranged from 712 to 1,245 kg CO2. t−1 grain.
The interaction of crop management practices and the nitrogen fertilization rate did not affect yield significantly (p > 0.05), however there was percentage increase in grain yield of 44 and 61 in SRI plants during wet and dry season respectively (Table 11). The average grain yield in treatment interaction was in the range of 4.5–8.1 t ha−1 and 3.0–7.7 t ha−1 during wet and dry season respectively. Average grain yield in SRI treatments (SRI-60N, SRI-90N, SRI-120N, SRI-150N) recorded the yield potential range for TXD 306 variety which (7–8 t ha−1) during wet season, while in CP only CP120N treatment reached the yield potential.
The increment in grain yield in the SRI treatment was largely attributed to increases in the number of spikelets per panicle and filled grain percentage (Table 12), the same was reported by other scholars. The yield in SRI plants could also be linked with the root characteristics i.e., higher volume and root weight (data not shown). Our results are in agreement with previous studies of other researcher's, such as Sandhu et al. (2017) reported strong association of root traits such as nodal root number, root dry weight with grain yield. Ashraf et al. (1999) reported that old seedlings results in CP practice lower rice yields because they suffer from stem and root injury during pulling. Previous studies in the study area with the same variety have reported different rice yield. Kahimba et al. (2013) reported 2.96–4.76 t ha−1 and yield increased by 24.3% in SRI compared to conventional practices and Reuben et al. (2016) reported grain yield ranged in 8.1–8.5 t ha−1 under SRI with the same variety.
Table 12. Effects of crop management practices and fertilizer N levels on panicle components of rice.
Thakur et al. (2014) found overall, grain yield with SRI was 49% higher than with CP, with yield enhanced at every N application dose. Thakur et al. (2021) reported increased rice yield by SRI up to 25–50% or more and Mati et al. (2021) in Kenya reported the increased rice yields in the range of 20–100%.
The number of panicles per hill was significant with SRI recording 37 and 22% higher than the CP in wet and dry seasons. Nitrogen levels and interactions with SRI or CP significantly affected the number of panicles (Table 12). The higher panicle weight percentages of 22.2 and 43.6% were recorded under SRI in wet and dry seasons, respectively. Panicle weight increased with an increase in N levels but not beyond 120 kg N−1 in wet season and 90 kg N ha−1 dry season. Panicle length was significantly (p < 0.05) affected by N levels and the length increased with increasing N levels in wet season. The number of panicle per hill was significantly (p < 0.05) affected by crop management practices in wet season, with SRI recording higher number of panicle per hill (15) compared with CP (9). Nitrogen levels and their interactions with SRI or CP significantly affected the number of panicles per hill. Spikelets per panicle were significantly influenced by crop management practices, with SRI recording higher number of spikelets per panicles in wet and dry seasons. Nitrogen levels also significantly affected the number of spikelets per panicle during dry season.
Effective tillers were significantly affected by CP, N levels and their interactions (p < 0.05) in wet and dry seasons but SRI recorded higher effective tillers over CP (Table 12). The filled grains per panicle were significantly affected by crop management practices (p < 0.05) in wet and dry seasons. Grain filling rate was significantly affected by crop management practices in wet season, with increased grain filling by 4.6 and 5.9% under SRI compared with CP in wet and dry season, respectively. There was significant effect of N levels in dry season. Previous studies have reported absence of significant effect of crop management practices on percentage of filled grains (Belder et al., 2004; Zheng et al., 2020).
The CMP significantly affected straw yield during dry season and SRI recorded increased straw yield by 33.3% over CP (Table 13). Straw yield increased with increase in N levels in wet and dry seasons. The highest straw yield was recorded in wet season (6.6 and 6.5 t ha−1) in an application of 150 Kg N ha−1, and with interactions of SRI and CP with 150 kg N ha−1. Harvest index (HI) was significantly affected by N levels during wet season, whereas the lowest HI of 0.5 was recorded in an application of 150 kg N ha−1. There was no interaction effects observed between treatments on the straw yields. Results also indicated that the dry weight of 1,000-grains was significantly affected by CP in wet season. However, there was no significant effect of N levels or their interactions with CP or SRI observed on the dry weight of 1,000 grains. Crop management practices significantly affected panicle weight and spikelets per panicle in wet and dry seasons, with higher values recorded under SRI.
Table 13. Effects of crop management practices and N levels on straw yield, harvest index, grain yield and 1,000 grains weight of rice.
Our results show that the treatments interaction of system of rice intensification and nitrogen rates significantly decreased CH4 and CO2 emissions from paddy rice in either rice seasons.
Conventional practice contributed to higher GWP and GHGI. System of rice intensification treatment reduced global warming potential, methane and carbon dioxide by 57.1, 59.2, and 25% over CP treatments, respectively. System of rice intensification and nitrogen fertilization at 90 kg N ha−1 could be practiced to sustain increase rice productivity while minimizing greenhouse gases intensity in the changing climatic conditions.
Our results suggest strong potential for system of rice intensification management practice to reduce the total GHG emissions from paddy rice, while maintaining rice yield.
The original contributions presented in the study are included in the article/Supplementary material, further inquiries can be directed to the corresponding author/s.
PAM set and managed experiments, collected data, and analyzed and wrote the first draft of manuscript. KK and AA reviewed and edited the manuscript. PM collected data, managed experiment, and reviewed first draft of manuscript. All authors have read and agreed to the published version of the manuscript.
We acknowledge the Center of Excellence Project: IDA Credit 5794-ET under the frame of Africa Centre of Excellence for supporting a PhD study in Climate Smart Agriculture and Biodiversity Conservation. We thank the Mkindo rice farmers for allocating the land as well as hosting our experiments. We also thank Ibrahim Paul, Nasoro Mohamed and all team for their field data collection.
The authors declare that the research was conducted in the absence of any commercial or financial relationships that could be construed as a potential conflict of interest.
All claims expressed in this article are solely those of the authors and do not necessarily represent those of their affiliated organizations, or those of the publisher, the editors and the reviewers. Any product that may be evaluated in this article, or claim that may be made by its manufacturer, is not guaranteed or endorsed by the publisher.
The Supplementary Material for this article can be found online at: https://www.frontiersin.org/articles/10.3389/fsufs.2022.868479/full#supplementary-material
Adounkpe, J., Ahoudji, O., and Sinsin, B. (2021). Assessment of the contribution of flooded rice cultivation systems to methane emissions in the lower Ouémé Valley, in Benin Republic. J. Agric. Chem. Environ. 10, 327–344. doi: 10.4236/jacen.2021.103021
Alexandratos, N., and Bruinsma, J. (2012). World agriculture towards 2030/2050: the 2012 revision, world agriculture towards 2030/2050: the 2012 revision. ESA Working Paper No. 12-03. Rome: FAO.
Ali, M. A., Inubushi, K., Kim, J., and Amin, S. (2019). Management of paddy soil towards low greenhouse gas emissions and sustainable rice production in the changing climatic conditions. Soil Contam. Altern. Sustain. Develop. 1–19.
Arunrat, N., Sereenonchai, S., and Pumijumnong, N. (2018). On-Farm evaluation of the potential use of greenhouse gas mitigation techniques for rice cultivation: A case study in Thailand. Climate 6, 36. doi: 10.3390/cli6020036
Ashraf, M., Khalid, A., and Ali, K. (1999). Effect of seedling age and density on growth and yield of rice in saline soil. Pakistan J. Biol. Sci. doi: 10.3923/pjbs.1999.860.862
Bajgai, Y., Yeshey, Y., De Mastro, G., Ghimiray, M., Chhogyel, N., Tshewang, S., et al. (2019). Influence of nitrogen application on wheat crop performance, soil properties, greenhouse gas emissions and carbon footprint in central Bhutan. Environ. Develop. 32, 100469. doi: 10.1016/j.envdev.2019.100469
Belder, P., Bouman, B. A. M., Cabangon, R., Guoan, L., Quilang, E. J. P., Yuanhua, L., et al. (2004). Effect of water-saving irrigation on rice yield and water use in typical lowland conditions in Asia. Agric. Water Manag. 65, 193–210. doi: 10.1016/j.agwat.2003.09.002
Bigaignon, L., Delon, C., Ndiaye, O., Galy-Lacaux, C., Serça, D., Guérin, F., et al. (2020). Understanding N2O emissions in african ecosystems: assessments from a semi-arid savanna grassland in senegal and sub-tropical agricultural fields in Kenya. Sustainability 12, 8875. doi: 10.3390/su12218875
Boateng, K. K., Obeng, G. Y., and Mensah, E. (2017). Rice cultivation and greenhouse gas emissions: a review and conceptual framework with reference to Ghana. Agriculture 7, 7. doi: 10.3390/agriculture7010007
Boateng, K. K., Obeng, G. Y., and Mensah, E. (2019). “Agricultural Greenhouse Gases from Sub-Saharan Africa,” in Greenhouse Gas Emissions, (Singapore: Springer), 73–85. doi: 10.1007/978-981-13-3272-2_6
Boateng, K. K., Obeng, G. Y., and Mensah, E. (2020). Eco-friendly yield and greenhouse gas emissions as affected by fertilization type in a tropical smallholder rice system, Ghana. Sustainability 12, 10239. doi: 10.3390/su122410239
Bray, R. H., and Kurtz, L. T. (1945). Determination of total, organic and available forms of phosphorus in soil. Soil Sci. 59, 39–45.
Bremner, J. M., and Mulvaney, C. S. (1982). “Total nitrogen,” in Methods of Soil Analysis; Part Agronomy Monograph 9 (Madison, WI: American Society of Agronomy), 1149–1170.
Cassman, K. G., Dobermann, A., and Walters, D. T. (2002). Agroecosystems, nitrogen-use efficiency, and nitrogen management. AMBIO 31, 132–140. doi: 10.1579/0044-7447-31.2.132
Chapman, H. D. (1982). “Cation-exchange capacity,” in Methods of Soil Analysis Part 3: Chemical Methods, 5th Edn, ed D.L. Sparks (Madison, WI: Soil Science of America Inc), 891–901.
Corton, T. M., Bajita, J. B., Grospe, F. S., Pamplona, R. R., Assis, C. A., Wassmann, R., et al. (2000). Methane emission from irrigated and intensively managed rice fields in Central Luzon (Philippines). Nutr. Cycl. Agroecosyst. 58, 37–53. doi: 10.1023/A:1009826131741
Datta, A., Yeluripati, J. B., Nayak, D. R., Mahata, K. R., Santra, S. C., and Adhya, T. K. (2013). Seasonalvariation of methaneflux from coastal saline ricefield with the application of differ-ent organic manures. Atmos. Environ. 66, 114–122. doi: 10.1016/j.atmosenv.2012.06.008
Elkahwagy, R., Gyanchandani, V., and Piselli, D. (2017). UNFCCC nationally determined contributions: climate change and trade. Center for Trade and Economic Integration (CTEI) Working Paper. doi: 10.2139/ssrn.2919692
FAO (2002). Fertilizer Use by Crop (5th ed.). Rome: FAOSTAT. Available online at: http://www.fao.org/faostat/en/ (accessed November 2020).
Fuhrmanna, I., He, Y., Lehndorff, E., Brüggemann, N., Amelung, W., Wassmann, R., et al. (2018). Nitrogen fertilizer fate after introducing maize and upland-rice into continuous paddy rice cropping systems. Agric. Ecosyst. Environ. 258, 162–171. doi: 10.1016/j.agee.2018.02.021
Gaihre, Y. K., Tirol-Padre, A., Wassmann, R., Aquino, E., Pangga, G. V., and Sta-Cruz, C. (2011). Spatial and temporal variations in methanefluxes from irrigated lowland ricefields. Phili Agric. Sci. 94, 335–342.
Gathorne, H., Reddy, A. D. N., Venkatanarayana, M., and White, B. H. (2013). A Life Cycle Assessment (LCA) of greenhouse gas emissions from SRI and flooded rice production in SE India. Taiwan Water Conserv. 61, 110125. doi: 10.35648/20.500.12413/11781/ii250
Gitonga, J. W. (2020). Evaluation of Greenhouse Gas Emissions and Water Productivity from Rice Production as Affected by Water Management and Soil Type in Mwea. Central Kenya (Master Thesis, JKUAT-COETEC).
Gowele, G. E., Mahoo, H. F., and Kahimba, F. C. (2020). Comparison of silicon status in rice grown under the system of rice intensification and flooding regime in mkindo irrigation scheme, Morogoro, Tanzania. Tanzania J. Agric. Sci. 19, 216–226.
Grewer, U., Bockel, L., and Bernoux, M. (2013). EX-ACT quick guidance Estimating and Targeting GHG Mitigation in Agriculture. (Rome: Food and Agriculture Organization of the United Nations)
Grewer, U., Bockel, L., Galford, G., Gurwick, N., Nash, J., Pirolli, G., et al. (2016). A methodology for greenhouse gas emission and carbon sequestration assessments in agriculture: supplemental materials for info note series analysing low emissions agricultural practices in USAID development projects CCAFS Working paper No. 187. (Copenhagen, Cali and Rome: CGIAR Research Program on Climate Change, Agriculture and Food Security, International Center for Tropical Agriculture and Food and Agriculture Organization of the United Nations).
Grewer, U., Nash, J., Gurwick, N., Bockel, L., Galford, G., Richards, M., et al. (2018). Analyzing the greenhouse gas impact potential of smallholder development actions across a global food security program. Environ. Res. Lett. 13, 044003. doi: 10.1088/1748-9326/aab0b0
Gupta, K., Kumar, R., Baruah, K. K., Hazarika, S., Karmakar, S., and Bordoloi, N. (2021). Greenhouse gas emission from rice fields: a review from Indian context. Environ. Sci. Pollut. Res. 28, 30551–30572. doi: 10.1007/s11356-021-13935-1
Gyanchandani, V. (2016). UNFCCC Nationally Determined Contributions: Climate Change and Trade. Available at: SSRN:3286257. doi: 10.2139/ssrn.3286257
Hadi, A., Inubushi, K., and Yagi, K. (2010). Effect of water management on greenhouse gas emissions and microbial properties of paddy soils in Japan and Indonesia. Paddy Water Environ. 8, 319–324. doi: 10.1007/s10333-010-0210-x
Haefele, S. M., Wopereis, M. C. S., Donovan, C., and Maubuisson, J. (2001). Improving the productivity and profitability of irrigated rice production in Mauritania. Eur. J. Agron. 14, 181–196. doi: 10.1016/S1161-0301(00)00094-0
Hidayah, S., Agustina, D. A., and Joubert, M. D. (2009). Intermittent irrigation in system of rice intensification, Potential as an adaptation and mitigation option of negative impacts of rice cultivation in irrigated paddy field, Experimental Station for Irrigation Team. Penelitian Irigasi Hemat Air pada Budidaya Padi dengan metode SRI di Petak Tersier, Tasikmalaya (MT 2/2009). Buku 22, Seri Penelitian Irigasi Hemat Air pada Budidaya Padi dengan metode SRI, Balai Irigasi. p. 1–11.
Hillier, J., Smith, P., and Bandel, T. (2013). “Farm-scale greenhouse gas emissions using the Cool Farm Tool: application of a generic farming emissions calculator in developing countries,” in Climate Change Mitigation and Agriculture, (Routledge), 245–254. doi: 10.4324/9780203144510-29
Hillier, J., Walter, C., Malin, D., Garcia-Suarez, T., Mila-i-Canals, L., and Smith. (2011). A farm-focused calculator for emissions from crop and livestock production. Environ. Model. Softw. 26, 1070–1078. doi: 10.1016/j.envsoft.2011.03.014
Hussain, S., Peng, S., Fahad, S., Khaliq, A., Huang, J., Cui, K., et al. (2015). Rice management interventions to mitigate greenhouse gas emissions: a review. Environ. Sci. Pollut. Res. 22, 3342–3360. doi: 10.1007/s11356-014-3760-4
IPCC (2007). “Climate change 2007: synthesis report Contribution of Working Groups I, II and III to the Fourth Assessment Report of the Intergovernmental Panel on Climate Change,” in Pachauri, RK and Reisinger A., eds., (Geneva, Switzerland: IPCC), 104.
IPCC (2014a). Contribution of Working Groups I, II and III to the 5th Assessment Report of the Intergovernmental Panel on Climate Change. Climate Change 2014: Synthesis Report. Geneva, Switzerland: IPCC. (2014).
IPCC (2014b). Climate change, 2014: Mitigation of Climate Change. in: Edenhofer, O., Pichs-Madruga, R., Sokona, Y., Farahani, E., Kadner, S., Seyboth, K., et al. (eds.) Contribution of Working Group III to the Fifth Assessment Report of the Intergovernmental Panel on Climate Change, (Cambridge, New York: Cambridge University Press), 811–922.
IPCC. (2019). 2006 IPCC Guidelines for National Greenhouse Gas Inventories, eds E. Calvo Buendia, K. Tanabe, A. Kranjc, J. Baasansuren, M. Fukuda, S. Ngarize, et al. (IPCC).
I. P. O. (2006). 2006 IPCC Guidelines for National Greenhouse Gas Inventories. Hayama; Kanagawa: Institute for Global Environmental Strategies.
Islam, S. M., Gaihre, Y. K., Islam, M. R., Akter, M., Al Mahmud, A., Singh, U., et al. (2020). Effects of water management on greenhouse gas emissions from farmers' rice fields in Bangladesh. Sci. Total Environ. 734, 139382. doi: 10.1016/j.scitotenv.2020.139382
Jain, N., Dubey, R., Dubey, D. S., Singh, J., Khanna, M., Pathak, H., et al. (2014). Mitigation of greenhouse gas emission with system of rice intensification in the Indo-Gangetic Plains. Paddy Water Environ. 12, 355–363. doi: 10.1007/s10333-013-0390-2
Janz, B., Weller, S., Kraus, D., Racela, H. R., Wassmann, R., Butterbach-Bahl, K., and Kiese, R. (2019). Greenhouse gas footprint of diversifying rice cropping systems: impactsofwater regime and organic amendments. Agric. Ecosyst. Environ. 270, 41–54. doi: 10.1016/j.agee.2018.10.011
Jiang, Y., Carrijo, D., Huang, S., Chen, J. I., Balaine, N., Zhang, W., et al. (2019). Water management to mitigate the global warming potential of rice systems: A global meta-analysis. Field Crop Res. 234, 47–54. doi: 10.1016/j.fcr.2019.02.010
Kahimba, F. C., Kombe, E. E., and Mahoo, H. F. (2013). The potential of system of rice intensification (SRI) to increase rice water productivity: a case of Mkindo irrigation scheme in Morogoro region, Tanzania. Tanzania J. Agric. Sci. 12.
Karki, S., Adviento-Borbe, M. A. A., Massey, J. H., and Reba, M. L. (2021). Assessing seasonal methane and nitrous oxide emissions from furrow-irrigated rice with cover crops. Agriculture 11, 261. doi: 10.3390/agriculture11030261
Katambara, Z., Kahimba, F. C., Mahoo, H. F., Mbungu, W. B., Mhenga, F., Reuben, et al. (2013). Adopting the system of rice intensification (SRI) in Tanzania: A review. Agric. Sci. 4, 7. doi: 10.4236/as.2013.48053
Kim, D. G., Thomas, A. D., Pelster, D., Rosenstock, T. S., and Sanz-Cobena, A. (2015). “Greenhouse gas emissions in natural and agricultural landsin sub-Saharan Africa: Synthesis of available data and suggestions for further studies,” in AGU Fall Meeting Abstracts, (vol. 2015, p. B11A−0406). doi: 10.5194/bgd-12-16479-2015
Kim, G. Y., Lee, S. B., Lee, J. S., Choi, E. J., and Ryu, J. H. (2012). Mitigation of greenhouse gases by water management of SRI (System of Rice Intensification) in rice paddy fields. Korean J. Soil Sci. Fertil. 45, 1173–1178. doi: 10.7745/KJSSF.2012.45.6.1173
Ku, H. H., Hayashi, K., Agbisit, R., and Villegas-Pangga, G. (2017).Evaluation of fertilizer and water management effect on rice performance and greenhouse gas intensity in different seasonal weather of tropical climate. Sci. Total Environ. 601, 1254–1262. doi: 10.1016/j.scitotenv.2017.05.277
Kumaraswamy, S., Rath, A. K., Ramakrishnan, B., and Sethunathan, N. (2000). Wetland rice soils as sources and sinks of methane: a review and prospects for research. Biol. Fertil. Soils 31, 449–461. doi: 10.1007/s003740000214
Lagomarsino, A., Agnelli, A. E., Linquist, B., Adviento-Borbe, M. A., Agnelli, A., Gavina, G., et al. (2016). Alternate wetting and drying of rice reduced CH4 emissions but triggered N2O peaks in a clayey soil of central Italy. Pedosphere 26, 533–548. doi: 10.1016/S1002-0160(15)60063-7
Lai, L., Wassmann, R., and Sander, B. O. (2021). Policy framework for myanmar rice production and in-depth study on greenhouse gas emissions. J. Earth Environ. Sci. Res. 185, 159. doi: 10.47363/JEESR/2021
Lampayan, R. M., Rejesus, R. M., Singleton, G. R., and Bouman, B. A. (2015). Adoption and economics of alternate wetting and drying water management for irrigated lowland rice. Field Crops Res. 170, 95–108. doi: 10.1016/j.fcr.2014.10.013
Li, J., Wan, Y., Wang, B., Waqas, M. A., Cai, W., Guo, C., et al. (2018). Combination of modified nitrogen fertilizers and water saving irrigation can reduce greenhouse gas emissions and increase rice yield. Geoderma 315, 1–10. doi: 10.1016/j.geoderma.2017.11.033
Lindsay, W. L., and Norvell, W. A. (1982). “Development of a DTPA soil test for zinc, iron, manganese, and copper,” in Methods of Soil Analysis Part 3: Chemical Methods, 5th Edn, ed D.L. Sparks (Madison, WI: Soil Science of America Inc), 421–428.
Linquist, B. A., Adviento-Borbe, M. A., Pittelkow, C. M., van Kessel, C., and van Groenigen, K. J. (2012). Fertilizer management practices and greenhouse gas emissions from rice systems: a quantitative review and analysis. Field Crops Res. 135, 10–21. doi: 10.1016/j.fcr.2012.06.007
Linquist, B. A., Anders, M. M., Adviento-Borbe, M. A. A., Chaney, R. L., Nalley, L. L., Da Rosa, E. F., et al. (2015). Reducing greenhouse gas emissions, water use, and grain arsenic levels in rice systems. Global Change Biol. 21, 407–417. doi: 10.1111/gcb.12701
Liu, J., You, L., Amini, M., Obersteiner, M., Herrero, M., Zehnder, A. J., et al. (2010). A high-resolution assessment on global nitrogen flows in cropland. Proc. Nat. Acad. Sci. 107, 8035–8040. doi: 10.1073/pnas.0913658107
Mati, B. M., Nyangau, W. W., Ndiiri, J. A., and Wanjogu, R. (2021). Enhancing production while saving water through the system of rice intensification (SRI) in Kenya's irrigation schemes. J. Agric. Sci. Technol. 20, 24–40.
Mclean, E. O. (1982). “Soil pH lime requirement,” in Method of Soil Analysis, Part 2, Chemical and Mineralogical Properties, 2nd Edn, eds A. L. Page, R. H. Miller, and D.R Keeney (Madison, WI: American Society of Agronomy), 561–573.
Minamikawa, K., and Yagi, K. (2009). “Possibility of water management for mitigating total emission of greenhouse gases from irrigated paddy fields,” in Climate Change and Crops, (Berlin, Heidelberg: Springer), 307–328. doi: 10.1007/978-3-540-88246-6_14
Myhre, G., Shindell, D., Bréon, F.-M., Collins, W., Fuglestvedt, J., Huang, J., et al. (2013). “Anthropogenic and natural radiative forcing. in climate change 2013: the physical science basis,” in Stocker, T.F., Qin, D., Plattner, G.-K., Tignor, M., Allen, S.K., Boschung, J., et al., (eds.) Contribution of Working Group I to the Fifth Assessment Report of the Intergovernmental Panel on Climate Change, (Cambridge, UK; New York, NY, USA: Cambridge University Press), 659–740. doi: 10.1017/CBO9781107415324.018
Nakano, Y., Bamba, I., Diagne, A., Otsuka, K., and Kajisa, K. (2011). The possibility of a rice green revolution in large-scale irrigation schemes in Sub-Saharan Africa (Policy Research Working Paper; no. WP5560). Washington, DC: World Bank. doi: 10.1596/1813-9450-5560
Nakano, Y., and Kajisa, K. (2013). The determinants of technology adoption: The case of the rice sector in Tanzania (JICA-RI Working Paper). Tokyo: JICA Research Institute
Nelson D. W. and Sommers, L. E.. (1982). “Total carbon, organic carbon, and organic matter,” in Methods of Soil Analysis Part 3: Chemical Methods: SSSA Book Series No. 5, 5th Edn, ed D. L. Sparks (Baltimore, MD: Soil Science of America Inc), 961–1010.
Nhamo, N., ROdenburg, J., Zenna, N., Makombe, G., and LuziKihupi, A. (2014). Narrowing the yield gap in East and Southern Africa: Using and adapting existing technologies. Agric. Syst. 131, 45–55. doi: 10.1016/j.agsy.2014.08.003
Niang, A., Becker, M., Ewert, F., Dieng, I., Gaiser, T., Tanaka, A., et al. (2017). Variability and determinants of yields in rice production systems of West Africa. Field Crops Res. 207, 1–12. doi: 10.1016/j.fcr.2017.02.014
Nyamadzawo, G., Wuta, M., Chirinda, N., Mujuru, L., and Smith, J. L. (2013). Greenhouse gas emissions from intermittently flooded (dambo) rice under different tillage practices in Chiota smallholder farming area of Zimbabwe. Atmosph. Clim. Sci. doi: 10.4236/acs.2013.34A003
Owino, C. N., Kitaka, N., Kipkemboi, J., and Ondiek, R. A. (2020). Assessment of greenhouse gases emission in smallholder rice paddies converted from Anyiko Wetland, Kenya. Front. Environ. Sci. 8, 80. doi: 10.3389/fenvs.2020.00080
Pelster, D., Rufino, M., Rosenstock, T., Mango, J., Saiz, G., Diaz-Pines, E., et al. (2017). Smallholder farms in eastern African tropical highlands have low soil greenhouse gas fluxes. Biogeosciences 14, 187–202. doi: 10.5194/bg-14-187-2017
Petersen, S. O. (2018). Greenhouse gas emissions from liquid dairy manure: Prediction and mitigation. J. Dairy Sci. 101, 6642–6654. doi: 10.3168/jds.2017-13301
Pramono, A., Adriany, T. A., and Susilawati, H. L. (2020). Mitigation scenario for reducing greenhouse gas emission from rice field by water management and rice cultivars. J. Trop Soils. 25, 53–60. doi: 10.5400/jts.2020.v25i2.53-60
Ramesh, T., and Rathika, S. (2020). Evaluation of rice cultivation systems for greenhouse gases emission and productivity. Int. J. Ecol. Environ. Sci. 2, 49–54.
Reuben, Katambara, Z., Kahimba, F. C., Mahoo, H. F., Mbungu, W. B., Mhenga, F., et al. (2016). Influence of transplanting age on paddy yield under the system of rice intensification. Agric. Sci. 7, 154–163. doi: 10.4236/as.2016.73015
Rosenstock, T. S., Mpanda, M., Pelster, D. E., Butterbach-Bahl, K., Rufino, M. C., Thiong'o, M., et al. (2016). Greenhouse gas fluxes from agricultural soils of Kenya and Tanzania. J. Geophys. Res. 121, 1568–1580. doi: 10.1002/2016JG003341
Sander, B. O., Samson, M., and Buresh, R. J. (2014). Methane and nitrous oxide emissions fromflooded ricefields as affected by water and straw management between rice crops. Geoderma 235, 355–362. doi: 10.1016/j.geoderma.2014.07.020
Sander, B. O., Schneider, Romasanta, R., Samoy-Pascual, K., Sibayan, E. B., Asis, C. A., et al. (2020). Potential of alternate wetting and drying irrigation practices for the mitigation of ghg emissions from rice fields: two cases in central luzon (Philippines). Agriculture 10, 350. doi: 10.3390/agriculture10080350
Sandhu, N., Subedi, S. R., Yadaw, R. B., Chaudhary, B., Prasai, H., Iftekharuddaula, K., et al. (2017). Root traits enhancing rice grain yield under alternate wetting and drying condition. Front. Plant Sci. 8, 1879. doi: 10.3389/fpls.2017.01879
Saunois, M., Stavert, A. R., Poulter, B., Bousquet, Canadell, J. G., Jackson, R. B., et al. (2020). The global methane budget 2000–2017. Earth Syst. Sci. Data. 12, 1561–1623. doi: 10.5194/essd-12-1561-2020
Shang, Q., Yang, X., Gao, C., Wu, Liu, J., Xu, Y., et al. (2011). Net annual global warming potential and greenhouse gas intensity in Chinese double rice-cropping systems: A 3-year field measurement in long-term fertilizer experiments. Glob. Chang. Biol. 17, 2196–2210. doi: 10.1111/j.1365-2486.2010.02374.x
Smartt, A. D., Brye, K. R., Rogers, C. W., Norman, R. J., Gbur, E. E., Hardke, J. T., et al. (2016). Previous crop and cultivar effects on methane emissions from drill-seeded, delayed-flood rice grown on a clay soil. Appl. Environ. Soil Sci. doi: 10.1155/2016/9542361
Smith, P., Clark, H., Dong, H., Elsiddig, E. A., Haberl, H., Harper, R., et al. (2014). “Agriculture, forestry and other land use (AFOLU),” in Edenhofer, O., Pichs-Madruga, R., Sokona, Y., Farahani, E., Kadner, S., Seyboth, K., et al. (eds.), Climate change, 2014: Mitigation of Climate Change. IPCC Working Group III Contribution to AR5, (Cambridge, UK: Cambridge University Press) 811–922. doi: 10.1017/CBO9781107415416.017
Sun, L., Song, C., Miao, Y., Qiao, T., and Gong, C. (2013). Temporal and spatial variability of methane emissions in a northern temperate marsh. Atmospheric Environ. 81, 356–363. doi: 10.1016/j.atmosenv.2013.09.033
Susilawati, H. L., Setyanto, P., and Kartikawati, R. Sutriadi, M. T. (2019). “The opportunity of direct seeding to mitigate greenhouse gas emission from paddy rice field,” in IOP Conference Series: Earth and Environmental Science, Vol. 393 (Bristol: IOP Publishing), 012042.
Tanaka, A., Diagne, M., and Saito, K. (2015). Causes of yield stagnation in irrigated lowland rice systems in the Senegal River Valley: Application of dichotomous decision tree analysis. Field Crops Res. 176, 99–107. doi: 10.1016/j.fcr.2015.02.020
Tanaka, A., Johnson, J., Senthilkumar, K., Akakpo, C., Segda, Z., Yameogo, L., et al. (2017). On-farm rice yield and its association with biophysical factors in sub-Saharan Africa. Eur. J. Agron. 85, 1–11. doi: 10.1016/j.eja.2016.12.010
Tanaka, A., Saito, K., Azoma, K., and Kobayashi, K. (2013). Factors affecting variation in farm yields of irrigated lowland rice in southern-central Benin. Eur. J. Agron. 44, 46–53. doi: 10.1016/j.eja.2012.08.002
Tesfaye, K., Takele, R., Sapkota, T. B., Khatri-Chhetri, A., Solomon, D., Stirling, C., et al. (2021). Model comparison and quantification of nitrous oxide emission and mitigation potential from maize and wheat fields at a global scale. Sci. Total Environ. 782, 146696. doi: 10.1016/j.scitotenv.2021.146696
Thakur, A. K., Mandal, K. G., Mohanty, R. K., and Uphoff, N. (2021). How agroecological rice intensification can assist in reaching the Sustainable Development Goals. Int. J. Agric. Sustain. 20, 216–230. doi: 10.1080/14735903.2021.1925462
Thakur, A. K., Mohanty, R. K., Patil, D. U., and Kumar, A. (2014). Impact of water management on yield and water productivity with system of rice intensification (SRI) and conventional transplanting system in rice. Paddy Water Environ. 12, 413–424. doi: 10.1007/s10333-013-0397-8
Tilman, D., Balzer, C., Hill, J., and Befort, B. L. (2011). Global food demand and the sustainable intensification of agriculture. Proc. Nat. Acad. Sci. 108, 20260–20264. doi: 10.1073/pnas.1116437108
Tilman, D., Cassman, K. G., Matson, A., Naylor, R., and Polasky, S. (2002). Agricultural sustainability and intensive production practices. Nature 418, 671–677. doi: 10.1038/nature01014
Tongwane, M. I., and Moeletsi, M. E. (2018). A review of greenhouse gas emissions from the agriculture sector in Africa. Agric. Syst. 166, 124–134. doi: 10.1016/j.agsy.2018.08.011
Tsujimoto, Y., Rakotoson, T., Tanaka, A., and Saito, K. (2019). Challenges and opportunities for improving N use efficiency for rice production in sub-Saharan Africa. Plant Prod. Sci. 22, 413–427. doi: 10.1080/1343943X.2019.1617638
UNFCCC (2015). Adoption of the Paris Agreement. I: Proposal by the President (Draft Decision). Geneva, Switzerland: United Nations Office.
van Beek, C. L., Meerburg, B. G., Schils, R. L., Verhagen, J., and Kuikman, J. (2010). Feeding the world's increasing population while limiting climate change impacts: linking N2O and CH4 emissions from agriculture to population growth. Environ. Sci. Policy 13, 89–96. doi: 10.1016/j.envsci.2009.11.001
Van Groenigen, K. J., Van Kessel, C., and Hungate, B. A. (2013). Increased greenhouse-gas intensity of rice production under future atmospheric conditions. Nat. Clim. Change 3, 288–291. doi: 10.1038/nclimate1712
van Oort, A. J., Saito, K., Tanaka, A., Amovin-Assagba, E., Van Bussel, L. G. J., van Wart, J., et al. (2015). Assessment of rice self-sufficiency in eight African countries in 2025. Global Food Secur. 5, 39–49. doi: 10.1016/j.gfs.2015.01.002
Vetter, S. H., Malin, D., and Smith, Hillier, J. (2018). The potential to reduce GHG emissions in egg production using a GHG calculator–A Cool Farm Tool case study. J. Cleaner Prod. 202, 1068–1076. doi: 10.1016/j.jclepro.2018.08.199
Wang, W., Dalal, R., Reeves, S., Butterbach-Bahl, K., and Kiese, R. (2011). Greenhouse gas fluxes from an Australian subtropical cropland under long-term contrasting management regimes. Glob. Chang. Biol. 17,3089–3101. doi: 10.1111/j.1365-2486.2011.02458.x
Wassmann, R., Pasco, R., Zerrudo, J., Ngo, D. M., Vo, T. B. T., and Sander, B. O. (2019). Introducing a new tool for greenhouse gas calculation tailored for cropland: rationale, operational framework and potential application. Carbon Manage. 10, 79–92. doi: 10.1080/17583004.2018.1553436
Win, E., Win, K. K., Bellingrath-Kimura, S. D., and Oo, A. Z. (2020). Greenhouse gas emissions, grain yield and water productivity: a paddy rice field case study based in Myanmar. Greenhouse Gases. 10, 884–897. doi: 10.1002/ghg.2011
Wopereis, M. C. S., Donovan, C., Nebié, B., Guindo, D., and N'Diaye, M. K. (1999). Soil fertility management in irrigated rice systems in the Sahel and Savanna regions of West Africa: Part I. Agronomic analysis. Field Crops Res. 61, 125–145.
Xu, Y., Ge, J., Tian, S., Li, S., Nguy-Robertson, A. L., Zhan, M., et al. (2015).Effects of water-saving irrigation practices and drought resistant rice variety on greenhouse gas emissions from a no-till paddy in the central lowlands of China. Sci. Total Environ. 505, 1043–1052. doi: 10.1016/j.scitotenv.2014.10.073
Yang, S., Peng, S., Xu, J., Luo, Y., and Li, D. (2012). Methane and nitrous oxide emissions from paddy field as affected by water-saving irrigation. Phys. Chem. Earth 53, 30–37. doi: 10.1016/j.pce.2011.08.020
Yao, Z., Zheng, X., Dong, H., Wang, R., Mei, B., and Zhu, J. (2012). A 3-year record of N2O and CH4 emissions from a sandy loam paddy during rice seasons as affected by different nitrogen application rates. Agric. Ecosyst. Environ. 152, 1–9. doi: 10.1016/j.agee.2012.02.004
Zhang, X., Xu, X., Liu, Y., Wang, J., and Xiong, Z. (2016). Global warming potential and greenhouse gas intensity in rice agriculture driven by high yields and nitrogen use efficiency. Biogeosciences 13, 2701–2714. doi: 10.5194/bg-13-2701-2016
Zhang, X., Zhou, S., Bi, J., Sun, H., Wang, C., and Zhang, J. (2021). Drought-resistance rice variety with water-saving management reduces greenhouse gas emissions from paddies while maintaining rice yields. Agric. Ecosyst. Environ. 320, 107592. doi: 10.1016/j.agee.2021.107592
Zheng, C., Zhang, Z., Hao, S., Chen, W., Pan, Y., and Wang, Z. (2020). Agronomic growth performance of super rice under water-saving irrigation methods with different water-controlled thresholds in different growth stages. Agronomy. 10, 239. doi: 10.3390/agronomy10020239
Zheng, H., Huang, H., Yao, L., Liu, J., He, H., and Tang, J. (2014). Impacts of rice varieties and management on yield-scaled greenhouse gas emissions from rice fields in China: A meta-analysis. Biogeosciences 11, 3685–3693. doi: 10.5194/bg-11-3685-2014
Keywords: greenhouse gas emission, system of rice intensification, management practices, climate change, global warming potential, conventional practice, rice
Citation: Mboyerwa PA, Kibret K, Mtakwa P and Aschalew A (2022) Greenhouse gas emissions in irrigated paddy rice as influenced by crop management practices and nitrogen fertilization rates in eastern Tanzania. Front. Sustain. Food Syst. 6:868479. doi: 10.3389/fsufs.2022.868479
Received: 07 February 2022; Accepted: 29 September 2022;
Published: 31 October 2022.
Edited by:
Felix Kwabena Donkor, University of South Africa, South AfricaReviewed by:
Joseph Onyango Gweyi, Kenyatta University, KenyaCopyright © 2022 Mboyerwa, Kibret, Mtakwa and Aschalew. This is an open-access article distributed under the terms of the Creative Commons Attribution License (CC BY). The use, distribution or reproduction in other forums is permitted, provided the original author(s) and the copyright owner(s) are credited and that the original publication in this journal is cited, in accordance with accepted academic practice. No use, distribution or reproduction is permitted which does not comply with these terms.
*Correspondence: Primitiva A. Mboyerwa, cHJpbWl0aXZhYW5kcmVhQHlhaG9vLmNvbQ==
Disclaimer: All claims expressed in this article are solely those of the authors and do not necessarily represent those of their affiliated organizations, or those of the publisher, the editors and the reviewers. Any product that may be evaluated in this article or claim that may be made by its manufacturer is not guaranteed or endorsed by the publisher.
Research integrity at Frontiers
Learn more about the work of our research integrity team to safeguard the quality of each article we publish.